- State Key Laboratory of Oral Diseases, National Clinical Research Center for Oral Diseases, West China Hospital of Stomatology, Sichuan University, Chengdu, China
Diabetes mellitus is a common systematic chronic disease amongst dental patients. The elevated glucose microenvironment can prolong the healing of tooth extraction sockets. Therefore, the promotion of healing up tooth extraction sockets is of great clinical importance to the patients with diabetes mellitus. The current evidence indicates the mechanism of the recovery period of extraction sockets in hyperglycaemia conditions from physiological, inflammation, immune, endocrine and neural aspects. New advancements have been made in varied curative approaches and drugs in the management of wound healing of tooth extraction sockets in diabetes. However, most of the interventions are still in the stage of animal experiments, and whether it can be put into clinical application still needs further explorations. Specifically, our work showed topical administration of plasma-rich growth factor, advanced platelet-rich fibrin, leukocyte- and platelet-rich fibrin and hyaluronic acid as well as maxillary immediate complete denture is regarded as a promising approach for clinical management of diabetic patients requiring extractions. Overall, recent studies present a blueprint for new advances in novel and effective approaches for this worldwide health ailment and tooth extraction sockets healing.
Introduction
Diabetes mellitus (DM) is recognized as an enormous menace to the general population globally, which affects 463 million adults (1). It is a systematic metabolic disorder characterized by defective insulin secretion and impaired insulin, resulting in microvascular complications and hyperglcemia (2). Diabetes is divided into diabetes mellitus type 1 (T1DM) and diabetes mellitus type 2 (T2DM), with T2DM making up 90% of cases worldwide and thus more relevant research (3). Patients with DM are associated with a high risk of hyperlipidemia, obesity, and healing disorders. Considering that diabetes ranks 3th in the most prevalent chronic disease in the oral field (4), number of diabetic patients experiencing oral manifestations exceeded 90% (5). Diabetic patients have a prevalence of missing teeth, prolonged wound healing, xerostomia, caries, burning mouth disorder, lichen planus, and even bacterial osteomyelitis of the jaw, which could increase the treatment difficulty and compromise the treatment outcome of various oral diseases (6–13). A population-based cohort study proved that diabetic patients have a higher risk of tooth extraction due to periodontal disease than non-diabetic patients in South Korea (p <.01) (6). The origin of the medication-related osteonecrosis of the jaws tends to be tooth extraction in elderly patients with uncontrolled diabetes (P < 0.0125) (14). Case reports proved that bacteraemia and fungal infection caused by diabetes-related tooth extraction seem to be a triggering factor for osteomyelitis and mucormycosis, respectively (15, 16).Therefore, elucidating the mechanism and investigating the approaches to promoting the healing of tooth extraction sockets is of great clinical importance, especially for the patients with DM. In this review, we systematically searched and appraised the current literature to summarize and discuss the mechanisms and managements of delayed extraction sockets in patients with diabetes.
Mechanistic insight into delayed tooth extraction socket healing among diabetic patients
The histological healing process in extraction-sockets is a four-stage process involving the blood clot phase, the inflammation phase of granulation tissue formation, the proliferation phase with woven bone formation and the modeling and remodeling phase, as shown below (Figure 1) (17, 18). Osteogenic tissue proliferates and bone maturity following trabecular bone formation occurs between 4 and 8 weeks after extraction (19, 20).
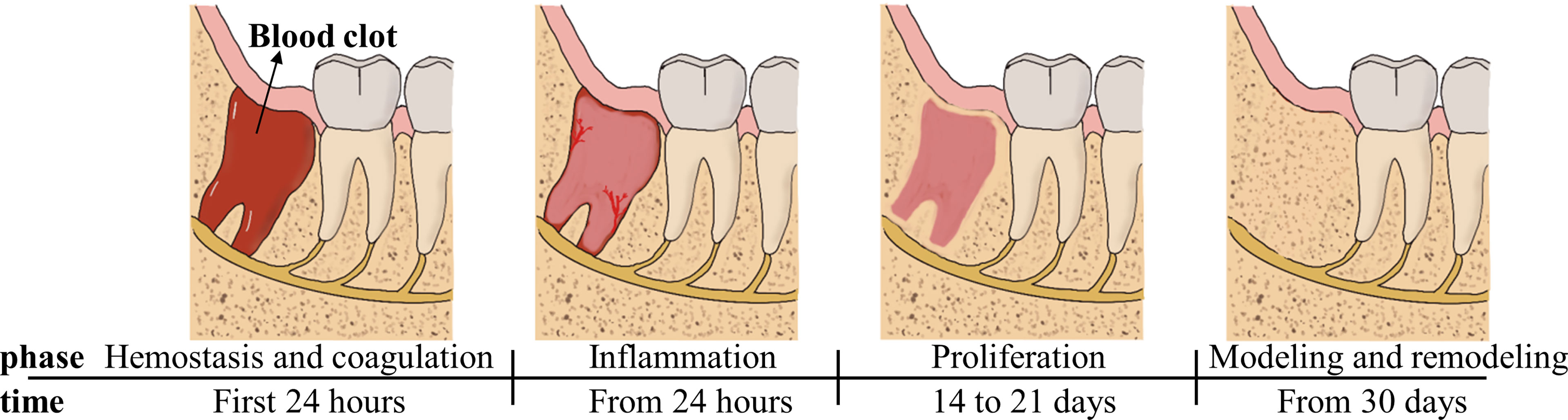
Figure 1 Main processes of wound healing occurring in the socket after tooth extraction depicted as four time-related phases.
Delayed tooth extraction socket (TES) healing were often found in patients with poorly controlled or untreated DM (21). Tooth extraction healing is slower for diabetic than the group without diabetes, particularly on day 7 post-operatively (22). However, not all studies have reached the conclusion that diabetics have increased delayed healing (23). In the study by Goss et al. there was no statistically significant difference in healing rate after tooth extraction in either T1DM or T2DM compared to non-diabetic patients, a result that supports the tendency for diabetic patients to recover well after tooth extraction when they are well controlled (24, 25). For instance it has been shown that the duration of bone healing is similar in diabetic and normal individuals (24). Still, due to the specificity of diabetes and the possibility of delayed-wound-healing risk after tooth extraction, it is of great value to understand the mechanisms involved and the potential treatments.
In recent years, the field of wound research has been broadened by an in-depth understanding of diabetes and its various aspects of physiological, inflammatory, immunological, endocrine, neurological mechanisms and microRNAs (miRNAs) associated with the healing of extracted tooth sockets (26). Long-standing wound healing in patients with diabetes is generally attributed to the abnormal expression of all the cells involved as well as the dysregulation of the expression of growth factors, cytokines required to coordinate the normal healing process as suggested by these research. Factors accounting for the healing process of diabetic extraction sockets is presented in Figure 2.
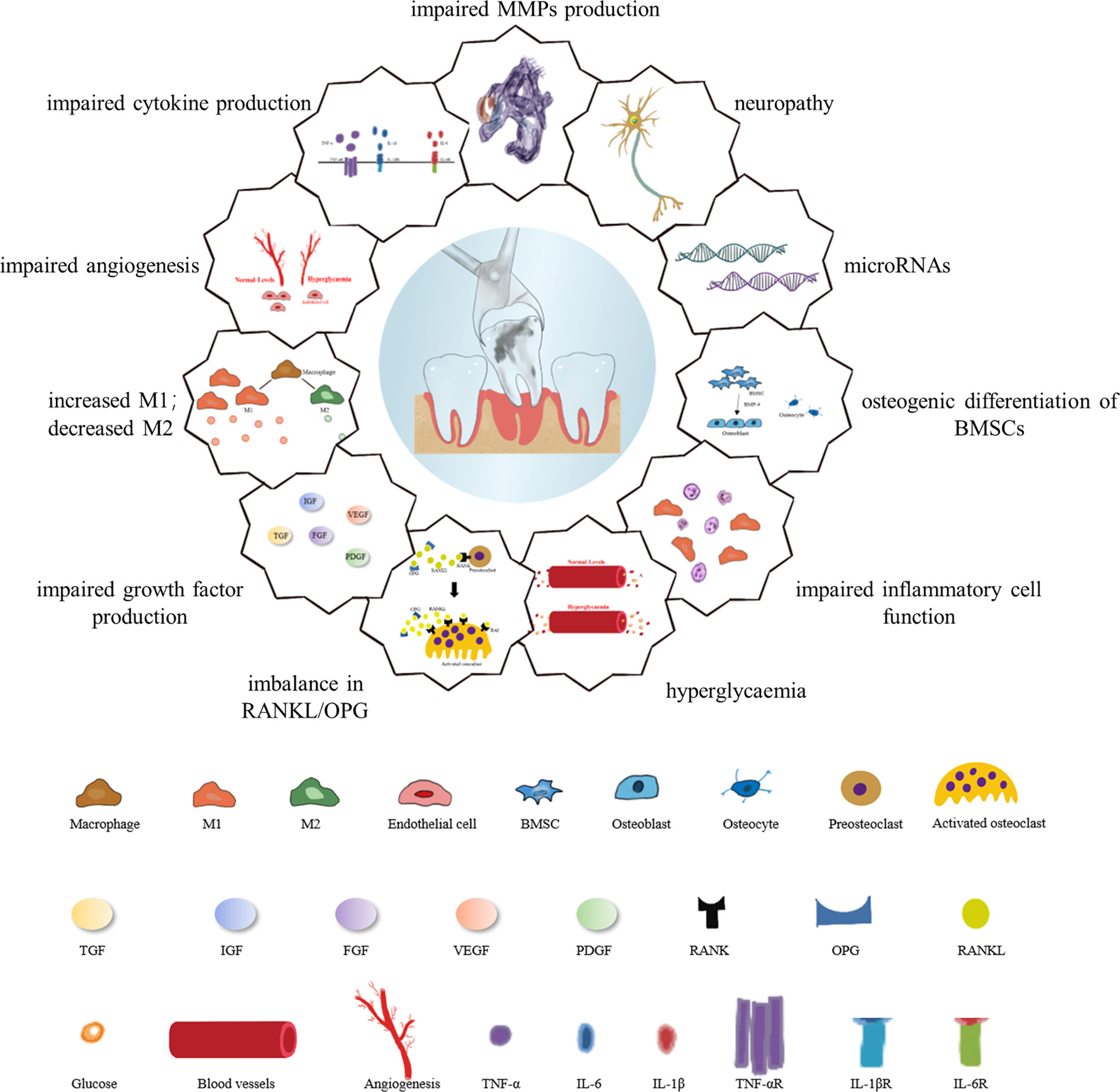
Figure 2 Factors responsible for the healing process of diabetic extraction sockets. Diabetes inhibits mitotic growth factor expression through epigenetic mechanisms; difficulty in wound healing after tooth extraction is associated with diminished osteogenic differentiation of mesenchymal stem cells, activation of matrix metalloproteinase-9, persistent imbalance of RANKL/OPG ratio, and reduced expression of neuropeptides. Hyperglycemia affects hormone receptor conversion as well as the formation of new blood vessels, and impaired angiogenesis not only hinders bone formation but also affects the rate of wound healing. Diabetic wounds are characterized by chronic inflammation due to high levels of reactive oxygen species, dysregulated M1/M2 macrophage polarization, and pro-inflammatory chemokines. High glucose levels have a negative impact on macrophage function, mainly in the form of dysregulated levels of cytokine secretion such as TNF-α, IL-6 and IL-1β, in addition to the inability of neutrophils to function in the inflammatory response phases of wound healing, migration, chemotaxis and adhesion. MicroRNAs also influence the different phases of diabetic wound healing.
Physiological mechanism
Healing of extraction sockets is a complex process involving the reconstruction of damaged soft and hard tissues. It embodies the proliferation and differentiation of osteocytes, as well as the synthesis and mineralization of extracellular matrix, resulting in bone formation and remodelling. These activities are regulated by various cytokines, comprising the transforming growth factor β (TGFΒ), the vascular endothelial growth factor (VEGF), the insulin-like growth factor (IGF) and the bone morphogenetic protein (BMP) (27). The increased recovery rate was observed through the local application of growth factors; however, the deficiency of growth factors in hyperglycaemia conditions caused a low level of wound healing in animal or clinical studies (28, 29). Decreased expression levels of these TGFΒ1-3, TGFβRII and TGFβRIII genes may be linked to impaired oral mucosa healing in diabetic mice (30). Diabetes-induced detrimental effects on TES healing under the palatal plate may be mitigated due to the rise in salivary VEGF elicited by T2DM in clinical trials (31). However, the presence of VEGF would be insufficient to produce new bone under hyperglycemic conditions. The bone formation is disrupted due to crosslinking of advanced glycation end products (AGEs) unfavorably, in spite of induction of VEGF-C and VEGF receptor-3 positivity in Akita mouse osteoblasts after extraction (19). IGF-1 could foster the osteogenic differentiation of apical papillae stem cells, which is likely to be induced by c-Jun N-terminal kinase and p38 mitogen-activated protein kinase signaling pathways (32). In addition, the concentration alterations in tissue growth factors, such as IGF-1, may be strongly correlated with wound healing of the epithelium in rats (33). Noticeably, non-enzymatic glycosylation of collagen in hyperglycaemic rats was found to impair the collagen metabolism, thus producing highly soluble and easily degradable collagen. In this case, the mechanical properties of the formed bone were weakened, and led to the delayed healing and increased alveolar destruction (34).
Interestingly, the gene expression profile of T2DM was distinguishable from control subjects (35). According to Liang et al. (36), 11 differentially expressed genes were substantially higher in the non-diabetic control group than in the T2DM group, and among these genes, BMP-4, which is significantly under-expressed in T2DM blood, is the most important gene regulating bone marrow mesenchymal stromal cells (MSCs) osteogenic differentiation based on gene ontology annotation and random forest analysis. Among BMP family, BMP-4 was shown bone-forming potential in rat tooth sockets (37). BMP-4, associated with bone morphogenetic protein receptor 1, enhances the osteogenic differentiation of stem cells via activation of Smad signaling (38). It is noteworthy that recombinant BMP4/7 has a higher potential to induce MSC differentiation than BMP4 (39). With high concentrations of glucose (25mmol/l), the levels of BMP-4, bone sialoprotein and osteopontin expression, expression of Shh and alkaline phosphatase (ALP) were greatly reduced compared with low glucose (5.5mmol/l) (40).
The nature of diabetic wounds that are resistant to healing is also connected to the involvement of matrix metalloproteinase (MMP). The higher activity of MMP-2 and MMP-9 in diabetic mice wounds is similar to that of hard-to-heal wounds caused by ulcers or burns (41), and subsequently studies have identified MMP-8 and MMP-9 from diabetic wounds and demonstrated that MMP-8 inhibits apoptosis and favors wound healing, while conversely MMP-9 promotes apoptosis and renders wounds unhealable in mice (42). Infection of wounds increases MMP-9 activity, facilitates macrophage infiltration and diminishes angiogenesis in animal and clinical experiments (43). Selective inhibition of MMP-9 together with locally applied active recombinant MMP-8 supports wound healing in diabetes in mice (44). Hyperglycaemia (25mmol/L) can affect the regulation of cellular Na+/K+ adenosine triphosphate enzyme activity, increase protein kinase C activity, influence the conversion of hormone receptors and the formation of new blood vessels in vitro (45, 46). This is corroborated by the fact that lower ATP concentrations in plasma are coupled with lower blood flow in T2DM patients compared to healthy subjects (47). High blood glucose (>13.9mmol/L) can also contribute to the production of AGEs as well as receptor for AGEs (RAGE) under metabolic disorders and inflammatory conditions in diabetic rats (48). In in vitro experiments, increased AGE levels elevate extracellular MMP inducer content and stimulate the secretion of MMP, accompanied by collagen degradation and a decrease in bone strength (49). Large amount of aldoses of AGEs has been found to cause dysfunction of the endothelial cells and extracellular matrix of the microvascular wall by covalently bounding to active amino groups, and damage blood vessels by up-grading oxidative stress and inducing monocytes to produce platelet-derived growth factors (50). Thus the blood vessels became pathologically permeable and inelastic, and block the blood flow (51).
Receptor activator of nuclear factor kappa B (RANK) and its ligand (RANKL), as well as the deceptive receptor osteoprotegerin (OPG), are the three main proteins of the RANKL/RANK/OPG signaling pathway encoded by TNFRSF11B (52). RANKL-RANK interaction increases osteoclast production, whereas OPG inhibits their binding. This pathway is famed for its roles in bone remodeling and may have an impact on the pathogenesis of T2DM women (53). For poorly controlled T2DM patients, a continual imbalance in RANKL/OPG ratio may be produced in periodontal tissues (54).
Angiogenesis is described as new vessel formation out of pre-existing ones and exerts its effects on wound healing (55). The functional vascular supply is responsible for proper ossification of newly deposited bone (56). Impaired angiogenesis in patients with hyperglycaemia affects the rate of wound healing, in addition to impeding bone formation (57). Over and above that, hypoxia-inducible factor 1α may stimulate angiogenesis and enhance new bone formation as a transcription factor in vitro (58). During bone repair, its expression is upregulated due to hypoxia, but its function of mediating angiogenesis and osteogenesis is suppressed due to high glucose conditions in diabetic mice (59).
Inflammation and immune mechanism
Alterations in inflammation levels and reductions in new connective tissue and bone formation played an essential role in diabetic oral wound healing. Diabetes suppresses mitogenic growth factor expression and increases pro-inflammatory cytokine expression mediated by epigenetic mechanisms (60). Chronic diabetic wounds are chronically inflamed due to a great deal of reactive oxygen species (ROS), dysregulated M1 macrophage polarization and pro-inflammatory chemokines in mice (61). TNF-α is acknowledged to stimulate inflammatory response by increasing the number of blood vessels and vessel density and regulating M1/M2 macrophage polarization in in vitro and animal studies (62–64). However, it is stated that elevated TNF-α and promoting inflammatory cytokines in a hyperglycemic state (>16.7mmol/L) instead spur bone resorption on the one hand and restrain bone formation on the other hand in rats (65). Runx2, important for the differentiation of osteoblasts and maturation of chondrocytes, is inhibited by pro-inflammatory cytokines in vitro (66, 67). A reduction of Runx2 diminished MSC differentiation and the production of osteoblast cells (68). Besides, granulocytes were unable to function during the inflammatory response stage of the wound healing, migration, chemotaxis and the adhesion of neutrophil in T1DM patients (69). The impaired neutrophil function, however, was found not related to the increased risk of short-term postoperative complications in T2DM (70). No correlation was found between extended wound epithelialization and reduced neutrophil function at three weeks postoperatively (70).
Uncontrolled DM patients are regarded as immunosuppressed, considering the negative impact of hyperglycaemia on the immune system. It has been confirmed that high blood sugar (25mmol/L) causes damage to the cellular immune response, inflammatory cytokines and microcirculation during the healing process (71, 72). The mechanism of impaired immune system is mainly related to immune cells, such as macrophages and granulocytes. High glucose levels (>16.7mmol/L) have a negative impact on the function of macrophages, mainly in the form of dysregulated secretion levels of cytokines such as TNF-α, IL-6 and IL-10, and decreased metabolic activity in T1DM mice (73); in combination with enhanced pro-inflammatory macrophages was found in T1DM mice in in vitro experiments, resulting in a higher risk of infection (74). Defects in phagocytosis may interfere with the inflammatory response and microbial uptake, causing accumulated debris in the wound and preventing the formation of granulation tissues both in vivo and in vitro (75). Notably, abnormal inflammatory responses coordinated by M1 or M2 macrophages are also usually associated with delayed healing. In a vitro co-culture model, M1 pro-inflammatory macrophages were found to act primarily by inhibiting capability of MSCs as well as the angiogenic ability of endothelial cells, while the opposite role was found for M2 anti-inflammatory macrophages (76). High glucose (25mmol/L) could drive M1 macrophage polarization via overproducing ROS under inflammatory stimulation in T1DM rats (77). The polarization of elevated M1 and reduced M2 macrophage may take responsibility for slowed TES healing in subjects with T2DM through aberrant expression of tumor necrosis factor-α (TNF-α) and peroxisome proliferator-activated receptor-γ (21).
Endocrine mechanism and neural mechanism
In diabetic patients, their hyperglycemic condition affects a wide range of cell functions, for example, the regulation of bone-forming differentiation. Osteoblast proliferation and differentiation can be inhibited by hyperglycemia (25mmol/L) through caspase-1-mediated pyroptosis in vivo and in vitro (78). It may cause osteoblast bone formation disorders and result in pathological changes, such as diminished bone formation and reduced alveolar bone height of tooth extraction wounds. It has been suggested that the increased expression of glucose transporter 1 might be part of the reasons for the inadequate mineralization of osteoblasts during hyperglycaemia in vitro (79). Excessive protein-linkedΒ-acetylglucosamine glycosylation (-GlcNAcylation) induced by O-GlcNAc transferasewith high glucose (46,60 mmol/L), glucosamine (2.5-5mmol/L) or N-acetylglucosamine (5mmol/L) leads to a reduction in RUNX2 gene expression and thus has an inhibitory effect on osteogenic differentiation in vitro (80). Furthermore, the weakening of MSC osteogenic differentiation might be an essential factor responsible for TES healing for T2DM pig models (81). Growth differentiation factor 11 was related to the inhibited osteogenic differentiation of MSCs in TES in patients with T2DM (82).
Sensory nerves contribute to inflammation and immune response, in particular, possess trophic-facilitating wound healing generally (83). Neuropeptides are neuromodulators involved in a variety of processes, diabetic wound healing released by sensory nerves included (84). Insufficiency of neurogenic mediators such as substance P (SP), secreted from sensory neurons, may participate in wound epithelization in mutant diabetic mice with delayed healing (85). Moreover, SP stimulates bone formation in osteoblasts by neurokinin-1 receptors at advanced stages of bone formation in rats (86). Diabetes can lead to autonomic and small sensory nerve fiber neuropathy and dysregulation of inflammation, as evidenced by reduced expression of neuropeptide and imbalance in pro- and anti-inflammatory cytokine responses (87). It has been found that the exogenous SP improved wound repair kinetics and suggested that the chronic trauma in DM patients may be attributable to downgraded levels of neuropeptide nutrition (85).
The function of microRNAs in the healing of diabetic wound
MiRNAs, regulating expression of mRNA, are a kind of short non-coding single-stranded RNA molecules (88). MiRNAs influence several physiological and pathological processes, the most notable being metabolism, proliferation, differentiation and apoptosis. Therefore, they are being investigated as vital markers at different stages of the wound healing process (89).
There are several miRNAs involved in the regulation of inflammatory phase of wound healing in a hyperglycemic environment. For example, inflammation in unhealed wounds of patients with T2DM affects plasma miRNA concentrations, whereas miR-191 affects angiogenesis through its target zonula occludens-1 in order to slow down the tissue reparative process (90). MiR-497, with its down-regulation activity for pro-inflammatory cytokines, to such factors as TNF-α, IL-1β, IL-6, is considered as a promising curative factor for diabetic wound healing in mice (91). MiR-129-2-3p at wound sites in type 2 diabetic mice may expedite wound healing by mediating the function of neutrophils (92).
Other miRNAs participating in angiogenesis and remodeling stages consist of miR-15b, miR-20b, miR-21, etc. Both 15b and 200b can inflict impaired angiogenesis by repressing the expression of VEGF in diabetic mice (93). In diabetic mice, knockdown of miR-20b-5p was found to significantly potentiate wound repair and facilitate wound angiogenesis by regulating the Wnt9b/β-catenin signaling pathway (94). It has been shown that miR-21 expression is engaged in early healing of the incisor extraction sockets in mice (95). Strauss et al. demonstrated that miR-21 knockout mice had approximately 15% reduced bone formation in the mesial and coronal portions of the extraction socket compared to wild-type controls (95). MiR-27b was revealed to prompt wound healing by rescuing damaged angiogenic cells in T2DM mice (96). For pigs and mice, anti-angiogenic MiR-92a, its inhibitor, possesses the ability to accelerate wound healing (97). In in vitro experiments, upregulated MiR-140-3p exosomes promoted the differentiation of MSCs into osteoblasts (98).
Nevertheless, the study of miRNAs and diabetic TES healing paves the way for miRNA-based dental regeneration strategies.
Potential interventions in the management of extraction sockets healing in patients with diabetes
Ideal interventions used in oral surgery should facilitate the repair of extraction sockets, and reduce the postoperative infection, pain and complications. Plenty of investigations have explored pathways to acceleration of TESs healing under high-glucose conditions based on molecular regulators of their activity, either directly or indirectly. It is encouraging to see that a considerable number of results have entered clinical trials, as shown in the table below (Table 1). Directly interacting targets include growth factors, BMPs, parathyroid Hormone (PTH), and stem cells. A variety of drugs may act indirectly on molecular targets by up- or down-regulating the expression of growth factors, MMP, collagen synthesis/degradation, pro- and anti-inflammatory cytokines, and pro-angiogenic factors. Drugs or natural products or formations of molecular targets that are involved on a direct or indirect basis in a proposed treatment will be described below.
Molecular targets
Local delivery of growth factors, as for instance by the delivery of platelet-derived growth factor (99), IGF (79), fibroblastic growth factor (105), has been verified to favor wound healing in poorly controlled diabetes. Systematic reviews and meta-analyses have found the efficacy of platelet derivatives to improve the wound healing and bone density, thereby stimulating the soft tissues and bone regeneration (106, 107). Platelet-rich plasma is dependent on platelets to exert great influence on healing. In a split-mouth study recruiting 34 patients with T1DM, the application of plasma-rich growth factor after extraction yielded remarkably diminished residual TES volumes and improved Healing Indices by accelerating the socket closure (epithelialization) and tissue maturation in diabetic patients (99). Another animal research study assessed the effect of topical application of autologous platelet-rich plasma on extraction wound and found that it prevents the medication-related osteonecrosis of the jaws (108). Activated platelet lysates induce OPG expression and stimulate soft tissue healing and osteoblast differentiation in rats (109). IGF-I was found to increase the volume of neoformed bone after tooth extraction in diabetic rats by regulating glucose transporter 1 expression, as well as increases osteoblast mineralization during extraction wound healing (79, 110). For those patients with insulin resistance, IGF-I treatment can be considered, but the effectiveness and safety of IGF-I for long-term use in the management of diabetes and complications involved need further studies (111). Hence, locally delivered growth factors to promote healing may be a potential therapy for the treatment of diabetic osteopathy.
Local hemostatics are beneficial in reducing underlying postsurgical bleeding and to pace healing (112). Leukocyte- and platelet-rich fibrin (L-PRF) enhanced bone density and reduced inflammation, used as a graft to fill the TES and stabilize the blood clot in patients (100). L-PRF alone or in combination with hyaluronic acid (HA) was effective in improving mucosal healing and preventing alveolar osteitis and infection following mandibular third molars extraction (101). However, it has also been the finding that L-PRF adds to the growth factors concentration in the TES but has no positive outcome on bone healing (113). Additionally, the finding demonstrated the potential of advanced platelet-rich fibrin (A-PRF) as a therapeutic biomaterial for bone regeneration after surgical extractions of third molars in clinical trials, but further studies with larger sample sizes and more systematic and reliable evaluation tools are necessary (102).
Treating the diabetic sockets with BMP may be useful to TES healing. Controlled local release of recombinant human BMP-2 dramatically promoted bone production in diabetic mice to near normality and potentiates bone rejuvenation in normal mice (114). BMP-6 can facilitate the osteoblast differentiation from MSCs and the chondrocyte maturation by signalling through type I and type II BMP receptors (115). The extra-alveolar tissue of diabetic rats showed a subcellular periosteal reaction by day 3, and a large amount of cartilage had been formed by day 7 following the application of BMP-6 (116). It has been reported that down-regulated BMP-6 in certain tissues such as myofibroblast progenitor cells in diabetic patients thereby inhibited the cartilage formation delaying the healing (117). Therefore, the topical application of BMP-6 is promising to reverse the healing inhibition of diabetes. Moreover, the level of expressed BMP-4, bone sialoprotein and osteopontin, ALP activity and the increased number of matrix mineralized nodules in MSCs correlated with the Lenti – Shh activated Shh signaling pathway; in vivo experiments revealed that Lenti – Shh invoked additional osteogenesis (40). The intraoral injection of the inhibitor of growth differentiation factor 11 has been found to promote the bone healing in the post-extraction site as well as the osteogenic differentiation of porcine MSCs (82). Furthermore, activating macrophages by mannose receptor clustering and enhancing M2 macrophage polarization were found to contribute to accelerated wound healing, increase the collagen expression and reduce the infection in hyperglycemic conditions in mice (118). Sustained Interleukin-4 released markedly enhanced osteogenic and angiogenic gene expression with improved socket healing in T2DM mice by inducing macrophage transformation towards M2 polarization (21).
PTH is an important hormone to regulate the bone metabolism. PTH has been shown to reduce the alveolar bone loss in the intermittent and systemic administrations by decreasing the RANKL/OPG ratio in diabetic rats (119). However, some studies found that PTH did not improve the post-extraction wound healing or stimulate the osseointegration in hyperglycemic rats, regardless of administration of PTH (intermittent versus continuous) (120). This can be explained by the overall inhibitory effect of high levels of AGEs and collagen cross-linking on bone formation under diabetic metabolism (121). The anabolic role of PTH in the repair after DM extraction needs to be confirmed by further studies.
Synthetic drugs
The acceleration of TES recovery with insulin or metformin has already been reported in previous research (34, 122). Insulin, a first-line drug in the clinical therapy of DM, can directly hasten TES healing by raising TGFβ-3 expression and lowering IGF-1R expression in diabetic rabbits (27). Moreover, the consequences of high blood glucose and metformin on peri-implant healing should be attached importance to. Metformin is the most commonly employed oral hypoglycemic agents; its benefit attributed to its preferential influence on endothelial cells, as well as its antioxidant and anti-inflammatory properties (123). Metformin not only remarkably reduced both intracellular ROS and apoptosis, but also increased osteoblast differentiation at varied glucose levels (0.99, 1.98, 3.96, and 7.92 g/L), which may be related to the promotion of Runx2 and IGF-1 expression in vitro (122). Noticeably, osteogenic differentiation potential of MSCs could be enhanced by metformin in T2DM patients through the BMP-4/Smad/Runx2 signaling pathway (36). Goto-Kakizaki rats with T2DM showed improved blood glucose and bone volume percentage, the number of trabecular, as well as bone density after using metformin (124).
Natural product-based treatment
Natural ingredients, namely obtained from natural sources, often stand for the topic of further research and have been exploited as an alternative therapy like spirulina, chitosan, flavonoids and many more. Chitosan is a deacetylated polysaccharide from chitin, which can accelerate new bone formation and enhance neovascularization in vivo (125). Besides chitosan, spirulina, a microalgae containing kaempferol, also has antioxidant and anti-inflammatory effects (126). Due to the fact that the addition of 12% spirulina and 20% chitosan to the dental socket of mice yielded an alkaline pH that was suited to ALP activity, the bone remodeling process can be completed by promoting an increase in osteoblast cells and a decrease in osteoclasts (127). Ellagic acid is a natural component that effectively prevents bone loss induced by tooth removal in diabetic rats; diabetic rats treated with ellagic acid express a stronger immunohistochemical response to fibroblastic growth factor-2 and ALP than non-treated diabetic rats (105).
Flavonoids are known as a natural component that can inhibit inflammation whilst speeding up wound healing. Morin, as a pleiotropic dietary flavonoid, may prevent bone histomorphological alterations in diabetic rats through a potential mechanism of the insulin/IGF-1 pathway (128). Extract of okra fruit containing flavonoid, possesses strong antioxidant and anti-inflammatory properties. Okra fruit extract (250 mg/kg) increased TGFΒ1 levels in post-extraction wounds of diabetic Wistar rats (129). Treatment of hyperglycemic diabetic rats with a new chemically modified curcumin 2.24 contributed to the alleviation of local and systemic inflammation and reduced bone loss, plus inhibition of collagenolytic MMPs as well as pro-inflammatory cytokines (130). A modified curcumin was found to accelerate skin wound healing in hyperglycemic rats induced by streptozotocin (131). Probiotics serve as a potential strategy to augment insulin sensitivity and minimize autoimmune responses by modifying intestinal flora and reducing inflammatory responses and oxidative stress (132). It is showed that exogenous SP favourably promotes wound healing kinetics in Mutant diabetic mice (85). Further, new bone formation was enhanced histomorphometrically when using deproteinized bovine bone mineral containing 10% collagen with hypoxia-inducible factor 1α in dogs (133). These materials provide a clue for latent auxiliary therapies in the management of post-extraction wound in patients with DM.
Other approaches
HA could be a reliable approach to wound closure. One study investigated the underlying role of HA, a component of extracellular matrix, in promoting TES healing in diabetic patients. In a randomized controlled split-mouth study including 30 patients with poorly controlled T2DM who required tooth extraction, 0.8% HA placed in post-extraction socket improved the wound healing, in particular on the first days after applying (103). In addition, sodium hyaluronate (HY) is the product of the neutralization of the carboxyl groups of HA, which has been proved to enhance the healing process in the extraction sockets of rats (134). Diabetic rats gained greater percentage of newly formed trabeculae in the post-extraction wound treated with HY or carbon nanotubes functionalized with HY (135).
Low-level laser therapy offered a good treatment option for TES healing in T2DM patients (136). Rat sockets irradiated by 808 nm or 660 nm laser had less inflammatory cell infiltration and more angiogenesis than unirradiated sockets apparently (137). Low-level laser therapy at 808 nm was able to considerably improve osteoid regeneration, while no substantial difference was observed in the amount of bone formation with 660 nm (137). Park et al. agreed that 980-nm laser irradiation in diabetic and normal rats for 1 minute per day contributed to early TES healing and further calcification with a high expression of Runx2 and collagen type I mRNA (138). Maxillary immediate complete denture has been considered as a feasible treatment for TES healing in T2DM patients with lower reduction of socket opening diameters, as it offers an opportunity to train chewing ability, and thus maintaining good nutrition in post-extraction period (104).
To date, the clinically safe and effective therapy to facilitate the healing of TESs in patients with DM is still lacking. Many clinical trials and animal experiments have explored the interventions for facilitating the healing of extraction sockets and improving clinical symptoms (24, 105). However, the efficacy of these methods is not satisfactory because of the complicated nature of diabetes, the fragility of the oral environment and short-term assessment. Well-designed large-scale multi-centre clinical trials are still required for the investigation of interventional wound healing diabetics.
Conclusions
This review investigated the mechanism and treatment of the extraction sockets healing process in diabetic patients. Approaches involving the growth factor, growth factors, BMP, PTH, stem cells, synthetic drugs, natural product, HA, Low-level laser therapy have been evaluated with limited achievement. Various clinical trials have been explored to enhance the healing process of post-extraction sockets under hyperglycemic conditions, including plasma-rich growth factor, L-PRF, A-PRF, HA, maxillary immediate complete denture. However, most of these interventions are mostly still in the stage of animal experiments, and further studies are still needed before they can be applied in clinical practices. In the light of these facts, they present a hope that new approaches development will further supervene for this worldwide health ailment and healing of tooth extraction sockets.
Author contributions
SY: researched data, wrote, reviewed, and edited the manuscript. YL: commentary. YW, ZW and CL: revised the manuscript. DS: critical review, funding acquisition. All authors contributed to the article and approved the submitted version.
Funding
This study was supported by the National Natural Science Foundation (82170970).
Conflict of interest
The authors declare that the research was conducted in the absence of any commercial or financial relationships that could be construed as a potential conflict of interest.
Publisher’s note
All claims expressed in this article are solely those of the authors and do not necessarily represent those of their affiliated organizations, or those of the publisher, the editors and the reviewers. Any product that may be evaluated in this article, or claim that may be made by its manufacturer, is not guaranteed or endorsed by the publisher.
References
1. Saeedi P, Petersohn I, Salpea P, Malanda B, Karuranga S, Unwin N, et al. Global and Regional Diabetes Prevalence Estimates for 2019 and Projections for 2030 and 2045: Results from the International Diabetes Federation Diabetes Atlas, 9(Th) Edition. Diabetes Res Clin Pract (2019) 157:107843. doi: 10.1016/j.diabres.2019.107843
2. Meldgaard T, Olesen SS, Farmer AD, Krogh K, Wendel AA, Brock B, et al. Diabetic Enteropathy: From Molecule to Mechanism-Based Treatment. J Diabetes Res (2018) 2018:3827301. doi: 10.1155/2018/3827301
3. Dogruel H, Balci MK. Development of Therapeutic Options on Type 2 Diabetes in Years: Glucagon-Like Peptide-1 Receptor Agonist's Role Intreatment; from the Past to Future. World J Diabetes (2019) 10(8):446–53. doi: 10.4239/wjd.v10.i8.446
4. Segura-Egea J, Martín-González J, Cabanillas-Balsera D, Fouad A, Velasco-Ortega E, López-López J. Association between Diabetes and the Prevalence of Radiolucent Periapical Lesions in Root-Filled Teeth: Systematic Review and Meta-Analysis. Clin Oral investigations (2016) 20(6):1133–41. doi: 10.1007/s00784-016-1805-4
5. Nazir MA, AlGhamdi L, AlKadi M, AlBeajan N, AlRashoudi L, AlHussan M. The Burden of Diabetes, Its Oral Complications and Their Prevention and Management. Open Access Maced J Med Sci (2018) 6(8):1545–53. doi: 10.3889/oamjms.2018.294
6. Yoo JJ, Kim DW, Kim MY, Kim YT, Yoon JH. The Effect of Diabetes on Tooth Loss Caused by Periodontal Disease: A Nationwide Population-Based Cohort Study in South Korea. J Periodontol (2019) 90(6):576–83. doi: 10.1002/jper.18-0480
7. López-Pintor RM, Casañas E, González-Serrano J, Serrano J, Ramírez L, de Arriba L, et al. Xerostomia, Hyposalivation, and Salivary Flow in Diabetes Patients. J Diabetes Res (2016) 2016:4372852. doi: 10.1155/2016/4372852
8. Lund Haheim L, Ronningen KS, Enersen M, Olsen I. The Predictive Role of Tooth Extractions, Oral Infections, and Hs-C-Reactive Protein for Mortality in Individuals with and without Diabetes: A Prospective Cohort Study of a 12 1/2-Year Follow-Up. J Diabetes Res (2017) 2017:9590740. doi: 10.1155/2017/9590740
9. Jimenez M, Hu FB, Marino M, Li Y, Joshipura KJ. Type 2 Diabetes Mellitus and 20 Year Incidence of Periodontitis and Tooth Loss. Diabetes Res Clin Pract (2012) 98(3):494–500. doi: 10.1016/j.diabres.2012.09.039
10. Yap SD, Hamblin PS, Bach L, Ekinci E, Wong R. Sodium Glucose Co-Transporter-2 Inhibitor-Induced Diabetic Ketoacidosis Following Tooth Extraction: Improving Awareness among Dental Practitioners. Aust Dent J (2021) 66(4):444–7. doi: 10.1111/adj.12872
11. Wang Y, Xing L, Yu H, Zhao L. Prevalence of Dental Caries in Children and Adolescents with Type 1 Diabetes: A Systematic Review and Meta-Analysis. BMC Oral Health (2019) 19(1):213. doi: 10.1186/s12903-019-0903-5
12. Genco RJ, Borgnakke WS. Diabetes as a Potential Risk for Periodontitis: Association Studies. Periodontol 2000 (2020) 83(1):40–5. doi: 10.1111/prd.12270
13. Otero Rey EM, Yáñez-Busto A, Rosa Henriques IF, López-López J, Blanco-Carrión A. Lichen Planus and Diabetes Mellitus: Systematic Review and Meta-Analysis. Oral Dis (2019) 25(5):1253–64. doi: 10.1111/odi.12977
14. Wang Q, Liu J, Qi S, Liao X, Liu D, Pan J. Clinical Analysis of Medication Related Osteonecrosis of the Jaws: A Growing Severe Complication in China. J Dental Sci (2018) 13(3):190–7. doi: 10.1016/j.jds.2017.12.003
15. Ribeiro LM, Carvalho S, Guimarães D, Vilela M, Guerra AS. Streptococcus Anginosus and Phalangeal Osteomyelitis: An Unusual Presentation. J Orthop Case Rep (2020) 10(9):19–22. doi: 10.13107/jocr.2020.v10.i09.1888
16. Gholinejad Ghadi N, Seifi Z, Shokohi T, Aghili SR, Nikkhah M, Vahedi Larijani L, et al. Fulminant Mucormycosis of Maxillary Sinuses after Dental Extraction Inpatients with Uncontrolled Diabetic: Two Case Reports. J Mycol Med (2018) 28(2):399–402. doi: 10.1016/j.mycmed.2018.01.003
17. Zhou S, Li G, Zhou T, Zhang S, Xue H, Geng J, et al. The Role of Ift140 in Early Bone Healing of Tooth Extraction Sockets. Oral Dis (2021). doi: 10.1111/odi.13833
18. de Sousa Gomes P, Daugela P, Poskevicius L, Mariano L, Fernandes MH. Molecular and Cellular Aspects of Socket Healing in the Absence and Presence of Graft Materials and Autologous Platelet Concentrates: A Focused Review. J Oral Maxillofac Res (2019) 10(3):e2. doi: 10.5037/jomr.2019.10302
19. Takahashi S, Kikuchi R, Ambe K, Nakagawa T, Takada S, Ohno T, et al. Lymphangiogenesis and Nos Localization in Healing Process after Tooth Extraction in Akita Mouse. Bull Tokyo Dent Coll (2016) 57(3):121–31. doi: 10.2209/tdcpublication.2016-0600
20. Devlin H, Sloan P. Early Bone Healing Events in the Human Extraction Socket. Int J Oral Maxillofac Surg (2002) 31(6):641–5. doi: 10.1054/ijom.2002.0292
21. Shen X, Shen X, Li B, Zhu W, Fu Y, Xu R, et al. Abnormal Macrophage Polarization Impedes the Healing of Diabetes-Associated Tooth Sockets. Bone (2021) 143:115618. doi: 10.1016/j.bone.2020.115618
22. Gadicherla S, Smriti K, Roy S, Pentapati KC, Rajan J, Walia A. Comparison of Extraction Socket Healing in Non-Diabetic, Prediabetic, and Type 2 Diabetic Patients. Clin Cosmet Investig Dent (2020) 12:291–6. doi: 10.2147/ccide.S264196
23. Aronovich S, Skope LW, Kelly JP, Kyriakides TC. The Relationship of Glycemic Control to the Outcomes of Dental Extractions. J Oral Maxillofac Surg (2010) 68(12):2955–61. doi: 10.1016/j.joms.2010.05.006
24. Huang S, Dang H, Huynh W, Sambrook PJ, Goss AN. The Healing of Dental Extraction Sockets in Patients with Type 2 Diabetes on Oral Hypoglycaemics: A Prospective Cohort. Aust Dent J (2013) 58(1):89–93. doi: 10.1111/adj.12029
25. Power DJ, Sambrook PJ, Goss AN. The Healing of Dental Extraction Sockets in Insulin-Dependent Diabetic Patients: A Prospective Controlled Observational Study. Aust Dent J (2019) 64(1):111–6. doi: 10.1111/adj.12669
26. De Angelis P, Manicone PF, Gasparini G, De Filippis I, Liguori MG, De Angelis S, et al. The Effect of Controlled Diabetes and Hyperglycemia on Implant Placement with Simultaneous Horizontal Guided Bone Regeneration: A Clinical Retrospective Analysis. BioMed Res Int (2021) 2021:9931505. doi: 10.1155/2021/9931505
27. Younis WH, Al-Rawi NH, Mohamed MA, Yaseen NY. Molecular Events on Tooth Socket Healing in Diabetic Rabbits. Br J Oral Maxillofac Surg (2013) 51(8):932–6. doi: 10.1016/j.bjoms.2013.08.014
28. Gökşen S, Balabanlı B, Coşkun-Cevher Ş.. Application of Platelet Derived Growth Factor-Bb and Diabetic Wound Healing: The Relationship with Oxidative Events. Free Radic Res (2017) 51(5):498–505. doi: 10.1080/10715762.2017.1327715
29. Pranskunas M, Galindo-Moreno P, Padial-Molina M. Extraction Socket Preservation Using Growth Factors and Stem Cells: A Systematic Review. J Oral Maxillofac Res (2019) 10(3):e7. doi: 10.5037/jomr.2019.10307
30. Yamano S, Kuo WP, Sukotjo C. Downregulated Gene Expression of TgfΒs in Diabetic Oral Wound Healing. J Craniomaxillofac Surg (2013) 41(2):e42–8. doi: 10.1016/j.jcms.2012.08.001
31. Radović K, Brković B, Roganović J, Ilić J, Milić Lemić A, Jovanović B. Salivary Vegf and Post-Extraction Wound Healing in Type 2 Diabetic Immediate Denture Wearers. Acta Odontol Scand (2022) 80(1):9–14. doi: 10.1080/00016357.2021.1930149
32. Ma S, Liu G, Jin L, Pang X, Wang Y, Wang Z, et al. Igf-1/Igf-1r/Hsa-Let-7c Axis Regulates the Committed Differentiation of Stem Cells from Apical Papilla. Sci Rep (2016) 6:36922. doi: 10.1038/srep36922
33. Gong F, Zhao F, Cheng SL, Ding D, Zhang BW, Li XL, et al. Effect of Insulin-Like Growth Factor-1 on Promoting Healing of Skin Ulcers in Diabetic Rats. J Biol Regul Homeost Agents (2019) 33(3):687–94.
34. Devlin H, Garland H, Sloan P. Healing of Tooth Extraction Sockets in Experimental Diabetes Mellitus. J Oral Maxillofac Surg (1996) 54(9):1087–91.
35. Grayson BL, Wang L, Aune TM. Peripheral Blood Gene Expression Profiles in Metabolic Syndrome, Coronary Artery Disease and Type 2 Diabetes. Genes Immun (2011) 12(5):341–51. doi: 10.1038/gene.2011.13
36. Liang C, Sun R, Xu Y, Geng W, Li J. Effect of the Abnormal Expression of Bmp-4 in the Blood of Diabetic Patients on the Osteogenic Differentiation Potential of Alveolar Bmscs and the Rescue Effect of Metformin: A Bioinformatics-Based Study. BioMed Res Int (2020) 2020:7626215. doi: 10.1155/2020/7626215
37. Ferreira CL, Abreu FA, Silva GA, Silveira FF, Barreto LB, Paulino Tde P, et al. TgfΒ 1 and Bmp-4 Carried by Liposomes Enhance the Healing Process in Alveolar Bone. Arch Oral Biol (2013) 58(6):646–56. doi: 10.1016/j.archoralbio.2012.11.013
38. Park ES, Woods DC, Tilly JL. Bone Morphogenetic Protein 4 Promotes Mammalian Oogonial Stem Cell Differentiation Via Smad1/5/8 Signaling. Fertil Steril (2013) 100(5):1468–75. doi: 10.1016/j.fertnstert.2013.07.1978
39. Yuan S, Pan Q, Liu W, Wu B, Han X, Bi Z. Recombinant Bmp 4/7 Fusion Protein Induces Differentiation of Bone Marrow Stem Cells. J Cell Biochem (2011) 112(10):3054–60. doi: 10.1002/jcb.23230
40. Jiang ZL, Jin H, Liu ZS, Liu MY, Cao XF, Jiang YY, et al. Lentiviral Mediated Shh Reverses the Adverse Effects of High Glucose on Osteoblast Function and Promotes Bone Formation Via Sonic Hedgehog Signaling. Mol Med Rep (2019) 20(4):3265–75. doi: 10.3892/mmr.2019.10540
41. Neely AN, Clendening CE, Gardner J, Greenhalgh DG. Gelatinase Activities in Wounds of Healing-Impaired Mice Versus Wounds of Non-Healing-Impaired Mice. J Burn Care Rehabil (2000) 21(5):395–402. doi: 10.1097/00004630-200021050-00001
42. Gooyit M, Peng Z, Wolter WR, Pi H, Ding D, Hesek D, et al. A Chemical Biological Strategy to Facilitate Diabetic Wound Healing. ACS Chem Biol (2014) 9(1):105–10. doi: 10.1021/cb4005468
43. Chang M, Nguyen TT. Strategy for Treatment of Infected Diabetic Foot Ulcers. Acc Chem Res (2021) 54(5):1080–93. doi: 10.1021/acs.accounts.0c00864
44. Gao M, Nguyen TT, Suckow MA, Wolter WR, Gooyit M, Mobashery S, et al. Acceleration of Diabetic Wound Healing Using a Novel Protease-Anti-Protease Combination Therapy. Proc Natl Acad Sci U.S.A. (2015) 112(49):15226–31. doi: 10.1073/pnas.1517847112
45. Müller HK, Kellerer M, Ermel B, Mühlhöfer A, Obermaier-Kusser B, Vogt B, et al. Prevention by Protein Kinase C Inhibitors of Glucose-Induced Insulin-Receptor Tyrosine Kinase Resistance in Rat Fat Cells. Diabetes (1991) 40(11):1440–8. doi: 10.2337/diab.40.11.1440
46. Avagimyan A. The Pathophysiological Basis of Diabetic Cardiomyopathy Development. Curr Probl Cardiol (2022), 101156. doi: 10.1016/j.cpcardiol.2022.101156
47. Groen MB, Knudsen TA, Finsen SH, Pedersen BK, Hellsten Y, Mortensen SP. Reduced Skeletal-Muscle Perfusion and Impaired Atp Release During Hypoxia and Exercise in Individuals with Type 2 Diabetes. Diabetologia (2019) 62(3):485–93. doi: 10.1007/s00125-018-4790-0
48. Chang PC, Chung MC, Wang YP, Chien LY, Lim JC, Liang K, et al. Patterns of Diabetic Periodontal Wound Repair: A Study Using Micro-Computed Tomography and Immunohistochemistry. J Periodontol (2012) 83(5):644–52. doi: 10.1902/jop.2011.110325
49. Li W, Ling W, Teng X, Quan C, Cai S, Hu S. Effect of Advanced Glycation End Products, Extracellular Matrix Metalloproteinase Inducer and Matrix Metalloproteinases on Type-I Collagen Metabolism. BioMed Rep (2016) 4(6):691–3. doi: 10.3892/br.2016.641
50. Yan SD, Schmidt AM, Anderson GM, Zhang J, Brett J, Zou YS, et al. Enhanced Cellular Oxidant Stress by the Interaction of Advanced Glycation End Products with Their Receptors/Binding Proteins. J Biol Chem (1994) 269(13):9889–97.
51. Brownlee M, Cerami A, Vlassara H. Advanced Glycosylation End Products in Tissue and the Biochemical Basis of Diabetic Complications. N Engl J Med (1988) 318(20):1315–21. doi: 10.1056/NEJM198805193182007
52. Yasuda H. Discovery of the Rankl/Rank/Opg System. J Bone Miner Metab (2021) 39(1):2–11. doi: 10.1007/s00774-020-01175-1
53. Duan P, Tu P, Si L, Hu W, Liu M, Liu J, et al. Gene Polymorphisms in the Rankl/Rank/Opg Pathway Are Associated with Type 2 Diabetes Mellitus in Southern Han Chinese Women. Genet Test Mol Biomarkers (2016) 20(6):285–90. doi: 10.1089/gtmb.2015.0306
54. Santos VR, Lima JA, Gonçalves TE, Bastos MF, Figueiredo LC, Shibli JA, et al. Receptor Activator of Nuclear Factor-Kappa B Ligand/Osteoprotegerin Ratio in Sites of Chronic Periodontitis of Subjects with Poorly and Well-Controlled Type 2 Diabetes. J Periodontol (2010) 81(10):1455–65. doi: 10.1902/jop.2010.100125
55. Gómez-Escudero J, Clemente C, García-Weber D, Acín-Pérez R, Millán J, Enríquez JA, et al. Pkm2 Regulates Endothelial Cell Junction Dynamics and Angiogenesis Via Atp Production. Sci Rep (2019) 9(1):15022. doi: 10.1038/s41598-019-50866-x
56. Wang Z, Hui A, Zhao H, Ye X, Zhang C, Wang A, et al. A Novel 3d-Bioprinted Porous Nano Attapulgite Scaffolds with Good Performance for Bone Regeneration. Int J Nanomedicine (2020) 15:6945–60. doi: 10.2147/ijn.S254094
57. Orchard E, Green W, Nair RP, Abreo F, Sunavala-Dossabhoy G. Sildenafil Transiently Delays Early Alveolar Healing of Tooth Extraction Sockets. Clin Surg (2017) 2.
58. Simon MP, Tournaire R, Pouyssegur J. The Angiopoietin-2 Gene of Endothelial Cells Is up-Regulated in Hypoxia by a Hif Binding Site Located in Its First Intron and by the Central Factors Gata-2 and Ets-1. J Cell Physiol (2008) 217(3):809–18. doi: 10.1002/jcp.21558
59. Oh SM, Shin JS, Kim IK, Kim JH, Moon JS, Lee SK, et al. Therapeutic Effects of Hif-1α on Bone Formation around Implants in Diabetic Mice Using Cell-Penetrating DNA-Binding Protein. Molecules (2019) 24(4). doi: 10.3390/molecules24040760
60. Ko KI, Sculean A, Graves DT. Diabetic Wound Healing in Soft and Hard Oral Tissues. Transl Res (2021) 236:72–86. doi: 10.1016/j.trsl.2021.05.001
61. Liu S, Zhang Q, Yu J, Shao N, Lu H, Guo J, et al. Absorbable Thioether Grafted Hyaluronic Acid Nanofibrous Hydrogel for Synergistic Modulation of Inflammation Microenvironment to Accelerate Chronic Diabetic Wound Healing. Adv Healthc Mater (2020) 9(11):e2000198. doi: 10.1002/adhm.202000198
62. Wu X, Xu W, Feng X, He Y, Liu X, Gao Y, et al. Tnf-a Mediated Inflammatory Macrophage Polarization Contributes to the Pathogenesis of Steroid-Induced Osteonecrosis in Mice. Int J Immunopathol Pharmacol (2015) 28(3):351–61. doi: 10.1177/0394632015593228
63. Brandt M, Gerke V, Betz T. Human Endothelial Cells Display a Rapid Tensional Stress Increase in Response to Tumor Necrosis Factor-Α. PloS One (2022) 17(6):e0270197. doi: 10.1371/journal.pone.0270197
64. Santamaria-Jr M, Bagne L, Zaniboni E, Santamaria MP, Jardini MAN, Felonato M, et al. Diabetes Mellitus and Periodontitis: Inflammatory Response in Orthodontic Tooth Movement. Orthod Craniofac Res (2020) 23(1):27–34. doi: 10.1111/ocr.12340
65. Malta FS, Garcia RP, Azarias JS, Ribeiro G, Miranda TS, Shibli JA, et al. Impact of Hyperglycemia and Treatment with Metformin on Ligature-Induced Bone Loss, Bone Repair and Expression of Bone Metabolism Transcription Factors. PloS One (2020) 15(8):e0237660. doi: 10.1371/journal.pone.0237660
66. Komori T. Runx2, an Inducer of Osteoblast and Chondrocyte Differentiation. Histochem Cell Biol (2018) 149(4):313–23. doi: 10.1007/s00418-018-1640-6
67. Vincent C, Findlay DM, Welldon KJ, Wijenayaka AR, Zheng TS, Haynes DR, et al. Pro-Inflammatory Cytokines Tnf-Related Weak Inducer of Apoptosis (Tweak) and Tnfalpha Induce the Mitogen-Activated Protein Kinase (Mapk)-Dependent Expression of Sclerostin in Human Osteoblasts. J Bone Miner Res (2009) 24(8):1434–49. doi: 10.1359/jbmr.090305
68. Komori T. Regulation of Proliferation, Differentiation and Functions of Osteoblasts by Runx2. Int J Mol Sci (2019) 20(7). doi: 10.3390/ijms20071694
69. Kjersem H, Hilsted J, Madsbad S, Wandall JH, Johansen KS, Borregaard N. Polymorphonuclear Leucocyte Dysfunction During Short Term Metabolic Changes from Normo- to Hyperglycemia in Type 1 (Insulin Dependent) Diabetic Patients. Infection (1988) 16(4):215–21. doi: 10.1007/bf01650754
70. Fernandes KS, Glick M, de Souza MS, Kokron CM, Gallottini M. Association between Immunologic Parameters, Glycemic Control, and Postextraction Complications in Patients with Type 2 Diabetes. J Am Dent Assoc (2015) 146(8):592–9. doi: 10.1016/j.adaj.2015.02.014
71. Muriach M, Bosch-Morell F, Arnal E, Alexander G, Blomhoff R, Romero FJ. Lutein Prevents the Effect of High Glucose Levels on Immune System Cells in Vivo and in Vitro. J Physiol Biochem (2008) 64(2):149–57. doi: 10.1007/bf03168243
72. Zou W, Liu B, Wang Y, Shi F, Pang S. Metformin Attenuates High Glucose-Induced Injury in Islet Microvascular Endothelial Cells. Bioengineered (2022) 13(2):4385–96. doi: 10.1080/21655979.2022.2033411
73. Galvão Tessaro FH, Ayala TS, Bella LM, Martins JO. Macrophages from a Type 1 Diabetes Mouse Model Present Dysregulated Pl3k/Akt, Erk 1/2 and Sapk/Jnk Levels. Immunobiology (2020) 225(2):151879. doi: 10.1016/j.imbio.2019.11.014
74. Luo Z, Soläng C, Larsson R, Singh K. Interleukin-35 Prevents the Elevation of the M1/M2 Ratio of Macrophages in Experimental Type 1 Diabetes. Int J Mol Sci (2022) 23(14). doi: 10.3390/ijms23147970
75. Li Q, Leng K, Liu Y, Sun H, Gao J, Ren Q, et al. The Impact of Hyperglycaemia on Pkm2-Mediated Nlrp3 Inflammasome/Stress Granule Signalling in Macrophages and Its Correlation with Plaque Vulnerability: An in Vivo and in Vitro Study. Metabolism (2020) 107:154231. doi: 10.1016/j.metabol.2020.154231
76. Li Y, Kong N, Li Z, Tian R, Liu X, Liu G, et al. Bone Marrow Macrophage M2 Polarization and Adipose-Derived Stem Cells Osteogenic Differentiation Synergistically Promote Rehabilitation of Bone Damage. J Cell Biochem (2019) 120(12):19891–901. doi: 10.1002/jcb.29297
77. Zhang B, Yang Y, Yi J, Zhao Z, Ye R. Hyperglycemia Modulates M1/M2 Macrophage Polarization Via Reactive Oxygen Species Overproduction in Ligature-Induced Periodontitis. J Periodontal Res (2021) 56(5):991–1005. doi: 10.1111/jre.12912
78. Yang L, Liu J, Shan Q, Geng G, Shao P. High Glucose Inhibits Proliferation and Differentiation of Osteoblast in Alveolar Bone by Inducing Pyroptosis. Biochem Biophys Res Commun (2020) 522(2):471–8. doi: 10.1016/j.bbrc.2019.11.080
79. Fang Y, Wang ZY, Mao Y, Xin HT, Ren GL, Bai XF. Effects of Insulin-Like Growth Factor I on the Development of Osteoblasts in Hyperglycemia. Diabetes Res Clin Pract (2006) 73(1):95–7. doi: 10.1016/j.diabres.2005.11.010
80. Gu H, Song M, Boonanantanasarn K, Baek K, Woo KM, Ryoo HM, et al. Conditions Inducing Excessive O-Glcnacylation Inhibit Bmp2-Induced Osteogenic Differentiation of C2c12 Cells. Int J Mol Sci (2018) 19(1). doi: 10.3390/ijms19010202
81. Zhang S, Song S, Wang S, Duan Y, Zhu W, Song Y. Type 2 Diabetes Affects Postextraction Socket Healing and Influences First-Stage Implant Surgery: A Study Based on Clinical and Animal Evidence. Clin Implant Dent Relat Res (2019) 21(3):436–45. doi: 10.1111/cid.12780
82. Wang S, Wang L, Shi S, Wang X, He C, Yuan L, et al. Inhibition of Gdf11 Could Promote Bone Healing in the Tooth Extraction Socket and Facilitate Mesenchymal Stem Cell Osteogenic Differentiation in T2dm Pigs. J Periodontol (2020) 91(12):1645–52. doi: 10.1002/jper.20-0011
83. Berggreen E, Sae-Lim V, Bletsa A, Heyeraas KJ. Effect of Denervation on Healing after Tooth Replantation in the Ferret. Acta Odontol Scand (2001) 59(6):379–85. doi: 10.1080/000163501317153239
84. Nowak NC, Menichella DM, Miller R, Paller AS. Cutaneous Innervation in Impaired Diabetic Wound Healing. Transl Res (2021) 236:87–108. doi: 10.1016/j.trsl.2021.05.003
85. Gibran NS, Jang YC, Isik FF, Greenhalgh DG, Muffley LA, Underwood RA, et al. Diminished Neuropeptide Levels Contribute to the Impaired Cutaneous Healing Response Associated with Diabetes Mellitus. J Surg Res (2002) 108(1):122–8. doi: 10.1006/jsre.2002.6525
86. Goto T, Nakao K, Gunjigake KK, Kido MA, Kobayashi S, Tanaka T. Substance P Stimulates Late-Stage Rat Osteoblastic Bone Formation through Neurokinin-1 Receptors. Neuropeptides (2007) 41(1):25–31. doi: 10.1016/j.npep.2006.11.002
87. Theocharidis G, Veves A. Autonomic Nerve Dysfunction and Impaired Diabetic Wound Healing: The Role of Neuropeptides. Auton Neurosci (2020) 223:102610. doi: 10.1016/j.autneu.2019.102610
88. Fabian MR, Sonenberg N, Filipowicz W. Regulation of Mrna Translation and Stability by Micrornas. Annu Rev Biochem (2010) 79:351–79. doi: 10.1146/annurev-biochem-060308-103103
89. Moura J, Børsheim E, Carvalho E. The Role of Micrornas in Diabetic Complications-Special Emphasis on Wound Healing. Genes (Basel) (2014) 5(4):926–56. doi: 10.3390/genes5040926
90. Dangwal S, Stratmann B, Bang C, Lorenzen JM, Kumarswamy R, Fiedler J, et al. Impairment of Wound Healing in Patients with Type 2 Diabetes Mellitus Influences Circulating Microrna Patterns Via Inflammatory Cytokines. Arterioscler Thromb Vasc Biol (2015) 35(6):1480–8. doi: 10.1161/atvbaha.114.305048
91. Ban E, Jeong S, Park M, Kwon H, Park J, Song EJ, et al. Accelerated Wound Healing in Diabetic Mice by Mirna-497 and Its Anti-Inflammatory Activity. BioMed Pharmacother (2020) 121:109613. doi: 10.1016/j.biopha.2019.109613
92. Umehara T, Mori R, Mace KA, Murase T, Abe Y, Yamamoto T, et al. Identification of Specific Mirnas in Neutrophils of Type 2 Diabetic Mice: Overexpression of Mirna-129-2-3p Accelerates Diabetic Wound Healing. Diabetes (2019) 68(3):617–30. doi: 10.2337/db18-0313
93. Pizzino G, Irrera N, Galfo F, Pallio G, Mannino F, D'Amore A, et al. Effects of the Antagomirs 15b and 200b on the Altered Healing Pattern of Diabetic Mice. Br J Pharmacol (2018) 175(4):644–55. doi: 10.1111/bph.14113
94. Xiong Y, Chen L, Yan C, Zhou W, Endo Y, Liu J, et al. Circulating Exosomal Mir-20b-5p Inhibition Restores Wnt9b Signaling and Reverses Diabetes-Associated Impaired Wound Healing. Small (2020) 16(3):e1904044. doi: 10.1002/smll.201904044
95. Strauss FJ, Stähli A, Kobatake R, Tangl S, Heimel P, Apaza Alccayhuaman KA, et al. Mirna-21 Deficiency Impairs Alveolar Socket Healing in Mice. J Periodontol (2020) 91(12):1664–72. doi: 10.1002/jper.19-0567
96. Wang JM, Tao J, Chen DD, Cai JJ, Irani K, Wang Q, et al. Microrna Mir-27b Rescues Bone Marrow-Derived Angiogenic Cell Function and Accelerates Wound Healing in Type 2 Diabetes Mellitus. Arterioscler Thromb Vasc Biol (2014) 34(1):99–109. doi: 10.1161/atvbaha.113.302104
97. Gallant-Behm CL, Piper J, Dickinson BA, Dalby CM, Pestano LA, Jackson AL. A Synthetic Microrna-92a Inhibitor (Mrg-110) Accelerates Angiogenesis and Wound Healing in Diabetic and Nondiabetic Wounds. Wound Repair Regener (2018) 26(4):311–23. doi: 10.1111/wrr.12660
98. Wang N, Liu X, Tang Z, Wei X, Dong H, Liu Y, et al. Increased Bmsc Exosomal Mir-140-3p Alleviates Bone Degradation and Promotes Bone Restoration by Targeting Plxnb1 in Diabetic Rats. J Nanobiotechnology (2022) 20(1):97. doi: 10.1186/s12951-022-01267-2
99. Mozzati M, Gallesio G, di Romana S, Bergamasco L, Pol R. Efficacy of Plasma-Rich Growth Factor in the Healing of Postextraction Sockets in Patients Affected by Insulin-Dependent Diabetes Mellitus. J Oral Maxillofac Surg Off J Am Assoc Oral Maxillofac Surgeons (2014) 72(3):456–62. doi: 10.1016/j.joms.2013.10.010
100. Ritto FG, Pimentel T, Canellas JVS, Junger B, Cruz M, Medeiros PJ. Randomized Double-Blind Clinical Trial Evaluation of Bone Healing after Third Molar Surgery with the Use of Leukocyte- and Platelet-Rich Fibrin. Int J Oral Maxillofac Surg (2019) 48(8):1088–93. doi: 10.1016/j.ijom.2019.01.020
101. Afat IM, Akdoğan ET, Gönül O. Effects of Leukocyte- and Platelet-Rich Fibrin Alone and Combined with Hyaluronic Acid on Early Soft Tissue Healing after Surgical Extraction of Impacted Mandibular Third Molars: A Prospective Clinical Study. J Craniomaxillofac Surg (2019) 47(2):280–6. doi: 10.1016/j.jcms.2018.11.023
102. Zahid TM, Nadershah M. Effect of Advanced Platelet-Rich Fibrin on Wound Healing after Third Molar Extraction: A Split-Mouth Randomized Double-Blind Study. J Contemp Dent Pract (2019) 20(10):1164–70.
103. Marin S, Popovic-Pejicic S, Radosevic-Caric B, Trtić N, Tatic Z, Selakovic S. Hyaluronic Acid Treatment Outcome on the Post-Extraction Wound Healing in Patients with Poorly Controlled Type 2 Diabetes: A Randomized Controlled Split-Mouth Study. Med Oral Patol Oral Cir Bucal (2020) 25(2):e154–e60. doi: 10.4317/medoral.23061
104. Radović K, Obradović-Djuričić K, Čairović A, Glišić M, Djurišić S. Prosthetic Treatment after Teeth Extractions in Patients with Type 2 Diabetes Mellitus. Srp Arh Celok Lek (2016) 144(9-10):474–7.
105. Al-Obaidi M, Al-Bayaty F, Al Batran R, Hussaini J, Khor G. Impact of Ellagic Acid in Bone Formation after Tooth Extraction: An Experimental Study on Diabetic Rats. TheScientificWorldJournal (2014) 2014:908098. doi: 10.1155/2014/908098
106. Canellas J, Ritto FG, Figueredo C, Fischer RG, de Oliveira GP, Thole AA, et al. Histomorphometric Evaluation of Different Grafting Materials Used for Alveolar Ridge Preservation: A Systematic Review and Network Meta-Analysis. Int J Oral Maxillofac Surg (2020) 49(6):797–810. doi: 10.1016/j.ijom.2019.10.007
107. Bao M, Du G, Zhang Y, Ma P, Cao Y, Li C. Application of Platelet-Rich Fibrin Derivatives for Mandibular Third Molar Extraction Related Post-Operative Sequelae: A Systematic Review and Network Meta-Analysis. J Oral Maxillofac Surg (2021) 79(12):2421–32. doi: 10.1016/j.joms.2021.07.006
108. Toro LF, de Mello-Neto JM, Santos F, Ferreira LC, Statkievicz C, Cintra LTA, et al. Application of Autologous Platelet-Rich Plasma on Tooth Extraction Site Prevents Occurence of Medication-Related Osteonecrosis of the Jaws in Rats. Sci Rep (2019) 9(1):22. doi: 10.1038/s41598-018-37063-y
109. Guo X, Lu H, Liu C, Zhang Y, Bi L. Effects of Super-Activated Platelet Lysate on Early Healing of Tooth Extraction Sockets in Rats. Drug Des Devel Ther (2022) 16:2213–27. doi: 10.2147/dddt.S363766
110. Fang Y, Wang LP, Du FL, Liu WJ, Ren GL. Effects of Insulin-Like Growth Factor I on Alveolar Bone Remodeling in Diabetic Rats. J Periodontal Res (2013) 48(2):144–50. doi: 10.1111/j.1600-0765.2012.01512.x
111. McDonald A, Williams RM, Regan FM, Semple RK, Dunger DB. Igf-I Treatment of Insulin Resistance. Eur J Endocrinol (2007) 157 Suppl 1:S51–6. doi: 10.1530/eje-07-0271
112. Brancaccio Y, Antonelli A, Barone S, Bennardo F, Fortunato L, Giudice A. Evaluation of Local Hemostatic Efficacy after Dental Extractions in Patients Taking Antiplatelet Drugs: A Randomized Clinical Trial. Clin Oral Investig (2021) 25(3):1159–67. doi: 10.1007/s00784-020-03420-3
113. Wang X, Fok MR, Pelekos G, Jin L, Tonetti MS. Increased Local Concentrations of Growth Factors from Leucocyte- and Platelet-Rich Fibrin Do Not Translate into Improved Alveolar Ridge Preservation: An Intra-Individual Mechanistic Randomized Controlled Trial. J Clin Periodontol (2022). doi: 10.1111/jcpe.13688
114. de Santana RB, Trackman PC. Effect of Targeted Delivery of Bone Morphogenetic Protein-2 on Bone Formation in Type 1 Diabetes. Int J Oral Maxillofac Implants (2015) 30(3):707–14. doi: 10.11607/jomi.3956
115. Ebisawa T, Tada K, Kitajima I, Tojo K, Sampath TK, Kawabata M, et al. Characterization of Bone Morphogenetic Protein-6 Signaling Pathways in Osteoblast Differentiation. J Cell Sci (1999) 112(Pt 20):3519–27. doi: 10.1242/jcs.112.20.3519
116. Shyng YC, Chi CY, Devlin H, Sloan P. Healing of Tooth Extraction Sockets in the Streptozotocin Diabetic Rat Model: Induction of Cartilage by Bmp-6. Growth Factors (2010) 28(6):447–51. doi: 10.3109/08977194.2010.527966
117. Nguyen TQ, Chon H, van Nieuwenhoven FA, Braam B, Verhaar MC, Goldschmeding R. Myofibroblast Progenitor Cells Are Increased in Number in Patients with Type 1 Diabetes and Express Less Bone Morphogenetic Protein 6: A Novel Clue to Adverse Tissue Remodelling? Diabetologia (2006) 49(5):1039–48. doi: 10.1007/s00125-006-0172-0
118. Gan J, Liu C, Li H, Wang S, Wang Z, Kang Z, et al. Accelerated Wound Healing in Diabetes by Reprogramming the Macrophages with Particle-Induced Clustering of the Mannose Receptors. Biomaterials (2019) 219:119340. doi: 10.1016/j.biomaterials.2019.119340
119. Chen H, Fu T, Ma Y, Wu X, Li X, Li X, et al. Intermittent Administration of Parathyroid Hormone Ameliorated Alveolar Bone Loss in Experimental Periodontitis in Streptozotocin-Induced Diabetic Rats. Arch Oral Biol (2017) 83:76–84. doi: 10.1016/j.archoralbio.2017.06.033
120. Xu L, Mei L, Zhao R, Yi J, Jiang Y, Li R, et al. The Effects of Intro-Oral Parathyroid Hormone on the Healing of Tooth Extraction Socket: An Experimental Study on Hyperglycemic Rats. J Appl Oral Sci (2020) 28:e20190690. doi: 10.1590/1678-7757-2019-0690
121. Altan MF, Kanter M, Donmez S, Kartal ME, Buyukbas S. Combination Therapy of Nigella Sativa and Human Parathyroid Hormone on Bone Mass, Biomechanical Behavior and Structure in Streptozotocin-Induced Diabetic Rats. Acta Histochem (2007) 109(4):304–14. doi: 10.1016/j.acthis.2007.02.006
122. Zhen D, Chen Y, Tang X. Metformin Reverses the Deleterious Effects of High Glucose on Osteoblast Function. J Diabetes Complications (2010) 24(5):334–44. doi: 10.1016/j.jdiacomp.2009.05.002
123. Markowicz-Piasecka M, Sadkowska A, Huttunen KM, Podsiedlik M, Mikiciuk-Olasik E, Sikora J. An Investigation into the Pleiotropic Activity of Metformin. A Glimpse of Haemostasis. Eur J Pharmacol (2020) 872:172984. doi: 10.1016/j.ejphar.2020.172984
124. Inouye KA, Bisch FC, Elsalanty ME, Zakhary I, Khashaba RM, Borke JL. Effect of Metformin on Periimplant Wound Healing in a Rat Model of Type 2 Diabetes. Implant Dent (2014) 23(3):319–27. doi: 10.1097/id.0000000000000069
125. Aguilar A, Zein N, Harmouch E, Hafdi B, Bornert F, Offner D, et al. Application of Chitosan in Bone and Dental Engineering. Molecules (2019) 24(16). doi: 10.3390/molecules24163009
126. Kang MS, Moon JH, Park SC, Jang YP, Choung SY. Spirulina Maxima Reduces Inflammation and Alveolar Bone Loss in Porphyromonas Gingivalis-Induced Periodontitis. Phytomedicine (2021) 81:153420. doi: 10.1016/j.phymed.2020.153420
127. Hendrijantini N, Rostiny R, Kuntjoro M, Sidharta K, Wiyono DSP, Anindyanari A, et al. The Effect of Combination Spirulina-Chitosan on Angiogenesis, Osteoclast, and Osteoblast Cells in Socket Models of Hyperglycemic Rattus Norvegicus. Contemp Clin Dent (2018) 9(4):582–6. doi: 10.4103/ccd.ccd_617_18
128. Abuohashish H, AlAsmari A, Mohany M, Ahmed M, Al-Rejaie S. Supplementation of Morin Restores the Altered Bone Histomorphometry in Hyperglycemic Rodents Via Regulation of Insulin/Igf-1 Signaling. Nutrients (2021) 13(7). doi: 10.3390/nu13072365
129. Luthfi M, Yuliati, Wijayanti EH, Abdul Razak FB, Irmalia WR. The Efficacy of Okra Fruit Extract on the Expression of Transforming Growth Factor Beta 1 in the Tooth Socket of Diabetic Wistar Rats. . Dent Res J (Isfahan) (2021) 18:91. doi: 10.4103/1735-3327.330872
130. Deng J, Golub LM, Lee HM, Raja V, Johnson F, Kucine A, et al. A Novel Modified-Curcumin Promotes Resolvin-Like Activity and Reduces Bone Loss in Diabetes-Induced Experimental Periodontitis. J Inflammation Res (2021) 14:5337–47. doi: 10.2147/jir.S330157
131. Zhang Y, McClain SA, Lee HM, Elburki MS, Yu H, Gu Y, et al. A Novel Chemically Modified Curcumin "Normalizes" Wound-Healing in Rats with Experimentally Induced Type I Diabetes: Initial Studies. J Diabetes Res (2016) 2016:5782904. doi: 10.1155/2016/5782904
132. Homayouni-Rad A, Soroush AR, Khalili L, Norouzi-Panahi L, Kasaie Z, Ejtahed HS. Diabetes Management by Probiotics: Current Knowledge and Future Pespective. Int J Vitam Nutr Res (2016) 86(3-4):215–27. doi: 10.1024/0300-9831/a000273
133. Lim HC, Thoma DS, Jeon M, Song JS, Lee SK, Jung UW. Effect of Hypoxia-Inducible Factor 1α on Early Healing in Extraction Sockets. BioMed Res Int (2018) 2018:8210637. doi: 10.1155/2018/8210637
134. Mendes RM, Silva GA, Lima MF, Calliari MV, Almeida AP, Alves JB, et al. Sodium Hyaluronate Accelerates the Healing Process in Tooth Sockets of Rats. Arch Oral Biol (2008) 53(12):1155–62. doi: 10.1016/j.archoralbio.2008.07.001
135. Sá MA, Andrade VB, Mendes RM, Caliari MV, Ladeira LO, Silva EE, et al. Carbon Nanotubes Functionalized with Sodium Hyaluronate Restore Bone Repair in Diabetic Rat Sockets. Oral Dis (2013) 19(5):484–93. doi: 10.1111/odi.12030
136. John SS, Mohanty S, Chaudhary Z, Sharma P, Kumari S, Verma A. Comparative Evaluation of Low Level Laser Therapy and Cryotherapy in Pain Control and Wound Healing Following Orthodontic Tooth Extraction: A Double Blind Study. J Craniomaxillofac Surg (2020) 48(3):251–60. doi: 10.1016/j.jcms.2020.01.012
137. Dalirsani Z, Ghazi N, Delavarian Z, Pakfetrat A, Esmaily H, Davaji M, et al. Effects of Diode Low-Level Laser Therapy on Healing of Tooth Extraction Sockets: A Histopathological Study in Diabetic Rats. Lasers Med Sci (2021) 36(7):1527–34. doi: 10.1007/s10103-021-03270-9
Keywords: tooth extraction, diabetic, healing, dental extraction sockets, insulin-dependent diabetic
Citation: Yang S, Li Y, Liu C, Wu Y, Wan Z and Shen D (2022) Pathogenesis and treatment of wound healing in patients with diabetes after tooth extraction. Front. Endocrinol. 13:949535. doi: 10.3389/fendo.2022.949535
Received: 21 May 2022; Accepted: 31 August 2022;
Published: 23 September 2022.
Edited by:
Xingwu Ran, Sichuan University, ChinaCopyright © 2022 Yang, Li, Liu, Wu, Wan and Shen. This is an open-access article distributed under the terms of the Creative Commons Attribution License (CC BY). The use, distribution or reproduction in other forums is permitted, provided the original author(s) and the copyright owner(s) are credited and that the original publication in this journal is cited, in accordance with accepted academic practice. No use, distribution or reproduction is permitted which does not comply with these terms.
*Correspondence: Daonan Shen, c2hlbmRhb25hbkAxMjYuY29t