- 1Faculty of Science and Engineering, Waseda University, Tokyo, Japan
- 2Institute of Physical Education, Keio University, Yokohama, Japan
- 3Graduate School of Advanced Science and Engineering, Waseda University, Tokyo, Japan
- 4Institute for Liberal Arts, Tokyo Institute of Technology, Tokyo, Japan
- 5Faculty of Sport Sciences, Waseda University, Saitama, Japan
- 6Research Center for Molecular Exercise Science, University of Physical Education, Budapest, Hungary
- 7Faculty of Sport Sciences, Surugadai University, Saitama, Japan
Background: Glucose and lipid tolerance reportedly exhibit diurnal variations, being lower in the evening than in the morning. Therefore, the effects of exercise on glucose and blood lipid levels at different times of the day may differ. This study aimed to investigate the effects of short-term endurance exercise intervention in the morning versus late afternoon on 24-h blood glucose variability and blood lipid levels.
Methods: Twelve healthy young men participated in a randomized crossover trial. The participants were assigned to morning (09:00–11:00) or late afternoon (16:00–18:00) endurance exercise for a week, consisting of supervised exercise sessions on Mondays, Wednesdays, and Fridays. In the morning and evening trials, the participants walked for 60 min on a treadmill at approximately 60% of maximal oxygen uptake (VO2max). Following a 2-week wash-out period, the participants performed the exercise training regimen at another time point. Continuous glucose monitoring was used to evaluate blood glucose fluctuations during each 24-h trial period. Blood samples were collected before and after each intervention to examine blood lipid and hormonal responses.
Results: Examination of the area under the curve (AUC) of the glucose level changes for 24 h after the late afternoon versus morning exercise intervention revealed significantly lower values for the former versus the latter (P < 0.01). The AUC of glucose level changes after each meal was also lower after the late afternoon versus morning intervention, and significantly lower values were observed in the late afternoon versus morning trial for breakfast and dinner (P < 0.05, P < 0.01). In addition, a significant decrease in triglycerides (TG) and TG/high-density lipoprotein cholesterol (HDL-C) was noted after versus before the late afternoon intervention (P < 0.05).
Conclusions: These results suggest that late afternoon endurance exercise is more effective than morning endurance exercise at improving 24-h glucose and triglyceride levels.
Introduction
The World Health Organization reported that an estimated 17.9 million people died from cardiovascular diseases (CVDs) in 2019, representing 32% of all global deaths. CVD is the leading cause of death globally (1). Hyperlipidemia and hyperglycemia are also independent risk factors of CVDs (2, 3). Type 2 diabetes and obesity are related to increased blood lipid and glucose levels (4, 5). Therefore, the prevention and improvement of hyperlipidemia and hyperglycemia are important for reducing the risk of CVDs.
Circadian rhythms in mammals are associated with the regulation of several physiological and biological functions, including body temperature, sleep-wake cycles, physical activity, hormone secretion, and metabolism, which are controlled by clock genes. This clock system collectively regulates a wide range of metabolic targets, including glucocorticoids (6), the master energy sensor adenosine monophosphate-activated protein kinase (AMPK) (7), the rate-limiting step in fatty acid and cholesterol synthesis (8, 9), and the hepatic cAMP response element-binding protein, which regulates gluconeogenesis (10). As a result, various metabolic processes, such as insulin sensitivity, insulin secretion, cholesterol synthesis, fat oxidation, and energy expenditure, are in rhythm throughout the 24-h daily cycle (11–13). In addition, the disruption of circadian rhythms is associated with the development of metabolic diseases such as obesity and diabetes. Therefore, the timing of exercise and nutritional intake should be considered accordingly to reduce the risk of obesity, diabetes, and other metabolic diseases.
It is well known that endurance exercise has a favorable effect on many established CVD-related risk factors (14, 15). Hence, exercise guidelines mention the exercise modality, intensity, duration, and frequency to prevent and improve hyperlipidemia and hyperglycemia (14, 15). However, no mention has been made regarding exercise timing. One reason for this is that the effects of exercise timing on organisms have not been adequately examined. However, glucose and lipid tolerance reportedly have diurnal variations, being lower in the evening than in the morning (16–18). Previous studies demonstrated that diurnal variations in glucose tolerance are so great that adults with normal glucose tolerance in the morning are metabolically equivalent to prediabetics in the evening (17, 19). In addition, postprandial triglyceride concentrations were higher in the evening than in the morning, possibly because of diurnal variations in lipid tolerance (20, 21). Therefore, different exercise timings exert different effects on glucose and blood lipid levels. Previous studies indicated that lipolysis and fat oxidation are higher in the evening versus morning acute endurance exercise (22, 23).
However, the effects of exercise at different times of day (e.g., morning versus late afternoon) on glucose levels and lipid markers have not been clarified. Thus, this study aimed to compare the effects of short-term endurance exercise performed in the morning versus late afternoon on glucose and blood lipid levels in healthy young men.
Methods
Participants
Twelve healthy young men aged ≥20 years (21.8 ± 0.2 years, mean ± SE) without regular exercise habits participated in the present study after providing informed consent. The participants were recruited only if they met the following inclusion criteria (1): no anti-obesity or anti-diabetic supplement use (2); no medical diagnosis of diabetes or dyslipidemia (3); no hypertension (systolic blood pressure >140 mmHg, diastolic blood pressure >90 mmHg); and (4) no use of blood glucose/lipid-lowering or related medications. Therefore, the study included only participants with no medication use, disease history, or smoking habits at the initial recruitment stage (Figure 1).
This study was conducted in Tokyo (Japan) between August 2019 and February 2021. The participants made no special lifestyle changes during the study period. This study was approved by the Ethics Committee of Waseda University (approval no. 2018-136 (1)) and conducted in accordance with the Declaration of Helsinki guidelines. The human trial in the present study was registered at https://center6.umin.ac.jp/cgi-bin/ctr/ctr_view_reg.cgi?recptno=R000042147 as UMIN000036994 .
Preliminary measurements
Height, body weight, and body fat percentage were measured at the participants’ first visit to the laboratory. All participants underwent an incremental exercise test to exhaustion on a treadmill to determine the maximal oxygen uptake (VO2max). The incremental exercise test used a treadmill (MAT-2700; Fukuda Denshi, Tokyo, Japan) and the Bruce protocol, in which the incline and speed were increased every 3 min (24). The results of the incremental exercise test were used to calculate the 60% VO2max value for each participant.
Endurance exercise intervention
A randomized crossover design was used in this study. Participants were assigned to either morning (9:00–11:00) or late afternoon (16:00–18:00) endurance exercise training for a week, which consisted of supervised exercise sessions on Mondays, Wednesdays, and Fridays. Exercise intensity was changed by adjusting the speed of the treadmill 5–10 min after the start of loading. Heart rate was measured using a heart rate sensor to align the exercise intensity for each exercise trial. (Polar H10; Polar, Tokyo, Japan). In the morning and late afternoon trials, the participants walked for 60 min on a treadmill at approximately 60% VO2max. Following a 2-week wash-out period, the participants performed the exercise training regimen in another trial (Figure 2). All participants were instructed to follow the same daily routine with a similar diet as much as possible during both trial periods.
Anthropometry
Body weight was measured to the nearest 0.1 kg using a digital balance (Inbody 270; Inbody Inc., Tokyo, Japan), and height was measured to the nearest 0.1 cm using a wall-mounted stadiometer (YS-OA; As One Corp., Tokyo, Japan). Body mass index was calculated as the weight in kilograms divided by the square of height in meters. Body fat Percentage was measured via direct segmental multi-frequency bioimpedance analysis (InBody270; Inbody Inc., Tokyo, Japan).
Measurement of physical activity
All participants were asked to wear a triaxial accelerometer (Active style Pro HJA-750C; Omron Corp., Kyoto, Japan) during the trial period. They wore the triaxial accelerometer each day at all times from morning to night except during showers and sleeping hours. We selected those wearers who wore the device for at least 600 min and averaged the data to calculate daily physical activity. We used moderate-to-vigorous physical activity (MVPA) and step counts required for the assessment (25).
Measurement of dietary and chronotype
To determine the chronotype of each participant (morningness to eveningness), a lifestyle survey was conducted using the Morningness-Eveningness Questionnaire (26). The MEQ has consisted of 19 questions related to sleep habits, sleepiness, and the preferred time for daily performance. The scores ranged from 16 to 86. Based on their scores, the participants were divided into the following three chronotype groups: morningness (score 59–86), intermediate (score 42–58), or eveningness (score 16–41). The Food Frequency Questionnaire was used to assess the participants’ dietary and nutritional intake. Most FFQs for Japanese people are highly effective for estimating nutrients (27). The FFQ asks about the frequency of food intake (once daily, once or twice a week, once or twice a month, etc.) and the approximate serving size (weight, volume, size). The average daily energy intake was depicted as kilocalories per day (kcal/day). The participants were asked to record the timing of each meal intake during each trial period (Table S1).
Measurement and analysis of glucose levels
All participants were asked to wear a continuous glucose monitoring system (FreeStyle Libre Pro; Abbott Laboratories, Chicago, IL, US) to collect the continuous measurement of glucose levels during the entire trial. Once applied, the system can continuously measure glucose levels in 15 min intervals for 14 days. The sensor was applied to the back of the upper arm. The sensor continually stores data on glucose levels. In both trials, 24-h glucose levels and area under the curve (AUC) were calculated. The 24-h glucose variability for each exercise trial was examined from Tuesday through Friday. The 24-h glucose variability from Saturday after each intervention to Thursday of the following week was also examined. The 24-h glucose variability measurements were taken from 00:00 h to 24:00 h on that day. Postprandial glucose fluctuations were examined up to 4-h postprandial based on each meal time that was recorded. The parameters used to determine glycemic variability included mean glucose level (MAX), minimum glucose level (MIN), mean amplitude of glycemic excursion (MAGE), and J-index. The J-index was calculated using the following formula (28): J-index = 0.001 × (mean ± standard deviation)2.
Measurement of blood glucose and lipid levels
Venous blood samples were collected at baseline and after each trial (Figure 2). The participants were required to abstain from any intense exercise for at least one day before the collection of the blood sample and fast for at least 10 h overnight. The blood samples were collected at 09:00–10:00, and blood samples after each trial intervention were drawn at least 48 h after the end of the last trial. Blood samples were collected in a tube containing thrombin and a heparin-neutralizing agent, and a tube containing ethylenediaminetetraacetic acid (EDTA)-Na2. After being collected, the blood intended for serum analysis was allowed to stand for 30 min at room temperature, whereas the blood intended for plasma analysis was centrifuged at 3500 rpm for 10 min. After centrifugation, the serum and plasma samples were extracted from the respective blood collection tubes and stored at −80°C until the assay. Plasma insulin, glucose, and serum blood lipids (triglycerides [TG]; high-density lipoprotein cholesterol [HDL-C]; low-density lipoprotein cholesterol [LDL-C]; apolipoprotein A1 [ApoA1]; apolipoprotein B [ApoB]) were analyzed by Kotobiken Medical Laboratories Inc. (Tokyo, Japan). Cholesterol uptake capacity (CUC) was analyzed by Sysmex Corporation (Hyogo, Japan).
Statistical analysis
Predictive Analytics Software for Windows (SPSS Japan Inc. Tokyo, Japan) was used for the data analysis. The total sample size was calculated to detect the medium effects. Using a medium effect size (f = 0.25), a sample size of 12 participants was deemed sufficient to provide 80% power to detect (α-error probability value set at 0.05) within–between interactions (two trials: morning and evening trials) using measures taken at morning and late afternoon trials time points (G*Power, version 3.1.9.2; Universitat Kiel, Germany). The normal or non-normal distributions of the data were analyzed using the Shapiro–Wilk test. To compare changes in the diurnal and postprandial glucose levels between trials, a two-way repeated-measures analysis of variance (ANOVA) was used (effects of trial and time were used as factors). Wilcoxon’s signed-rank test was used for the 24-h AUC per day of the intervention period for each trial, for which normality and equal variances were not assumed. Repeated one-way ANOVA was also performed to compare blood parameters at baseline versus after the intervention for each trial. The Friedman test was used for TG and TG/HDL-C, for which normality and equal variances were not assumed. In addition, a paired t-test was used to compare glucose parameters and AUC during the intervention period in both trials. Statistical significance was set at P < 0.05.
Results
Characteristics of study participants
Participant characteristics are shown in Table 1.
Comparison of physical activity levels and mealtime
There were no significant inter-trial differences in the amount of physical activity (step count and MVPA) or mealtime during any trial period (Table S1).
Comparison of the heart rate during the exercise in each trial
There was no difference in heart rate between the morning and late afternoon trials during exercise (Figure S1).
Comparison of diurnal changes in glucose concentrations and AUC of each trial
There were no significant differences in 24-h glucose variability during the intervention period in either trial (Figure S2A). The AUC of blood glucose variability during the intervention period was lower in the late afternoon trial; however, the difference was not statistically significant (Figure S2B). The daily AUC for each trial did not differ significantly between trials (Figure S3). On the other hand, in the 24-h blood glucose variation after the intervention of each trial, the late afternoon trial showed lower values than the morning trial (trial, P = 0.002; time, P < 0.001; interaction, P = 0.571), and the AUC was significantly lower (P < 0.01) (Figures 3A, B). In addition, the daily AUC after the intervention for each trial was lower in the late afternoon versus morning trial, with significantly lower Thursday AUC values (Figure 3C; P < 0.05).
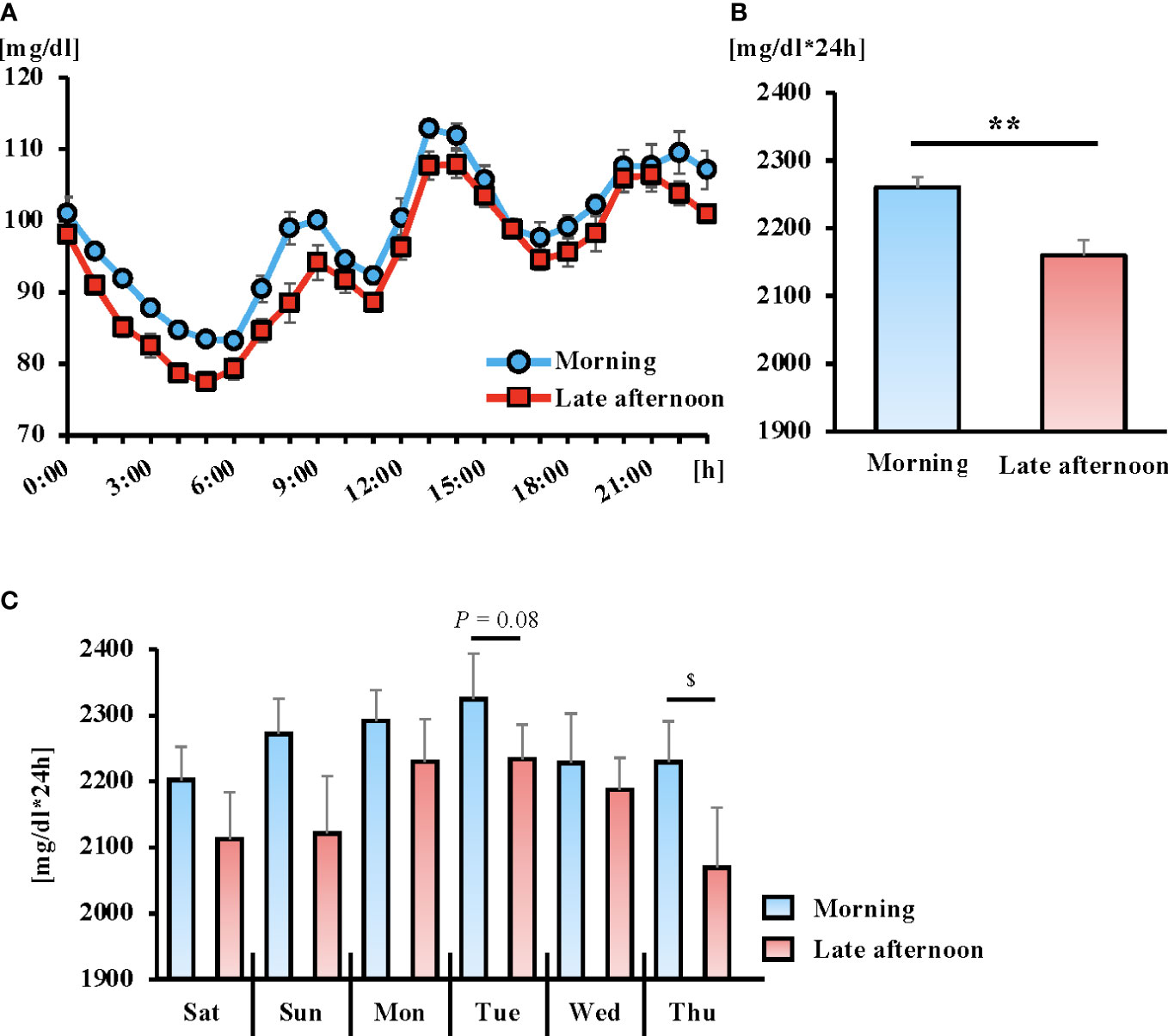
Figure 3 The 6-day average of the 24-hour blood glucose concentrations (A) and area under the curve (AUC) (B) following the exercise intervention. Daily AUC for 6 days following the exercise intervention (C). Blue and red lines indicate the morning and late afternoon trials, respectively. All data are presented as mean ± standard error. **P < 0.01 compared to the level in the Morning trial (paired t-test). $P < 0.05 compared to the level in the Morning trial (Wilcoxon). Blood glucose fluctuations were calculated from the 24-hour blood glucose fluctuations after the intervention for each trial (from Saturday to next Thursday). The 24-h glucose variability measurements were taken from 00:00 h to 24:00 h on that day.
Comparison of postprandial glucose concentrations and AUC
Postprandial blood glucose fluctuations were lower in the late afternoon versus morning trial for all three meals. The AUC after breakfast and dinner was significantly lower in the late afternoon versus morning trial (Figure 4; P < 0.05, P < 0.01, respectively).
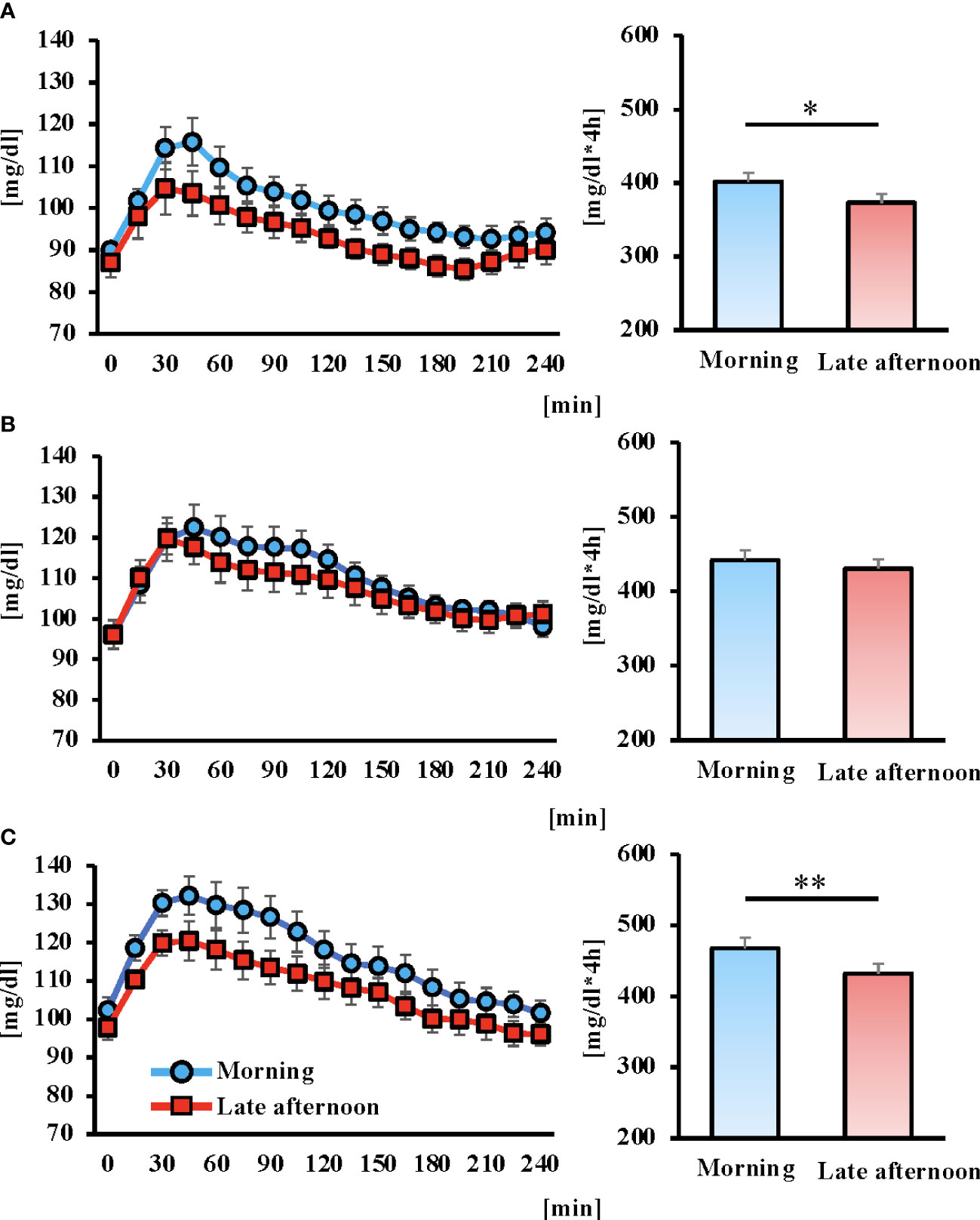
Figure 4 Concentrations of glucose of postprandial 4 hours and area under the curve (AUC) for 6 days after intervention in each trial. Breakfast (A), lunch (B) Dinner (C), and the blue and red lines indicate the morning and late afternoon trials, respectively. All data are presented as mean ± standard error. *P < 0.05, **P < 0.01 compared to the level in the Morning trial (paired t-test).
Comparison of glucose parameters between trials
The mean, minimum, and J-index values were significantly lower in the late afternoon versus morning trial (P < 0.01), whereas there were no significant differences in MAX and MAGE between trials (Table 2).
Blood lipids and parameters before versus after intervention by trial
A significant decrease in TG and TG/HDL-C ratio after the intervention was noted in the late afternoon trial only (Figure 5; P < 0.05, both). However, there were no significant changes in glucose, insulin, HDL-C, LDL-C, ApoA1, ApoB, ApoB/Apo A1, or CUC in either trial (Table 3).
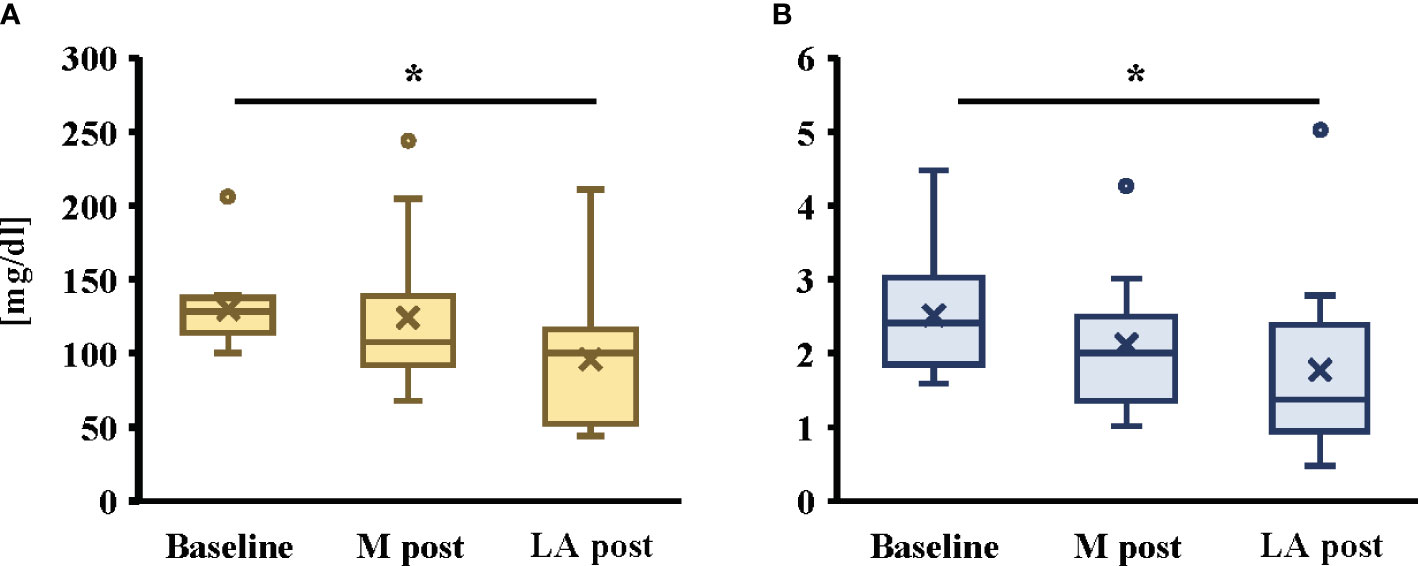
Figure 5 Changes in TG (A) and TG/HDL-C ratio (B) by morning or late afternoon of short-term endurance exercise. All data are presented as mean ± standard error. *P < 0.05 compared to the level in the Morning trial (Friedman). TG: triglycerides, HDL-C: high-density lipoprotein cholesterol, M post: After the intervention of morning trial, LA post: After the intervention of late afternoon trial. The participants were required to abstain from any intense exercise for at least one day before the collection of the blood sample and fast for at least 10 h overnight. The blood samples were collected at 09:00–10:00, and blood samples after each trial intervention were drawn at least 48 h after the end of the last trial.
Discussion
The present study examined the effects of short-term morning or late afternoon endurance exercise on diurnal variations in blood glucose and lipid levels in healthy young men. The diurnal variation in blood glucose levels after the exercise intervention was lower in the late afternoon versus morning trial. Blood glucose fluctuations using AUC showed significantly lower values in the late afternoon versus morning trial. The AUC of blood glucose fluctuation after each meal also showed lower values in the late afternoon than morning trial for all three meals and significantly lower values in the late afternoon versus morning trial for breakfast and dinner. In addition, only the late afternoon trial showed significant reductions in TG and TG/HDL-C levels after versus before the intervention. These results suggest that late afternoon endurance exercise may be more effective than morning endurance exercise at improving 24-h glucose and triglyceride levels.
The insulin-sensitizing effect of exercise is explained by the AMPK-mediated translocation of GLUT4 to the subsarcolemmal membrane, thereby promoting insulin-stimulated glucose uptake into muscle cells (29). Indeed, previous studies in humans reported that the interaction between exercise-induced AMPK phosphorylation and the subsequent insulin-stimulated activation of GLUT4 alleviates 24-h hyperglycemia (30, 31). In contrast, glucose tolerance exhibits diurnal variation and is reportedly lower in the evening versus morning (32). In addition, insulin sensitivity and beta cell responsiveness to glucose, which are thought to contribute to decreased glucose tolerance, are lower at dinner than at breakfast (33, 34). Thus, temporal variations in insulin sensitivity and the time dependence of the insulin secretion rate are thought to contribute to the diurnal variation in glucose metabolism. Therefore, the effects of exercise on blood glucose fluctuations at different times of day may differ. In the present study, diurnal fluctuations in blood glucose levels were lower in the late afternoon versus morning trial. A previous study of patients with type 2 diabetes reported that afternoon high-intensity interval training improved glycemic profiles more effectively than morning training (35). These findings are consistent with data from mice showing that glycolytic activation is more specific to exercise during the early active phase than during the early rest phase (36). Therefore, these results show that glycemic control during endurance exercise is more effective in the late afternoon versus morning.
The mechanism that promotes glucose initiatives in exercise has been shown to involve myokines, which are secreted by muscle contraction during exercise. IL-6, one of the myokines, has been shown to have multifunctional properties with important roles in regulating immune and inflammatory responses and is secreted in a manner dependent on exercise intensity and duration of exercise (37). Furthermore, IL-6 has been shown to be involved in glucose metabolism, facilitating the uptake of blood glucose into muscle cells; IL-6 induces GLUT4 translocation onto the plasma membrane by activating AMPK in muscle cells (38). The resulting translocation of GLUT4 receptors to the muscle cell membrane promotes blood glucose uptake and lowers blood glucose levels. The previous study has shown that changes in blood IL-6 concentrations after acute endurance exercise are higher in the evening than in the morning (22). Therefore, differences in the change in IL-6 concentrations after morning or late afternoon endurance exercise may have influenced the results. Another possibility is its association with insulin secretory function. However, blood insulin levels in the morning or late afternoon endurance exercise were not significantly different between trials. Therefore, the effect of insulin secretory function is considered to be small. On the other hand, previous studies have shown that short-term endurance exercise increases glucagon-like peptide-1 (GLP-1) and improves beta-cell function (39). Furthermore, a previous study has shown that the endocrine function of incretin extends beyond its stimulatory effect on insulin secretion, and GLP-1/glucose-dependent insulinotropic polypeptide (GIP) receptors are also found in skeletal muscle, a cell outside the pancreas (40). In other words, incretins have been shown to regulate glucose uptake into muscle in an insulin-independent manner. Therefore, late afternoon endurance exercise may have affected diurnal blood glucose fluctuations by improving beta cells and increasing incretin secretion. However, the present study did not include myokine or incretin, and more detailed studies are needed in the future.
The effect of glycemic control on short-term endurance exercise lasts for up to a week. Muscle sensitivity to insulin stimulation increases over several hours (up to 48 h) after exercise (41). In addition to the effects of acute exercise, insulin activity is reportedly enhanced over a longer period with repeated exercise (41). The 24-h blood glucose variability after the endurance exercise intervention in this study was lower in the late afternoon than morning trial. Furthermore, the effect lasted for approximately 1 week. Phosphatidylinositol 3-kinase (PI 3-kinase) is an important step in the regulation of glucose transport, and its activation is required for insulin-mediated glucose transport in insulin-sensitive tissues such as skeletal muscle (42, 43). Indeed, PI 3-kinase plays a specific role in insulin-stimulated glucose transport (42, 43). Short-term exercise training reportedly enhances insulin-stimulated PI 3-kinase activity in humans and rodents (44, 45). These findings suggest that enhanced insulin signaling may contribute to the increased insulin action of exercise in human skeletal muscles. Thus, insulin action may be more enhanced after late afternoon versus morning endurance exercise.
TG and cholesterol synthesis show diurnal variations in which their levels are higher at night (46, 47). In other words, lipid tolerance is lower in the evening than in the morning. In fact, evening statin intake more effectively improves cholesterol levels (48). Therefore, the effect of exercise on blood lipid levels may vary according to time of day. TG level is an independent risk factor for CVDs. Epidemiological studies have shown a higher prevalence of dyslipidemia in patients with versus those without CVDs, indicating an association between elevated serum TG levels and an increased risk of CVDs (49, 50). In the present study, TG levels decreased significantly after versus before the intervention only in the late afternoon endurance exercise trial. Previous studies demonstrated that exercise improves the risk of CVD, including dyslipidemia and hyperglycemia (51, 52). Another previous study examined the effects of different exercise timings over 12 weeks on blood lipids and inflammatory markers in 330 patients with cardiovascular disease. The results showed more improvement in LDL-C with evening exercise compared to morning exercise (53). This indicates that the effect of exercise on blood lipid levels may vary according to time of day.
Dyslipidemia is a major risk factor for CVDs, and exercise is a beneficial way to improve blood lipid levels (54). The decrease in TG levels observed in this study may be due to lipoprotein lipase (LPL). In a previous study, exercise reportedly increased LPL mRNA expression and decreased blood TG levels (55). Furthermore, LPL activity in response to a meal load was lower in the evening than in the morning (56). Therefore, the effect of late afternoon exercise on TG levels may have been more pronounced by promoting LPL activity. However, the contribution of LPL to reducing blood TG levels obtained in this study has not been examined and requires further investigation.
No differences in HDL-C or LDL-C levels were found in morning versus late afternoon endurance exercise. A previous study demonstrated that exercise can improve HDL-C levels (57). On the other hand, a sufficient training volume is necessary to improve blood cholesterol levels, while total energy expenditure and intensity are important factors related to improvement (52). The short-term endurance exercise focused on in this study may have been insufficient to cause changes in blood cholesterol because its type and intensity differed from those in the previous study. Furthermore, the diurnal values of TG fluctuate by 33–63%, whereas LDL fluctuates by less than 10%, and HDL-C shows no or very small diurnal fluctuations (18, 20, 58). ApoA1 and ApoB also have diurnal fluctuations of 24 and 12 h, respectively, but their diurnal fluctuations are small (5–6%) (18). Therefore, their effects on morning or late afternoon endurance exercise may be small.
This study has several limitations. First, it included only healthy young men. In a previous study, men were shown to have up to twice as high postprandial TG concentrations and greater diurnal variation than women (59). These sex-based differences are thought to be due to the effects of estrogen on lipid metabolism (60). Therefore, differences in the time of day of exercise implementation to TG may show different results according to sex. Second, a previous study has shown similar diurnal variation in glucose tolerance in patients with type 2 diabetes between morning and evening (61). A previous study in overweight with type 2 diabetes and pre-diabetic subjects showed similar effects on glycemic control during a 12-week morning and evening exercise intervention (62). Therefore, different subjects may have different effects on glycemic variability at different times of day during exercise. Third, the time of exercise intervention used in this study differs from previous studies (22, 23, 62). Hormones and other factors involved in energy metabolism have been shown to have diurnal variations (22, 63). Therefore, the effects on glucose fluctuations and blood lipids may differ depending on the time of day when exercise is performed. Finally, phosphorylation of the AMPK subunit complexes during exercise exhibits diurnal variation and is highly dependent on exercise intensity and type (64, 65). Hence, it is necessary to examine the effects of exercise on blood glucose fluctuations at different exercise times and consider exercise intensity and type.
Conclusion
In conclusion, our study findings demonstrated that short-term evening endurance exercise was more effective than morning endurance exercise at controlling blood glucose levels during the day, an effect that lasted for approximately 1 week. Evening exercise was more effective at improving blood lipid levels than morning exercise.
Data availability statement
The original contributions presented in this study are included in the article/Supplementary Material, and further inquiries can be directed to the corresponding author.
Ethics statement
The studies involving human participants were reviewed and approved by Ethics Committee for Humans at Waseda University. The patients/participants provided their written informed consent to participate in this study.
Author contributions
The authors’ responsibilities were as follows: H-KK, SF, and SS, conceptualization; SF and H-KK, data curation; H-KK, SF, and MT, formal analysis; H-KK and SS, funding acquisition; H-KK, SF, HC, TN, and MT, investigation; H-KK, MT, and SS, study methodology; H-KK and SS, project administration; SS and H-KK, project resources; SS, supervision; H-KK and SF, visualization; H-KK, SF, and SS, writing—original draft; MT, TI, ZR, and SZS, writing, review, and editing. All authors have read and approved the final manuscript for publication.
Funding
This work was supported by the Japan Society for the Promotion of Science (KAKENHI grant numbers 20K19689 and 18K17940) to H-KK and 19H01089 to SS), and the JST-Mirai Program (JMPJM120D5 for SS), Japan. The funder was not involved in the study design; data collection, analysis, and interpretation; writing of this article; or the decision to submit the article for publication.
Conflict of interest
The authors declare that the research was conducted in the absence of any commercial or financial relationships that could be construed as a potential conflict of interest.
Publisher’s note
All claims expressed in this article are solely those of the authors and do not necessarily represent those of their affiliated organizations, or those of the publisher, the editors and the reviewers. Any product that may be evaluated in this article, or claim that may be made by its manufacturer, is not guaranteed or endorsed by the publisher.
Supplementary material
The Supplementary Material for this article can be found online at: https://www.frontiersin.org/articles/10.3389/fendo.2022.957239/full#supplementary-material
References
1. Organization WH. Cardiovascular diseases (CVDs) (2021). Available at: https://www.who.int/news-room/fact-sheets/detail/cardiovascular-diseases-(cvds)#:~:text=Cardiovascular%20diseases%20(CVDs)%20are%20the,%2D%20and%20middle%2Dincome%20countries.
2. Borén J, Matikainen N, Adiels M, Taskinen MR. Postprandial hypertriglyceridemia as a coronary risk factor. Clin Chim Acta (2014) 431:131–42. doi: 10.1016/j.cca.2014.01.015
3. Pirillo A, Norata GD, Catapano AL. Postprandial lipemia as a cardiometabolic risk factor. Curr Med Res Opin (2014) 30(8):1489–503. doi: 10.1185/03007995.2014.909394
4. Tomlinson B, Chan P, Lam CWK. Postprandial hyperlipidemia as a risk factor in patients with type 2 diabetes. Expert Rev Endocrinol Metab (2020) 15(3):147–57. doi: 10.1080/17446651.2020.1750949
5. Lairon D, Defoort C. Effects of nutrients on postprandial lipemia. Curr Vasc Pharmacol (2011) 9(3):309–12. doi: 10.2174/157016111795495576
6. Balsalobre A, Brown SA, Marcacci L, Tronche F, Kellendonk C, Reichardt HM, et al. Resetting of circadian time in peripheral tissues by glucocorticoid signaling. Science. (2000) 289(5488):2344–7. doi: 10.1126/science.289.5488.2344
7. Jordan SD, Lamia KA. AMPK at the crossroads of circadian clocks and metabolism. Mol Cell Endocrinol (2013) 366(2):163–9. doi: 10.1016/j.mce.2012.06.017
8. Sitaula S, Zhang J, Ruiz F, Burris TP. Rev-erb regulation of cholesterologenesis. Biochem Pharmacol (2017) 131:68–77. doi: 10.1016/j.bcp.2017.02.006
9. Feng D, Liu T, Sun Z, Bugge A, Mullican SE, Alenghat T, et al. A circadian rhythm orchestrated by histone deacetylase 3 controls hepatic lipid metabolism. Science. (2011) 331(6022):1315–9. doi: 10.1126/science.1198125
10. Zhang EE, Liu Y, Dentin R, Pongsawakul PY, Liu AC, Hirota T, et al. Cryptochrome mediates circadian regulation of cAMP signaling and hepatic gluconeogenesis. Nat Med (2010) 16(10):1152–6. doi: 10.1038/nm.2214
11. Li MD, Li CM, Wang Z. The role of circadian clocks in metabolic disease. Yale J Biol Med (2012) 85(3):387–401.
12. Gerhart-Hines Z, Lazar MA. Circadian metabolism in the light of evolution. Endocr Rev (2015) 36(3):289–304. doi: 10.1210/er.2015-1007
13. Marcheva B, Ramsey KM, Peek CB, Affinati A, Maury E, Bass J. Circadian clocks and metabolism. Handb Exp Pharmacol (2013) 217):127–55. doi: 10.1007/978-3-642-25950-0_6
14. Haskell WL, Lee IM, Pate RR, Powell KE, Blair SN, Franklin BA, et al. Physical activity and public health: updated recommendation for adults from the American college of sports medicine and the American heart association. Med Sci Sports Exerc. (2007) 39(8):1423–34. doi: 10.1249/mss.0b013e3180616b27
15. Garber CE, Blissmer B, Deschenes MR, Franklin BA, Lamonte MJ, Lee IM, et al. American College of sports medicine position stand. quantity and quality of exercise for developing and maintaining cardiorespiratory, musculoskeletal, and neuromotor fitness in apparently healthy adults: guidance for prescribing exercise. Med Sci Sports Exerc. (2011) 43(7):1334–59. doi: 10.1249/MSS.0b013e318213fefb
16. Lindgren O, Mari A, Deacon CF, Carr RD, Winzell MS, Vikman J, et al. Differential islet and incretin hormone responses in morning versus afternoon after standardized meal in healthy men. J Clin Endocrinol Metab (2009) 94(8):2887–92. doi: 10.1210/jc.2009-0366
17. Jarrett RJ, Baker IA, Keen H, Oakley NW. Diurnal variation in oral glucose tolerance: blood sugar and plasma insulin levels morning, afternoon, and evening. Br Med J (1972) 1(5794):199–201. doi: 10.1136/bmj.1.5794.199
18. Rivera-Coll A, Fuentes-Arderiu X, Díez-Noguera A. Circadian rhythmic variations in serum concentrations of clinically important lipids. Clin Chem (1994) 40(8):1549–53. doi: 10.1093/clinchem/40.8.1549
19. Carroll KF, Nestel PJ. Diurnal variation in glucose tolerance and in insulin secretion in man. Diabetes. (1973) 22(5):333–48. doi: 10.2337/diab.22.5.333
20. Sennels HP, Jørgensen HL, Fahrenkrug J. Diurnal changes of biochemical metabolic markers in healthy young males - the bispebjerg study of diurnal variations. Scand J Clin Lab Invest. (2015) 75(8):686–92. doi: 10.3109/00365513.2015.1080385
21. van Oostrom AJ, Castro Cabezas M, Harmelink MJ, Twickler TB, Remijnse TA, Erkelens DW. [Diurnal triglyceride profiles in 30 young healthy men as a function of diet, fasting triglyceride levels, body composition and insulin sensitivity]. Ned Tijdschr Geneeskd. (1999) 143(37):1868–72.
22. Kim HK, Konishi M, Takahashi M, Tabata H, Endo N, Numao S, et al. Effects of acute endurance exercise performed in the morning and evening on inflammatory cytokine and metabolic hormone responses. PloS One (2015) 10(9):e0137567. doi: 10.1371/journal.pone.0137567
23. Mohebbi H, Azizi M. Maximal fat oxidation at the different exercise intensity in obese and normal weight men in the morning and evening. J Sport Exerc Psychol (2011) 6(1):49–58. doi: 10.4100/jhse.2011.61.06
24. Kim HK, Ando K, Tabata H, Konishi M, Takahashi M, Nishimaki M, et al. Effects of different intensities of endurance exercise in morning and evening on the lipid metabolism response. J Sports Sci Med (2016) 15(3):467–76.
25. Mâsse LC, Fuemmeler BF, Anderson CB, Matthews CE, Trost SG, Catellier DJ, et al. Accelerometer data reduction: a comparison of four reduction algorithms on select outcome variables. Med Sci Sports Exerc. (2005) 37(11 Suppl):S544–54. doi: 10.1249/01.mss.0000185674.09066.8a
26. Horne JA, Ostberg O. A self-assessment questionnaire to determine morningness-eveningness in human circadian rhythms. Int J Chronobiol. (1976) 4(2):97–110.
27. Wakai K. A review of food frequency questionnaires developed and validated in Japan. J Epidemiol (2009) 19(1):1–11. doi: 10.2188/jea.je20081007
28. Wójcicki JM. "J"-index. a new proposition of the assessment of current glucose control in diabetic patients. . Horm Metab Res (1995) 27(1):41–2. doi: 10.1055/s-2007-979906
29. Kjøbsted R, Munk-Hansen N, Birk JB, Foretz M, Viollet B, Björnholm M, et al. Enhanced muscle insulin sensitivity after Contraction/Exercise is mediated by AMPK. Diabetes. (2017) 66(3):598–612. doi: 10.2337/db16-0530
30. Wojtaszewski JF, Hansen BF, Kiens B, Richter EA. Insulin signaling in human skeletal muscle: time course and effect of exercise. Diabetes. (1997) 46(11):1775–81. doi: 10.2337/diab.46.11.1775
31. Wojtaszewski JF, Hansen BF, Kiens GB, Markuns JF, Goodyear LJ, Richter EA. Insulin signaling and insulin sensitivity after exercise in human skeletal muscle. Diabetes. (2000) 49(3):325–31. doi: 10.2337/diabetes.49.3.325
32. Stenvers DJ, Scheer F, Schrauwen P, la Fleur SE, Kalsbeek A. Circadian clocks and insulin resistance. Nat Rev Endocrinol (2019) 15(2):75–89. doi: 10.1038/s41574-018-0122-1
33. Takahashi M, Ozaki M, Kang MI, Sasaki H, Fukazawa M, Iwakami T, et al. Effects of meal timing on postprandial glucose metabolism and blood metabolites in healthy adults. Nutrients (2018) 10(11):1763 doi: 10.3390/nu10111763
34. Van Cauter E, Polonsky KS, Scheen AJ. Roles of circadian rhythmicity and sleep in human glucose regulation. Endocr Rev (1997) 18(5):716–38. doi: 10.1210/edrv.18.5.0317
35. Savikj M, Gabriel BM, Alm PS, Smith J, Caidahl K, Björnholm M, et al. Afternoon exercise is more efficacious than morning exercise at improving blood glucose levels in individuals with type 2 diabetes: a randomised crossover trial. Diabetologia. (2019) 62(2):233–7. doi: 10.1007/s00125-018-4767-z
36. Sato S, Basse AL, Schönke M, Chen S, Samad M, Altıntaş A, et al. Time of exercise specifies the impact on muscle metabolic pathways and systemic energy homeostasis. Cell Metab (2019) 30(1):92–110.e4. doi: 10.1016/j.cmet.2019.03.013
37. Pedersen BK, Febbraio MA. Muscle as an endocrine organ: focus on muscle-derived interleukin-6. Physiol Rev (2008) 88(4):1379–406. doi: 10.1152/physrev.90100.2007
38. Pedersen BK, Febbraio MA. Muscles, exercise and obesity: skeletal muscle as a secretory organ. Nat Rev Endocrinol (2012) 8(8):457–65. doi: 10.1038/nrendo.2012.49
39. Malin SK, Francois ME, Eichner NZM, Gilbertson NM, Heiston EM, Fabris C, et al. Impact of short-term exercise training intensity on β-cell function in older obese adults with prediabetes. J Appl Physiol (1985) 125(6):1979–86. doi: 10.1152/japplphysiol.00680.2018
40. Abdulla H, Phillips B, Smith K, Wilkinson D, Atherton PJ, Idris I. Physiological mechanisms of action of incretin and insulin in regulating skeletal muscle metabolism. Curr Diabetes Rev (2014) 10(5):327–35. doi: 10.2174/1573399810666141017153749
41. Wojtaszewski JF, Richter EA. Effects of acute exercise and training on insulin action and sensitivity: focus on molecular mechanisms in muscle. Essays Biochem (2006) 42:31–46. doi: 10.1042/bse0420031
42. Shepherd PR, Withers DJ, Siddle K. Phosphoinositide 3-kinase: the key switch mechanism in insulin signalling. Biochem J (1998) 333(Pt 3):471–90. doi: 10.1042/bj3330471
43. Terauchi Y, Tsuji Y, Satoh S, Minoura H, Murakami K, Okuno A, et al. Increased insulin sensitivity and hypoglycaemia in mice lacking the p85 alpha subunit of phosphoinositide 3-kinase. Nat Genet (1999) 21(2):230–5. doi: 10.1038/6023
44. Chibalin AV, Yu M, Ryder JW, Song XM, Galuska D, Krook A, et al. Exercise-induced changes in expression and activity of proteins involved in insulin signal transduction in skeletal muscle: differential effects on insulin-receptor substrates 1 and 2. Proc Natl Acad Sci U S A. (2000) 97(1):38–43. doi: 10.1073/pnas.97.1.38
45. Houmard JA, Shaw CD, Hickey MS, Tanner CJ. Effect of short-term exercise training on insulin-stimulated PI 3-kinase activity in human skeletal muscle. Am J Physiol (1999) 277(6):E1055–60. doi: 10.1152/ajpendo.1999.277.6.E1055
46. Edwards PA, Muroya H, Gould RG. In vivo demonstration of the circadian thythm of cholesterol biosynthesis in the liver and intestine of the rat. J Lipid Res (1972) 13(3):396–401. doi: 10.1016/S0022-2275(20)39403-7
47. Bremner WF, Sothern RB, Kanabrocki EL, Ryan M, McCormick JB, Dawson S, et al. Relation between circadian patterns in levels of circulating lipoprotein(a), fibrinogen, platelets, and related lipid variables in men. Am Heart J (2000) 139(1 Pt 1):164–73. doi: 10.1016/s0002-8703(00)90324-7
48. Saito Y, Yoshida S, Nakaya N, Hata Y, Goto Y. Comparison between morning and evening doses of simvastatin in hyperlipidemic subjects. a double-blind comparative study. Arterioscler Thromb (1991) 11(4):816–26. doi: 10.1161/01.atv.11.4.816
49. Gotto AM, Gorry GA, Thompson JR, Cole JS, Trost R, Yeshurun D, et al. Relationship between plasma lipid concentrations and coronary artery disease in 496 patients. Circulation. (1977) 56(5):875–83. doi: 10.1161/01.cir.56.5.875
50. Brunner D, Altman S, Loebl K, Schwartz S, Levin S. Serum cholesterol and triglycerides in patients suffering from ischemic heart disease and in healthy subjects. Atherosclerosis. (1977) 28(2):197–204. doi: 10.1016/0021-9150(77)90157-5
51. Chodzko-Zajko WJ, Proctor DN, Fiatarone Singh MA, Minson CT, Nigg CR, Salem GJ, et al. American College of sports medicine position stand. exercise and physical activity for older adults. Med Sci Sports Exerc. (2009) 41(7):1510–30. doi: 10.1249/MSS.0b013e3181a0c95c
52. Mann S, Beedie C, Jimenez A. Differential effects of aerobic exercise, resistance training and combined exercise modalities on cholesterol and the lipid profile: review, synthesis and recommendations. Sports Med (2014) 44(2):211–21. doi: 10.1007/s40279-013-0110-5
53. Lian XQ, Zhao D, Zhu M, Wang ZM, Gao W, Zhao H, et al. The influence of regular walking at different times of day on blood lipids and inflammatory markers in sedentary patients with coronary artery disease. Prev Med (2014) 58:64–9. doi: 10.1016/j.ypmed.2013.10.020
54. Lin X, Zhang X, Guo J, Roberts CK, McKenzie S, Wu WC, et al. Effects of exercise training on cardiorespiratory fitness and biomarkers of cardiometabolic health: A systematic review and meta-analysis of randomized controlled trials. J Am Heart Assoc (2015) 4(7): e002014. doi: 10.1161/jaha.115.002014
55. Seip RL, Angelopoulos TJ, Semenkovich CF. Exercise induces human lipoprotein lipase gene expression in skeletal muscle but not adipose tissue. Am J Physiol. (1995) 268(2 pt 1): E229-236. doi: 10.1152/ajpendo.1995.268.2.E229
56. Arasaradnam MP, Morgan L, Wright J, Gama R. Diurnal variation in lipoprotein lipase activity. Ann Clin Biochem (2002) 39(Pt 2):136–9. doi: 10.1258/0004563021901883
57. Kodama S, Tanaka S, Saito K, Shu M, Sone Y, Onitake F, et al. Effect of aerobic exercise training on serum levels of high-density lipoprotein cholesterol: a meta-analysis. Arch Intern Med (2007) 167(10):999–1008. doi: 10.1001/archinte.167.10.999
58. van Kerkhof LW, Van Dycke KC, Jansen EH, Beekhof PK, van Oostrom CT, Ruskovska T, et al. Diurnal variation of hormonal and lipid biomarkers in a molecular epidemiology-like setting. PloS One (2015) 10(8):e0135652. doi: 10.1371/journal.pone.0135652
59. Demacker PN, Schade RW, Jansen RT, Van 't Laar A. Intra-individual variation of serum cholesterol, triglycerides and high density lipoprotein cholesterol in normal humans. Atherosclerosis. (1982) 45(3):259–66. doi: 10.1016/0021-9150(82)90227-1
60. Halkes CJ, Castro Cabezas M, van Wijk JP, Erkelens DW. Gender differences in diurnal triglyceridemia in lean and overweight subjects. Int J Obes Relat Metab Disord (2001) 25(12):1767–74. doi: 10.1038/sj.ijo.0801831
61. Jarrett RJ, Keen H. Diurnal variation of oral glucose tolerance: a possible pointer to the evolution of diabetes mellitus. Br Med J (1969) 2(5653):341–4. doi: 10.1136/bmj.2.5653.341
62. Teo SYM, Kanaley JA, Guelfi KJ, Marston KJ, Fairchild TJ. The effect of exercise timing on glycemic control: A randomized clinical trial. Med Sci Sports Exerc. (2020) 52(2):323–34. doi: 10.1249/mss.0000000000002139
63. Scheer FA, Hu K, Evoniuk H, Kelly EE, Malhotra A, Hilton MF, et al. Impact of the human circadian system, exercise, and their interaction on cardiovascular function. Proc Natl Acad Sci U S A. (2010) 107(47):20541–6. doi: 10.1073/pnas.1006749107
64. Wojtaszewski JF, Nielsen P, Hansen BF, Richter EA, Kiens B. Isoform-specific and exercise intensity-dependent activation of 5'-AMP-activated protein kinase in human skeletal muscle. J Physiol (2000) 528 Pt 1(Pt 1):221–6. doi: 10.1111/j.1469-7793.2000.t01-1-00221.x
65. Treebak JT, Birk JB, Rose AJ, Kiens B, Richter EA, Wojtaszewski JF. AS160 phosphorylation is associated with activation of alpha2beta2gamma1- but not alpha2beta2gamma3-AMPK trimeric complex in skeletal muscle during exercise in humans. Am J Physiol Endocrinol Metab (2007) 292(3):E715–22. doi: 10.1152/ajpendo.00380.2006
Keywords: chrono-exercise, exercise timing, endurance exercise, glucose levels, triglycerides
Citation: Kim H-K, Furuhashi S, Takahashi M, Chijiki H, Nanba T, Inami T, Radak Z, Sakamoto S and Shibata S (2022) Late-afternoon endurance exercise is more effective than morning endurance exercise at improving 24-h glucose and blood lipid levels. Front. Endocrinol. 13:957239. doi: 10.3389/fendo.2022.957239
Received: 30 May 2022; Accepted: 04 July 2022;
Published: 19 July 2022.
Edited by:
Hiroshi Yoshida, Jikei University Kashiwa Hospital, JapanReviewed by:
Yoshitaka Hashimoto, Kyoto Prefectural University of Medicine, JapanStephen C Kolwicz Jr., Ursinus College, United States
Shinji Koba, Showa University, Japan
Copyright © 2022 Kim, Furuhashi, Takahashi, Chijiki, Nanba, Inami, Radak, Sakamoto and Shibata. This is an open-access article distributed under the terms of the Creative Commons Attribution License (CC BY). The use, distribution or reproduction in other forums is permitted, provided the original author(s) and the copyright owner(s) are credited and that the original publication in this journal is cited, in accordance with accepted academic practice. No use, distribution or reproduction is permitted which does not comply with these terms.
*Correspondence: Hyeon-Ki Kim, gusrlgusrl82@gmail.com
†These authors have contributed equally to this work