- 1Center for Cohort Studies, Graduate School of Medical Sciences, Kyushu University, Fukuoka, Japan
- 2Department of Medicine and Clinical Science, Graduate School of Medical Sciences, Kyushu University, Fukuoka, Japan
- 3Department of Nephrology, Fukuoka Red Cross Hospital, Fukuoka, Japan
Fibroblast growth factor (FGF)23 is a bone-derived phosphotropic hormone that regulates phosphate and mineral homeostasis. Recent studies have provided evidence that a high plasma concentration of FGF23 is associated with cardiac disease, including left ventricular hypertrophy (LVH), heart failure, atrial fibrillation, and cardiac death. Experimental studies have shown that FGF23 activates fibroblast growth factor receptor 4 (FGFR4)/phospholipase Cγ/calcineurin/nuclear factor of activated T-cells signaling in cardiomyocytes and induces cardiac hypertrophy in rodents. Activation of FGFR4 by FGF23 normally requires the co-receptor α-klotho, and klotho-independent signaling occurs only under conditions characterized by extremely high FGF23 concentrations. Recent studies have demonstrated that FGF23 activates the renin-angiotensin-aldosterone system (RAAS) and induces LVH, at least in part as a result of lower vitamin D activation. Moreover, crosstalk between FGF23 and RAAS results in the induction of cardiac hypertrophy and fibrosis. In this review, we summarize the results of studies regarding the relationships between FGF23 and cardiac events, and describe the potential direct and indirect mechanisms whereby FGF23 induces LVH.
1 Structure and function of fibroblast growth factor 23
Fibroblast growth factors (FGFs) are polypeptide growth factors with a broad range of biological functions, including the regulation of embryonic development, organogenesis, metabolism, angiogenesis, mitogenesis, and cellular differentiation (1, 2). The FGF family comprises 22 members, and FGF 23 belongs to the FGF 19 subfamily, and because this a circulating hormone, it is termed endocrine FGF (3). FGF23 is a 251-amino acid protein and is principally synthesized by osteoblasts and osteocytes. The classical target organs of FGF23 are the kidney and parathyroid glands, and FGF23 has its physiological effects through fibroblast growth factor receptors (FGFRs)1, 2, and 4, with α-klotho acting as a co-factor (4). The C-terminus of FGF23 contains the binding site for α-klotho and the N-terminus contains the binding site for FGFRs. The O-linked proprotein convertase cleavage site is stabilized through O-linked glycosylation by N-acetylgalactosaminyltransferase 3 (GALNT3), which protects FGF23 against proteolytic cleavage (5). FGF23 acts on the kidney via FGFR1c/α-klotho-mediated signaling to regulate phosphate excretion and vitamin D metabolism. Dietary phosphate intake stimulates the production and secretion of FGF23 by osteocytes, and FGF23 reduces phosphate reabsorption by reducing the expression of the sodium/phosphate co-transporters NaPi-2a and NaPi-2c in the proximal tubules of the kidney (6). In addition, FGF23 reduces the synthesis of active vitamin D by downregulating 1α-hydroxylase (CYP27B1) and upregulating 24-hydroxylase (CYP27B1) (7). FGF23 also inhibits the secretion of parathyroid hormone (PTH) by the parathyroid gland (8). It is normally secreted by osteocytes, but under pathological conditions, FGF23 can be secreted by the heart (9–14), liver (15), kidney (16), macrophages (17), or bone marrow (18).
2 FGF23 and cardiac events
Cardiovascular disease is the leading cause of mortality worldwide and is highly prevalent in the general population (19). In 2008, the circulating FGF23 concentrations of patients undergoing hemodialysis were reported to be associated with mortality for the first time (20). Since then, a large number of clinical studies have shown that high plasma concentrations of FGF23 are associated with left ventricular hypertrophy (LVH), heart failure, and mortality in the general population, and especially in patients with chronic kidney disease (CKD). In the present review, we summarize the published clinical evidence regarding the relationships between FGF23 and cardiac events and then discuss the effects of FGF23 on the heart.
2.1 FGF23 and LVH
Several previous studies have shown an association between high circulating concentrations of FGF23 and a high risk of LVH in the general population (21–24) and patients (25–32). In addition, there is a particularly strong association in patients with CKD (25, 26, 28, 30, 32). High circulating FGF23 concentrations are associated with concentric hypertrophy rather than eccentric hypertrophy (21, 30). These findings suggest that FGF23 might increase ventricular wall thickness. Finally, a recent clinical study showed that etelcalcetide reduces the circulating concentration of FGF23 and inhibits the progression of LVH (33). In this study, the concentration of FGF23, but not the levels of renin-angiotensin-aldosterone system (RAAS)-related parameters, showed a clear association with left ventricular mass index (34).
2.2 FGF23 and heart failure
Previous observational studies have demonstrated that the circulating concentration of FGF23 is related to heart failure (HF) (22, 29, 35–45), and this association appears to be stronger in patients with CKD (36, 37, 44, 46), but is not affected by adjustment for kidney function (22, 35, 37, 39, 41–44). Some previous studies have shown significant relationships between high circulating FGF23 concentration and low ejection fraction (27, 28, 45, 47–49). In addition, high FGF23 concentration was shown to be associated with new-onset heart failure in a cohort study of members of the general population (43). FGF23 may induce HF through FGF23-associated LVH, and Andersen et al. reported that the circulating concentrations of FGF23 in patients with HF are significantly higher than those in healthy individuals (50). In this study, the expression of FGF23 in the ventricles of patients with HF did not exceed those of heathy controls (50), but several other studies have shown higher expression of FGF23 in the myocardia of patients with LVH and in rodent models of LVH (11–14). Genetically high FGF23 concentrations have also been shown to be associated with a higher risk of heart failure in a biobank cohort (51). Thus, the circulating concentration of FGF23 and its myocardial expression may be associated with HF.
2.3 FGF23 and atrial fibrillation
Numerous studies have shown a relationship between FGF23 concentration and atrial fibrillation (AF) (23, 39, 47, 52–55). The Multi-Ethnic Study of Atherosclerosis (MESA) and Cardiovascular Health Study (CHS) revealed that high serum concentrations of FGF23 are associated with the incidence of AF, even after adjustment for estimated glomerular filtration rate and other cardiovascular risk factors (52, 53). In contrast, the Atherosclerosis Risk in Communities (ARIC) study showed that the baseline serum FGF23 concentration is not associated with the risk of AF after adjustment for potential confounders (52). However, a meta-analysis showed that high concentrations of FGF23 are associated with a higher risk of AF (52). Recently, Graves et al. demonstrated that FGF23 prolongs the QTc interval and induces ventricular arrhythmias via the FGFR4 pathway in mice (56). It is thought that FGF23 induces LVH, leading to cardiac remodeling, which may explain the arrhythmogenesis.
2.4 FGF23 and myocardial infarction
Some previous studies have demonstrated that FGF23 concentration is associated with the incidence of myocardial infarction (MI) (22, 37), whereas others have shown no association (35, 57, 58). Thus, this remains an area of controversy, but the principal effect of FGF23 on the heart is likely to be the induction of LVH, which may exacerbate HF and AF.
2.5 FGF23 and cardiovascular mortality
Several previous studies have shown an association between high serum concentrations of FGF23 and cardiovascular mortality (37, 45, 59–64). Furthermore, the circulating concentrations of FGF23 are significantly higher in non-surviving patients with myocardial infarction and heart failure (65). A linear dose-response relationship between FGF23 concentration and cardiovascular mortality for concentrations of FGF23 of >50 pg/mL has been demonstrated (37, 59). FGF23 concentrations are stable over time in the majority of patients with CKD; however, individuals with rising FGF23 concentrations were shown to be at a higher risk of death than those with stable FGF23 concentrations (66). These findings imply that high FGF23 concentration is associated with cardiovascular mortality owing to HF and AF.
3 Mechanisms of FGF23-induced LVH
Numerous studies have demonstrated that high FGF23 concentrations are associated with LVH in humans (21–25, 27–30). In 2011, Faul et al. demonstrated experimentally that the intramyocardial injection of FGF23 in mice induces LVH (26). Cardiomyocytes express FGFR4, but α-klotho is not expressed in the heart. α-klotho increases the binding affinity of FGFR to FGF23 by ~20-fold (67); therefore, the binding affinity of FGF23 for FGFR4 in the absence of α-klotho is weaker than in its presence. Thus, both direct and indirect mechanisms of the effect of FGF23 on the heart must be discussed to fully understand how FGF23 influences the progression of LVH.
3.1 Mechanism for the direct effect of FGF23 on LVH
Faul and colleagues have demonstrated that the injection of recombinant FGF23 induces LVH in an FGFR-dependent, but α-klotho-independent, manner (26). They and other researchers have shown that FGF23 increases the expression of pro-hypertrophic genes in cardiomyocytes (12, 26, 68), regulates calcium homeostasis in cardiomyocytes (69), increases intracellular calcium concentration, and promotes the contractility of cardiomyocytes (70) (Table 1). FGF23 activates FGFR4/phospholipase Cγ/calcineurin/nuclear factor of activated T-cells (NFAT) signaling in cardiomyocytes and induces cardiac hypertrophy in rodents (71). In addition, Han et al. have shown that the cardiac-specific deletion of FGFR4 attenuates FGF23-induced LVH in mice (74). These results are consistent with FGF23 directly stimulating cardiomyocytes via FGFR4 to induce LVH. Klotho-independent signaling is only activated in the presence of a high FGF23 concentration (67). The upregulation of intracardiac FGF23 expression using an adeno-associated virus (AAV) was found not to induce LVH in healthy mice (75). In addition, high-phosphate diet-induced LVH in mice, which is mediated through high serum FGF23 concentrations, was found to be reversed by the normalization of the serum FGF23 concentration (72). Therefore, this direct effect of FGF23 on the heart is likely to occur only under pathological conditions, such as in a CKD-related milieu.
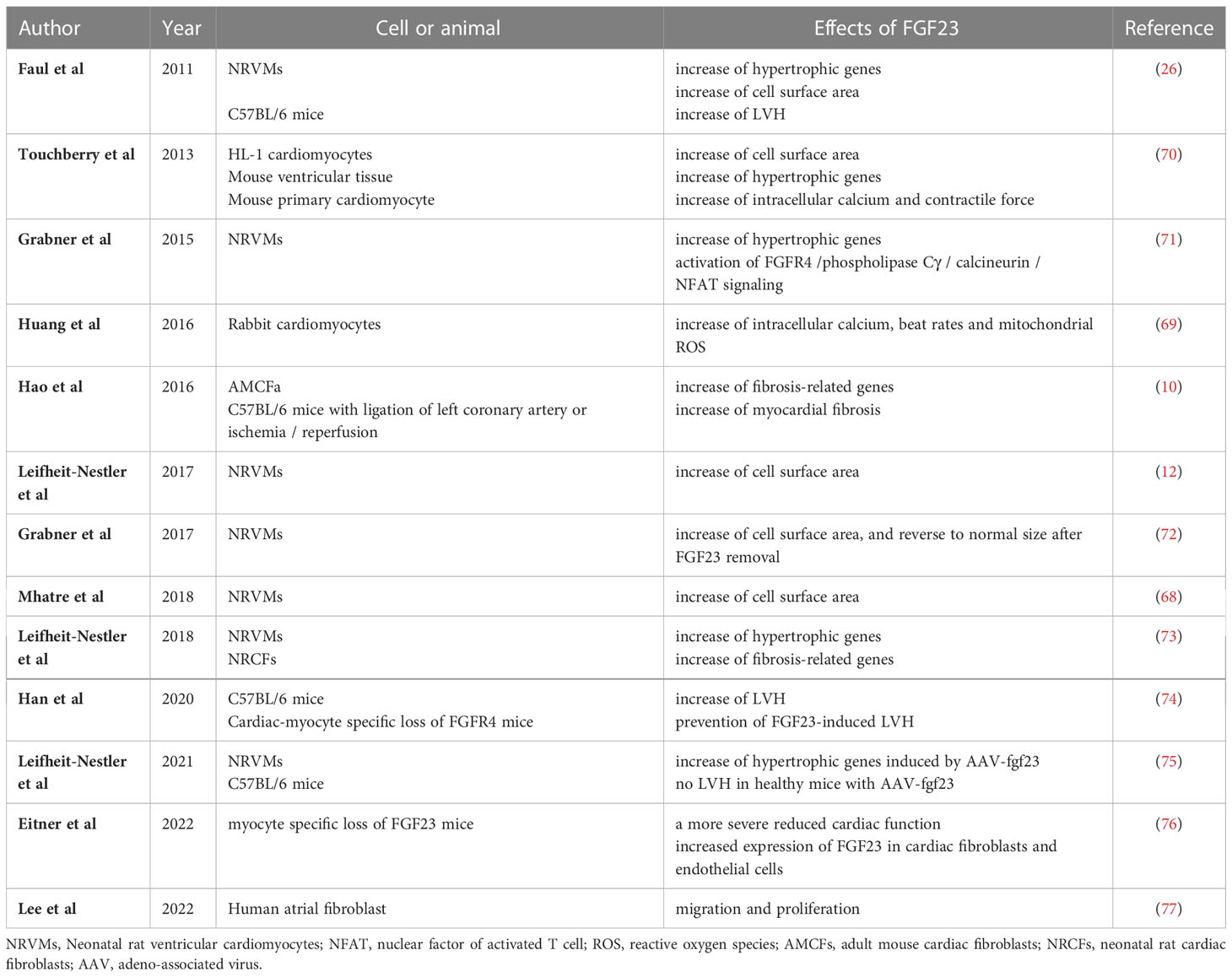
Table 1 Summary of the results of experimental studies regarding the direct effects of FGF23 on LVH and myocardial fibrosis.
Several previous studies have demonstrated that the expression of FGF23 in cardiomyocytes is high under pathological conditions. The expression of FGF23 and FGFR4 in the heart has been shown to be associated with LVH using autopsy samples collected from patients with CKD (11), and the expression of FGF23 is high in the heart following MI (9). Transverse aortic constriction (TAC)-induced LVH causes an increase in the expression of FGF23 in the heart (10, 13, 14). In addition, rats that undergo nephrectomy express FGF23 in their hearts (12). Inflammation regulates the expression of FGF23 through HIF1α stabilization in osteocytes (78). Finally, the uremic toxin indoxyl sulfate induces cardiac hypertrophy through the FGF23-FGFR4 signaling pathway (79). These results suggest that inflammation caused by HF or uremic toxins may induce the expression of FGF23 in cardiomyocytes (Figure 1).
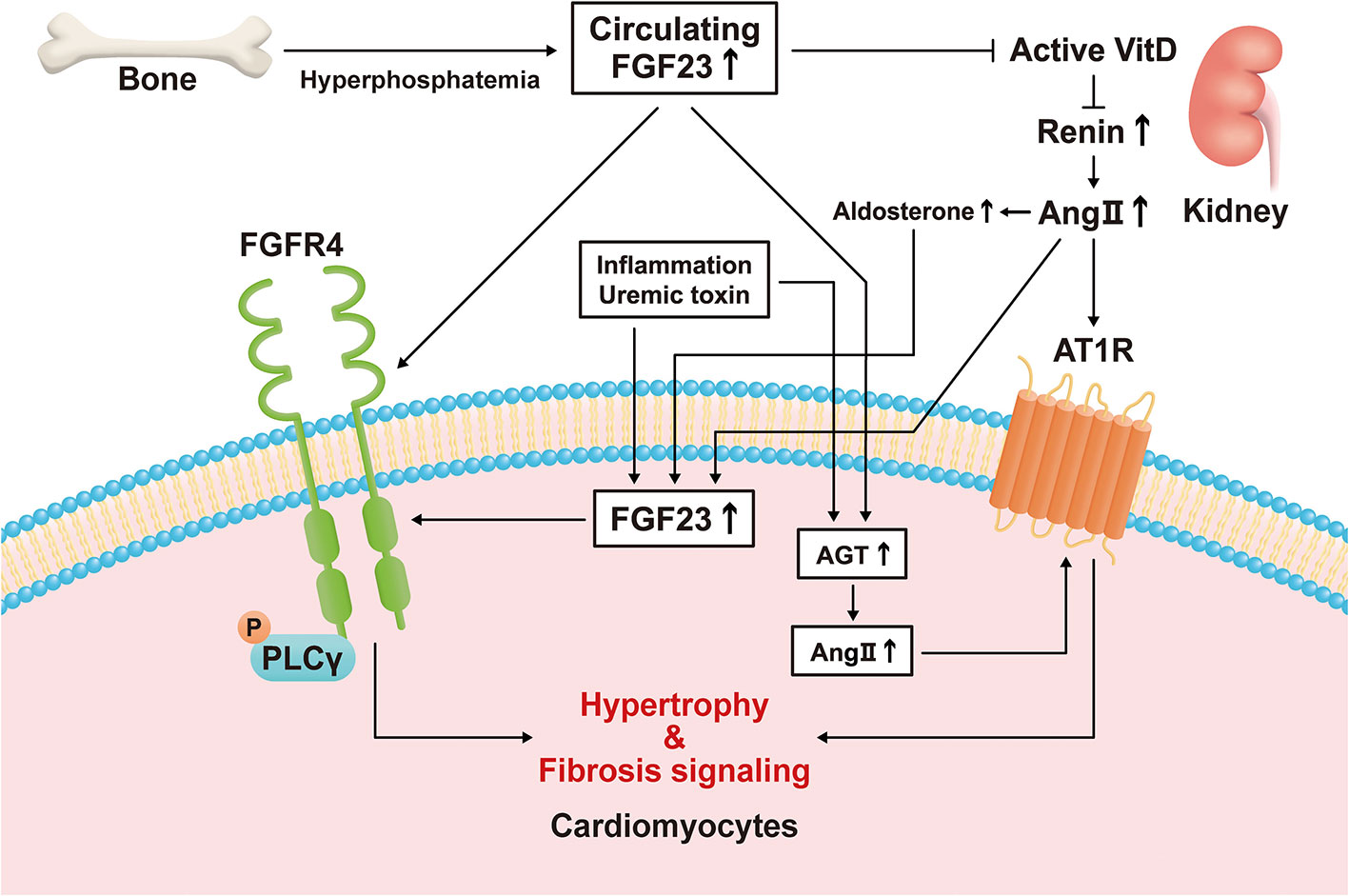
Figure 1 Direct and indirect mechanisms mediate the effects of fibroblast growth factor (FGF)23 on left ventricular hypertrophy. Hyperphosphatemia induces an increase in circulating FGF23 concentration by increasing its secretion by bone. FGF23 stimulates hypertrophic signaling via fibroblast growth factor receptor (FGFR)4 in cardiomyocytes. FGF23 also suppresses active vitamin D (VitD) synthesis in the kidney, and the activation of VitD is lower in a chronic kidney disease (CKD)-related milieu. Active VitD inhibits renin activity in the kidney and heart and increases serum angiotensin II (Ang II) concentration and its cardiac expression. Ang II binds to angiotensin II receptor type 1 (AT1R) in cardiomyocytes, causing cardiac hypertrophy and fibrosis. Inflammatory cytokines, a uremic milieu, Ang II, and aldosterone induce FGF23 transcription in cardiomyocytes. Circulating FGF23 also causes an increase in local angiotensinogen and Ang II expression in cardiomyocytes, leading to hypertrophy and fibrosis.
Previous studies have also shown that FGFR4 mediates LVH (71, 72, 74). FGFR4 knockout mice attenuated the progression of LVH which was induced by high phosphate diet, FGF23, and aging (71, 72, 74). The expression of FGFR4 in the heart changes under pathological conditions. Hao et al. showed that the expression of FGFR4 increases in the heart after ischemic reperfusion (10), and cardiac FGFR4 expression was shown to be markedly upregulated in the hearts of patients with LVH (11). Finally, cardiac overexpression of FGF23 using an adeno-associated virus (AAV) in mice was found to increase the expression of FGFR4 (75). These findings imply that the level of FGFR4 expression in the heart may contribute to the progression of LVH.
FGF23 has also been reported to induce myocardial fibrosis. Hao et al. demonstrated that it is expressed in cardiac fibroblasts and its overexpression in the heart induces cardiac fibrosis through the activation of β-catenin and TGF-β in mice (10). FGF23 induces pro-fibrotic signaling, involving TGFβ/Smad complexes, in cardiac fibroblasts (73, 80). In addition, FGFR1 has been reported to contribute to the FGF23-induced proliferation and migration of cardiac fibroblasts (77). Recently, Eitner et al. demonstrated that the myocyte-specific deletion of FGF23 mice with TAC-induced LVH impairs cardiac function and is associated with higher expression of FGF23 in fibroblasts and endothelial cells (76). These findings suggest that cardiac myocyte-derived FGF23 is needed to maintain cardiac function and that cardiac fibroblasts and endothelial cells might represent important sources of FGF23 for the progression of LVH under pathological conditions. Together, these results are consistent with the hypothesis that FGF23/FGFR induces fibrotic signaling in cardiac fibroblasts.
Previous studies have shown that FGF23 has effects on other types of cardiovascular cells. High serum FGF23 concentrations are associated with endothelial dysfunction in patients with CKD (81), and FGF23 has been shown to cause the release of nitric oxide (NO) and the formation of reactive oxygen species (ROS) in human coronary artery endothelial cells (82). Pro-inflammatory M1 macrophages express FGF23 (17), and FGF23 has been shown to increase the production of the pro-inflammatory cytokine TNF-α by M0 macrophages and to reduce arginase-1 expression in M2 macrophages (17). Thus, FGF23 can be expressed in many cell types, including myocytes, fibroblasts, endothelial cells, and cardiac macrophages under pathological conditions, and the paracrine effects of FGF23 secreted by these cells, in addition to the effects of circulating FGF23, may influence pathological cardiac remodeling (83).
3.2 Mechanism of the indirect effect of FGF23 on LVH
There are several hypotheses regarding how an indirect mechanism might mediate the effect of FGF23 on LVH (Figure 1 and Table 2). Slavic et al. reported that TAC increases the circulating FGF23 concentration and the cardiac expression of FGF23 in mice (13). Okamoto et al. also demonstrated that the LVH induced by TAC is associated with high cardiac FGF23 expression and RAAS activation (88). However, genetic ablation of Fgf23 does not affect TAC-induced LVH and spironolactone inhibits LVH following TAC (13). Leifheit-Nestler et al. showed that both angiotensin II and aldosterone induce FGF23 expression in cardiomyocytes (73), and Mhatre et al. showed that both FGF23 and angiotensin II stimulate an increase in cytoplasmic Ca2+ in cardiomyocytes and induce LVH (68). Finally, Böckmann et al. revealed that FGF23 induces the expression of angiotensinogen gene in cardiomyocytes and angiotensin-converting enzyme in cardiac fibroblasts, activates the cardiac RAAS, and promotes LVH (85). Thus, the RAAS plays an important role in the development of LVH, and FGF23 is associated with cardiac RAAS activation in LVH.
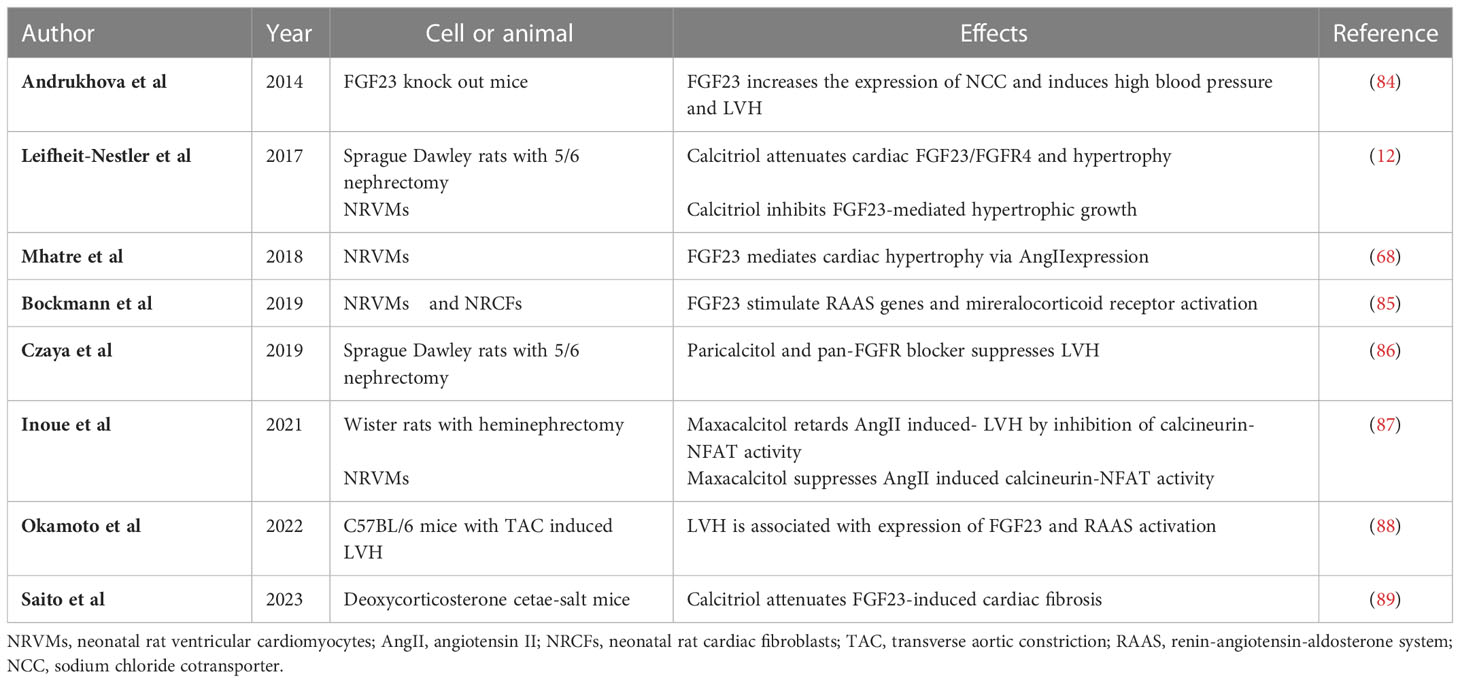
Table 2 Summary of the results of experimental studies regarding the indirect effects of FGF23 on LVH and myocardial fibrosis.
Active vitamin D inhibits RAAS-associated gene expression and reduces cardiac fibrosis (90–92). It also increases the serum FGF23 concentration, but inhibits FGF23-FGFR4 signaling in the heart and reduces LVH (12). Active vitamin D has been shown to retard the progression of LVH by inhibiting calcineurin/NFAT activity (87). In addition, active vitamin D and a pan-FGFR blocker have additive effects to further slow LVH (86). Recently, Saito et al. have shown that active vitamin D attenuates FGF23-induced cardiac fibrosis and improves diastolic function by inhibiting TGF-β signaling in deoxycorticosterone acetate and salt-treated mice (89). Finally, FGF23 reduces the synthesis of active vitamin D in the kidney (7). Thus, the downregulation of active vitamin D secondary to a high serum FGF23 concentration may contribute to the progression of LVH.
One previous study showed an effect of FGF23 on the sodium chloride co-transporter NCC. Specifically, FGF23 directly increased the expression of NCC in the distal renal tubules and sodium reabsorption in mice (84). This result suggests that FGF23 might induce volume expansion and high blood pressure through the upregulation of NCC, thereby contributing to LVH.
4 Conclusion
FGF23 contributes to the progression of LVH through direct and indirect mechanisms. However, the progression of LVH is also affected by active vitamin D, the RAAS, blood pressure, and other factors. In a CKD-related milieu in particular, high expression of FGF23 in osteocytes and cardiomyocytes may contribute to LVH progression via FGFR4 and angiotensin II receptor type 1 signaling.
Author contributions
TN contributed to provide the concept of review and drafting the manuscript. HK contributed to critical revision of the manuscript. MT contributed to provide the concept of review and contributed to critical revision of the manuscript. All authors contributed to the article and approved the submitted version.
Funding
This work was supported by JSPS KAKENHI Grant Number 21K08232. All the authors have declared no competing interest.
Acknowledgments
We thank Mark Cleasby, PhD from Edanz (https://jp.edanz.com/ac) for editing a draft of this manuscript.
Conflict of interest
The authors declare that the research was conducted in the absence of any commercial or financial relationships that could be construed as a potential conflict of interest.
Publisher’s note
All claims expressed in this article are solely those of the authors and do not necessarily represent those of their affiliated organizations, or those of the publisher, the editors and the reviewers. Any product that may be evaluated in this article, or claim that may be made by its manufacturer, is not guaranteed or endorsed by the publisher.
References
2. Martin A, David V, Quarles LD. Regulation and function of the FGF23/klotho endocrine pathways. Physiol Rev (2012) 92(1):131–55. doi: 10.1152/physrev.00002.2011
3. Hu MC, Shiizaki K, Kuro-o M, Moe OW. Fibroblast growth factor 23 and klotho: Physiology and pathophysiology of an endocrine network of mineral metabolism. Annu Rev Physiol (2013) 75:503–33. doi: 10.1146/annurev-physiol-030212-183727
4. Urakawa I, Yamazaki Y, Shimada T, Iijima K, Hasegawa H, Okawa K, et al. Klotho converts canonical FGF receptor into a specific receptor for FGF23. Nature (2006) 444(7120):770–4. doi: 10.1038/nature05315
5. Tagliabracci VS, Engel JL, Wiley SE, Xiao J, Gonzalez DJ, Nidumanda Appaiah H, et al. Dynamic regulation of FGF23 by Fam20C phosphorylation, GalNAc-T3 glycosylation, and furin proteolysis. Proc Natl Acad Sci U.S.A. (2014) 111(15):5520–5. doi: 10.1073/pnas.1402218111
6. Shimada T, Kakitani M, Yamazaki Y, Hasegawa H, Takeuchi Y, Fujita T, et al. Targeted ablation of Fgf23 demonstrates an essential physiological role of FGF23 in phosphate and vitamin d metabolism. J Clin Invest (2004) 113(4):561–8. doi: 10.1172/JCI200419081
7. Shimada T, Hasegawa H, Yamazaki Y, Muto T, Hino R, Takeuchi Y, et al. FGF-23 is a potent regulator of vitamin d metabolism and phosphate homeostasis. J Bone Miner Res (2004) 19(3):429–35. doi: 10.1359/JBMR.0301264
8. Ben-Dov IZ, Galitzer H, Lavi-Moshayoff V, Goetz R, Kuro-o M, Mohammadi M, et al. The parathyroid is a target organ for FGF23 in rats. J Clin Invest (2007) 117(12):4003–8. doi: 10.1172/JCI32409
9. Andrukhova O, Slavic S, Odorfer KI, Erben RG. Experimental myocardial infarction upregulates circulating fibroblast growth factor-23. J Bone Miner Res (2015) 30(10):1831–9. doi: 10.1002/jbmr.2527
10. Hao H, Li X, Li Q, Lin H, Chen Z, Xie J, et al. FGF23 promotes myocardial fibrosis in mice through activation of beta-catenin. Oncotarget (2016) 7(40):64649–64. doi: 10.18632/oncotarget.11623
11. Leifheit-Nestler M, Grosse Siemer R, Flasbart K, Richter B, Kirchhoff F, Ziegler WH, et al. Induction of cardiac FGF23/FGFR4 expression is associated with left ventricular hypertrophy in patients with chronic kidney disease. Nephrol Dial Transplant (2016) 31(7):1088–99. doi: 10.1093/ndt/gfv421
12. Leifheit-Nestler M, Grabner A, Hermann L, Richter B, Schmitz K, Fischer DC, et al. Vitamin d treatment attenuates cardiac FGF23/FGFR4 signaling and hypertrophy in uremic rats. Nephrol Dial Transplant (2017) 32(9):1493–503. doi: 10.1093/ndt/gfw454
13. Slavic S, Ford K, Modert M, Becirovic A, Handschuh S, Baierl A, et al. Genetic ablation of Fgf23 or klotho does not modulate experimental heart hypertrophy induced by pressure overload. Sci Rep (2017) 7(1):11298. doi: 10.1038/s41598-017-10140-4
14. Matsui I, Oka T, Kusunoki Y, Mori D, Hashimoto N, Matsumoto A, et al. Cardiac hypertrophy elevates serum levels of fibroblast growth factor 23. Kidney Int (2018) 94(1):60–71. doi: 10.1016/j.kint.2018.02.018
15. Bienaime F, Ambolet A, Aussilhou B, Brazier F, Fouchard M, Viau A, et al. Hepatic production of fibroblast growth factor 23 in autosomal dominant polycystic kidney disease. J Clin Endocrinol Metab (2018) 103(6):2319–28. doi: 10.1210/jc.2018-00123
16. Sugiura H, Matsushita A, Futaya M, Teraoka A, Akiyama KI, Usui N, et al. Fibroblast growth factor 23 is upregulated in the kidney in a chronic kidney disease rat model. PLoS One (2018) 13(3):e0191706. doi: 10.1371/journal.pone.0191706
17. Han X, Li L, Yang J, King G, Xiao Z, Quarles LD. Counter-regulatory paracrine actions of FGF-23 and 1,25(OH)2 d in macrophages. FEBS Lett (2016) 590(1):53–67. doi: 10.1002/1873-3468.12040
18. Toro L, Barrientos V, Leon P, Rojas M, Gonzalez M, Gonzalez-Ibanez A, et al. Erythropoietin induces bone marrow and plasma fibroblast growth factor 23 during acute kidney injury. Kidney Int (2018) 93(5):1131–41. doi: 10.1016/j.kint.2017.11.018
19. Roger VL, Go AS, Lloyd-Jones DM, Benjamin EJ, Berry JD, Borden WB, et al. Heart disease and stroke statistics–2012 update: A report from the American heart association. Circulation (2012) 125(1):e2–e220.
20. Gutierrez OM, Mannstadt M, Isakova T, Rauh-Hain JA, Tamez H, Shah A, et al. Fibroblast growth factor 23 and mortality among patients undergoing hemodialysis. N Eng J Med (2008) 359(6):584–92. doi: 10.1056/NEJMoa0706130
21. Mirza MA, Larsson A, Melhus H, Lind L, Larsson TE. Serum intact FGF23 associate with left ventricular mass, hypertrophy and geometry in an elderly population. Atherosclerosis (2009) 207(2):546–51. doi: 10.1016/j.atherosclerosis.2009.05.013
22. Kestenbaum B, Sachs MC, Hoofnagle AN, Siscovick DS, Ix JH, Robinson-Cohen C, et al. Fibroblast growth factor-23 and cardiovascular disease in the general population: The multi-ethnic study of atherosclerosis. Circ Heart Fail (2014) 7(3):409–17. doi: 10.1161/CIRCHEARTFAILURE.113.000952
23. Masson S, Agabiti N, Vago T, Miceli M, Mayer F, Letizia T, et al. The fibroblast growth factor-23 and vitamin d emerge as nontraditional risk factors and may affect cardiovascular risk. J Intern Med (2015) 277(3):318–30. doi: 10.1111/joim.12232
24. Patel RB, Ning H, de Boer IH, Kestenbaum B, Lima JAC, Mehta R, et al. Fibroblast growth factor 23 and long-term cardiac function: The multi-ethnic study of atherosclerosis. circ cardiovasc imaging. Circ Cardiovasc Imaging (2020) 13(11):e011925. doi: 10.1161/CIRCIMAGING.120.011925
25. Gutierrez OM, Januzzi JL, Isakova T, Laliberte K, Smith K, Collerone G, et al. Fibroblast growth factor 23 and left ventricular hypertrophy in chronic kidney disease. Circulation (2009) 119(19):2545–52. doi: 10.1161/CIRCULATIONAHA.108.844506
26. Faul C, Amaral AP, Oskouei B, Hu MC, Sloan A, Isakova T, et al. FGF23 induces left ventricular hypertrophy. J Clin Invest (2011) 121(11):4393–408. doi: 10.1172/JCI46122
27. Shibata K, Fujita S, Morita H, Okamoto Y, Sohmiya K, Hoshiga M, et al. Association between circulating fibroblast growth factor 23, alpha-klotho, and the left ventricular ejection fraction and left ventricular mass in cardiology inpatients. PLoS One (2013) 8(9):e73184.
28. Agarwal I, Ide N, Ix JH, Kestenbaum B, Lanske B, Schiller NB, et al. Fibroblast growth factor-23 and cardiac structure and function. J Am Heart Assoc (2014) 3(1):e000584. doi: 10.1161/JAHA.113.000584
29. Cheng N, He Y, Dang A, Lv N, Wang X, Li H. Association between plasma fibroblast growth factor 23 and left ventricular mass index in patients with takayasu arteritis. Clin Rheumatol (2020) 39(5):1591–9. doi: 10.1007/s10067-019-04895-6
30. Silva AP, Mendes F, Carias E, Goncalves RB, Fragoso A, Dias C, et al. Plasmatic klotho and FGF23 levels as biomarkers of CKD-associated cardiac disease in type 2 diabetic patients. Int J Mol Sci (2019) 20(7):1536. doi: 10.3390/ijms20071536
31. Akhabue E, Wong M, Mehta R, Isakova T, Wolf M, Yancy C, et al. Fibroblast growth factor-23 and subclinical markers of cardiac dysfunction: The coronary artery risk development in young adults (CARDIA) study. Am Heart J (2022) 245:10–8. doi: 10.1016/j.ahj.2021.11.009
32. Halim A, Burney HN, Li X, Li Y, Tomkins C, Siedlecki AM, et al. FGF23 and cardiovascular structure and function in advanced chronic kidney disease. Kidney (2022) 3(9):1529–41. doi: 10.34067/KID.0002192022
33. Dorr K, Kammer M, Reindl-Schwaighofer R, Lorenz M, Prikoszovich T, Marculescu R, et al. Randomized trial of etelcalcetide for cardiac hypertrophy in hemodialysis. Circ Res (2021) 128(11):1616–25. doi: 10.1161/CIRCRESAHA.120.318556
34. Dorr K, Kammer M, Reindl-Schwaighofer R, Lorenz M, Marculescu R, Poglitsch M, et al. The effect of FGF23 on cardiac hypertrophy is not mediated by systemic renin-angiotensin- aldosterone system in hemodialysis. Front Med (Lausanne) (2022) 9:878730. doi: 10.3389/fmed.2022.878730
35. Parker BD, Schurgers LJ, Brandenburg VM, Christenson RH, Vermeer C, Ketteler M, et al. The associations of fibroblast growth factor 23 and uncarboxylated matrix gla protein with mortality in coronary artery disease: The heart and soul study. Ann Intern Med (2010) 152(10):640–8. doi: 10.7326/0003-4819-152-10-201005180-00004
36. Ix JH, Katz R, Kestenbaum BR, de Boer IH, Chonchol M, Mukamal KJ, et al. Fibroblast growth factor-23 and death, heart failure, and cardiovascular events in community-living individuals: CHS (Cardiovascular health study). J Am Coll Cardiol (2012) 60(3):200–7. doi: 10.1016/j.jacc.2012.03.040
37. Lutsey PL, Alonso A, Selvin E, Pankow JS, Michos ED, Agarwal SK, et al. Fibroblast growth factor-23 and incident coronary heart disease, heart failure, and cardiovascular mortality: The atherosclerosis risk in communities study. J Am Heart Assoc (2014) 3(3):e000936. doi: 10.1161/JAHA.114.000936
38. di Giuseppe R, Buijsse B, Hirche F, Wirth J, Arregui M, Westphal S, et al. Plasma fibroblast growth factor 23, parathyroid hormone, 25-hydroxyvitamin D3, and risk of heart failure: A prospective, case-cohort study. J Clin Endocrinol Metab (2014) 99(3):947–55. doi: 10.1210/jc.2013-2963
39. Ter Maaten JM, Voors AA, Damman K, van der Meer P, Anker SD, Cleland JG, et al. Fibroblast growth factor 23 is related to profiles indicating volume overload, poor therapy optimization and prognosis in patients with new-onset and worsening heart failure. Int J Cardiol (2018) 253:84–90. doi: 10.1016/j.ijcard.2017.10.010
40. Ferreira JP, Verdonschot J, Collier T, Wang P, Pizard A, Bar C, et al. Proteomic bioprofiles and mechanistic pathways of progression to heart failure. Circ Heart Fail (2019) 12(5):e005897. doi: 10.1161/CIRCHEARTFAILURE.118.005897
41. Robinson-Cohen C, Shlipak M, Sarnak M, Katz R, Peralta C, Young B, et al. Impact of race on the association of mineral metabolism with heart failure: The multi-ethnic study of atherosclerosis. J Clin Endocrinol Metab (2020) 105(4):e1144–e1151. doi: 10.1210/clinem/dgz218
42. Paul S, Wong M, Akhabue E, Mehta RC, Kramer H, Isakova T, et al. Fibroblast growth factor 23 and incident cardiovascular disease and mortality in middle-aged adults. J Am Heart Assoc (2021) 10(16):e020196. doi: 10.1161/JAHA.120.020196
43. Binnenmars SH, Hoogslag GE, Yeung SMH, Brouwers FP, Bakker SJL, van Gilst WH, et al. Fibroblast growth factor 23 and risk of new onset heart failure with preserved or reduced ejection fraction: The PREVEND study. J Am Heart Assoc (2022) 11(15):e024952. doi: 10.1161/JAHA.121.024952
44. Mendonca L, Bigotte Vieira M, Neves JS. Association of combined fractional excretion of phosphate and FGF23 with heart failure and cardiovascular events in moderate and advanced renal disease. J Nephrol (2023) 36(1):55–67. doi: 10.1007/s40620-022-01358-1
45. Cornelissen A, Florescu R, Kneizeh K, Cornelissen C, Brandenburg V, Liehn E, et al. Intact fibroblast growth factor 23 levels and outcome prediction in patients with acute heart failure. Sci Rep (2021) 11(1):15507. doi: 10.1038/s41598-021-94780-7
46. Janus SE, Hajjari J, Chami T, Mously H, Badhwar AK, Karnib M, et al. Multi-variable biomarker approach in identifying incident heart failure in chronic kidney disease: results from the chronic renal insufficiency cohort study. Eur J Heart Fail (2022) 24(6):988–95. doi: 10.1002/ejhf.2543
47. Seiler S, Cremers B, Rebling NM, Hornof F, Jeken J, Kersting S, et al. The phosphatonin fibroblast growth factor 23 links calcium-phosphate metabolism with left-ventricular dysfunction and atrial fibrillation. Eur Heart J (2011) 32(21):2688–96. doi: 10.1093/eurheartj/ehr215
48. Poelzl G, Trenkler C, Kliebhan J, Wuertinger P, Seger C, Kaser S, et al. FGF23 is associated with disease severity and prognosis in chronic heart failure. Eur J Clin Invest (2014) 44(12):1150–8. doi: 10.1111/eci.12349
49. Song T, Fu Y, Wang Y, Li W, Zhao J, Wang X, et al. FGF-23 correlates with endocrine and metabolism dysregulation, worse cardiac and renal function, inflammation level, stenosis degree, and independently predicts in-stent restenosis risk in coronary heart disease patients underwent drug-eluting-stent PCI. BMC Cardiovasc Disord (2021) 21(1):24. doi: 10.1186/s12872-020-01839-w
50. Andersen IA, Huntley BK, Sandberg SS, Heublein DM, Burnett JC Jr. Elevation of circulating but not myocardial FGF23 in human acute decompensated heart failure. Nephrol Dial Transplant (2016) 31(5):767–72. doi: 10.1093/ndt/gfv398
51. Akwo E, Pike MM, Ertuglu LA, Vartanian N, Farber-Eger E, Lipworth L, et al. Association of genetically predicted fibroblast growth factor-23 with heart failure: A mendelian randomization study. Clin J Am Soc Nephrol (2022) 17(8):1183–93. doi: 10.2215/CJN.00960122
52. Alonso A, Misialek JR, Eckfeldt JH, Selvin E, Coresh J, Chen LY, et al. Circulating fibroblast growth factor-23 and the incidence of atrial fibrillation: The atherosclerosis risk in communities study. J Am Heart Assoc (2014) 3(5):e001082. doi: 10.1161/JAHA.114.001082
53. Mathew JS, Sachs MC, Katz R, Patton KK, Heckbert SR, Hoofnagle AN, et al. Fibroblast growth factor-23 and incident atrial fibrillation: The multi-ethnic study of atherosclerosis (MESA) and the cardiovascular health study (CHS). Circulation (2014) 130(4):298–307. doi: 10.1161/CIRCULATIONAHA.113.005499
54. Panwar B, Jenny NS, Howard VJ, Wadley VG, Muntner P, Kissela BM, et al. Fibroblast growth factor 23 and risk of incident stroke in community-living adults. Stroke (2015) 46(2):322–8. doi: 10.1161/STROKEAHA.114.007489
55. Chua W, Purmah Y, Cardoso VR, Gkoutos GV, Tull SP, Neculau G, et al. Data-driven discovery and validation of circulating blood-based biomarkers associated with prevalent atrial fibrillation. Eur Heart J (2019) 40(16):1268–76. doi: 10.1093/eurheartj/ehy815
56. Graves JM, Vallejo JA, Hamill CS, Wang D, Ahuja R, Patel S, et al. Fibroblast growth factor 23 (FGF23) induces ventricular arrhythmias and prolongs QTc interval in mice in an FGF receptor 4-dependent manner. Am J Physiol Heart Circ Physiol (2021) 320(6):H2283–H94. doi: 10.1152/ajpheart.00798.2020
57. Taylor EN, Rimm EB, Stampfer MJ, Curhan GC. Plasma fibroblast growth factor 23, parathyroid hormone, phosphorus, and risk of coronary heart disease. Am Heart J (2011) 161(5):956–62. doi: 10.1016/j.ahj.2011.02.012
58. Takahashi H, Ozeki M, Fujisaka T, Morita H, Fujita SI, Takeda Y, et al. Changes in serum fibroblast growth factor 23 in patients with acute myocardial infarction. Circ J (2018) 82(3):767–74. doi: 10.1253/circj.CJ-17-0826
59. Arnlov J, Carlsson AC, Sundstrom J, Ingelsson E, Larsson A, Lind L, et al. Higher fibroblast growth factor-23 increases the risk of all-cause and cardiovascular mortality in the community. Kidney Int (2013) 83(1):160–6. doi: 10.1038/ki.2012.327
60. Brandenburg VM, Kleber ME, Vervloet MG, Tomaschitz A, Pilz S, Stojakovic T, et al. Fibroblast growth factor 23 (FGF23) and mortality: The ludwigshafen risk and cardiovascular health study. Atherosclerosis (2014) 237(1):53–9. doi: 10.1016/j.atherosclerosis.2014.08.037
61. Haring R, Enserro D, Xanthakis V, Mitchell GF, Benjamin EJ, Hamburg NM, et al. Plasma fibroblast growth factor 23: Clinical correlates and association with cardiovascular disease and mortality in the framingham heart study. J Am Heart Assoc (2016) 5(7):e003486. doi: 10.1161/JAHA.116.003486
62. Souma N, Isakova T, Lipiszko D, Sacco RL, Elkind MS, DeRosa JT, et al. Fibroblast growth factor 23 and cause-specific mortality in the general population: The northern Manhattan study. J Clin Endocrinol Metab (2016) 101(10):3779–86. doi: 10.1210/jc.2016-2215
63. Sharma S, Katz R, Dubin RF, Drew DA, Gutierrez OM, Shlipak MG, et al. FGF23 and cause-specific mortality in community-living individuals-the health, aging, and body composition study. J Am Geriatr Soc (2021) 69(3):711–7. doi: 10.1111/jgs.16910
64. Westerberg PA, Tivesten A, Karlsson MK, Mellstrom D, Orwoll E, Ohlsson C, et al. Fibroblast growth factor 23, mineral metabolism and mortality among elderly men (Swedish MrOs). BMC Nephrol (2013) 14:85. doi: 10.1186/1471-2369-14-85
65. Cornelissen A, Florescu R, Kneizeh K, Cornelissen C, Liehn E, Brandenburg V, et al. Fibroblast growth factor 23 and outcome prediction in patients with acute myocardial infarction. J Clin Med (2022) 11(3):601. doi: 10.3390/jcm11030601
66. Isakova T, Cai X, Lee J, Xie D, Wang X, Mehta R, et al. Longitudinal FGF23 trajectories and mortality in patients with CKD. J Am Soc Nephrol (2018) 29(2):579–90. doi: 10.1681/ASN.2017070772
67. Goetz R, Ohnishi M, Kir S, Kurosu H, Wang L, Pastor J, et al. Conversion of a paracrine fibroblast growth factor into an endocrine fibroblast growth factor. J Biol Chem (2012) 287(34):29134–46. doi: 10.1074/jbc.M112.342980
68. Mhatre KN, Wakula P, Klein O, Bisping E, Volkl J, Pieske B, et al. Crosstalk between FGF23- and angiotensin II-mediated Ca(2+) signaling in pathological cardiac hypertrophy. Cell Mol Life Sci (2018) 75(23):4403–16. doi: 10.1007/s00018-018-2885-x
69. Huang SY, Chen YC, Kao YH, Hsieh MH, Lin YK, Chung CC, et al. Fibroblast growth factor 23 dysregulates late sodium current and calcium homeostasis with enhanced arrhythmogenesis in pulmonary vein cardiomyocytes. Oncotarget (2016) 7(43):69231–42. doi: 10.18632/oncotarget.12470
70. Touchberry CD, Green TM, Tchikrizov V, Mannix JE, Mao TF, Carney BW, et al. FGF23 is a novel regulator of intracellular calcium and cardiac contractility in addition to cardiac hypertrophy. Am J Physiol Endocrinol Metab (2013) 304(8):E863–73. doi: 10.1152/ajpendo.00596.2012
71. Grabner A, Amaral AP, Schramm K, Singh S, Sloan A, Yanucil C, et al. Activation of cardiac fibroblast growth factor receptor 4 causes left ventricular hypertrophy. Cell Metab (2015) 22(6):1020–32. doi: 10.1016/j.cmet.2015.09.002
72. Grabner A, Schramm K, Silswal N, Hendrix M, Yanucil C, Czaya B, et al. FGF23/FGFR4-mediated left ventricular hypertrophy is reversible. Sci Rep (2017) 7(1):1993. doi: 10.1038/s41598-017-02068-6
73. Leifheit-Nestler M, Kirchhoff F, Nespor J, Richter B, Soetje B, Klintschar M, et al. Fibroblast growth factor 23 is induced by an activated renin-angiotensin-aldosterone system in cardiac myocytes and promotes the pro-fibrotic crosstalk between cardiac myocytes and fibroblasts. Nephrol Dial Transplant (2018) 33(10):1722–34. doi: 10.1093/ndt/gfy006
74. Han X, Cai C, Xiao Z, Quarles LD. FGF23 induced left ventricular hypertrophy mediated by FGFR4 signaling in the myocardium is attenuated by soluble klotho in mice. J Mol Cell Cardiol (2020) 138:66–74. doi: 10.1016/j.yjmcc.2019.11.149
75. Leifheit-Nestler M, Wagner MA, Richter B, Piepert C, Eitner F, Bockmann I, et al. Cardiac fibroblast growth factor 23 excess does not induce left ventricular hypertrophy in healthy mice. Front Cell Dev Biol (2021) 9:745892. doi: 10.3389/fcell.2021.745892
76. Eitner F, Richter B, Schwanen S, Szaroszyk M, Vogt I, Grund A, et al. Comprehensive expression analysis of cardiac fibroblast growth factor 23 in health and pressure-induced cardiac hypertrophy. Front Cell Dev Biol (2021) 9:791479. doi: 10.3389/fcell.2021.791479
77. Lee TW, Chung CC, Lee TI, Lin YK, Kao YH, Chen YJ. Fibroblast growth factor 23 stimulates cardiac fibroblast activity through phospholipase c-mediated calcium signaling. Int J Mol Sci (2021) 23(1):166. doi: 10.3390/ijms23010166
78. David V, Martin A, Isakova T, Spaulding C, Qi L, Ramirez V, et al. Inflammation and functional iron deficiency regulate fibroblast growth factor 23 production. Kidney Int (2016) 89(1):135–46. doi: 10.1038/ki.2015.290
79. Kishimoto H, Nakano T, Torisu K, Tokumoto M, Uchida, Yamada S, et al. Indoxyl sulfate induces cardiomyocyte hypertrophy via FGF23-FGFR4 signaling pathway. Front Cardiovasc Med (2023), in press. doi: 10.3389/fcvm.2023.990422
80. Kuga K, Kusakari Y, Uesugi K, Semba K, Urashima T, Akaike T, et al. Fibrosis growth factor 23 is a promoting factor for cardiac fibrosis in the presence of transforming growth factor-beta1. PLoS One (2020) 15(4):e0231905.
81. Yilmaz MI, Sonmez A, Saglam M, Yaman H, Kilic S, Demirkaya E, et al. FGF-23 and vascular dysfunction in patients with stage 3 and 4 chronic kidney disease. Kidney Int (2010) 78(7):679–85. doi: 10.1038/ki.2010.194
82. Richter B, Haller J, Haffner D, Leifheit-Nestler M. Klotho modulates FGF23-mediated NO synthesis and oxidative stress in human coronary artery endothelial cells. Pflugers Arch (2016) 468(9):1621–35. doi: 10.1007/s00424-016-1858-x
83. Leifheit-Nestler M, Haffner D. Paracrine effects of FGF23 on the heart. Front Endocrinol (Lausanne) (2018) 9:278. doi: 10.3389/fendo.2018.00278
84. Andrukhova O, Slavic S, Smorodchenko A, Zeitz U, Shalhoub V, Lanske B, et al. FGF23 regulates renal sodium handling and blood pressure. EMBO Mol Med (2014) 6(6):744–59. doi: 10.1002/emmm.201303716
85. Bockmann I, Lischka J, Richter B, Deppe J, Rahn A, Fischer DC, et al. FGF23-mediated activation of local RAAS promotes cardiac hypertrophy and fibrosis. Int J Mol Sci (2019) 20(18):4634. doi: 10.3390/ijms20184634
86. Czaya B, Seeherunvong W, Singh S, Yanucil C, Ruiz P, Quiroz Y, et al. Cardioprotective effects of paricalcitol alone and in combination with FGF23 receptor inhibition in chronic renal failure: Experimental and clinical studies. Am J Hypertens (2019) 32(1):34–44. doi: 10.1093/ajh/hpy154
87. Inoue K, Matsui I, Hamano T, Okuda K, Tsukamoto Y, Matsumoto A, et al. Maxacalcitol (22-oxacalcitriol (OCT)) retards progression of left ventricular hypertrophy with renal dysfunction through inhibition of calcineurin-NFAT activity. Cardiovasc Drugs Ther (2021) 35(2):381–97. doi: 10.1007/s10557-020-07111-9
88. Okamoto K, Fujii H, Watanabe K, Goto S, Kono K, Nishi S. Changes of FGF23 and the renin-Angiotensin-System in Male mouse models of chronic kidney disease and cardiac hypertrophy. J Endocr Soc (2022) 6(2):bvab187. doi: 10.1210/jendso/bvab187
89. Saito T, Mizobuchi M, Kato T, Ogata H, Koiwa F, Honda H. Fibroblast growth factor 23 exacerbates cardiac fibrosis in deoxycorticosterone acetate-salt mice with hypertention. Lab Invest (2023) 103(1). doi: 10.1016/j.labinv.2022.100003
90. Li YC, Kong J, Wei M, Chen ZF, Liu SQ, Cao LP. 1,25-dihydroxyvitamin D(3) is a negative endocrine regulator of the renin-angiotensin system. J Clin Invest (2002) 110(2):229–38. doi: 10.1172/JCI0215219
91. Mizobuchi M, Nakamura H, Tokumoto M, Finch J, Morrissey J, Liapis H, et al. Myocardial effects of VDR activators in renal failure. J Steroid Biochem Mol Biol (2010) 121(1-2):188–92. doi: 10.1016/j.jsbmb.2010.03.026
Keywords: FGF23, Heart, Left ventricle hypertrophy, FGFR4, cardiac event
Citation: Nakano T, Kishimoto H and Tokumoto M (2023) Direct and indirect effects of fibroblast growth factor 23 on the heart. Front. Endocrinol. 14:1059179. doi: 10.3389/fendo.2023.1059179
Received: 01 October 2022; Accepted: 30 January 2023;
Published: 24 February 2023.
Edited by:
Noriko Ide, Harvard University, United StatesReviewed by:
Christian Faul, University of Alabama at Birmingham, United StatesHiroya Ohta, Tokushima Bunri University, Japan
Copyright © 2023 Nakano, Kishimoto and Tokumoto. This is an open-access article distributed under the terms of the Creative Commons Attribution License (CC BY). The use, distribution or reproduction in other forums is permitted, provided the original author(s) and the copyright owner(s) are credited and that the original publication in this journal is cited, in accordance with accepted academic practice. No use, distribution or reproduction is permitted which does not comply with these terms.
*Correspondence: Toshiaki Nakano, bmFrYW5vLnRvc2hpYWtpLjQ1NUBtLmt5dXNodS11LmFjLmpw