- 1Institute for Clinical Chemistry, Laboratory Medicine and Transfusion Medicine, General Hospital Nuremberg and Paracelsus Medical University, Nuremberg, Germany
- 2Instituto de Neurobiología, Universidad Nacional Autónoma de México (UNAM), Campus UNAM-Juriquilla, Querétaro, Mexico
Diabetic retinopathy (DR) and diabetic macular edema (DME) are major causes for visual loss in adults. Nearly half of the world’s population with diabetes has some degree of DR, and DME is a major cause of visual impairment in these patients. Severe vision loss occurs because of tractional retinal detachment due to retinal neovascularization, but the most common cause of moderate vision loss occurs in DME where excessive vascular permeability leads to the exudation and accumulation of extracellular fluid and proteins in the macula. Metabolic control stands as an effective mean for controlling retinal vascular alterations in some but not all patients with diabetes, and the search of other modifiable factors affecting the risk for diabetic microvascular complications is warranted. Prolactin (PRL) and its proteolytic fragment, vasoinhibin, have emerged as endogenous regulators of retinal blood vessels. PRL acquires antiangiogenic and anti-vasopermeability properties after undergoing proteolytic cleavage to vasoinhibin, which helps restrict the vascularization of ocular organs and, upon disruption, promotes retinal vascular alterations characteristic of DR and DME. Evidence is linking PRL (and other pituitary hormones) and vasoinhibin to DR and recent preclinical and clinical evidence supports their translation into novel therapeutic approaches.
Diabetic Retinopathy is a common cause of vision loss and blindness
Most patients with longstanding diabetes mellitus develop microvascular complications of diabetes, namely nephropathy, neuropathy, and retinopathy. DR is a highly specific neurovascular complication of diabetes and is the most frequent cause of new blindness among adults aged 20-74 years in developed countries (1, 2). DR advances from mild nonproliferative abnormalities with increased vasopermeability and microaneurysms to moderate and severe stages characterized by the growth of new blood vessels in the retina and the posterior surface of the vitreous. Fibrous tissue may exert tension on the retina and cause retinal detachment. The new blood vessels may bleed and cause preretinal and vitreous hemorrhage. A macular edema causing central vision impairment may occur because of increased vasopermeability and capillary nonperfusion (3). Major risk factors include the duration of diabetes, HbA1c levels, and blood pressure (3, 4). The onset of puberty and pregnancy increase the risk of progression of DR. Tertiary prevention of DR includes laser photocoagulation for proliferative diabetic retinopathy (PDR), anti-VEGF therapy for DME and PDR, and vitrectomy in advanced DR (5). Various pathophysiological and pathobiochemical pathways directly linked to chronic hyperglycemia which lead to a disorganization and breakdown of the blood-retinal-barrier are involved in the manifestation of DR and DME, including an activation of protein kinase C (6) and the accumulation of advanced glycation end products (7). However, there are patient populations with type 1 diabetes of extreme duration who do not develop diabetic complications and appear to be protected by unknown factors (8, 9). This contrasts with other studies, which usually report that >90% of patients with type 1 diabetes will eventually develop retinopathy (10). Also, there was a lack of association between glycemic control and prevalence of reported microvascular complications (11). Consistently, the total glycemic exposure (A1C and duration of diabetes) explained only 11% of the variation in risk in the Diabetes Control and Complications Trial (DCCT) cohort, where retinopathy progression was studied in conventional and intensive treatment groups (12). It is thus acknowledged that significant numbers of patients with diabetes can live without severe complications, likely due to factors that can neutralize the adverse effects of hyperglycemia or other unknown protective factors which prevent the development of diabetic complications (11). Hormonal factors are predisposed to confer protective effects against microvascular complications through their effects on organ function, repair and maintenance of homeostasis, the control of growth, and their capacity to adapt their levels and action in response to demand or to pathologic stimuli. The investigation of pituitary hormones is therefore warranted.
Pituitary infarction revealed an involvement of pituitary hormones in diabetic retinopathy
A role of pituitary hormones in the etiopathology of DR emerged soon after the observation that infarction or insufficiency of the anterior lobe of the pituitary, can result in hypoglycemia and high sensitivity to administered insulin, known as the Houssay-Biasotti phenomenon. In fact, infarction, or insufficiency of the pituitary gland, also known as Simmond’s disease, can lead to terminal hypoglycemia, as reported in a series of early case studies (13, 14). Pituitary infarction can also occur after severe peri- or postpartum hemorrhage, as described by Sheehan (Sheehan’s syndrome). In all instances, examples of cessation or regression of diabetic retinopathy was observed. Soon thereafter, pituitary ablations, stalk sections, and destruction by irradiation were introduced for treating diabetic retinopathy but became obsolete in the face of the harmful effects that were associated with these procedures and the following anterior pituitary insufficiency. The beneficial effects of pituitary insufficiency were attributed to the cessation of growth hormone secretion and consecutively lower insulin-like growth factor I (IGF-I) levels, however, the overall resumé of repeated cross-sectional, longitudinal, and prospective studies on the relationship between circulating IGF-I levels and DR did not establish a clear role for the GH/IGF-I axis (15). Patients with acromegaly and diabetes mellitus do not have a higher prevalence of DR (16) and patients with diabetes and congenital IGF-I deficiency (Laron syndrome) or GH gene deletion can develop DR (17, 18). Disparate data are available on circulating IGF-I levels and DR progression during pregnancy, with studies finding or not finding an association of IGF-I levels with DR during pregnancy (19, 20). On the other hand, it is known that an acute reduction of chronic hyperglycemia can accelerate DR, and that this deterioration is preceded by an upregulation of serum IGF-I (21). Both, GH, and IGF-I are present in the vitreous and the levels of IGF-I are higher in the vitreous of patients with retinal neovascularization (22, 23). Mechanistically, IGF-I has mitogenic and differentiating effects on cultured retinal endothelial cells (24) and on retinal capillaries (25), and can induce neovascularization in the avascular rabbit cornea (26). IGF-I and its receptor, as well as IGF binding proteins are distributed throughout the retina, and IGF-I mRNA has been detected in the ganglion cell layer, the inner nuclear layer and in the outer limiting membrane (27, 28). The total IGF-I distribution in ocular tissues is therefore a combination of local expression and systemic uptake. Altogether, the contribution of local and circulating IGF-I in diabetic retinopathy remains to be understood, can be interpreted as rather “permissive” than causal (17) and therapeutic interventions into the GH/IGF-I axis did not yield sufficient evidence in clinical studies to be considered in the current treatment recommendations for DR (5). Attesting to the heterogeneity and variation in pathomechanisms of proliferative retinopathies across the lifespan, ample evidence demonstrates the key role of IGF-I in retinopathy of prematurity (29–32).
Circulating PRL levels change in diabetes
Another pituitary hormone which attracted attention in respect to its involvement in DR is PRL. Not long after the radioimmunoassay for PRL became available, which allowed the measurement of circulating PRL concentrations (33, 34), PRL was evaluated in patients without DR and DR at various stages. Early reports found higher PRL levels in patients with diabetes but without severe DR and hypothesized about the potential function of PRL as a protective factor in DR, and about some potential treatment based on the stimulation of PRL secretion (35, 36). Indeed, pituitary stalk section results in minimized GH secretion with subsequent decline of IGF-I levels but result in higher PRL-secretion due to a disinhibition of lactotroph PRL secretion by the disruption of dopamine transport through the pituitary stalk (37). The beneficial effects of pituitary stalk sections could therefore have been not only due to the reduction of IGF-I levels, but also due to an increase in circulating PRL. Comparable with IGF-I levels, various results were reported in which the association of PRL levels with DR presence and severity was not confirmed (38–41). A mechanism of action for protective effects of PRL levels was also missing. PRL exerts a diverse array of biological functions beyond its essential role in lactation (42–44), a fact which has received little attention in clinical medicine in the past, where the relevance of PRL is acknowledged in prolactinoma and secondary amenorrhea. Regarding diabetes and its complications, there is a new trend towards the recognition of PRL as an important metabolic hormone, directly involved in beta-cell function and survival, and the regulation of insulin sensitivity and resistance, respectively (45). Higher PRL levels are associated with higher insulin sensitivity and a lower incidence of type 2 diabetes mellitus, which led to a re-evaluation of current thresholds for normal PRL levels and hyperprolactinemia (45). It was proposed to re-define the interpretation of PRL levels beyond the upper threshold of 25 ng/ml where a homeostatic functionally increased transient hyperprolactinemia (homeoFIT) can be assumed, the suggested term for an elevation of PRL levels which may constitute a physiological response to increased metabolic demand (reviewed in ref. 45).
The PRL/vasoinhibin axis controls ocular angiogenesis and vascular function
A new perspective on the role of PRL in DR began to evolve when the antiangiogenic effects of an enzymatically cleaved 16 kDa N-terminal fragment of human PRL were discovered (46), and a direct pathophysiological implication towards the regulation of blood vessel growth emerged. It became evident that the 16 kDa N-terminal fragment is not the only fragment with antiangiogenic effects, and that multiple isoforms with a large variation in molecular mass exist, their size being determined by the PRL-cleaving enzyme and its cleavage site location within the PRL molecule. The isoforms were collectively called vasoinhibin (47–49), including similar proteins generated by the proteolytic cleavage of GH and placental lactogen (PL) (50, 51). A strong role of vasoinhibin as a regulator of ocular angiogenesis and vascular function evolved, and with reference to existing reviews (52–55), and 11 years after PRL and vasoinhibin were first portrayed as endogenous players in DR (56), the following discussion will focus on key principles and significant developments in the recent years (Table 1). The new understanding of circulating PRL levels in terms of homeoFIT-levels is relevant when considering the role of PRL and vasoinhibin in DR, as in partial disagreement to the early studies between 1970 and 1985, there appeared to be an association between circulating PRL levels and DR, reported by Arnold et al. in 2010 (62). The PRL levels were higher in patients with diabetes and no retinopathy (compared to healthy controls) and higher in patients with diabetes and non-proliferative DR than in patients with PDR (62). The PRL levels in the patients with diabetes were above the conventional threshold of 25 ng/ml, and therefore in the homeoFIT-range. In addition to answering to increased metabolic demand, PRL levels in the homeoFIT-range may also, through their proteolytic conversion to vasoinhibin, contribute to control the function and growth of ocular blood vessels. Interestingly, uncleaved PRL is protective in the retina and required for maintaining retinal functionality in mice during aging and has potential therapeutic value against age-related retinal disorders (68, 69). Short PRL isoforms are expressed in the canine retina undergoing retinal degeneration (70). A clinical study in patients with a prolactinoma using optical coherence tomography revealed a reduced thickness of the chorioretinal layers in patients with prolactinoma compared to controls (71). Patients with DR have a higher renal elimination of PRL (72) and the circulating levels of vasoinhibin are reduced in patients with DR (63).
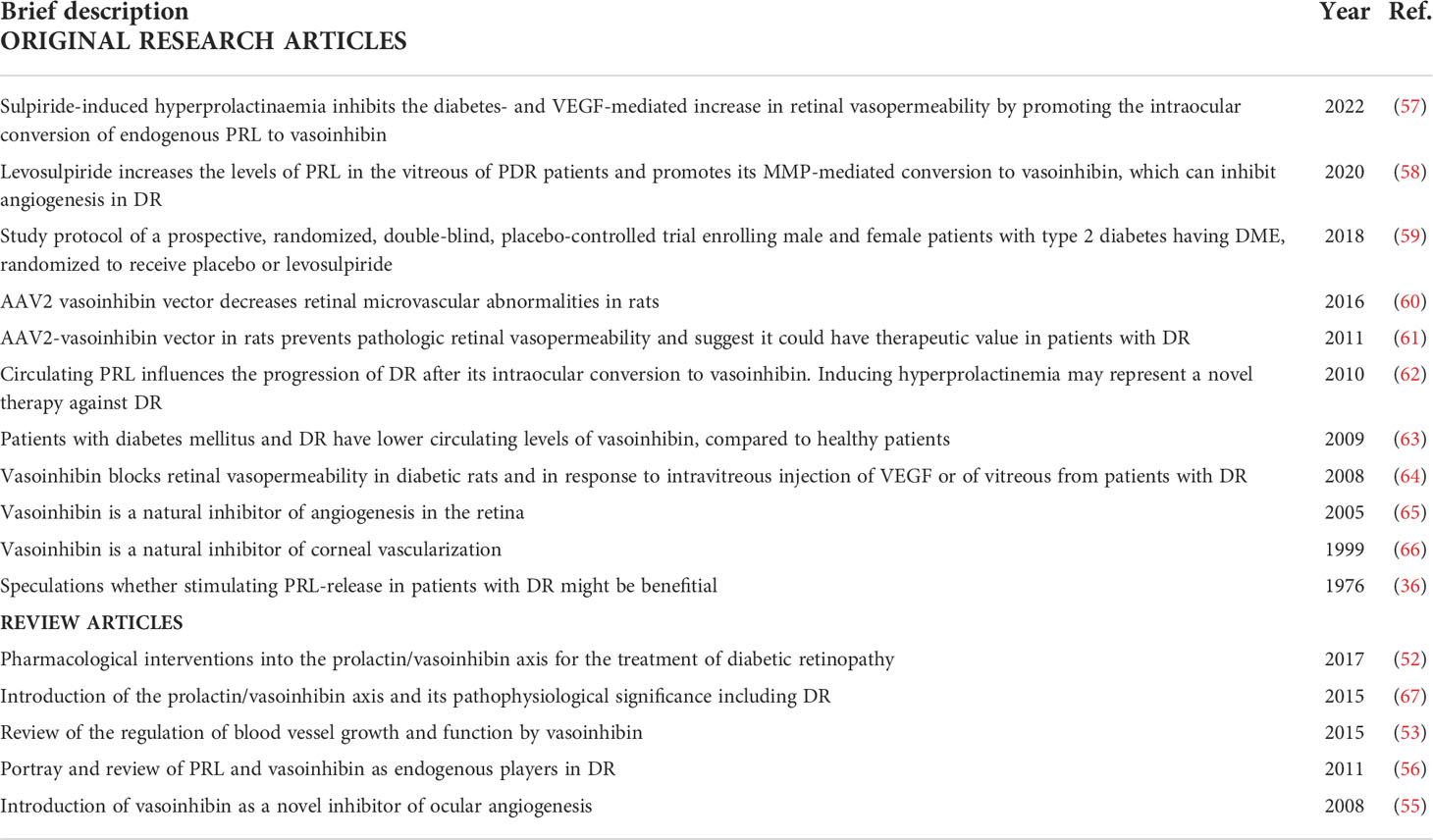
Table 1 Landmark original research articles and reviews highlighting the involvement of the prolactin/vasoinhibin axis in diabetic retinopathy.
The principle underlying vasoinhibin accumulation in the retina – or in other tissues – is that of an endocrine axis in which the levels of vasoinhibin are controlled by regulatory mechanisms at the hypothalamo-, the pituitary-, and the local level. The vasoinhibin levels depend on the availability and amount of secreted and circulating PRL (hypothalamo-pituitary level), and on the hypothalamo, pituitary, and peripheral tissue distribution and activities of PRL-cleaving proteases (local level). This hormonal axis was described as the PRL/vasoinhibin axis of which the vasculature is a major target tissue (53, 67). The cleavage sites in PRL through which vasoinhibin is generated are conserved in vertebrates (47, 67, 73) and high affinity cleavages sites evolved, most likely as a gain of function under positive selection, as a unique feature of higher primates (74). The cleavage of PRL to generate vasoinhibin occurs in the wider context of a hormone-metabolism junction, through which specifically cleaved hormones regulate essential functions to maintain homeostasis at the organismal, tissue, or organ levels (75, 76). The PRL/vasoinhibin axis contributes to maintaining corneal avascularity (66), restricts retinal vasculature (65), and is disrupted in retinopathy of prematurity (77, 78). In rodents, hyperprolactinemia leads to vasoinhibin accumulation in the retina and reduces both VEGF-induced and diabetes-induced retinal vasopermeability (57, 62, 64); an effect also demonstrated by vasoinhibin gene transfer which not only prevented (61) but also reversed (60) excessive retinal vasopermeability and oxygen-induced retinal angiogenesis (79).
The bioactive site in vasoinhibin, through which the antiangiogenic and antivasopermeability effects of the molecule are mediated, is a short, conserved three-residue motif consisting of residues His46-Gly47-Arg48 which becomes active after the proteolytic cleavage of PRL to vasoinhibin (80). Molecular dynamics simulations predicted the three-dimensional structure of vasoinhibin comprising a three-helix bundle with a tendency to form dimers or multimers, which also complicated the experimental resolution of the vasoinhibin three-dimensional structure (73, 81, 82). Vasoinhibin signals through various binding partners such as a specific high affinity binding site on endothelial cells (83), integrin alpha5 beta1 (84), or plasminogen activator inhibitor 1, urokinase, and urokinase receptor multicomponent complex (85) to trigger intracellular signaling pathways that result in its effects on endothelial cells but a classical hormone receptor has not been identified. The circulating levels of vasoinhibin are unknown due to the absence of a quantitative vasoinhibin assay for human serum, which is why immunoprecipitation followed by SDS-PAGE and Western blotting is still the only more frequently used method for the evaluation of vasoinhibin in clinical samples (77). Alternative methods using a lab-on-a chip technology or mass spectrometry were reported (63, 86, 87) but did not establish themselves thereafter. The lack of monoclonal anti-vasoinhibin antibodies able to discriminate between PRL and vasoinhibin prevented attempts to develop a sandwich enzyme-linked immunosorbent assay (ELISA). Fortunately, monoclonal antibodies were recently developed, and their evaluation for an ELISA by which the levels of vasoinhibin could be quantified is underway (88). However, Western blot evaluation of vasoinhibin in clinical samples is supported by the measurement of its antiangiogenic properties in the presence or absence of anti-PRL antibodies that neutralize vasoinhibin action (58, 89).
A clinical trial investigates the elevation of PRL-levels in patients with diabetic retinopathy
Increased, hypoxia-driven expression of VEGF, produced by the retinal pigment epithelium, by endothelial cells, pericytes and other retinal cells, with consecutive enrichment in the retina and vitreous is a major driver of DME and PDR as it contributes to rupturing the blood-retinal barrier and induces angiogenesis which results in pathological neovascularization. The healthy vitreous is one of the few naturally avascular structures but is invaded by blood vessels in PDR. Not only the elevation of growth factors facilitates its invasion by neovessels, the impaired production or insufficient upregulation of natural blood vessel inhibitors responsible for maintaining the avascular state of the vitreous are relevant as well (90). The healthy vitreous humor as such is antiangiogenic and inhibits tumor neovascularization (91), and angiogenesis in various other models, for example the retinal-extract induced angiogenesis in the chick chorioallantoic membrane (CAM) assay (92).
As mentioned, hyperprolactinemia leads to vasoinhibin accumulation in the retina of rats and prevents and reverses diabetes-induced blood retinal barrier breakdown and ischemia-induced angiogenesis by inhibiting vasopermeability and by targeting the retinal pigment epithelial cells in the outer blood retinal barrier (62, 93). These insights triggered the development of a randomized clinical trial, in which levosulpiride is evaluated as a medical treatment in patients with PDR and DME (59) (Figure 1). Levosulpiride is a dopamine D2 receptor blocker which is used as a prokinetic drug in patients with diabetic gastroparesis, where enteric inhibitory dopaminergic D2 receptor antagonism can have prokinetic effects. At the pituitary level D2 receptor antagonism with levosulpiride evokes hyperprolactinemia (94). One arm of the clinical study includes patients with PDR undergoing vitrectomy, with and without prior treatment with levosulpiride and subsequent laboratory evaluation of the vitreous fluid. Levosulpiride treatment increased PRL and vasoinhibin in the vitreous, and the vitreous from levosulpiride-treated patients with PDR, but not from placebo-treated patients with PDR, inhibited the basic fibroblast growth factor (bFGF) and VEGF-induced proliferation of endothelial cells in culture (58). The conversion of PRL to vasoinhibin was mediated by matrix metalloprotease (MMP) present in the vitreous fluid and was higher in patients without diabetes than in patients with PDR (58). This result is the first partial outcome of the clinical study which provided a proof-of-concept that treatment with levosulpiride is appropriate to elevate intraocular PRL and vasoinhibin levels. Further proof-of-concept was shown by an in vivo study in rats with streptozotocin-induced diabetes, in which racemic sulpiride increased ocular vasoinhibin levels and inhibited retinal hypervasopermeability (57). The other arms of the trial that also comprise patients with DME are awaiting completion and the publication of the results are expected soon.
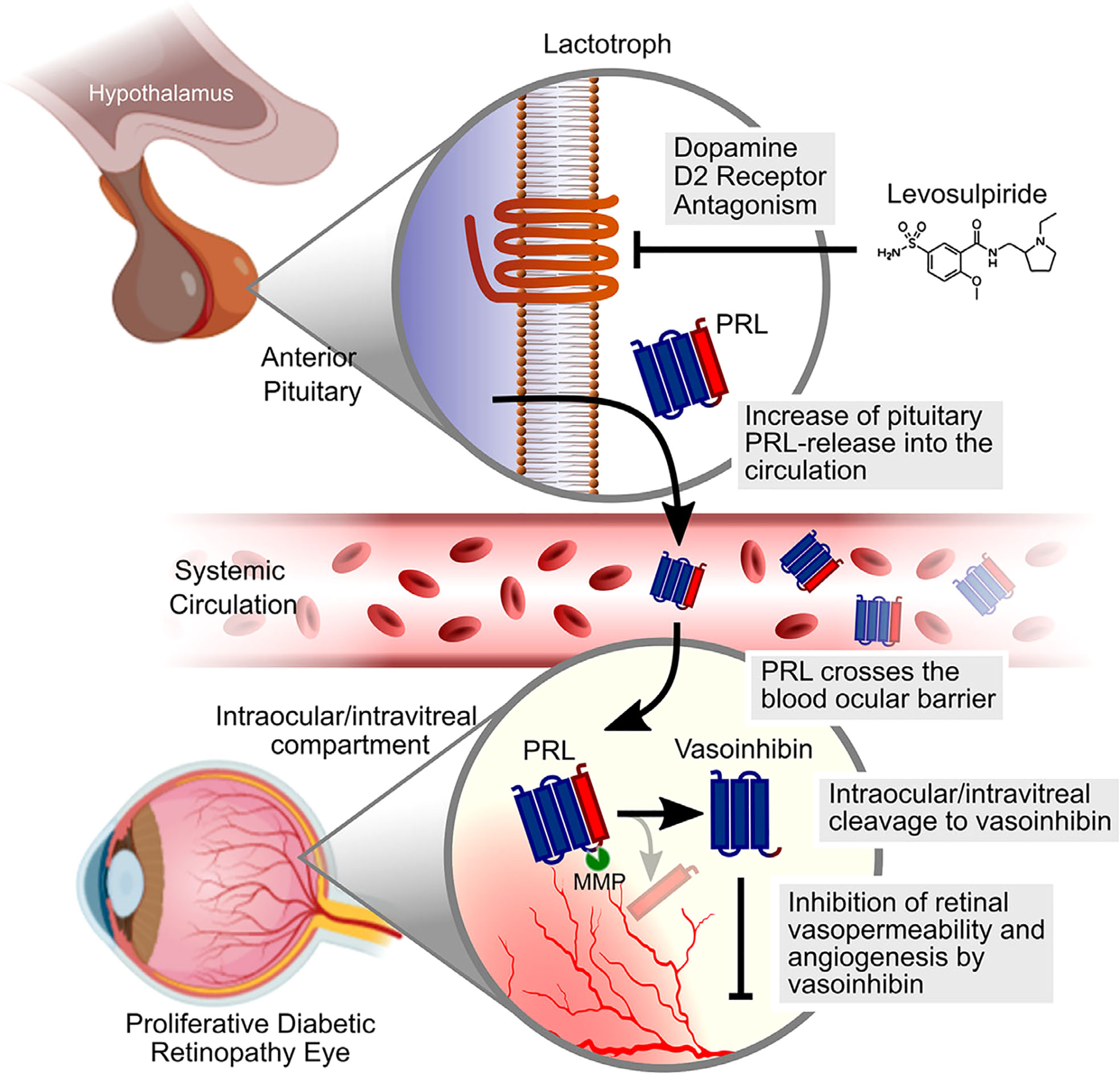
Figure 1 Schematic representation of the mechanism by which levosulpiride therapy could limit the progression of DME and DR. Levosulpiride, a dopamine D2 receptor antagonist, blocks dopamine D2 receptors located in the membrane of anterior pituitary cells that produce PRL (lactotrophs). Given that hypothalamic dopamine inhibits the release of PRL, levosulpiride leads to high levels of PRL in the circulation (hyperprolactinemia) which, in turn, favor PRL penetration across the blood–ocular barrier. MMPs in the intraocular/vitreous compartment cleave PRL to vasoinhibin, which can reduce retinal vasopermeability and angiogenesis in DME and DR. Scheme was partly created with Biorender.com. The original figure was published by Nunez-Amaro et al. (58) under the Creative Commons Attribution-Non-Commercial-NoDerivatives 4.0 International License (https://creativecommons.org/licenses/by-nc-nd/4.0/). The figure was not modified.
PRL and vasoinhibin are endogenous players in diabetic retinopathy with translational potential
By the providing the retina and the vitreous with PRL and antiangiogenic vasoinhibin, the PRL/vasoinhibin axis contributes to the physiological restricted and avascular states of the retina and vitreous body, respectively. The natural antiangiogenic capacity of the vitreous is impaired in DR, namely by the upregulation of factors stimulating blood vessel growth, but likewise by the downregulation of inhibitors. The downregulation includes a reduced MMP-mediated conversion of PRL to vasoinhibin in DR and facilitates an increase in retinal blood vessel permeability and neovascularization growing into the vitreous, with concurrent manifestation of edema, bleeding, tractional retinal detachment, and clinically loss of vision and blindness. Preclinical experimental and clinical proof-of-concept studies revealed the translational potential of raising systemic PRL levels to elevate ocular PRL levels and enhance the generation of vasoinhibin in the vitreous. The PRL/vasoinhibin axis and its regulation in diabetes is among the factors beyond glycemic exposure which may determine the risk of DME, and DR. Therapeutic interventions are currently evaluated in a clinical trial and will show whether patients with diabetes benefit from raising circulating PRL levels. The new clinical perspective of PRL in metabolism and its contribution to the control of blood vessel growth and function via the PRL/vasoinhibin axis is attesting to the clinical significance of PRL beyond reproduction-associated functions.
Author contributions
JT wrote the manuscript, TB and CC edited the manuscript. All authors approved the final version. All authors contributed to the article and approved the submitted version.
Funding
This study was supported by the National Council of Science and Technology (CONACYT, grants 289568 and A1-S-9620B) and UNAM (grant 405PC) to CC.
Conflict of interest
The authors declare that the research was conducted in the absence of any commercial or financial relationships that could be construed as a potential conflict of interest.
Publisher’s note
All claims expressed in this article are solely those of the authors and do not necessarily represent those of their affiliated organizations, or those of the publisher, the editors and the reviewers. Any product that may be evaluated in this article, or claim that may be made by its manufacturer, is not guaranteed or endorsed by the publisher.
References
1. Yau JW, Rogers SL, Kawasaki R, Lamoureux EL, Kowalski JW, Bek T, et al. Global prevalence and major risk factors of diabetic retinopathy. Diabetes Care (2012) 35(3):556–64. doi: 10.2337/dc11-1909
2. Boyer DS, Hopkins JJ, Sorof J, Ehrlich JS. Anti-vascular endothelial growth factor therapy for diabetic macular edema. Ther Adv Endocrinol Metab (2013) 4(6):151–69. doi: 10.1177/2042018813512360
3. Cheung N, Mitchell P, Wong TY. Diabetic retinopathy. Lancet (2010) 376(9735):124–36. doi: 10.1016/S0140-6736(09)62124-3
4. Stratton IM, Kohner EM, Aldington SJ, Turner RC, Holman RR, Manley SE, et al. UKPDS 50: risk factors for incidence and progression of retinopathy in type II diabetes over 6 years from diagnosis. Diabetologia (2001) 44(2):156–63. doi: 10.1007/s001250051594
5. Solomon SD, Chew E, Duh EJ, Sobrin L, Sun JK, VanderBeek BL, et al. Diabetic retinopathy: A position statement by the American diabetes association. Diabetes Care (2017) 40(3):412–8. doi: 10.2337/dc16-2641
6. Lee TS, MacGregor LC, Fluharty SJ, King GL. Differential regulation of protein kinase c and (Na,K)-adenosine triphosphatase activities by elevated glucose levels in retinal capillary endothelial cells. J Clin Invest (1989) 83(1):90–4. doi: 10.1172/JCI113889
7. Chibber R, Molinatti PA, Rosatto N, Lambourne B, Kohner EM. Toxic action of advanced glycation end products on cultured retinal capillary pericytes and endothelial cells: relevance to diabetic retinopathy. Diabetologia (1997) 40(2):156–64. doi: 10.1007/s001250050657
8. Sun JK, Keenan HA, Cavallerano JD, Asztalos BF, Schaefer EJ, Sell DR, et al. Protection from retinopathy and other complications in patients with type 1 diabetes of extreme duration: the joslin 50-year medalist study. Diabetes Care (2011) 34(4):968–74. doi: 10.2337/dc10-1675
9. Hirsch IB, Brownlee M. Beyond hemoglobin A1c–need for additional markers of risk for diabetic microvascular complications. JAMA J Am Med Assoc (2010) 303(22):2291–2. doi: 10.1001/jama.2010.785
10. Klein R, Klein BE, Moss SE, Davis MD, DeMets DL. The Wisconsin epidemiologic study of diabetic retinopathy. II. prevalence and risk of diabetic retinopathy when age at diagnosis is less than 30 years. Arch Ophthalmol (1984) 102(4):520–6. doi: 10.1001/archopht.1984.01040030398010
11. Keenan HA, Costacou T, Sun JK, Doria A, Cavellerano J, Coney J, et al. Clinical factors associated with resistance to microvascular complications in diabetic patients of extreme disease duration: the 50-year medalist study. Diabetes Care (2007) 30(8):1995–7. doi: 10.2337/dc06-2222
12. Lachin JM, Genuth S, Nathan DM, Zinman B, Rutledge BN, Group DER. Effect of glycemic exposure on the risk of microvascular complications in the diabetes control and complications trial–revisited. Diabetes (2008) 57(4):995–1001. doi: 10.2337/db07-1618
13. Poulsen JE. Diabetes and anterior pituitary insufficiency. final course and postmortem study of a diabetic patient with sheehan's syndrome. Diabetes (1966) 15(2):73–7. doi: 10.2337/diab.15.2.73
14. Poulsen JE. Recovery from retinopathy in a case of diabetes with simmonds' disease. Diabetes (1953) 2(1):7–12. doi: 10.2337/diab.2.1.7
15. Frystyk J. The growth hormone hypothesis - 2005 revision. Hormone Metab Res = Hormon- und Stoffwechselforschung = Hormones metabolisme (2005) 37 Suppl 1:44–8. doi: 10.1055/s-2005-861362
16. Azzoug S, Chentli F. Diabetic retinopathy in acromegaly. Indian J Endocrinol Metab (2014) 18(3):407–9. doi: 10.4103/2230-8210.131207
17. Laron Z, Weinberger D. Diabetic retinopathy in two patients with congenital IGF-I deficiency (Laron syndrome). Eur J Endocrinol / Eur Fed Endocrine Societies (2004) 151(1):103–6. doi: 10.1530/eje.0.1510103
18. Laron Z, Weinberger D. Diabetic retinopathy, nephropathy and cardiovascular disease in a patient with GH gene deletion. Clin Endocrinol (2005) 63(6):699–700. doi: 10.1111/j.1365-2265.2005.02402.x
19. Loukovaara S, Immonen IJ, Koistinen R, Rutanen EM, Hiilesmaa V, Loukovaara M, et al. The insulin-like growth factor system and type 1 diabetic retinopathy during pregnancy. J Diabetes its complications (2005) 19(5):297–304. doi: 10.1016/j.jdiacomp.2005.03.004
20. Ringholm L, Vestgaard M, Laugesen CS, Juul A, Damm P, Mathiesen ER. Pregnancy-induced increase in circulating IGF-I is associated with progression of diabetic retinopathy in women with type 1 diabetes. Growth hormone IGF Res Off J Growth Hormone Res Soc Int IGF Res Soc (2011) 21(1):25–30. doi: 10.1016/j.ghir.2010.12.001
21. Chantelau E. Evidence that upregulation of serum IGF-1 concentration can trigger acceleration of diabetic retinopathy. Br J Ophthalmol (1998) 82(7):725–30. doi: 10.1136/bjo.82.7.725
22. Harvey S, Parker E, Macdonald I, Sanders EJ. Growth hormone is present in the human retina and vitreous fluid. Neurosci Lett (2009) 455(3):199–202. doi: 10.1016/j.neulet.2009.03.073
23. Grant M, Russell B, Fitzgerald C, Merimee TJ. Insulin-like growth factors in vitreous. studies in control and diabetic subjects with neovascularization. Diabetes (1986) 35(4):416–20. doi: 10.2337/diab.35.4.416
24. Giannini S, Cresci B, Manuelli C, Fujita-Yamaguchi Y, Romagnani P, Mohan S, et al. Insulin-like growth factor binding protein production in bovine retinal endothelial cells. Metabolism: Clin Exp (1997) 46(12):1367–79. doi: 10.1016/s0026-0495(97)90134-7
25. King GL, Goodman AD, Buzney S, Moses A, Kahn CR. Receptors and growth-promoting effects of insulin and insulinlike growth factors on cells from bovine retinal capillaries and aorta. J Clin Invest (1985) 75(3):1028–36. doi: 10.1172/JCI111764
26. Grant MB, Caballero S, Millard WJ. Inhibition of IGF-I and b-FGF stimulated growth of human retinal endothelial cells by the somatostatin analogue, octreotide: a potential treatment for ocular neovascularization. Regul Pept (1993) 48(1-2):267–78. doi: 10.1016/0167-0115(93)90356-d
27. Burren CP, Berka JL, Edmondson SR, Werther GA, Batch JA. Localization of mRNAs for insulin-like growth factor-I (IGF-I), IGF-I receptor, and IGF binding proteins in rat eye. Invest Ophthalmol Visual Sci (1996) 37(7):1459–68.
28. Ocrant I, Valentino KL, King MG, Wimpy TH, Rosenfeld RG, Baskin DG. Localization and structural characterization of insulin-like growth factor receptors in mammalian retina. Endocrinology (1989) 125(5):2407–13. doi: 10.1210/endo-125-5-2407
29. Hellstrom A, Svensson E, Carlsson B, Niklasson A, Albertsson-Wikland K. Reduced retinal vascularization in children with growth hormone deficiency. J Clin Endocrinol Metab (1999) 84(2):795–8. doi: 10.1210/jcem.84.2.5484
30. Hellstrom A, Perruzzi C, Ju M, Engstrom E, Hard AL, Liu JL, et al. Low IGF-I suppresses VEGF-survival signaling in retinal endothelial cells: direct correlation with clinical retinopathy of prematurity. Proc Natl Acad Sci United States America (2001) 98(10):5804–8. doi: 10.1073/pnas.101113998
31. Hellstrom A, Engstrom E, Hard AL, Albertsson-Wikland K, Carlsson B, Niklasson A, et al. Postnatal serum insulin-like growth factor I deficiency is associated with retinopathy of prematurity and other complications of premature birth. Pediatrics (2003) 112(5):1016–20. doi: 10.1542/peds.112.5.1016
32. Lee JE, Perruzzi C, Ju M, Engstrom E, Hard A, Liu J, et al. Low IGF-1 suppresses VEGF-survival signaling in retinal endothelial cells: direct correlation with clinical retinopathy of prematurity. Survey Ophthalmol (2001) 98:5804–8. doi: 10.1016/s0039-6257(02)00455-1
33. Hwang P, Guyda H, Friesen H. A radioimmunoassay for human prolactin. Proc Natl Acad Sci United States America (1971) 68(8):1902–6. doi: 10.1073/pnas.68.8.1902
34. Sinha YN, Selby FW, Lewis UJ, VanderLaan WP. A homologous radioimmunoassay for human prolactin. J Clin Endocrinol Metab (1973) 36(3):509–16. doi: 10.1210/jcem-36-3-509
35. Hunter PR, Anderson J, Lunn TA, Horrobin DF, Boyns AR, Cole EN. Letter: Diabetic retinopathy and prolactin. Lancet (1974) 1(7868):1237. doi: 10.1016/s0140-6736(74)91060-5
36. Harter M, Balarac N, Pourcher P, Koslowski JM, Krebs B, Ramaioli A. Diabetic retinopathy and prolactin. Lancet (1976) 2(7992):961–2. doi: 10.1016/s0140-6736(76)90923-5
37. Turkington RW, Underwood LE, Van Wyk JJ. Elevated serum prolactin levels after pituitary-stalk section in man. New Engl J Med (1971) 285(13):707–10. doi: 10.1056/NEJM197109232851302
38. Larinkari J, Laatikainen L, Ranta T, Moronen P, Pesonen K, Laatikainen T. Metabolic control and serum hormone levels in relation to retinopathy in diabetic pregnancy. Diabetologia (1982) 22(5):327–32. doi: 10.1007/BF00253576
39. Froland A, Hagen C, Lauritzen E. Diabetic retinopathy and prolactin. Lancet (1977) 1(8001):45. doi: 10.1016/s0140-6736(77)91683-x
40. Cerasola GA, Donatelli M, Sinagra D, Russo V, Amico LM, Lodato G. Study of pituitary secretion in relation to retinopathy in patients with juvenile diabetes mellitus. Acta diabetologica latina (1981) 18(4):319–28. doi: 10.1007/BF02042815
41. Mooradian AD, Morley JE, Billington CJ, Slag MF, Elson MK, Shafer RB. Hyperprolactinaemia in male diabetics. Postgraduate Med J (1985) 61(711):11–4. doi: 10.1136/pgmj.61.711.11
42. Bole-Feysot C, Goffin V, Edery M, Binart N, Kelly PA. Prolactin (PRL) and its receptor: actions, signal transduction pathways and phenotypes observed in PRL receptor knockout mice. Endocrine Rev (1998) 19(3):225–68. doi: 10.1210/edrv.19.3.0334
43. Freeman ME, Kanyicska B, Lerant A, Nagy G. Prolactin: structure, function, and regulation of secretion. Physiol Rev (2000) 80(4):1523–631. doi: 10.1152/physrev.2000.80.4.1523
44. Ben-Jonathan N, LaPensee CR, LaPensee EW. What can we learn from rodents about prolactin in humans? Endocrine Rev (2008) 29(1):1–41. doi: 10.1210/er.2007-0017
45. Macotela Y, Triebel J, Clapp C. Time for a new perspective on prolactin in metabolism. Trends Endocrinol metabolism: TEM (2020) 31(4):276–86. doi: 10.1016/j.tem.2020.01.004
46. Clapp C, Martial JA, Guzman RC, Rentier-Delure F, Weiner RI. The 16-kilodalton n-terminal fragment of human prolactin is a potent inhibitor of angiogenesis. Endocrinology (1993) 133(3):1292–9. doi: 10.1210/endo.133.3.7689950
47. Macotela Y, Aguilar MB, Guzman-Morales J, Rivera JC, Zermeno C, Lopez-Barrera F, et al. Matrix metalloproteases from chondrocytes generate an antiangiogenic 16 kDa prolactin. J Cell Sci (2006) 119(Pt 9):1790–800. doi: 10.1242/jcs.02887
48. Triebel J, Robles JP, Zamora M, de la Escalera GM, Bertsch T, Clapp C. Regulator of angiogenesis and vascular function: A 2019 update of the vasoinhibin nomenclature. Front Endocrinol (2019) 10:214. doi: 10.3389/fendo.2019.00214
49. Triebel J, Bertsch T, de la Escalera GM, Clapp C. On the path toward classifying hormones of the vasoinhibin-family. Front Endocrinol (2015) 6:16. doi: 10.3389/fendo.2015.00016
50. Struman I, Bentzien F, Lee H, Mainfroid V, D'Angelo G, Goffin V, et al. Opposing actions of intact and n-terminal fragments of the human prolactin/growth hormone family members on angiogenesis: an efficient mechanism for the regulation of angiogenesis. Proc Natl Acad Sci United States America (1999) 96(4):1246–51. doi: 10.1073/pnas.96.4.1246
51. Markl-Hahn H, Neugebauer L, Lenke L, Ecker S, Merz T, McCook O, et al. Human placental tissue contains a placental lactogen-derived vasoinhibin. J Endocr Soc (2022) 6(4):bvac029. doi: 10.1210/jendso/bvac029
52. Triebel J, Robles-Osorio ML, Garcia-Franco R, de la Escalera GM, Clapp C, Bertsch T. From bench to bedside: Translating the Prolactin/Vasoinhibin axis. Front Endocrinol (2017) 8:342. doi: 10.3389/fendo.2017.00342
53. Clapp C, Thebault S, Macotela Y, Moreno-Carranza B, Triebel J, de la Escalera GM. Regulation of blood vessels by prolactin and vasoinhibins. Adv Exp Med Biol (2015) 846:83–95. doi: 10.1007/978-3-319-12114-7_4
54. Clapp C, Thebault S, Jeziorski MC, de la Escalera GM. Peptide hormone regulation of angiogenesis. Physiol Rev (2009) 89(4):1177–215. doi: 10.1152/physrev.00024.2009
55. Clapp C, Thebault S, Arnold E, Garcia C, Rivera JC, de la Escalera GM. Vasoinhibins: novel inhibitors of ocular angiogenesis. Am J Physiol Endocrinol Metab (2008) 295(4):E772–8. doi: 10.1152/ajpendo.90358.2008
56. Triebel J, Macotela Y, de la Escalera GM, Clapp C. Prolactin and vasoinhibins: Endogenous players in diabetic retinopathy. IUBMB Life (2011) 63(10):806–10. doi: 10.1002/iub.518
57. Adan-Castro E, Siqueiros-Marquez L, Ramirez-Hernandez G, Diaz-Lezama N, Ruiz-Herrera X, Nunez FF, et al. Sulpiride-induced hyperprolactinaemia increases retinal vasoinhibin and protects against diabetic retinopathy in rats. J Neuroendocrinol (2022) 34(4):e13091. doi: 10.1111/jne.13091
58. Nunez-Amaro CD, Moreno-Vega AI, Adan-Castro E, Zamora M, Garcia-Franco R, Ramirez-Neria P, et al. Levosulpiride increases the levels of prolactin and antiangiogenic vasoinhibin in the vitreous of patients with proliferative diabetic retinopathy. Transl Vis Sci Technol (2020) 9(9):27. doi: 10.1167/tvst.9.9.27
59. Robles-Osorio ML, Garcia-Franco R, Nunez-Amaro CD, Mira-Lorenzo X, Ramirez-Neria P, Hernandez W, et al. Basis and design of a randomized clinical trial to evaluate the effect of levosulpiride on retinal alterations in patients with diabetic retinopathy and diabetic macular edema. Front Endocrinol (2018) 9:242. doi: 10.3389/fendo.2018.00242
60. Diaz-Lezama N, Wu Z, Adan-Castro E, Arnold E, Vazquez-Membrillo M, Arredondo-Zamarripa D, et al. Diabetes enhances the efficacy of AAV2 vectors in the retina: therapeutic effect of AAV2 encoding vasoinhibin and soluble VEGF receptor 1. Lab investigation J Tech Methods Pathol (2016) 96(3):283–95. doi: 10.1038/labinvest.2015.135
61. Ramirez M, Wu Z, Moreno-Carranza B, Jeziorski MC, Arnold E, Diaz-Lezama N, et al. Vasoinhibin gene transfer by adenoassociated virus type 2 protects against VEGF- and diabetes-induced retinal vasopermeability. Invest Ophthalmol Visual Sci (2011) 52(12):8944–50. doi: 10.1167/iovs.11-8190
62. Arnold E, Rivera JC, Thebault S, Moreno-Paramo D, Quiroz-Mercado H, Quintanar-Stephano A, et al. High levels of serum prolactin protect against diabetic retinopathy by increasing ocular vasoinhibins. Diabetes (2010) 59(12):3192–7. doi: 10.2337/db10-0873
63. Triebel J, Huefner M, Ramadori G. Investigation of prolactin-related vasoinhibin in sera from patients with diabetic retinopathy. Eur J Endocrinol / Eur Fed Endocrine Societies (2009) 161(2):345–53. doi: 10.1530/EJE-09-0130
64. Garcia C, Aranda J, Arnold E, Thebault S, Macotela Y, Lopez-Casillas F, et al. Vasoinhibins prevent retinal vasopermeability associated with diabetic retinopathy in rats via protein phosphatase 2A-dependent eNOS inactivation. J Clin Invest (2008) 118(6):2291–300. doi: 10.1172/JCI34508
65. Aranda J, Rivera JC, Jeziorski MC, Riesgo-Escovar J, Nava G, Lopez-Barrera F, et al. Prolactins are natural inhibitors of angiogenesis in the retina. Invest Ophthalmol Visual Sci (2005) 46(8):2947–53. doi: 10.1167/iovs.05-0173
66. Duenas Z, Torner L, Corbacho AM, Ochoa A, Gutierrez-Ospina G, Lopez-Barrera F, et al. Inhibition of rat corneal angiogenesis by 16-kDa prolactin and by endogenous prolactin-like molecules. Invest Ophthalmol Visual Sci (1999) 40(11):2498–505.
67. Triebel J, Bertsch T, Bollheimer C, Rios-Barrera D, Pearce CF, Hufner M, et al. Principles of the prolactin/vasoinhibin axis. Am J Physiol Regulatory Integr Comp Physiol (2015) 309(10):R1193–203. doi: 10.1152/ajpregu.00256.2015
68. Arnold E, Thebault S, Arona RM, de la Escalera GM, Clapp C. Prolactin mitigates deficiencies of retinal function associated with aging. Neurobiol Aging (2020) 85:38–48. doi: 10.1016/j.neurobiolaging.2019.10.002
69. Melendez Garcia R, Arredondo Zamarripa D, Arnold E, Ruiz-Herrera X, Noguez Imm R, Baeza Cruz G, et al. Prolactin protects retinal pigment epithelium by inhibiting sirtuin 2-dependent cell death. EBioMedicine (2016) 7:35–49. doi: 10.1016/j.ebiom.2016.03.048
70. Sudharsan R, Murgiano L, Tang HY, Olsen TW, Chavali VRM, Aguirre GD, et al. Short prolactin isoforms are expressed in photoreceptors of canine retinas undergoing retinal degeneration. Sci Rep (2021) 11(1):460. doi: 10.1038/s41598-020-80691-6
71. Ogmen BE, Ugurlu N, Faki S, Polat SB, Ersoy R, Cakir B. Retinal layers in prolactinoma patients: a spectral-domain optical coherence tomography study. Int Ophthalmol (2021) 41(4):1373–9. doi: 10.1007/s10792-021-01701-8
72. Triebel J, Moreno-Vega AI, Vazquez-Membrillo M, Nava G, Garcia-Franco R, Lopez-Star E, et al. High prolactin excretion in patients with diabetes mellitus and impaired renal function. Clin Lab (2015) 61(7):709–16. doi: 10.7754/clin.lab.2014.141237
73. Zamora M, Robles JP, Aguilar MB, Romero-Gomez SJ, Bertsch T, de la Escalera GM, et al. Thrombin cleaves prolactin into a potent 5.6-kDa vasoinhibin: Implication for tissue repair. Endocrinology (2021) 162(12):1–15. doi: 10.1210/endocr/bqab177
74. Leuchs A, Davies N, Friedrich C, Trier S, Clapp C, Bertsch T, et al. A comparative phylogenetic analysis of prolactin cleavage sites for the generation of vasoinhibin in vertebrates. Gen Comp Endocrinol (2022) 320:114011. doi: 10.1016/j.ygcen.2022.114011
75. Triebel J, Robles JP, Zamora M, Clapp C, Bertsch T. New horizons in specific hormone proteolysis. Trends Endocrinol metabolism: TEM (2022) 33(6):371–7. doi: 10.1016/j.tem.2022.03.004
76. Campbell KL, Haspel N, Gath C, Kurniatash N, Nouduri Akkiraju I, Stuffers N, et al. Protein hormone fragmentation in intercellular signaling: hormones as nested information systems. Biol Reprod (2021) 104(4):887–901. doi: 10.1093/biolre/ioaa234
77. Zepeda-Romero LC, Vazquez-Membrillo M, Adan-Castro E, Gomez-Aguayo F, Gutierrez-Padilla JA, Angulo-Castellanos E, et al. Higher prolactin and vasoinhibin serum levels associated with incidence and progression of retinopathy of prematurity. Pediatr Res (2017) 81(3):473–9. doi: 10.1038/pr.2016.241
78. Duenas Z, Rivera JC, Quiroz-Mercado H, Aranda J, Macotela Y, Montes de Oca P, et al. Prolactin in eyes of patients with retinopathy of prematurity: implications for vascular regression. Invest Ophthalmol Visual Sci (2004) 45(7):2049–55. doi: 10.1167/iovs.03-1346
79. Pan H, Nguyen NQ, Yoshida H, Bentzien F, Shaw LC, Rentier-Delrue F, et al. Molecular targeting of antiangiogenic factor 16K hPRL inhibits oxygen-induced retinopathy in mice. Invest Ophthalmol Visual Sci (2004) 45(7):2413–9. doi: 10.1167/iovs.03-1001
80. Robles JP, Zamora M, Siqueiros-Marquez L, Adan-Castro E, Ramirez-Hernandez G, Nunez FF, et al. The HGR motif is the antiangiogenic determinant of vasoinhibin: implications for a therapeutic orally active oligopeptide. Angiogenesis (2022) 25(1):57–70. doi: 10.1007/s10456-021-09800-x
81. Robles JP, Zamora M, Velasco-Bolom JL, Tovar M, Garduno-Juarez R, Bertsch T, et al. Vasoinhibin comprises a three-helix bundle and its antiangiogenic domain is located within the first 79 residues. Sci Rep (2018) 8(1):17111. doi: 10.1038/s41598-018-35383-7
82. Triebel J, Schauer N, del RPF, Aguilar M, Robles J-P, lEGM de, et al. The vasoinhibin solution structure appears unfolded, dynamic, and features aggregation. Endocrine Abstracts (2016) 41:EP861. doi: 10.1530/endoabs.41.EP861
83. Clapp C, Weiner RI. A specific, high affinity, saturable binding site for the 16-kilodalton fragment of prolactin on capillary endothelial cells. Endocrinology (1992) 130(3):1380–6. doi: 10.1210/endo.130.3.1311239
84. Morohoshi K, Mochinaga R, Watanabe T, Nakajima R, Harigaya T. 16 kDa vasoinhibin binds to integrin alpha5 beta1 on endothelial cells to induce apoptosis. Endocrine connections (2018) 7(5):630–6. doi: 10.1530/EC-18-0116
85. Bajou K, Herkenne S, Thijssen VL, D'Amico S, Nguyen NQ, Bouche A, et al. PAI-1 mediates the antiangiogenic and profibrinolytic effects of 16K prolactin. Nat Med (2014) 20(7):741–7. doi: 10.1038/nm.3552
86. Waybright TJ, Xu X, Faupel-Badger JM, Xiao Z. Preparation of human serum for prolactin measurement by multiple reaction monitoring mass spectrometry. Methods Mol Biol (2013) 1002:195–203. doi: 10.1007/978-1-62703-360-2_16
87. Nakajima R, Ishida M, Kamiya CA, Yoshimatsu J, Suzuki M, Hirota A, et al. Elevated vasoinhibin derived from prolactin and cathepsin d activities in sera of patients with preeclampsia. Hypertens Res (2015) 38(12):899–901. doi: 10.1038/hr.2015.99
88. Muller N, Robles JP, Zamora M, Ebnet J, Markl-Hahn H, de la Escalera GM, et al. Development of vasoinhibin-specific monoclonal antibodies. Front Endocrinol (2021) 12:645085. doi: 10.3389/fendo.2021.645085
89. Gonzalez C, Parra A, Ramirez-Peredo J, Garcia C, Rivera JC, Macotela Y, et al. Elevated vasoinhibins may contribute to endothelial cell dysfunction and low birth weight in preeclampsia. Lab investigation J Tech Methods Pathol (2007) 87(10):1009–17. doi: 10.1038/labinvest.3700662
90. Aranda J, Motiejunaite R, Silva P, Aiello LP, Kazlauskas A. Regression activity that is naturally present in vitreous becomes ineffective as patients develop proliferative diabetic retinopathy. Diabetologia (2013) 56(6):1444–53. doi: 10.1007/s00125-013-2884-2
91. Felton SM, Brown GC, Felberg NT, Federman JL. Vitreous inhibition of tumor neovascularization. Arch Ophthalmol (1979) 97(9):1710–3. doi: 10.1001/archopht.1979.01020020278019
92. Lutty GA, Thompson DC, Gallup JY, Mello RJ, Patz A, Fenselau A. Vitreous: an inhibitor of retinal extract-induced neovascularization. Invest Ophthalmol Visual Sci (1983) 24(1):52–6.
93. Arredondo Zamarripa D, Diaz-Lezama N, Melendez Garcia R, Chavez Balderas J, Adan N, Ledesma-Colunga MG, et al. Vasoinhibins regulate the inner and outer blood-retinal barrier and limit retinal oxidative stress. Front Cell Neurosci (2014) 8:333. doi: 10.3389/fncel.2014.00333
Keywords: vasoinhibin, PRL, diabetic retinopathy, diabetic macular edema, diabetes, levosulpiride
Citation: Triebel J, Bertsch T and Clapp C (2022) Prolactin and vasoinhibin are endogenous players in diabetic retinopathy revisited. Front. Endocrinol. 13:994898. doi: 10.3389/fendo.2022.994898
Received: 15 July 2022; Accepted: 18 August 2022;
Published: 09 September 2022.
Edited by:
Damasia Becu-Villalobos, CONICET Instituto de Biología y Medicina Experimental (IBYME), ArgentinaReviewed by:
Vera Popovic-Brkic, University of Belgrade, SerbiaCarolina Cristina, National University of Northwestern Buenos Aires, Argentina
Copyright © 2022 Triebel, Bertsch and Clapp. This is an open-access article distributed under the terms of the Creative Commons Attribution License (CC BY). The use, distribution or reproduction in other forums is permitted, provided the original author(s) and the copyright owner(s) are credited and that the original publication in this journal is cited, in accordance with accepted academic practice. No use, distribution or reproduction is permitted which does not comply with these terms.
*Correspondence: Jakob Triebel, amFrb2IudHJpZWJlbEBnbXguZGU=
†ORCID: Jakob Triebel, orcid.org/0000-0002-1989-8645, Carmen Clapp, orcid.org/0000-0002-7670-6718