- 1Department of Parasitology, Guangdong Medical University, Zhanjiang, Guangdong, China
- 2Department of Pharmacy, Affiliated Hospital of Guangdong Medical University, Zhanjiang, China
Background: Bromodomain and extracellular terminal (BET) family (including BRD2, BRD3, and BRD4) is considered to be a major driver of cancer cell growth and a new target for cancer therapy. Currently, more than 30 targeted inhibitors have shown significant inhibitory effects against various tumors in preclinical and clinical trials. However, the expression levels, gene regulatory networks, prognostic value, and target prediction of BRD2, BRD3, and BRD4 in adrenocortical carcinoma (ACC) have not been fully elucidated. Therefore, this study aimed to systematically analyze the expression, gene regulatory network, prognostic value, and target prediction of BRD2, BRD3, and BRD4 in patients with ACC, and elucidated the association between BET family expression and ACC. We also provided useful information on BRD2, BRD3, and BRD4 and potential new targets for the clinical treatment of ACC.
Methods: We systematically analyzed the expression, prognosis, gene regulatory network, and regulatory targets of BRD2, BRD3, and BRD4 in ACC using multiple online databases, including cBioPortal, TRRUST, GeneMANIA, GEPIA, Metascape, UALCAN, LinkedOmics, and TIMER.
Results: The expression levels of BRD3 and BRD4 were significantly upregulated in ACC patients at different cancer stages. Moreover, the expression of BRD4 was significantly correlated with the pathological stage of ACC. ACC patients with low BRD2, BRD3, and BRD4 expressions had longer survival than patients with high BRD2, BRD3, and BRD4 expressions. The expression of BRD2, BRD3, and BRD4 was altered by 5%, 5%, and 12% in 75 ACC patients, respectively. The frequency of gene alterations in the 50 most frequently altered BRD2, BRD3, and BRD4 neighboring genes in these ACC patients were ≥25.00%, ≥25.00%, and ≥44.44%, respectively. BRD2, BRD3, and BRD4 and their neighboring genes form a complex network of interactions mainly through co-expression, physical interactions, and shared protein domains. Molecular functions related to BRD2, BRD3, and BRD4 and their neighboring genes mainly include protein-macromolecule adaptor activity, cell adhesion molecule binding, and aromatase activity. Chemokine signaling pathway, thiamine metabolism, and olfactory transduction were found to be enriched as per the KEGG pathway analysis. SP1, NPM1, STAT3, and TP53 are key transcription factors for BRD2, BRD4, and their neighboring genes. MiR-142-3P, miR-484, and miR-519C were the main miRNA targets of BRD2, BRD3, BRD4, and their neighboring genes. We analyzed the mRNA sequencing data from 79 patients with ACC and found that ZSCAN12, DHX16, PRPF4B, EHMT1, CDK5RAP2, POMT1, WIZ, ZNF543, and AKAP8 were the top nine genes whose expression were positively associated with BRD2, BRD3, and BRD4 expression. The expression level of BRD2, BRD3, and BRD4 positively correlated with B cell and dendritic cell infiltration levels. BRD4-targeted drug PFI-1 and (BRD2, BRD3, and BRD4)-targeted drug I-BET-151 may have good inhibitory effects on the SW13 cell line.
Conclusions: The findings of this study provide a partial basis for the role of BRD2, BRD3, and BRD4 in the occurrence and development of ACC. In addition, this study also provides new potential therapeutic targets for ACC, which can serve as a reference for future basic and clinical research.
1 Introduction
Adrenal cortical carcinoma (ACC) is a sporadic adrenocortical endocrine tumor with an annual incidence of 0.5 to 2 cases per million. The number of cases in the female population generally outnumbers that of the male population (1.5:1) (1, 2). The clinical manifestations of ACC can be in three different forms. Symptoms related to hormone excess occur in 60% of the patients with ACC. Another 20% of patients with ACC have abdominal pain or fullness due to tumor growth, and the remaining 20% have unrelated symptoms detected by abdominal imaging (3–5). The prognosis of ACC is also extremely poor since the clinical presentation of ACC is often difficult to determine, and most patients are diagnosed in the late metastatic stage of the disease. Although ACC patients with the locoregional disease are treated with surgery, approximately 75% of patients experience recurrence after treatment (6). In addition, for advanced metastatic disease, the median overall survival was 12–15 months, and the 5-year overall survival was <15% (7, 8). Patients with ACC did not benefit from improvements in overall tumor treatment when compared to those with other tumor types. Evidence suggests that chemotherapy and radiation therapy are ineffective in most ACC cases. Mitotane, the only drug currently approved by the U.S. Food and Drug Administration for ACC, is usually only temporarily effective, and its use is often accompanied by significant side effects (9, 10). Complete tumor resection remains the only curative treatment (11). Therefore, in the current situation of uncertain therapeutic effects, limited toxic drugs, and high risk of disease recurrence, it is important to systematically analyze targeted prediction and prognostic markers in patients with ACC.
Bromodomain and extracellular terminal (BET) family consists of four members, including BRD2, BRD3, BRD4, and BRDT, which play key roles in various cell processes, including cell cycle, apoptosis, migration, and invasion (12). Therefore, the BET family can enhance its carcinogenic function by increasing its expression or promoting the transcriptional activity of carcinogenic factors. BET family is over-expressed in a variety of human cancers, which are closely related to human carcinogenesis (13). They are considered to be attractive therapeutic targets for selectively inhibiting cancer patients (14). However, BRDT is primarily expressed in germ cells, and BRD2, BRD3, and BRD4 are ubiquitously expressed. Among them, BRD4 is the most extensively studied in tumor research. BRD2, BRD3, and BRD4 can specifically recognize acetylated lysine residues and regulate the replication and transcription of related genes, thereby affecting key processes in cell carcinogenesis (15). They have two tandem bromodomains (BD1 and BD2) that regulate gene expression by binding to promoters and super-enhancer regions and promoting transcriptional elongation in cancer. The inhibition of BRD2, BRD3, and BRD4 shortens signaling between super-enhancer regions and oncogene target promoters, resulting in cell-specific inhibition of oncogene expression and ultimately cancer cell death. They contain multiple acetylated residues and activate transcription by binding to acetylated lysine residues on target proteins and mediating chromatin decompression (16). Studies have reported that BRD4 inhibitors treat cancer by interfering with the interaction between BRD4 and acetyl lysine on target proteins (17). Currently, more than 30 targeted inhibitors have shown significant inhibitory effects against various tumors in preclinical and clinical trials (18). Therefore, BRD2, BRD3, and BRD4 may be biomarkers for some cancers, and the development of their inhibitors may be a potential strategy for cancer treatment.
The role of BRD2, BRD3, and BRD4 in ACC is not well understood. Therefore, this study systematically analyzed the expression, gene regulatory network, prognostic value, and target prediction of BRD2, BRD3, and BRD4 in ACC patients, elucidated the association between BET family and ACC, and identified potential new targets for ACC therapy.
2 Materials and methods
2.1 UALCAN analysis
UALCAN (http://ualcan.path.uab.edu/analysis.html) is an online professional database for analyzing tumor gene expression levels. We used UALCAN to analyze the expression levels of BRD2, BRD3, and BRD4 in ACC patients with different cancer stages. The “Expression Analysis” module of UALCAN database was used to analyze TCGA gene expression data, and the screening criteria were set as: (1) gene: BRD2, BRD3, and BRD4; (2) dataset: ACC; (3) 77 ACC patients (9 in stage 1, 37 in stage 2, 16 in stage 3, and 15 in stage 4); and threshold setting conditions: P-value cutoff = 0.05. The Student’s t-test was used for comparative analysis.
2.2 GEPIA
Gene Expression Profiling (GEPIA) (http://gepia.cancer-pku.cn/index.html) is a free online platform for analyzing the correlation of gene expression levels with the tumor pathological stage and prognostic value. We used GEPIA to analyze the pathological stage correlation and prognostic value of the expression level of BRD2, BRD3, and BRD4 in patients with ACC. The screening criteria were: (1) Gene: BRD2, BRD3, and BRD4; (2) dataset: ACC; and (3) threshold setting conditions: P-value cutoff = 0.05. The Student’s t-test was used to analyze the expression of BRD2, BRD3, BRD4, and the pathological stage of ACC. The Kaplan–Meier curve was used to analyze the prognosis of patients with ACC.
2.3 cBioPortal analysis
cBioPortal (http://cbioportal.org) is an online professional database used to analyze genetic alterations in tumors. We used the cBioPortal database to analyze genetic alterations in BRD2, BRD3, BRD4, and their neighboring genes. A total of 75 ACC samples were analyzed, and mRNA expression z-scores were obtained relative to all samples (log RNA Seq V2 RSEM) using a z-score threshold of ±2.0.
2.4 STRING analysis
STRING (https://string-db.org/cgi/input.pl) is an online professional database for analyzing protein-protein interactions (PPI). We used STRING to build a low-confidence level (0.150) PPI network interaction and screening criteria for species defined as humans.
2.5 GeneMANIA analysis
GeneMANIA (http://www.genemania.org) is a free professional tool for analyzing gene functions. We used GeneMANIA to explore the function of BRD2, BRD3, BRD4, and the top 50 altered neighboring genes, respectively.
2.6 Metascape analysis
Metascape (https://metascape.org) is a professional-free tool for analyzing gene Gene Ontology (GO) functions and Kyoto Encyclopedia of Genes and Genomes (KEGG) pathway enrichment. We used Metascape to analyze the GO function and KEGG pathway enrichment of BRD2, BRD3, BRD4, and their altered neighboring genes in ACC.
2.7 TRRUST analysis
TRRUST (https://www.grnpedia.org/trrust/) is an online professional database that analyzes gene transcription regulators. We used the TRRUST database to analyze the transcriptional regulators of BRD2, BRD3, BRD4, and their altered neighboring genes in patients with ACC.
2.8 LinkedOmics analysis
LinkedOmics (http://www.linkedomics.org/) is a free online platform for analyzing miRNA target enrichment and differentially-expressed genes (DEGs) associated with tumor genes. We used the LinkedOmics database to analyze the miRNA target enrichment and differentially-expressed genes associated with BRD2, BRD3, and BRD4.
2.9 Timer analysis
TIMER (https://cistrome.shinyapps.io/timer/) is a specialized database for systematically analyzing tumor genes associated with infiltrating immune cells. We used TIMER to analyze the correlation between BRD2, BRD3, and BRD4 expression with immune cell infiltration levels.
2.10 Genomics of drug sensitivity in cancer analysis
The Genomics of Drug Sensitivity in Cancer (http://www.cancerRxgene.org) is a specialized public database for obtaining information on antitumor drug sensitivity. We used this database to find targeted drugs of BRD2, BRD3, and BRD4, and predict their anti-ACC activity.
3 Results
3.1 BET family expression in patients with ACC
We first compared the expression levels of BRD2, BRD3, and BRD4 in ACC patients with different cancer stages and found that the BRD3 (stage 4) and BRD4 transcript levels were significantly upregulated in patients with ACC (P < 0.05) (Figures 1B, C). However, BRD2 transcript levels did not change (Figures 1A). In addition, we evaluated the correlation between the differential expression of BRD2, BRD3, and BRD4 with the pathological stage of ACC. We found a significant correlation between the expression of BRD4 and the pathological stage of patients with ACC (P = 0.0104) (Supplementary Figure 1C). Finally, we used GEPIA to assess the prognostic value of BRD2, BRD3, and BRD4 expressions in ACC patients. Our results showed that ACC patients with low BRD3 and BRD4 expressions had longer overall survival than those with high BRD3 (P = 0.0054) (Figure 1F) and BRD4 expressions (P = 0.0066) (Figure 1H). Similarly, ACC patients with low BRD2, BRD3, and BRD4 expressions had longer disease-free survival than those with high BRD2 (P = 0.0042) (Figure 1E), BRD3 (P = 0.00012) (Figure 1G), and BRD4 expressions (P = 3.9e-5) (Figure 1I). However, BRD2 expressions had no effect on the overall survival of ACC patients (Figure 1D).
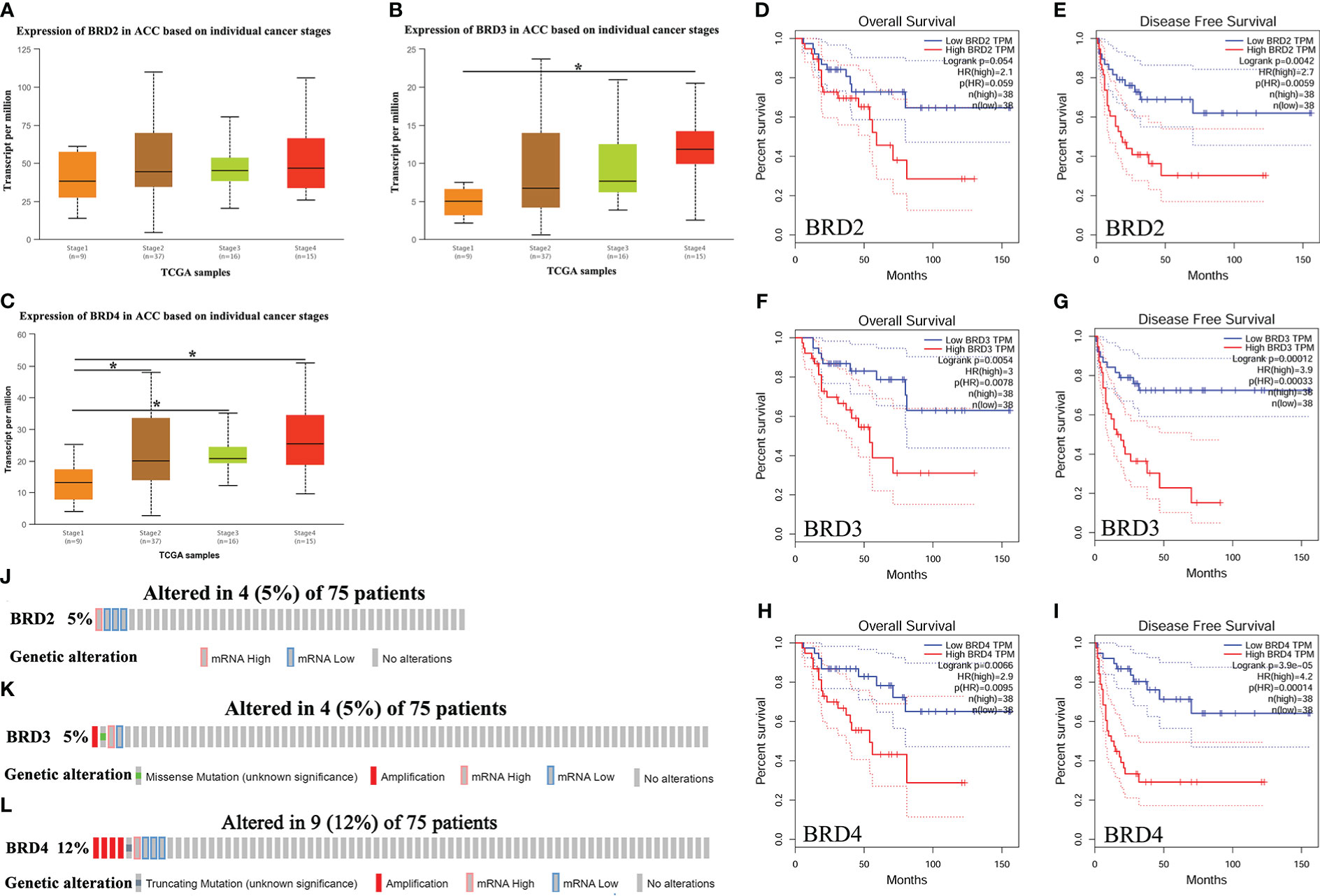
Figure 1 The expression, prognostic value, and genetic alteration of BET family in ACC patients (UALCAN, GEPIA, and cBioPortal). (A) The expression of BRD2 in ACC patients based on individual cancer stages (UALCAN); (B) The expression of BRD3 in ACC patients based on individual cancer stages (UALCAN); (C) The expression of BRD4 in ACC patients based on individual cancer stages (UALCAN); (D) The overall survival curve of BRD2 in patients with ACC (GEPIA); (E) The disease-free survival cure of BRD2 in patients with ACC (GEPIA); (F) The overall survival curve of BRD3 in patients with ACC (GEPIA); (G) The disease-free survival cure of BRD3 in patients with ACC (GEPIA); (H) The overall survival curve of BRD4 in patients with ACC (GEPIA); (I) The disease-free survival cure of BRD4 in patients with ACC (GEPIA). (J) Genetic alteration of BRD2 in ACC (cBioPortal); (K) Genetic alteration of BRD3 in ACC (cBioPortal); (L) Genetic alteration of BRD4 in ACC (cBioPortal). Data are expresssed as mean ± SE. *P < 0.05.
3.2 Genetic alteration of the BET family in patients with ACC
We further assessed genetic alterations in BRD2, BRD3, and BRD4 in 75 patients with ACC using The Cancer Genome Atlas (TCGA). We found that the expression of BRD2 and BRD3 was altered by 5% in patients with ACC, with the type of genetic alteration mainly including high and low RNA levels (Figures 1J, K). However, the expression of BRD4 was altered by 12% in patients with ACC, with the type of genetic alteration mainly including truncating mutation, amplification, and high and low RNA levels (Figure 1L).
3.3 Neighboring gene alteration and BET family interaction network in patients with ACC
We evaluated the alterations in the neighboring genes of BRD2, BRD3, and BRD4 in patients with ACC using the cBioPortal. Our results showed a gene alteration frequency of ≥25.00% for 50 of the most frequently altered neighboring genes of BRD2 and BRD3 in patients with ACC (Tables 1, 2). However, we found a gene alteration frequency of ≥ 44.44% for the 50 most frequently altered neighboring genes of BRD4 in patients with ACC (Table 3). Furthermore, the most frequently altered neighboring genes of BRD2, BRD3, and BRD4 in patients with ACC were CLDN23 (75.00%), PLEC (75.00%), MAL2 (75.00%), SYNE1 (75.00%), GPRIN2 (75.00%), ADAMTS13 (50.00%), NOTCH3 (55.56%), ANGPTL6 (44.44%), and C19ORF38 (44.44%) (Tables 1- 3).
We further evaluated the potential interactions between BRD2, BRD3, BRD4, and their neighboring genes. We found that 37 nodes and 100 edges were obtained in the constructed PPI network of BRD2 and its neighboring genes in patients with ACC (Figure 2A). Furthermore, BRD2 and its neighboring genes were linked to a complex interaction network (66 genes and 118 edges) through prediction, co-expression, physical interactions, co-localization, and shared protein domains (Figure 2B). We found that 41 nodes and 126 edges were obtained in the constructed PPI network of BRD3 and its neighboring genes in patients with ACC (Figure 2C). BRD3 and its neighboring genes were linked to a complex interaction network (69 genes and 221 edges) through co-expression, physical interactions, shared protein domains, genetic interactions, and co-localization (Figure 2D). Our results showed that 38 nodes and 128 edges were obtained in the constructed PPI network of BRD4 and its neighboring genes in patients with ACC (Figure 2E). BRD4 and its neighboring genes were linked to a complex interaction network (62 genes and 233 edges) through co-expression, physical interactions, shared protein domains, and colocalization (Figure 2F).
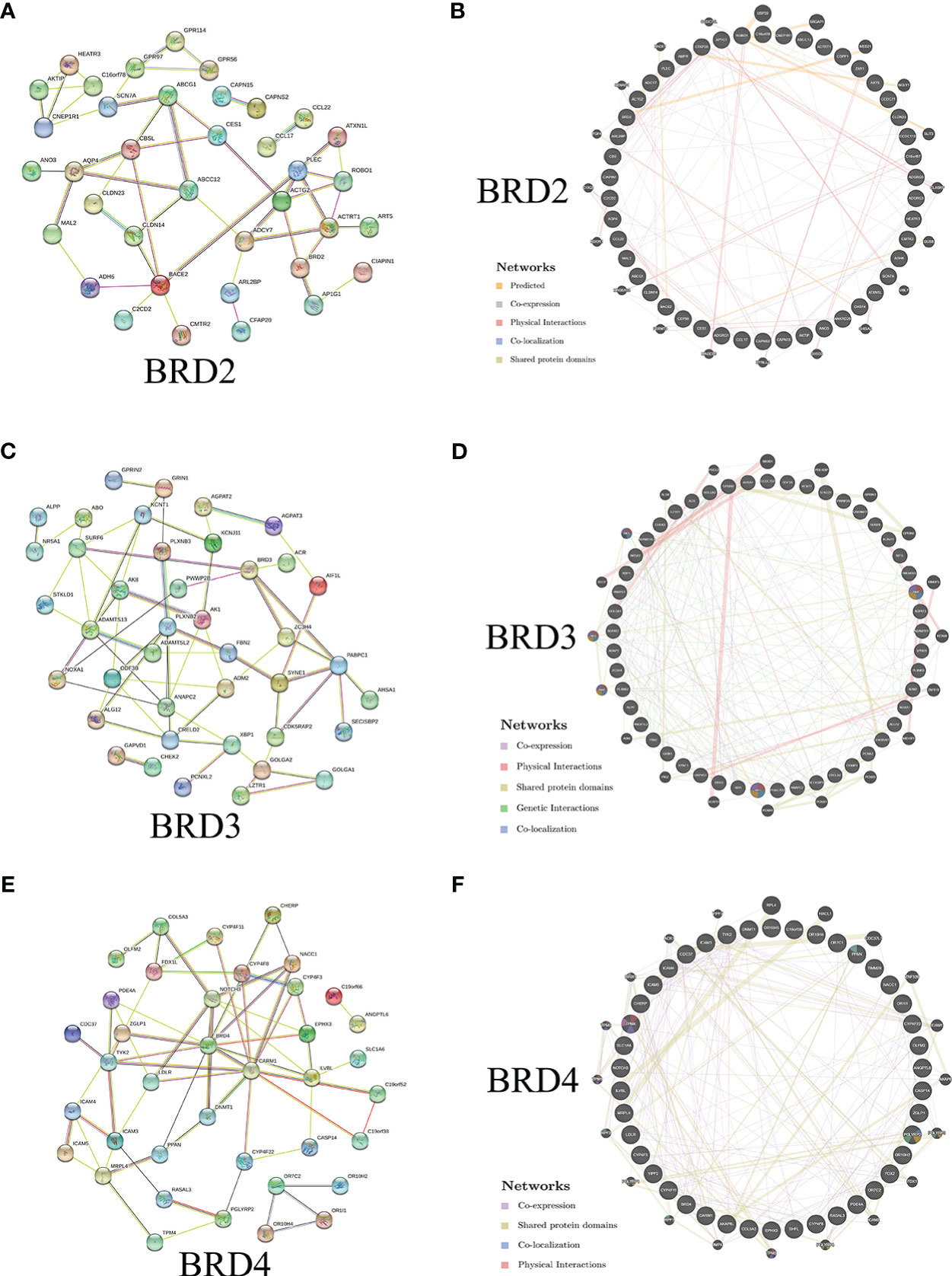
Figure 2 Interaction analyses of BET family and their neighboring genes in ACC (STRING and GeneMANIA). (A) PPI network of BRD2 and its neighboring genes in patients with ACC (STRING); (B) Network analyses of BRD2 and its neighboring genes in patients with ACC (GeneMANIA); (C) PPI network of BRD3 and its neighboring genes in patients with ACC (STRING); (D) Network analyses of BRD3 and its neighboring genes in patients with ACC (GeneMANIA); (E) PPI network of BRD4 and its neighboring genes in patients with ACC (STRING); (F) Network analyses of BRD4 and its neighboring genes in patients with ACC (GeneMANIA).
3.4 GO and KEGG pathway enrichment analyses
We further performed the GO and KEGG pathway enrichment analyses of BRD2, BRD3, BRD4, and the top 50 altered neighboring genes in ACC patients using Metascape. We found that biological processes related to BRD2 and its neighboring genes in patients with ACC mainly include adenylate cyclase-activating G protein-coupled receptor signaling pathway, generation of precursor metabolites and energy, and lymphocyte chemotaxis (Figure 3A). Their molecular functions include protein-macromolecule adaptor activity, endopeptidase activity, and channel activity (Figure 3B). Their cellular components related to BRD2 and its neighboring genes in patients with ACC mainly include glial cell projection, trans-Golgi network, and perinuclear region of cytoplasm (Figure 3C). Chemokine signaling pathway was found to be enriched as per the KEGG pathway analysis (Figure 3D). Furthermore, our results showed that biological processes related to BRD3 and its neighboring genes in patients with ACC mainly include angiogenesis, peptide metabolic process, and microtubule cytoskeleton organization involved in mitosis (Figure 3E). Their molecular functions include cell adhesion molecule binding, calcium ion binding, and voltage-gated monoatomic ion channel activity (Figure 3F). Their cellular components related to BRD3 and its neighboring genes in patients with ACC mainly include extracellular matrix, Golgi membrane, and polymeric cytoskeletal fiber (Figure 3G). Thiamine metabolism was found to be enriched as per the KEGG pathway analysis (Figure 3H). Finally, we found that biological processes related to BRD4 and its neighboring genes in patients with ACC mainly include sensory perception of smell, icosanoid metabolic processes, positive regulation of histone modification, and phagocytosis (Figure 3I). Their molecular functions include aromatase activity, G protein-coupled serotonin receptor activity, and integrin binding (Figure 3J). Olfactory transduction was found to be enriched as per the KEGG pathway analysis (Figure 3K).
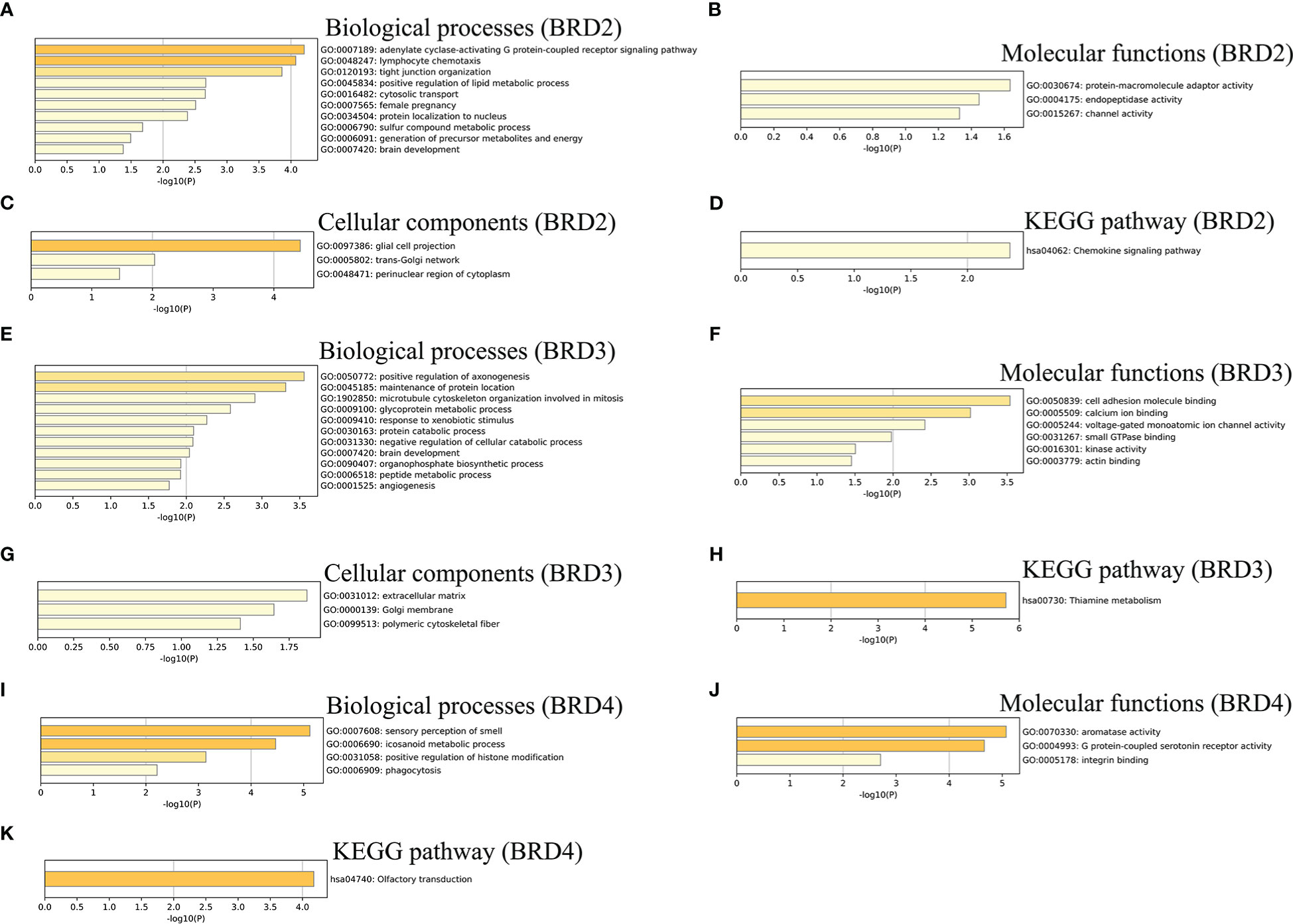
Figure 3 GO function and KEGG pathways enrichment analyses of BET family and their neighboring genes in ACC (metascape). (A) Biological processes of BRD2 and its neighboring genes; (B) Molecular functionsf BRD2 and its neighboring genes; (C) Cellular components of BRD2 and its neighboring genes; (D) KEGG pathway analysis of BRD2 and its neighboring genes; (E) Biological processes of BRD3 and its neighboring genes; (F) Molecular functionsf BRD3 and its neighboring genes; (G) Cellular components of BRD3 and its neighboring genes; (H) KEGG pathway analysis of BRD3 and its neighboring genes; (I) Biological processes of BRD4 and its neighboring genes; (J) Molecular functionsf BRD4 and its neighboring genes; (K) KEGG pathway analysis of BRD4 and its neighboring genes.
3.5 Transcription factor and miRNA targets regulating BET family expression in patients with ACC
We used TRRUST to analyze the key regulatory factors of BRD2, BRD3, and BRD4 in patients with ACC. Our results showed that SP1 may be the key transcription factor of BRD2 and its neighboring genes in patients with ACC (P < 0.05) (Table 4). Among them, ADH6, BACE2, CBS, CES1, and CHST4 may be the main regulatory genes of SP1. Furthermore, we found that NPM1, STAT3, and TP53 may be the key transcription factors of BRD4 and its neighboring genes in patients with ACC (P < 0.05) (Table 4). Among them, BRD4 and DNMT1 may be the main regulatory genes of NPM1. STAT3 regulates the functions of DNMT1 and TYK2. Moreover, CARM1 and DNMT1 may be the main regulatory genes for TP53. Next, we analyzed the miRNA targets of BRD2, BRD3, and BRD4 using LinkedOmics. The top three miRNA targets of BRD2 in patients with ACC may be (ACACTAC) miR-142-3P, (GTGACTT) miR-224, and (TAATGTG) miR-323 (FDP < 0.01) (Table 5). (GAGCCTG) miR-484, (GCACCTT) miR-18A, miR-18B, and (GTCTTCC) miR-7 may be the top three miRNA targets of BRD3 in patients with ACC (FDP < 0.05) (Table 5). (TGCACTT) miR-519C, miR-519B, miR-519A, (GCACTTT) miR-17-5P, miR-20A, miR-106A, miR-106B, miR-20B, miR-519D, and (ATGTACA) miR-493 may be the top three miRNA targets of BRD4 in patients with ACC (FDP = 0) (Table 5).
3.6 Correlation of differentially expressed genes and BET family expression in patients with ACC
We analyzed the mRNA sequencing data from 79 patients with ACC using the LinkedOmics TCGA database. In patients with ACC, 19,339 genes were closely related to BRD2, BRD3, and BRD4 (Figures 4A, D, and G). Among them, positive and negative genes (9,647 and 9,692, 9,115 and 10,224, 10,028 and 9,311) were found correlate with BRD2, BRD3, and BRD4 expressions, respectively (Figures 4A, 4D and G). Moreover, we found that 50 genes had a notable positive or negative correlation with BRD2, BRD3, and BRD4 expressions in patients with ACC (P < 0.05) (Figures 4B, C, E, F, H, and I). Among them, BRD2 expression was strongly positively associated with the expression of ZSCAN12 (Pearson correlation coefficient (PCO) = 0.7111, P = 2.092e–13; Figure 4J), DHX16 (PCO = 0.6981, P = 8.628e–13; Figure 4K), and PRPF4B (PCO = 0.6937, P = 1.371e–12; Figure 4L). However, the expression of BRD3 was positively associated with the expression of EHMT1 (PCO = 0.8005, P = 8.586e–19; Figure 4M), CDK5RAP2 (Pearson correlation = 0.7338, P = 1.437e–14; Figure 4N), and POMT1 (PCO = 0.7237, P = 4.921e–14; Figure 4O). Furthermore, WIZ (PCO = 0.7339, P = 1.418e-14; Figure 4P), ZNF543 (PCO = 0.6332, P = 3.8e-10; Figure 4Q), and AKAP8 (PCO = 0.6325, P = 4.027e-10; Figure 4R) were the top three genes whose expressions were positively correlated with the expression of BRD4.
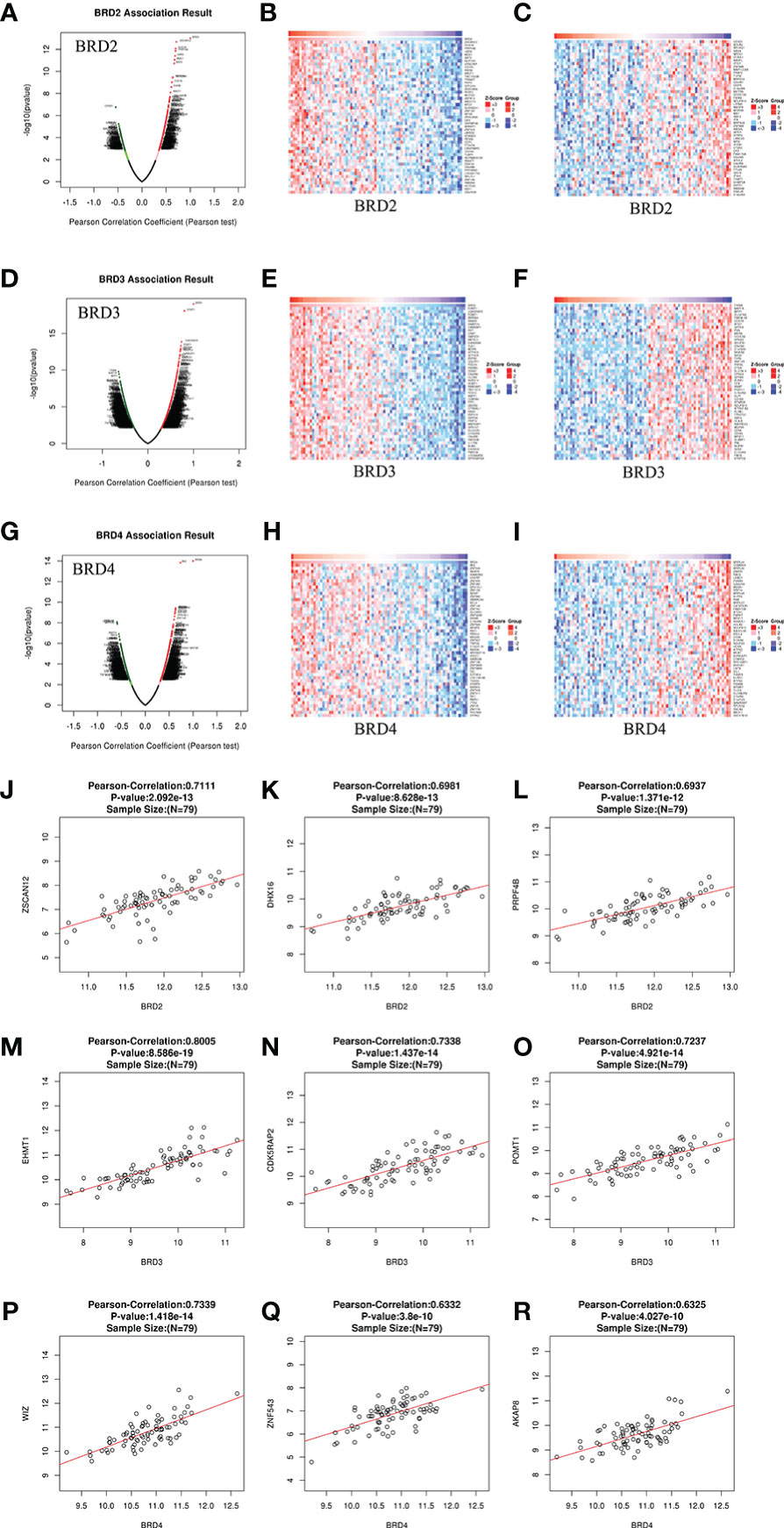
Figure 4 Genes differentially expressed in correlation with BET family expression in ACC (LinkedOmics). (A) Pearson test was used to analyze correlations between BRD2 expression and genes differentially expressed in patients with ACC; (B, C) Heatmaps showing genes positively and negatively correlated, respectively, with BRD2 in patients with ACC (top 50 genes); (D) Pearson test was used to analyze correlations between BRD3 expression and genes differentially expressed in patients with ACC; (E, F) Heatmaps showing genes positively and negatively correlated, respectively, with BRD3 in patients with ACC (top 50 genes); (G) Pearson test was used to analyze correlations between BRD4 expression and genes differentially expressed in patients with ACC; (H, I) Heatmaps showing genes positively and negatively correlated, respectively, with BRD4 in patients with ACC (top 50 genes). (J, K, L) The scatter plots show Pearson’s correlation of BRD2 expression with expression of ZSCAN12, DHX16, and PRPF4B, respectively, in patients with ACC; (M, N, O) The scatter plots show Pearson’s correlation of BRD3 expression with expression of EHMT1, CDK5RAP2, and POMT1, respectively, in patients with ACC; (P, Q, R) The scatter plots show Pearson’s correlation of BRD4 expression with expression of WIZ, ZNF543, and AKAP8, respectively, in patients with ACC.
3.7 Immune cell infiltration and BET family expression in patients with ACC
We used TIMER to evaluate the relationship between immune cell infiltration levels and BRD2, BRD3, and BRD4 expressions in patients with ACC. Our results showed that the expression levels of BRD2, BRD3, and BRD4 in patients with ACC were positively correlated mainly with B-cell and dendritic cell infiltration levels (P<0.05; Figures 5A-C).
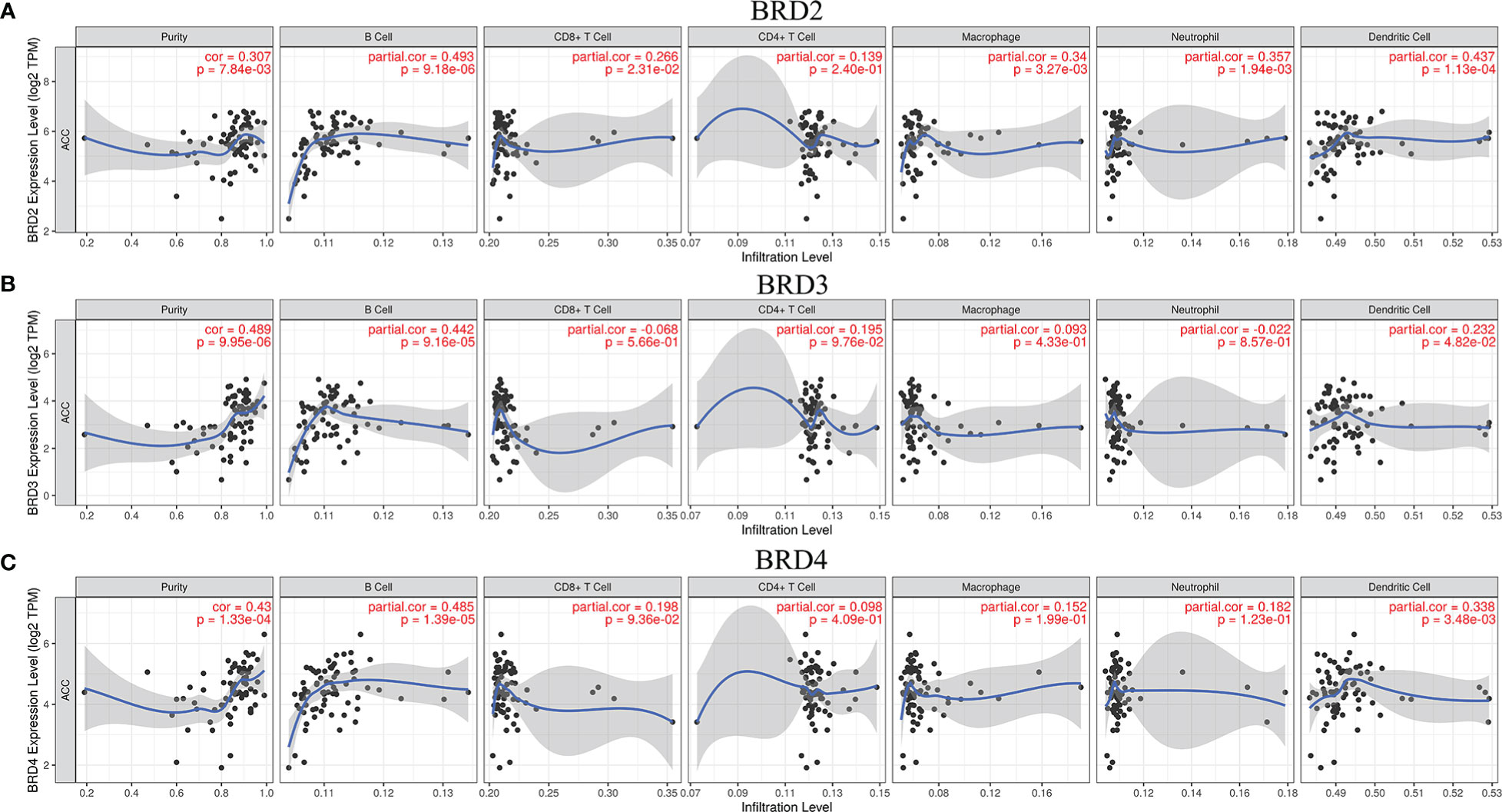
Figure 5 The correlation between BET family expression and immune cell infiltration levels in ACC (TIMER). (A) BRD2; (B) BRD3; (C) BRD4.
3.8 BET family targeting Drugs
We used the Genomics of Drug Sensitivity in Cancer database to evaluate the inhibitory effect of BRD4-targeted drug PFI-1 and (BRD2, BRD3, and BRD4)-targeted drug I-BET-151 on ACC cell lines. PFI-1 inhibited 914 cell lines with area under the curve (AUC) values greater than 0.548 (Figure 6B). It had a good inhibitory effect on these cell lines (0.820 ≤ IC50 (μM) ≤ 367) (Figure 6A). Furthermore, we found that PFI-1 had good inhibitory effect on SW13 (a cell line of ACC) (AUC values =0.863, IC50 (μM) = 8.66) (Figures 6C, D). However, I-BET-151 inhibited 899 cell lines with area under the curve (AUC) values greater than 0.200 (Figure 6F). It had a good inhibitory effect on these cell lines (0.0922 ≤ IC50 (μM) ≤ 301) (Figure 6E). Furthermore, we found that I-BET-151 had a good inhibitory effect on SW13 (AUC values =0.680559, IC50 (μM) = 1.999834) (Figures 6G, H).
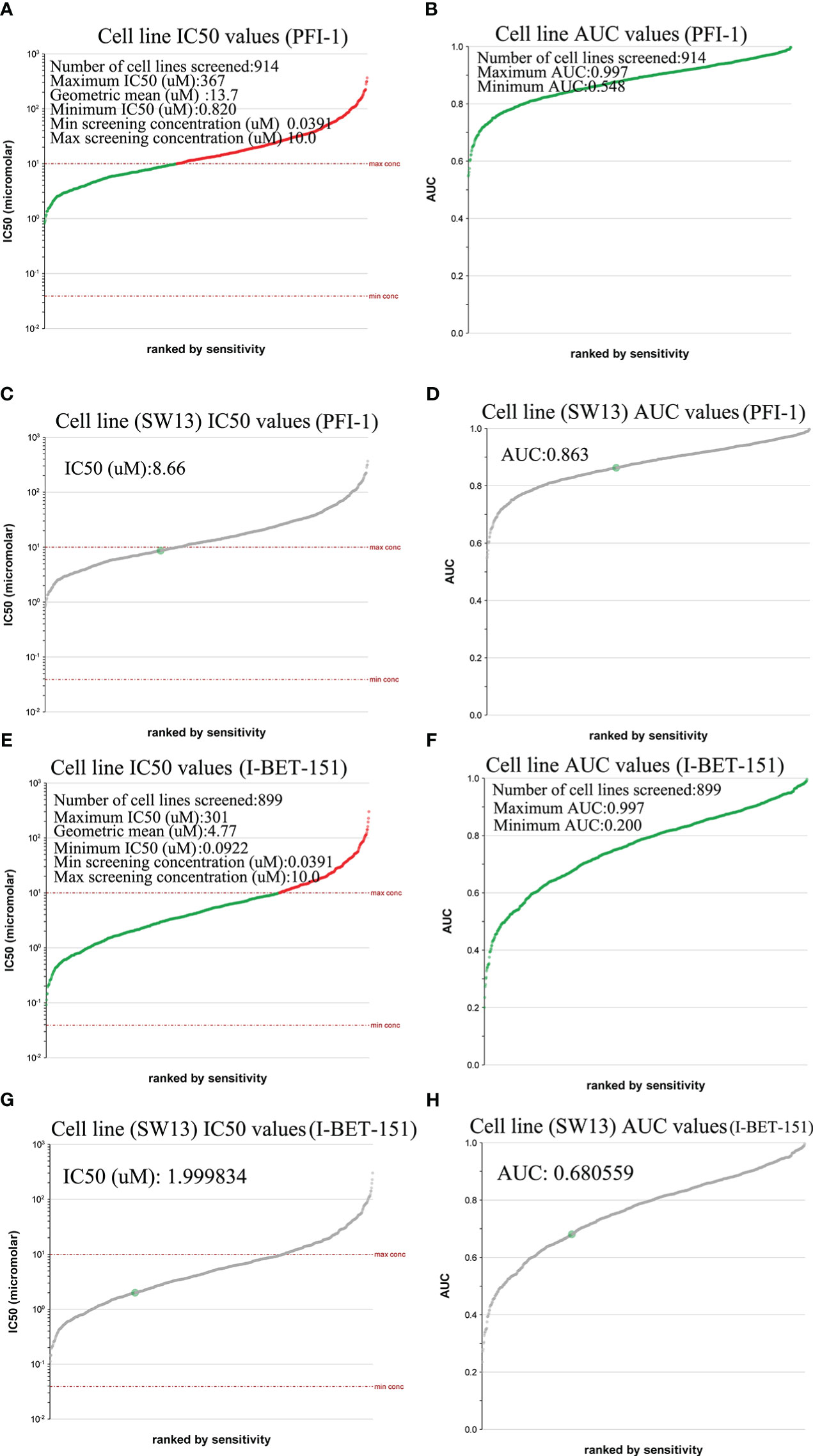
Figure 6 IC50 evaluation of PFI-1 and I-BET-151 in different tissue types of cancer (Genomics of Drug Sensitivity in Cancer). (A) Cell line IC50 values of PFI-1; (B) Cell line AUC values of PFI-1; (C) SW13 cell line IC50 values of PFI-1; (D) SW13cell line AUC values of PFI-1. (E) Cell line IC50 values of I-BET-151; (F) Cell line AUC values of I-BET-151; (G) SW13 Cell line IC50 values of I-BET-151; (H) SW13cell line AUC values of I-BET-151.
4 Discussion
The expressions of BRD2 and BRD3 in patients with ACC still remain unclear. BRD4 expression is reported to be significantly upregulated in ACC (19). However, the expression of BRD4 has not been reported for the individual cancer stages of patients with ACC. We first compared the expression levels of BRD2, BRD3, and BRD4 in ACC patients with different cancer stages and found that BRD3 and BRD4 transcript levels were significantly upregulated in patients with ACC. Furthermore, we found a significant positive correlation between the expression of BRD4 and the pathological stage of patients with ACC. In cancer patients with high BRD4 expression, increased BRD4 activity is associated with higher expression of oncogenes such as MYC, NOTCH3, and NRG1 (20). BRD4-driven oncogenes promote tumor cell proliferation, metastasis, and increased chemoresistance (20). Our results also revealed that the expression of BRD2, BRD3, and BRD4 was altered by 5%, 5%, and 12%, respectively, in patients with ACC, with the type of genetic alteration mainly including high and low RNA levels. Increased expression of BRD3 and BRD4 caused by genetic changes may also be an important factor. However, this requires further investigation. Finally, we assessed the prognostic value of BRD2, BRD3, and BRD4 expression in ACC patients. Our results showed that ACC patients with low BRD2, BRD3, and BRD4 expression had longer survival than those with high BRD2, BRD3, and BRD4 expression. BRD2, BRD3, and BRD4 may serve as potential prognostic markers in patients with ACC.
Our results showed a gene alteration frequency of ≥25.00%, ≥25.00%, and ≥44.44% for the 50 most frequently altered neighboring genes of BRD2, BRD3, and BRD4, respectively, in patients with ACC. We further evaluated the potential interactions between BRD2, BRD3, BRD4, and their neighboring genes. We found that BRD2, BRD3, BRD4, and their neighboring genes were linked to a complex interaction network through co-expression, physical interactions, and shared protein domains. Next, we evaluated the functions of BRD2, BRD3, and BRD4 and their neighboring genes. Our results showed that the biological processes related to BRD2 and its neighboring genes mainly include adenylate cyclase-activating G protein-coupled receptor signaling pathway, generation of precursor metabolites and energy, and lymphocyte chemotaxis. These biological processes affecting tumor proliferation, invasion, and metastasis have been reported (21–23). Furthermore, the biological processes related to BRD3 and its neighboring genes mainly include angiogenesis and microtubule cytoskeleton organization involved in mitosis. Abnormal angiogenesis and mitosis are the key factors of tumor occurrence and development (24, 25). Moreover, our results showed that the biological processes related to BRD4 and its neighboring genes mainly include positive regulation of histone modification and phagocytosis. Histone modification is a reversible process mediated by epigenetic enzymes (26). Histone methylation and acetylation are two important chemical modifications. They play important roles in transcriptional activation/inactivation, chromosomal packaging, and DNA damage/repair (27). The molecular functions of BRD2 and its neighboring genes include endopeptidase activity and channel activity. Various endopeptidase activities affect tumorigenesis and prognosis. Prolyl endopeptidase is a serine peptidase involved in the differentiation, development, and proliferation of various tissues. Recent studies have shown that the expression and activity of this cytoplasmic enzyme increases in colorectal cancer and affects the prognosis of colon cancer patients (28). The molecular functions of BRD3 and its neighboring genes include cell adhesion molecule binding, calcium ion binding, and voltage-gated monoatomic ion channel activity. Cell adhesion is a key mediator of cancer progression and promotes cancer hallmarks including immune escape and metastatic spread (29). Calcium (Ca) ion is a key secondary messenger in excitable and unexcitable cells. Ca signaling has received limited attention as a potential target for anticancer therapy (30). However, the molecular functions of BRD4 and its neighboring genes include aromatase activity, G protein-coupled serotonin receptor activity, and integrin binding. Recently, it has been proved that ACC cell overexpress aromatase and estrogen receptor, and estrogen synthesized by aromatase can enhance the proliferation of ACC cells (31). ACC can cause Cushing’s syndrome. However, some studies have shown that several receptors, including G protein-coupled serotonin receptors, are frequently co-expressed in patients with Cushing’s syndrome (32). Integrins are cell-adhesion receptors. However, integrin expression patterns are frequently altered in cancer. The expression of certain integrins is associated with increased metastasis and decreased cancer (33). Chemokine signaling pathway, thiamine metabolism, and olfactory transduction were enriched as per the KEGG pathway analysis for BRD2, BRD3, BRD4, and their neighboring genes. The tumor microenvironment consists of stromal cells and tumor cells, which interact with each other through complex crosstalk mediated by a variety of growth factors, cytokines, and chemokines. Chemokines play a key role in promoting tumor cell proliferation (34). Thiamine supplementation may contribute to tumor cell survival, proliferation, and chemotherapy resistance. However, some studies suggest that thiamine may exhibit some antitumor effects. The role of thiamine in cancer is controversial (35). Olfactory receptors specifically expressed in certain cancer cells, such as prostate-specific G protein-coupled receptor 1 (PSGR1), are significantly elevated in prostate cancer. NDRG1 affects oncogenic signaling pathways and tumor progression (36). There is no relevant research on whether olfactory receptors are specifically expressed in ACC patients. Taken together, the functions and signaling pathways involving BRD2, BRD3, BRD4, and their neighboring genes may be involved in the occurrence and progression of ACC. The regulation of these genes and signaling pathways may be a potential treatment strategy for ACC.
Next, we explored the transcription factor and miRNA targets of BRD2, BRD3, and BRD4 in patients with ACC. We found that SP1, NPM1, STAT3, and TP53 are the key transcription factors of BRD2, BRD4, and their neighboring genes in patients with ACC. SP1 is a well-known member of the transcription factor family, which plays an important role in cell growth, differentiation, apoptosis, and carcinogenesis (37). However, its role in the ACC has not been reported. Studies have shown that STAT3 can promote angiogenesis in patients with ACC, thereby making STAT3 a selective target for molecular-targeted therapy of ACC (38). ACC is a rare tumor type associated with TP53 mutations. Studies have shown that genetic susceptibility caused by mutations in TP53 is associated with approximately 50% of childhood ACC cases but only 3–6% of adult cases (39). The relationship between NPM1 and ACC has not yet been reported, but may be a new potential therapeutic target for ACC. Furthermore, our results showed that miR-142-3P, miR-224, miR-323, miR-484, miR-18A, miR-18B, miR-7, miR-519C, miR-519B, miR-519A, miR-17-5P, miR-20A, miR-106A, miR-106B, miR-20B, miR-519D, and miR-493 are targets of BRD2, BRD3, and BRD4 in patients with ACC. They are associated with tumor cell proliferation, migration, invasion, and drug resistance, and may be promising targets for cancer therapy (40–42). However, their relationship with ACC has not yet been reported. Our results suggest that these transcription factor and miRNA targets of BRD2, BRD3, BRD4, and their neighboring genes may be potentially therapeutic in treating ACC.
We explored the correlation between the differentially expressed genes and BET family expression in patients with ACC. We found that the expression of 19,339 genes was correlated with BRD2, BRD3, and BRD4 expression. Among them, ZSCAN12, DHX16, PRPF4B, EHMT1, CDK5RAP2, POMT1, WIZ, ZNF543, and AKAP8 were the top nine genes whose expressions were positively correlated with the expression of BRD2, BRD3, and BRD4. Therefore, targeting these cells may provide additional therapy for ACC. Tumor immune infiltration is closely related to the clinical prognosis (43). As expected, the expression levels of BRD2, BRD3, and BRD4 in patients with ACC positively correlated with B cell and dendritic cell infiltration levels. Targeting BRD2, BRD3, and BRD4 or their related regulatory targets may be a feasible strategy for reducing immune cell infiltration levels in patients with ACC. We further evaluated the inhibitory effect of BRD4-targeting drug PFI-1 and (BRD2, BRD3, and BRD4)-targeted drug I-BET-151 on ACC lines. We found that PFI-1 and I-BET-151 inhibited 914 and 899 cancer cell lines, respectively. Among them, I-BET-151 had a better inhibitory effect on the SW13 cell line than PFI-1. In short, PFI-1 and I-BET-151 exhibited broad-spectrum inhibitory effects on cancer cells. Therefore, finding inhibitors of BRD2, BRD3, and BRD4 or their regulatory targets may be an important strategy for the treatment of ACC.
In conclusion, this study systematically analyzed the expression, gene regulatory network, prognostic value, and target prediction of BRD2, BRD3, and BRD4 in patients with ACC, elucidated the association between BRD2, BRD3, BRD4 and ACC, and provided insights into the mechanism and treatment of ACC.
Data availability statement
The original contributions presented in the study are included in the article/Supplementary Material. Further inquiries can be directed to the corresponding authors.
Author contributions
LD and YS performed data analysis work and aided in writing the manuscript. YS designed the study and assisted in writing the manuscript. QL, ZZ, JC, ZS, QX, XL, YC, LL, JZ edited the manuscript. All authors contributed to the article and approved the submitted version.
Funding
This work was supported by Guangdong province ordinary colleges and universities young innovative talents project (4SG21202G), national pharmaceutical economic information network science and technology communication innovation project of chinese pharmaceutical association (CMEI2021KPYJ00310), postdoctoral foundation of Guangdong medical university (4SG22292G), and the project of financial fund science and technology special competitive allocation of Zhanjiang (Zhanke[2010]174).
Conflict of interest
The authors declare that the research was conducted in the absence of any commercial or financial relationships that could be construed as a potential conflict of interest.
Publisher’s note
All claims expressed in this article are solely those of the authors and do not necessarily represent those of their affiliated organizations, or those of the publisher, the editors and the reviewers. Any product that may be evaluated in this article, or claim that may be made by its manufacturer, is not guaranteed or endorsed by the publisher.
Supplementary material
The Supplementary Material for this article can be found online at: https://www.frontiersin.org/articles/10.3389/fendo.2023.1089531/full#supplementary-material
Supplementary Figure 1 | Correlation between the expression of BET family and the pathological stage of ACC (GEPIA). (A) BRD2; (B) BRD3; (C) BRD4.
References
1. Golden SH, Robinson KA, Saldanha I, Anton B, Ladenson PW. Clinical review: prevalence and incidence of endocrine and metabolic disorders in the united states: a comprehensive review. J Clin Endocrinol Metab (2009) 94:1853e1878. doi: 10.1210/jc.2008-2291
2. Else T, Kim AC, Sabolch A, Raymond VM, Kandathil A, Caoili EM, et al. Adrenocortical carcinoma. Endocr Rev (2014) 35:282e326. doi: 10.1210/er.2013-1029
3. Clay MR, Pinto EM, Fishbein L, Else T, Kiseljak-Vassiliades K. Pathological and genetic stratification for management of adrenocortical carcinoma. J Clin Endocrinol Metab (2022) 107(4):1159–69. doi: 10.1210/clinem/dgab866
4. Puglisi S, Perotti P, Pia A, Reimondo G, Terzolo M. Adrenocortical carcinoma with hypercortisolism. Endocrinol Metab Clin North Am (2018) 47:395e407. doi: 10.1016/j.ecl.2018.02.003
5. Fassnacht M, Allolio B. Clinical management of adrenocortical carcinoma. Best Pract Res Clin Endocrinol Metab (2009) 23:273e289. doi: 10.1016/j.beem.2008.10.008
6. Glenn JA, Else T, Hughes DT, Cohen MS, Jolly S, Giordano TJ, et al. Longitudinal patterns of recurrence in patients with adrenocortical carcinoma. Surgery. (2019) 165:186e195. doi: 10.1016/j.surg.2018.04.068
7. Fassnacht M, Kroiss M, Allolio B. Update in adrenocortical carcinoma. J Clin Endocrinol Metab (2013) 98:4551e4564. doi: 10.1210/jc.2013-3020
8. Baur J, Buntemeyer TO, Megerle F, Deutschbein T, Spitzweg C, Quinkler M, et al. Outcome after resection of adrenocortical carcinoma liver metastases: a retrospective study. BMC Cancer. (2017) 17:522. doi: 10.1186/s12885-017-3506-z
9. Postlewait LM, Ethun CG, Tran TB, Prescott JD, Pawlik TM, Wang TS, et al. Outcomes of adjuvant mitotane after resection of adrenocortical carcinoma: a 13-institution study by the US adrenocortical carcinoma group. J Am Coll Surg (2016) 222:480e490. doi: 10.1016/j.jamcollsurg.2015.12.013
10. Kroiss M, Quinkler M, Lutz WK, Allolio B, Fassnacht M. Drug interactions with mitotane by induction of CYP3A4 metabolism in the clinical management of adrenocortical carcinoma. Clin Endocrinol (Oxf). (2011) 75:585e591. doi: 10.1111/j.1365-2265.2011.04214.x
11. Mir MC, Klink JC, Guillotreau J, Long JA, Miocinovic R, Kaouk JH, et al. Comparative outcomes of laparoscopic and open adrenalectomy for adrenocortical carcinoma: single, high-volume center experience. Ann Surg Oncol (2013) 20:1456e1461. doi: 10.1245/s10434-012-2760-1
12. Belkina AC, Denis GV. BET domain co-regulators in obesity, inflammation and cancer. Nat Rev Cancer. (2012) 12(7):465–77. doi: 10.1038/nrc3256
13. French CA, Miyoshi I, Kubonishi I, Grier HE, Perez-Atayde AR, Fletcher JA. BRD4-NUT fusion oncogene: a novel mechanism in aggressive carcinoma. Cancer Res (2003) 63(2):304–7.
14. Dai X, Gan W, Li X, Wang S, Zhang W, Huang L, et al. Prostate cancer-associated SPOP mutations confer resistance to BET inhibitors through stabilization of BRD4. Nat Med (2017) 23(9):1063–71. doi: 10.1038/nm.4378
15. Wu SY, Chiang CM. The double bromodomain-containing chromatin adaptor Brd4 and transcriptional regulation. J Biol Chem (2007) 282(18):13141–5. doi: 10.1074/jbc.R700001200
16. Choudhary C, Kumar C, Gnad F, Nielsen ML, Rehman M, Walther TC, et al. Lysine acetylation targets protein complexes and co-regulates major cellular functions. Science. (2009) 325(5942):834–40. doi: 10.1126/science.1175371
17. Liu Z, Wang P, Chen H, Wold EA, Tian B, Brasier AR, et al. Drug discovery targeting bromodomain-containing protein 4. J Med Chem (2017) 60(11):4533–58. doi: 10.1021/acs.jmedchem.6b01761
18. Liu N, Ling R, Tang X, Yu Y, Zhou Y, Chen D. Post-translational modifications of BRD4: Therapeutic targets for tumor. Front Oncol (2022) 12:847701. doi: 10.3389/fonc.2022.847701
19. Zhu DD, Yu XB, Jiang W, Zhu Y. Pathological stage-associated non-coding RNA long intergenic non-protein coding RNA 1234 (LINC01234) participation in cell cycle regulation in adrenocortical carcinoma through bromodomain-containing protein 4 (BRD4) expression mediation via sponging microRNA (miR)-140-3p. Bioengineered. (2022) 13(5):13607–21. doi: 10.1080/21655979.2022.2081464
20. Drumond-Bock AL, Bieniasz M. The role of distinct BRD4 isoforms and their contribution to high-grade serous ovarian carcinoma pathogenesis. Mol Cancer. (2021) 20(1):145. doi: 10.1186/s12943-021-01424-5
21. Zia F, Fagarasan M, Bitar K, Coy DH, Pisegna JR, Wank SA, et al. Pituitary adenylate cyclase activating peptide receptors regulate the growth of non-small cell lung cancer cells. Cancer Res (1995) 55(21):4886–91.
22. Griffiths HBS, Williams C, King SJ, Allison SJ. Nicotinamide adenine dinucleotide (NAD+): essential redox metabolite, co-substrate and an anti-cancer and anti-ageing therapeutic target. Biochem Soc Trans (2020) 48(3):733–44. doi: 10.1042/BST20190033
23. Farhood B, Najafi M, Mortezaee K. CD8+ cytotoxic T lymphocytes in cancer immunotherapy: A review. J Cell Physiol (2019) 234(6):8509–21. doi: 10.1002/jcp.27782
24. Situ Y, Xu Q, Deng L, Zhu Y, Gao R, Lei L, et al. System analysis of VEGFA in renal cell carcinoma: The expression, prognosis, gene regulation network and regulation targets. Int J Biol Markers. (2022) 37(1):90–101. doi: 10.1177/17246008211063501
25. Lopes D, Maiato H. The tubulin code in mitosis and cancer. Cells. (2020) 9(11):2356. doi: 10.3390/cells9112356
26. Zou C, Mallampalli RK. Regulation of histone modifying enzymes by the ubiquitin-proteasome system. Biochim Biophys Acta (2014) 1843(4):694–702. doi: 10.1016/j.bbamcr.2013.12.016
27. Greer EL, Shi Y. Histone methylation: a dynamic mark in health, disease and inheritance. Nat Rev Genet (2012) 13(5):343–57. doi: 10.1038/nrg3173
28. Larrinaga G, Perez I, Blanco L, Sanz B, Errarte P, Beitia M, et al. Prolyl endopeptidase activity is correlated with colorectal cancer prognosis. Int J Med Sci (2014) 11(2):199–208. doi: 10.7150/ijms.7178
29. Läubli H, Borsig L. Altered cell adhesion and glycosylation promote cancer immune suppression and metastasis. Front Immunol (2019) 10:2120. doi: 10.3389/fimmu.2019.02120
30. Marchi S, Giorgi C, Galluzzi L, Pinton P. Ca2+ fluxes and cancer. Mol Cell (2020) 78(6):1055–69. doi: 10.1016/j.molcel.2020.04.017
31. Barzon L, Masi G, Pacenti M, Trevisan M, Fallo F, Remo A, et al. Expression of aromatase and estrogen receptors in human adrenocortical tumors. Virchows Arch (2008) 452(2):181–91. doi: 10.1007/s00428-007-0542-0
32. El Ghorayeb N, Bourdeau I, Lacroix A. Multiple aberrant hormone receptors in cushing's syndrome. Eur J Endocrinol (2015) 173(4):M45–60. doi: 10.1530/EJE-15-0200
33. Yousefi H, Vatanmakanian M, Mahdiannasser M, Mashouri L, Alahari NV, Monjezi MR, et al. Understanding the role of integrins in breast cancer invasion, metastasis, angiogenesis, and drug resistance. Oncogene. (2021) 40(6):1043–63. doi: 10.1038/s41388-020-01588-2
34. Hussain M, Adah D, Tariq M, Lu Y, Zhang J, Liu J. CXCL13/CXCR5 signaling axis in cancer. Life Sci (2019) 227:175–86. doi: 10.1016/j.lfs.2019.04.053
35. Lu'o'ng KV, Nguyễn LT. The role of thiamine in cancer: possible genetic and cellular signaling mechanisms. Cancer Genomics Proteomics. (2013) 10(4):169–85.
36. Wiese H, Gelis L, Wiese S, Reichenbach C, Jovancevic N, Osterloh M, et al. Quantitative phosphoproteomics reveals the protein tyrosine kinase Pyk2 as a central effector of olfactory receptor signaling in prostate cancer cells. Biochim Biophys Acta (2015) 1854(6):632–40. doi: 10.1016/j.bbapap.2014.09.002
37. Beishline K, Azizkhan-Clifford J. Sp1 and the 'hallmarks of cancer'. FEBS J (2015) 282(2):224–58. doi: 10.1111/febs.13148
38. Zhu Y, Xu Y, Chen D, Zhang C, Rui W, Zhao J, et al. Expression of STAT3 and IGF2 in adrenocortical carcinoma and its relationship with angiogenesis. Clin Transl Oncol (2014) 16(7):644–9. doi: 10.1007/s12094-013-1130-1
39. Bulzico D, Torres DC, Ferreira GM, Pires BRB, de Faria PAS, Hassan R, et al. A novel TP53 mutation associated with TWIST1 and SIP1 expression in an aggressive adrenocortical carcinoma. Endocr Pathol (2017) 28(4):326–31. doi: 10.1007/s12022-017-9482-7
40. Liang X, Wang Y, Shi H, Dong M, Han H, Li Q. Nucleolin-targeting AS1411 aptamer-modified micelle for the Co-delivery of doxorubicin and miR-519c to improve the therapeutic efficacy in hepatocellular carcinoma treatment. Int J Nanomedicine. (2021) 16:2569–84. doi: 10.2147/IJN.S304526
41. Cheng G, Li Y, Liu Z, Song X. lncRNA PSMA3-AS1 promotes the progression of non-small cell lung cancer through targeting miR-17-5p/PD-L1. Adv Clin Exp Med (2021) 30(10):1043–50. doi: 10.17219/acem/138624
42. Zhen Q, Zhang Y, Gao L, Wang R, Chu W, Zhao X, et al. MiR-519d-3p enhances the sensitivity of non-small-cell lung cancer to tyrosine kinase inhibitors. Mamm Genome. (2021) 32(6):508–16. doi: 10.1007/s00335-021-09919-8
Keywords: BRD4, adrenocortical carcinoma, target prediction, gene regulation network, PFI-1, BRD3, BRD2
Citation: Situ Y, Liang Q, Zeng Z, Chen J, Shao Z, Xu Q, Lu X, Cui Y, Zhang J, Lu L and Deng L (2023) Systematic analysis of the BET family in adrenocortical carcinoma: The expression, prognosis, gene regulation network, and regulation targets. Front. Endocrinol. 14:1089531. doi: 10.3389/fendo.2023.1089531
Received: 04 November 2022; Accepted: 19 January 2023;
Published: 30 January 2023.
Edited by:
Anna Perri, Magna Græcia University of Catanzaro, ItalyReviewed by:
Vincenzo Pezzi, University of Calabria, ItalyAmit Kumar Singh, National Cancer Institute (NIH), United States
Copyright © 2023 Situ, Liang, Zeng, Chen, Shao, Xu, Lu, Cui, Zhang, Lu and Deng. This is an open-access article distributed under the terms of the Creative Commons Attribution License (CC BY). The use, distribution or reproduction in other forums is permitted, provided the original author(s) and the copyright owner(s) are credited and that the original publication in this journal is cited, in accordance with accepted academic practice. No use, distribution or reproduction is permitted which does not comply with these terms.
*Correspondence: Yongli Situ, styl1987@126.com; Li Deng, dengli@gdmu.edu.cn