- 1The First Affifiliated Hospital, Anhui Medical University, Hefei, China
- 2Inflammation and Immune Mediated Diseases Laboratory of Anhui Province, Hefei, China
- 3Anhui Institute of Innovative Drugs, Hefei, China
- 4School of Pharmacy, Anhui Medical University, Hefei, China
- 5Institute of clinical pharmacology, Anhui Medical University, Hefei, China
- 6Geriatric Department, The First Affifiliated Hospital of USTC, Division of Life Sciences and Medicine, University of Science and Technology of China, Hefei, China
Gouty arthritis is a common inflammatory disease. The condition is triggered by a disorder of uric acid metabolism, which causes urate deposition and gout flares. MicroRNAs are a class of conserved small non-coding RNAs that bind to the 3’ untranslated region (UTR) of mRNA and regulate the expression of a variety of proteins at the post-transcriptional level. In recent years, attention has been focused on the role of miRNAs in various inflammatory diseases, including gouty arthritis. It is thought that miRNAs may regulate immune function and inflammatory responses, thereby influencing the onset and progression of the disease. This article mainly reviewed the roles of miRNAs in the pathogenesis of gouty arthritis and prospected their potential as diagnostic and prognostic relevant biomarkers and as possible therapeutic targets.
1 Introduction
Gouty arthritis (GA) is an inflammatory joint disease with a prevalence of 3.9% of all adults in the United States, 5.2% for men, and 2.7% for women (1). As a disorder of uric acid metabolism, this disease is mainly caused by the deposition of monosodium urate crystals (MSU) in the joint capsule, bursa, bone, and cartilage, ultimately causing joint damage and even deformity (2). With gout flares, the pain increases and seriously affects the patient’s quality of life. In addition, gout is closely associated with metabolic comorbidities that can lead to myocardial infarction, type 2 diabetes, chronic kidney disease, and premature death (3, 4). The treatment of gouty arthritis attacks is mainly to control pain and suppress joint inflammation, such as the use of non-steroidal anti-inflammatory drugs, glucocorticoids, etc. The long-term management of patients with gouty arthritis focuses on uric acid-lowering therapy to reverse hyperuricemia and thus prevent gout attacks (5, 6). Despite new treatment strategies and a good understanding of the pathogenesis of gouty arthritis, recurrent attacks continue to occur after treatment (7).
MiRNA is a conserved short-stranded non-coding RNA of approximately 23nt in length, and they were first identified in Caenorhabditis elegans in 1993 (8). miRNAs are first transcribed in the nucleus as longer primary miRNAs (primary miRNA, pri-miRNA), then processed in the nucleus by Drosha into hairpin RNAs of 60-70 nucleotides, i.e. precursor miRNAs (miRNA precusor, pre-miRNA), which are transported out of the nucleus with the help of the Exprotin-5 complex and sheared in the cytoplasm by Dicer to become mature miRNAs (9, 10).Current studies have shown that miRNAs are highly conserved evolutionarily (11). A miRNA can regulate the activity or stability of multiple target genes by recognizing and inducing the assembly of the RNA silencing complex (RISC) with the miRNA response element (MRE) in the mRNA 3’ untranslated region (UTR) region of the target gene, and multiple microRNAs can also synergistically regulate the same target gene (12, 13). More than 1,000 miRNAs have been identified in human cells, while more than 500 microRNAs in the human body (14). Although the functions of miRNAs are not fully understood, relevant studies have shown that miRNAs are involved in various processes, including cell differentiation, metabolism, and inflammation (15).
Recent research has demonstrated that miRNA plays an essential role in the pathogenesis of common nonautoimmune inflammatory diseases, including gouty arthritis (16). Although some studies have attempted to elucidate the crucial role of miRNAs in the pathogenesis of gouty arthritis, their analyses have always been conducted in a single direction. They have not diversified to integrate multiple fields of study. Therefore, this paper reviews the various regulatory mechanisms of miRNAs in developing gout, including its relationship with uric acid metabolism, classical inflammatory signaling pathways, and bone erosion. On this foundation, we considered the promise of miRNA as a potential diagnostic and prognostic marker for gout and as a therapeutic target.
2 Overview of gouty arthritis
Gouty arthritis (GA) is characterized by swelling and heat pain in one side of the joint, causing joint dysfunction, deformity, and even disability (17). Epidemiology reports the current range of gout incidence at 0.6-2.8 per 1000 people per year. The prevalence of gout has continued to increase worldwide in recent decades, probably due to the aging of society’s population and changes in dietary patterns (18–20). The development of gout is based on four pathophysiological stages, the first two of which are hyperuricemia and the formation and deposition of sodium urate crystals, the leading causes of which are disorders of purine metabolism and dysregulation uric acid secretion (Figure 1). The latter two components are mainly gout flares triggered by acute inflammatory reactions and irreversible bone erosion caused by the deposition of tophi in advanced gout (21).
Usually, the intake of purines is converted by xanthine oxidase in the liver to uric acid, which is excreted mainly through the kidneys (22). The renal tubular transporters such as OAT4 and GLUT9 are responsible for the reabsorption of uric acid (23, 24), while ABCG2 and ABCC4 are responsible for the secretion of uric acid (25, 26). And once a high purine diet such as alcohol, seafood, and meat is accompanied by impaired renal excretion function, it will lead to hyperuricemia. Once a high purine diet is accompanied by inadequate excretion by the kidneys, it can lead to hyperuricemia (27, 28).
Due to the high concentration of urate, along with other physical and chemical environments, MSU is generated and deposited in the joint cavity, which in turn acts as foreign bodies to recruit neutrophils and macrophages and initiate toll-like receptor (TLR) and NLRP3 inflammasome signaling pathways to activate innate immunity (29–31). Current studies suggest that the acute flares of gout depend on two switches, one of which is the activation of the TLR2/4-NF-κB signaling pathway within macrophages or monocytes, which promotes the synthesis of pro-IL1-β and significant components of the inflammasome, and that this activation is associated with the influence of large amounts of free fatty acids, intestinal flora or other microorganisms (32, 33). Stimulating sodium urate crystals is another critical point of activation of gout inflammation, which activates the NLRP3 inflammatory pathway by promoting the assembly of inflammatory vesicles, thereby promoting the conversion of pro-caspase-1 to caspase-1 and the release of large amounts of pro-inflammatory factors such as IL-1β (34–36).
These pro-inflammatory factors recruit more neutrophils to exacerbate the inflammatory response; however, along with the inflammatory death of large numbers of neutrophils, activated neutrophils release extracellularly depolymerized chromatin and intracellular granule proteins, called neutrophil extracellular traps (NETs), to trap and break down inflammatory factors to relieve gout flares (37, 38). This chronic inflammatory response develops into the advanced disease characterized by tophi (Figure 1), a microenvironment of adaptive and innate immune cells, MSU, and fibroblasts, promoting bone resorption by osteoclasts and reducing bone formation osteoblasts, and ultimately causes bone erosion (39, 40).
3 The function and mechanism of miRNAs in GA
Extensive studies illustrate that abnormal expression in miRNAs occurs during the pathophysiology of gouty arthritis (15, 41, 42). Only 10% of the population with high uric acid has positive signs of gouty arthritis, which may be associated with different genetic backgrounds, and miRNA sequence alterations affect the genetic susceptibility background (43). In addition to this, human genome-wide association studies (GWAS) have identified many loci associated with hyperuricemia and gout, and these single nucleotide polymorphism (SNP) loci are mainly associated with the coding of uric acid transporter genes (44, 45). Further studies have illustrated the ability of miRNAs to regulate inflammatory immune-related processes in gouty arthritis (46, 47).
Over the past years, attempts have been made to identify aberrantly expressed MiRNAs in gout to explore the role of these molecules in the pathogenesis of gout. In 2014 Tae-Jong Kim et al. first investigated the role of Mir-155 in acute gouty arthritis (48). So far, studies on gouty arthritis have been divided into omics-based high-throughput studies and in vitro cellular models stimulated with MSU or in vivo animal models. A large number of meaningful results have now been identified. Therefore, we will summarize the relationship between miRNAs and gout pathogenesis in terms of hyperuricemia, inflammatory immunity, and bone erosion and look at their diagnostic and therapeutic value based on the last five years of publication and older but more classic literature
3.1 Involvement of miRNAs in the hyperuricemia
Hyperuricemia (HUA) is the prodromal stage of gout attack and a common clinical feature in the course of gouty arthritis. The inability to excrete uric acid from purines promptly leads to a series of disturbances in the metabolic environment and can even cause damage to liver and kidney function (49). As the body’s primary urate handling organ, the kidney generally relies on renal tubular urate transport proteins, such as URAT1, GLUT9, and ABCG2, to regulate uric acid excretion (26, 44). It was reported that C421A polymorphism enhanced the degradation of ABCG2 in a miRNA-dependent manner and that the use of inhibitors of miR-519c and miR-328 reversed this translational repression (50). Sun, W. et al. reported that Xie-Zhuo-Chu-Bi-Fang could upregulate miR-34a and downregulate URAT1 to treat hyperuricemia (51). In addition, miR-143-3p can directly target the 3’UTR of GLUT9 in renal tubular epithelial cells to reduce uric acid reabsorption and inflammatory response (52). In a clinical study, miR-155 was elevated in the serum of HUA patients, and subjects with urate deposition had higher miR-155 than those without deposition findings (53). In vitro experiments also revealed that miR-155 was elevated in high-uric acid-stimulated venous endothelial cells (HUVEC) and inhibited eNOS expression causing endothelial cell dysfunction (54).
Similarly, hyperuricemic stimulation led to the downregulation of miR-92a, thereby inhibiting vascular neogenesis through the KLF2-VEGFA axis (55). Hong Q et al. also found that miR-663 could act on the transcript of TGF-β1 to regulate PTEN to inhibit endothelial cell migration (56). These studies also suggest that high uric acid causes cardiovascular damage and explains the correlation between gout and cardiovascular disease such as myocardial infarction. And miRNAs also play a precise regulatory role in liver and kidney function damage caused by excessive uric acid. Uric acid damages renal interstitial fibroblasts by downregulating miR-9 and causing activation of NF-KB and JAK-STAT pathways (57). Besides, Chen, S et al. also reported that overexpression of miR149-5p inhibited FGF21 expression and attenuated uric acid-induced lipid deposition in hepatocytes (58). Chi, K et al. found that HOTAIR competitively binds miR-22 in hyperuricemia to regulate NLRP3 inflammasome activation to promote endothelial cell pyroptosis and exacerbate renal injury (59). Recent studies have also reported a decrease in miR-30b and an increase in IL-6R in serum urine and kidney tissue in a mouse model of HUA (60). The above study we summarized in the Table 1. In summary, miRNAs are involved in the development of hyperuricemia and play an important role, and targeting miRNA processing may provide new insights for the future treatment of hyperuricemia.
3.2 Role of miRNAs in the regulation of immune-inflammatory responses
3.2.1 miRNAs and TLR2/4/MyD88/NF-KB pathway in GA
The onset of gouty arthritis results from an inflammatory immune response triggered by MSU deposition. Two pathways mediate the main molecular mechanisms: activation of the TLR-related NF/KB signaling pathway and activation of the inflammatory vesicle NLRP3, respectively. The former is mainly microbial or free fatty acids activating Toll-like pattern recognition receptors, mainly TLR2/4, recognizing the downstream signaling molecule myeloid differentiation factor 88 (MyD88) for intracellular signaling and finally leading to the activation of NF-KB (31, 35, 61). Numerous studies have illustrated the significant correlation between miR-192 and NF-KB pathway. For example, miR-192-5p effectively alleviated tumor progression by inhibiting the IRAK1/NF-κB pathway in endometrial cancer (62, 63). Recently, Lian, C et al. found that miR-192-5p in MSU-treated synovial fluid mononuclear cells (SMFCs) and THP-1 could target TLR4 to inhibit NF-KB pathway activation reducing TNF-a and IL-1β release (Figure 2) (64). MiR-146a is the first regulator that is involved in innate immunity. It has been reported that miR-146a can regulate key downstream adaptor molecules of TLR in sepsis by complementary pairing with the 3’UTR base sequence of TNF receptor-associated factor 6 (TRAF6) and IL-1 receptor-associated kinase 1 (IRAK1) genes, thereby inhibiting the activity of TLR signaling pathway and thus inhibiting NF- κB signaling pathway to exert inflammatory suppressive effects (65). Another study indicates miR-146a alleviates inflammation in acute gouty arthritis in rats via TLR4/MyD88/NF-KB signaling pathway. And further study demonstrated that miR-146a knockout mice promoted the development of gouty arthritis by upregulating TRAF6 and IRAK-1 expression compared to wild type (Figure 2) (66, 67). Ma, T et al. illustrated that MicroRNA-302b could directly bind to the 3’ UTR of IRAK4 and EphA2 in an in vivo and in vitro model of gout to inhibit activation of the NF-KB pathway to reduce IL-1β (Figure 2) (68). Therefore, targeting the TLR-mediated NF-KB pathway via miRNA can be a promising approach for GA treatment.
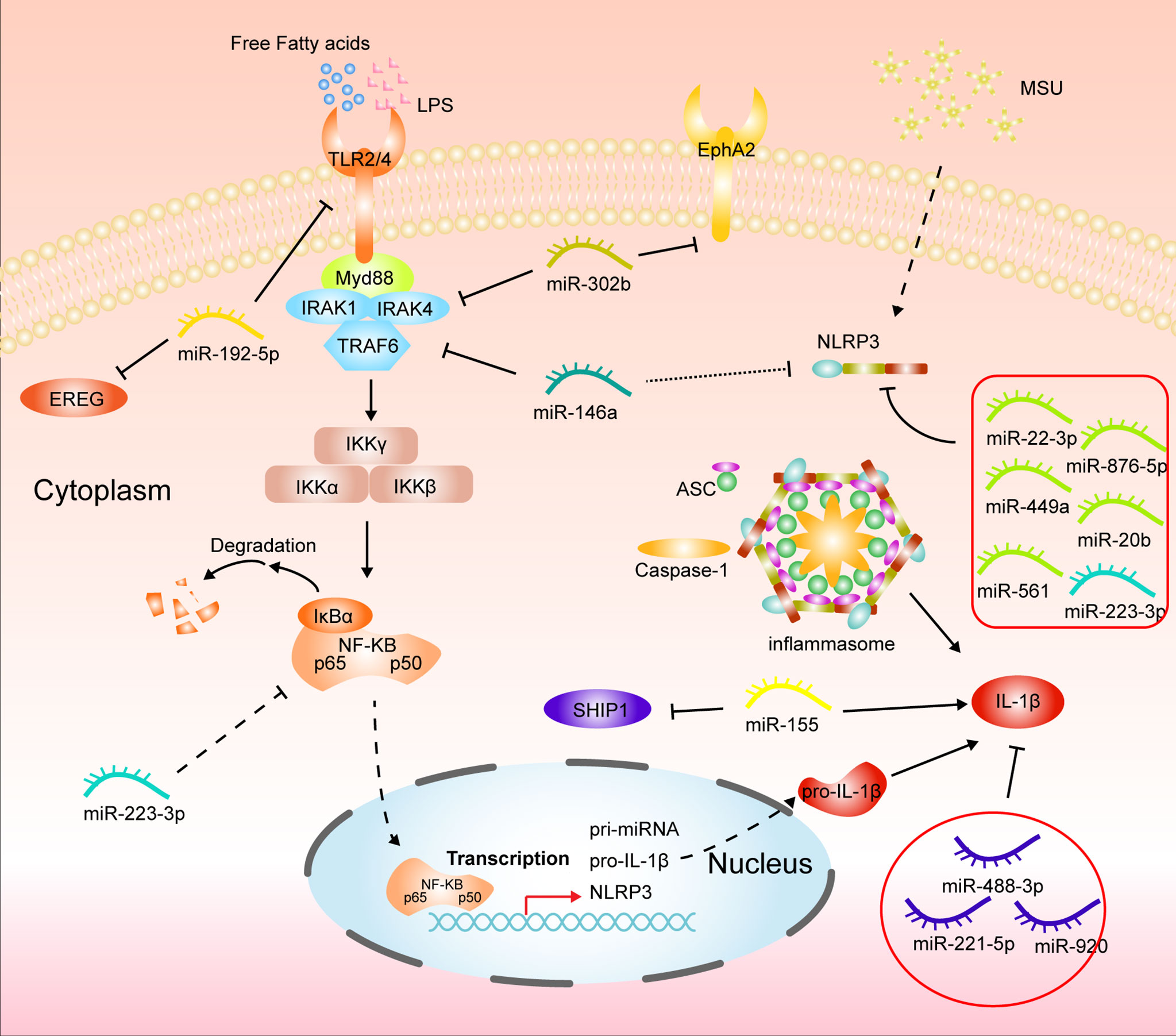
Figure 2 Mechanisms of miRNAs in gouty arthritis regulating inflammatory pathways in monocytes/macrophages.
3.2.2 miRNAs and NLRP3 inflammasome pathway in GA
NLRP3 is the most comprehensive subfamily studied in the nucleotide-binding oligomerization domain-like receptor (69). NLRP3 inflammasome comprises NLRP3, recruitment domain, an adaptor protein, apoptosis-associated spot like protein (ASC), and caspase-1, expressed in many immune cells. In gouty arthritis, MSU crystal acts as a ligand to bind and activate NLRP3 inflammasome. After the conformational change of NLRP3 protein, it polymerizes with ATP to form a protein oligomer. It then recruits pro-caspase-1 and ASC through its effector domain PYD, The caspase-1 precursor was automatically catalyzed into the active form, and proIL-1β was enzymolysis into IL-1β (70, 71). Current studies have confirmed that miRNAs are critical regulators of the NLRP3 inflammasome pathway (72). In recent years, it has been reported that circHIPK3 can act as a molecular sponge to adsorb miR-561 to competitively bind NLRP3 mRNA to reduce inflammation in GA (64). Upregulation of miR-20b expression and downregulation of NLRP3 protein was also found in macrophages with HOTAIR knockdown (73). Wang, X et al. reported that miR-223-3p and miR-22-3p could reduce inflammatory effects in monocytes and mouse models of gout by interacting with the 3’ untranslated region segment of NLRP3 mRNA (74). And Tian, J et al. also found that miR-223 could target NLRP3 mRNA in MSU-induced rat models and fibroblast-like synoviocytes to inhibit inflammation and cellular pyroptosis. Further studies confirmed that miR-223 deficiency exacerbated the swelling index of MSU-induced joint inflammation and intensified inflammatory cell infiltration and cytokine release, including IL-1β and MCP-1, compared to WT mice (75, 76). Similarly, the expression of NLRP3 was dramatically upregulated in Bone marrow-derived macrophages (BMDMs) from miR-146a KO mice (67). Besides, the active ingredients of some Chinese traditional medicines also exert anti-inflammatory effects in GA by regulating the miRNA/NLRP3 axis. Total glucosides of paeony alleviate in vitro MSU-induced inflammation in macrophage THP-1 via the MALAT1/miR-876-5p/NLRP3 cascade pathway. Wang Y et al. also reported that Tripterine alleviates GA by modulating miR-449a to act directly on NLRP3 mRNA and inhibit its expression (Figure 2) (77, 78).
3.2.3 miRNAs and other mechanisms of inflammation in GA
In GA, when inflammatory pathways in macrophages are activated, they are polarized toward the M1 phenotype and release large amounts of pro-inflammatory factors such as TNF-a, IL-1β, and MCP-1. miRNAs also play a regulatory role in this process. MiR-449a and miR-192-5p can target NLRP3 and epiregulin (EREG) to inhibit macrophage M1 polarization in gout (77, 79). Apart from this, miRNA-488-3p and -920 both can interact with the 3’UTR of IL1-β to exert anti-inflammatory effects (80). Li, G. et al. also reported that miR-221-5p represses IL1-β expression in acute gouty arthritis to regulate the inflammatory response (81). Although Jin, H. M et al. reported that overexpression of miR-155 in vitro reduced SHIP-1 levels and promoted MSU-induced TNF-a and IL-1β production (Figure 2), it was later indicated that there was no remission of GA in miR-155 knockout mice compared to wild type (48, 82). Inflammatory factors tend to infiltrating immune cells, such as neutrophils, which on the one hand, worsen inflammation.
On the other hand, large numbers of neutrophils accumulate inflammatory death, chromatin remodeling, and ejection outside the cells to form NETs, called NETosis. These aggregated NETs can trap pro-inflammatory factors and act in conjunction with some anti-inflammatory factors to reduce the development of inflammation. Yet, few studies on the relationship between miRNAs and NETs in gout. Recently, it has been shown that MSU stimulation can significantly increase miR-3146 expression in neutrophils, accompanied by the formation of many NETs. In contrast, treating rats with antagomir-3146 reduced NETs formation and relief of joint swelling and inflammation, suggesting that early NETs formation exacerbates GA and that miR-3146 plays a role vital role before NETs formation (83). However, the current research on NETs is still inadequate, and how miRNAs regulate the development of NETs in gout is still unclear. Further studies are needed to reveal the potential mechanisms to help people understand gout and identify potential therapeutic targets. We conclude with a summary of miRNAs involved in inflammatory immunity. (Table 2)
3.3 The regulatory role of miRNAs in bone erosion
There is irreversible joint damage and deformity in advanced gout, mainly due to local cartilage damage and bone erosion caused by tophi (40). The primary mechanism is that MSU disrupts the balance between osteoblasts for bone formation and osteoclasts for bone resorption, decreases the activity of osteoblasts, promotes the aggregation and differentiation of osteoclasts, and promotes the development of inflammation and bone damage (84–86). Extensive studies have confirmed the involvement of miRNAs in the development of bone erosion. For instance, miR-20a targets RANKL through the TLR4/p38 pathway, hindering osteoclast proliferation and differentiation (87). Sujitha, S et al. found that miR-23a altered the expression level of LRP5 through RNA interference and contributed to a decrease in bone loss and an increase in calcium retention (88). In addition, Najm, A. et al. also demonstrated that miR-17 inhibits the autocrine effects of the IL-6 family in vivo by directly targeting JAK1 and STAT3 to exert anti-inflammatory and anti-bone erosion effects (89). There are few studies on miRNAs affecting bone erosion in gouty arthritis. Only An L et al. reported that miR-192-5p could inhibit MSU-induced EREG expression in GA mice to alleviate bone erosion (79). This case suggests that the underlying molecular mechanisms of miRNAs affecting bone erosion in GA remain to be explored.
4 Application of miRNAs in clinical diagnosis and treatment of GA
First of all, miRNA widely exists in a variety of body fluids, such as whole human blood (90), urine (91) saliva (92). Secondly, miRNA is stable in body fluid in a specific secretion mode, and it is easy to extract tissue samples without invasion. Even under changing environmental conditions, miRNA can stably exist. Because of its specificity, sensitivity, and stable expression in a wide range of diseases, MiRNA has early diagnostic capabilities and is rapid and accurate (92). In studies of gouty arthritis, several miRNAs are up-or down-regulated, and some of these miRNAs also vary with the extent of the disease. BohatáJ et al. found elevated levels of five circulating miRNAs, miR-17, miR-18a, miR-30c, miR-142, and miR-223, in the plasma of patients with GA and HUA (93). In addition, it has been reported that miR-221 is lowly expressed in the serum of AGA patients and the receiver operating curve (ROC) applied to the diagnostic value analysis showed an area under the curve of 0.884 (81). Therefore miRNAs have the potential to become markers for gout diagnosis.
The treatment of GA is mainly divided into anti-inflammatory and analgesic, and uric acid lowering. The primary treatment for gout attacks is cortisol, non-steroidal anti-inflammatory drugs (including selective and non-selective COX2 inhibitors), and low-dose colchicine to control pain and lessen inflammation (94–97). Although IL-1 inhibitors can effectively control gout attacks, they are usually reserved for patients with intolerable side effects or contraindications to first-line anti-inflammatory therapy (98, 99). The first-line uric acid-lowering therapy drug is allopurinol, a xanthine oxidase inhibitor. Still, patients who do not respond to or are intolerant of allopurinol are treated with febuxostat (6, 100). Probenecid, sulfinpyrazone, and benzbromarone can be used as monotherapy or combined with xanthine oxidase inhibitors by promoting uric acid excretion (101, 102). Liu, P et al. found that colchicine upregulated mir-223-3p and downregulated IL-1β, and etoricoxib treated AGA by upregulating miR-451a and downregulating COX-2 (103). A recent study reported that two novel hexapeptides (GPAGPR and GPSGRP) found in Apostichopus japonicus hydrolysates inhibit uric acid biosynthesis and reabsorption. The expression profiles of GPSGRP-treated HUA model mice were analyzed, and 21 differentially expressed miRNAs were identified (104).
Chinese medicine or natural products have been developed to treat gout arthritis in recent years. The drugs for acute gout arthritis have severe adverse reactions such as bone marrow suppression, liver cell damage, and gastrointestinal bleeding (105). Traditional Chinese medicine has some advantages in terms of low toxicity and adverse reactions. Wang Y et al. indicated that both Chuan Hu Tong Feng Compound and Allopurinol upregulated miR-486-5p, miR-339-5p, and miR-361-5p expression and decreased CCL2 and CXCL8 protein levels in patients with chronic gouty arthritis (106). Another research proved that benzbromarone and Xiezhuo Chubi Decoction reduced uric acid levels by increasing the expression levels of miR-34a and miR-146a (107). In addition, like Tripterine (77), Total glucosides of paeony (78) and Epigallocatechin (57) were also reported to regulate the expression of miR-449a, miR-876, and miR-9, respectively, to alleviate the progression of GA. Similarly, Li, X et al. reported that Noni (Morinda citrifolia L.) fruit Juice also modulates miRNA and pro-inflammatory factors to treat MSU-induced AGA in mice (108).
Although miRNA has much fundamental research on the treatment of arthritis, there are still many problems in the transition from mechanism research to clinical application. Therefore, miRNAs related to gout treatment need further exploration and development.
5 Future expectation
Since the discovery of miRNAs, their wide range of biological effects have been gradually revealed, also indicating that they play an important regulatory role in various cellular activities. Based on recent research results in related fields, in this section, we will look at the future directions of miRNA research and the prospects of clinical applications.
Exosomes, a cellular vesicle structure widely found in body fluids, have been identified for the presence of miRNAs. In recent years, several studies have demonstrated that miRNAs regulate inflammatory immunity and tumor progression through exosomes as vectors. Jiang, K et al. found that peripheral-derived exosome-mediated miR-155 promoted the polarization and proliferation of macrophage M1 and activated the NF-KB pathway to promote the release of inflammatory factors TNF-α and IL-6 in an acute lung injury model in mice (109). Similarly, Yingying Cao et al. reported that Enterotoxigenic Bacteroides fragilis (ETBF) promotes intestinal inflammation and malignancy by inhibiting exosome-encapsulated miR-149 (110). Naïve bone marrow-derived macrophages produce exosomes with anti-inflammatory miRNAs that target receptor macrophages to promote their M2 polarization and alleviate inflammation (111). Therefore, it is natural to speculate that circulating exosomes contain miRNAs that may influence the development of GA by regulating macrophage polarization or other key molecules of inflammation. Furthermore, such exosome-derived miRNAs have great application in both the diagnosis and treatment of GA.
In addition, nanomaterials as carriers of drug-targeted delivery systems have become a hot research topic due to the development of the interdisciplinary intersection of materials science and medicine in recent years. Since miRNA mimics are not resistant to nucleic acid endonucleases and are prone to degradation in circulation, nanomaterials can be used to wrap miRNAs for targeted therapy. Wang, F et al. reported that microRNA-31 bound to adriamycin-loaded mesoporous silica nanoparticles would be used to target tumor cells high in MTEF4 expression to promote mitochondrial apoptosis (112). Moreover, Ahir, M. et al. also reported that the use of mesoporous silica nanoparticles as co-delivery carriers of miR-34a-Mimic and antisense-miR-10b on tumor cells effectively inhibited tumor growth and metastasis in triple-negative breast cancer (113). Although nanomaterials are being studied as potential therapies for tumors and inflammatory diseases, they may still cause an immune response in the body and exacerbate inflammation. Therefore the development of low inflammatory response nanomaterials for the treatment of inflammatory diseases remains a great challenge. With the development of basic science and technology, gene-editing technology is becoming more and more mature and is expected to be used for the treatment of many diseases. CRISPR/Cas9 technology, which won the Nobel Prize, has brought a revolution to the life science field. Recently, Zhou, W et al. reported that CRISPR/Cas9-mediated knockdown of miRNA-363 effectively promoted apoptosis in diffuse large B-cell lymphoma cell lines in response to adriamycin-induced apoptosis (114). And, Yu Toyoda et al. also utilized CRISPR/cas9 to construct knockout mice to identify the role of GLUT12 in regulating blood urate levels (115). Therefore, the use of CRISPR/cas9-mediated miRNA knockdown to suppress inflammation in gouty arthritis still holds great promise for research.
6 Conclusion
In recent years, miRNAs have become a hot topic in biomedical research. Current studies have shown that miRNAs are closely associated with the development and progression of gouty arthritis, and miRNAs play an essential role in the post-transcriptional regulation process of genes. Despite the large number of studies reporting miRNA regulation of the gouty inflammatory process, there is still a significant gap in gouty arthritis, especially in bone erosion.
The underlying mechanisms of self-remission of gout as a recurrent chronic disease and the formation of gouty stones remain unclear. What is neutrophil-associated NETosis in the inflammatory process, and is it the culprit that exacerbates inflammation or relieves it leading to recurrent gout attacks?
Although gout is already a treatable rheumatic disease, the side effects of drugs are still evident, and targeting miRNAs may provide a new idea and insight for gout treatment. In the future, miRNA is expected to be a marker for diagnosing gouty arthritis or a target for drug therapy. However, further studies are still needed. Therefore, the search for relevant miRNAs and further study of their mechanisms are essential for diagnosing and treating gouty arthritis.
Author contributions
TX and JWu conceived and designed the article, ZL and FY wrote the manuscript, SH and JWa reviewed the literature, BC, LL and JY revised the article, and YY, CY, YH, and SW proofread the language. All authors contributed to the article and approved the submitted version.
Funding
This study was supported by the youth scientific research fund of Anhui Medical University (2021xkj275, chaired by zhipan Luo) and the school level quality project of Anhui Medical University (2021xjyxm24, chaired by zhipan Luo).
Conflict of interest
The authors declare that the research was conducted in the absence of any commercial or financial relationships that could be construed as a potential conflict of interest.
Publisher’s note
All claims expressed in this article are solely those of the authors and do not necessarily represent those of their affiliated organizations, or those of the publisher, the editors and the reviewers. Any product that may be evaluated in this article, or claim that may be made by its manufacturer, is not guaranteed or endorsed by the publisher.
References
1. Chen-Xu M, Yokose C, Rai SK, Pillinger MH, Choi HK. Contemporary prevalence of gout and hyperuricemia in the united states and decadal trends: the national health and nutrition examination survey, 2007-2016. Arthritis Rheumatol (2019) 71:991–9. doi: 10.1002/art.40807
2. Perez-Ruiz F, Marimon E, Chinchilla SP. Hyperuricaemia with deposition: latest evidence and therapeutic approach. Ther Adv Musculoskelet Dis (2015) 7:225–33. doi: 10.1177/1759720X15599734
3. Fisher MC, Rai SK, Lu N, Zhang Y, Choi HK. The unclosing premature mortality gap in gout: a general population-based study. Ann Rheum Dis (2017) 76:1289–94. doi: 10.1136/annrheumdis-2016-210588
4. Roughley MJ, Belcher J, Mallen CD, Roddy E. Gout and risk of chronic kidney disease and nephrolithiasis: meta-analysis of observational studies. Arthritis Res Ther (2015) 17:90. doi: 10.1186/s13075-015-0610-9
5. Richette P, Doherty M, Pascual E, Barskova V, Becce F, Castaneda-Sanabria J, et al. 2016 Updated EULAR evidence-based recommendations for the management of gout. Ann Rheum Dis (2017) 76:29–42. doi: 10.1136/annrheumdis-2016-209707
6. FitzGerald JD, Dalbeth N, Mikuls T, Brignardello-Petersen R, Guyatt G, Abeles AM, et al. 2020 American College of rheumatology guideline for the management of gout. Arthritis Rheumatol (2020) 72:879–95. doi: 10.1002/art.41247
7. Tin A, Li Y, Brody JA, Nutile T, Chu AY, Huffman JE, et al. Large-Scale whole-exome sequencing association studies identify rare functional variants influencing serum urate levels. Nat Commun (2018) 9:4228. doi: 10.1038/s41467-018-06620-4
8. Cai Y, Yu X, Hu S, Yu J. A brief review on the mechanisms of miRNA regulation. Genomics Proteomics Bioinf (2009) 7:147–54. doi: 10.1016/S1672-0229(08)60044-3
9. Cheng J, Roden CA, Pan W, Zhu S, Baccei A, Pan X, et al. A molecular chipper technology for CRISPR sgRNA library generation and functional mapping of noncoding regions. Nat Commun (2016) 7:11178. doi: 10.1038/ncomms11178
10. Suzuki HI, Katsura A, Miyazono K. A role of uridylation pathway for blockade of let-7 microRNA biogenesis by Lin28B. Cancer Sci (2015) 106:1174–81. doi: 10.1111/cas.12721
11. Chen GR, Sive H, Bartel DP. A seed mismatch enhances Argonaute2-catalyzed cleavage and partially rescues severely impaired cleavage found in fish. Mol Cell (2017) 68:1095–107 e5. doi: 10.1016/j.molcel.2017.11.032
12. Bronisz A, Rooj AK, Krawczynski K, Peruzzi P, Salinska E, Nakano I, et al. The nuclear DICER-circular RNA complex drives the deregulation of the glioblastoma cell microRNAome. Sci Adv (2020) 6(51):eabc0221. doi: 10.1126/sciadv.abc0221
13. Grasso G, Higuchi T, Mac V, Barbier J, Helsmoortel M, Lorenzi C, et al. NF90 modulates processing of a subset of human pri-miRNAs. Nucleic Acids Res (2020) 48:6874–88. doi: 10.1093/nar/gkaa386
14. Londin E, Loher P, Telonis AG, Quann K, Clark P, Jing Y, et al. Analysis of 13 cell types reveals evidence for the expression of numerous novel primate- and tissue-specific microRNAs. Proc Natl Acad Sci U S A. (2015) 112:E1106–15. doi: 10.1073/pnas.1420955112
15. Papanagnou P, Stivarou T, Tsironi M. The role of mirnas in common inflammatory arthropathies: osteoarthritis and gouty arthritis. Biomolecules. (2016) 6(4):44. doi: 10.3390/biom6040044
16. Alevizos I, Illei GG. MicroRNAs as biomarkers in rheumatic diseases. Nat Rev Rheumatol (2010) 6:391–8. doi: 10.1038/nrrheum.2010.81
17. Richette P, Doherty M, Pascual E, Barskova V, Becce F, Castaneda J, et al. 2018 Updated european league against rheumatism evidence-based recommendations for the diagnosis of gout. Ann Rheum Dis (2020) 79:31–8. doi: 10.1136/annrheumdis-2019-215315
18. Ragab G, Elshahaly M, Bardin T. Gout: An old disease in new perspective - a review. J Adv Res (2017) 8:495–511. doi: 10.1016/j.jare.2017.04.008
19. Kim JW, Kwak SG, Lee H, Kim SK, Choe JY, Park SH. Prevalence and incidence of gout in Korea: data from the national health claims database 2007-2015. Rheumatol Int (2017) 37:1499–506. doi: 10.1007/s00296-017-3768-4
20. Dehlin M, Drivelegka P, Sigurdardottir V, Svärd A, Jacobsson LT. Incidence and prevalence of gout in Western Sweden. Arthritis Res Ther (2016) 18:164. doi: 10.1186/s13075-016-1062-6
21. Dalbeth N, Stamp L. Hyperuricaemia and gout: time for a new staging system? Ann Rheum Dis (2014) 73:1598–600. doi: 10.1136/annrheumdis-2014-205304
22. Han M, Park HC, Kim H, Jo HA, Huh H, Jang JY, et al. Hyperuricemia and deterioration of renal function in autosomal dominant polycystic kidney disease. BMC Nephrol (2014) 15:63. doi: 10.1186/1471-2369-15-63
23. Vitart V, Rudan I, Hayward C, Gray NK, Floyd J, Palmer CN, et al. SLC2A9 is a newly identified urate transporter influencing serum urate concentration, urate excretion and gout. Nat Genet (2008) 40:437–42. doi: 10.1038/ng.106
24. Ekaratanawong S, Anzai N, Jutabha P, Miyazaki H, Noshiro R, Takeda M, et al. Human organic anion transporter 4 is a renal apical organic anion/dicarboxylate exchanger in the proximal tubules. J Pharmacol Sci (2004) 94:297–304. doi: 10.1254/jphs.94.297
25. Van Aubel RA, Smeets PH, van den Heuvel JJ, Russel FG. Human organic anion transporter MRP4 (ABCC4) is an efflux pump for the purine end metabolite urate with multiple allosteric substrate binding sites. Am J Physiol Renal Physiol (2005) 288:F327–33. doi: 10.1152/ajprenal.00133.2004
26. Woodward OM, Köttgen A, Coresh J, Boerwinkle E, Guggino WB, Köttgen M. Identification of a urate transporter, ABCG2, with a common functional polymorphism causing gout. Proc Natl Acad Sci U S A. (2009) 106:10338–42. doi: 10.1073/pnas.0901249106
27. Choi HK, Atkinson K, Karlson EW, Willett W, Curhan G. Purine-rich foods, dairy and protein intake, and the risk of gout in men. N Engl J Med (2004) 350:1093–103. doi: 10.1056/NEJMoa035700
28. Choi HK, Atkinson K, Karlson EW, Willett W, Curhan G. Alcohol intake and risk of incident gout in men: a prospective study. Lancet. (2004) 363:1277–81. doi: 10.1016/S0140-6736(04)16000-5
29. Cronstein BN, Sunkureddi P. Mechanistic aspects of inflammation and clinical management of inflammation in acute gouty arthritis. J Clin Rheumatol (2013) 19:19–29. doi: 10.1097/RHU.0b013e31827d8790
30. Martinon F, Pétrilli V, Mayor A, Tardivel A, Tschopp J. Gout-associated uric acid crystals activate the NALP3 inflammasome. Nature. (2006) 440:237–41. doi: 10.1038/nature04516
31. Liu-Bryan R, Scott P, Sydlaske A, Rose DM, Terkeltaub R. Innate immunity conferred by toll-like receptors 2 and 4 and myeloid differentiation factor 88 expression is pivotal to monosodium urate monohydrate crystal-induced inflammation. Arthritis Rheumatol (2005) 52:2936–46. doi: 10.1002/art.21238
32. Joosten LA, Netea MG, Mylona E, Koenders MI, Malireddi RK, Oosting M, et al. Engagement of fatty acids with toll-like receptor 2 drives interleukin-1β production via the ASC/caspase 1 pathway in monosodium urate monohydrate crystal-induced gouty arthritis. Arthritis Rheumatol (2010) 62:3237–48. doi: 10.1002/art.27667
33. Vieira AT, Macia L, Galvão I, Martins FS, Canesso MC, Amaral FA, et al. A role for gut microbiota and the metabolite-sensing receptor GPR43 in a murine model of gout. Arthritis Rheumatol (2015) 67:1646–56. doi: 10.1002/art.39107
34. So AK, Martinon F. Inflammation in gout: mechanisms and therapeutic targets. Nat Rev Rheumatol (2017) 13:639–47. doi: 10.1038/nrrheum.2017.155
35. Bauernfeind FG, Horvath G, Stutz A, Alnemri ES, MacDonald K, Speert D, et al. Cutting edge: NF-kappaB activating pattern recognition and cytokine receptors license NLRP3 inflammasome activation by regulating NLRP3 expression. J Immunol (2009) 183:787–91. doi: 10.4049/jimmunol.0901363
36. Martinon F, Burns K, Tschopp J. The inflammasome: a molecular platform triggering activation of inflammatory caspases and processing of proIL-beta. Mol Cell (2002) 10:417–26. doi: 10.1016/S1097-2765(02)00599-3
37. Schauer C, Janko C, Munoz LE, Zhao Y, Kienhöfer D, Frey B, et al. Aggregated neutrophil extracellular traps limit inflammation by degrading cytokines and chemokines. Nat Med (2014) 20:511–7. doi: 10.1038/nm.3547
38. Sil P, Wicklum H, Surell C, Rada B. Macrophage-derived IL-1β enhances monosodium urate crystal-triggered NET formation. Inflammation Res (2017) 66:227–37. doi: 10.1007/s00011-016-1008-0
39. Palmer DG, Highton J, Hessian PA. Development of the gout tophus. hypothesis Am J Clin Pathol (1989) 91:190–5. doi: 10.1093/ajcp/91.2.190
40. Dalbeth N, Clark B, Gregory K, Gamble G, Sheehan T, Doyle A, et al. Mechanisms of bone erosion in gout: a quantitative analysis using plain radiography and computed tomography. Ann Rheum Dis (2009) 68:1290–5. doi: 10.1136/ard.2008.094201
41. Wang Y, Xu D, Wang B, Hou X. Could MicroRNAs be regulators of gout pathogenesis? Cell Physiol Biochem (2015) 36:2085–92. doi: 10.1159/000430176
42. Xu YT, Leng YR, Liu MM, Dong RF, Bian J, Yuan LL, et al. MicroRNA and long noncoding RNA involvement in gout and prospects for treatment. Int Immunopharmacol (2020) 87:106842. doi: 10.1016/j.intimp.2020.106842
44. Anzai N, Jutabha P, Amonpatumrat-Takahashi S, Sakurai H. Recent advances in renal urate transport: characterization of candidate transporters indicated by genome-wide association studies. Clin Exp Nephrol (2012) 16:89–95. doi: 10.1007/s10157-011-0532-z
45. Kawamura Y, Nakaoka H, Nakayama A, Okada Y, Yamamoto K, Higashino T, et al. Genome-wide association study revealed novel loci which aggravate asymptomatic hyperuricaemia into gout. Ann Rheum Dis (2019) 78:1430–7. doi: 10.1136/annrheumdis-2019-215521
46. Dalbeth N, Pool B, Shaw OM, Harper JL, Tan P, Franklin C, et al. Role of miR-146a in regulation of the acute inflammatory response to monosodium urate crystals. Ann Rheum Dis (2015) 74:786–90. doi: 10.1136/annrheumdis-2014-205409
47. Li X, Pan Y, Li W, Guan P, You C. The role of noncoding rnas in gout. Endocrinology. (2020) 161(11):bqaa165. doi: 10.1210/endocr/bqaa165
48. Jin HM, Kim TJ, Choi JH, Kim MJ, Cho YN, Nam KI, et al. MicroRNA-155 as a proinflammatory regulator via SHIP-1 down-regulation in acute gouty arthritis. Arthritis Res Ther (2014) 16:R88. doi: 10.1186/ar4531
49. Bussler S, Penke M, Flemming G, Elhassan YS, Kratzsch J, Sergeyev E, et al. Novel insights in the metabolic syndrome in childhood and adolescence. Horm Res Paediatr (2017) 88:181–93. doi: 10.1159/000479510
50. Ripperger A, Benndorf RA. The C421A (Q141K) polymorphism enhances the 3’-untranslated region (3’-UTR)-dependent regulation of ATP-binding cassette transporter ABCG2. Biochem Pharmacol (2016) 104:139–47. doi: 10.1016/j.bcp.2016.02.011
51. Sun WF, Zhu MM, Li J, Zhang XX, Liu YW, Wu XR, et al. Effects of xie-Zhuo-Chu-Bi-Fang on miR-34a and URAT1 and their relationship in hyperuricemic mice. J Ethnopharmacol (2015) 161:163–9. doi: 10.1016/j.jep.2014.12.001
52. Zhou Z, Dong Y, Zhou H, Liu J, Zhao W. MiR-143-3p directly targets GLUT9 to reduce uric acid reabsorption and inflammatory response of renal tubular epithelial cells. Biochem Biophys Res Commun (2019) 517:413–20. doi: 10.1016/j.bbrc.2019.07.114
53. Estevez-Garcia IO, Gallegos-Nava S, Vera-Pérez E, Silveira LH, Ventura-Ríos L, Vancini G, et al. Levels of cytokines and micrornas in individuals with asymptomatic hyperuricemia and ultrasonographic findings of gout: a bench-to-bedside approach. Arthritis Care Res (Hoboken) (2018) 70:1814–21. doi: 10.1002/acr.23549
54. Zhang X, Hong Q, Hou K, Wang Y, Wu D, Chen X. [High concentration uric acid regulates endothelial function via miR-155]. Nan Fang Yi Ke Da Xue Xue Bao. (2013) 33:1141–5.
55. Yu S, Hong Q, Wang Y, Hou K, Wang L, Zhang Y, et al. High concentrations of uric acid inhibit angiogenesis via regulation of the krüppel-like factor 2-vascular endothelial growth factor-a axis by miR-92a. Circ J (2015) 79:2487–98. doi: 10.1253/circj.CJ-15-0283
56. Hong Q, Yu S, Geng X, Duan L, Zheng W, Fan M, et al. High concentrations of uric acid inhibit endothelial cell migration via mir-663 which regulates phosphatase and tensin homolog by targeting transforming growth factor-β1. Microcirculation. (2015) 22:306–14. doi: 10.1111/micc.12200
57. Chen LL, Xu Y. Epigallocatechin gallate attenuates uric acid-induced injury in rat renal interstitial fibroblasts NRK-49F by up-regulation of miR-9. Eur Rev Med Pharmacol Sci (2018) 22:7458–69. doi: 10.26355/eurrev_201811_16287
58. Chen S, Chen D, Yang H, Wang X, Wang J, Xu C. Uric acid induced hepatocytes lipid accumulation through regulation of miR-149-5p/FGF21 axis. BMC Gastroenterol (2020) 20:39. doi: 10.1186/s12876-020-01189-z
59. Chi K, Geng X, Liu C, Zhang Y, Cui J, Cai G, et al. LncRNA-HOTAIR promotes endothelial cell pyroptosis by regulating the miR-22/NLRP3 axis in hyperuricaemia. J Cell Mol Med (2021) 25:8504–21. doi: 10.1111/jcmm.16812
60. Zhong X, Chen Y, Yao C, Xu L, Peng Y, Yang Q, et al. MicroRNA-30b participates in the pathological process of hyperuricemia by regulating interleukin-6 receptor. Nucleosides Nucleotides Nucleic Acids (2020) 39:1162–78. doi: 10.1080/15257770.2020.1780439
61. Stottmeier B, Dick TP. Redox sensitivity of the MyD88 immune signaling adapter. Free Radic Biol Med (2016) 101:93–101. doi: 10.1016/j.freeradbiomed.2016.10.004
62. Wang Y, Ma H, Li Y, Su R. MiR-192-5p-modified tumor-associated macrophages-derived exosome suppressed endometrial cancer progression through targeting irak1/nf-kappab signaling. Reprod Sci (2022) 29:436–47. doi: 10.1007/s43032-021-00789-8
63. Doukas SG, Vageli DP, Sasaki CT. NF-kappaB inhibition reverses acidic bile-induced miR-21, miR-155, miR-192, miR-34a, miR-375 and miR-451a deregulations in human hypopharyngeal cells. J Cell Mol Med (2018) 22:2922–34. doi: 10.1111/jcmm.13591
64. Lian C, Sun J, Guan W, Zhang L, Zhang X, Yang L, et al. Circular rna circhipk3 activates macrophage nlrp3 inflammasome and tlr4 pathway in gouty arthritis via sponging mir-561 and mir-192. Inflammation. (2021) 44:2065–77. doi: 10.1007/s10753-021-01483-2
65. Gao M, Wang X, Zhang X, Ha T, Ma H, Liu L, et al. Attenuation of cardiac dysfunction in polymicrobial sepsis by microrna-146a is mediated via targeting of irak1 and traf6 expression. J Immunol (2015) 195:672–82. doi: 10.4049/jimmunol.1403155
66. Chen X, Gao Q, Zhou L, Wang Y, Sun RR, Zhang ZY. MiR-146a alleviates inflammation of acute gouty arthritis rats through TLR4/MyD88 signal transduction pathway. Eur Rev Med Pharmacol Sci (2019) 23:9230–7. doi: 10.26355/eurrev_201911_19415
67. Zhang QB, Qing YF, Yin CC, Zhou L, Liu XS, Mi QS, et al. Mice with miR-146a deficiency develop severe gouty arthritis via dysregulation of TRAF 6, IRAK 1 and NALP3 inflammasome. Arthritis Res Ther (2018) 20:45. doi: 10.1186/s13075-018-1546-7
68. Ma T, Liu X, Cen Z, Xin C, Guo M, Zou C, et al. MicroRNA-302b negatively regulates IL-1β production in response to MSU crystals by targeting IRAK4 and EphA2. Arthritis Res Ther (2018) 20:34. doi: 10.1186/s13075-018-1528-9
69. Schroder K, Tschopp J. The inflammasomes. Cell. (2010) 140:821–32. doi: 10.1016/j.cell.2010.01.040
70. Kingsbury SR, Conaghan PG, McDermott MF. The role of the NLRP3 inflammasome in gout. J Inflammation Res (2011) 4:39–49. doi: 10.2147/JIR.S11330
71. Renaudin F, Orliaguet L, Castelli F, Fenaille F, Prignon A, Alzaid F, et al. Gout and pseudo-gout-related crystals promote GLUT1-mediated glycolysis that governs NLRP3 and interleukin-1beta activation on macrophages. Ann Rheum Dis (2020) 79:1506–14. doi: 10.1136/annrheumdis-2020-217342
72. Chen S, Sun B. Negative regulation of NLRP3 inflammasome signaling. Protein Cell (2013) 4:251–8. doi: 10.1007/s13238-013-2128-8
73. Liu YF, Xing GL, Chen Z, Tu SH. Long non-coding RNA HOTAIR knockdown alleviates gouty arthritis through miR-20b upregulation and NLRP3 downregulation. Cell Cycle (2021) 20:332–44. doi: 10.1080/15384101.2021.1874696
74. Wang X, Chi J, Dong B, Xu L, Zhou Y, Huang Y, et al. MiR-223-3p and miR-22-3p inhibit monosodium urate-induced gouty inflammation by targeting NLRP3. Int J Rheum Dis (2021) 24:599–607. doi: 10.1111/1756-185X.14089
75. Yang QB, Li LQ, Zhang QB, He YL, Mi QS, Zhou JG. microRNA-223 deficiency exacerbates acute inflammatory response to monosodium urate crystals by targeting nlrp3. J Inflammation Res (2021) 14:1845–58. doi: 10.2147/JIR.S307796
76. Tian J, Zhou D, Xiang L, Liu X, Zhang H, Wang B, et al. MiR-223-3p inhibits inflammation and pyroptosis in monosodium urate-induced rats and fibroblast-like synoviocytes by targeting NLRP3. Clin Exp Immunol (2021) 204:396–410. doi: 10.1111/cei.13587
77. Wang Y. Tripterine ameliorates monosodium urate crystal-induced gouty arthritis by altering macrophage polarization via the miR-449a/NLRP3 axis. Inflammation Res (2021) 70:323–41. doi: 10.1007/s00011-021-01439-0
78. Meng Q, Meng W, Bian H, Zheng F, Gu H, Zuo R, et al. Total glucosides of paeony protects THP-1 macrophages against monosodium urate-induced inflammation via MALAT1/miR-876-5p/NLRP3 signaling cascade in gouty arthritis. BioMed Pharmacother (2021) 138:111413. doi: 10.1016/j.biopha.2021.111413
79. An L, Yin F. MiR-192-5p suppresses M1 macrophage polarization via epiregulin (EREG) downregulation in gouty arthritis. Tissue Cell (2021) 73:101669. doi: 10.1016/j.tice.2021.101669
80. Zhou W, Wang Y, Wu R, He Y, Su Q, Shi G. MicroRNA-488 and -920 regulate the production of proinflammatory cytokines in acute gouty arthritis. Arthritis Res Ther (2017) 19:203. doi: 10.1186/s13075-017-1418-6
81. Li G, Zhang H, Ma H, Qu S, Xing Q, Wang G. MiR-221-5p is involved in the regulation of inflammatory responses in acute gouty arthritis by targeting IL-1β. Int J Rheum Dis (2021) 24:335–40. doi: 10.1111/1756-185X.14028
82. Yang Q, Zhang Q, Qing Y, Zhou L, Mi Q, Zhou J. miR-155 is dispensable in monosodium urate-induced gouty inflammation in mice. Arthritis Res Ther (2018) 20:144. doi: 10.1186/s13075-018-1550-y
83. Shan L, Yang D, Feng F, Zhu D, Li X. miR-3146 induces neutrophil extracellular traps to aggravate gout flare. J Clin Lab Anal (2021) 35:e24032. doi: 10.1002/jcla.24032
84. Dalbeth N, Smith T, Nicolson B, Clark B, Callon K, Naot D, et al. Enhanced osteoclastogenesis in patients with tophaceous gout: urate crystals promote osteoclast development through interactions with stromal cells. Arthritis Rheumatol (2008) 58:1854–65. doi: 10.1002/art.23488
85. Chhana A, Callon KE, Pool B, Naot D, Watson M, Gamble GD, et al. Monosodium urate monohydrate crystals inhibit osteoblast viability and function: implications for development of bone erosion in gout. Ann Rheum Dis (2011) 70:1684–91. doi: 10.1136/ard.2010.144774
86. Chhana A, Pool B, Callon KE, Tay ML, Musson D, Naot D, et al. Monosodium urate crystals reduce osteocyte viability and indirectly promote a shift in osteocyte function towards a proinflammatory and proresorptive state. Arthritis Res Ther (2018) 20:208. doi: 10.1186/s13075-018-1704-y
87. Kong XH, Shi SF, Hu HJ, Wang JX. MicroRNA-20a suppresses RANKL-modulated osteoclastogenesis and prevents bone erosion in mice with rheumatoid arthritis through the TLR4/p38 pathway. J Biol Regul Homeost Agents (2021) 35:921–31. doi: 10.23812/20-604-A
88. Sujitha S, Dinesh P, Rasool M. Berberine encapsulated PEG-coated liposomes attenuate Wnt1/β-catenin signaling in rheumatoid arthritis via miR-23a activation. Eur J Pharm Biopharm (2020) 149:170–91. doi: 10.1016/j.ejpb.2020.02.007
89. Najm A, Masson FM, Preuss P, Georges S, Ory B, Quillard T, et al. MicroRNA-17-5p reduces inflammation and bone erosions in mice with collagen-induced arthritis and directly targets the JAK/STAT pathway in rheumatoid arthritis fibroblast-like synoviocytes. Arthritis Rheumatol (2020) 72:2030–9. doi: 10.1002/art.41441
90. Katsuura S, Kuwano Y, Yamagishi N, Kurokawa K, Kajita K, Akaike Y, et al. MicroRNAs miR-144/144* and miR-16 in peripheral blood are potential biomarkers for naturalistic stress in healthy Japanese medical students. Neurosci Lett (2012) 516:79–84. doi: 10.1016/j.neulet.2012.03.062
91. Ritter A, Hirschfeld M, Berner K, Jaeger M, Grundner-Culemann F, Schlosser P, et al. Discovery of potential serum and urine-based microRNA as minimally-invasive biomarkers for breast and gynecological cancer. Cancer biomark (2020) 27:225–42. doi: 10.3233/CBM-190575
92. Cressatti M, Juwara L, Galindez JM, Velly AM, Nkurunziza ES, Marier S, et al. Salivary microR-153 and microR-223 levels as potential diagnostic biomarkers of idiopathic parkinson’s disease. Mov Disord (2020) 35:468–77. doi: 10.1002/mds.27935
93. Bohatá J, Horváthová V, Pavlíková M, Stibůrková B. Circulating microRNA alternations in primary hyperuricemia and gout. Arthritis Res Ther (2021) 23:186. doi: 10.1186/s13075-021-02569-w
94. Roddy E, Clarkson K, Blagojevic-Bucknall M, Mehta R, Oppong R, Avery A, et al. Open-label randomised pragmatic trial (CONTACT) comparing naproxen and low-dose colchicine for the treatment of gout flares in primary care. Ann Rheum Dis (2020) 79:276–84. doi: 10.1136/annrheumdis-2019-216154
95. Rainer TH, Cheng CH, Janssens HJ, Man CY, Tam LS, Choi YF, et al. Oral prednisolone in the treatment of acute gout: A pragmatic, multicenter, double-blind, randomized trial. Ann Intern Med (2016) 164:464–71. doi: 10.7326/M14-2070
96. Janssens HJ, Janssen M, van de Lisdonk EH, van Riel PL, van Weel C. Use of oral prednisolone or naproxen for the treatment of gout arthritis: a double-blind, randomised equivalence trial. Lancet. (2008) 371:1854–60. doi: 10.1016/S0140-6736(08)60799-0
97. Man CY, Cheung IT, Cameron PA, Rainer TH. Comparison of oral prednisolone/paracetamol and oral indomethacin/paracetamol combination therapy in the treatment of acute goutlike arthritis: a double-blind, randomized, controlled trial. Ann Emerg Med (2007) 49:670–7. doi: 10.1016/j.annemergmed.2006.11.014
98. Janssen CA, Oude Voshaar MAH, Vonkeman HE, Jansen T, Janssen M, Kok MR, et al. Anakinra for the treatment of acute gout flares: a randomized, double-blind, placebo-controlled, active-comparator, non-inferiority trial. Rheumatol (Oxford) (2019) 8:8. doi: 10.1093/rheumatology/key402
99. Schlesinger N, Alten RE, Bardin T, Schumacher HR, Bloch M, Gimona A, et al. Canakinumab for acute gouty arthritis in patients with limited treatment options: results from two randomised, multicentre, active-controlled, double-blind trials and their initial extensions. Ann Rheum Dis (2012) 71:1839–48. doi: 10.1136/annrheumdis-2011-200908
100. White WB, Saag KG, Becker MA, Borer JS, Gorelick PB, Whelton A, et al. Cardiovascular safety of febuxostat or allopurinol in patients with gout. N Engl J Med (2018) 378:1200–10. doi: 10.1056/NEJMoa1710895
101. Reinders MK, Haagsma C, Jansen TL, van Roon EN, Delsing J, van de Laar MA, et al. A randomised controlled trial on the efficacy and tolerability with dose escalation of allopurinol 300-600 mg/day versus benzbromarone 100-200 mg/day in patients with gout. Ann Rheum Dis (2009) 68:892–7. doi: 10.1136/ard.2008.091462
102. Reinders MK, van Roon EN, Jansen TL, Delsing J, Griep EN, Hoekstra M, et al. Efficacy and tolerability of urate-lowering drugs in gout: a randomised controlled trial of benzbromarone versus probenecid after failure of allopurinol. Ann Rheum Dis (2009) 68:51–6. doi: 10.1136/ard.2007.083071
103. Liu P, Chen Y, Wang B, Wang Z, Li C, Wang Y. Expression of microRNAs in the plasma of patients with acute gouty arthritis and the effects of colchicine and etoricoxib on the differential expression of microRNAs. Arch Med Sci (2019) 15:1047–55. doi: 10.5114/aoms.2018.75502
104. Fan S, Huang Y, Lu G, Sun N, Wang R, Lu C, et al. Novel anti-hyperuricemic hexapeptides derived from apostichopus japonicus hydrolysate and their modulation effects on the gut microbiota and host microRNA profile. Food Funct (2022) 13(7):3865–78. doi: 10.1039/D1FO03981D
105. Martin WJ, Herst PM, Chia EW, Harper JL. Sesquiterpene dialdehydes inhibit MSU crystal-induced superoxide production by infiltrating neutrophils in an in vivo model of gouty inflammation. Free Radic Biol Med (2009) 47:616–21. doi: 10.1016/j.freeradbiomed.2009.05.035
106. Wang Y, Dong L, Liu P, Chen Y, Jia S, Wang Y. A randomized controlled trial of chuanhutongfeng mixture for the treatment of chronic gouty arthritis by regulating miRNAs. Evid Based Complement Alternat Med (2019) 2019:5917269. doi: 10.1155/2019/5917269
107. Sun WF, Zhang XX, Sun FY, Xu W, Liang J, Feng SM, et al. MicroRNA expression patterns of the kidney in hyperuricemia mice treated with xiezhuo chubi decoction. Chin J Integr Med (2011) 17:35–42. doi: 10.1007/s11655-011-0605-6
108. Li X, Liu Y, Shan Y, Wang Y, Li Z, Bi Y, et al. MicroRNAs involved in the therapeutic functions of noni (Morinda citrifolia l.) fruit juice in the treatment of acute gouty arthritis in mice induced with monosodium urate. Foods (2021) 10(7):1638. doi: 10.3390/foods10071638
109. Jiang K, Yang J, Guo S, Zhao G, Wu H, Deng G. Peripheral circulating exosome-mediated delivery of mir-155 as a novel mechanism for acute lung inflammation. Mol Ther (2019) 27:1758–71. doi: 10.1016/j.ymthe.2019.07.003
110. Cao Y, Wang Z, Yan Y, Ji L, He J, Xuan B, et al. Enterotoxigenic bacteroidesfragilis promotes intestinal inflammation and malignancy by inhibiting exosome-packaged mir-149-3p. Gastroenterology. (2021) 161:1552–66 e12. doi: 10.1053/j.gastro.2021.08.003
111. Bouchareychas L, Duong P, Covarrubias S, Alsop E, Phu TA, Chung A, et al. Macrophage exosomes resolve atherosclerosis by regulating hematopoiesis and inflammation via microrna cargo. Cell Rep (2020) 32:107881. doi: 10.1016/j.celrep.2020.107881
112. Wang F, Zhang L, Bai X, Cao X, Jiao X, Huang Y, et al. Stimuli-responsive nanocarrier for co-delivery of mir-31 and doxorubicin to suppress high mtef4 cancer. ACS Appl Mater Interfaces (2018) 10:22767–75. doi: 10.1021/acsami.8b07698
113. Ahir M, Upadhyay P, Ghosh A, Sarker S, Bhattacharya S, Gupta P, et al. Delivery of dual miRNA through CD44-targeted mesoporous silica nanoparticles for enhanced and effective triple-negative breast cancer therapy. Biomater Sci (2020) 8:2939–54. doi: 10.1039/D0BM00015A
114. Zhou W, Xu Y, Zhang J, Zhang P, Yao Z, Yan Z, et al. MiRNA-363-3p/DUSP10/JNK axis mediates chemoresistance by enhancing DNA damage repair in diffuse large b-cell lymphoma. Leukemia (2022) 36(7):1861–9. doi: 10.1038/s41375-022-01565-6
Keywords: MicroRNA, gouty arthritis, hyperuricemia, cellular signaling pathway, biomarker
Citation: Luo Z, Yang F, Hong S, Wang J, Chen B, Li L, Yang J, Yao Y, Yang C, Hu Y, Wang S, Xu T and Wu J (2022) Role of microRNA alternation in the pathogenesis of gouty arthritis. Front. Endocrinol. 13:967769. doi: 10.3389/fendo.2022.967769
Received: 13 June 2022; Accepted: 19 July 2022;
Published: 11 August 2022.
Edited by:
Isabelle Jéru, Assistance Publique Hopitaux De Paris, FranceReviewed by:
Fengjie Sun, Georgia Gwinnett College, United StatesMaojie Wang, Guangdong Provincial Hospital of Chinese Medicine, China
Copyright © 2022 Luo, Yang, Hong, Wang, Chen, Li, Yang, Yao, Yang, Hu, Wang, Xu and Wu. This is an open-access article distributed under the terms of the Creative Commons Attribution License (CC BY). The use, distribution or reproduction in other forums is permitted, provided the original author(s) and the copyright owner(s) are credited and that the original publication in this journal is cited, in accordance with accepted academic practice. No use, distribution or reproduction is permitted which does not comply with these terms.
*Correspondence: Tao Xu, xutao@ahmu.edu.cn; Jun Wu, wujunahslyy@163.com
†These authors have contributed equally to this work