- 1Key Laboratory for Space Bioscience and Biotechnology, School of Life Sciences, Northwestern Polytechnical University, Xi’an, China
- 2Xi’an International Medical Center Hospital Affiliated to Northwest University, Xi’an, China
- 3Department of Physical Education, Northwestern Polytechnical University, Xi’an, China
- 4Research Center of Special Environmental Biomechanics and Medical Engineering, Northwestern Polytechnical University, Xi’an, China
Irisin, out-membrane part of fibronectin type III domain–containing 5 protein (FNDC5), was activated by Peroxisome proliferator-activated receptor γ (PPARγ) coactivator-1α (PGC-1α) during physical exercise in skeletal muscle tissues. Most studies have reported that the concentration of irisin is highly associated with health status. For instance, the level of irisin is significantly lower in patients with obesity, osteoporosis/fractures, muscle atrophy, Alzheimer’s disease, and cardiovascular diseases (CVDs) but higher in patients with cancer. Irisin can bind to its receptor integrin αV/β5 to induce browning of white fat, maintain glucose stability, keep bone homeostasis, and alleviate cardiac injury. However, it is unclear whether it works by directly binding to its receptors to regulate muscle regeneration, promote neurogenesis, keep liver glucose homeostasis, and inhibit cancer development. Supplementation of recombinant irisin or exercise-activated irisin might be a successful strategy to fight obesity, osteoporosis, muscle atrophy, liver injury, and CVDs in one go. Here, we summarize the publications of FNDC5/irisin from PubMed/Medline, Scopus, and Web of Science until March 2022, and we review the role of FNDC5/irisin in physiology and pathology.
1 Introduction
The protein sequence of fibronectin type III domain–containing 5 protein (FNDC5) contains a signal peptide [for endoplasmic reticulum (ER) targeting nascent FNDC5] (1), a hydrophobic transmembrane domain, a fibronectin III domain (the main part of irisin in the extracellular), and a carboxyterminal domain in the cytoplasm. After being N-glycosylated at the two potential sites—Asn36 and Asn81 (mouse) (2) or Asn7 and Asn52 (human) (3) in the ER—and cleaved by disintegrin and metallopeptidase domain (ADAM) family proteins such as ADAM10 (4), irisin is secreted to the blood circulation.
The physiological role of irisin in inducing thermogenic beige fat genesis to control energy metabolism was first described by Spiegelman and his teammates in 2012 in Nature (5). After that, irisin has also been found to promote liver glycogen synthesis and inhibit liver gluconeogenesis to maintain glucose homeostasis (6, 7). Later, the function of irisin in nerve system was found in improving cognition, learning, and memory (8). Moreover, irisin also contributes to maintaining musculoskeletal homeostasis by binding with integrin αVβ5 (9, 10). In recent years, researchers have also revealed that irisin reduces the risk of cancers (11) and cardiovascular diseases (CVDs) (12).
In this review, we summarized the up-to-date publications on irisin. We hope that it will help to understand the mechanisms of irisin and provide clues for the clinical application of irisin in diseases.
2.1. Role of irisin in inducing white fat browning
The upregulation of FNDC5/irisin under aerobic exercise (5, 8, 13) or cold-induced shivering (14) induces the “browning” of white fat via increasing the expression of thermogenic genes such as Prdm16, Dio2, cidea, Cox-7a, PGC-1α, and UCP-1 in white fat. The activated beige fat dissipated energy in the form of heat by absorbing the excessive energy substrates (fatty acid or glucose), which improved obesity and type 2 diabetes mellitus (T2DM). On the basis of the single-cell RNA-seq method, Kajimura and his workmates revealed that irisin induced adipocyte progenitor cells (APCs) toward de novo beige fat biogenesis and proliferation by activating a complex of CD81 and αVβ1/β5 integrins to phosphorylate Focal adhesion kinase 1 (FAK, Tyr397) signaling (15, 16). Moreover, injection of recombinant irisin (r-irisin; 0.5 mg/g/day) into diet-induced obese (DIO) mice for 14 days resulted in activating thermogenic genes expression and browning of subcutaneous white fat, thereby reducing body weight and improving glucose metabolism (3). In vitro studies suggested that irisin induced the expression of UCP1 and other thermogenic genes in primary inguinal adipocytes and 3T3-L1 preadipocytes through activating p38 mitogen-activated protein kinase (p38 MAPK) and extracellular signal–related kinase 1/2 (ERK1/2) signaling pathways (3). Inhibition of P38 and ERK1/2 expression could block the upregulation of Uncoupling protein 1 (UCP-1) by irisin, which was called “Irisin ERKs the Fat” by Wu et al. (17). In conclusion, irisin can promote the proliferation and differentiation of beige APCs and the browning of mature white fat.
Most studies have shown that irisin expression is significantly lower in individuals with DIO or T2DM than normal individuals (6, 18–20). However, there are also opposite results, for instance, the concentration of irisin in the blood of obese individuals is higher than that of thin individuals (normal) (21–23) under sedentary conditions. A meta-analysis with 1,005 obese patients and 1,242 control subjects showed that obese individuals had a higher circulating irisin level (24). In the development of obesity, the high level of irisin was probably derived from the increased in white adipose tissue (WAT) (25), as the expression of FNDC5/irisin was decreased in muscle tissues (26), as well as in brown adipose tissue and other tissues. However, if irisin concentration is indeed elevated in obese individuals, then why is not irisin doing its job of burning and “ERK-ing” the fat? In obese adipose tissues, is it reduced in the expression of its receptor αVβ1/β5 integrins or the sensitivity? This controversial phenomenon was also assumed as “irisin resistance”. Clearly, these questions require further exploration in the future.
2.2. Role of irisin in the liver
The liver is the main site of gluconeogenesis and glycogenesis to maintain energy metabolism. Irisin expression was significantly reduced in subjects with steatohepatitis (6, 18, 19) or in mouse models of ischemia-reperfusion (I/R)–induced liver injury (27, 28). Long-term exercise-induced irisin or supplementation of exogenous r-irisin could protect the liver from non-alcoholic fatty liver disease (NAFLD) (6, 7), liver glucose disorder (29, 30), or I/R-induced liver injury (31), which embodied the potential role of irisin in muscle-liver cross-talk (32).
Hong et al. (33) revealed that injection of r-irisin into DIO mice for 2 weeks inhibited hepatic cholesterol synthesis via activating Adenosine 5‘-monophosphate (AMP)-activated protein kinase (AMPK, Thr172) and inhibiting sterol regulatory element-binding transcription factor 2 (SREBP2) expression. Hepatic glucose homeostasis is closely related to hepatic gluconeogenesis and glycogen synthesis. Studies have found that irisin reduces the expression of phosphoenolpyruvate carboxykinase (PEPCK) and glucose-6-phosphatase (G6Pase)–mediated gluconeogenesis in the liver, which can be weakened by suppressing AMPK Small interfering RNA (AMPK siRNA), suggesting that irisin inhibits gluconeogenesis through activating AMPK-PEPCK/G6Pase pathway (30). Similar results also revealed that irisin inhibited glucosamine (GlcN) or palmitate-induced primary hepatocyte insulin resistance by activating phosphatidylinositol 3-kinase (PI3K)/Protein kinase B (Akt)/forkhead box protein O1 (FOXO1)–mediated PEPCK and G6Pase; meanwhile, irisin augments liver glycogenesis through PI3K/Akt/GSK3–glycogen synthase (GS) signaling pathway (29). A recent study has revealed that exercise-induced irisin competitively inhibits the binding of myeloid differentiation factor 2 (MD2) and Toll-like receptor 4 by forming a complex with MD2 in liver cells and thus inhibits the inflammatory response, which may contribute to the improvement of NAFLD by reducing liver steatosis and fibrosis through exercise (34).
I/R is a leading reason of liver injury after liver resection or transplantation (35), which highly associated with liver steatosis (36). During I/R, irisin expression was significantly reduced in serum and liver tissues (27, 31). Intravenous injection of irisin (250 μg/kg) significantly attenuated the I/R-induced decrease of mitochondria, the increment of apoptotic liver cells, high expression of inflammatory factors, and oxidative stress in the liver (27). Studies have found that supplemental irisin can bind to integrin αVβ5 and activate the downstream AMPK-UCP2 pathway to protect intestinal epithelial cells against I/R-induced cell apoptosis and oxidative stress (37).
In conclusion, irisin acts as an anti-obesity and anti-diabetic factor via regulating glucose and cholesterol synthesis metabolism in the liver. It enhances liver glycogen synthesis by activating PI3K/Akt/GSK3-GS pathway and inhibits gluconeogenesis via activating AMPK-PEPCK/G6Pase as well as PI3K/Akt/FoxO1-PEPCK and G6Pase pathway in the liver. In addition, irisin alleviates inflammation and oxidative stress in liver injury induced by I/R. However, there are still some problems that are not clear enough. The role of irisin receptor in the liver is rarely reported, and whether irisin first activates receptors on the surface of hepatocyte and then regulates glucose and lipid metabolism is still unclear.
2.3. Role of irisin in nerves
FNDC5/irisin as a novel therapeutic factor capable of improving cognition, learning, and memory function (38), which has been proved in brain injury caused in cerebral ischemia (39), stroke (40), and anxiety (41). The mediator role of FNDC5/irisin in the brain was first described by Spiegelman and his co-workers in 2013 (8); it was shown that RNA interference (RNAi)-mediated knockdown of FNDC5 reduced brain-derived neurotrophic factor [BDNF; key factor for neuronal cell survival, synaptic plasticity, dendritic arborization, and synaptogenesis (42, 43)]; reversely, increasing irisin levels in the blood by delivery FNDC5 with adenovirus increased the expression of BDNF and other neuroprotective factors, which opened a new avenue in skeletal muscle-brain cross-talk.
Moreover, fndc5 knockout Knock out (KO) mice had abnormal morphology and function of dentate gyrus neurons (part of the hippocampus), and the cognitive function of the mutant mice was significantly inhibited. Direct or peripheral delivery of irisin to the dentate gyrus was sufficient to ameliorate the cognitive deficits and neuropathology of the mice (44). In the mouse cerebral ischemia model induced by middle cerebral artery occlusion, the level of irisin was negatively correlated with the cerebral infarction volume and brain function injury score, whereas in those treated with r-irisin, the cerebral infarction volume, nerve function injury, and brain edema of the mice were significantly improved, which were related with phosphorylation of ERK1/2-Akt–mediated inflammation (39). Recently, integrin αV/β5 in the hippocampus and cortex was detected (45), which makes it available that irisin combines with integrin αV/β5 to exert the protective effect on brain; however, the specific mechanism remains to be further explored.
In addition, Lourenco et al. showed that the FNDC5/irisin expression was reduced in hippocampi and cerebrospinal fluid of Alzheimer’s disease (AD) models. Inhibition of FNDC5 in the brain impaired the memory system of mice and blocked the neuroprotective effect of physical exercise on synaptic plasticity and memory. Conversely, the increased irisin levels in AD mice improved synaptic plasticity and memory (38). In vitro, r-irisin prevented neurons from Aβ- (25–35)–induced cell toxicity via attenuating IL-6– and IL-1β–mediated inflammation status (46). Furthermore, irisin was the upstream regulator of BDNF, which attenuated the learning and memory deficits as well as the cytotoxic response against Aβ toxicity in AD (47, 48). Thus, the activation of the irisin–BDNF axis may be a potential therapeutic target for AD (49).
In conclusion, the expression of irisin was decreased in patients with brain injury. Exogenous r-irisin supplementation significantly protects nerves and enhances memory and cognitive function. Thus, irisin can be used as a potential target for the treatment of stroke, cerebral ischemia, AD, and other brain injuries.
2.4. Role of irisin in bone
2.4.1. Effects of irisin on bone tissues
Musculoskeletal interaction is one of research hot spots in recent years (50, 51). Colaianni et al. (52) found that conditioned medium (CM) from primary myoblasts of mice after 3 weeks of exercise induced a higher degree of osteoblast differentiation in vitro than that under resting conditions, and adding neutralizing antibody of FNDC5/irisin into the CM significantly reduced the expression of alkaline phosphatase (ALP) and collagen I (Col I) in osteoblasts. That was the first to establish that irisin secreted from muscles has a positive regulatory effect on bone during physical exercise. After that, Zhang et al. (53) found that 2 weeks of free wheel-running exercise increased the expression of osteogenic markers such as osterix (Osx), bone sialoprotein (BSP), and osteocalcin (OCN), as well as FNDC5/irisin in bone tissues. Irisin receptor integrin αV mediated the modulation of irisin on bone during exercise. Eight weeks of running exercise–inhibited ovariectomized (OVX) induced the reduction of femoral trabecular and cortical bone mineral density (BMD) (54), and exercise improved bone microarchitecture and increased the number of ALP-positive cells in OVX mice, whereas twice-weekly injection of cyclo RGDyk polypeptide drugs (anti-irisin receptor integrin αV agents) weakened the improvement effects of exercise (55).
The concentration of FNDC5/irisin was strongly correlated with BMD and bone homeostasis. A cross-sectional and case-control study showed that low concentrations of irisin in serum were related to hip fractures and osteoporosis in postmenopausal women (56, 57). FNDC5/irisin deletion in osteoblast lineage resulted in a lower bone density and delayed bone development and mineralization in mice, FNDC5/irisin KO also blocked the increment of cortical bone thickness by 4 days of voluntary wheel-running exercise (58). Systemic FNDC5 KO mice resulted in low bone strength and mass than Wild type (WT) mice (59), and global FNDC5/irisin KO also completely blocked OVX-induced osteocytic osteolysis and trabecular bone loss (60).
2.4.2 Role of irisin in osteoblasts
Bone modeling and remodeling require the balance of osteoblasts-induced bone formation and osteoclasts-induced bone resorption (61). Irisin activates osteogenic gene expression and induces bone formation. Injecting r-irisin (100 μg/kg/weeks, 4 weeks) significantly increased the mRNA expression of activating transcription factor 4 (Atf4) in bone marrow and phosphoprotein 1 (osteopontin, Spp1) in the whole tibia, indicating that irisin shifted from mesenchymal stem cell commitment toward osteoblast lineage and increased bone formation; in vitro experiments showed that r-irisin upregulated osteoblast marker genes like Bmp2/4, Spp1, Runt-related transcription factor-2 (Runx2), Alp, and Atf4, as well as phosphorylation of ERK1/2 in bone marrow stromal cells (62).
Furthermore, administration of r-irisin (100 ng/ml) induced differentiation and mineralization of primary rat osteoblasts and MC3T3-E1 cells by increasing the expression of osteoblast transcription regulators and differentiation marker, which was blocked by inhibiting p38 and ERK1/2 expression (63). Physical exercise activated Akt-β-catenin (essential for osteoblastic differentiation (64)) and induced ALP-positive cells increment, and these effects were abolished by tail vein injecting integrin αV inhibitor (55), which suggested that irisin increased bone mass by binding to osteoblast surface receptors and activating the Akt/β-catenin-Alp pathway. Recently, Xue et al. (65). also got a similar result in preosteoblasts.
2.4.3. Role of irisin in osteoclasts
Irisin protects bone microstructure by stimulating osteoblasts production and inhibiting the differentiation of osteoclasts to establish a “new balance”. Injection of r-irisin into OVX-induced mice significantly increased the number of osteoblasts on the surface of trabeculae bone while inhibiting the number of osteoclasts and decreasing the concentration of tartrate-resistant acid phosphatase (TRAP; marker of osteoclasts) (66, 67).
In addition, supplementation of r-irisin (20 nmol/L) in pre-osteoclastic RAW264.7 cells for 4 days resulted in the decrease of osteoclast differentiation markers (53). Moreover, Ma et al. (68) showed that irisin promoted the proliferation of two osteoclast precursor cells (RAW264.7 cells and mouse bone marrow monocytes) via activating p38-MAPK and c-Jun N-terminal kinase (JNK) signaling pathways but significantly downregulated osteoclasts differentiation markers, as well as decreased hydroxyapatite resorption pits and TRAP+ multinucleated cell numbers. However, there were also some different results. Estell et al. (69). found that administration of irisin (2–10 ng/ml) promoted the differentiation of mouse bone marrow progenitors toward osteoclasts and that overexpression of fndc5 in mice promoted the differentiation and resorption of osteoclasts, which resulted in lower bone mass.
2.4.4. Role of irisin in osteocytes
Osteocytes accounted for more than 90% of bone cells and played crucial roles in bone homeostasis. Irisin prevents bone loss and osteoporosis by robustly inhibiting osteocytic apoptosis. Spiegelman and his workmates revealed for the first time that irisin bound directly to osteocytes by integrin receptors (αVβ1/β5) and that inhibition of integrin αV receptor expression significantly inhibited the activation of SOST in bone cells by r-irisin. Injection of r-irisin (100 μg/kg) in vivo improved disuse-induced low viability and apoptosis of osteocytes and a high rate of empty lacunae (70). Furthermore, they found that irisin rapidly activated the expression of Atf4 and inhibited apoptosis by activating ERK1/2 in MLO-Y4 osteocytes, which contributed to bone development (70, 71).
In summary, irisin regulates bone regeneration and homeostasis, which reflects the key regulatory role of muscle on bone (72). We summarized the effects of irisin in bone tissue cells in Table 1.
2.5. Role of irisin in skeletal muscle
AMPK–PGC-1α (PPARγ coactivator-1α)–FNDC5 axis is the most important pathway for irisin synthesis. During exercise, the Ca2+ level is increased significantly in the muscle cytoplasm along with skeletal muscle contraction and then stimulates the phosphorylation of AMPK (78), which, in turn, enhances PGC-1α and regulates transcription of downstream factors such as fndc5. Shan et al. revealed that myostatin (MSTN) KO in skeletal muscle significantly increased PGC-1α and FNDC5/irisin expression, and the high level of irisin increased the browning of WAT in MSTN−/− mice (79). Moreover, Ge et al. found that myostatin inhibited FNDC5/irisin expression by increasing miR-34a (80).
The blood circulation level of irisin has been identified as a biomarker for muscle mass and performance (81). For example, the concentration of irisin in patients with sarcopenia and pre-sarcopenia was lower compared with that in non-sarcopenic participants (82, 83). Exposure to an ambient hypoxic environment can cause skeletal muscle loss and atrophy, along with the low concentration of irisin in blood circulation both in humans (84) and mice (85), which could be one of the reasons of muscle atrophy induced by hypoxia (86). However, interestingly, knockdown of fndc5 in skeletal muscle still performed equal muscle mass, development, growth, regeneration, and strength compared with WT mice. Although, there was no difference in cardiotoxin-induced muscle injury between fndc5-mutant and WT mice (87).
Multiple studies showed that exogenous r-irisin improved skeletal muscle loss and atrophy. Colaianni et al. revealed that r-irisin prevented hindlimb unloading-induced muscle mass decline and decrease of myosin type II expression (10). In addition, in vitro, fndc5 gene expression and irisin concentration were positively correlated with the process of differentiation of C2C12 myotubes; r-irisin supplementation increased human primary skeletal muscle cell growth and hypertrophy by increasing insulin-like growth factor 1(IGF-1)/PGC1α4 and decreasing myostatin through activating ERK1/2 pathway (88). Another study from Reza et al. showed that r-irisin increased myogenic differentiation and myoblast fusion via activating IL-6 signaling pathway, and r-irisin treatment also improved denervation-induced muscle injury by increasing protein synthesis through the ERK1/2 pathway (9). Irisin treatment (100 ng/ml, 24 h) also prevented dexamethasone-induced atrophy in C2C12 myotubes by upregulating IGF-1 and attenuating proteolytic activity through dephosphorylation of FoxO3α-mediated ubiquitin-proteasome overactivity (89).
In short, irisin is mainly produced by muscle tissue via Ca2+–AMPK–PGC-1α–FNDC5 pathway. It induces the expression of myoblasts by activating downstream ERK1/2 and IL-6 pathways in an autocrine manner, which plays key regulatory role in muscle growth and differentiation. However, there are still some problems, such as whether the receptor is still integrin αV/β5 on the surface of muscle cells. In addition, the role of integrin αV/β5 in exercise-induced muscle hyperplasia and hypertrophy is unclear.
2.6. Role of irisin in articular cartilage
Osteoarthritis (OA) is a degenerative joint injury characterized by joint pain, progressive cartilaginous degeneration, and stiffness, which poses a great challenge to the physical health of the patients (90). It was stated that FNDC5/irisin activated by moderate physical exercise played a key role in alleviating symptoms and the process of OA such as progressive cartilaginous degeneration, synovial inflammation, and osteophyte formation (71, 91, 92). Studies have shown that the expression of FNDC5/irisin is reduced in patients with osteoarthritic cartilage (93) or synovial fluid (94) compared with that in healthy subjects. In addition, FNDC5 KO mice accelerated anterior cruciate ligament transection–induced OA progression; conversely, FNDC5 knock-in attenuated OA progression (95).
Direct intraarticular injection of irisin may be more effective as almost no blood vessels pass through cartilage. Destabilized medial meniscus (DMM)–induced OA mice were directly injected intra-articular with r-irisin for 8 weeks; the results showed that irisin prevented articular cartilage loss and ameliorated irregular gait; moreover, administration of irisin increased autophagy flux and survival of chondrocytes in DMM-induced OA mice by increasing the expression of LC3 and proliferating cell nuclear antigen (93). In vitro, Col II and tissue inhibitor of matrix metalloproteinase (MMP)–1 and –3 expression was significantly increased, and Col X (hypertrophic chondrocyte–related gene) and MMP-1 and MMP-13 expression significantly decreased by adding r-irisin to human primary chondrocytes for 7 days, indicating that the addition of irisin contributes to maintaining partial stability of the extracellular matrix (ECM) of cartilage (96). This mechanism may be related to irisin, lowering the activation of p38, JNK, and Akt in chondrocytes (96, 97). Recently, Jia et al. (98). found that exercise-activated irisin alleviated OA chondrocyte inflammation by inhibiting PI3K/Akt/Nuclear factor kappa B (NF-κB) signaling pathway and suppressing the NOD-like receptor protein 3 (NLRP3) and caspase-1–mediated pyroptosis. In addition, in vitro, r-irisin treatment (5 and 10 ng/ml, 24 h) attenuated IL-lβ–induced PI3K/Akt/NF-κB p65 cascade and blocked the nuclear translocation of NF-κB p65. Furthermore, irisin supplementation also improved the inflammatory status of OA by reducing the expression of inflammation factors such as IL-1β (95, 99), TNF-α (92), IL-6, and IL-1 (96).
In summary, the expression of irisin was reduced in patients with OA, and moderate physical exercise could alleviate OA by activating irisin (92). The therapeutic effect of irisin is mainly reflected in reducing the inflammatory state of damaged cartilage and increasing the autophagy flux; additionally, intraarticular injection of r-irisin may be effective for rehabilitating patients with OA.
2.7. Role of irisin in cancer
Cancer is one of the leading causes of human death. Regular exercise helps reducing the risk of cancer (100); as an exercise gene (101), the role of FNDC5/irisin in the occurrence and prevention of cancer has received extensive attention (102). Most studies have shown an elevated irisin expression in cancer (103–105). However, a few studies also reported that irisin expression is reduced in patients with cancer (106). Therefore, more research studies are needed to explore the role of irisin in cancer.
In vitro, r-irisin inhibited the proliferation, migration, invasion, and epithelial-to-mesenchymal transition (EMT) in lung cancer (11), epithelial ovarian cancer (107), and pancreatic cancer (PC) (108) cells by inhibiting PI3K/Akt- and Signal transducer and activator of transcription 3 (STAT3)-mediated (109) downstream Snail expression (an important role in stimulating EMT).
Irisin induces the arrest of cancer cell division and inhibits cell growth. Huang et al. revealed that irisin induced G (2)/M cell cycle arrest and increased the expression of P21 and tissue factor pathway inhibitor 2, thereby inhibiting the proliferation and invasion of glioblastoma multiforme cells (91). Similarly, Liu et al. found that the receptor of irisin also existed on the surface of PC cells; supplementation of both non-glycosylated and glycosylated r-irisin in PCs could induce G1 arrest and inhibit the growth of PC via activating AMPK and inhibiting mTOR expression (110); these results indicate that irisin can affect tumor tissues and exert antitumor properties. However, there is still not enough evidence that irisin can directly act on the integrins on tumor cells to inhibit the development of EMT or tumor proliferation (111).
Overall, irisin has a wide application prospect for the treatment of cancer. Irisin inhibited the proliferation, migration, and invasion of tumor cells by inhibiting PI3K/Akt- and STAT3-mediated Snail/EMT pathways. In addition, irisin also inhibited tumor growth by inducing G1 or G (2)/M cell cycle arrest through AMPK/mTOR pathway.
2.8. Role of irisin in myocardium and blood vessel
CVDs include hypertension, coronary artery disease, myocardial infarction, heart failure, atherosclerosis, and myocardial I/R injury, which are the leading cause of human death worldwide (112). Regular exercise can reduce the risk of CVDs, and irisin may play a crucial role in it. Studies have found that the expression of irisin in patients with CVDs is significantly lower than that in healthy people (113–117). Li et al. revealed that resistance exercise could activate the release of irisin from skeletal muscle and then stimulate the AMPK-PINK1/Parkin-LC3/P62 signaling pathway, which regulated mitophagy and inhibited oxidative stress in the myocardium (12). In vitro, studies have shown that irisin binds directly to the endothelial cell surface receptor integrin αV/β5, thereby phosphorylating AMPK (Thr172) and activating PGC-1α (induce mitochondrial biogenesis) and mitochondrial transcription factor A (a key activator of mitochondrial transcription and a participant in mitochondrial genome replication).
Cardiac hypertrophy progresses to heart failure; irisin can significantly improve myocardial hypertrophy. Qing et al. showed that administration of r-irisin could attenuate angiotensin II (Ang II)–induced cardiomyocyte hypertrophy, in vitro, and that treatment of irisin in transverse aortic constriction (TAC)–induced cardiac hypertrophy murine, in vivo, significantly suppressed cardiac hypertrophy and fibrosis by phosphorylating AMPK (Thr172) and inhibiting the phosphorylation of mTOR (Ser2448). However, the expression of irisin increased in the hypertrophic heart and serum during this period, which may be a stress response from the body, as the elevated irisin could decrease endothelial damage by suppressing oxidative stress and inflammation (4, 118). Yue et al. found that r-irisin protected myocardial hypertrophic mice induced by TAC or Ang II–treated cardiomyocytes via inhibiting NLRP3-mediated pyroptosis (119).
The therapeutic role of irisin on cardiac hypertrophy was also reflected in the improvement of autophagy flux and induction of protective autophagy. Li et al. found that supplementation of irisin in Ang II–treated cardiomyocytes significantly increased the expression of LC3II and decreased P62 expression and activated the phosphorylation of AMPK (Thr172) and ULK1 (Ser555), thereby reducing cardiomyocyte apoptosis, and this protection will be reversed by autophagy inhibitor such as 3-methyladenine, autophagy-related 5 siRNA (ATG5), and chloroquine; moreover, blockage of AMPK and ULK1 also abrogated autophagy flux and indicted irisin-induced protective autophagy in cardiac hypertrophy via activating AMPK-ULK1 pathway (120, 121).
Growing evidence suggests that the content of irisin in patients with atherosclerosis is significantly lower than that in normal controls (122–124), and irisin supplementation has a significant effect on the treatment and improvement of atherosclerosis. For example, irisin supplementation can significantly improve endothelial dysfunction, decrease endothelial apoptosis, and predominantly decrease atherosclerotic plaque area in nicotine or streptozotocin-induced apolipoprotein E-Null [apoE(−/−)] atherosclerosis mice (125). Here, we enumerated the role of irisin in atherosclerosis disease in Table 2.
Overall, the integrin αVβ5 on the endothelial cell surface could be activated by FNDC5/irisin. As a key energy sensor to maintain energy balance and mitochondrial hemostasis (131), AMPK mediated the effect of FNDC5/irisin on mitophagy, oxidative stress, and mitochondrial biogenesis, thereby improving myocardial hypertrophy, myocardial infarction, atherosclerosis, and other cardiac diseases, which reflecting the protection of regular exercise on cardiac health.
2 Conclusions
In this review, we systematically summarized the roles of FNDC5/irisin in fat, liver, nerve, bone, skeletal muscle, articular cartilage, cancer, and angiocarpy. Irisin, as a muscle factor secreted by exercise, plays an extremely important role in regulating fat browning, improving liver and systemic glucose metabolism, maintaining musculoskeletal homeostasis, promoting synaptic growth, and inhibiting the progression of cancer. The mechanism of irisin is mainly through first directly binding to its receptor integrin αV/β1/5 and then activating AMPK, FAK, and MAPK signaling pathways. Collectively, potential mechanisms and signaling pathways for the actions of irisin in musculoskeletal and pathological tissues are shown in Figures 1 and 2, respectively.
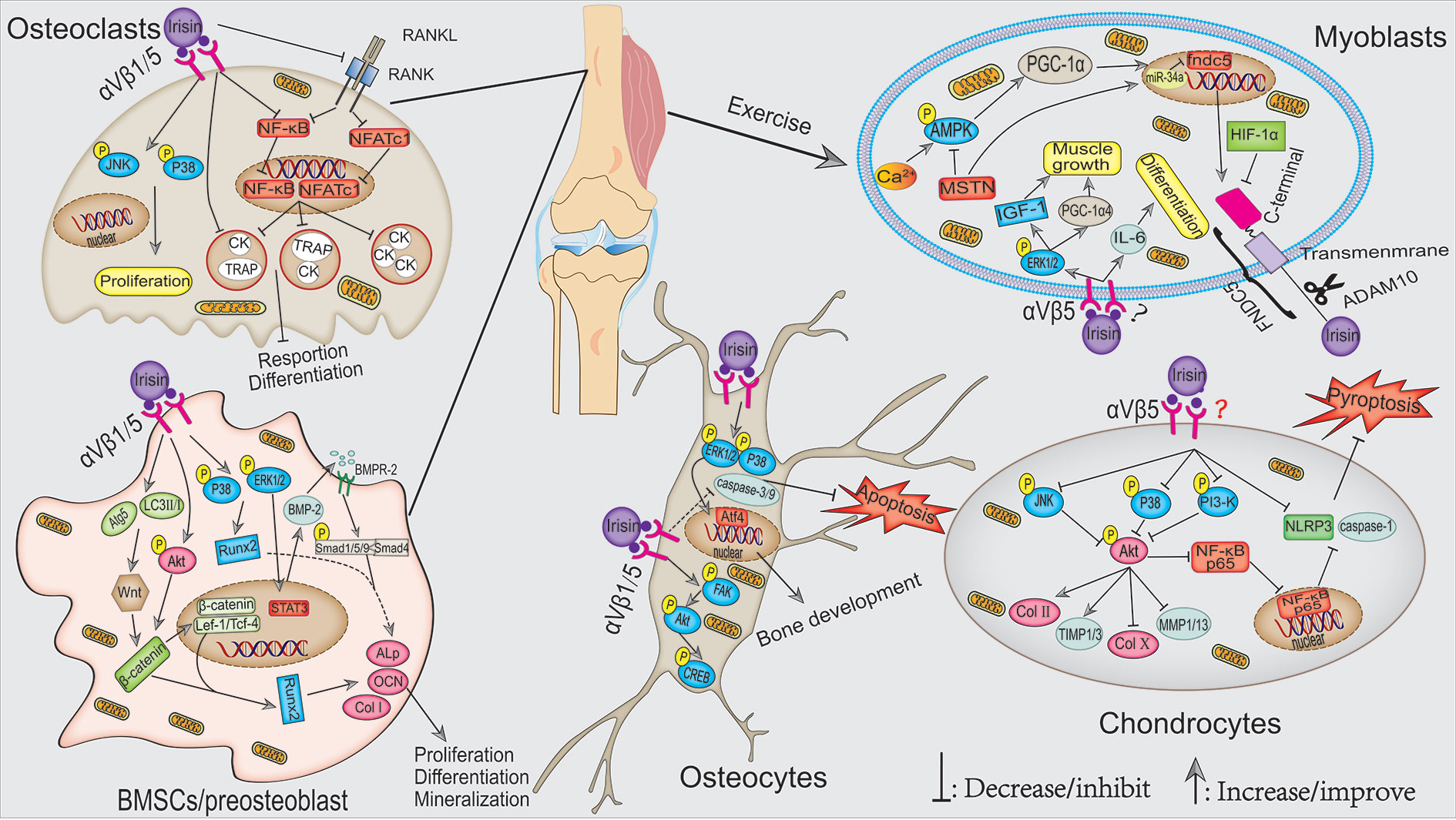
Figure 1 Potential mechanisms signaling pathways for the actions of irisin in musculoskeletal. During exercise, the elevated Ca2+ in muscle cytoplasm-induced activation of the AMPK–PGC-1α–FNDC5 axis is the main pathway for irisin synthesis. In addition, irisin, in turn, can stimulate muscle growth and myoblast differentiation via ERK1/2–IGF-1/MSTN and IL-6 signaling pathways, respectively. Multiple pathways mediated exercise-induced irisin and r-irisin–activated osteoblast differentiation and mineralization, e.g., p38/ERK1/2, Akt-β-catenin, and Wnt-β-catenin–mediated activation of ALP/OCN/Col I pathways. In osteoclast, irisin induced its proliferation through activating the p38/JNK pathway. In addition, irisin also inhibited the NF-κB and NFATc1 levels in the nucleus, thus inhibiting the expression of osteoclast differentiation marker genes. As for osteocytes, irisin inhibited osteocyte apoptosis by inhibiting caspase-9 and caspase-3 expression, which probably through activating p38/ERK1/2. Furthermore, moderate exercise-activated irisin or r-irisin could alleviate OA by maintaining ECM stabilization and reducing inflammatory response through p38/JNK-Akt and PI3K/Akt/NF-κB signaling pathway, respectively.

Figure 2 Potential mechanisms signaling pathways for the actions of irisin in the pathological tissues. Irisin protects against DIO by inducing the recruitment of beige fat to dissipate energy into heat. This mechanism is involved in p38 MAPK and ERK1/2 pathways, as well as FAK-mediated beige APCs proliferation. In addition, irisin attenuated diet-induced metabolic disorders, including NAFLD and hepatic steatosis by promoting the synthesis of liver glycogen via PI3K/Akt/GSK3-GS and inhibiting the generation of liver gluconeogenesis through AMPK-PEPCK/G6Pase and PI3K/Akt/FOXO1-mediated PEPCK/G6Pase pathways. In brain tissues, irisin promoted cognition and neuro development via inhibiting the inflammatory response and activating BDNF-mediated nerve cell survival, differentiation, and plasticity. Moreover, irisin affects the proliferation, migration, and invasion of tumor cells probably by binding integrin αV/β5–mediated PI3K/Akt-Snail-EMT and AMPK-mTOR pathways, which has great therapeutic prospects for inhibiting cancer development. Moreover, exercise-induced irisin can also reduce the risk of cardiovascular diseases. In cardiomyocytes, irisin stimulated AMPK-mediated autophagy and mitobiogenesis by binding to its receptor integrin αV/β5, thereby relieving cardiac hypertrophy and injury.
There are still some unsolved questions, for example, the concentration of irisin in pathophysiological conditions that are highly controversial; some studies suggest that the irisin level rises in patients with obesity or cancer, but why does irisin not play a role in burning and “ERK-ing” the fat as well as inhibiting the development of cancer? Perhaps, its receptor sensitivity and number are reduced under these pathological conditions, which resulted in “irisin resistance”. At this point, high concentration of irisin may not come from muscle tissue but from newly increased fat or cancer tissues; perhaps, due to the decreased activity and expression of its receptor, irisin could not play a substantial role even if the concentration increased. In addition, whether irisin that directly binds to receptors on the surface of chondrocytes, myoblasts, cancer cells, and hepatocytes plays a regulatory role is still unclear, and relevant studies are limited. Therefore, it may be necessary to further explore the role of irisin by detecting the expression of its receptor integrin αV/β1/5 in these pathological and physiological tissues.
Here, we summarized the progress and mechanism of FNDC5/irisin in physiological and pathological conditions, and we analyzed the shortcomings of current research of FNDC5/irisin. We hope that this review may provide an available reference for FNDC5/irisin research.
Author contributions
SL and FC were mainly involved in data analysis and manuscript drafting, KN and DW helped to draw the mechanic images. ZW and PF made final checks on the manuscript and data. HX provided major theoretical knowledge and relevant suggestions. All authors contributed to the article and approved the submitted version.
Funding
This work was supported by the National Natural Science Foundation of China (grant numbers 81772409 and 32001055) and the Innovation Foundation for Doctor Dissertation of Northwestern Polytechnical University (grant/award number CX2021098).
Acknowledgments
Many thanks to Hassan Siddique (University of Science and Technology of China) for checking the grammar.
Conflict of interest
The authors declare that the research was conducted in the absence of any commercial or financial relationships that could be construed as a potential conflict of interest.
Publisher’s note
All claims expressed in this article are solely those of the authors and do not necessarily represent those of their affiliated organizations, or those of the publisher, the editors and the reviewers. Any product that may be evaluated in this article, or claim that may be made by its manufacturer, is not guaranteed or endorsed by the publisher.
Abbreviations
UCP-1, uncoupling protein 1; Dio2, type II iodothyronine deiodinase 2; Cidea, cell death activator; Prdm16, PR domain-containing 16; Cox-7a, cytochrome c oxidase subunit 7a; PINK1, PTEN-induced putative kinase 1; RANKL, receptor activator for nuclear factor–κB ligand; RANK, receptor activator of nuclear factor–κB; NFATc1, nuclear factor of activated T cell 1; TRAP, tartrate-resistant acid phosphatase; CK, cathepsin K; IGF1, insulin-like growth factor 1; FOXO1, forkhead box protein O1; SOST, Sclerostin.
References
1. Nie Y, Dai B, Guo X, Liu D. Cleavage of FNDC5 and insights into its maturation process. Mol Cell Endocrinol (2020) 510(15):110840. doi: 10.1016/j.mce.2020.110840
2. Nie Y, Liu D. N-glycosylation is required for FDNC5 stabilization and irisin secretion. Biochem J (2017) 474(18):3167–77. doi: 10.1042/BCJ20170241
3. Zhang Y, Li R, Meng Y, Li S, Donelan W, Zhao Y, et al. Irisin stimulates browning of white adipocytes through mitogen-activated protein kinase p38 MAP kinase and ERK MAP kinase signaling. Diabetes (2014) 63(2):514–25. doi: 10.2337/db13-1106
4. Yu Q, Kou W, Xu X, Zhou S, Luan P, Xu X, et al. FNDC5/Irisin inhibits pathological cardiac hypertrophy. Clin Sci (Lond) (2019) 133(5):611–27. doi: 10.1042/CS20190016
5. Bostrom P, Wu J, Jedrychowski MP, Korde A, Ye L, Lo JC, et al. A PGC1-alpha-dependent myokine that drives brown-fat-like development of white fat and thermogenesis. Nature (2012) 481(7382):463–8. doi: 10.1038/nature10777
6. Stergios AP, Jannis K, Athanasios DA, Eleni VG. Irisin in patients with nonalcoholic fatty liver disease. Clin MCJM Exp (2014) 63(2):207–17. doi: 10.1016/j.metabol.2013.09.013
7. Canivet CM, Bonnafous S, Rousseau D, Pierre SL, L-G S, Patouraux S, et al. Hepatic FNDC5 is a potential local protective factor against non-alcoholic fatty liver. Biochimica et biophysica acta Molecular basis of disease (2020) 1866(5):165705. doi: 10.1016/j.bbadis.2020.165705
8. Wrann CD, White JP, Salogiannnis J, Laznik-Bogoslavski D, Wu J, Ma D, et al. Exercise induces hippocampal BDNF through a PGC-1alpha/FNDC5 pathway. Cell Metab (2013) 18(5):649–59. doi: 10.1016/j.cmet.2013.09.008
9. Reza MM, Subramaniyam N, Sim CM, Ge X, Sathiakumar D, McFarlane C, et al. Irisin is a pro-myogenic factor that induces skeletal muscle hypertrophy and rescues denervation-induced atrophy. Nat Commun (2017) 8(1):1104. doi: 10.1038/s41467-017-01131-0
10. Colaianni G, Mongelli T, Cuscito C, Pignataro P, Lippo L, Spiro G, et al. Irisin prevents and restores bone loss and muscle atrophy in hind-limb suspended mice. Sci Rep (2017) 7(1):2811. doi: 10.1038/s41598-017-02557-8
11. Shao L, Li H, Chen J, Song H, Zhang Y, Wu F, et al. Irisin suppresses the migration, proliferation, and invasion of lung cancer cells via inhibition of epithelial-to-mesenchymal transition. Biochem. Biophys. Res. Commun. (2017) 485(3):598–605. doi: 10.1016/j.bbrc.2016.12.084
12. Li H, Qin S, Liang Q, Xi Y, Bo W, Cai M, et al. Exercise training enhances myocardial mitophagy and improves cardiac function via Irisin/FNDC5-PINK1/Parkin pathway in MI mice. Biomedicines (2021) 9(6):701. doi: 10.3390/biomedicines9060701
13. Jedrychowski MP, Wrann CD, Paulo JA, Gerber KK, Szpyt J, Robinson MM, et al. Detection and quantitation of circulating human irisin by tandem mass spectrometry. Cell Metab (2015) 22(4):734–40. doi: 10.1016/j.cmet.2015.08.001
14. Lee P, Linderman JD, Smith S, Brychta RJ, Wang J, Idelson C, et al. Irisin and FGF21 are cold-induced endocrine activators of brown fat function in humans. Cell Metab (2014) 19(2):302–9. doi: 10.1016/j.cmet.2013.12.017
15. Oguri Y, Shinoda K, Kim H, Alba DL, Bolus WR, Wang Q, et al. CD81 controls beige fat progenitor cell growth and energy balance via FAK signaling. Cell (2020) 182(3):563–77 e20. doi: 10.1016/j.cell.2020.06.021
16. Cohen P, Kajimura S. The cellular and functional complexity of thermogenic fat. Nat Rev Mol Cell Biol (2021) 22(6):393–409. doi: 10.1038/s41580-021-00350-0
18. Mehrnoosh S, Nariman M, Solaleh E, Reza F. Lower circulating irisin is associated with nonalcoholic fatty liver disease and type 2 diabetes. Diabetes PHJ syndr m (2017) 11(Suppl 1):S467–S72. doi: 10.1016/j.dsx.2017.03.037
19. Zhang HJ, Zhang XF, Ma ZM, Pan LL, Chen Z, Han HW, et al. Irisin is inversely associated with intrahepatic triglyceride contents in obese adults. J Hepatol (2013) 59(3):557–62. doi: 10.1016/j.jhep.2013.04.030
20. Jiang S, Piao L, Ma EB, Ha H, Huh JY. Associations of circulating irisin with FNDC5 expression in fat and muscle in type 1 and type 2 diabetic mice. Biomolecules (2021) 11(2):322. doi: 10.3390/biom11020322
21. Dokumacioglu E, Iskender H, Sahin A, Erturk EY, Kaynar O. Serum levels of nesfatin-1 and irisin in obese children. Eur Cytokine Netw (2020) 31(1):39–43. doi: 10.1684/ecn.2020.0444
22. Stengel A, Hofmann T, G-S M, E U, Kobelt P, Klapp B. Circulating levels of irisin in patients with anorexia nervosa and different stages of obesity–correlation with body mass index. Peptides (2013) 39:125–30. doi: 10.1016/j.peptides.2012.11.014
23. Pardo M, Crujeiras AB, Amit M, Aguera Z, Jiménez-Murcia S, B R, et al. Association of irisin with fat mass, resting energy expenditure, and daily activity in conditions of extreme body mass index. Int J Endocrinol (2014) 2014:857270. doi: 10.1155/2014/857270
24. Jia J, Yu F, Wei WP, Yang P, Zhang R, Sheng Y, et al. Relationship between circulating irisin levels and overweight/obesity: A meta-analysis. World J Clin Cases (2019) 7(12):1444–55. doi: 10.12998/wjcc.v7.i12.1444
25. Crujeiras AB, Pardo M, Casanueva FF. Irisin: 'fat' or artefact. Clin Endocrinol (Oxf) (2015) 82(4):467–74. doi: 10.1111/cen.12627
26. Moreno-Navarrete JM, Ortega F, Serrano M, Guera E, Pardo G, Tinahones F, et al. Irisin is expressed and produced by human muscle and adipose tissue in association with obesity and insulin resistance. J Clin Endocrinol Metab (2013) 98(4):E769–78. doi: 10.1210/jc.2012-2749
27. Bi J, Zhang J, Ren Y, Du Z, Li Q, Wang Y, et al. Irisin alleviates liver ischemia-reperfusion injury by inhibiting excessive mitochondrial fission, promoting mitochondrial biogenesis and decreasing oxidative stress. Redox Biol (2019) 20:296–306. doi: 10.1016/j.redox.2018.10.019
28. Fan X, Du J, Wang MH, Li JM, Yang B, Chen Y, et al. Irisin contributes to the hepatoprotection of dexmedetomidine during intestinal Ischemia/Reperfusion. Oxid Med Cell Longev (2019) 2019:7857082. doi: 10.1155/2019/7857082
29. Liu TY, Shi CX, Gao R, Sun HJ, Xiong XQ, Ding L, et al. Irisin inhibits hepatic gluconeogenesis and increases glycogen synthesis via the PI3K/Akt pathway in type 2 diabetic mice and hepatocytes. Clin Sci (Lond) (2015) 129(10):839–50. doi: 10.1042/CS20150009
30. Xin C, Liu J, Zhang J, Zhu D, Wang H, Xiong L, et al. Irisin improves fatty acid oxidation and glucose utilization in type 2 diabetes by regulating the AMPK signaling pathway. Int J Obes (Lond) (2016) 40(3):443–51. doi: 10.1038/ijo.2015.199
31. Bi J, Yang L, Wang T, Zhang J, Li T, Ren Y, et al. Irisin improves autophagy of aged hepatocytes via increasing telomerase activity in liver injury. Oxid Med Cell Longev (2020) 2020:6946037. doi: 10.1155/2020/6946037
32. Pinto AP, Ropelle ER, Quadrilatero J, da Silva ASR. Physical exercise and liver autophagy: Potential roles of IL6 and irisin. Exercise Rev ss (2021) 50(2):89–96. doi: 10.1249/JES.0000000000000278
33. Tang H, Yu R, Liu S, Huwatibieke B, Li Z, Zhang W. Irisin inhibits hepatic cholesterol synthesis via AMPK-SREBP2 signaling. EBioMedicine (2016) 6:139–48. doi: 10.1016/j.ebiom.2016.02.041
34. Zhu W, Sahar NE, Javaid HMA, Pak ES, Liang G, Wang Y, et al. Exercise-induced irisin decreases inflammation and improves NAFLD by competitive binding with MD2. Cells (2021) 10(12):3306. doi: 10.3390/cells10123306
35. Nastos C, Kalimeris K, Papoutsidakis N, Tasoulis MK, Lykoudis PM, Thedoraki K, et al. Global consequences of liver ischemia/reperfusion injury. Oxid Med Cell Longev (2014) 2014:906965. doi: 10.1155/2014/906965
36. Bi J, Zhang J, Ren Y, Du Z, Li Q, Wang Y, et al. Corrigendum to "Irisin alleviates liver ischemia-reperfusion injury by inhibiting excessive mitochondrial fission, promoting mitochondrial biogenesis and decreasing oxidative stress" [Redox biol. 20 (2019) 296-306]. Redox Biol (2019) 26:101193. doi: 10.1016/j.redox.2019.101193
37. Bi J, Zhang J, Ren Y, Du Z, Li T, Wang T, et al. Irisin reverses intestinal epithelial barrier dysfunction during intestinal injury via binding to the integrin αVβ5 receptor. J Cell Mol Med (2020) 24(1):996–1009. doi: 10.1111/jcmm.14811
38. Lourenco MV, Frozza RL, Freitas GB de, Zhang H, Kimcheski GC, Ribeiro FC, et al. Exercise-linked FNDC5/irisin rescues synaptic plasticity and memory defects in alzheimer's models. Nat Med (2019) 25(1):165–75. doi: 10.1038/s41591-018-0275-4
39. Li DJ, Li YH, Yuan HB, Qu LF. The novel exercise-induced hormone irisin protects against neuronal injury via activation of the akt and ERK1/2 signaling pathways and contributes to the neuroprotection of physical exercise in cerebral ischemia. Clin Exp (2017) 68:31–42. doi: 10.1016/j.metabol.2016.12.003
40. Liu Y, Zhu C, Guo J, Chen Y, Meng C. The neuroprotective effect of irisin in ischemic stroke. Front Aging Neurosci (2020) 12:588958. doi: 10.3389/fnagi.2020.588958
41. Uysal N, Yuksel O, Kizildag S, Yuce Z, Gumus H, Karakilic A, et al. Regular aerobic exercise correlates with reduced anxiety and incresed levels of irisin in brain and white adipose tissue. Front Aging Neurosci (2018) 676:92–7. doi: 10.1016/j.neulet.2018.04.023
42. Greenberg ME, Xu B, Lu B, Hempstead BL. New insights in the biology of BDNF synthesis and release: implications in CNS function. J Neurosci (2009) 29(41):12764–7. doi: 10.1523/JNEUROSCI.3566-09.2009
43. Park H, Poo MM. Neurotrophin regulation of neural circuit development and function. J Neurosci (2013) 14(1):7–23. doi: 10.1038/nrn3379
44. Islam MR, Valaris S, Young MF, Haley EB, Luo R, Bond SF, et al. Exercise hormone irisin is a critical regulator of cognitive function. Nat Metab (2021) 3(8):1058–70. doi: 10.1038/s42255-021-00438-z
45. Jackson TC, Gorse K, Herrmann JR, Kochanek PM. Hippocampal and prefrontal cortical brain tissue levels of irisin and GDF15 receptor subunits in children. J Neurobiol (2021) 58(5):2145–57. doi: 10.1007/s12035-020-02250-4
46. Wang K, Li H, Wang H, Wang JH, Song F. Irisin exerts neuroprotective effects on cultured neurons by regulating astrocytes. J Mo Inflammation (2018) 2018:9070341. doi: 10.1155/2018/9070341
47. Aranciba S, Silhol M, Mouliére F, Meffre J, Höllinger I, Maurice T, et al. Protective effect of BDNF against beta-amyloid induced neurotoxicity in vitro and in vivo in rats. Journal: Neurobiol Dis (2008) 31(3):316–26. doi: 10.1016/j.nbd.2008.05.012
48. Zhang L, Fang Y, Lian Y, Chen Y, Wu T, Zheng Y, et al. Brain-derived neurotrophic factor ameliorates learning deficits in a rat model of alzheimer's disease induced by aβ1-42. PLoS One (2015) 10(4):. doi: 10.1371/journal.pone.0122415
49. Kim OY, Song J. The role of irisin in alzheimer's disease. J Clin Med (2018) 7(11):407. doi: 10.3390/jcm7110407
50. Pedersen BK, Febbraio MA. Muscles, exercise and obesity: skeletal muscle as a secretory organ. Nat Rev Endocrinol (2012) 8(8):457–65. doi: 10.1038/nrendo.2012.49
51. Giudice J, Taylor JM. Muscle as a paracrine and endocrine organ. Curr Opin Pharmacol (2017) 34:49–55. doi: 10.1016/j.coph.2017.05.005
52. Colaianni G, Cuscito C, Mongelli T, Oranger A, Mori G, Brunetti G, et al. Irisin enhances osteoblast differentiation in vitro. Int J Endocrinol (2014) 2014:902186. doi: 10.1155/2014/902186
53. Zhang J, Valverde P, Zhu X, Murray D, Wu Y, Yu L, et al. Exercise-induced irisin in bone and systemic irisin administration reveal new regulatory mechanisms of bone metabolism. Bone Res (2017) 5:16056. doi: 10.1038/boneres.2016.56
54. Kawao N, Iemura S, Kawaguchi M, Mizukami Y, Takafuji Y, Kaji H, et al. Role of irisin in effects of chronic exercise on muscle and bone in ovariectomized mice. Jo Bone (2021) 39(4):547–57. doi: 10.1007/s00774-020-01201-2
55. Zhao R, Zhou Y, Li J, Lin J, Cui W, Peng Y, et al. Irisin regulating skeletal response to endurance exercise in ovariectomized mice by promoting akt/β-catenin pathway. Front Physiol (2021) 12:639066. doi: 10.3389/fphys.2021.639066
56. Liu K, Jing P, Liu Z, Wang Y, Han Z, Wang Y, et al. Serum levels of irisin in postmenopausal women with osteoporotic hip fractures. Cytokine (2021) 148:155708. doi: 10.1016/j.cyto.2021.155708
57. Roomi AB, Nori W, Hamed RM. Lower serum irisin levels are associated with increased osteoporosis and oxidative stress in postmenopausal. Rep Biochem Mol Biol (2021) 10(1):13–9. doi: 10.52547/rbmb.10.1.13
58. Zhu X, Li X, Wang X, Chen T, Tao F, Liu C, et al. Irisin deficiency disturbs bone metabolism. J Cell Physiol (2021) 236(1):664–76. doi: 10.1002/jcp.29894
59. Luo Y, Qiao X, Ma Y, Deng H, Xu CC, Xu L. Disordered metabolism in mice lacking irisin. Sci Rep (2020) 10(1):17368. doi: 10.1038/s41598-020-74588-7
60. Kim H, Wrann CD, Jedrychowski M, Vidoni S, Kitase Y, Nagano K, et al. Irisin mediates effects on bone and fat via alphaV integrin receptors. Cell (2018) 175(7):1756–68 e17. doi: 10.1016/j.cell.2018.10.025
61. Ma Q, Liang M, Wu Y, Luo F, Ma Z, Dong S, et al. Osteoclast-derived apoptotic bodies couple bone resorption and formation in bone remodeling. Bone Res (2021) 9(1):5. doi: 10.1038/s41413-020-00121-1
62. Colaianni G, Cuscito C, Mongelli T, Pignataro P, Buccoliero C, Liu P, et al. The myokine irisin increases cortical bone mass. Proc Natl Acad Sci U.S.A. (2015) 112(39):12157–62. doi: 10.1073/pnas.1516622112
63. Qiao X, Nie Y, Ma Y, Chen Y, Cheng R, Yin W, et al. Irisin promotes osteoblast proliferation and differentiation via activating the MAP kinase signaling pathways. Sci Rep (2016) 6:18732. doi: 10.1038/srep18732
64. Sunters A, Armstrong VJ, Zaman G, Kypta RM, Kawao Y, Lanyon LE, et al. Mechano-transduction in osteoblastic cells involves strain-regulated estrogen receptor alpha-mediated control of insulin-like growth factor (IGF) I receptor sensitivity to ambient IGF, leading to phosphatidylinositol 3-kinase/AKT-dependent Wnt/LRP5 receptor-independent activation of beta-catenin signaling. J Biol Chem (2010) 285(12):8743–58. doi: 10.1074/jbc.M109.027086
65. Xue Y, Hu S, Chen C, He J, Sun J, Jin Y, et al. Myokine irisin promotes osteogenesis by activating BMP/SMAD signaling via alphaV integrin and regulates bone mass in mice. Int J Biol Sci (2022) 18(2):572–84. doi: 10.7150/ijbs.63505
66. Luo Y, Ma Y, Qiao X, Zeng R, Cheng R, Nie Y, et al. Irisin ameliorates bone loss in ovariectomized mice. Climacteric (2020) 23(5):496–504. doi: 10.1080/13697137.2020.1745768
67. Morgan EN, Alsharidah AS, Mousa AM, Edrees HM. Irisin has a protective role against osteoporosis in ovariectomized rats. BioMed Res Int (2021) 2021:5570229. doi: 10.1155/2021/5570229
68. Ma Y, Qiao X, Zeng R, Cheng R, Zhang J, Luo Y, et al. Irisin promotes proliferation but inhibits differentiation in osteoclast precursor cells. FASEB J (2018) 32(11):5813–5823. doi: 10.1096/fj.201700983RR
69. Estell EG, Le PT, Vegting Y, Kim H, Wrann C, Bouxsein ML, et al. Irisin directly stimulates osteoclastogenesis and bone resorption in vitro and in vivo. Elife (2020) 9:e58172. doi: 10.7554/eLife.58172
70. Storlino G, Colaianni G, Sanesi L, Lippo L, Brunetti G, Errede M, et al. Irisin prevents disuse-induced osteocyte apoptosis. J Bone Miner Res (2020) 35(4):766–75. doi: 10.1002/jbmr.3944
71. He Z, Li H, Han X, Zhou F, Du J, Yang Y, et al. Irisin inhibits osteocyte apoptosis by activating the erk signaling pathway in vitro and attenuates ALCT-induced osteoarthritis in mice. Bone (2020) 141:115573. doi: 10.1016/j.bone.2020.115573
72. Colaianni G, Mongelli T, Colucci S, Cinti S, Grano M. Crosstalk between muscle and bone Via the muscle-myokine irisin. Curr Osteoporos Rep (2016) 14(4):132–7. doi: 10.1007/s11914-016-0313-4
73. Chen X, Sun K, Zhao S, Geng T, Fan X, Sun S, et al. Irisin promotes osteogenic differentiation of bone marrow mesenchymal stem cells by activating autophagy via the wnt//β-catenin signal pathway. Cytokine (2020) 136:155292. doi: 10.1016/j.cyto.2020.155292
74. Colaianni G, Errede M, Sanesi L, Notarnicola A, Celi M, Zerlotin R, et al. Irisin correlates positively with BMD in a cohort of older adult patients and downregulates the senescent marker p21 in osteoblasts. J Bone Miner Res (2021) 36(2):305–14. doi: 10.1002/jbmr.4192
75. Colucci S, Colaianni G, Brunetti G, Ferranti F, Mascetti G, Mori G, et al. Irisin prevents microgravity-induced impairment of osteoblast differentiation in vitro during the space flight CRS-14 mission. FASEB J (2020) 34(8):10096–106. doi: 10.1096/fj.202000216R
76. Chen Z, Zhang Y, Zhao F, Yin C, Yang C, Wang X, et al. Recombinant irisin prevents the reduction of osteoblast differentiation induced by stimulated microgravity through increasing β-catenin expression. Int J Mol Sci (2020) 21(4):1259. doi: 10.3390/ijms21041259
77. Metzger CE, Narayanan SA, Phan PH, Bloomfield SA. Hindlimb unloading causes regional loading-dependent changes in osteocyte inflammatory cytokines that are modulated by exogenous irisin treatment. Microgravity (2020) 6:28. doi: 10.1038/s41526-020-00118-4
78. Jessen N, Goodyear LJ. Contraction signaling to glucose transport in skeletal muscle. J Appl Physiol (2005) 99(1):330–7. doi: 10.1152/japplphysiol.00175.2005
79. Shan T, Liang X, Bi P, Kuang S. Myostatin knockout drives browning of white adipose tissue through activating the AMPK-PGC1alpha-Fndc5 pathway in muscle. FASEB J (2013) 27(5):1981–9. doi: 10.1096/fj.12-225755
80. Ge X, Sathiakumar D, Lua BJ, Kukreti H, Lee M, McFarlane C. Myostatin signals through miR-34a to regulate Fndc5 expression and browning of white adipocytes. Int J Obes (Lond) (2017) 41(1):137–48. doi: 10.1038/ijo.2016.110
81. Chang JS, Kim TH, Nguyen TT, Park KS, Kim N, Kong ID. Circulating irisin levels as a predictive biomarker for sarcopenia: A cross-sectional community-based study. Geriatr Gerontol Int (2017) 17(11):2266–73. doi: 10.1111/ggi.13030
82. Park HS, Kim HC, Zhang D, Yeom H, Lim SK. The novel myokine irisin: clinical implications and potential role as a biomarker for sarcopenia in postmenopausal women. Endocrine (2019) 64(2):341–8. doi: 10.1007/s12020-018-1814-y
83. Zhao M, Zhou X, Yuan C, Li R, Ma Y, Tang X. Association between serum irisin concentrations and sarcopenia in patients with liver cirrhosis: a cross-sectional study. Sci Rep (2020) 10(1):16093. doi: 10.1038/s41598-020-73176-z
84. Sliwicka E, Cison T, Kasprzak Z, Nowak A, Pilaczynska-Szczesniak L. Serum irisin and myostatin levels after 2 weeks of high-altitude climbing. PloS One (2017) 12(7):e0181259. doi: 10.1371/journal.pone.0181259
85. Liu S, Fu P, Ning K, Wang R, Yang B, Chen J, et al. HIF-1alpha negatively regulates irisin expression which involves in muscle atrophy induced by hypoxia. Int J Mol Sci (2022) 23(2):887. doi: 10.3390/ijms23020887
86. Liu S, Fu P, Ning K, Wang R, Yang B, Chen J, et al. HIF-1α negatively regulates irisin expression which involves in muscle atrophy induced by hypoxia. Int J Mol Sci (2022) 23(2):887. doi: 10.3390/ijms23020887
87. Xiong Y, Wu Z, Zhang B, Wang C, Mao F, Liu X, et al. Fndc5 loss-of-function attenuates exercise-induced browning of white adipose tissue in mice. FASEB J (2019) 33(5):5876–86. doi: 10.1096/fj.201801754RR
88. Huh JY, Dincer F, Mesfum E, Mantzoros CS. Irisin stimulates muscle growth-related genes and regulates adipocyte differentiation and metabolism in humans. Int J Obes (Lond) (2014) 38(12):1538–44. doi: 10.1038/ijo.2014.42
89. Chang JS, Kong ID. Irisin prevents dexamethasone-induced atrophy in C2C12 myotubes. Pflugers Arch (2020) 472(4):495–502. doi: 10.1007/s00424-020-02367-4
90. Vadala G, Di Giacomo G, Ambrosio L, Cannata F, Cicione C, Papalia R, et al. Irisin recovers osteoarthritic chondrocytes In vitro. Cells (2020) 9(6):1478. doi: 10.3390/cells9061478
91. Huang CW, Chang YH, Lee HH, Wu JY, Huang JX, Chung YH, et al. Irisin, an exercise myokine, potently suppresses tumor proliferation, invasion, and growth in glioma. FASEB J (2020) 34(7):9678–93. doi: 10.1096/fj.202000573RR
92. Park J, Bae J, Lee J. Complex exercise improves anti-inflammatory and anabolic effects in osteoarthritis-induced sarcopenia in elderly women. Healthcare (Basel) (2021) 9(6):711. doi: 10.3390/healthcare9060711
93. Wang FS, Kuo CW, Ko JY, Chen YS, Wang SY, Ke HJ, et al. Irisin mitigates oxidative stress, chondrocyte dysfunction and osteoarthritis development through regulating mitochondrial integrity and autophagy. (2020) 9(9):810. doi: 10.3390/antiox9090810
94. Mao Y, Xu W, Xie Z, Dong Q. Association of irisin and CRP levels with the radiographic severity of knee osteoarthritis. Genet Test Mol Biomarkers (2016) 20(2):86–9. doi: 10.1089/gtmb.2015.0170
95. Li X, Zhu X, Wu H, Dyke TEV, Xu X, Morgan EF, et al. Roles and mechanisms of irisin in attenuating pathological features of osteoarthritis. Front Cell Dev Biol (2021) 9:703670. doi: 10.3389/fcell.2021.703670
96. Vadalà G, Giacomo G, Ambrosio L, Cannata F, Cicione C, Papalia R, et al. Irisin recovers osteoarthritic chondrocytes in vitro. Cells (2020) 9(6):1478. doi: 10.3390/cells9061478
97. Lee AS, Ellman MB, Yan D, Kroin JS, Cole BJ, Wijnen AJv, et al. A current review of molecular mechanisms regarding osteoarthritis and pain. Gene (2013) 527(2):440–7. doi: 10.1016/j.gene.2013.05.069
98. Jia S, Yang Y, Bai Y, Wei Y, Zhang H, Tian Y, et al. Mechanical stimulation protects against chondrocyte pyroptosis through irisin-induced suppression of PI3K/Akt/NF-kappaB signal pathway in osteoarthritis. Front Cell Dev Biol (2022) 10:797855. doi: 10.3389/fcell.2022.797855
99. Li X, Liu Y, Liu Q, Wang S, Ma Y, Jin Q. Recombinant human irisin regulated collagen II, matrix metalloproteinase-13 and the wnt/β-catenin and NF-κB signaling pathways in interleukin-1β-induced human SW1353 cells. Experimetal (2020) 19(4):2879–86. doi: 10.3892/etm.2020.8562
100. Lakosli SG, Eves ND, Douglas PS, Jones LW. Exercise rehabilitation in patients with cancer. Nat Rev Clin Oncol (2012) 9(5):288–96. doi: 10.1038/nrclinonc.2012.27
101. Timmons JA, Baar K, Davidsen PK, Atherton PJ. Is irisin a human exercise gene? Nature (2012) 488(7413):E9–10. doi: 10.1038/nature11364
102. Tsiani E, Tsakiridis N, Kouvelioti R, Jaglanian A, Klentrou P. Current evidence of the role of the myokine irisin in cancer. Cancers (Basel). (2021) 13(11):2628. doi: 10.3390/cancers13112628
103. Shi G, Tang N, Qui J, Zhang D, Huang F, Cheng Y, et al. Irisin stimulates cell proliferation and invasion by targeting the PI3K/AKT pathway in human hepatocellular carcinoma. Biochem. Biophys. Res. Commun (2017) 493(1):585–91. doi: 10.1016/j.bbrc.2017.08.148
104. Pinkowska A, Nowinska K, Ciesielska U, Podhorska-Okolow M. Irisin association with ki-67, MCM3 and MT-I/II in squamous cell carcinomas of the larynx. Biomolecules (2021) 12(1):52. doi: 10.3390/biom12010052
105. Ugur K, Aydin S, Kuloglu T, Artas G, Kocdor MA, Sahin İ, et al. Comparison of irisin hormone expression between thyroid cancer tissues and oncocytic variant cells. Cancer Manag Res (2019) 11:2595–603. doi: 10.2147/CMAR.S201979
106. Provatopoulou X, Georgiou GP, Kalogera E, Kalles V, Matiatou MA, Papapanagiotou I, et al. Serum irisin levels are lower in patients with breast cancer: association with disease diagnosis and tumor characteristics. BMC Cancer (2015) 15:898. doi: 10.1186/s12885-015-1898-1
107. Zhu T, Zhang W, Zhang Y, Lu E, Liu H, Liu X, et al. Irisin/FNDC5 inhibits the epithelial-mesenchymal transition of epithelial ovarian cancer cells via the PI3K/Akt pathway. Arch Gynecol Obstet (2022) 306(3):841–850. doi: 10.1007/s00404-022-06770-3
108. Zhang D, Zhang P, Li L, Tang N, Huang F, Kong X, et al. Irisin functions to inhibit malignant growth of human pancreatic cancer cells via downregulation of the PI3K/AKT signaling pathway. Onco Targets Ther (2019) 12:7243–9. doi: 10.2147/OTT.S214260
109. Kong G, Jiang Y, Sun X, Cao Z, Zhang G, Zhao Z, et al. Irisin reverses the IL-6 induced epithelial-mesenchymal transition in osteosarcoma cell migration and invasion through the STAT3/Snail signaling pathway. Oncol Rep (2017) 38(5):2647–56. doi: 10.3892/or.2017.5973
110. Liu J, Song N, Huang Y, Chen Y. Irisin inhibits pancreatic cancer cell growth via the AMPK-mTOR pathway. Sci Rep (2018) 8(1):15247. doi: 10.1038/s41598-018-33229-w
111. Park EJ, Myint PK, Ito A, Appiah MG, Darkwah S, Kawamoto E, et al. Integrin-ligand interactions in inflammation, cancer, and metabolic disease: Insights into the multifaceted roles of an emerging ligand irisin. Front Cell Dev Biol (2020) 8:588066. doi: 10.3389/fcell.2020.588066
112. Sattar N, Gill JMR, Alazawi W. Improving prevention strategies for cardiometabolic disease. Nat Med (2020) 26(3):320–5. doi: 10.1038/s41591-020-0786-7
113. El-Lebedy DH, Ibrahim AA, Ashmawy IO. Novel adipokines vaspin and irisin as risk biomarkers for cardiovascular diseases in type 2 diabetes mellitus. Diabetes Metab Syndr (2018) 12(5):643–8. doi: 10.1016/j.dsx.2018.04.025
114. Yin C, Hu W, Wang M, Lv W, Jia T, Xiao Y, et al. Irisin as a mediator between obesity and vascular inflammation in Chinese children and adolescents. J Nutr Metab (2020) 30(2):320–9. doi: 10.1016/j.numecd.2019.09.025
115. Dong X, Fu W, Deng Y, Jia L, Lin N, Li W, et al. Lower serum irisin levels are associated with the increasing mortality of cardiovascular and cerebrovascular diseases in hemodialysis patients. Ann Palliat Med (2021) 10(6):6052–61. doi: 10.21037/apm-21-406
116. Bi J, Zhang J, Ren Y, Du Z, Zhang Y, Liu C, et al. Exercise hormone irisin mitigates endothelial barrier dysfunction and microvascular leakage-related diseases. JCI Insight (2020) 5(13):e136277. doi: 10.1172/jci.insight.136277
117. Lin C, Guo Y, Xia Y, Li C, Xu X, Qi T, et al. FNDC5/Irisin attenuates diabetic cardiomyopathy in a type 2 diabetes mouse model by activation of integrin αV/β5-AKT signaling and reduction of oxidative/nitrosative stress. J Mol Cell Cardiol (2021) 160:27–41. doi: 10.1016/j.yjmcc.2021.06.013
118. Ho MY, Wang CY. Role of irisin in myocardial infarction, heart failure, and cardiac hypertrophy. Cells (2021) 10(8):2103. doi: 10.3390/cells10082103
119. Yue R, Zheng Z, Luo Y, Wang X, Lv M, Qin D, et al. NLRP3-mediated pyroptosis aggravates pressure overload-induced cardiac hypertrophy, fibrosis, and dysfunction in mice: cardioprotective role of irisin. Cell Death Discov (2021) 7(1):50. doi: 10.1038/s41420-021-00434-y
120. Li R, Wang X, Wu S, Wu Y, Chen H, Xin J, et al. Irisin ameliorates angiotensin II-induced cardiomyocyte apoptosis through autophagy. J Cell Physiol (2019) 234(10):17578–88. doi: 10.1002/jcp.28382
121. Li RL, Wu SS, Wu Y, Wang XX, Chen HY, Xin JJ, et al. Irisin alleviates pressure overload-induced cardiac hypertrophy by inducing protective autophagy via mTOR-independent activation of the AMPK-ULK1 pathway. J Mol Cell Cardiol (2018) 121:242–55. doi: 10.1016/j.yjmcc.2018.07.250
122. Saadeldin MK, Elshaer SS, Emara IA, Maged M, Abdel-Aziz AK. Serum sclerostin and irisin as predictive markers for atherosclerosis in Egyptian type II diabetic female patients: A case control study. PloS One (2018) 13(11):e0206761. doi: 10.1371/journal.pone.0206761
123. Guo W, Zhang B, Wang X. Lower irisin levels in coronary artery disease: a meta-analysis. J Minerva Endocrinologica (2020) 45(1):61–9. doi: 10.23736/S0391-1977.17.02663-3
124. Remuzgo-Martínez S, Rueda-Gotor J, Pulito-Cueto V, López-Mejías R, Corrales A, Lera-Gómez L, et al. Irisin as a novel biomarker of subclinical atherosclerosis. Cardiovasc Risk Severe Dis Axial Spondyloarthritis (2022) 13:894171. doi: 10.3389/fimmu.2022.894171
125. Chen J, Li K, Shao J, Lai Z, Gao R, Wang C, et al. Irisin suppresses nicotine-mediated atherosclerosis by attenuating endothelial cell migration, proliferation. Cell Cycle Arrest Cell Senescence (2022) 9:851603. doi: 10.3389/fcvm.2022.851603
126. Li K, Chen J, Wang C, Shao J, Lai Z, Yu X, et al. Irisin ameliorates nicotine-mediated atherosclerosis via inhibition of the PI3K pathway. Ann Transl Med (2021) 9(9):805. doi: 10.21037/atm-21-2072
127. Zhang M, Xu Y, Jiang L. Irisin attenuates oxidized low-density lipoprotein impaired angiogenesis through AKT/mTOR/S6K1/Nrf2 pathway. J Cell Physiol (2019) 234(10):18951–62. doi: 10.1002/jcp.28535
128. Lu J, Xiang G, Liu M, Mei W, Xiang L, Dong J. Irisin protects against endothelial injury and ameliorates atherosclerosis in apolipoprotein e-null diabetic mice. Atherosclerosis (2015) 243(2):438–48. doi: 10.1016/j.atherosclerosis.2015.10.020
129. Zheng G, Li H, Zhang T, Yang L, Yao S, Chan S, et al. Irisin protects macrophages from oxidized low density lipoprotein-induced apoptosis by inhibiting the endoplasmic reticulum stress pathway. Saudi J Biol Sci (2018) 25(5):849–57. doi: 10.1016/j.sjbs.2017.08.018
130. Deng X, Huang W, Peng J, Zhu TT, Sun XL, Zhou XY, et al. Irisin alleviates advanced glycation end products-induced inflammation and endothelial dysfunction via inhibiting ROS-NLRP3 inflammasome signaling. Inflammation (2018) 41(1):260–75. doi: 10.1007/s10753-017-0685-3
Keywords: irisin, beige fat, musculoskeletal homeostasis, cancer, liver, cardiovascular diseases
Citation: Liu S, Cui F, Ning K, Wang Z, Fu P, Wang D and Xu H (2022) Role of irisin in physiology and pathology. Front. Endocrinol. 13:962968. doi: 10.3389/fendo.2022.962968
Received: 07 June 2022; Accepted: 07 September 2022;
Published: 26 September 2022.
Edited by:
Isabelle Jéru, Assistance Publique Hopitaux De Paris, FranceReviewed by:
In Deok Kong, Yonsei University, South KoreaJian Yang, Third Affiliated Hospital of Chongqing Medical University, China
Copyright © 2022 Liu, Cui, Ning, Wang, Fu, Wang and Xu. This is an open-access article distributed under the terms of the Creative Commons Attribution License (CC BY). The use, distribution or reproduction in other forums is permitted, provided the original author(s) and the copyright owner(s) are credited and that the original publication in this journal is cited, in accordance with accepted academic practice. No use, distribution or reproduction is permitted which does not comply with these terms.
*Correspondence: Huiyun Xu, celldon@nwpu.edu.cn; Dongen Wang, wde2017@nwpu.edu.cn
†These authors have contributed equally to this work