- 1Department of Orthopaedics, Shanghai Tenth People’s Hospital, School of Medicine, Tongji University, Shanghai, China
- 2Shanghai Key Laboratory for Prevention and Treatment of Bone and Joint Diseases, Shanghai Institute of Traumatology and Orthopaedics, Ruijin Hospital, Shanghai Jiao Tong University School of Medicine, Shanghai, China
Osteoporosis (OP) is a systemic disease characterized by bone metabolism imbalance and bone microstructure destruction, which causes serious social and economic burden. At present, the diagnosis and treatment of OP mainly rely on imaging combined with drugs. However, the existing pathogenic mechanisms, diagnosis and treatment strategies for OP are not clear and effective enough, and the disease progression that cannot reflect OP further restricts its effective treatment. The application of metabolomics has facilitated the study of OP, further exploring the mechanism and behavior of bone cells, prevention, and treatment of the disease from various metabolic perspectives, finally realizing the possibility of a holistic approach. In this review, we focus on the application of metabolomics in OP research, especially the newer systematic application of metabolomics and treatment with herbal medicine and their extracts. In addition, the prospects of clinical transformation in related fields are also discussed. The aim of this study is to highlight the use of metabolomics in OP research, especially in exploring the pathogenesis of OP and the therapeutic mechanisms of natural herbal medicine, for the benefit of interdisciplinary researchers including clinicians, biologists, and materials engineers.
1. Introduction
OP is a systemic metabolic bone disease characterized by damage to the microstructure of bone tissue and reduced bone mass, resulting in increased bone fragility and susceptibility to fractures. The main clinical manifestations of OP are height loss and hunchback, which increases the risk of fractures in multiple parts such as the hip and spine, and the probability of fracture varies from country to country (1–3). Fractures are predicted to occur in women over age 50 and in one in five men, resulting in limited quality of life and increased morbidity and mortality (4–6). Therefore, with the increasing aging of the population, complications such as osteoporotic fractures have become a worldwide problem, and the economic burden is increasing exponentially. Therefore, it is particularly important to comprehensively prevent and treat OP from the aspects of pathogenic mechanism, prevention, and treatment.
At present, OP has received great attention and extensive research, and a routine prevention and treatment system has also been formed in clinical practice (7–11). For instance, for the prevention of OP, patients are mainly encouraged to take calcium and vitamin D supplements early in clinical practice, and increase the time of sunlight exposure. However, this method has poor specificity and effectiveness, and lacks pertinence and targeting, making it difficult to effectively slow down the development of bone loss. At the same time, dual energy X-ray is insufficient in revealing the strength and structure of the bone tissue (12, 13). Although various drugs are developed in clinical OP treatment via confirmed mechanisms, including hormone replacement, alendronate sodium, parathyroid hormone, and RANKL inhibitors, etc. (14–16), the osteoporotic symptom still hard to completely reverse. Therefore, more therapeutic clues, especially metabolic ones should be concerned in the field of OP prevention.
The collection of small-molecule chemical entities involved in metabolic forms the metabolome. Metabolomics has been redefined from a simple biomarker identification tool to a technique for discovering active drivers of biological processes (17–19). It detects multiple metabolite changes during environmental exposure in a high-throughput form and are closely related to pathological phenotype, especially for multifunctional disease such as OP (20, 21). Therefore, metabolomics emerges an increasingly important role in the systematic study of OP. It is worth noting that, using metabolomics, the functions of traditional Chinese medicines such as Epimedium, Gushudan on OP treatment have been explored. However, there is a lack of systematic induction and research on the metabolic mechanism of various natural herbs in the treatment of OP.
Thus, metabolomics plays an important role in multi-field research of OP, which can deeply explore many problems closely related to OP and provide a new approach for comprehensive research and evaluation of OP. This review systematized various applications of metabolomics in OP research, including mechanism exploration, prevention, prediction, and drug treatment effect. In particular, we focused on the key metabolite changes in OP and after treatment with natural herbal medicines. Lots of metabolites are summarized to correlated with OP treatment, which could be useful for clinical transformation in related fields.
2. Abnormal metabolism in OP
Bone undergoes a constantly active metabolic cycle which is essential to maintain a healthy bone composition through the deposition and absorption of bone matrix and minerals. Imbalance and/or dysregulation of specific biochemical cascades of enzymes involved in protein, fat, and carbohydrates in bone metabolism can lead to various types of osteocyte dysfunction and related metabolic bone disease (22, 23).
Postmenopausal OP is characterized by loss of estrogen that leads to metabolic disorders in bone tissue. Metabolomics assays are factors associated with biological or metabolic status, and these metabolites are highly correlated pathogenic mechanisms of postmenopausal OP (24–31). On the other hand, abnormal differentiation of bone mesenchymal stem cells is related to the occurrence of senile OP. What’s more, under the influence of endogenous and exogenous factors such as hormone abuse, menopause, and aging, BMS over-differentiate into fat cells instead of osteoblasts, which often leads to bone metabolism imbalance and even OP. Therefore, a comprehensive understanding of the cellular metabolism and functional changes of bone marrow mesenchymal stem cells with aging is of great significance for the mechanism exploration and clinical treatment of senile OP.
There is increasing evidence that some of the causative factors are modifiable risk factors for OP, such as abnormal drug intake, high fat, and abnormal hormone levels. Studies have shown that these substances can induce secondary OP by regulating changes in metabolite levels (32–38).
Cholesterol participates in many cellular structures, and hydroxysterols, steroid hormones and bile acids play an important role in the formation of cell membranes. Therefore, dysfunction in cholesterol synthesis associates with various diseases and disorders (39, 40). In the OP model, the precise control of cholesterol synthesis and transport is affected, evidenced by the abnormal level of isoprene and squalene. Fatty acid metabolism involves a series of enzymes that degrade fatty acids into bioactive substrates to synthesize straight chain fatty acids, which are stored in adipose tissue as triglycerides (41, 42). In postmenopausal OP, fatty acyl-coa and pyruvate are converted to acetyl-coa by glycolysis, and subsequent metabolic pathways for synthesis of NADH, guanosine triphosphate and amino acids are disrupted (42, 43). Glycogen is easily mobilized as a long-branched polymer of glucose residues and can be broken down into glucose to provide the body with the required energy. Human osteoblasts and bone marrow mesenchymal stem cells in patients with secondary OP can be manifested as abnormal glucose metabolism of creatine, glucokinase and phosphorylase (44).
Therefore, in recent years, it has been found that there are many metabolic pathways in OP, related with abnormal bone remodeling. However, the changes of these metabolites and pathways and their roles in the pathogenesis of OP remain unclear (44, 45).
3. Metabolomics sample preparation and platform technologies
3.1 Metabolomics sample preparation
OP can be classified as primary or secondary according to its cause. For primary OP, biological samples can be collected from postmenopausal women and the elderly in clinical studies, whereas animal models could be accomplished by surgical ovaries resection (46, 47). For secondary OP, most samples are come from animal models, including glucocorticoids injection, fixation, special diet and retinoic acid lavage (45).
In sample preparation for metabolomics of OP, the following samples are usually included: urine, plasma, serum, osteocytes, bone cells, etc (48, 49). Urine samples are usually centrifuged directly without any dilution treatment, or diluted with pure water. Protein-rich serum and plasma are deproteinized with organic solvents such as acetonitrile and methanol. In metabolomics analysis, plasma and serum samples also require the use of silylation reagents such as trifluoroacetamide and trimethylsilane to improve the stability of metabolites. Proteins in blood samples are ultrafiltered through high molecular weight cut-off filters. The pH of the sample has a significant effect on the chemical shifts observed in NMR spectroscopy, so it is important to control the pH of the biofluid sample. To provide a stable environment for urine samples, a phosphate buffer (pH 6.8) is usually used (50–52).
For osteoblast samples, the cell pellet was resuspended in water, and then the membrane was broken with ultrasound and extracted with cold water mixed with methanol (53). After the above extraction process, samples are diluted or centrifuged in mobile phase, evaporated to dryness, and finally resuspended in a compatible solvent for further injection into metabolomics-related systems (17, 54). To obtain accurate metabolites, cells need to stop their metabolic activity almost immediately, and the classical methods include enzymatic denaturation and freezing (55). After extraction of metabolites from bone tissue samples, the tissue is pressed between metal plates in the presence of liquid nitrogen for rapid collection and rapid freezing of bone tissue. Care must be taken before and during sampling to avoid metabolic changes, which may be caused by anesthesia and other procedures (56).
3.2 Metabolomics platform technologies
After preparing the relevant samples, it is important to choose a suitable protocol and platform technologies in exploring the metabolomics. Currently, LC/MS (liquid chromatography/MS), GC/MS (gas chromatography/MS) and 1H NMR (nuclear magnetic resonance) are the main tools for exploring OP metabolomics (1, 57, 58). 1H NMR is suitable for the preliminary exploration of metabolomics, where most compounds can be detected. This method does not damage the sample, pre-treatment is relatively simple and can be used for quantitative data analysis. At now, 1H NMR still plays an important role in metabolomics because of its high ability in detecting the metabolites and elucidate structures in vivo. Its disadvantage is the narrow detection window and lack of sensitivity (59, 60).
MS has great advantages over 1H NMR in terms of sensitivity and specificity, and MS can detect potential new biomarkers associated with OP and has a good ability to detect metabolites at low concentrations and without parental interference, which, combined with modern high-resolution MS, plays an important role in the study of metabolomics. However, it also has the disadvantage of poor homogeneity and integrity (61, 62). Chromatography can be used for the separation of complex osteoporotic compounds and has promising applications. Another advantage of chromatography is the possibility of separating isomers. The addition of chromatography can improve the detection of low concentrations of metabolites, increase the coverage of metabolomics, and improve the quantitative accuracy of MS, but it also has disadvantages, such as insufficient qualitative capabilities. There are many kinds of chromatographic derivatives, such as reversed-phase liquid chromatography (RPLC), high performance liquid chromatography (HPLC), reversed-phase liquid chromatography (RPLC) hydrophilic liquid chromatography (HILC), and ultra-high energy liquid chromatography (UHPLC) (54). Currently, different chromatographic methods are often combined with MS to complement each other. It is often used in combination with GC/MS and LC/MS and has a wide range of applications in exploring OP with high sensitivity and good selectivity. It also allows the quantitative and qualitative analysis of complex metabolic compounds (57, 63, 64).
4. Metabolomics in OP pathogenesis research
In recent years, using metabolomics, the pathogenesis of OP has been comprehensively studied. OP can lead to profound metabolic changes in bone marrow and bone, involving many different metabolites and metabolic pathways (65, 66), as shown in Figure 1. The related mechanisms mainly involve amino acid metabolism, lipid metabolism, glucose metabolism, energy metabolism, etc. Lipid metabolism plays an important role in the pathogenesis of senile OP. In addition, lipid metabolism in idiopathic OP is also disturbed. Secondary OP has a clear etiology, and its metabolization-related pathogenesis varies from disease to disease, usually involving lipid metabolism, amino acid metabolism, mitochondrial energy metabolism, etc. (24–38, 67, 68), as shown in Table 1.
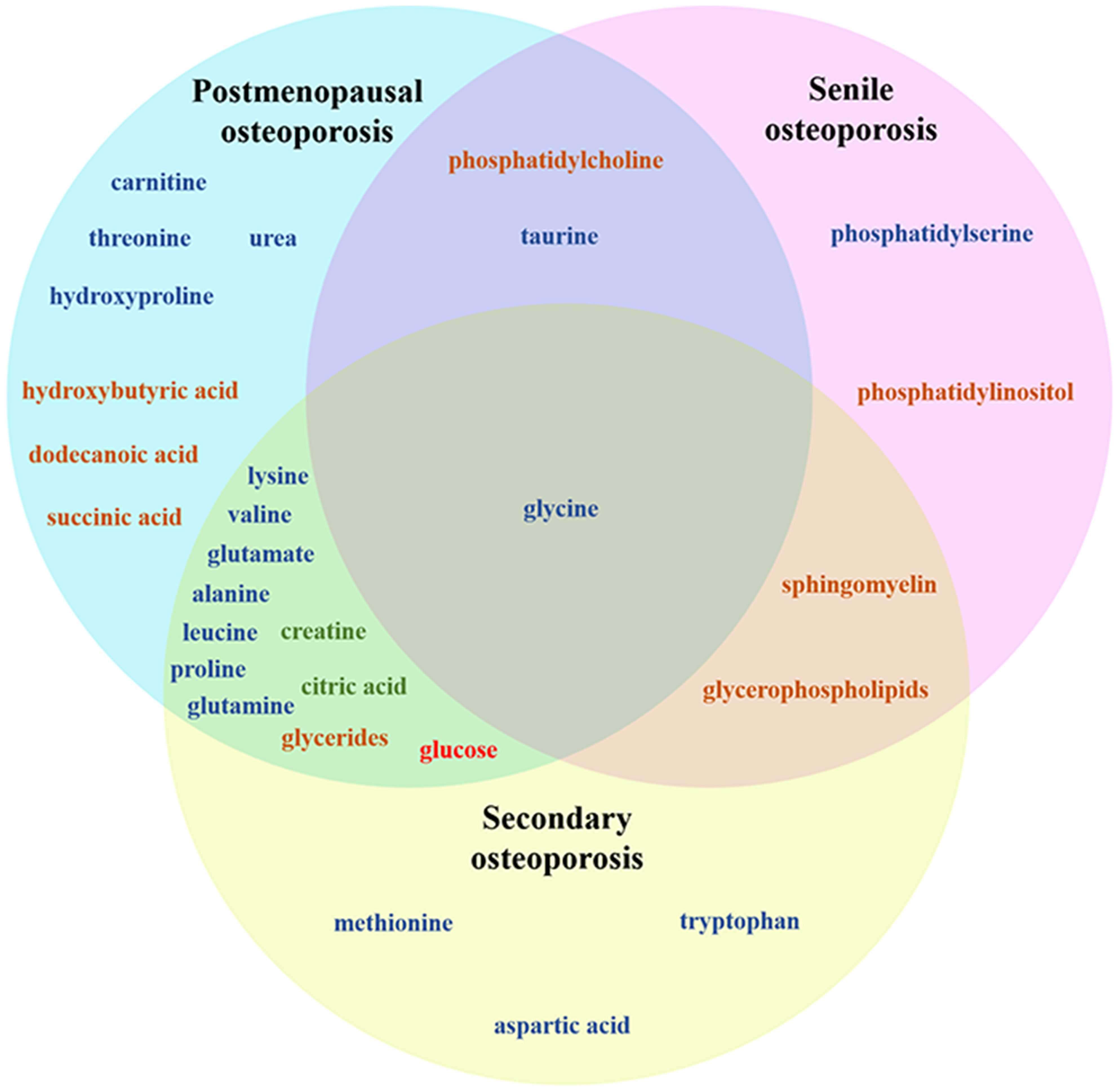
Figure 1 Venn diagram of metabolite changes in different types of OP pathogenesis. Dark blue: Amino acid metabolism product, dark yellow: Lipid metabolism product, dark green: Energy metabolism product, red: sugar metabolite.
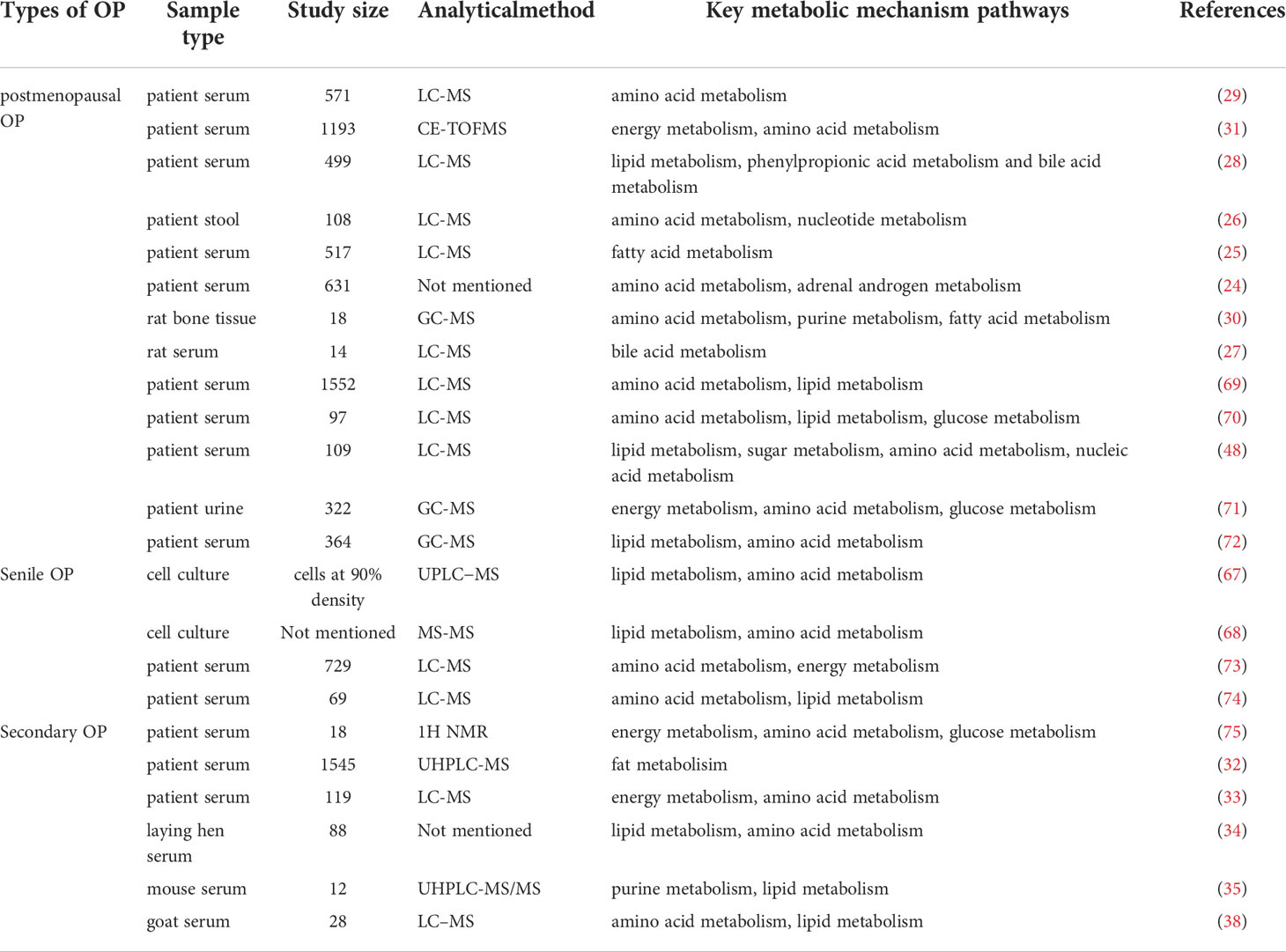
Table 1 The application of metabolomics in exploring the pathogenic mechanism of various types of OP.
4.1 Different types of metabolism
4.1.1 Amino acid metabolism
The metabolism of amino acids in the body includes two aspects. On the one hand, it is mainly used to synthesize proteins, polypeptides, and other nitrogen-containing substances unique to the body itself. In addition, amino acids can be decomposed into amines, α-keto acids, and carbon dioxide through a series of combined deamination, deamination, decarboxylation, and transamination effects. These carbon dioxide, alpha-keto acids and amines can be converted into lipids, non-essential amino acids, and sugars, and can also be oxidized to release energy using the tricarboxylic acid cycle, while producing water and carbon dioxide. Therefore, amino acid metabolism plays an important role in the pathogenesis of OP. Some studies have consistently demonstrated that some substances in amino acid metabolism might related with the pathogenesis of OP. Scientist studied in the metabolism of middle-aged Japanese women showed that lysine was correlated with the menopausal status of women, and increased gradually with the change of premenopausal, perimenopausal and postmenopausal (31). In addition, the effects of glucocorticoid-induced short-and long-term OP on lipids and plasma metabolites was investigated in ovariectomized sheep. Lysine was also found to distinguish between normal and low bone mineral density (BMD) groups (38). What’s more, a GC-MS analysis in metabolic profiles of postmenopausal OP progression in 364 Chinese women reflected the epochal changes of lysine in the pathogenesis of OP (72).
Novel metabolite changed in middle-aged Japanese women were studied. The study found that carnitine was associated with women’s menopausal status and gradually increased with the change of menopause (31). The metabolites and metabolic pathways associated with changes in BMD in postmenopausal and perimenopausal women with OP was systematically investigated. Carnitine significantly affects changes in BMD (25). In addition, patient serum samples are efficiently analyzed by metabolomics using untargeted MS. The study found that compared with the control group, the carnitine content of the OP group was significantly imbalanced (74). These studies would help to establish that the pathogenic mechanism of healthy bones and OP was closely related to metabolite carnitine.
Metabolomics techniques were used to discover differences in metabolites of bone metabolic disorders between healthy volunteers and osteoporotic patients. Abnormal metabolism of valine might serve as a key mechanism of OP (31, 38, 75). The relationship between OP and amino acid metabolism was further explored. The results of the study indicate that the change of the metabolite glutamate may play an important role in the pathogenesis of OP (26, 36, 70).
In addition, some recent studies have also suggested that the following amino acids may be closely related to the pathogenesis of OP, including: alanine (30, 36, 75), tryptophan (34, 38, 72), methionine (36, 38, 70), phosphatidylserine (67, 68), urea (28, 30), glycine (69, 73, 75), threonine (69, 70), leucine (69, 75), proline (31, 70, 75), aspartic acid (36, 75), hydroxyproline (31, 72), taurine (31, 71, 73), glutamine (31, 75), as shown in Table 2.
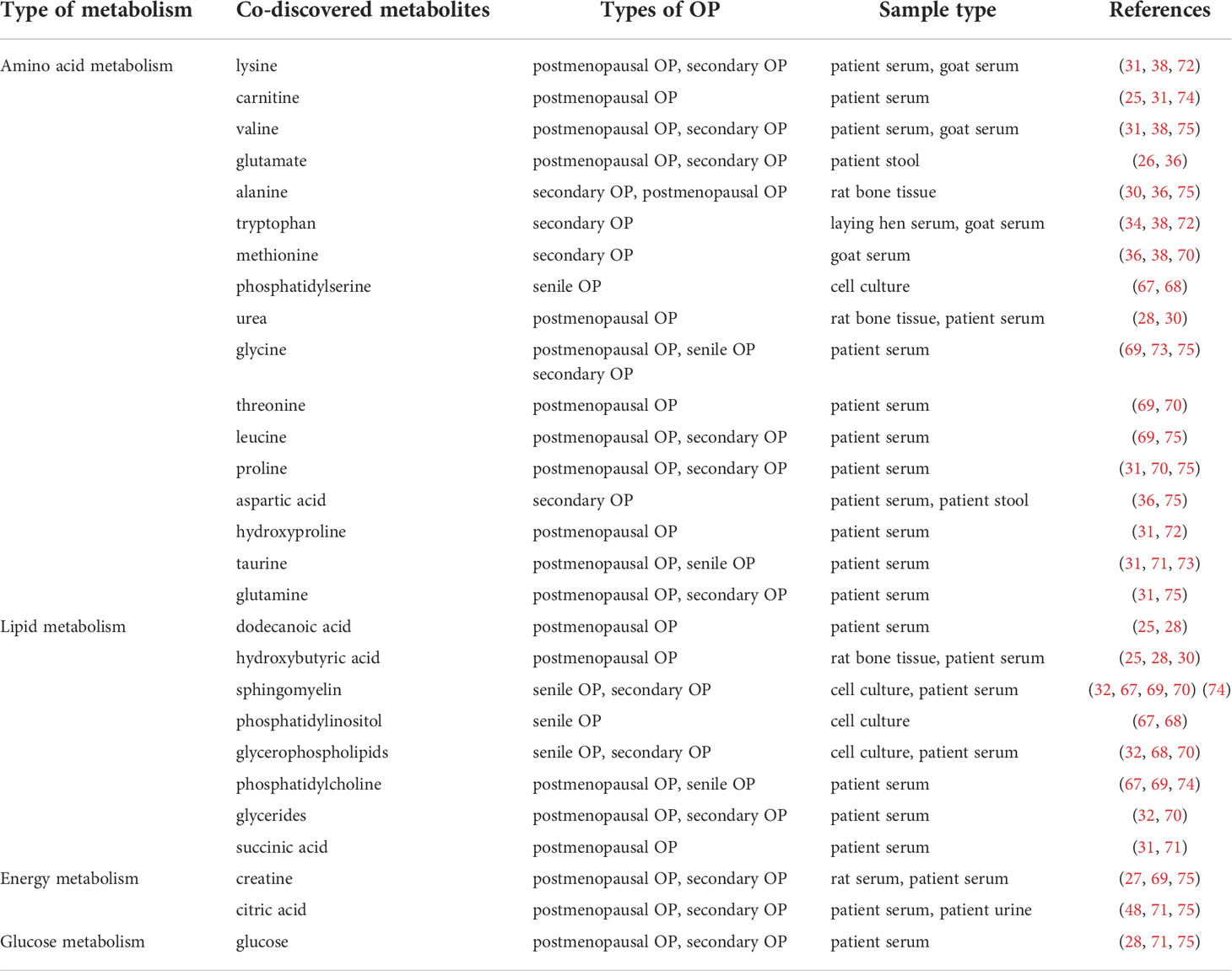
Table 2 Metabolomics is used to explore mechanisms of OP, with the same metabolites found in different studies.
4.1.2 Lipid metabolism
Lipids are an important material basis for maintaining cell function and cell proliferation. Several studies have found that some lipid metabolites have been found many times in various samples of OP, which might play an important role in the pathogenesis of OP.
Senescence-related lipid metabolism might play an important role in the abnormal differentiation of BMSCs. The declining trend of sphingomyelin describes lipid responses that might lead to abnormal differentiation of bone marrow-derived mesenchymal stem cells during aging (67). Mendelian randomization analysis showed that sphingomyelin was inversely associated with BMD (32). In Singaporean Chinese postmenopausal women, the association between blood lipids and femoral neck BMD was explored using metabolomics. There was a significant correlation between sphingomyelin and BMD reduction (69). In addition, other studies have also explored the association between BMD and sphingomyelin, indicating that sphingomyelin plays a key role in the pathogenesis of OP (70, 74).
Metabolomics with OP bone marrow and bone also showed that hydroxybutyric acid biosynthesis was disturbed. Assessment of differential metabolites improves understanding of metabolic relationships between kidney-bone axis and tissues in ovariectomized rats. Using a metabolomic approach, serum samples from early menopausal and perimenopausal women were analyzed. These results suggested that hydroxybutyric acid might play a role in the mechanism of osteoporotic bone remodeling (25, 28, 30).
The lipidomic strategy was used to observe the expression of related enzymes and lipids in the membranes of MSCs of different ages and proliferation states. Several studies have found that the changes of glycerophospholipids are closely related to the metabolic function mechanism mediated by bone marrow mesenchymal stem cells (32, 68, 70). Serum samples were analyzed using an untargeted MS-based metabolomic approach. Phosphatidylcholine metabolites were significantly dysregulated in the OP group compared with the control group. This metabolome will contribute to the study of disease mechanisms that promote bone health and OP progression (67, 69, 74).
In addition, other lipid metabolites, such as Glycerides (32, 70), succinic acid (31, 71), dodecanoic acid (37, 40), phosphatidylinositol (67, 68), etc., have been proved by many studies to be closely related to the pathogenesis of OP, as shown in Table 2.
4.1.3 Other metabolism
In recent years, the pathogenesis of OP has been continuously explored by means of metabolomics, and some key metabolites of energy metabolism and glucose metabolism also play an important role in the mechanism of OP.
A postmenopausal OP mouse model was used to compare metabolome changes in the control and OP groups. Metabolites creatine was significantly different (27). The pathogenesis of OP is revealed from the perspective of microbe-gut-metabolic bone axis regulation, which provided a new entry point for the pathogenesis of OP. OP-related metabolomic markers were examined to reveal underlying mechanisms of OP. Creatine has changed significantly (69). In addition, metabolomic techniques were used to discover differences in metabolites of bone metabolic disorders between healthy volunteers and diabetic patients. Changes in creatine levels were also found (75). Therefore, the metabolic abnormalities of creatine might serve as a key substance in the underlying pathogenic mechanism of OP.
Metabolites with significant differences between estrogen levels and BMD. Metabolite citric acid changes were useful markers of bone loss and/or estrogen deficiency (48). Metabolomics techniques were used to discover differences in metabolites of bone metabolic disorders between healthy volunteers and diabetic patients. Citric acid level was also significantly changed (75). This metabolite abnormality could be used as a key indicator of the pathogenesis of diabetic OP. Pathological features of postmenopausal OP were revealed, and metabolic pathways and biomarkers that might be associated with OP were explored. citric acid was also found to be a potential biomarker of OP (71). Therefore, citric acid was related to the pathogenesis of OP.
Using metabolomic profiling methods, metabolic alterations in postmenopausal women and elderly OP compared with healthy people were analyzed. These studies all found that glucose played a role in the mechanism of osteoporotic bone remodeling (28, 71, 75).
OP is a classic age-related disease that is often considered a “silent disease” because there are no symptoms for many years before a fracture occurs. Therefore, it is of great practical significance to deeply study the pathogenic mechanism of OP from the perspective of metabolism, which can further promote the early prevention, diagnosis, and intervention of OP from the perspective of mechanism (1–3). To sum up, many studies have shown that OP will experience various metabolite changes in various stages of the disease, including amino acid metabolism, lipid metabolism and energy metabolism (40, 69, 71). These different studies all found some of the same metabolites for the above metabolic pathways. Therefore, these same metabolites played important roles in the pathogenesis of OP, and future monitoring of changes in these metabolites by metabolomics is important to achieve further research in OP.
4.2 Factors associated with the metabolomic outcome of OP
OP is a heterogeneous disease. Therefore, vitamin D, diabetes, race, and other factors should be considered when studying OP using metabolomic approaches. Vitamin D inhibits osteoclast recruitment, prevents estrogen deficiency, and enhances osteoblast precursor cell proliferation and osteoblast activity (76). A series of studies found that vitamin D levels can significantly alter amino acids, energy metabolism, levels of sugars and their derivatives, and organic acids in patients with OP, thus affecting the metabolomic outcome of OP (77, 78). In addition, different blood calcium levels affected the metabolism of lipids and amino acids such as taurine, glycerophospholipids and glycine, thus causing changes in the metabolomic outcome of OP, which ultimately affected BMD and bone degeneration (79, 80). Recent studies have found that temperature increases the total amount of polyamines in the body, and that inhibition of polyamine biosynthesis in the body limited the protective effect on bone (81).
Severe metabolic disturbances in diabetes can lead to OP. Findings suggest that diabetes mellitus combined with OP will further lead to significant changes in amino acid metabolism and energy metabolism, such as tricarboxylic acid cycle products and various branched-chain amino acids (75). OP combined with osteoarthritis will further alter the phospholipid precursors, energy metabolism and amino acid metabolites of OP (82). In addition, lipoprotein and amino acid metabolites are significantly different when OP is associated with atherosclerosis (83).
Ethnic differences are also important factors influencing metabolomic outcomes in OP. The association between lipid and amino acid metabolites and BMD changes was significant in Asian women with OP in China and Singapore. In particular, dodecanoic acid played an important role in metabolites (25, 70). However, TwinsUK-based studies have mainly identified amino acid and hormone metabolites and found a causal relationship between them and BMD. Lipid metabolites and other amino acid metabolites were different from those in Asians (24). In addition, these studies have shown that the severity of OP varies among ethnic groups. Therefore, the changes in the levels of osteoporotic metabolites and the occurrence and development of OP explored by metabolomics are different based on different races (24, 25, 70).
As a secondary OP, OP caused by intestinal flora has attracted more and more attention in recent years. The microorganisms in the gastrointestinal tract are collectively referred to as the gut flora and consist of approximately 10 trillion bacteria (84). Recent studies have provided substantial evidence for the existence of a “gut microbiota-metabolite-bone axis”, and OP is closely associated with the development and progression of gut microbiota imbalances (26, 27, 35, 85–87). He et al (26) combined LC-MS metabolomics with 16S rRNA gene sequencing. The results showed that mutations in gut bacteria such as Klebsiella and Clostridium interfered with changes in the metabolic levels of acetylmannosamine, type I collagen C-terminal peptide and collagenogenic peptide, and mediated postmenopausal bone loss. Other studies have found that OP was associated with the functional, taxonomic and β-diversity composition of the gut microbiota. Oscillating bacteria, Brucella, Actinomyces and other intestinal flora acted mainly on the metabolism of tryptophan and tyrosine and the degradation of isoleucine, leucine and valine, thus negatively regulating BMD in OP (86). Wen et al. (27) found that the onset and progression of OP is closely related to the metabolic regulation of the intestinal flora. The gut microbiota is one of the important pathogenic factors of OP and regulates the pathogenesis of OP through the microbial-gut metabolic-bone axis. Liu et al. (85) found that the effect of ethanol intake on the gut microbiota mainly increased the ratio of firmicutes and Bacteroidetes, which led to an increase in 5-hydroxytryptamine and inhibited the mineralization and proliferation of osteoblast-associated cells, thus affecting BMD. In addition, high lipids led to a significant increase in the relative abundance of bacteria, with a decrease in B. phenotypicus, B. actinomycetemcomitans and the bacteria of lipolysis and purine metabolism, which decrease the BMD (35). In addition, bone loss induced by salivary microbiota through the “oral-gut axis” in patients with periodontitis may be related to tryptophan metabolism and lipid degradation (88). These studies will contribute to a better understanding of the mechanisms and relationships between changes in osteoporotic metabolite levels and the gut microbiota, and how the gut microbiota is involved in and mediates the development and progression of OP, making gut microbiota regulation a new therapeutic strategy to promote healthy bone development.
Therefore, vitamin D, blood calcium, temperature, race, diabetes, osteoarthritis, atherosclerosis, gut microbiota and other factors both influence the metabolomic outcome of OP. In the future, it will be a meaningful research direction to further pay attention to and integrate various factors such as age, BMI, smoking and physical activity to explore the changes of metabolomics in OP.
5. Metabolomics for the development of OP therapeutics
At present, the therapeutic mechanism of mature OP drugs such as alendronate sodium, teriparatide, calcitriol, etc. and the therapeutic effect of biomaterials have been systematically and deeply studied. There are also many studies and reviews summarized these drugs. In this review, we systematically summarized the OP treatment drugs that have been studied more in recent years, especially natural herbal medicine (Figure 2), and related extracts (Table 3), which have been found both protect bone from osteoporosis, but the mechanism need to be further explored. The introduction of metabolomics provides a good platform for the study of these drugs in regulating the biochemical metabolism of bone tissue, and can further explore the side effects, efficacy, and dose effect of their therapeutic methods. We provide a series of novel OP treatments to be developed and even laid a solid foundation for clinical transformation.
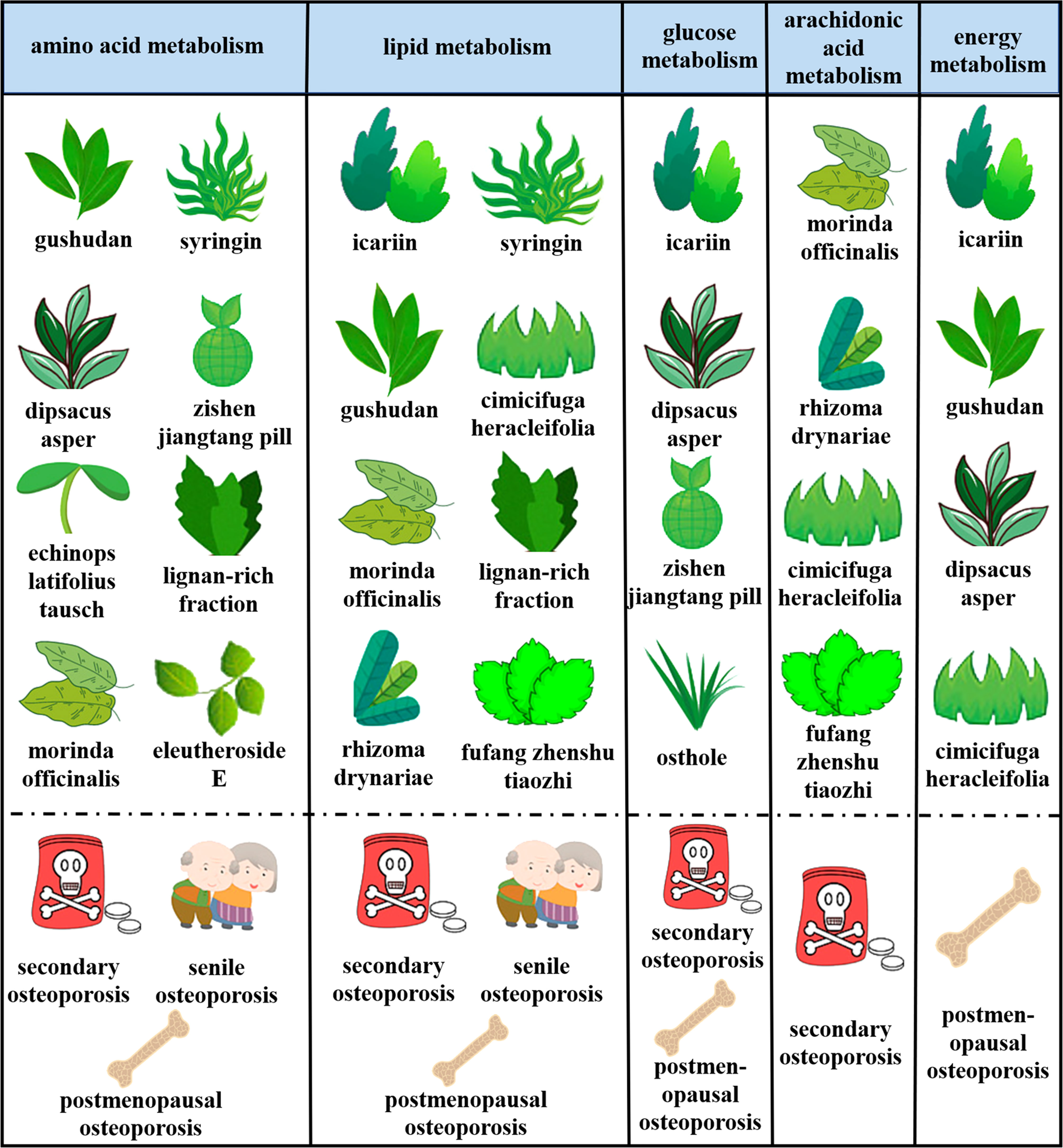
Figure 2 Metabolic pathways of different types of natural herbal action and therapeutic effects on different types of OP.
5.1 Natural herbal medicine
5.1.1 Natural compound herbal medicine
XianLingGuBaoJiaoNang was used to prevent and treat OP. However, there was no comprehensive metabolic profile of XianLingGuBaoJiaoNang. The results showed that cleavage and deglycosylation of glycosylated groups were the main metabolic pathways of various glycosides. Notably, amino acid binding was first found in the metabolism of pentene-flavonoid glycosides in the intestinal flora of rats (117).
The mechanism of Zishen Jiangtang Pill maintaining blood glucose and BMD is still unclear. These results indicate that Zishen Jiangtang Pill could effectively improve abnormal bone metabolism and glucose metabolism in diabetic OP, and was expected to be an effective alternative drug for the treatment of diabetic OP (101). Fufang Zhenshu Tiaozhi could treat hyperlipidemia and OP caused by glucocorticoid. Fufang Zhenshu Tiaozhi had a protective effect on senile OP, and its mechanism might be related to the interference of arachidonic acid metabolism, glycerophospholipin and sphingomyelin (104).
Xie et al (107) studied the effect of QingEWan on intestinal microflora in rats with OP. The levels of butyric acid, propionic acid and acetic acid were increased. In addition, QingEWan could regulate intestinal flora and improve OP.
5.1.2 Natural single herbal medicine
Gushudan is a kind of traditional Chinese medicine preparation designed for secondary OP. Yuan et al (93) discussed the anti-OP effect of Gushudan on hormone-induced OP rats and its mechanism, and identified 40 different metabolites, mainly involving energy metabolism, amino acid metabolism, intestinal flora metabolism and fat metabolism. Using UPLC-MS technology of metabolomics, the overall therapeutic effect of Gushudan on secondary OP was effectively explored by detecting urine blood samples (94). By 1H NMR metabolomics method, 27 differential metabolites were found after Gushudan treatment. It was further proved that Gushudan may ultimately treat OP by changing these metabolites (95). Through a non-targeted metabolomic approach, Gushudan was further used to explore the therapeutic effect and related mechanism of secondary OP. The results showed that energy, fat, and amino acid metabolism play a huge role in this pathway (96). These correlation studies have systematically explored the therapeutic effect and metabolic mechanism of Gushudan. Metabolomics was also used to explore the mechanism of OP according to the above section. Several studies simultaneously found that the pathogenesis of OP is closely related to lipid metabolism, amino acid metabolism and energy metabolism. We summarized some key and jointly validated metabolites related with Gushudan in the Table 3. In combination with the regulation of Gushudan on metabolites of OP, we found that Gushudan significantly regulated taurine, creatine, Valine, tryptophan, and Leucine metabolites of OP (Table 3).
Tao et al (97) found that the Dipsacus asper treatment group had abnormal metabolic pathways. Dipsacus asper segment of liquor could treat and prevent OP by intervening energy metabolism, carbohydrate metabolism and amino acid metabolism in the body.
Wang et al (98) discussed the effects of Echinops latifolius Tausch on ovariectomized rats and the metabolic pathways involved in the changes in trabecular microstructure in OP. Echinops latifolius Tausch effected on bone trabecular microstructure of castrated rats may be related to intervention of glycerophospholipids.
Morinda officinalis and its chemical constituents could prevent OP caused by aging and estrogen deficiency. Metabolomics analysis showed that 37 different metabolites were present in the control group compared with the dexamethasone group, and 20 of them were significantly reversed after treatment with Morinda officinalis. Further Western blot analysis and metabolic pathway enrichment showed that Morinda officinalis prevented bone loss mainly through interference with arachidonic acid metabolism (99).
The mechanism of Rhizoma Drynariae anti-OP was still unclear. Using metabolomics, some potential biomarkers involving nine metabolic pathways were identified. These experimental results showed that Rhizoma Drynariae can prevent and treat OP by regulating the above-mentioned metabolic pathways, and provided a new theoretical basis for natural herbal medicines (100).
Cimicifuga heracleifolia was a traditional American herb that promises to counteract the ills of menopause. Serum metabolite composition was analyzed by serum metabolomics. The results showed that Cimicifuga heracleifolia has the effect of lowering blood lipid and anti-OP on climacteric syndrome. At the same time, its potential in improving metabolic disorders caused by postmenopausal OP was found (102).
Radices rehmanniae or dry Radices rehmanniae could prevent postmenopausal OP and senile OP. In metabolomics studies, 10 cases were significantly reversed after Radices rehmanniae treatment. These metabolites were mainly involved in amino acid metabolism, sex hormone regulation and steroid hormone biosynthesis. The mechanism of Radices rehmanniae action might be related to steroid hormone biosynthesis (108).
Rubus coreanus Vinegar had a good effect on postmenopausal OP. Of note, the Rubus coreanus Vinegar group had slightly increased levels of tryptophan, phenylalanine, lysophosphatidylcholine, glucose, and butyric acid compared with the postmenopausal OP group. Rubus coreanus Vinegar might be an effective natural substitute for prevention of postmenopausal OP (115).
5.1.3 Natural herbal medicinal extracts
Icariin, the main component of icariin flavonoid glycoside, has been widely confirmed to have anti-OP effect. Some studies combined 1H NMR metabolomics and proteomics, and elucidated 8 metabolites in serum and 23 proteins in femur which were significantly changed (89). After a single oral administration of Epimedium, Cheng et al (90) determined the metabolites in rat urine, plasma, feces, and bile. The results also showed that the main metabolic pathways of icariin in rats were glycosylation and glycoaldehyde acidification after glycosylation. Pan et al (91) systematically analyzed the metabolomics characteristics of glucocorticoid-induced OP model rats. Huang et al (92) discussed the therapeutic effect and mechanism of icariin on low bone density in cage laying hens. Icariin mainly interfered with fat metabolism, taurine metabolism and pyrimidine metabolism of laying hens, resulting in increased BMD in old laying hens. Cobined these study of metabolomics applied to OP, we found that alanine, creatine, Taurine, Glycine, and β-glucose metabolites of the pathogenesis of OP were significantly regulated (Table 3).
Syringin had strong anti-OP activity, but the specific mechanism of its anti-OP was still unclear. High resolution mass spectrometry (MS) showed that metabolic pathways were closely related to catecholamine biosynthesis, butyric acid metabolism, glycine, tyrosine, methionine, and serine metabolism (52).
The part of Lignan-rich fraction in lignan was a traditional Chinese medicine used to treat bone diseases in China. Studies were conducted to identify potentially related metabolic pathways and metabolites. Studies have shown that Lignan-rich fraction can effectively restore amino acid-related tryptophan metabolism, lipids, and antioxidant systems (103).
Si et al (105) discussed the efficacy of osthole treatment. In the ovariectomized OP model, 28 metabolites were identified as biomarkers, some of which had significant regulatory effects.
In a study, the interventional effect of Eleutheroside E on postmenopausal OP was evaluated by analyzing the related metabolic network, potential biomarkers, and urinary metabolic profile of postmenopausal osteoporotic rats. This study explained the metabolic effects and pharmacological mechanisms of Eleutheroside E on postmenopausal OP (106).
Some studies have shown that Achyranthes polysaccharides can treat OP through various ways. This study evaluated the effect of Achyranthes bidentata polysaccharides on OP based on metabolomics analysis. Achyranthes bidentata polysaccharides had good potential in the treatment of OP (50). Metabolomics highly integrates the “top-down” integration strategy, and responds to various functional pathways and indicators through changes in metabolic pathways, networks, and end products, to understand the overall trend of system change. Therefore, using metabolomics methods, natural herbal formulations and extracts have received more extensive research and attention.
5.2 Hormone drugs
Estradiol is the main clinical drug for OP treatment. Wei et al. discussed metabolic changes in myogenic OP and the therapeutic effects of estradiol. The analysis showed that the changes of oxidative phosphorylation, tryptophan metabolism, glycerol phospholipid metabolism, thermogenesis, histidinine metabolism, arginine biosynthesis and purine metabolism were the most common pathogenic factors of myogenic OP (110). Liu et al. studied the response of osteoclast metabolites to estradiol using a metabolomics-based approach (111). Some 27 metabolites such as amino acids and lipid derivatives were significantly altered after estrogen action. The metabolomic pathway enrichment analysis showed that estrogen affects glycerophospholipid metabolism and played a therapeutic role in OP. Estradiol-induced changed in phosphatidylcholine-sterol acyltransferase activity, methyldialdehyde and malondialdehyde further affected glycerophospholipid metabolism. Studies have shown that estradiol is highly conditioned dependent on osteoclast metabolism.
5.3 Gut microbes
So far, fecal microbiota transplantation and probiotic supplementation have gradually attracted the attention of scholars as a new organ transplantation method in the alleviation and even treatment of OP. This approach aims to alter the abundance and composition of gut microbes in the recipient’s gut, thus affecting the metabolite levels in the body for the treatment of OP (118). Zhang et al (119) showed that gut microbiota treatment increased the levels of propionic and acetic acids, optimized the abundance and composition of the gut microbiota, inhibited the production of excess osteoclasts, and prevented bone loss in postmenopausal osteoporotic rats. Lactobacillus reassorts intestinal flora and alters metabolite composition, particularly lysophosphatidylcholine levels. Lactobacillus might be an effective and safe treatment strategy in some types of osteoporotic diseases (112). Lactic acid bacteria significantly reduced bone loss in older women with low bone density. Lactobacillus-regulated metabolites are involved in a variety of metabolic pathways, including acylcarnitine, peptide and lipid metabolism, as well as amino acid metabolism (113).
In addition, various drugs and bioactive substances could indirectly treat OP by directly modulating the abundance and composition of the intestinal microbiota. Calcium supplementation could increase the number of propionibacteria and immobile bacilli in the feces, thus affecting the concentration of short-chain fatty acids. Inulin could significantly increase the number of bifidobacteria and cocci, acting on the production of single-chain fatty acids and ultimately improving the mechanical strength, bone mineral content and BMD of the femur (120). lignan-rich induced high abundance of actinomycetes and restores microbial composition, which reduced abnormal lipid metabolism, prevents glucose tolerance, improves liver function, and reduced the risk of OP in castrated rats (121). Gushudan promoted the production of lactobacilli, which in turn acted on the production of lysine, acetate, and butyrate, ultimately acting as an anti-OP agent (122). Temperature exposure could reduce rumen bacteria and digestive cocci and increase lactic acid bacteria, lactobacilli and Lybacilaceae, thereby leading to changes in spermidine, spermine and polyamine levels and increasing bone strength (81). Qinga pill could change the composition of Firmicutes, Verumobacteria and Bacteroides in intestinal flora, and increase the content of butyric acid, propionic acid, and acetic acid in intestinal flora. The combination of anti-OP drugs and gut microbiota might be a new treatment for OP (107). In addition, Achyranthes achyranthes could regulate the levels of polyunsaturated fatty acids, lipids, glucose, and amino acids by acting on Escherichia coli, Roche, and anaerobic bacteria, thus exerting an anti-OP effect (123).
5.4 Other treatment strategies
Mao et al (80) investigated whether calcium supplementation can reduce bone loss in rats caused by calcium restriction and estrogen deficiency. The results of metabolomics analysis suggested that calcium supplementation was a metabolic pathway closely related to glycerophospholipid metabolism, and that the effect of calcium supplementation on OP might be due to increased estrogen levels, resulting in changes in metabolite levels, and ultimately increased BMD, thereby reducing bone degeneration.
In a population of postmenopausal women with OP, the effect of tocotrienols on metabolites was assessed using patient serum systems. When treated with tocotrienols, oxidative stress and inhibition of inflammation were significantly modulated resulting in a significant reduction in bone loss in patients (114).
Wang et al (116) discussed the efficacy of bone marrow mesenchymal stem cells in the treatment of OP in ovariectomized mice. Stem cell therapy could be intertransformed by glucuronic acid and pentose, metabase and taurine metabolism, and arachidonic acid metabolism. This study laied a foundation for the study of bone marrow mesenchymal stem cells as a treatment strategy for OP.
Chondroitin sulfate calcium complex was considered to have in vitro bone health activity. It was found that intervention with calcium chondroitin sulfate could alter fecal metabolite composition and intestinal microflora of castrated rats. Correlation analysis showed that certain intestinal flora was significantly associated with metabolite-rich and OP phenotypes (109).
As mentioned above, metabolomics has made a lot of progress in developing new treatments for OP. From the perspective of biochemical metabolism mechanism, in-depth research has been conducted on how various drugs such as Chinese herbal medicine, polysaccharides, hormones, and Lactobacillus act on metabolic reprogramming of the body and play a therapeutic role in OP (52, 89, 110, 113). Among a variety of Chinese herbal medicines, studies on the regulation of icariin and Gushudan on various OP metabolism are comprehensive and in-depth, involving fat metabolism, sugar metabolism, amino acid metabolism, pyrimidine metabolism, taurine metabolism and intestinal microflora disorders, etc (89, 93). Notably, we found that metabolites of the pathogenesis of OP, including Taurine, creatine, Valine, Tryptophan, Leucine, Alanine, creatine, Taurine, Glycine, and β-glucose were significantly regulated by icariin (90, 92). Therefore, further research on the therapeutic mechanisms of these two drugs should be more attached for clinical application.
6. Application of metabolomics in other researches of OP
The imbalance of bone resorption and bone formation caused by osteoclasts relatively active, leading to OP and accompanied by various metabolic disorders (124, 125). Therefore, specific changes of markers in various samples such as blood, tissue, and urine of patients with OP will reflect the characteristics of metabolic disorders of bone tissue, which can support the prevention and prediction of the disease (126, 127). Subtle changes in metabolites can be revealed by metabolomics, but these changes have not yet resulted in changes in bone density or structure. Furthermore, substances produced as the end products of metabolic activity are factors related to biological or metabolic states. Therefore, these specific metabolic markers are highly sensitive markers for the prevention and prediction of OP specific pathologic states.
6.1 Prevention
It is extremely important to explore some risk factors that reflect abnormal bone metabolism, and they can be used for early prevention of OP. For example, nutrition is closely related to BMD values in children and adulthood, therefore, rational nutritional intervention and treatment are crucial for the prevention of OP, which can further reduce the risk of osteoporotic fractures. Mangano et al (128) used an untargeted metabolomics approach to determine the biochemical factors driving the relationship between vegetable and fruit intake and the risk of OP. Vegetables and fruits can inhibit the synthesis pathway of lipid metabolites, and lead to increased concentrations of other metabolites in the body, thereby stimulating estrogen synthesis and slowing the progression of OP. Dietary prevention strategies with adequate intake of dark green leafy vegetables, berries, and melons are associated with significant improvements in OP development and progression in both men and women. Chau et al (129) studied metabolites associated with coffee and assessed their association with OP. The results showed that 12 serum metabolites were positively correlated with coffee intake, among which fenugreek, 3-hydroxypyridine sulfate and quinic acid had the strongest correlation. Of these metabolites, 11 were known to be involved in coffee intake, and six of them were involved in caffeine metabolism. In addition, explosion to some metals and heavy metals may also lead to bone metabolism disorders (130). 1 μM cadmium significantly affected the malate-aspartate and citric acid cycles, and 10 μM cadmium significantly affected the pyrimidine, alanine, glutamate, glucose-alanine, and citric acid cycles.
6.2 Prediction
Predicting OP is critical for people to maintain bone health and improve their overall quality of life. Existing series of risk factors are difficult to predict complicated OP risk. In recent years, through metabolomics technology, some studies have found that several types of metabolites can be used as potential predictive markers of OP. Kong et al (131) conducted a survey with an average follow-up of 9 years. In a community cohort study, high spermidine concentrations were associated with an increased risk of osteoporotic fractures. With further validation studies, spermidine baseline concentration may be a new alternative marker for OP associated brittle fractures. Therefore, spermidine and its related metabolites may be reliable predictors of OP. Untargeted metabolomics analysis was performed on serum samples from 32 normal controls and 32 patients with OP. Hyocholic acids plays an important role in the development of OP and may be a potential marker. Hyocholic acids may be a new target for predicting OP (132). OP is a chronic disease that manifests insidiously and is age-related, often not detected until after a fracture. Therefore, some studies have established a sensitive, accurate, and rapid predictive test method, and the related aminobutyric acid enantiomers and isomers are accurately detected and used to predict the progression of OP (133). Serum (R) -3-aminoiso-butyric acid and γ -aminobutyric acid were positively correlated with physical activity in young, lean women. This study opens new possibilities for aminobutyric acid as a potential predictor of OP.
7.Technological innovations in metabolomics and multi-omics integration to explore OP
With the rapid development of metabolomics in the field of OP, a series of traditional testing and analysis techniques and methods have drawbacks. Therefore, in recent years, technological innovations have been made in many aspects of metabolomics in the process of exploring OP diseases, and certain progress has been made. Furthermore, OP is a multi-factorial disease. Therefore, it is of great practical significance for OP to integrate metabolomics and multi-omics data for a comprehensive and systematic exploration. Wang et al (134) proposed a simple method to correlate the relative retention time of peaks in chromatograms with the intrinsic peaks and to assess their off-target performance using an LC-MS dataset obtained from plasma samples of rats with OP. The relative retention time method have fewer missing values, low peak intensity relative standard deviation, and good pattern recognition performance, which showed great potential in future metabolomics research. To improve the interpretability of the multiregional orthogonal projection model, they integrated targeted analyses of oxygen lipids, metabolomics, fatty acids, sphingolipids, and transcriptome. Clinical closure was also used for analysis. They identified OP genes associated with dysregulation of inhaled glucocorticoid metabolites, providing insights into the mechanism of BMD loss in asthma patients taking glucocorticoids. These results suggested that a combination of multi-block associative variable selection with multi-block orthogonal projection and interactive visualization techniques could generate hypotheses from multi-omics studies and inform biology (135). Yier et al (51) studied the anti-OP effects of oleanolic acid and used metabolomics methods to predict the mechanism of action. Oleanolic acid and methionine, cysteine metabolism, isoleucine, valine, phenylalanine, tryptophan, leucine and tyrosine biosynthesis, linoleic acid metabolism and other metabolic pathways were significantly affected. Using the new analytical platform, they will further understand the relationship between the therapeutic effect of oleanolic acid in improving OP and glucocorticoid-induced lipid metabolism, molecular transport, and metabolic changes in rats with dysglycemia.
The combination of metabolomic and metallomic methods to study OP is also one of the research hotspots in recent years. Tao et al (136) developed metabolomic and metallomic methods to explain the biochemical basis of the anti-OP effects of salt and raw achyranthes. Iron, manganese, zinc, glycine, ammonia cycle, alanine metabolism, arginine, galactose metabolism, copper, selenium, serine metabolism, lactose degradation, proline metabolism and urea cycle were increased. The combination of metabolomics and metallomics with pattern recognition and enrichment analysis of metabolites provided a useful tool for revealing the mechanism of action of traditional Chinese medicine. As a Chinese medicine prescription for clinical treatment of OP, it had the function of improving renal function and strengthening muscles and bones. Metabolic analysis identified 17 potential biomarkers associated with OP, including β -aminobutyric acid, glucose, arachidonic acid, and malic acid. Metallomic analysis showed that there were seven metal elements in rat kidney tissue: arsenic, iron, manganese, barium, molybdenum, selenium, and zinc. Metabolic pathways mainly included amino acid metabolism and glycolysis of the neurotransmitter. The combination of renal metabolomics and metallomics could effectively supplement the study of urine and blood metabolomics, which can not only effectively explore the pathogenesis of OP, but also explore the therapeutic mechanism of Gushudan on the disease (137).
Using bioinformatics methods, it was found that osteoblast differentiation was associated with an increased requirement for proline, and highlighted the strong demand of proline for osteoblast differentiation and bone formation (138). Kodriˇc et al. combined a variety of perspectives, including metabolomics, transcriptomics, proteomics, and genomics. The intersections were then analyzed to identify the common pathways or molecules that played an important role in OP prediction, prevention, diagnosis, or treatment (139). Combined with cell metabolomics and network biology analysis, fatty acid metabolism and galactose metabolism might be the main pathways affected by jujube side treatment (140). The pharmacological effects of naringin on OP remain unclear. Metabolomics analysis showed that 21 species were significantly regulated by naringin, including: pyruvate, amino acids, glycerophospholipids, polyunsaturated fatty acid metabolism, etc. Naringin was associated with changes in expression of 13 important protein targets by network predictive pharmacologic analysis. This revealed that the combination of network pharmacology and high-throughput metabolomics can further explore the metabolic mechanism (141). Heat exposure improves BMD and thus strength in castrated mice, primarily due to improved trabecular bone thickness, bone connection density, and bone volume. Comprehensive metabolomics and metagenomic analysis showed that temperature promoted bacterial polyamines biosynthesis, resulting in increased levels of total polyamines in vivo. The results of the study showed that the supplementation of spermidine enhanced bone density, and at the same time, the synthesis of polyamines in the body was inhibited (81). Tween-2 decoction is a Mongolian medicine for postmenopausal OP rats. Researchers combine untargeted metabolomics and network pharmacology and identified three key protein targets - hydroxysteroid dehydrogenase, cytochrome, and vitamin D receptors. Network pharmacology suggested that major changes in vitamin B6 metabolism were related to vitamin D receptor targets. Thus, Tween-2 decoction on postmenopausal OP rats might be related to down-regulation of vitamin D receptor (142).
In conclusion, the organic combination of metabolomics and bioinformatics, genetics, genomics, transcriptome, proteome, network biology, metagenomics, network pharmacology has made a lot of progress, realizing the systematic exploration of OP prevention, detection, and treatment (135, 137, 139, 141).
8. Challenges of clinical translation of metabolomics in OP research
Over the past decade, metabolomics has been increasingly used to identify biomarkers in disease and is currently considered a very powerful tool with great clinical translational potential (143). The development and utilization of metabolomics has enabled in-depth study of the metabolic characteristics of clinical disease, thereby optimizing disease mechanism exploration, prevention, prediction, and treatment monitoring. In theory, metabolomics can target metabolic therapy based on the metabolic dependence of OP to improve the specificity of clinical treatment (48, 49). From disease prediction to treatment, metabolomics opens new opportunities for comprehensive OP research. However, the clinical development and mass application of metabolomics still need to overcome some challenges and difficulties.
So far, non-targeted and targeted metabolomics have been widely used in OP disease mechanism and treatment research, especially natural Chinese herbal medicine. However, they need to overcome many obstacles and challenges before they can achieve clinical translation and widespread application (144). To overcome these drawbacks, a variety of complementary methods should be adopted to conduct metabolome research. At this time, more advanced instruments and platforms are required, which are difficult to achieve in both clinical and general laboratories. After obtaining a large amount of data, professional data processing and analysis software is often required, which requires certain professional skills of analysts, especially for non-targeted metabolomics. During data analysis, when the choice of the peak selection algorithm is changed, the data results will vary slightly. In addition, rational and rigorous experimental design is essential for analyzing large metabolomic datasets, which is also critical for the choice of statistical analysis (1, 57, 58). Therefore, targeting large-scale metabolomics research and clinical practice requires interdisciplinary collaboration and efforts of biologists, statisticians, and chemists. It is worth noting that, because metabolomics requires more high-end instrument platforms and specialized data processing algorithms, how to achieve standardization of clinical-level laboratory execution is crucial. In addition, the uniform standardization of institutional reporting and data analysis for metabolomics is another important challenge. Currently, most metabolomics studies produce relatively quantitative results. Absolute quantification is critical when normalizing across platforms. Previously, the Metabolomics Association had launched a standards initiative for a unified standard for metabolomics data communication. However, many published datasets still fall short of these criteria due to a lack of consensus among laboratories (145).
The results suggest that metabolomics can be used for prevention and diagnostic clinical treatment of OP. According to the results of a series of studies, these metabolites are indeed associated with OP. However, it remains to be determined whether these differential metabolites play a causal role in the pathogenic and pharmacological mechanisms of OP or are merely early manifestations of preclinical disease (57, 64). Therefore, patients with other diseases within 2 years of OP diagnosis should be excluded when exploring whether these differential metabolites play a direct causal role. In addition, the key point is that metabolomics generally uses plasma, urine, etc. of organisms for more overall evaluation and detection. At this time, it is difficult to distinguish between the metabolome changes caused by OP and those caused by other factors (146). Therefore, metabolomic analysis needs to effectively address these biological confounding effects in order to be better utilized in various studies of OP.
9. Conclusions
OP is a systemic metabolic disease. Metabolomics can effectively reveal the specific metabolic mechanisms of OP and the metabolic trajectories related to treatment response. In this review, the metabolomics of OP pathogenesis and metabolomics of natural herbal medicine are elaborated and summarized systematically. Some clinical translational studies have shown that metabolomics is a valuable tool to predict the therapeutic effect of osteoprotective agents and natural herbal medicine on OP recovery or to evaluate their side effects on normal bone function. In addition, metabolomics combined with gut microbiota studies have provided convincing evidence in the study of OP metabolism. In the future, the integration of gut microbiota and host may lead to more research breakthroughs and clinical application in the OP study. Therefore, metabolomics has good exploration value and clinical transformation prospect in many fields with many advantages in the study of OP.
However, the application of metabolomics in OP research still has some limitations. The multiple factors such as food intake, microbiota activity, the liver and muscle work together influence the levels of various metabolites. Therefore, which metabolites in urine, plasma, serum, bone tissue of OP patients can accurately reflect the development of OP in clinical application is still in the urgent exploration stage. In addition, the clinical transformation limitations of metabolomics are further reflected by the cellular heterogeneity. Thus, what metabolomics has in common with other omics approaches is that each technique alone does not capture a complete view of OP. Therefore, it might be helpful to combine metabolomics with other omics studies to further improve its selectivity and the effectiveness of clinical transformation. Further, the results of OP metabolomics may be affected by age, BMI, smoking, physical activity, gender, and other factors. At present, there is a lack of relevant targeted studies, and the extent and mechanism of the effects need to be clarified, which is a series direction for further investigation in the future. It is worth noting that the application of metabolomics technology in common clinical diseases is becoming more and more popular, but its application in the field of OP started late. At present, most of them stay in the stage of animal experiment, there are huge differences between animal experiment and clinical research, and there is still a long distance in clinical transformation.
Author contributions
MC and KY conceived and revised the manuscript. ZZ and ZC wrote the manuscript. AC participated in the literature search and related data sorting during the revision. In addition, all authors contributed to the article and approved the submitted version.
Funding
This work was supported by National Natural Science Foundation of China (82070902, 82272176), Shanghai Science and Technology Commission (19411963100), and Shanghai Jiao Tong University “Medical and Research” Program (ZH2018ZDA04).
Conflict of interest
The authors declare that the research was conducted in the absence of any commercial or financial relationships that could be construed as a potential conflict of interest.
Publisher’s note
All claims expressed in this article are solely those of the authors and do not necessarily represent those of their affiliated organizations, or those of the publisher, the editors and the reviewers. Any product that may be evaluated in this article, or claim that may be made by its manufacturer, is not guaranteed or endorsed by the publisher.
References
1. Yang TL, Shen H, Liu AQ, Dong SS, Zhang L, Deng FY, et al. A road map for understanding molecular and genetic determinants of osteoporosis. Nat Rev Endocrinol (2020) 16:91–103. doi: 10.1038/s41574-019-0282-7
2. Compston JE, McClung MR, Leslie WD. Osteoporosis. Lancet (2019) 393:364–76. doi: 10.1016/S0140-6736(18)32112-3
3. Noh JY, Yang Y, Jung H. Molecular mechanisms and emerging therapeutics for osteoporosis. Int J Mol Sci (2020) 21:7623. doi: 10.3390/ijms21207623
4. Khosla S, Hopbauer LC. Osteoporosis treatment: recent developments and ongoing challenges. Lancet Diabetes Endocrinol (2017) 5:898–907. doi: 10.1016/S2213-8587(17)30188-2
5. Curry SJ, Krist AH, Owens DK, Barry MJ, Caughey AB, Davidson KW, et al. Screening for osteoporosis to prevent fractures US preventive services task force recommendation statement. Jama-Journal Am Med Assoc (2018) 319:2521–31. doi: 10.1001/jama.2018.7498
6. Swank KR, Furness JE, Baker EA, Gehrke CK, Biebelhausen SP, Baker KC. Metabolomic profiling in the characterization of degenerative bone and joint diseases. Metabolites (2020) 10:223. doi: 10.3390/metabo10060223
7. Black DM, Rosen CJ. Clinical practice. postmenopausal osteoporosis. New Engl J Med (2016) 374:254–62. doi: 10.1056/NEJMcp1513724
8. Miller PD. Management of severe osteoporosis. Expert Opin pharmacotherapy (2016) 17:473–88. doi: 10.1517/14656566.2016.1124856
9. Kerschan-Schindl K. Prevention and rehabilitation of osteoporosis. Wiener medizinische Wochenschrift (1946) (2016) 166:22–7. doi: 10.1007/s10354-015-0417-y
10. Dominguez LJ, Farruggia M, Veronese N, Barbagallo M. Vitamin d sources, metabolism, and deficiency: Available compounds and guidelines for its treatment. Metabolites (2021) 11:255. doi: 10.3390/metabo11040255
11. Chandra A, Rajawat J. Skeletal aging and osteoporosis: Mechanisms and therapeutics. Int J Mol Sci (2021) 22:3553. doi: 10.3390/ijms22073553
12. Marra M, Sammarco R, De Lorenzo A, Iellamo F, Siervo M, Pietrobelli A, et al. Assessment of body composition in health and disease using bioelectrical impedance analysis (BIA) and dual energy X-ray absorptiometry (DXA): A critical overview. Contrast media Mol Imaging (2019) 2019:3548284. doi: 10.1155/2019/3548284
13. Laskey MA. Dual-energy X-ray absorptiometry and body composition. Nutr (Burbank Los Angeles County Calif.) (1996) 12:45–51. doi: 10.1016/0899-9007(95)00017-8
14. Anthamatten A, Parish A. Clinical update on osteoporosis. J midwifery women's Health (2019) 64:265–75. doi: 10.1111/jmwh.12954
15. Arceo-Mendoza RM, Camacho PM. Postmenopausal osteoporosis: Latest guidelines. Endocrinol Metab Clinics North America (2021) 50:167–78. doi: 10.1016/j.ecl.2021.03.009
16. Watts NB. Postmenopausal osteoporosis: A clinical review. J women's Health (2002) (2018) 27:1093–6. doi: 10.1089/jwh.2017.6706
17. Rinschen MM, Ivanisevic J, Giera M, Siuzdak G. Identification of bioactive metabolites using activity metabolomics. Nat Rev Mol Cell Biol (2019) 20:353–67. doi: 10.1038/s41580-019-0108-4
18. Muthubharathi BC, Gowripriya T, Balamurugan K. Metabolomics: small molecules that matter more. Mol Omics (2021) 17:210–29. doi: 10.1039/D0MO00176G
19. Wishart DS. NMR metabolomics: A look ahead. J magnetic resonance (San Diego Calif. 1997) (2019) 306:155–61. doi: 10.1016/j.jmr.2019.07.013
20. Fan J, Jahed V, Klavins K. Metabolomics in bone research. Metabolites (2021) 11:343. doi: 10.3390/metabo11070434
21. Yang YJ, Kim DJ. An overview of the molecular mechanisms contributing to musculoskeletal disorders in chronic liver disease: Osteoporosis, sarcopenia, and osteoporotic sarcopenia. Int J Mol Sci (2021) 22:2604. doi: 10.3390/ijms22052604
22. Si J, Wang C, Zhang D, Wang B, Zhou Y. Osteopontin in bone metabolism and bone diseases. Med Sci monitor Int Med J Exp Clin Res (2020) 26:e919159. doi: 10.12659/MSM.919159
23. Suzuki A, Minamide M, Iwaya C, Ogata K, Iwata J. Role of metabolism in bone development and homeostasis. Int J Mol Sci (2020) 21:8992. doi: 10.3390/ijms21238992
24. Moayyeri A, Cheung CL, Tan KC, Morris JA, Cerani A, Mohney RP, et al. Metabolomic pathways to osteoporosis in middle-aged women: A genome-Metabolome-Wide mendelian randomization study. J Bone mineral Res Off J Am Soc Bone Mineral Res (2018) 33:643–50. doi: 10.1002/jbmr.3358
25. Gong R, Xiao HM, Zhang YH, Zhao Q, Su KJ, Lin X, et al. Identification and functional characterization of metabolites for bone mass in peri- and postmenopausal Chinese women. J Clin Endocrinol Metab (2021) 106:e3159–77. doi: 10.1210/clinem/dgab146
26. He J, Xu S, Zhang B, Xiao C, Chen Z, Si F, et al. Gut microbiota and metabolite alterations associated with reduced bone mineral density or bone metabolic indexes in postmenopausal osteoporosis. Aging (2020) 12:8583–604. doi: 10.18632/aging.103168
27. Wen K, Tao L, Tao Z, Meng Y, Zhou S, Chen J, et al. Fecal and serum metabolomic signatures and microbial community profiling of postmenopausal osteoporosis mice model. Front Cell infection Microbiol (2020) 10:535310. doi: 10.3389/fcimb.2020.535310
28. Greenbaum J, Lin X, Su KJ, Gong R, Shen H, Shen J, et al. Integration of the human gut microbiome and serum metabolome reveals novel biological factors involved in the regulation of bone mineral density. Front Cell infection Microbiol (2022) 12:853499. doi: 10.3389/fcimb.2022.853499
29. Miyamoto T, Hirayama A, Sato Y, Koboyashi T, Katsuyama E, Kanagawa H, et al. A serum metabolomics-based profile in low bone mineral density postmenopausal women. Bone (2017) 95:1–4. doi: 10.1016/j.bone.2016.10.027
30. Li X, Wang Y, Gao M, Bao B, Cao Y, Cheng F, et al. Metabolomics-driven of relationships among kidney, bone marrow and bone of rats with postmenopausal osteoporosis. Bone (2022) 156:116306. doi: 10.1016/j.bone.2021.116306
31. Watanabe K, Iida M, Harada S, Kato S, Kuwabara K, Kurihara A, et al. Metabolic profiling of charged metabolites in association with menopausal status in Japanese community-dwelling midlife women: Tsuruoka metabolomic cohort study. Maturitas (2022) 155:54–62. doi: 10.1016/j.maturitas.2021.10.004
32. Mishra BH, Mishra PP, Mononen N, Hilvo M, Sievänen H, Juonala M, et al. Uncovering the shared lipidomic markers of subclinical osteoporosis-atherosclerosis comorbidity: The young finns study. Bone (2021) 151:116030. doi: 10.1016/j.bone.2021.116030
33. Qiu C, Yu F, Su K, Zhao Q, Zhang L, Xu C, et al. Multi-omics data integration for identifying osteoporosis biomarkers and their biological interaction and causal mechanisms. iScience (2020) 23:100847. doi: 10.1016/j.isci.2020.100847
34. Ye J, Chi X, Wang J, Shen Z, Li S, Xu S. High fat induces activation of the tryptophan-ERK-CREB pathway and promotes bone absorption in cage layers. Poultry Sci (2021) 100:101149. doi: 10.1016/j.psj.2021.101149
35. Lu L, Tang M, Li J, Xie Y, Li Y, Xie J, et al. Gut microbiota and serum metabolic signatures of high-Fat-Induced bone loss in mice. Front Cell infection Microbiol (2021) 11:788576. doi: 10.3389/fcimb.2021.788576
36. Kang H, Guo Q, Dong Y, Peng R, Song K, Wang J, et al. Inhibition of MAT2A suppresses osteoclastogenesis and prevents ovariectomy-induced bone loss. FASEB J Off Publ Fed Am Societies Exp Biol (2022) 36:e22167. doi: 10.1096/fj.202101205RR
37. Baba M, Endoh M, Ma W, Toyama H, Hirayama A, Nishikawa K, et al. Folliculin regulates osteoclastogenesis through metabolic regulation. J Bone mineral Res Off J Am Soc Bone Mineral Res (2018) 33:1785–98. doi: 10.1002/jbmr.3477
38. Cabrera D, Kruger M, Wolber FM, Roy NC, Fraser K. Effects of short- and long-term glucocorticoid-induced osteoporosis on plasma metabolome and lipidome of ovariectomized sheep. BMC musculoskeletal Disord (2020) 21:349. doi: 10.1186/s12891-020-03362-7
39. Porter FD, Herman GE. Malformation syndromes caused by disorders of cholesterol synthesis. J Lipid Res (2011) 52:6–34. doi: 10.1194/jlr.R009548
40. Song Y, Liu J, Zhao K, Gao L, Zhao J. Cholesterol-induced toxicity: An integrated view of the role of cholesterol in multiple diseases. Cell Metab (2021) 33:1911–25. doi: 10.1016/j.cmet.2021.09.001
41. Christianson MS, Shen W. Osteoporosis prevention and management: nonpharmacologic and lifestyle options. Clin obstetrics gynecology (2013) 56:703–10. doi: 10.1097/GRF.0b013e3182a9d15a
42. Lee WC, Guntur AR, Long F, Rosen CJ. Energy metabolism of the osteoblast: Implications for osteoporosis. Endocrine Rev (2017) 38:255–66. doi: 10.1210/er.2017-00064
43. Wang Y, Deng P, Liu Y, Wu Y, Chen Y, Guo Y, et al. Alpha-ketoglutarate ameliorates age-related osteoporosis via regulating histone methylations. Nat Commun (2020) 11:5596. doi: 10.1038/s41467-020-19360-1
44. Burda P, Hochuli M. Hepatic glycogen storage disorders: what have we learned in recent years? Curr Opin Clin Nutr Metab Care (2015) 18:415–21. doi: 10.1097/MCO.0000000000000181
45. Turner RT, Maran A, Lotinun S, Hefferan T, Evans GL, Zhang M, et al. Animal models for osteoporosis. Rev endocrine Metab Disord (2001) 2:117–27. doi: 10.1023/A:1010067326811
46. Komori T. Animal models for osteoporosis. Eur J Pharmacol (2015) 759:287–94. doi: 10.1016/j.ejphar.2015.03.028
47. Zhang Z, Ren H, Shen G, Qiu T, Liang D, Yang Z, et al. Animal models for glucocorticoid-induced postmenopausal osteoporosis: An updated review. Biomedicine pharmacotherapy = Biomedecine pharmacotherapie (2016) 84:438–46. doi: 10.1016/j.biopha.2016.09.045
48. Miyamoto T, Hirayama A, Sato Y, Koboyashi T, Katsuyama E, Kanagawa H, et al. Metabolomics-based profiles predictive of low bone mass in menopausal women. Bone Rep (2018) 9:11–8. doi: 10.1016/j.bonr.2018.06.004
49. Lv H, Jiang F, Guan D, Lu C, Guo B, Chan C, et al. Metabolomics and its application in the development of discovering biomarkers for osteoporosis research. Int J Mol Sci (2016) 17:2018. doi: 10.3390/ijms17122018
50. Zhang M, Wang Y, Zhang Q, Wang C, Zhang D, Wan JB, et al. UPLC/Q-TOF-MS-based metabolomics study of the anti-osteoporosis effects of achyranthes bidentata polysaccharides in ovariectomized rats. Int J Biol macromolecules (2018) 112:433–41. doi: 10.1016/j.ijbiomac.2018.01.204
51. Xu Y, Chen S, Yu T, Qiao J, Sun G. High-throughput metabolomics investigates anti-osteoporosis activity of oleanolic acid via regulating metabolic networks using ultra-performance liquid chromatography coupled with mass spectrometry. Phytomedicine Int J phytotherapy phytopharmacology (2018) 51:68–76. doi: 10.1016/j.phymed.2018.09.235
52. Shi Z, Zou S, Shen Z, Luan F, Yan J. High-throughput metabolomics using UPLC/Q-TOF-MS coupled with multivariate data analysis reveals the effect and mechanism of syringin against ovariectomized osteoporosis. J chromatography B Analytical Technol Biomed Life Sci (2021) 1183:122957. doi: 10.1016/j.jchromb.2021.122957
53. Wishart DS. Emerging applications of metabolomics in drug discovery and precision medicine. Nat Rev Drug Discovery (2016) 15:473–84. doi: 10.1038/nrd.2016.32
54. Musilova J, Glatz Z. Metabolomics - basic concepts, strategies and methodologies. Chem Listy (2011) 105:745–51.
55. Alseekh S, Aharoni A, Brotman Y, Contrepois K, D'Auria J, J. Ewald CEJ, et al. Mass spectrometry-based metabolomics: a guide for annotation, quantification and best reporting practices. Nat Methods (2021) 18:747–56. doi: 10.1038/s41592-021-01197-1
56. Johnson CH, Ivanisevic J, Siuzdak G. Metabolomics: beyond biomarkers and towards mechanisms. Nat Rev Mol Cell Biol (2016) 17:451–9. doi: 10.1038/nrm.2016.25
57. Podgórska B, Wielogórska-Partyka M, Godzień J, Siemińska J, Ciborowski M, Szelachowska M, et al. Applications of metabolomics in calcium metabolism disorders in humans. Int J Mol Sci (2022) 23:10407. doi: 10.3390/ijms231810407
58. Yan M, Xu G. Current and future perspectives of functional metabolomics in disease studies-a review. Anal Chim Acta (2018) 1037:41–54. doi: 10.1016/j.aca.2018.04.006
59. You YS, Lin CY, Liang HJ, Lee SH, Tsai KS, Chiou JM, et al. Association between the metabolome and low bone mineral density in Taiwanese women determined by (1)H NMR spectroscopy. J Bone mineral Res Off J Am Soc Bone Mineral Res (2014) 29:212–22. doi: 10.1002/jbmr.2018
60. Wishart DS, Cheng LL, Copié V, Edison AS, Eghbalnia HR, Hoch JC, et al. NMR and metabolomics-a roadmap for the future. Metabolites (2022) 12:678. doi: 10.3390/metabo12080678
61. Ramautar R, de Jong GJ. Recent developments in liquid-phase separation techniques for metabolomics. Bioanalysis (2014) 6:1011–26. doi: 10.4155/bio.14.51
62. Chen CJ, Lee DY, Yu J, Lin YN, Lin TM. Recent advances in LC-MS-based metabolomics for clinical biomarker discovery. Mass spectrometry Rev (2022):e21785. doi: 10.1002/mas.21785
63. Chen Y, Li EM, Xu LY. Guide to metabolomics analysis: A bioinformatics workflow. Metabolites (2022) 12:357. doi: 10.3390/metabo12040357
64. D'Alessandro A, Giardina B, Gevi F, Timperio AM, Zolla L. Clinical metabolomics: the next stage of clinical biochemistry. Blood Transfusion (2012) 10:S19–24. doi: 10.2450/2012.005S
65. Li J, Chen X, Lu L, Yu X. The relationship between bone marrow adipose tissue and bone metabolism in postmenopausal osteoporosis. Cytokine Growth factor Rev (2020) 52:88–98. doi: 10.1016/j.cytogfr.2020.02.003
66. Armas LA, Recker RR. Pathophysiology of osteoporosis: new mechanistic insights. Endocrinol Metab Clinics North America (2012) 41:475–86. doi: 10.1016/j.ecl.2012.04.006
67. Lu X, Chen Y, Wang H, Bai Y, Zhao J, Zhang X, et al. Integrated lipidomics and transcriptomics characterization upon aging-related changes of lipid species and pathways in human bone marrow mesenchymal stem cells. J Proteome Res (2019) 18:2065–77. doi: 10.1021/acs.jproteome.8b00936
68. Kilpinen L, Tigistu-Sahle F, Oja S, Greco D, Parmar A, Saavalainen P, et al. Aging bone marrow mesenchymal stromal cells have altered membrane glycerophospholipid composition and functionality. J Lipid Res (2013) 54:622–35. doi: 10.1194/jlr.M030650
69. Zhang X, Xu H, Li GH, Long MT, Cheung CL, Vasan RS, et al. Metabolomics insights into osteoporosis through association with bone mineral density. J Bone mineral Res Off J Am Soc Bone Mineral Res (2021) 36:729–38. doi: 10.1002/jbmr.4240
70. Cabrera D, Kruger M, Wolber FM, Roy NC, Totman JJ, Henry CJ, et al. Association of plasma lipids and polar metabolites with low bone mineral density in Singaporean-Chinese menopausal women: A pilot study. Int J Environ Res Public Health (2018) 15:1045. doi: 10.3390/ijerph15051045
71. Yu L, Qi H, An G, Bao J, Ma B, Zhu J, et al. Association between metabolic profiles in urine and bone mineral density of pre- and postmenopausal Chinese women. Menopause (New York N.Y.) (2019) 26:94–102. doi: 10.1097/GME.0000000000001158
72. Qi H, Bao J, An G, Ouyang G, Zhang P, Wang C, et al. Association between the metabolome and bone mineral density in pre- and post-menopausal Chinese women using GC-MS. Mol Biosyst (2016) 12:2265–75. doi: 10.1039/C6MB00181E
73. Miyamoto K, Hirayama A, Sato Y, Ikeda S, Maruyama M, Soga T, et al. A metabolomic profile predictive of new osteoporosis or sarcopenia development. Metabolites (2021) 11:278. doi: 10.3390/metabo11050278
74. Aleidi SM, Alnehmi EA, Alshaker M, Masood A, Benabdelkamel H, Al-Ansari MM, et al. A distinctive human metabolomics alteration associated with osteopenic and osteoporotic patients. Metabolites (2021) 11:628. doi: 10.3390/metabo11090628
75. Liang WD, Huang PJ, Xiong LH, Zhou S, Ye RY, Liu JR, et al. Metabolomics and its application in the mechanism analysis on diabetic bone metabolic abnormality. Eur Rev Med Pharmacol Sci (2020) 24:9591–600. doi: 10.26355/eurrev_202009_23047
76. Erben RG, Mosekilde L, Thomsen JS, Weber K, Stahr K, Leyshon A, et al. Prevention of bone loss in ovariectomized rats by combined treatment with risedronate and 1alpha,25-dihydroxyvitamin D3. J Bone mineral Res Off J Am Soc Bone Mineral Res (2002) 17:1498–511. doi: 10.1359/jbmr.2002.17.8.1498
77. Elsayyad NME, Gomaa I, Salem MA, Amer R, El-Laithy HM. Efficient lung-targeted delivery of risedronate sodium/vitamin D3 conjugated PAMAM-G5 dendrimers for managing osteoporosis: Pharmacodynamics, molecular pathways and metabolomics considerations. Life Sci (2022) 309:121001. doi: 10.1016/j.lfs.2022.121001
78. Chen SY, Yu HT, Kao JP, Yang CC, Chiang SS, Mishchuk DO, et al. Consumption of vitamin D2 enhanced mushrooms is associated with improved bone health. J Nutr Biochem (2015) 26:696–703. doi: 10.1016/j.jnutbio.2015.01.006
79. Wang M, Yang X, Wang F, Li R, Ning H, Na L, et al. Calcium-deficiency assessment and biomarker identification by an integrated urinary metabonomics analysis. BMC Med (2013) 11:86. doi: 10.1186/1741-7015-11-86
80. Mao H, Wang W, Shi L, Chen C, Han C, Zhao J, et al. Metabolomics and physiological analysis of the effect of calcium supplements on reducing bone loss in ovariectomized rats by increasing estradiol levels. Nutr Metab (2021) 18:76. doi: 10.1186/s12986-021-00602-y
81. Chevalier C, Kieser S, Çolakoğlu M, Hadadi N, Brun J, Rigo D, et al. Warmth prevents bone loss through the gut microbiota. Cell Metab (2020) 32:575–590.e7. doi: 10.1016/j.cmet.2020.08.012
82. Pertusa C, Mifsut D, Morales JM, Tarín JJ, Cano A, Monleón D, et al. Metabolomic analysis of severe osteoarthritis in a Spanish population of women compared to healthy and osteoporotic subjects. Metabolites 12 (2022) 12:677. doi: 10.3390/metabo12080677
83. Värri M, Niskanen L, Tuomainen TP, Honkanen R, Kröger H, Tuppurainen MT. Metabolite profiling of osteoporosis and atherosclerosis in postmenopausal women: A cross-sectional study. Vasc Health Risk Manage (2020) 16:515–24. doi: 10.2147/VHRM.S279028
84. He Y, Chen Y. The potential mechanism of the microbiota-gut-bone axis in osteoporosis: a review. Osteoporosis Int J established as result cooperation between Eur Foundation Osteoporosis Natl Osteoporosis Foundation USA (2022). doi: 10.1007/s00198-022-06557-x
85. Liu Z, Xu X, Shen Y, Hao Y, Cui W, Li W, et al. Altered gut microbiota and metabolites profile are associated with reduced bone metabolism in ethanol-induced osteoporosis. Cell Prolif (2022) 55:e13245. doi: 10.1111/cpr.13245
86. Ling CW, Miao Z, Xiao ML, Zhou H, Jiang Z, Fu Y, et al. The association of gut microbiota with osteoporosis is mediated by amino acid metabolism: Multiomics in a Large cohort. J Clin Endocrinol Metab (2021) 106:e3852–64. doi: 10.1210/clinem/dgab492
87. Gong J, He L, Zou Q, Zhao Y, Zhang B, Xia R, et al. Association of serum 25-hydroxyvitamin d (25(OH)D) levels with the gut microbiota and metabolites in postmenopausal women in China. Microbial Cell factories (2022) 21:137. doi: 10.1186/s12934-022-01858-6
88. Wang N, Zheng L, Qian J, Wang M, Li L, Huang Y, et al. Salivary microbiota of periodontitis aggravates bone loss in ovariectomized rats. Front Cell infection Microbiol (2022) 12:983608. doi: 10.3389/fcimb.2022.983608
89. Xue L, Jiang Y, Han T, Zhang N, Qin L, Xin H, et al. Comparative proteomic and metabolomic analysis reveal the antiosteoporotic molecular mechanism of icariin from epimedium brevicornu maxim. J ethnopharmacology (2016) 192:370–81. doi: 10.1016/j.jep.2016.07.037
90. Cheng T, Sheng T, Yi Y, Zhang T, Han H. Metabolism profiles of icariin in rats using ultra-high performance liquid chromatography coupled with quadrupole time-of-flight tandem mass spectrometry and in vitro enzymatic study. J chromatography B Analytical Technol Biomed Life Sci (2016) 1033-1034:353–60. doi: 10.1016/j.jchromb.2016.09.010
91. Pan S, Chen A, Han Z, Wang Y, Lu X, Yang Y. (1)H NMR-based metabonomic study on the effects of epimedium on glucocorticoid-induced osteoporosis. J chromatography B Analytical Technol Biomed Life Sci (2016) 1038:118–26. doi: 10.1016/j.jchromb.2016.10.015
92. Huang J, Hu Y, Tong X, Zhang L, Yu Z, Zhou Z. Untargeted metabolomics revealed therapeutic mechanisms of icariin on low bone mineral density in older caged laying hens. Food Funct (2020) 11:3201–12. doi: 10.1039/C9FO02882J
93. Yuan X, Wen J, Jia H, Tong L, Zhao J, Zhao L, et al. Integrated metabolomic analysis for intervention effects of gushudan on glucocorticoid-induced osteoporostic rat plasma based on RP/HILIC-UHPLC-Q-Orbitrap HRMS. Analytical Biochem (2020) 591:113559. doi: 10.1016/j.ab.2019.113559
94. Huang Y, Bo Y, Wu X, Wang Q, Qin F, Zhao L, et al. An intergated serum and urinary metabonomic research based on UPLC-MS and therapeutic effects of gushudan on prednisolone-induced osteoporosis rats. J chromatography B Analytical Technol Biomed Life Sci (2016) 1027:119–30. doi: 10.1016/j.jchromb.2016.05.019
95. Liu S, Yuan X, Ma C, Zhao J, Xiong Z. (1)H-NMR-based urinary metabolomic analysis for the preventive effects of gushudan on glucocorticoid-induced osteoporosis rats. Analytical Biochem (2020) 610:113992. doi: 10.1016/j.ab.2020.113992
96. Wu X, Huang Y, Sun J, Wen Y, Qin F, Zhao L, et al. A HILIC-UHPLC-MS/MS untargeted urinary metabonomics combined with quantitative analysis of five polar biomarkers on osteoporosis rats after oral administration of gushudan. J chromatography B Analytical Technol Biomed Life Sci (2018) 1072:40–9. doi: 10.1016/j.jchromb.2017.10.005
97. Tao Y, Chen X, Li W, Cai B, Di L, Shi L, et al. Global and untargeted metabolomics evidence of the protective effect of different extracts of dipsacus asper wall. ex C.B. Clarke on estrogen deficiency after ovariectomia in rats. J ethnopharmacology (2017) 199:20–9. doi: 10.1016/j.jep.2017.01.050
98. Wang J, Dong X, Ma F, Li C, Bu R, Lu J, et al. Metabolomics profiling reveals echinops latifolius tausch improves the trabecular micro-architecture of ovariectomized rats mainly via intervening amino acids and glycerophospholipids metabolism. J ethnopharmacology (2020) 260:113018. doi: 10.1016/j.jep.2020.113018
99. Xia T, Dong X, Lin L, Jiang Y, Ma X, Xin H, et al. Metabolomics profiling provides valuable insights into the underlying mechanisms of morinda officinalis on protecting glucocorticoid-induced osteoporosis. J Pharm Biomed Anal (2019) 166:336–46. doi: 10.1016/j.jpba.2019.01.019
100. Jiang YC, Li YF, Zhou L, Zhang DP. UPLC-MS metabolomics method provides valuable insights into the effect and underlying mechanisms of rhizoma drynariae protecting osteoporosis. J chromatography B Analytical Technol Biomed Life Sci (2020) 1152:122262. doi: 10.1016/j.jchromb.2020.122262
101. Li H, Chu S, Zhao H, Liu D, Liu X, Qu X, et al. Effect of zishen jiangtang pill, a Chinese herbal product, on rats with diabetic osteoporosis. Evidence-Based complementary Altern Med eCAM (2018) 2018:7201914. doi: 10.1155/2018/7201914
102. Miao LY, Chu TTH, Li P, Jiang Y, Li HJ. Cimicifuga heracleifolia is therapeutically similar to black cohosh in relieving menopausal symptoms: evidence from pharmacological and metabolomics studies. Chin J Natural Medicines (2019) 17:435–45. doi: 10.1016/S1875-5364(19)30051-2
103. Xiao HH, Sham TT, Chan CO, Li MH, Chen X, Wu QC, et al. A metabolomics study on the bone protective effects of a lignan-rich fraction from sambucus williamsii ramulus in aged rats. Front Pharmacol (2018) 9:932. doi: 10.3389/fphar.2018.00932
104. Luo D, Li J, Chen K, Rong X, Guo J. Untargeted metabolomics reveals the protective effect of fufang zhenshu tiaozhi (FTZ) on aging-induced osteoporosis in mice. Front Pharmacol (2018) 9:1483. doi: 10.3389/fphar.2018.01483
105. Si Z, Zhou S, Shen Z, Luan F. High-throughput metabolomics discovers metabolic biomarkers and pathways to evaluating the efficacy and exploring potential mechanisms of osthole against osteoporosis based on UPLC/Q-TOF-MS coupled with multivariate data analysis. Front Pharmacol (2020) 11:741. doi: 10.3389/fphar.2020.00741
106. Ma YS, Hou ZJ, Li Y, Zheng BB, Wang JM, Wang WB. Unveiling the pharmacological mechanisms of eleutheroside e against postmenopausal osteoporosis through UPLC-Q/TOF-MS-Based metabolomics. Front Pharmacol (2020) 11:1316. doi: 10.3389/fphar.2020.01316
107. Xie H, Hua Z, Guo M, Lin S, Zhou Y, Weng Z, et al. Gut microbiota and metabonomics used to explore the mechanism of qing'e pills in alleviating osteoporosis. Pharm Biol (2022) 60:785–800. doi: 10.1080/13880209.2022.2056208
108. Xia T, Dong X, Jiang Y, Lin L, Dong Z, Shen Y, et al. Metabolomics profiling reveals rehmanniae radix preparata extract protects against glucocorticoid-induced osteoporosis mainly via intervening steroid hormone biosynthesis. Molecules (Basel Switzerland) (2019) 24:253. doi: 10.3390/molecules24020253
109. Shen Q, Zhang C, Qin X, Zhang H, Zhang Z, Richel A. Modulation of gut microbiota by chondroitin sulfate calcium complex during alleviation of osteoporosis in ovariectomized rats. Carbohydr polymers (2021) 266:118099. doi: 10.1016/j.carbpol.2021.118099
110. Wei Z, Ge F, Che Y, Wu S, Dong X, Song D. Metabolomics coupled with pathway analysis provides insights into sarco-osteoporosis metabolic alterations and estrogen therapeutic effects in mice. Biomolecules (2021) 12:41. doi: 10.3390/biom12010041
111. Liu X, Liu Y, Cheng M, Zhang X, Xiao H. A metabolomics study of the inhibitory effect of 17-beta-estradiol on osteoclast proliferation and differentiation. Mol Biosyst (2015) 11:635–46. doi: 10.1039/C4MB00528G
112. Liu H, Gu R, Li W, Zhou W, Cong Z, Xue J, et al. Lactobacillus rhamnosus GG attenuates tenofovir disoproxil fumarate-induced bone loss in male mice via gut-microbiota-dependent anti-inflammation. Ther Adv chronic Dis (2019) 10:2040622319860653. doi: 10.1177/2040622319860653
113. Li P, Sundh D, Ji B, Lappa D, Ye L, Nielsen J, et al. Metabolic alterations in older women with low bone mineral density supplemented with lactobacillus reuteri. JBMR plus (2021) 5:e10478. doi: 10.1002/jbm4.10478
114. Shen CL, Mo H, Dunn DM, Watkins BA. Tocotrienol supplementation led to higher serum levels of lysophospholipids but lower acylcarnitines in postmenopausal women: A randomized double-blinded placebo-controlled clinical trial. Front Nutr (2021) 8:766711. doi: 10.3389/fnut.2021.766711
115. Lee MY, Kim HY, Singh D, Yeo SH, Baek SY, Park YK, et al. Metabolite profiling reveals the effect of dietary rubus coreanus vinegar on ovariectomy-induced osteoporosis in a rat model. Molecules (Basel Switzerland) (2016) 21:149. doi: 10.3390/molecules21020149
116. Wang W, Wang Y, Hu J, Duan H, Wang Z, Yin L, et al. Untargeted metabolomics reveal the protective effect of bone marrow mesenchymal stem cell transplantation against ovariectomy-induced osteoporosis in mice. Cell Transplant (2022) 31:9636897221079745. doi: 10.1177/09636897221079745
117. Gao MX, Tang XY, Zhang FX, Yao ZH, Yao XS, Dai Y. Biotransformation and metabolic profile of xian-Ling-Gu-Bao capsule, a traditional Chinese medicine prescription, with rat intestinal microflora by ultra-performance liquid chromatography coupled with quadrupole time-of-flight tandem mass spectrometry analysis. Biomed Chromatogr BMC (2018) 32:e4160. doi: 10.1002/bmc.4160
118. Ooijevaar RE, Terveer EM, Verspaget HW, Kuijper EJ, Keller JJ. Clinical application and potential of fecal microbiota transplantation. Annu Rev Med (2019) 70:335–51. doi: 10.1146/annurev-med-111717-122956
119. Zhang YW, Cao MM, Li YJ, Lu PP, Dai GC, Zhang M, et al. Fecal microbiota transplantation ameliorates bone loss in mice with ovariectomy-induced osteoporosis via modulating gut microbiota and metabolic function. J orthopaedic translation (2022) 37:46–60. doi: 10.1016/j.jot.2022.08.003
120. He W, Xie Z, Thøgersen R, Rasmussen MK, Zachariassen LF, Jørgensen NR, et al. Effects of calcium source, inulin, and lactose on gut-bone associations in an ovarierectomized rat model. Mol Nutr Food Res (2022) 66:e2100883. doi: 10.1002/mnfr.202100883
121. Xiao HH, Lu L, Poon CC, Chan CO, Wang LJ, Zhu YX, et al. The lignan-rich fraction from sambucus williamsii hance ameliorates dyslipidemia and insulin resistance and modulates gut microbiota composition in ovariectomized rats. Biomedicine pharmacotherapy = Biomedecine pharmacotherapie (2021) 137:111372. doi: 10.1016/j.biopha.2021.111372
122. Tong L, Feng Q, Lu Q, Zhang J, Xiong Z. Combined (1)H NMR fecal metabolomics and 16S rRNA gene sequencing to reveal the protective effects of gushudan on kidney-yang-deficiency-syndrome rats via gut-kidney axis. J Pharm Biomed Anal (2022) 217:114843. doi: 10.1016/j.jpba.2022.114843
123. Yin Y, Zhu F, Pan M, Bao J, Liu Q, Tao Y. A multi-omics analysis reveals anti-osteoporosis mechanism of four components from crude and salt-processed achyranthes bidentata blume in ovariectomized rats. Molecules (Basel Switzerland) (2022) 27:5012. doi: 10.3390/molecules27155012
124. Kitaura H, Marahleh A, Ohori F, Noguchi T, Shen WR, Qi J, et al. Osteocyte-related cytokines regulate osteoclast formation and bone resorption. Int J Mol Sci (2020) 21:5169. doi: 10.3390/ijms21145169
125. An J, Yang H, Zhang Q, Liu C, Zhao J, Zhang L, et al. Natural products for treatment of osteoporosis: The effects and mechanisms on promoting osteoblast-mediated bone formation. Life Sci (2016) 147:46–58. doi: 10.1016/j.lfs.2016.01.024
126. Karlamangla AS, Burnett-Bowie SM, Crandall CJ. Bone health during the menopause transition and beyond. Obstetrics gynecology Clinics North America (2018) 45:695–708. doi: 10.1016/j.ogc.2018.07.012
127. Eastell R, Szulc P. Use of bone turnover markers in postmenopausal osteoporosis. Lancet Diabetes Endocrinol (2017) 5:908–23. doi: 10.1016/S2213-8587(17)30184-5
128. Mangano KM, Noel SE, Lai CQ, Christensen JJ, Ordovas JM, Dawson-Hughes B, et al. Diet-derived fruit and vegetable metabolites show sex-specific inverse relationships to osteoporosis status. Bone (2021) 144:115780. doi: 10.1016/j.bone.2020.115780
129. Chau YP, Au PCM, Li GHY, Sing CW, Cheng VKF, Tan KCB, et al. Serum metabolome of coffee consumption and its association with bone mineral density: The Hong Kong osteoporosis study. J Clin Endocrinol Metab (2020) 105:dgz210. doi: 10.1210/clinem/dgz210
130. Tian J, Li Z, Wang L, Qiu D, Zhang X, Xin X, et al. Metabolic signatures for safety assessment of low-level cadmium exposure on human osteoblast-like cells. Ecotoxicology Environ Saf (2021) 207:111257. doi: 10.1016/j.ecoenv.2020.111257
131. Kong SH, Kim JH, Shin CS. Serum spermidine as a novel potential predictor for fragility fractures. J Clin Endocrinol Metab (2021) 106:e582–91. doi: 10.1210/clinem/dgaa745
132. Deng D, Pan C, Wu Z, Sun Y, Liu C, Xiang H, et al. An integrated metabolomic study of osteoporosis: Discovery and quantification of hyocholic acids as candidate markers. Front Pharmacol (2021) 12:725341. doi: 10.3389/fphar.2021.725341
133. Wang Z, Bian L, Mo C, Shen H, Zhao LJ, Su KJ, et al. Quantification of aminobutyric acids and their clinical applications as biomarkers for osteoporosis. Commun Biol (2020) 3:39. doi: 10.1038/s42003-020-0766-y
134. Wang Y, Ma L, Zhang M, Chen M, Li P, He C, et al. A simple method for peak alignment using relative retention time related to an inherent peak in liquid chromatography-mass spectrometry-based metabolomics. J chromatographic Sci (2019) 57:9–16. doi: 10.1093/chromsci/bmy074
135. Reinke SN, Galindo-Prieto B, Skotare T, Broadhurst DI, Singhania A, Horowitz D, et al. OnPLS-based multi-block data integration: A multivariate approach to interrogating biological interactions in asthma. Analytical Chem (2018) 90:13400–8. doi: 10.1021/acs.analchem.8b03205
136. Tao Y, Huang S, Yan J, Li W, Cai B. Integrated metallomic and metabolomic profiling of plasma and tissues provides deep insights into the protective effect of raw and salt-processed achyranthes bidentata blume extract in ovariectomia rats. J ethnopharmacology (2019) 234:85–95. doi: 10.1016/j.jep.2019.01.033
137. Jia H, Yuan X, Liu S, Feng Q, Zhao J, Zhao L, et al. Integrated renal metabolomic and metallomic profiling revealed protective effect and metabolic mechanism of gushudan on glucocorticoid-induced osteoporotic rat based on GC-MS and ICP-MS. J Pharm Biomed Anal (2021) 193:113705. doi: 10.1016/j.jpba.2020.113705
138. Shen L, Yu Y, Zhou Y, Pruett-Miller SM, Zhang GF, Karner CM. SLC38A2 provides proline to fulfill unique synthetic demands arising during osteoblast differentiation and bone formation. eLife (2022) 11:e76963. doi: 10.7554/eLife.76963.sa2
139. Kodrič K, Čamernik K, Černe D, Komadina R, Marc J. P4 medicine and osteoporosis: a systematic review. Wiener klinische Wochenschrift (2016) 128:480–91. doi: 10.1007/s00508-016-1125-3
140. Wang YF, Chang YY, Zhang XM, Gao MT, Zhang QL, Li X, et al. Salidroside protects against osteoporosis in ovariectomized rats by inhibiting oxidative stress and promoting osteogenesis via Nrf2 activation. Phytomedicine Int J phytotherapy phytopharmacology (2022) 99:154020. doi: 10.1016/j.phymed.2022.154020
141. Li Y, Liu J, Zhou H, Liu J, Xue X, Wang L, et al. Liquid chromatography-mass spectrometry method for discovering the metabolic markers to reveal the potential therapeutic effects of naringin on osteoporosis. J chromatography B Analytical Technol Biomed Life Sci (2022) 1194:123170. doi: 10.1016/j.jchromb.2022.123170
142. Yang F, Dong X, Ma F, Xu F, Liu J, Lu J, et al. The interventional effects of tubson-2 decoction on ovariectomized rats as determined by a combination of network pharmacology and metabolomics. Front Pharmacol (2020) 11:581991. doi: 10.3389/fphar.2020.581991
143. Goodpaster BH, Sparks LM. Metabolic flexibility in health and disease. Cell Metab (2017) 25:1027–36. doi: 10.1016/j.cmet.2017.04.015
144. Masoodi M, Gastaldelli A, Hyötyläinen T, Arretxe E, Alonso C, Gaggini M, et al. Metabolomics and lipidomics in NAFLD: biomarkers and non-invasive diagnostic tests. Nat Rev Gastroenterol Hepatol (2021) 18:835–56. doi: 10.1038/s41575-021-00502-9
145. Cui L, Lu H, Lee YH. Challenges and emergent solutions for LC-MS/MS based untargeted metabolomics in diseases. Mass spectrometry Rev (2018) 37:772–92. doi: 10.1002/mas.21562
Keywords: metabolomics, osteoporosis, pathogenic mechanism, herbal medicine, treatment
Citation: Zhao Z, Cai Z, Chen A, Cai M and Yang K (2022) Application of metabolomics in osteoporosis research. Front. Endocrinol. 13:993253. doi: 10.3389/fendo.2022.993253
Received: 13 July 2022; Accepted: 28 October 2022;
Published: 14 November 2022.
Edited by:
Rupesh K. Srivastava, All India Institute of Medical Sciences, IndiaReviewed by:
Pradyumna Kumar Mishra, ICMR-National Institute for Research in Environmental Health, IndiaHamid Yousf Dar, School of Medicine, Emory University, United States
Copyright © 2022 Zhao, Cai, Chen, Cai and Yang. This is an open-access article distributed under the terms of the Creative Commons Attribution License (CC BY). The use, distribution or reproduction in other forums is permitted, provided the original author(s) and the copyright owner(s) are credited and that the original publication in this journal is cited, in accordance with accepted academic practice. No use, distribution or reproduction is permitted which does not comply with these terms.
*Correspondence: Ming Cai, Y21kb2N0b3JAdG9uZ2ppLmVkdS5jbg==; Kai Yang, Y3lhbi5rYWlAc2hzbXUuZWR1LmNu