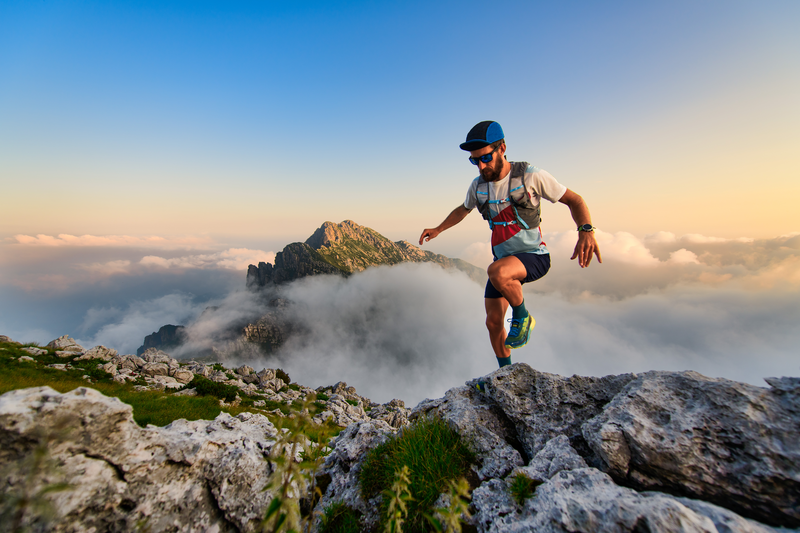
95% of researchers rate our articles as excellent or good
Learn more about the work of our research integrity team to safeguard the quality of each article we publish.
Find out more
MINI REVIEW article
Front. Endocrinol. , 10 February 2023
Sec. Molecular and Structural Endocrinology
Volume 14 - 2023 | https://doi.org/10.3389/fendo.2023.1106529
This article is part of the Research Topic Interorgan Crosstalk Mediated by Exerkines: the Role of Exercise in Health and Disease View all 5 articles
Human beings lead largely sedentary lives. From an evolutionary perspective, such lifestyle is not beneficial to health. Exercise can promote many enabling pathways, particularly through circulating exerkines, to optimize individual health and quality of life. Such benefits might explain the protective effects of exercise against aging and noncommunicable diseases. Nevertheless, the miRNA-mediated molecular mechanisms and exerkine interorgan crosstalk that underlie the beneficial effects of exercise remain poorly understood. In this mini review, we focused on the exerkine, irisin, mainly produced by muscle contraction during adaptation to exercise and its beneficial effects on body homeostasis. Herein, the complex role of irisin in metabolism and inflammation is described, including its subsequent effects on thermogenesis through browning to control obesity and improve glycemic regulation for diabetes mellitus control, its potential to improve cognitive function (via brain derived neurotrophic factor), and its pathways of action and role in aging.
Despite millions of years of human evolution, the intricate genetic mechanisms and transposable chromosome elements have not adapted to the average low-level physical activity performed by modern Homo sapiens sapiens (1). Our ancestors, such as hunter-gatherers, engaged in intense physical activity to search for food and evade predators (2). As a result, physical activity was performed up to 6-9 hours per day in South Africa and Ache in Paraguay, including walking and running (3). According to prior studies, men in the Tsimane group engaged in 120 min/day of moderate or vigorous physical activity while women engaged in 100 min/day of such activity (4). Modern society has introduced human beings to a sedentary lifestyle. Currently, human beings spend hours seated in front of overbright screens, avoid using the stairs, even to go two floors up, and prefer the delivery of food to their homes, instead of taking healthy short walks to the grocery store (5). Self-directed “physically active” individuals only exercise for 45-60 minutes per session, twice or three-times per week (6). The slow but constantly forged genes of humans were not designed for such low levels of activity. In terms of evolutionary metabolic/physiological adaptations, humans were designed to move frequently, run, and fight for food, and survive in an often-unfriendly environment (7). In developed countries, the vast availability of calories in addition to sedentary lifestyles has led to a high incidence of modern life (chronic) diseases, such as obesity, diabetes, heart disease, cancers, and many other complex psychosomatic and inflammatory disorders (8).
Regular exercise is important to the general health of humans. Individual judgment and scientific data indicate that exercise is the best non-pharmacological intervention (combined with good nutrition) to assure a good quality of life at any age (9–11). Physical exercise promotes cardiovascular efficiency, activates protein and energy metabolism through progressive endocrine adjustments, optimizes immune responses, and provides cognitive benefits, especially in committed individuals who engage in regular exercise programs (12). Although the benefits of exercise are known to be dependent on the intensity and duration of the training sessions adjusted to the physiological conditions of the participants (13), understanding the cellular pathways that regulate/revert/avoid extended post-exercise inflammatory condition has become a very important target (14).
Exercise affects all organs, tissues, and cells in the human body. Several molecular events are particularly important for eliciting health-related physiological adaptations, including cellular redox rebalancing, which involves the production of reactive oxygen/nitrogen species (ROS/RNS) counteracted by antioxidant defenses (15), skeletal muscle hypertrophy (including fiber-type distinctions) (15), mitochondrial biogenesis, fission-fusion dynamics (16), angiogenesis (17), and other mechanisms (18). However, the precise cellular and molecular mechanisms that underlie the beneficial effects of exercise remain unclear. Myokines constitute a new regulatory component that may play a role in exercise-induced adaptation (16–21). In this mini review, we sought to elucidate how myokines, in particular the exerkine, irisin, act in body homeostasis and under pathological conditions.
The skeletal muscle is the most abundant tissue in the human body and is responsible to produce strength, balance, and movement (21, 22). The skeletal muscle is also known as an endocrine, paracrine, and autocrine organ that produces the myokines required for integrative responses in the human body (19, 23, 24).
The skeletal muscle releases myokines, which are signaling molecules that promote an integrative crosstalk between the muscle and other organs, resulting in physiological benefits from exercise (25–28). Physical inactivity causes chronic inflammation, mainly owing to the inappropriate release of cytokines from redox-imbalanced (or injured) tissues or overstimulation of inflammatory cells, such as neutrophils and macrophages (29). Interestingly, several exercise protocols have been proven to reduce the mortality risk of individuals with a previous sedentary lifestyle, an effect that could be directly attributed to the signaling adaptation of key myokines. For instance, interleukin (IL)-6, the first and most studied myokine, is produced via muscle contraction during exercise training sessions and is released in circulation to induce lipolysis in adipose tissue and glycogenolysis in the liver. IL-6 increases the expression of IL-1ra, which can be observed within 1 to 2 h after 2.5 hours of exercise at 75% VO2max. IL-10 levels were found to peak at 45 and 72 h after resistance training and cycle ergometer at 60% VO2max, and at 4 h after a marathon (30, 31). Therefore, the expression intensity and circulating levels of the myokine, IL-6, and other factors, such as tumor necrosis factor-alpha (TNF-α) and C-reactive protein (CRP) (32, 33) are associated with muscle-derived signals for energy supply and post-exercise inflammatory responses (34, 35).
A novel jargon used in clinical and sports medicine, “exerkines,” may better define this myokine action in response to acute or chronic exercise (20) Among several previously reported cytokines (such as IL-6), key signaling agents have been highlighted from contractile muscles, such as angiopoietin-like 4 (ANGPTL4), apelin, brain-derived neurotrophic factor (BDNF), CCL2 (or MCP-1), CX3CL1 of fractalkine (FKN), fibroblast growth factor 21 (FGF21), IL-7, IL-8, IL-15, myostatin, secreted protein acidic and cysteine-rich (SPARC), leukemia inhibitory factor (LIF), meteorin-like protein (Metrnl), and irisin (36). These cytokines have multiple effects on the body, ranging from cardiovascular functions, with proven interactions with vascular endothelial growth factor (VEGF) and nitric oxide (NO•), to their pivotal participation in the triggering of post-exercise inflammation (37–40).
The skeletal muscle releases non-coding RNAs, especially microRNAs (miRNAs), which have been identified as new regulatory components that may play a role in exercise-induced adaptations. However, the function of these RNAs in circulation remains unclear (41). miRNAs are a group of endogenous small non-coding RNAs that are 18–25 nucleotides in length. miRNAs regulate gene expression at the post-transcriptional level through messenger RNA degradation or translational inhibition (42). Once released by skeletal muscle cells, circulating miRNAs are stable, easily detectable, and may regulate gene expression in target cells and tissues as a novel mode of intercellular communication comparable to that exhibited by myokines. Accordingly, specific circulating miRNAs are altered in response to different acute and chronic exercise protocols in healthy and diseased populations (43). miRNA-133 is a muscle-enriched miRNA that regulates myogenesis in vitro by increasing myoblast proliferation. The expression level of miRNA-133 in skeletal muscles is sensitive to muscle contraction in response to several types of endurance exercise. As a result, miRNA-133 is a suitable candidate for potential post-exercise regulation at the plasma level (41). Therefore, exercise-induced changes in circulating miRNAs are dependent on muscle mass, angiogenesis, inflammation, ischemia, and hypoxia (44).
Of all the active exerkines, irisin has been demonstrated as one of the main protagonists in the modulation of blood pressure control via the NO•-dependent pathways. Moreover, irisin is expected to be a potential therapeutic agent for the treatment of non-communicable diseases and their related conditions and is regulated by the expression of some miRNAs (45). As a result, irisin is the focus of our review.
Irisin is a relatively small peptide with 112 amino acids and a specific domain for the transmembrane protein, fibronectin (FNDC5). The start codon of the human FNDC5 gene is reported to be atypical ATA rather than ATG in rodents. Further, the translation efficiency of the human FNDC5 gene constructed with the ATA start codon is impaired. Other hominid species, such as Denisovan and Neanderthal, also display a loss in FNDC5 gene expression (46). Since the first discovery of irisin, scientists have tried to understand and determine the cleavage protein for FNDC5 (46). In fact, by using an improved mass spectrometry technique with synthetic peptides rich in heavy stable isotopes as internal standards, Jedrychowski et al. found that irisin is mainly expressed in the non-canonical start codon of FNDC5 (47). Based on recent studies, irisin binds to proteins of the αV class of integrins. Further biophysical studies revealed interacting surfaces between irisin and αVβ5 integrin (48). Chemical inhibition of αV integrins blocks the signaling pathway activated by irisin in both osteocytes and fat cells (49). These studies suggest the existence of the membrane receptors of irisin, as predicted by some scholars, with αVβ5 integrin as the receptor of irisin in osteocytes, adipocytes, and enterocytes (48, 49). However, the presence of irisin receptors in other cells requires further studies. In addition, the results of the irisin assays must be interpreted with caution as after ten years, the baseline values for western blotting and ELISA are associated with a large variance and problems with reproducibility may arise among lots (46, 50).”
In adipose tissue, FNDC5 exhibits its activity via uncoupling protein type-1 (UCP-1), which promotes the so-called “browning” process of adipose tissue. This activity is associated with an increase in the mitochondrial-rich adipocyte population within the fat tissue, ultimately leading to increased heat production and energy expenditure by these cells (51, 52). Interestingly, some miRNAs, such as miRNA-19b and miRNA-140, are suggested to downregulate irisin expression, thereby promoting weight loss by reducing energy expenditure (53, 54).
Irisin is produced in various tissues. In animals, irisin production was identified in the muscle, liver, pancreas, lung, adrenal glands, central nervous system (CNS), and kidney (53), whereas in humans, its production was identified in other tissues, such as adipose, bone, cardiomyocytes, and sebaceous glands. However, contracting skeletal muscles are the main sources of irisin production in the human body (54). In addition to its ability to induce mitochondrial biogenesis, irisin can regulate oxidative metabolism in different cell types via autocrine, paracrine, and endocrine mechanisms (55, 56). Notably, ROS/RNS production, and consequently the redox status in active skeletal muscles, is influenced by irisin activity (57, 58).
Skeletal muscle contraction induces the transcription and activation of the transcription coactivator, peroxisome proliferator-activated receptor gamma (PPAR-γ) type 1 alpha (PPARGC1A, also known as PGC-1α), a master regulator of genes involved in metabolism. The overexpression of PGC-1α increases irisin production via cleavage of the FNDC5 factor, stimulating mitochondrial biogenesis, oxidative phosphorylation, and oxygen consumption rate (55, 56). In response to exercise, irisin regulation depends on the specific training protocol (intensity, duration, and type of exercise), age, sex, training status, and muscle mass. Short bouts of intensive exercise acutely increase serum irisin levels in children and adults; however, irisin levels do not differ following prolonged (6 weeks) or chronic (1 year) exercise (57).
The activity of the FNDC5/irisin protein activates MAP-kinase cascades, and upon activation, the differentiation of neural cells and osteocytes is achieved, concomitant with the enhancement of glucose uptake by muscles and subsequent “browning” of white adipose tissues (58). Among the several MAPK pathways, the most conventional routes are c-Jun N-terminal kinases 1-3 (JNK1-3), extracellular signal-regulated kinase 1 and 2 (ERK1/2), p38 isoforms (α, β, γ, and δ), and ERK5 families. Less understood pathways, such as ERK3/4 and ERK7/8, and stress-activated protein kinases (SAPK1A, 1 B, 1C) (59, 60). Zhang et al. (2014) showed that chemical inhibition of the p38 or ERK pathways causes a significant reduction in the action of irisin on the adipocyte UCP-1 protein (61).
The exercise-induced irisin effect can differ in middle-aged, older, and young adults. Miyamoto-Mikami et al. showed that 8-weeks of endurance training promoted higher serum irisin expression in healthy middle-aged and older people (65 ± 8 years old) but not in young people (21 ± 1 years old). In contrast, younger and older adults displayed a similar irisin response to an acute bout of circuit training. Irisin levels were also found to have a significant correlation with a less visceral adipose tissue but not with whole-body fat mass (62) In a recent systematic review and meta-analysis, exercise training was found to significantly increase circulating irisin, and decrease insulin, glucose, and insulin resistance. Notably, the employed exercise training protocol may be associated with different irisin expression, suggesting that irisin level significantly increased when resistance training and resistance training combined with aerobic training were applied, while insulin level decreased when aerobic training and combined training were employed, especially in patients with type 2 diabetes and prediabetes (63). In another study, different muscles and training types were compared among mice. Based on the results, the slow-twitch muscle produced more irisin than the fast-twitch muscles; however, the training type (aerobic or anaerobic) did not affect irisin production (64)
Beyond its pivotal role in energy metabolism and mitochondrial biogenesis, FNDC5/irisin exerts inhibitory effects on inflammation by inducing hyperphosphorylation of MAPKs and reducing the release of pro-inflammatory cytokines (65). In addition, the association between irisin and nervous system function has been revealed during neural differentiation, when the loss of ERK1/2 function causes a significant decrease in the expression of both FNDC5/irisin and BDNF (66).
Overall, FNDC5/irisin can act as an important regulator of cellular communication, especially between muscle and other tissues. As suggested by Maalouf and Khoury (2019), FNDC5/irisin serves as a remarkable potential pharmacological target for inflammation and energy metabolism control (67). Figure 1 summarizes the effects of irisin on several organs and tissues.
Figure 1 The exercise stimulates the peroxisome proliferator-activated receptor gamma coactivator 1 (PCG1)-alpha transcription, which in turn drives the expression of fibronectin type III domain-containing protein 5 (FNDC5), a membrane protein that is cleaved and secreted as irisin. Irisin acts on various human organs and tissues; which together orchestrate whole-body metabolism by regulating bone remodeling, "browning" of mature white adipocytes in response to exercise, glucose metabolism and insulin sensitivity in skeletal muscle, neuroplasticity, insulin sensitivity, and improving hepatic glucose and lipid metabolism. Irisin improves redox balance and inflammation. ATP, adenosine triphosphate; AMPK, AMP-activated protein kinase; ANGPTL4, angiopoietin-like 4; BDNF, brain derived neurotrophic factor; MCP-1, monocyte chemoattractant protein-1; FGF21, fibroblast growth factor 21; LIF, leukemia inhibitory factor; Metrnl, meteorin-like protein; SPARC, secreted protein acidic and cysteine-rich; ROS, reactive oxygen species; RNS, reactive nitrogen species; SOD, dismutase superoxide; GSH-PX, glutathione peroxidase and CAT-9, catalase.
Obesity and type-2 diabetes mellitus are accompanied by the upregulation of inflammatory markers that substantially contribute to an overproduction of ROS/RNS, favoring insulin resistance in peripheral tissues and impaired insulin secretion from the pancreas (68, 69). As previously mentioned, irisin has a prominent capacity to modulate peripheral energy metabolism. Further, higher systemic irisin levels have been demonstrated to ameliorate glucose tolerance and mitigate insulin resistance (70). These effects of irisin can be observed through the upregulation of thioredoxin 2 (Trx2) and the blocked expression of thioredoxin-interacting protein (Txnip), consequently reducing the number of β-cells. Accordingly, irisin administration can help us understand its possible protective effect (71). Irisin was demonstrated to stimulate glucose uptake in skeletal muscle cells by increasing the phosphorylation of AMPK, thereby activating p38, which leads to the translocation of GLUT4 from the perinuclear region to the plasma membrane (72). This antidiabetic effect might be related to the ability of exercise-derived irisin to reduce or modulate inflammation. The anti-inflammatory action of irisin is reported to be particularly prominent in circulating immune cells, such as macrophages and neutrophils. Similarly, Mazur-Bialy et al. (73) demonstrated that irisin not only improves macrophage phagocytosis but also reduces ROS/RNS production in activated immune cells, which has a remarkable impact on inflammatory responses. The same researchers reinforced that the anti-inflammatory properties of irisin could be attributed to its ability to downregulate the downstream pathways of TLR4/MyD88 in RAW 264.7 macrophages (65, 73). The researchers also demonstrated the ability of irisin to inhibit the expression and release of inflammatory mediators in a coculture of adipocytes and macrophages (73). These researchers also showed that the Nf-r2/Heme oxygenase-1 (HO-1) pathways can be stimulated, consequently leading to less effects of ROS in the cell. Exercise promotes higher expression of NO, a factor that can stimulate HO-1; therefore, the antioxidant effects could be related to the Nf-r2/HO-1 pathways in the system (74). Treatment with irisin was found to stimulate antioxidants (SOD, GSH-Px, and CAT-9), ultimately reducing the levels of H2O2 in macrophages in vitro (75).
Interestingly, some studies have already revealed that irisin can reduce the progression of inflammation associated with inflammatory bowel disease (IBD) by reducing pro-inflammatory cytokine release (76, 77). IBD is related to reduced bone mineral density (DMO) induced by inflammation, and treatment with irisin induces bone formation and reduces the TNF-α+. Thus, irisin could reduce bowel inflammation and sclerostin (SOST) production, and reinvigorate DMO through osteoprotegerin (OPG) stimulation (46, 77). The volume intensity suggested a moderate intensity (60% VO2max) for 1 has no gastrointestinal changes were observed and intestinal inflammation was reduced (78) More studies are needed to delimit an exercise protocol for the treatment of these patients. Beyond these remarkable findings, higher levels of exercise-derived irisin can induce the expression of several anti-inflammatory proteins in the brain, particularly BDNF, in the hippocampus, a well-known brain segment associated with memory. Overall, irisin has putative applications in reverting the cognitive decline associated with Alzheimer’s disease (already demonstrated using experimental models) through exercise (79).
In a previous study, hepatic-released irisin was demonstrated to function as a paracrine/autocrine factor that inhibits lipogenesis and gluconeogenesis via the adenosine 5’-monophosphate (AMP)-activated protein kinase pathway by reducing the expression of phosphoenolpyruvate carboxyinase (Pepck) and glucose-6-phosphate (G6P) genes (80, 81). Accordingly, irisin could be a new treatment for the diabetic population.
In summary, both in vivo and in vitro studies revealed that irisin has remarkable anti-inflammatory properties as it modulates cytokine production, induces MAPK cascade factors (NF-kB), and reduces oxidative stress in different contexts. However, further mechanistic studies directly addressing the effects of irisin on ROS/RNS production and antioxidant defenses are needed.
Irisin is a myokine linked to many age-related diseases and neurological disorders (82–89). Studies in gerontology demonstrated that the pro-inflammatory response of adipose tissue contrasts with the anti-inflammatory action of exercise-induced myokines, such as irisin, released by contractile muscle tissue (23, 90). Unfortunately, only few studies have combined these variables into the same model. In 2020, Rashid et. al. analyzed the response of irisin to long-term moderate physical exercise (91). Their findings highlighted the influence of long-term exercise on metabolic mediators. After long-term physical exercise, irisin was demonstrated to improve glucose homeostasis, which was correlated with better glucose regulation, less insulin resistance, and consequently obesity. The protocol was tested in two groups: normal weight group (BMI < 25 kg/m2) and obese group (BMI ≥ 30 kg/m2). Notably, resistance training was adopted in this study. As aging comorbidities were consistently supported by the physiological pattern of an unhealthy aging lifestyle (87, 88), these studies could be perceived as early explorations into the positive effects of exercise-regulated irisin levels in the elderly population. To the best of our knowledge, this study is the only report of this information.
Numerous data have suggested the putative effect of increased irisin levels on reverting or retarding obesity and aging progression, based on renowned biomarkers, such as glycemia and total cholesterol levels (92). Previous studies have shown that irisin can act as a regulatory factor to control diabetes and obesity during aging (93).
Obesity and diabetes can increase the risk of cognitive ageing (94) and dementia (95). These cognitive conditions represent a significant challenge for the scientific and clinical communities (14). Exercise can have beneficial effects on brain health (9–11). Based on strong evidence, irisin plays a crucial role in the cognitive benefits of exercise. Prior studies also shed light on irisin as a potential therapeutic agent for some cognitive disorders (88, 96, 97). Cognitive function was previously thought to be improved by the increased expression of BDNF, which is stimulated by exercise-induced irisin and lactate (98, 99). Diabetes may also induce neuroinflammation, and consequently, cognitive deterioration and reduced memory. Treatment with irisin was found to block the p38, STAT3 and Nf-κB proteins, reducing diabetes-induced neuroinflammation in the brain of mice (100) Another study revealed that 100 nm/L irisin promoted cell proliferation via the STAT3 pathway (101). Synaptogenesis, neurogenesis, and long-term potentiation can be molecular pathways that explain the BDNF-mediated improvement in neuroplasticity via irisin (102). Moreover, a higher expression of BDNF affects the levels of dopamine, serotonin, and melatonin in several brain regions, preventing many of the symptoms observed in individuals with depression (65) and directly benefitting learning and memory via the dopamine pathways (103). When BDNF is stimulated by lactate (via SIRT1 pathway activation), memory and learning functions are substantially improved compared with instances of lower lactate concentrations (98). Notably, such findings were transversal and enthusiastically received from the psychological scientific community. However, lactate levels may reflect exercise intensity, which may be mainly responsible for the reported associations with improved memory function. Other benefits of exercise on brain function and the prevention of dementia include improved cerebral perfusion, improved metabolism (104), and reduced inflammation.
Immunological analysis must be performed to understand how the MAPK, AMPK, and TLR4/MyD88 intercellular pathways and exercise-induced irisin can benefit systemic inflammatory conditions (105). Recently, Papadopoulos, et al. reported that irisin released by muscles during aerobic exercise is an active agent in the AMPK/Akt-eNOS/NO• pathway (106).
Overall, the current evidence reinforces the hypothesis that irisin at optimal levels could be the main agent responsible for the long-term health benefits in regularly exercising individuals. Acute exercise can increase the concentration of circulating irisin, while chronic exercise can improve irisin metabolic dynamics and selectively increase circulating irisin concentrations. The effects of irisin may mediate some beneficial effects of exercise, such as the enhanced oxidation of fatty acids and heat production, leading to increased energy expenditure, glucose homeostasis, weight reduction, mitochondrial biogenesis, angiogenesis, improved cognition function, muscle fiber shifting, and prevention of muscular atrophy in aging and metabolic diseases (Figure 2). These effects can be regulated by distinct molecular pathways that permeate redox signaling and miRNA-mediated ncRNAs. However, the exact mechanisms remain unclear as the available data are barely consistent. Therefore, it is important to highlight how irisin can be used in the future as a diagnostic tool and possible treatment for the population. Further studies are necessary based on the metabolic, physiological, and cognitive limitations imposed by aging and pathological processes.
Figure 2 Irisin secreted by skeletal muscle during the exercise reaches blood circulation and in general ameliorates the mitochondrial biogenesis, angiogenesis, endothelial cell proliferation and browning. Such benefits might explain the protective effects of exercise against type-2 Diabetes Mellitus, inflammation, oxidative stress and neurodegenerative disease. PGC1-α, peroxisome proliferator-activated receptor gamma coactivator 1 alpha; FNDC5', fibronectin type III domain-containing protein 5; TLR4, toll-like receptor 4; MyD88, myeloid differentiation primary response 88; HO-1, heme oxygenase-1; SOD, dismutase superoxide; GSH-Px, peroxidase glutathione; CAT-9, catalase; H202, oxygen peroxide; BDNF, brain-derived neurotrophic factor and NF-kB, factor nuclear kappa B.
Herein, we addressed the mediators elicited during exercise for the maintenance of good health. We focused on the myokines produced by contracting muscles, which are also known as exerkines. Some of these mediators, including miRNAs, are released into the circulation, thereby improving cell-to-cell communication. Herein, the role of irisin in metabolism and inflammation was revealed, including its subsequent effects on obesity and diabetes mellitus, cognitive function, and compromised immune function. Further studies are necessary to demonstrate the possibility of biomarker-led diagnostics, conduct further mechanistic assessments, and identify novel treatment regimes.
Conceptualization, CT, BP, MB, and TF. writing, CT, BP, MB, AB, PB, CM, GF, PV. writing—review and editing, CT, BP, MB, AB, PB, CM, GF, PV, EO, EH and TF. visualization, CT, BP, MB, AB, PB, CM, GF, PV, EO, EH and TF. supervision, TF. All authors have read and agreed to the published version of the manuscript. All authors contributed to the article and approved the submitted version.
This work was supported by the Coordenação de Aperfeiçoamento de Pessoal de Nível Superior (CAPES) through the “Programa de Suporte a Pós-Graduação de Instituições de Ensino Particulares (PROSUP) and CAPES-PROEX” (grant number: 0001 and #88887.484856/2020-00), Brazil; the São Paulo Research Foundation (FAPESP: #2015/17275-8, #2017/06032-2, and #2022/03138-2); and the National Council for Scientific and Technological Development (CNPq: #409629/2021-9, #311839/2021-5, and #313376/2021-2). The authors would also like to thank the National funding by FCT- Foundation for Science and Technology, P.I., through the institutional scientific employment program-contract (CEECINST/00077/2021).
The authors declare that the research was conducted in the absence of any commercial or financial relationships that could be construed as a potential conflict of interest.
All claims expressed in this article are solely those of the authors and do not necessarily represent those of their affiliated organizations, or those of the publisher, the editors and the reviewers. Any product that may be evaluated in this article, or claim that may be made by its manufacturer, is not guaranteed or endorsed by the publisher.
1. Weaver TD. Neutral theory and the evolution of human physical form: an introduction to models and applications. J Anthropol Sci (2018) 96:7–26. doi: 10.4436/JASS.96009
2. Gardner C, Cole DC, Ryan L. Public health for the hunter-gatherer in us all. Can J Public Health (2020) 111:701. doi: 10.17269/S41997-020-00341-2
3. Comparative primate energetics and hominid evolution - PubMed. Available at: https://ncbi.nlm.nih.gov/9066904/ (Accessed December 4, 2022).
4. Pontzer H, Wood BM, Raichlen DA. Hunter-gatherers as models in public health. Obes Rev (2018) 19 Suppl 1:24–35. doi: 10.1111/OBR.12785
5. Freese J, Klement RJ, Ruiz-Núñez B, Schwarz S, Lötzerich H. The sedentary (r)evolution: Have we lost our metabolic flexibility? F1000Res (2017) 6. doi: 10.12688/F1000RESEARCH.12724.2
6. Prentice AM. Early influences on human energy regulation: Thrifty genotypes and thrifty phenotypes. Physiol Behav (2005) 86:640–5. doi: 10.1016/J.PHYSBEH.2005.08.055
7. Longman DP, Wells JCK, Stock JT. Human athletic paleobiology; using sport as a model to investigate human evolutionary adaptation. Am J Phys Anthropol (2020) 171 Suppl 70:42–59. doi: 10.1002/AJPA.23992
8. Wells JCK. The evolution of human fatness and susceptibility to obesity: An ethological approach. Biol Rev Camb Philos Soc (2006) 81:183–205. doi: 10.1017/S1464793105006974
9. Wu NN, Tian H, Chen P, Wang D, Ren J, Zhang Y. Physical exercise and selective autophagy: Benefit and risk on cardiovascular health. Cells (2019) 8. doi: 10.3390/CELLS8111436
10. Bernardo BC, Ooi JYY, Weeks KL, Patterson NL, McMullen JR. Understanding key mechanisms of exercise-induced cardiac protection to mitigate disease: Current knowledge and emerging concepts. Physiol Rev (2018) 98:419–75. doi: 10.1152/PHYSREV.00043.2016
11. Gao Z, Wang R. Children’s motor skill competence, physical activity, fitness, andhealthpromotion. J Sport Health Sci (2019) 8:95. doi: 10.1016/J.JSHS.2018.12.002
12. Global action plan on physical activity 2018–2030: more active people for a healthier world. Available at: https://www.who.int/publications/i/item/978924151418 (Accessed July 28, 2022).
13. Chomistek AK, Chasman DI, Cook NR, Rimm EB, Lee IM. Physical activity, genes for physical fitness, and risk of coronary heart disease. Med Sci Sports Exerc (2013) 45:691–7. doi: 10.1249/MSS.0B013E3182784E9F
14. de Barros MP, Bachi ALL, dos Santos J, Lambertucci RH, Ishihara R, Polotow TG, et al. The poorly conducted orchestra of steroid hormones, oxidative stress and inflammation in frailty needs a maestro: Regular physical exercise. Exp Gerontol (2021) 155. doi: 10.1016/J.EXGER.2021.111562
15. Schoenfeld BJ, Grgic J, Ogborn D, Krieger JW. Strength and hypertrophy adaptations between low- vs. high-load resistance training: A systematic review and meta-analysis. J Strength Cond Res (2017) 31:3508–23. doi: 10.1519/JSC.0000000000002200
16. Trewin AJ, Berry BJ, Wojtovich AP. Exercise and mitochondrial dynamics: Keeping in shape with ROS and AMPK. Antioxidants (2018) 7. doi: 10.3390/ANTIOX7010007
17. Fan Z, Turiel G, Ardicoglu R, Ghobrial M, Masschelein E, Kocijan T, et al. Exercise-induced angiogenesis is dependent on metabolically primed ATF3/4+ endothelial cells. Cell Metab (2021) 33:1793–1807.e9. doi: 10.1016/J.CMET.2021.07.015
18. Gomez-Cabrera MC, Carretero A, Millan-Domingo F, Garcia-Dominguez E, Correas AG, Olaso-Gonzalez G, et al. Redox-related biomarkers in physical exercise. Redox Biol (2021) 42. doi: 10.1016/J.REDOX.2021.101956
19. Severinsen MCK, Pedersen BK. Muscle-organ crosstalk: The emerging roles of myokines. Endocr Rev (2020) 41:594–609. doi: 10.1210/ENDREV/BNAA016
20. Gomez-Cabrera MC, Salvador-Pascual A, Cabo H, Ferrando B, Vina J. Redox modulation of mitochondriogenesis in exercise. does antioxidant supplementation blunt the benefits of exercise training? Free Radic Biol Med (2015) 86:37–46. doi: 10.1016/J.FREERADBIOMED.2015.04.006
21. Lee JH, Jun HS. Role of myokines in regulating skeletal muscle mass and function. Front Physiol (2019) 10:42/BIBTEX. doi: 10.3389/FPHYS.2019.00042/BIBTEX
22. di Liegro CM, Schiera G, Proia P, di Liegro I. Physical activity and brain health. Genes (Basel) (2019) 10:720. doi: 10.3390/GENES10090720
23. Pedersen BK, Febbraio MA. Muscles, exercise and obesity: Skeletal muscle as a secretory organ. Nat Rev Endocrinol (2012) 8:457–65. doi: 10.1038/NRENDO.2012.49
24. Whitham M, Febbraio MA. The ever-expanding myokinome: Discovery challenges and therapeutic implications. Nat Rev Drug Discovery (2016) 15:719–29. doi: 10.1038/NRD.2016.153
25. Morland C, Andersson KA, Haugen ØP, Hadzic A, Kleppa L, Gille A, et al. Exercise induces cerebral VEGF and angiogenesis via the lactate receptor HCAR1. Nat Commun (2017) 8:1–9. doi: 10.1038/NCOMMS15557
26. Moon HY, Becke A, Berron D, Becker B, Sah N, Benoni G, et al. Running-induced systemic cathepsin b secretion is associated with memory function. Cell Metab (2016) 24:332–40. doi: 10.1016/J.CMET.2016.05.025
27. Pedersen BK. Physical activity and muscle-brain crosstalk. Nat Rev Endocrinol (2019) 15:383–92. doi: 10.1038/S41574-019-0174-X
28. Lee B, Shin M, Park Y, Won SY, Cho KS. Physical exercise-induced myokines in neurodegenerative diseases. Int J Mol Sci (2021) 22:5795. doi: 10.3390/IJMS22115795
29. Khansari N, Shakiba Y, Mahmoudi M. Chronic inflammation and oxidative stress as a major cause of age-related diseases and cancer. Recent Pat Inflammation Allergy Drug Discov (2009) 3:73–80. doi: 10.2174/187221309787158371
30. Steensberg A, Fischer CP, Keller C, Møller K, Pedersen BK. IL-6 enhances plasma IL-1ra, IL-10, and cortisol in humans. Am J Physiol Endocrinol Metab (2003) 285:E433–7. doi: 10.1152/AJPENDO.00074.2003
31. Moldoveanu AI, Shephard RJ, Shek PN. The cytokine response to physical activity and training. Sports Med (2001) 31:115–44. doi: 10.2165/00007256-200131020-00004
32. Bachi ALL, Barros MP, Vieira RP, Rocha GA, de Andrade PBM, Victorino AB, et al. Combined exercise training performed by elderly women reduces redox indexes and proinflammatory cytokines related to atherogenesis. Oxid Med Cell Longev (2019) 2019. doi: 10.1155/2019/6469213
33. Sadjapong U, Yodkeeree S, Sungkarat S, Siviroj P. Multicomponent exercise program reduces frailty and inflammatory biomarkers and improves physical performance in community-dwelling older adults: A randomized controlled trial. Int J Environ Res Public Health 2020 Vol 17 Page 3760 (2020) 17:3760. doi: 10.3390/IJERPH17113760
34. Bruunsgaard H, Galbo H, Halkjaer-Kristensen J, Johansen TL, MacLean DA, Pedersen BK. Exercise-induced increase in serum interleukin-6 in humans is related to muscle damage. J Physiol (1997) 499:833–41. doi: 10.1113/JPHYSIOL.1997.SP021972
35. Gonzalez-Gil AM, Elizondo-Montemayor L. The role of exercise in the interplay between myokines, hepatokines, osteokines, adipokines, and modulation of inflammation for energy substrate redistribution and fat mass loss: A review. Nutrients (2020) 12:1. doi: 10.3390/NU12061899
36. Piccirillo R. Exercise-induced myokines with therapeutic potential for muscle wasting. Front Physiol (2019) 10:287/BIBTEX. doi: 10.3389/FPHYS.2019.00287/BIBTEX
37. Chow LS, Gerszten RE, Taylor JM, Pedersen BK, van Praag H, Trappe S, et al. Exerkines in health, resilience and disease. Nat Rev Endocrinol (2022) 18:273–89. doi: 10.1038/S41574-022-00641-2
38. Gao Y, Galis ZS. Exploring the role of endothelial cell resilience in cardiovascular health and disease. Arterioscler Thromb Vasc Biol (2021) 41:179–85. doi: 10.1161/ATVBAHA.120.314346
39. Leuchtmann AB, Adak V, Dilbaz S, Handschin C. The role of the skeletal muscle secretome in mediating endurance and resistance training adaptations. Front Physiol (2021) 12:709807. doi: 10.3389/FPHYS.2021.709807
40. Tejero J, Shiva S, Gladwin MT. Sources of vascular nitric oxide and reactive oxygen species and their regulation. Physiol Rev (2019) 99:311–79. doi: 10.1152/PHYSREV.00036.2017
41. Russell AP, Lamon S. Exercise, skeletal muscle and circulating microRNAs. Prog Mol Biol Transl Sci (2015) 135:471–96. doi: 10.1016/BS.PMBTS.2015.07.018
42. Fernandes T, Baraúna VG, Negrão CE, Ian Phillips M, Oliveira EM. Aerobic exercise training promotes physiological cardiac remodeling involving a set of microRNAs. Am J Physiol Heart Circ Physiol (2015) 309:H543–52. doi: 10.1152/AJPHEART.00899.2014
43. Gomes CPC, de Gonzalo-Calvo D, Toro R, Fernandes T, Theisen D, Wang DZ, et al. Non-coding RNAs and exercise: Pathophysiological role and clinical application in the cardiovascular system. Clin Sci (Lond) (2018) 132:925–42. doi: 10.1042/CS20171463
44. Banzet S, Chennaoui M, Girard O, Racinais S, Drogou C, Chalabi H, et al. Changes in circulating microRNAs levels with exercise modality. J Appl Physiol (1985) (2013) 115:1237–44. doi: 10.1152/JAPPLPHYSIOL.00075.2013
45. Maciorkowska M, Musiałowska D, Małyszko J. Adropin and irisin in arterial hypertension, diabetes mellitus and chronic kidney disease. Adv Clin Exp Med (2019) 28:1571–1575. doi: 10.17219/ACEM/104551
46. Maak S, Norheim F, Drevon CA, Erickson HP. Progress and challenges in the biology of FNDC5 and irisin. Endocr Rev (2021) 42:436–56. doi: 10.1210/ENDREV/BNAB003
47. Jedrychowski MP, Wrann CD, Paulo JA, Gerber KK, Szpyt J, Robinson MM, et al. Detection and quantitation of circulating human irisin by tandem mass spectrometry. Cell Metab (2015) 22:734–40. doi: 10.1016/J.CMET.2015.08.001
48. Bi J, Zhang J, Ren Y, Du Z, Li T, Wang T, et al. Irisin reverses intestinal epithelial barrier dysfunction during intestinal injury via binding to the integrin αVβ5 receptor. J Cell Mol Med (2020) 24:996. doi: 10.1111/JCMM.14811
49. Kim H, Wrann CD, Jedrychowski M, Vidoni S, Kitase Y, Nagano K, et al. Irisin mediates effects on bone and fat via αV integrin receptors. Cell (2018) 175:1756–1768.e17. doi: 10.1016/J.CELL.2018.10.025
50. Ma C, Ding H, Deng Y, Liu H, Xiong X, Yang Y. Irisin: A new code uncover the relationship of skeletal muscle and cardiovascular health during exercise. Front Physiol (2021) 12:620608. doi: 10.3389/FPHYS.2021.620608
51. Askari H, Rajani SF, Poorebrahim M, Haghi-Aminjan H, Raeis-Abdollahi E, Abdollahi M. A glance at the therapeutic potential of irisin against diseases involving inflammation, oxidative stress, and apoptosis: An introductory review. Pharmacol Res (2018) 129:44–55. doi: 10.1016/J.PHRS.2018.01.012
52. Zhang Y, Xie C, Wang H, Foss RM, Clare M, George EV, et al. Irisin exerts dual effects on browning and adipogenesis of human white adipocytes. Am J Physiol Endocrinol Metab (2016) 311:E530–41. doi: 10.1152/AJPENDO.00094.2016
53. Peng Q, Wang X, Wu K, Liu K, Wang S, Chen X. Irisin attenuates H2O2-induced apoptosis in cardiomyocytes via microRNA-19b/AKT/mTOR signaling pathway. Int J Clin Exp Pathol (2017) 10:7707.
54. Parr EB, Camera DM, Burke LM, Phillips SM, Coffey VG, Hawley JA. Circulating MicroRNA responses between ‘High’ and ‘Low’ responders to a 16-wk diet and exercise weight loss intervention. PLos One (2016) 11. doi: 10.1371/JOURNAL.PONE.0152545
55. Lebleu VS, O’Connell JT, Gonzalez Herrera KN, Wikman H, Pantel K, Haigis MC, et al. PGC-1α mediates mitochondrial biogenesis and oxidative phosphorylation in cancer cells to promote metastasis. Nat Cell Biol (2014) 16:992–1003. doi: 10.1038/NCB3039
56. Boström P, Wu J, Jedrychowski MP, Korde A, Ye L, Lo JC, et al. A PGC1-α-dependent myokine that drives brown-fat-like development of white fat and thermogenesis. Nature (2012) 481:463–8. doi: 10.1038/NATURE10777
57. Löffler D, Müller U, Scheuermann K, Friebe D, Gesing J, Bielitz J, et al. Serum irisin levels are regulated by acute strenuous exercise. J Clin Endocrinol Metab (2015) 100:1289–99. doi: 10.1210/JC.2014-2932
58. Rabiee F, Lachinani L, Ghaedi S, Nasr-Esfahani MH, Megraw TL, Ghaedi K. New insights into the cellular activities of Fndc5/Irisin and its signaling pathways. Cell Biosci (2020) 10:1–10. doi: 10.1186/S13578-020-00413-3
59. Sun Y, Liu WZ, Liu T, Feng X, Yang N, Zhou HF. Signaling pathway of MAPK/ERK in cell proliferation, differentiation, migration, senescence and apoptosis. J Recept Signal Transduct Res (2015) 35:600–4. doi: 10.3109/10799893.2015.1030412
60. Cargnello M, Roux PP. Activation and function of the MAPKs and their substrates, the MAPK-activated protein kinases. Microbiol Mol Biol Rev (2011) 75:50. doi: 10.1128/MMBR.00031-10
61. Zhang Y, Li R, Meng Y, Li S, Donelan W, Zhao Y, et al. Irisin stimulates browning of white adipocytes through mitogen-activated protein kinase p38 MAP kinase and ERK MAP kinase signaling. Diabetes (2014) 63:514–25. doi: 10.2337/DB13-1106
62. Miyamoto-Mikami E, Sato K, Kurihara T, Hasegawa N, Fujie S, Fujita S, et al. Endurance training-induced increase in circulating irisin levels is associated with reduction of abdominal visceral fat in middle-aged and older adults. PLos One (2015) 10. doi: 10.1371/JOURNAL.PONE.0120354
63. Rahimi GRM, Hejazi K, Hofmeister M. The effect of exercise interventions on irisin level: a systematic review and meta-analysis of randomized controlled trials. EXCLI J (2022) 21:524–39. doi: 10.17179/EXCLI2022-4703
64. Lavi G, Horwitz A, Einstein O, Zipori R, Gross O, Birk R. Fndc5/irisin is regulated by myogenesis stage, irisin, muscle type and training. Am J Transl Res (2022) 14:7063.
65. Mazur-Bialy AI, Pocheć E, Zarawski M. Anti-inflammatory properties of irisin, mediator of physical activity, are connected with TLR4/MyD88 signaling pathway activation. Int J Mol Sci (2017) 18:701. doi: 10.3390/IJMS18040701
66. Hosseini Farahabadi SS, Ghaedi K, Ghazvini Zadegan F, Karbalaie K, Rabiee F, Nematollahi M, et al. ERK1/2 is a key regulator of Fndc5 and PGC1α expression during neural differentiation of mESCs. Neuroscience (2015) 297:252–61. doi: 10.1016/J.NEUROSCIENCE.2015.03.069
67. Maalouf GE, El Khoury D. Exercise-induced irisin, the fat browning myokine, as a potential anticancer agent. J Obes (2019) 2019. doi: 10.1155/2019/6561726
68. Li H, Wang F, Yang M, Sun J, Zhao Y, Tang D. The effect of irisin as a metabolic regulator and its therapeutic potential for obesity. Int J Endocrinol (2021) 2021. doi: 10.1155/2021/6572342
69. de Sousa RAL, Improta-Caria AC, Souza BS de F. Exercise–linked irisin: Consequences on mental and cardiovascular health in type 2 diabetes. Int J Mol Sci (2021) 22:1–15. doi: 10.3390/IJMS22042199
70. Lu J, Xiang G, Liu M, Mei W, Xiang L, Dong J. Irisin protects against endothelial injury and ameliorates atherosclerosis in apolipoprotein e-null diabetic mice. Atherosclerosis (2015) 243:438–48. doi: 10.1016/J.ATHEROSCLEROSIS.2015.10.020
71. Liu C, Zhou J, Xu Y, Gong S, Zhu Y, Zhang H, et al. Irisin ameliorates oxidative stress-induced injury in pancreatic beta-cells by inhibiting txnip and inducing Stat3-Trx2 pathway activation. Oxid Med Cell Longev (2022) 2022. doi: 10.1155/2022/4674215
72. Lee HJ, Lee JO, Kim N, Kim JK, Kim HI, Lee YW, et al. Irisin, a novel myokine, regulates glucose uptake in skeletal muscle cells via AMPK. Mol Endocrinol (2015) 29:873–81. doi: 10.1210/ME.2014-1353
73. Mazur-Bialy AI. Superiority of the non-glycosylated form over the glycosylated form of irisin in the attenuation of adipocytic meta-inflammation: A potential factor in the fight against insulin resistance. Biomolecules (2019) 9:394. doi: 10.3390/BIOM9090394
74. Mazur-Bialy AI, Pocheć E. The time-course of antioxidant irisin activity: Role of the Nrf2/HO-1/HMGB1 axis. Antioxidants (Basel) (2021) 10:1–13. doi: 10.3390/ANTIOX10010088
75. Mazur-Bialy AI, Kozlowska K, Pochec E, Bilski J, Brzozowski T. Myokine irisin-induced protection against oxidative stress in vitro. involvement of heme oxygenase-1 and antioxidazing enzymes superoxide dismutase-2 and glutathione peroxidase. J Physiol Pharmacol (2018) 69:117–25. doi: 10.26402/JPP.2018.1.13
76. Metzger CE, Narayanan SA, Elizondo JP, Carter AM, Zawieja DC, Hogan HA, et al. DSS-induced colitis produces inflammation-induced bone loss while irisin treatment mitigates the inflammatory state in both gut and bone. Sci Rep (2019) 9:1–15. doi: 10.1038/s41598-019-51550-w
77. Anand Narayanan S, Metzger CE, Bloomfield SA, Zawieja DC. Inflammation-induced lymphatic architecture and bone turnover changes are ameliorated by irisin treatment in chronic inflammatory bowel disease. FASEB J (2018) 32:4848–61. doi: 10.1096/FJ.201800178R
78. Bilski J, Mazur-Bialy A, Brzozowski B, Magierowski M, Zahradnik-Bilska J, Wójcik D, et al. Can exercise affect the course of inflammatory bowel disease? experimental and clinical evidence. Pharmacol Rep (2016) 68:827–36. doi: 10.1016/J.PHAREP.2016.04.009
79. Lourenco MV, Frozza RL, de Freitas GB, Zhang H, Kincheski GC, Ribeiro FC, et al. Exercise-linked FNDC5/irisin rescues synaptic plasticity and memory defects in alzheimer’s models. Nat Med (2019) 25:165–75. doi: 10.1038/S41591-018-0275-4
80. Mo L, Shen J, Liu Q, Zhang Y, Kuang J, Pu S, et al. Irisin is regulated by car in liver and is a mediator of hepatic glucose and lipid metabolism. Mol Endocrinol (2016) 30:533–42. doi: 10.1210/ME.2015-1292/SUPPL_FILE/ME-15-1292.PDF
81. Xin C, Liu J, Zhang J, Zhu D, Wang H, Xiong L, et al. Irisin improves fatty acid oxidation and glucose utilization in type 2 diabetes by regulating the AMPK signaling pathway. Int J Obes (Lond) (2016) 40:443–51. doi: 10.1038/IJO.2015.199
82. Fatouros IG. Is irisin the new player in exercise-induced adaptations or not? a 2017 update. Clin Chem Lab Med (2018) 56:525–48. doi: 10.1515/CCLM-2017-0674/ASSET/GRAPHIC/J_CCLM-2017-0674_FIG_001.JPG
83. Jandova T, Buendía-Romero A, Polanska H, Hola V, Rihova M, Vetrovsky T, et al. Long-term effect of exercise on irisin blood levels-systematic review and meta-analysis. Healthcare (Basel) (2021) 9:1438. doi: 10.3390/HEALTHCARE9111438
84. McPhee JS, French DP, Jackson D, Nazroo J, Pendleton N, Degens H. Physical activity in older age: perspectives for healthy ageing and frailty. Biogerontology (2016) 17:567. doi: 10.1007/S10522-016-9641-0
85. Martinez Munoz IY, Camarillo Romero EDS, Garduno Garcia JJ. Irisin a novel metabolic biomarker: Present knowledge and future directions. Int J Endocrinol (2018) 2018. doi: 10.1155/2018/7816806
86. Ruan Q, Huang Y, Yang L, Ruan J, Gu W, Zhang X, et al. The effects of both age and sex on irisin levels in paired plasma and cerebrospinal fluid in healthy humans. Peptides (NY) (2019) 113:41–51. doi: 10.1016/J.PEPTIDES.2019.01.004
87. Marrano N, Biondi G, Borrelli A, Cignarelli A, Perrini S, Laviola L, et al. Irisin and incretin hormones: Similarities, differences, and implications in type 2 diabetes and obesity. Biomolecules (2021) 11:1–23. doi: 10.3390/BIOM11020286
88. de Freitas GB, Lourenco MV, de Felice FG. Protective actions of exercise-related FNDC5/Irisin in memory and alzheimer’s disease. J Neurochem (2020) 155:602–11. doi: 10.1111/JNC.15039
89. Zerlotin R, Oranger A, Pignataro P, Dicarlo M, Maselli F, Mori G, et al. Irisin and secondary osteoporosis in humans. Int J Mol Sci (2022) 23:690. doi: 10.3390/IJMS23020690
90. Marra F, Bertolani C. Adipokines in liver diseases. Hepatology (2009) 50:957–69. doi: 10.1002/HEP.23046
91. Rashid FA, Abbas HJ, Naser NA, Addai Ali H. Effect of long-term moderate physical exercise on irisin between normal weight and obese men. ScientificWorldJournal (2020) 2020. doi: 10.1155/2020/1897027
92. Leustean L, Preda C, Teodoriu L, Mihalache L, Arhire L, Ungureanu MC. Role of irisin in endocrine and metabolic disorders–possible new therapeutic agent? Appl Sci (2021) 11:5579. doi: 10.3390/APP11125579
93. Arhire LI, Mihalache L, Covasa M. Irisin: A hope in understanding and managing obesity and metabolic syndrome. Front Endocrinol (Lausanne) (2019) 10:524. doi: 10.3389/FENDO.2019.00524
94. Marseglia A, Darin-Mattsson A, Skoog J, Rydén L, Hadarsson-Bodin T, Kern S, et al. Metabolic syndrome is associated with poor cognition: A population-based study of 70-Year-Old adults without dementia. Journals Gerontology: Ser A (2021) 76:2275–83. doi: 10.1093/GERONA/GLAB195
95. Ma’u E, Cullum S, Cheung G, Livingston PG, Mukadam N. Differences in the potential for dementia prevention between major ethnic groups within one country: A cross sectional analysis of population attributable fraction of potentially modifiable risk factors in new Zealand. Lancet Reg Health West Pac (2021) 13:100191. doi: 10.1016/J.LANWPC.2021.100191
96. Islam MR, Valaris S, Young MF, Haley EB, Luo R, Bond SF, et al. Author correction: Exercise hormone irisin is a critical regulator of cognitive function. Nat Metab (2021) 3:1432–2. doi: 10.1038/s42255-021-00476-7
97. Valenzuela PL, Castillo-García A, Morales JS, de la Villa P, Hampel H, Emanuele E, et al. Exercise benefits on alzheimer’s disease: State-of-the-science. Ageing Res Rev (2020) 62. doi: 10.1016/J.ARR.2020.101108
98. El Hayek L, Khalifeh M, Zibara V, Abi Assaad R, Emmanuel N, Karnib N, et al. Lactate mediates the effects of exercise on learning and memory through SIRT1-dependent activation of hippocampal brain-derived neurotrophic factor (BDNF). J Neurosci (2019) 39:2369. doi: 10.1523/JNEUROSCI.1661-18.2019
99. Wrann CD, White JP, Salogiannnis J, Laznik-Bogoslavski D, Wu J, Ma D, et al. Exercise induces hippocampal BDNF through a PGC-1α/FNDC5 pathway. Cell Metab (2013) 18:649–59. doi: 10.1016/J.CMET.2013.09.008
100. Wang K, Song F, Xu K, Liu Z, Han S, Li F, et al. Irisin attenuates neuroinflammation and prevents the memory and cognitive deterioration in streptozotocin-induced diabetic mice. Mediators Inflammation (2019) 2019. doi: 10.1155/2019/1567179
101. Moon HS, Dincer F, Mantzoros CS. Pharmacological concentrations of irisin increase cell proliferation without influencing markers of neurite outgrowth and synaptogenesis in mouse H19-7 hippocampal cell lines. Metabolism (2013) 62:1131–6. doi: 10.1016/J.METABOL.2013.04.007
102. Müller P, Duderstadt Y, Lessmann V, Müller NG. Lactate and BDNF: Key mediators of exercise induced neuroplasticity? J Clin Med (2020) 9:1136. doi: 10.3390/JCM9041136
103. Puig MV, Rose J, Schmidt R, Freund N. Dopamine modulation of learning and memory in the prefrontal cortex: Insights from studies in primates, rodents, and birds. Front Neural Circuits (2014) 8:93. doi: 10.3389/FNCIR.2014.00093
104. Matura S, Fleckenstein J, Deichmann R, Engeroff T, Füzéki E, Hattingen E, et al. Effects of aerobic exercise on brain metabolism and grey matter volume in older adults: Results of the randomised controlled SMART trial. Transl Psychiatry (2017) 7:e1172. doi: 10.1038/TP.2017.135
105. Alves HR, Lomba GSB, Gonçalves-de-Albuquerque CF, Burth P. Irisin, exercise, and COVID-19. Front Endocrinol (Lausanne) (2022) 13:879066. doi: 10.3389/FENDO.2022.879066
Keywords: skeletal muscle, exercise, exerkines, myokines, oxidative stress, inflammaging
Citation: Trettel CdS, Pelozin BRdA, Barros MP, Bachi ALL, Braga PGS, Momesso CM, Furtado GE, Valente PA, Oliveira EM, Hogervorst E and Fernandes T (2023) Irisin: An anti-inflammatory exerkine in aging and redox-mediated comorbidities. Front. Endocrinol. 14:1106529. doi: 10.3389/fendo.2023.1106529
Received: 23 November 2022; Accepted: 16 January 2023;
Published: 10 February 2023.
Edited by:
Joanna Jaworska, Medical University of Gdansk, PolandReviewed by:
José Rodrigo Pauli, State University of Campinas, BrazilCopyright © 2023 Trettel, Pelozin, Barros, Bachi, Braga, Momesso, Furtado, Valente, Oliveira, Hogervorst and Fernandes. This is an open-access article distributed under the terms of the Creative Commons Attribution License (CC BY). The use, distribution or reproduction in other forums is permitted, provided the original author(s) and the copyright owner(s) are credited and that the original publication in this journal is cited, in accordance with accepted academic practice. No use, distribution or reproduction is permitted which does not comply with these terms.
*Correspondence: Tiago Fernandes, dGlmZXJuYW5kZXNAdXNwLmJy
†These authors have contributed equally to this work and share first authorship
‡ORCID: Caio dos Santos Trettel, orcid.org/0000-0003-1188-9661
Bruno Rocha de Avila Pelozin, orcid.org/0000-0002-9066-0488
Marcelo Paes Barros, orcid.org/0000-0003-3565-8331
André Luis Lacerda Bachi, orcid.org/0000-0001-8266-1416
Pedro Gabriel Senger Braga, orcid.org/0000-0002-6690-6345
César Miguel Momesso, orcid.org/0000-0003-3565-8331
Guilherme Eustáquio Furtado, orcid.org/0000-0001-8327-1567
Pedro Afonso Valente, orcid.org/0000-0003-4204-7870
Edilamar Menezes Oliveira, orcid.org/0000-0002-7101-312X
Eef Hogervorst, orcid.org/0000-0001-9729-4989
Tiago Fernandes, orcid.org/0000-0002-9057-3878
Disclaimer: All claims expressed in this article are solely those of the authors and do not necessarily represent those of their affiliated organizations, or those of the publisher, the editors and the reviewers. Any product that may be evaluated in this article or claim that may be made by its manufacturer is not guaranteed or endorsed by the publisher.
Research integrity at Frontiers
Learn more about the work of our research integrity team to safeguard the quality of each article we publish.