- 1Department of Pharmacy, Deqing People’s Hospital, Huzhou, China
- 2Department of Orthopedics, Shandong Provincial Hospital Affiliated to Shandong First Medical University, Jinan, China
- 3Department of Orthopedics, Sun Yat-Sen Memorial Hospital of Sun Yat-Sen University, Guangzhou, China
- 4Department of Pharmacology, School of Pharmaceutical Sciences, Cheeloo College of Medicine, Shandong University, Jinan, China
- 5Suzhou Research Institute, Shandong University, Suzhou, China
Osteoporosis is a systemic metabolic disease, mainly characterized by reduced bone mineral density and destruction of bone tissue microstructure. However, the molecular mechanisms of osteoporosis need further investigation and exploration. Increasing studies have reported that circular RNAs (circRNAs), a novel type of RNA molecule, play crucial roles in various physiological and pathological processes and bone-related diseases. Based on an in-depth understanding of their roles in bone development, we summarized the multiple regulatory roles and underlying mechanisms of circRNA–miRNA–mRNA networks in the treatment of osteoporosis, associated with bone marrow mesenchymal stem cells (BMSCs), osteoblasts, and osteoclasts. Deeper insights into the vital roles of circRNA–miRNA–mRNA networks can provide new directions and insights for developing novel diagnostic biomarkers and therapeutic targets in the treatment of osteoporosis.
1 Introduction
Osteoporosis (OP) is a common systemic metabolic disease, with high morbidity among the elderly especially in postmenopausal women (1). It is identified by the decreased bone toughness and altered bone microarchitecture, leading to an increase in skeletal fragility and fracture risk (2). Fracture is the most serious consequence of osteoporosis in the elderly, which easily leads to myeloid fracture, vertebral compression fracture, and other bone disorders. Osteoporosis has become one of the important causes of disability and death in the elderly due to its high incidence, high surgical risk, and poor prognosis (3, 4). With the aging of the population, osteoporosis has become a global health problem; it has been estimated that approximately 200 million individuals are affected by different levels of osteoporosis. The process of bone strength damage occurs silently and progressively, and there are no symptoms except fracture generally. Thus, the phenomenon not only has a socioeconomic impact and increases the healthcare cost related to osteoporosis, but also causes a heavy burden to patients with osteoporosis and their families (5, 6). However, there is no specific medicine to cure the disease in clinical practice, and the existing therapies can merely retard the process of osteoporosis and reduce the risk of fractures. Thus, it is imminently necessary to search for novel and effective therapeutics for osteoporosis.
Bone is a multifunctional, highly mineralized connective tissue acting as the center for the locomotory system and providing structural support for all internal organs. It is worth noting that bone is also a storeroom of calcium and phosphorus, which are prerequisite to maintain mineral homeostasis. Bone tissue metabolism is particularly active, and it endures constant remodeling during the whole life process. Bone remodeling is the process in which old bones are replaced by new bones, thereby maintaining bone mineral homeostasis and strength via a coupling between osteoblast-induced bone formation and osteoclast-mediated bone resorption (7–9). When the bones are affected by unfavorable factors, including aging, malnutrition, alcohol abuse, estrogen reduction, and connected adverse reactions to medications, the balance between bone formation and resorption will be broken, which is the main cause of generation osteoporosis (10, 11). However, because of a series of endogenous and exogenous factors included in bone metabolism, the particular molecular mechanism underlying the development of osteoporosis remains unclear (12–14).
Non-coding RNAs (ncRNAs), which are the novel focus point in most bioscience research, such as circular RNAs (circRNAs), microRNAs (miRNAs), and long ncRNAs (lncRNAs), have vital roles as regulators in various disease progressions, including osteoporosis (15, 16). CircRNAs, which widely exist in diverse organisms, emerge as a novel type of RNAs with a covalently closed-loop structure, implicated in the regulation of various biological activities, including cell growth, signaling, and multiple physiological and pathological responses (17). For example, circ_0000854, a novel circRNA, was detected to accelerate hepatocellular carcinoma (HCC) progression via the miR-1294/IRGQ axis, and silencing circ_0000854 suppresses cancer cell malignant behaviors, providing unique regulatory targets for HCC pathogenesis (18). Circ_SMG6 aggravated the resultant myocardial ischemia/reperfusion (I/R) injury, which might be related to the circ_SMG6-miR-138-5p-EGR1 network (19). This pathway can provide a novel therapeutic target to myocardial I/R injury (19). Meanwhile, emerging studies indicate that several circRNAs and miRNAs have been found to participate in the regulation of bone marrow mesenchymal stem cell (BMSC) differentiation and osteoporosis pathogenesis. It has been reported that circRNAs act as molecular sponges of miRNAs by competing for the rich miRNA-binding sites and interfering its expression with target messenger RNAs (mRNAs), which has been validated in various particular bone cellular activities (20). Moreover, the growing number of evidence proves that circRNA–miRNA–mRNA networks are a series of novel regulators in bone development (Tables 1 and Table 2) (Figure 1). In this review, we comprehensively searched the PubMed database (https://pubmed.ncbi.nlm.nih.gov/) with the combined keywords “circRNA”, “miRNA”, and “osteoporosis”, and summarized the roles of circRNA–miRNA–mRNA networks in the development and treatment of osteoporosis.
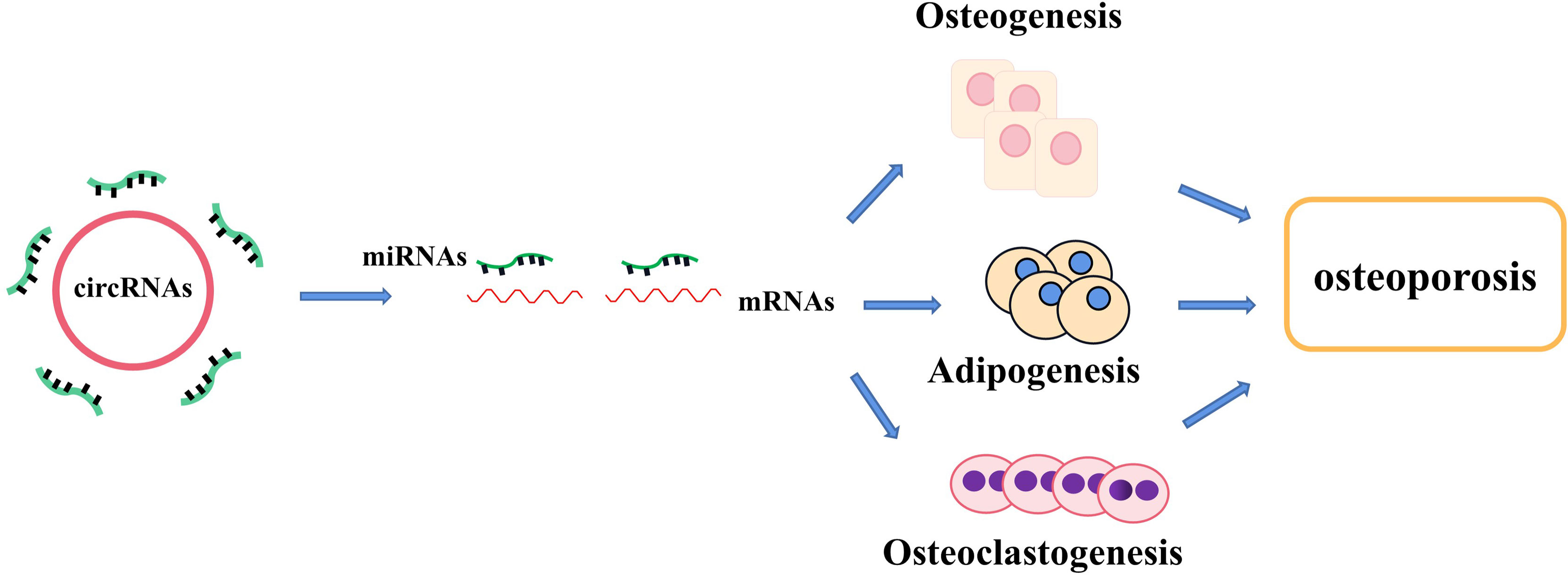
Figure 1 The regulatory roles of circRNA–miRNA–mRNA networks in bone development and bone homeostasis. CircRNA–miRNA–mRNA networks regulate the osteogenesis, adipogenesis, and osteoclastogenesis in several types of cells and provide a therapeutical approach to treating abnormal bone metabolism including osteoporosis.
2 The roles of circRNA–miRNA–mRNA networks in the development of osteoporosis
2.1 Biogenesis and function of circRNAs
CircRNAs are a new type of non-coding RNAs with a closed continuous loop structure covalently via the concatenating of the 3’-poly(A) tails and 5’-end capping splice sites, which were first found in viroid in 1976 and widely distributed in nature (63). Compared with linear RNAs, circRNAs are more stable to exonuclease RNase Rand and highly conserved molecules (64). CircRNAs can be roughly classified into three categories: intronic circRNAs, exonic circRNAs, and exon–intron circRNAs, on the basis of the genome origin and their means of generation. Because of splicing errors, circRNAs were previously considered as nonfunctional by-products (64). In recent years, increasing circRNAs were investigated to function in a class of biological processes, such as cell proliferation, differentiation, and apoptosis (50, 61). According to a recent report, circFAM120B, as a tumor suppressor, hampers cell proliferation, metastasis, and invasion in esophageal squamous cell carcinoma (ESCC) (65). CircUbe3a could facilitate the cell proliferation, migration, and phenotypic transformation of cardiac fibroblasts (CFs) and then exacerbate myocardial fibrosis after acute myocardial infarction (66). Meanwhile, circRNAs have also been unexpectedly proven to take part in the regulation of bone microarchitecture, pathogenesis, and therapies of osteoporosis. Recently, a report has indicated that circ_0005564 significantly increased the mRNA levels of osteogenic differentiation markers, including RUNX2, OPN, and OCN, and then played energetic roles in osteoporosis (67). Nevertheless, the specific roles of various circRNAs in osteoporosis and the related underlying mechanisms need further exploration.
CircRNAs act as regulators in different essential physiological processes by regulating gene transcription, participating in translation, and acting as miRNA sponges. For instance, in a recent report, circStag1 was found to be related to osteoporosis (68). Overexpression of circStag1 could significantly promote the osteogenic capability in BMSCs, and researchers found that circStag1 directly interplays with an RNA-binding protein, named human antigen R (HuR), to mechanistically promote the regeneration of bone-related tissue (68).
2.2 Biogenesis and function of miRNAs
miRNAs are a kind of stem ring endogenous small non-coding RNA molecules, about 22 nucleotides, and present in all eukaryotic cells (69). miRNAs play a key role in the translation and expression of gene in organisms post-transcriptionally via combining the 3’-UTR region of mRNA and then silencing the expression of target genes (70). The expression of miRNAs exerts the characteristics of tissue specificity and growth process specificity, and they participate in cell proliferation, differentiation, apoptosis, and other development. When the content of some miRNAs changes abnormally, the corresponding diseases will be induced subsequently on account of the abnormal expression levels of target genes (71). It has been reported in the literature that the disorder of several miRNAs is closely related to the occurrence and development of many types of diseases (72, 73). For instance, in cancer, miRNAs can act not only as oncogenes but also as tumor suppressors. At the same time, miRNAs can also be used as markers in the process of cancers, providing new targets for cancer therapy (74). In recent years, the roles of miRNAs in osteoporosis have gradually been recognized. miRNAs can regulate bone metabolism by regulating osteogenic differentiation, osteoclast differentiation, and maturation. Therefore, the abnormal expression of miRNAs, related to bone metabolism, must be closely related to the occurrence of bone-related diseases, such as osteoporosis. According to the study, the level of miR-27a has been verified to be reduced in the disease process of osteoporosis and during adipogenic differentiation (75). Further research found that silencing of miR-27a could decrease bone formation in vivo. Therefore, in-depth study of these miRNAs will be helpful to understand the pathogenesis of bone replacement and osteoporosis and search the clinical diagnosis and treatment of osteoporosis.
2.3 The effects of circRNA–miRNA–mRNA networks as signaling pathway factors in bone development
2.3.1 BMP signaling pathways
Bone morphogenetic proteins (BMPs), one of the members of transforming growth factor-β (TGF-β) superfamily, undertake a pivotal role in regenerating osteogenic differentiation of osteoblast by Smads-related or non-Smads-related pathways. BMP receptors can receive information from BMP ligands, form a complex with co-Smad4 through phosphorylating R-Smad1/5/8, and affect the transcriptional expression of downstream target genes. According to the research, BMP2, BMP6, BMP7, and BMP14 (also named to as GDF5) have been affirmed to be correlated with bone homeostasis (Figure 2).
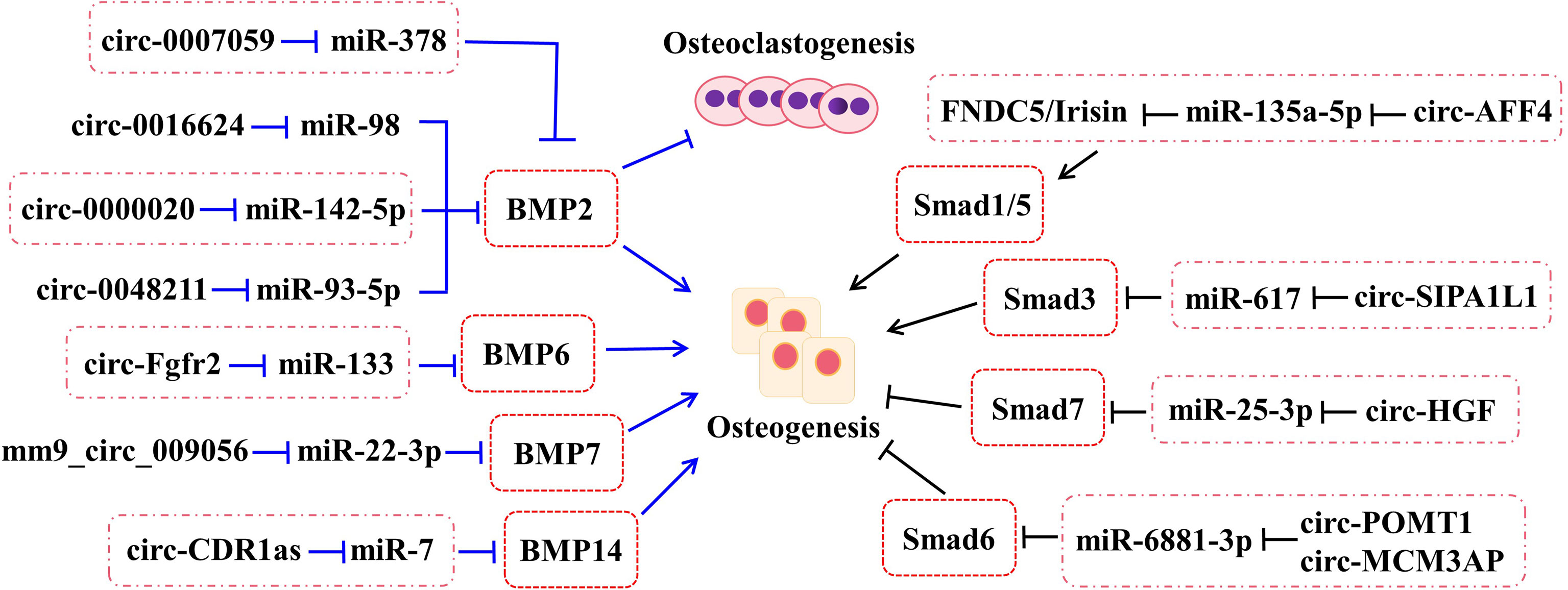
Figure 2 The roles of circRNA–miRNA–mRNA networks related to BMP and Smad pathways in bone development. The circRNA–miRNA–mRNA networks exert the potentials to regulate the bone development by BMP and Smad pathways.
2.3.1.1 BMP2
As a positive cytokine, bone morphogenetic protein-2 (BMP2) is involved in a variety of cellular activities, which is known to induce osteogenic differentiation and regulate bone development and fracture repair. For example, it is confirmed that circ-0000020 could promote osteogenic differentiation, retard the progress of osteoporosis, and upregulate the expression of BMP2 via sponging miR-142-5p as ceRNA (22). Inversely, the silence of circ-0000020 significantly decreased the expression of osteogenic markers, reduced the mineralization ability, and enhanced the apoptosis levels of BMSCs (22). Postmenopausal osteoporosis (PMO), one type of osteoporosis, caused by reduction of estrogen, dramatically reduces the quality of later life of postmenopausal women. According to the study, circ-0007059 was found by screened different circRNA expression levels in PMO patients via RNA-seq and bioinformatics analysis (24). Meanwhile, overexpression of circ-0007059 could attenuate osteoclastogenesis in hBMSCs in vitro (24). Further mechanism studies demonstrated that circ-0007059 directly targeted miR-378, which, in turn, targeted BMP2 (24). Hence, circ-0007059 was verified to function as a novel target in osteoclastogenesis via the miR-378/BMP2 signaling pathway (24). Furthermore, circRNA-0048211 could upregulate the expression of osteogenic genes during bone remodeling, including RUNX2, OPN, and OCN, and alleviate the progression of PMO through the circRNA-0048211/miRNA-93-5p/BMP2 regulatory network (23). The expression levels of circRNA-19142 and circRNA-5846 were observably upregulated in the BMP2-induced osteogenesis group compared with the control group (76). miR-7067-5p was confirmed to the co-targeted miRNAs of the two circRNAs by Venny analysis (76). Both circRNA-19142 and circRNA-5846 have been found to be involved in osteogenic activity through the circRNA-19142/cirRNAc-5846-miRNA-mRNA axis (76). Similarly, the circRNA-0016624/miR-98/BMP2 axis could prevent osteoporosis and offer a novel insight into therapeutic strategy (21).
2.3.1.2 BMP6
CircRNA-Fgfr2 could sponge miR-133 and regulate the expression of bone morphogenetic protein-6 (BMP6) (27). For further validation, overexpression of circRNA-Fgfr2 in rDFCs restrained the expression of miR-133; on the contrary, the level of BMP6 was increased (27). Furthermore, during osteogenic induction, the positive regulation of the circRNA-Fgfr2/miR-133/BMP6 regulatory pathway has been verified (27).
2.3.1.3 BMP7
Bone morphogenetic protein-7 (BMP7) belongs to the BMP family and possesses equivalent osteogenesis function. The calcitonin gene-related peptide (CGRP) could obviously promote the osteogenesis of MC3T3 cells and the expression of mm9_circ_009056 was significantly increased in the CGRP-induced cells (26). Furthermore, it could accelerate the expression levels of miR-22-3p following silencing mm9_circ_009056, but decrease the mRNA and protein levels of BMP7 (26). This phenomenon suggested that mm9_circ_009056 might act as a sponge for miR-22-3p to regulate the expression of BMP7 and then affect osteogenesis-related genes.
2.3.1.4 GDF5
Growth differentiation factor (GDF) 5, also called bone morphogenetic protein-14 (BMP14), has been reported to participate in the process of osteogenic differentiation. The cerebellar degeneration-related protein 1 transcript (CDR1as), as a newly reported circRNA, has been discovered to be involved in the osteogenic differentiation of periodontal ligament stem cells (PDLSCs). By in vivo and in vitro assays, they found that circ-CDR1as plays the role of a miR-7 sponge, leading to upregulate the level of GDF5 and phosphorylate the Smad1/5/8 and p38 mitogen-activated protein kinases (p38 MAPK) mechanistically (77).
2.3.1.5 Others
According to the latest studies, several circRNAs play a part in bone formation-related diseases through the Smad pathway (Figure 2). For example, circ_AFF4 sponges miR-135a-5p and modulates osteoblast differentiation by activating the Smad1/5 pathway via the miR-135a-5p/FNDC5/Irisin network in BMSCs (28). Both circPOMT1 and circMCM3AP have been demonstrated to crosstalk with hsa-miR-6881-3p. Moreover, hsa-miR-6881-3p possibly inhibits Smad6 to activate the BMP signaling pathway and influences osteogenesis of hASCs (52). At the same time, circRNA-SIPA1L1 accelerates osteogenesis in DPSCs via adsorbing miR-617 and further activating the Smad3 pathway (29). Moreover, circHGF, which was significantly upregulated in osteonecrosis of the femoral head (ONFH) sample group compared with the control group by circRNA microarray assay, restrained the cell proliferation and differentiation in BMSCs by acting on the miR-25-3p/Smad7 regulatory pathway (53).
2.3.2 RUNX signaling pathways
2.3.2.1 RUNX2
Runt-related transcription factor 2 (RUNX2), as a critical osteogenic marker gene, is broadly used to observe the osteogenic differentiation process. RUNX2 is involved in osteoblast differentiation through coordinating multiple signaling pathways, including BMPs, TGFs, Wnts, and hedgehogs. In the meantime, the upregulated level of RUNX2 can activate the expression of downstream Osterix, Col I, ALP, and other osteogenic genes. Yin et al. showed that the expression level of circRUNX2, derived from osteoporotic bone tissues, was downregulated (30). CircRUNX2 could be combined with miR-203 and compete for binding sites on miR-203 with RUNX2 (30). Overexpression of circRUNX2 could promote the osteogenic differentiation and restrain the progression of osteoporosis (30). A subsequent study has confirmed that circ-VANGL1, miR-217, and RUNX2 are correlated by the dual-luciferase reporter gene assay (78). At the same time, overexpression of circ-VANGL1 could lead to the increase of miR-217 and the decrease of RUNX2 and then the acceleration of osteogenic differentiation (78). Moreover, the expressions of bone formation-related genes were upregulated and ALP activity was increased by overexpressing circ-VANGL1 in hBMSCs (78). The reduced osteogenic ability is one of the pivotal causes of age-related osteoporosis in BMSCs. Overexpression of hsa_circ_0006215 could enhance the expression of the osteogenesis-related genes, and the condition in the hsa_circ_0006215 knockdown group was reversed (25). The results of luciferase reporter and RNA pull-down assays revealed the relationship between hsa_circ_0006215 and miR-942-5p and RUNX2 (25). The results also showed that hsa_circ_0006215 could regulate the RUNX2 and VEGF expression via targeting miR-942-5p and then promote the osteogenic differentiation (25). Similarly, the current studies show that circRNA-23525 promotes osteogenic differentiation by sponging miR-30a-3p to regulate RUNX2 expression in ADSCs (35). Meanwhile, circ_0011269 functions as a ceRNA binding to miR-122 and then regulates the expression of RUNX2 and accelerates osteoporosis progression (31).
2.3.2.2 RUNX1 and RUNX3
Hsa_circ_0026827, which is increased typically during the osteoblast differentiation of human dental pulp stem cells (hDPSCs), was surprisingly found to promote heterotopic bone formation in vivo. The mechanism of action is via Beclin1 and the RUNX1 signal channels by binding to miR-188-3p, providing a novel thinking for osteoporosis research (34).
It has been proven that hsa_circ_0005752 could regulate osteogenic differentiation, as verified by alkaline phosphatase (ALP) and alizarin red S (ARS) staining assays (32). The hsa_circ_0005752/miR-496/MDM2 network plays a significant role in accelerating osteogenic differentiation. It is worth noting that RUNX3 could enhance the level of hsa_circ_0005752 and then promote osteogenic differentiation (32). On the contrary, knockdown of hsa_circ_0005752 partially antagonizes the function (32). In the meantime, hsa_circRNA_33287 may also affect the osteogenesis under the control of the ceRNA mechanism in maxillary sinus membrane stem cells (MSMSCs), which combines miR-214-3p and Runx3 by forming the hsa_circRNA_33287/miR-214-3p/Runx3 circuit (33). These pathways furnish novel mentalities for bone regeneration and therapeutics for bone disease treatment, such as osteoporosis.
2.3.3 Wnts
The interrelation between circRNA–miRNA–mRNA networks and Wnt signaling during osteogenic differentiation has been revealed (Figure 3). Wnt pathways are divided into the canonical and non-canonical Wnt/β-Catenin pathway.
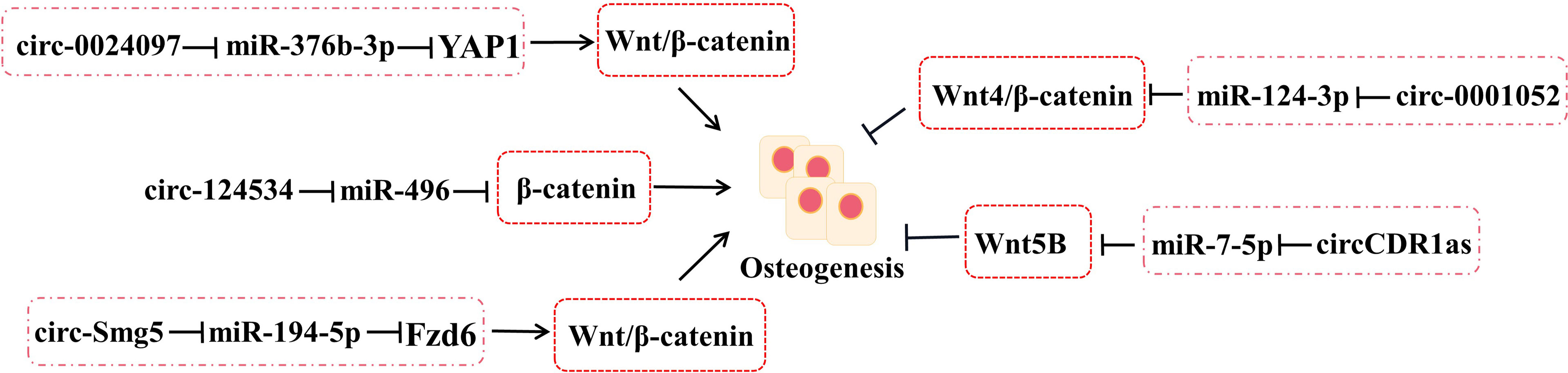
Figure 3 The roles of circRNA–miRNA–mRNA networks related to Wnt/β-catenin pathways in bone development. CircRNA–miRNA–mRNA networks participate in maintaining the bone development via Wnt/β-catenin pathways.
Yes-associated protein 1 (YAP1), which belongs to YAP family, plays an important part in increasing bone mass and retarding bone microstructure degeneration in BMSCs and MC3T3-E1. Circ_0024097 could directly target miR-376b-3p and then attenuate the osteoporosis via both the circ_0024097/miR-376b-3p/YAP1 network and the Wnt/β-catenin pathway (37). Furthermore, WIF-1 acts as a rescue regulatory factor to change the positive effects of circ_0024097 overexpression on osteogenic differentiation, which is the inhibitor of the Wnt/β-catenin pathway (37). Another interesting circRNA for restraining osteoporosis is circRNA-124534, which promotes hDPSC bone regeneration in vitro and in vivo (39). Similarly, it works through the miR-496/β-catenin pathway to enhance the osteogenic differentiation (39). At the same time, circSmg5 could improve osteoblast differentiation via targeting the miR-194-5p/Fzd6 network to activate the Wnt/β-catenin pathway in BMSCs (38).
Several Wnts pertain to the non-canonical Wnt pathway, such as Wnt4, Wnt5a, and Wnt5b. According to the report, circRNA_0001052 is clarified to negatively regulate BMSC proliferation by binding to miR-124-3p as a sponger via the Wnt4/β-catenin pathway (54). It is worth mentioning that the low-level laser irradiation (LLLI) could reverse the process and provide a potential therapeutic to osteoporosis (54). The balance between osteogenic and adipogenic differentiation plays an important role in bone repair in BMSCs. miR-7-5p could link with CDR1as and WNT5B to modulate the osteogenic/adipogenic differentiation disorder, which may provide a novel direction for the molecular mechanisms of bone metabolism-related diseases (55).
3 The treatment of osteoporosis
3.1 Prevention
Osteoporosis is a type of chronic disease that is hardly cured at the present stage. Therefore, it is essential to prevent osteoporosis in advance. Calcium and vitamin D are essential components of skeletons and also the basic strategy for prevention and treatment of osteoporosis, especially beneficial for reducing the risk of fractures in the elderly. Simultaneously, vitamin D is significant in the treatment of anti-resorption and anabolic bone formation. However, when dietary calcium and vitamin D are insufficient to meet the demand, the balance is affected. It is widely agreed upon that food supplementation or the use of drug supplements is necessary to improve calcium and vitamin D levels and then prevent the development of osteoporosis (79, 80). It is widely established that the progress of bone loss may be significantly delayed by focusing on a diet of milk and dairy products, leading to a healthy lifestyle, and taking appropriate calcium and vitamin supplements (81, 82).
3.2 Routine drug therapy
According to the mechanism of function, anti-osteoporosis drugs can be divided into bone resorption inhibitors, bone formation promoters, dual-action regulators, and others. Bone resorption inhibitors are constituted by various drugs, including estrogens, selective estrogen receptor modulators (SERMs), calcitonin, and bisphosphonates. While parathyroid hormone (PTH) and analogues belong to bone formation promoters and strontium salt drugs play dual roles in regulating the new bone formation (Table 3).
3.2.1 Estrogens and SERMs
Estrogen is a steroid sex hormone produced by the endocrine system, which is involved in the physiological and pathological processes of bone tissue and plays an important role in the process of bone reconstruction. Estrogen reduces bone resorption via inhibiting the function of osteoclasts, and similarly selective estrogen receptor modulators are synthetic non-hormonal substances that play the same role in bone metabolism. The typical representative, raloxifene, can significantly increase the bone density and reduce the risk of fracture. However, the risk of breast cancer will increase because of long-term estrogen use. Therefore, long-term estrogen treatment is not recommended (85).
3.2.2 Calcitonin
Calcitonin is a type of peptide hormone derived from thyroid cells and plays a vital role in the treatment of osteoporosis by directly inhibiting the differentiation and proliferation of osteoclasts. Then, calcitonin reduces the number of osteoclasts and destroys the dynamic balance of bone homeostasis. Calcitonin could also increase the bone density and relieve pain in osteoporosis. However, adverse reactions occur in several patients, and the effect of calcitonin on osteoclasts is transient, which exerts the obvious promotion in postmenopausal women (95).
3.2.3 Strontium salts
Strontium salts exhibit multidirectional effects on bone tissue, not only promoting osteogenesis but also inhibiting bone resorption and contributing to reduced possibility of fracture. However, similar to major existing drugs, the disadvantage is obvious. The risk of myocardial infarction will dramatically increase after long-term administration in postmenopausal women (96). Strontium ranelate (SR) is a type of anti-osteoporosis drug with a multidirectional mechanism of action caused by strontium ions, which is similar to calcium in physical and chemical functions, and then plays a key role in regulating bone metabolism via the calcium-sensing receptor (CaSR) (92).
3.3 Novel drug therapies
In recent years, with more in-depth research on the pathogenesis of osteoporosis, a series of therapeutic targets has been developed, and new drugs have been gradually applied in clinical trials.
3.3.1 PTH analogues
Essentially, PTH is a single-chain polypeptide secreted by the main cells of the parathyroid gland and joins in the regulative process of calcium homeostasis. Discontinuous action of PTH will stimulate bone formation and increase bone mass, but long-term action will lead to opposite effects, which are bone resorption enhancement and bone mass reduction (97). Recombinant human parathyroid hormone 1-34 (PTH1-34), which is also called teriparatide, is an active fragment of PTH. Teriparatide is the representative drug in osteoporosis and significantly reduces both vertebral and non-vertebral fractures in postmenopausal patients with osteoporosis (98).
3.3.2 RANKL inhibitors
Denosumab, approved in 2010, competitively binds to receptor activator of NF-κB ligand (RANKL), thereby preventing merging with its receptor, RANK, and then inhibits osteoclast differentiation and activation, also reducing the osteoclastic activity. In clinical studies, it has been found that denosumab brings positive effects to higher spine BMD in the patient with postmenopausal osteoporosis. Dinoselmer is easy to use and a large number of clinical trials have proved that it can significantly reduce bone turnover markers (87, 99). However, discontinuation of denosumab can result in a rebound of the markers and even loss of accrued BMD.
3.3.3 Cathepsin K Inhibitors
Cathepsin K is a member of the family of cysteine proteases, which has the highest expression and bone dissolution in osteoclasts. It decomposes bone tissue by degrading osseocolla and promotes the formation of cavities in bone, and then generates bone homeostasis disorder. Fortunately, two cathepsin K inhibitors, named odanacatib and ONO-5334, have been currently applied in the clinical treatment of osteoporosis. Such medicines will offer new therapeutic options for osteoporosis patients. Odanacatib is a reversibly selective, orally administered cathepsin K inhibitor. Odanacatib could reduce bone resorption and increase BMD and eventually achieve the goal of treating the disease via inhibiting the absorptive activity of osteoclasts, rather than decreasing their number (100). The other inhibitor, ONO-5334, is affirmed to exert the action of increasing BMD and bone strength parameters in the osteoporosis models of ovariectomized monkey and is associated with suppressing bone resorption markers in clinical settings (88). Hence, ONO-5334 was demonstrated as a late-model mode of function which could be used as a potential agent for the treatment of osteoporosis.
3.3.4 Sclerostin antibodies
Sclerostin, a regulatory factor that affects bone remodeling, is secreted only by mature osteocytes and restrains osteogenesis by binding low-density lipoprotein receptor-related protein 5 (LRP5) and LRP6 receptors to inhibit the Wnt/β-catenin pathway (91). Sclerostin monoclonal antibodies, such as romosozumab and blosozumab, have been verified to inhibit sclerotin activity and reduce the sclerotin inhibition of Wnt signaling in multiple animal models. In the meantime, romosozumab could significantly increase the bone mass and reduce fracture risk by reducing bone resorption and promoting bone formation in comparison with alendronate at the lumbar spine (101, 102). Sclerostin antibody shows promise in the treatment of established osteoporosis, although there are some existing problems that need to be solved, including optimal duration and order of administration (103).
3.3.5 CircRNA–miRNA–mRNA networks
As the research progressed, the role of circRNA–miRNA–mRNA networks in regulating bone metabolism balance and diagnosing bone-related diseases, and even in providing new ways of treatment to osteoporosis, was gradually proposed. For example, both the circ_0001795/miR-339-5p/YAP1 axis and the hsa_circ_0006766/miR-4739/Notch2 axis could attenuate osteoporosis progression and could be used as potential candidate therapeutic targets of bone regenerative medicine (36, 45). On the contrary, the circ_0006873-miR-142-5p-PTEN/Akt signaling pathway and the hsa_circ_0006859/miR-431-5p/ROCK1 signaling pathway could reverse the positive effects of osteogenic differentiation and promote the development of osteoporosis, which may provide treatment approaches for osteoporosis (56, 57). Overall, these results are helpful in exploring the pathogenesis of osteoporosis and in providing new strategies for its treatment.
4 Conclusions and perspectives
Above all, osteoporosis is a kind of complex multiple-factor chronic disease that needs further research to solve the problem of pathogenesis. Along with the advancement of osteoblast and osteoclast biology research, researchers have found a variety of regulatory transcription factors and signal pathways in the development of osteoporosis, including circRNA–miRNA–mRNA networks. The circRNA-associated ceRNA networks are closely related to the pathogenesis of osteoporosis by regulating osteoporosis-related key genes. Based on these findings on circRNA–miRNA–mRNA networks in osteoporosis, subsequent studies are needed to verify the application of circRNA–miRNA–mRNA networks in the prevention and treatment for osteoporosis. Targeting key circRNAs or miRNAs, identified as potentially crucial mechanisms underlying osteoporosis, might be a novel therapeutic method for osteoporosis.
Plenty of evidence suggest that these networks play significant roles in modulating the osteogenic/adipogenic differentiation in numerous types of cells and then dedicate to the osteoporosis. These findings are dramatically helpful to improve the current understanding of circRNA–miRNA–mRNA networks’ function in osteogenesis. In addition, existing drugs have obvious adverse reactions or are not suitable for long-term use. Therefore, the adverse reactions limit the longer and widespread use of these drugs. With the emergence of more in-depth studies, new target drugs are gradually proposed, which might be more effective in maintaining the dynamic balance in bone reconstruction, reducing the patient’s pain and improving the quality of life. Meanwhile, the corresponding therapeutics of circRNA–miRNA–mRNA networks are hoped to be applied in clinic and might be novel therapeutic targets in osteoporotic patients. As we mentioned, overexpression or knockdown of key circRNA/miRNAs might be a novel therapeutic strategy for osteoporosis. These important circRNA/miRNAs may be applied as a potential target to develop promising anti-osteoporotic drugs. However, the functions of more potential circRNA–miRNA–mRNA networks in the development of osteoporosis should be verified. A deep understanding of the osteoporosis-specific dysregulated circRNA–miRNA–mRNA network-mediated gene regulation in the development of osteoporosis is necessary to lay a firm foundation to develop promising therapeutic targets for osteoporosis.
Author contributions
MG conceived the review paper with the guidance of YL and BL. MG wrote the original draft. MG, ZZ, JS, BL, and YL reviewed and edited the paper. All authors have read and agreed to the published version of the manuscript. All authors contributed to the article and approved the submitted version.
Funding
This work was supported by the Postdoctoral Science Foundation of China (2021M691956), the Natural Science Foundation of Shandong Province (ZR2021QH087), the Medicine and Health Science and Technology Development Plan Project of Shandong Province (202002040581), the Natural Science Foundation of Jiangsu Province (BK20210110), and the Science and Technology Development Project of Suzhou (SYG202119).
Conflict of interest
The authors declare that the research was conducted in the absence of any commercial or financial relationships that could be construed as a potential conflict of interest.
Publisher’s note
All claims expressed in this article are solely those of the authors and do not necessarily represent those of their affiliated organizations, or those of the publisher, the editors and the reviewers. Any product that may be evaluated in this article, or claim that may be made by its manufacturer, is not guaranteed or endorsed by the publisher.
References
1. Yang F, Yang L, Li Y, Yan G, Feng C, Liu T, et al. Melatonin protects bone marrow mesenchymal stem cells against iron overload-induced aberrant differentiation and senescence. J Pineal Res (2017) 63(3):e12422. doi: 10.1111/jpi.12422
2. Li Y, Meng L, Zhao B. The roles of N6-methyladenosine methylation in the regulation of bone development, bone remodeling and osteoporosis. Pharmacol Ther (2022) 238:108174. doi: 10.1016/j.pharmthera.2022.108174
3. Lee KS, Lee J, Kim HK, Yeom SH, Woo CH, Jung YJ, et al. Extracellular vesicles from adipose tissue-derived stem cells alleviate osteoporosis through osteoprotegerin and miR-21-5p. J Extracell Vesicles (2021) 10(12):e12152. doi: 10.1002/jev2.12152
4. Shen JJ, Zhang CH, Chen ZW, Wang ZX, Yang DC, Zhang FL, et al. LncRNA HOTAIR inhibited osteogenic differentiation of BMSCs by regulating wnt/beta-catenin pathway. Eur Rev Med Pharmacol Sci (2019) 23(17):7232–46.
5. Boschitsch EP, Durchschlag E, Dimai HP. Age-related prevalence of osteoporosis and fragility fractures: real-world data from an Austrian menopause and osteoporosis clinic. Climacteric (2017) 20(2):157–63. doi: 10.1080/13697137.2017.1282452
6. Pisani P, Renna MD, Conversano F, Casciaro E, Di Paola M, Quarta E, et al. Major osteoporotic fragility fractures: Risk factor updates and societal impact. World J Orthop (2016) 7(3):171–81. doi: 10.5312/wjo.v7.i3.171
7. Zhang Z, Song Y, Wang SI, Ha SH, Jang KY, Park BH, et al. Osteoblasts/Osteocytes sirtuin6 is vital to preventing ischemic osteonecrosis through targeting VDR-RANKL signaling. J Bone Miner Res (2021) 36(3):579–90. doi: 10.1002/jbmr.4207
8. Kim SH, Zhang Z, Moon YJ, Park IW, Cho YG, Jeon R, et al. SPA0355 prevents ovariectomy-induced bone loss in mice. Korean J Physiol Pharmacol (2019) 23(1):47–54. doi: 10.4196/kjpp.2019.23.1.47
9. Moon YJ, Zhang Z, Bang IH, Kwon OK, Yoon SJ, Kim JR, et al. Sirtuin 6 in preosteoclasts suppresses age- and estrogen deficiency-related bone loss by stabilizing estrogen receptor alpha. Cell Death Differ (2019) 26(11):2358–70. doi: 10.1038/s41418-019-0306-9
10. Li Y, Feng C, Gao M, Jin M, Liu T, Yuan Y, et al. MicroRNA-92b-5p modulates melatonin-mediated osteogenic differentiation of bone marrow mesenchymal stem cells by targeting ICAM-1. J Cell Mol Med (2019) 23(9):6140–53. doi: 10.1111/jcmm.14490
11. Li Y, Yang F, Gao M, Gong R, Jin M, Liu T, et al. miR-149-3p regulates the switch between adipogenic and osteogenic differentiation of BMSCs by targeting FTO. Mol Ther Nucleic Acids (2019) 17:590–600. doi: 10.1016/j.omtn.2019.06.023
12. Kumar G, Roger PM. From crosstalk between immune and bone cells to bone erosion in infection. Int J Mol Sci (2019) 20(20):5154. doi: 10.3390/ijms20205154
13. Zhou T, Yang Y, Chen Q, Xie L. Glutamine metabolism is essential for stemness of bone marrow mesenchymal stem cells and bone homeostasis. Stem Cells Int (2019) 2019:8928934. doi: 10.1155/2019/8928934
14. Zhou T, Gai Z, Gao X, Li L. The potential mechanism of exercise combined with natural extracts to prevent and treat postmenopausal osteoporosis. J Healthc Eng (2021) 2021:2852661. doi: 10.1155/2021/2852661
15. Yang L, Li Y, Gong R, Gao M, Feng C, Liu T, et al. The long non-coding RNA-ORLNC1 regulates bone mass by directing mesenchymal stem cell fate. Mol Ther (2019) 27(2):394–410. doi: 10.1016/j.ymthe.2018.11.019
16. Zou J, Sun J, Chen H, Fan X, Qiu Z, Li Y, et al. The regulatory roles of miR-26a in the development of fracture and osteoblasts. Ann Transl Med (2022) 10(2):37. doi: 10.21037/atm-21-6101
17. Kristensen LS, Jakobsen T, Hager H, Kjems J. The emerging roles of circRNAs in cancer and oncology. Nat Rev Clin Oncol (2022) 19(3):188–206. doi: 10.1038/s41571-021-00585-y
18. Lin G, Li J, Chen K, Wang A, Guo C. Circ_0000854 regulates the progression of hepatocellular carcinoma through miR-1294 /IRGQ axis. Clin Immunol (2022) 238:109007. doi: 10.1016/j.clim.2022.109007
19. Huang C, Qu Y, Feng F, Zhang H, Shu L, Zhu X, et al. Cardioprotective effect of circ_SMG6 knockdown against myocardial Ischemia/Reperfusion injury correlates with miR-138-5p-Mediated EGR1/TLR4/TRIF inactivation. Oxid Med Cell Longev (2022) 2022:1927260. doi: 10.1155/2022/1927260
20. Xiong DD, Dang YW, Lin P, Wen DY, He RQ, Luo DZ, et al. A circRNA-miRNA-mRNA network identification for exploring underlying pathogenesis and therapy strategy of hepatocellular carcinoma. J Transl Med (2018) 16(1):220. doi: 10.1186/s12967-018-1593-5
21. Yu L, Liu Y. circRNA_0016624 could sponge miR-98 to regulate BMP2 expression in postmenopausal osteoporosis. Biochem Biophys Res Commun (2019) 516(2):546–50. doi: 10.1016/j.bbrc.2019.06.087
22. Zhou R, Miao S, Xu J, Sun L, Chen Y. Circular RNA circ_0000020 promotes osteogenic differentiation to reduce osteoporosis via sponging microRNA miR-142-5p to up-regulate bone morphogenetic protein BMP2. Bioengineered (2021) 12(1):3824–36. doi: 10.1080/21655979.2021.1949514
23. Qiao L, Li CG, Liu D. CircRNA_0048211 protects postmenopausal osteoporosis through targeting miRNA-93-5p to regulate BMP2. Eur Rev Med Pharmacol Sci (2020) 24(7):3459–66. doi: 10.26355/eurrev_202004_20804
24. Liu S, Wang C, Bai J, Li X, Yuan J, Shi Z, et al. Involvement of circRNA_0007059 in the regulation of postmenopausal osteoporosis by promoting the microRNA-378/BMP-2 axis. Cell Biol Int (2021) 45(2):447–55. doi: 10.1002/cbin.11502
25. Ji H, Cui X, Yang Y, Zhou X. CircRNA hsa_circ_0006215 promotes osteogenic differentiation of BMSCs and enhances osteogenesis-angiogenesis coupling by competitively binding to miR-942-5p and regulating RUNX2 and VEGF. Aging (Albany NY) (2021) 13(7):10275–88. doi: 10.18632/aging.202791
26. Wu C, Zheng Z, Ren W, Deng T, Li Y, Yang L, et al. Mm9_circ_009056 enhances osteogenesis by targeting BMP7 via CGRP-mediated miR-22-3p. Biochem Biophys Res Commun (2018) 501(1):199–205. doi: 10.1016/j.bbrc.2018.04.215
27. Du Y, Li J, Hou Y, Chen C, Long W, Jiang H. Alteration of circular RNA expression in rat dental follicle cells during osteogenic differentiation. J Cell Biochem (2019) 120(8):13289–301. doi: 10.1002/jcb.28603
28. Liu C, Liu AS, Zhong D, Wang CG, Yu M, Zhang HW, et al. Circular RNA AFF4 modulates osteogenic differentiation in BM-MSCs by activating SMAD1/5 pathway through miR-135a-5p/FNDC5/Irisin axis. Cell Death Dis (2021) 12(7):631. doi: 10.1038/s41419-021-03877-4
29. Ge X, Li Z, Zhou Z, Xia Y, Bian M, Yu J. Circular RNA SIPA1L1 promotes osteogenesis via regulating the miR-617/Smad3 axis in dental pulp stem cells. Stem Cell Res Ther (2020) 11(1):364. doi: 10.1186/s13287-020-01877-3
30. Yin Q, Wang J, Fu Q, Gu S, Rui Y. CircRUNX2 through has-miR-203 regulates RUNX2 to prevent osteoporosis. J Cell Mol Med (2018) 22(12):6112–21. doi: 10.1111/jcmm.13888
31. Xu X, Chen Y, Tan B, Wang D, Yuan Z, Wang F. Circular RNA circ_0011269 sponges miR-122 to regulate RUNX2 expression and promotes osteoporosis progression. J Cell Biochem (2020) 121(12):4819–26. doi: 10.1002/jcb.29709
32. Wang M, Huan Y, Li X, Li J, Lv G. RUNX3 derived hsa_circ_0005752 accelerates the osteogenic differentiation of adipose-derived stem cells via the miR-496/MDM2-p53 pathway. Regener Ther (2021) 18:430–40. doi: 10.1016/j.reth.2021.09.006
33. Peng W, Zhu S, Chen J, Wang J, Rong Q, Chen S. Hsa_circRNA_33287 promotes the osteogenic differentiation of maxillary sinus membrane stem cells via miR-214-3p/Runx3. BioMed Pharmacother (2019) 109:1709–17. doi: 10.1016/j.biopha.2018.10.159
34. Ji F, Zhu L, Pan J, Shen Z, Yang Z, Wang J, et al. hsa_circ_0026827 promotes osteoblast differentiation of human dental pulp stem cells through the Beclin1 and RUNX1 signaling pathways by sponging miR-188-3p. Front Cell Dev Biol (2020) 8:470. doi: 10.3389/fcell.2020.00470
35. Guo Z, Zhao L, Ji S, Long T, Huang Y, Ju R, et al. CircRNA-23525 regulates osteogenic differentiation of adipose-derived mesenchymal stem cells via miR-30a-3p. Cell Tissue Res (2021) 383(2):795–807. doi: 10.1007/s00441-020-03305-7
36. Li M, Li C, Zheng H, Zhou Z, Yang W, Gong Y, et al. CircRNA_0001795 sponges miRNA-339-5p to regulate yes-associated protein 1 expression and attenuate osteoporosis progression. Bioengineered (2022) 13(2):2803–15. doi: 10.1080/21655979.2021.2022074
37. Huang Y, Xiao D, Huang S, Zhuang J, Zheng X, Chang Y, et al. Circular RNA YAP1 attenuates osteoporosis through up-regulation of YAP1 and activation of wnt/beta-catenin pathway. BioMed Pharmacother (2020) 129:110365. doi: 10.1016/j.biopha.2020.110365
38. Lu Y, Liu YK, Wan FY, Shi S, Tao R. CircSmg5 stimulates the osteogenic differentiation of bone marrow mesenchymal stem cells by targeting the miR-194-5p/Fzd6 axis and beta-catenin signaling. Environ Toxicol (2022) 37(3):593–602. doi: 10.1002/tox.23425
39. Ji F, Pan J, Shen Z, Yang Z, Wang J, Bai X, et al. The circular RNA circRNA124534 promotes osteogenic differentiation of human dental pulp stem cells through modulation of the miR-496/beta-Catenin pathway. Front Cell Dev Biol (2020) 8:230. doi: 10.3389/fcell.2020.00230
40. Wang XB, Li PB, Guo SF, Yang QS, Chen ZX, Wang D, et al. circRNA_0006393 promotes osteogenesis in glucocorticoidinduced osteoporosis by sponging miR1455p and upregulating FOXO1. Mol Med Rep (2019) 20(3):2851–8. doi: 10.3892/mmr.2019.10497
41. Shen W, Sun B, Zhou C, Ming W, Zhang S, Wu X. CircFOXP1/FOXP1 promotes osteogenic differentiation in adipose-derived mesenchymal stem cells and bone regeneration in osteoporosis via miR-33a-5p. J Cell Mol Med (2020) 24(21):12513–24. doi: 10.1111/jcmm.15792
42. Cao G, Meng X, Han X, Li J. Exosomes derived from circRNA Rtn4-modified BMSCs attenuate TNF-alpha-induced cytotoxicity and apoptosis in murine MC3T3-E1 cells by sponging miR-146a. Biosci Rep (2020) 40(5):BSR20193436. doi: 10.1042/BSR20193436
43. Wen J, Guan Z, Yu B, Guo J, Shi Y, Hu L. Circular RNA hsa_circ_0076906 competes with OGN for miR-1305 biding site to alleviate the progression of osteoporosis. Int J Biochem Cell Biol (2020) 122:105719. doi: 10.1016/j.biocel.2020.105719
44. Li F, Wu H, Zou G, Cang D, Shen Y. Circular RNA_0062582 promotes osteogenic differentiation of human bone marrow mesenchymal stem cells via regulation of microRNA-145/CBFB axis. Bioengineered (2021) 12(1):1952–63. doi: 10.1080/21655979.2021.1921553
45. Guo Z, Xie M, Zou Y, Liang Q, Liu F, Su J, et al. Circular RNA Hsa_circ_0006766 targets microRNA miR-4739 to regulate osteogenic differentiation of human bone marrow mesenchymal stem cells. Bioengineered (2021) 12(1):5679–87. doi: 10.1080/21655979.2021.1967712
46. Zhang D, Ni N, Wang Y, Tang Z, Gao H, Ju Y, et al. CircRNA-vgll3 promotes osteogenic differentiation of adipose-derived mesenchymal stem cells via modulating miRNA-dependent integrin alpha5 expression. Cell Death Differ (2021) 28(1):283–302. doi: 10.1038/s41418-020-0600-6
47. Mi B, Xiong Y, Chen L, Yan C, Endo Y, Liu Y, et al. CircRNA AFF4 promotes osteoblast cells proliferation and inhibits apoptosis via the mir-7223-5p/PIK3R1 axis. Aging (Albany NY) (2019) 11(24):11988–2001. doi: 10.18632/aging.102524
48. Zhai Q, Zhao Y, Wang L, Dai Y, Zhao P, Xiang X, et al. CircRNA hsa_circ_0008500 acts as a miR-1301-3p sponge to promote osteoblast mineralization by upregulating PADI4. Front Cell Dev Biol (2020) 8:602731. doi: 10.3389/fcell.2020.602731
49. Ouyang Z, Tan T, Zhang X, Wan J, Zhou Y, Jiang G, et al. CircRNA hsa_circ_0074834 promotes the osteogenesis-angiogenesis coupling process in bone mesenchymal stem cells (BMSCs) by acting as a ceRNA for miR-942-5p. Cell Death Dis (2019) 10(12):932. doi: 10.1038/s41419-019-2161-5
50. Li Y, Bian M, Zhou Z, Wu X, Ge X, Xiao T, et al. Circular RNA SIPA1L1 regulates osteoblastic differentiation of stem cells from apical papilla via miR-204-5p/ALPL pathway. Stem Cell Res Ther (2020) 11(1):461. doi: 10.1186/s13287-020-01970-7
51. Chia W, Liu J, Huang YG, Zhang C. A circular RNA derived from DAB1 promotes cell proliferation and osteogenic differentiation of BMSCs via RBPJ/DAB1 axis. Cell Death Dis (2020) 11(5):372. doi: 10.1038/s41419-020-2572-3
52. Huang XQ, Cen X, Sun WT, Xia K, Yu LY, Liu J, et al. CircPOMT1 and circMCM3AP inhibit osteogenic differentiation of human adipose-derived stem cells by targeting miR-6881-3p. Am J Transl Res (2019) 11(8):4776–88.
53. Feng X, Xiang Q, Jia J, Guo T, Liao Z, Yang S, et al. CircHGF suppressed cell proliferation and osteogenic differentiation of BMSCs in ONFH via inhibiting miR-25-3p binding to SMAD7. Mol Ther Nucleic Acids (2022) 28:99–113. doi: 10.1016/j.omtn.2022.02.017
54. Liu N, Lu W, Qu X, Zhu C. LLLI promotes BMSC proliferation through circRNA_0001052/miR-124-3p. Lasers Med Sci (2022) 37(2):849–56. doi: 10.1007/s10103-021-03322-0
55. Chen G, Wang Q, Li Z, Yang Q, Liu Y, Du Z, et al. Circular RNA CDR1as promotes adipogenic and suppresses osteogenic differentiation of BMSCs in steroid-induced osteonecrosis of the femoral head. Bone (2020) 133:115258. doi: 10.1016/j.bone.2020.115258
56. Lv G, Chen Y, Cheng Z, Lin L, Shen H. Circ_0006873 sponges miR-142-5p to inhibit osteoblastic differentiation of hBMSCs via regulating PTEN/Akt signaling pathway. Ann Clin Lab Sci (2022) 52(1):48–59.
57. Zhi F, Ding Y, Wang R, Yang Y, Luo K, Hua F. Exosomal hsa_circ_0006859 is a potential biomarker for postmenopausal osteoporosis and enhances adipogenic versus osteogenic differentiation in human bone marrow mesenchymal stem cells by sponging miR-431-5p. Stem Cell Res Ther (2021) 12(1):157. doi: 10.1186/s13287-021-02214-y
58. Miao F, Yin BH, Zhang X, Xue DD, Ma C. CircRNA_009934 induces osteoclast bone resorption via silencing miR-5107. Eur Rev Med Pharmacol Sci (2020) 24(14):7580–8. doi: 10.26355/eurrev_202007_22256
59. Zhang Y, Jia S, Wei Q, Zhuang Z, Li J, Fan Y, et al. CircRNA_25487 inhibits bone repair in trauma-induced osteonecrosis of femoral head by sponging miR-134-3p through p21. Regener Ther (2021) 16:23–31. doi: 10.1016/j.reth.2020.12.003
60. Xu Y, Sun L, Hu J, Xu S. Knockdown of hsa_circ_0001275 reverses dexamethasone-induced osteoblast growth inhibition via mediation of miR-377/CDKN1B axis. PloS One (2021) 16(5):e0252126. doi: 10.1371/journal.pone.0252126
61. Chen X, Ouyang Z, Shen Y, Liu B, Zhang Q, Wan L, et al. CircRNA_28313/miR-195a/CSF1 axis modulates osteoclast differentiation to affect OVX-induced bone absorption in mice. RNA Biol (2019) 16(9):1249–62. doi: 10.1080/15476286.2019.1624470
62. Wang X, Chen T, Deng Z, Gao W, Liang T, Qiu X, et al. Melatonin promotes bone marrow mesenchymal stem cell osteogenic differentiation and prevents osteoporosis development through modulating circ_0003865 that sponges miR-3653-3p. Stem Cell Res Ther (2021) 12(1):150. doi: 10.1186/s13287-020-02070-2
63. Zhang Q, Wang W, Zhou Q, Chen C, Yuan W, Liu J, et al. Roles of circRNAs in the tumour microenvironment. Mol Cancer (2020) 19(1):14. doi: 10.1186/s12943-019-1125-9
64. Yang X, Ye T, Liu H, Lv P, Duan C, Wu X, et al. Expression profiles, biological functions and clinical significance of circRNAs in bladder cancer. Mol Cancer (2021) 20(1):4. doi: 10.1186/s12943-020-01300-8
65. Song H, Tian D, Sun J, Mao X, Kong W, Xu D, et al. circFAM120B functions as a tumor suppressor in esophageal squamous cell carcinoma via the miR-661/PPM1L axis and the PKR/p38 MAPK/EMT pathway. Cell Death Dis (2022) 13(4):361. doi: 10.1038/s41419-022-04818-5
66. Wang Y, Li C, Zhao R, Qiu Z, Shen C, Wang Z, et al. CircUbe3a from M2 macrophage-derived small extracellular vesicles mediates myocardial fibrosis after acute myocardial infarction. Theranostics (2021) 11(13):6315–33. doi: 10.7150/thno.52843
67. Liu Z, Liu Q, Chen S, Su H, Jiang T. Circular RNA Circ_0005564 promotes osteogenic differentiation of bone marrow mesenchymal cells in osteoporosis. Bioengineered (2021) 12(1):4911–23. doi: 10.1080/21655979.2021.1959865
68. Chen G, Long C, Wang S, Wang Z, Chen X, Tang W, et al. Circular RNA circStag1 promotes bone regeneration by interacting with HuR. Bone Res (2022) 10(1):32. doi: 10.1038/s41413-022-00208-x
69. Rupaimoole R, Slack FJ. MicroRNA therapeutics: towards a new era for the management of cancer and other diseases. Nat Rev Drug Discovery (2017) 16(3):203–22. doi: 10.1038/nrd.2016.246
70. Fabian MR, Sonenberg N. The mechanics of miRNA-mediated gene silencing: a look under the hood of miRISC. Nat Struct Mol Biol (2012) 19(6):586–93. doi: 10.1038/nsmb.2296
71. Krol J, Loedige I, Filipowicz W. The widespread regulation of microRNA biogenesis, function and decay. Nat Rev Genet (2010) 11(9):597–610. doi: 10.1038/nrg2843
72. Gebert LFR, MacRae IJ. Regulation of microRNA function in animals. Nat Rev Mol Cell Biol (2019) 20(1):21–37. doi: 10.1038/s41580-018-0045-7
73. Bravo Vazquez LA, Moreno Becerril MY, Mora Hernandez EO, Leon Carmona GG, Aguirre Padilla ME, Chakraborty S, et al. The emerging role of MicroRNAs in bone diseases and their therapeutic potential. Molecules (2021) 27(1):211. doi: 10.3390/molecules27010211
74. Pandey M, Mukhopadhyay A, Sharawat SK, Kumar S. Role of microRNAs in regulating cell proliferation, metastasis and chemoresistance and their applications as cancer biomarkers in small cell lung cancer. Biochim Biophys Acta Rev Cancer (2021) 1876(1):188552. doi: 10.1016/j.bbcan.2021.188552
75. You L, Pan L, Chen L, Gu W, Chen J. MiR-27a is essential for the shift from osteogenic differentiation to adipogenic differentiation of mesenchymal stem cells in postmenopausal osteoporosis. Cell Physiol Biochem (2016) 39(1):253–65. doi: 10.1159/000445621
76. Qian DY, Yan GB, Bai B, Chen Y, Zhang SJ, Yao YC, et al. Differential circRNA expression profiles during the BMP2-induced osteogenic differentiation of MC3T3-E1 cells. BioMed Pharmacother (2017) 90:492–9. doi: 10.1016/j.biopha.2017.03.051
77. Li X, Zheng Y, Zheng Y, Huang Y, Zhang Y, Jia L, et al. Circular RNA CDR1as regulates osteoblastic differentiation of periodontal ligament stem cells via the miR-7/GDF5/SMAD and p38 MAPK signaling pathway. Stem Cell Res Ther (2018) 9(1):232. doi: 10.1186/s13287-018-0976-0
78. Yang L, Zeng Z, Kang N, Yang JC, Wei X, Hai Y. Circ-VANGL1 promotes the progression of osteoporosis by absorbing miRNA-217 to regulate RUNX2 expression. Eur Rev Med Pharmacol Sci (2019) 23(3):949–57. doi: 10.26355/eurrev_201902_16981
79. Dominguez LJ, Farruggia M, Veronese N, Barbagallo M. Vitamin d sources, metabolism, and deficiency: Available compounds and guidelines for its treatment. Metabolites (2021) 11(4):255. doi: 10.3390/metabo11040255
80. Barnsley J, Buckland G, Chan PE, Ong A, Ramos AS, Baxter M, et al. Pathophysiology and treatment of osteoporosis: challenges for clinical practice in older people. Aging Clin Exp Res (2021) 33(4):759–73. doi: 10.1007/s40520-021-01817-y
81. Tanski W, Kosiorowska J, Szymanska-Chabowska A. Osteoporosis - risk factors, pharmaceutical and non-pharmaceutical treatment. Eur Rev Med Pharmacol Sci (2021) 25(9):3557–66. doi: 10.26355/eurrev_202105_25838
82. Ratajczak AE, Zawada A, Rychter AM, Dobrowolska A, Krela-Kazmierczak I. Milk and dairy products: Good or bad for human bone? practical dietary recommendations for the prevention and management of osteoporosis. Nutrients (2021) 13(4):1329. doi: 10.3390/nu13041329
83. Andreopoulou P, Bockman RS. Management of postmenopausal osteoporosis. Annu Rev Med (2015) 66:329–42. doi: 10.1146/annurev-med-070313-022841
84. D'Amelio P, Isaia GC. The use of raloxifene in osteoporosis treatment. Expert Opin Pharmacother (2013) 14(7):949–56. doi: 10.1517/14656566.2013.782002
85. Li N, Gong YC, Chen J. A meta-analysis of the therapeutic effect of intranasal salmon calcitonin on osteoporosis. Eur J Med Res (2021) 26(1):140. doi: 10.1186/s40001-021-00610-x
86. Ebrahimpour A, Sadighi M, Hoveidaei AH, Chehrassan M, Minaei R, Vahedi H, et al. Surgical treatment for bisphosphonate-related atypical femoral fracture: A systematic review. Arch Bone Jt Surg (2021) 9(3):283–96. doi: 10.22038/abjs.2020.52698.2608
87. Migliorini F, Maffulli N, Colarossi G, Eschweiler J, Tingart M, Betsch M. Effect of drugs on bone mineral density in postmenopausal osteoporosis: a Bayesian network meta-analysis. J Orthop Surg Res (2021) 16(1):533. doi: 10.1186/s13018-021-02678-x
88. Eastell R, Nagase S, Small M, Boonen S, Spector T, Ohyama M, et al. Effect of ONO-5334 on bone mineral density and biochemical markers of bone turnover in postmenopausal osteoporosis: 2-year results from the OCEAN study. J Bone Miner Res (2014) 29(2):458–66. doi: 10.1002/jbmr.2047
89. Cipriani C, Pepe J, Silva BC, Rubin MR, Cusano NE, McMahon DJ, et al. Comparative effect of rhPTH(1-84) on bone mineral density and trabecular bone score in hypoparathyroidism and postmenopausal osteoporosis. J Bone Miner Res (2018) 33(12):2132–9. doi: 10.1002/jbmr.3554
90. Dempster DW, Zhou H, Rao SD, Recknor C, Miller PD, Leder BZ, et al. Early effects of abaloparatide on bone formation and resorption indices in postmenopausal women with osteoporosis. J Bone Miner Res (2021) 36(4):644–53. doi: 10.1002/jbmr.4243
91. Gatti D, Viapiana O, Fracassi E, Idolazzi L, Dartizio C, Povino MR, et al. Sclerostin and DKK1 in postmenopausal osteoporosis treated with denosumab. J Bone Miner Res (2012) 27(11):2259–63. doi: 10.1002/jbmr.1681
92. Kolodziejska B, Stepien N, Kolmas J. The influence of strontium on bone tissue metabolism and its application in osteoporosis treatment. Int J Mol Sci (2021) 22(12):6564. doi: 10.3390/ijms22126564
93. Aditya S, Rattan A. Sclerostin inhibition: A novel target for the treatment of postmenopausal osteoporosis. J Midlife Health (2021) 12(4):267–75. doi: 10.4103/jmh.JMH_106_20
94. Xie B, Chen S, Xu Y, Han W, Hu R, Chen M, et al. The impact of glucagon-like peptide 1 receptor agonists on bone metabolism and its possible mechanisms in osteoporosis treatment. Front Pharmacol (2021) 12:697442. doi: 10.3389/fphar.2021.697442
95. Binkley N, Bolognese M, Sidorowicz-Bialynicka A, Vally T, Trout R, Miller C, et al. A phase 3 trial of the efficacy and safety of oral recombinant calcitonin: the oral calcitonin in postmenopausal osteoporosis (ORACAL) trial. J Bone Miner Res (2012) 27(8):1821–9. doi: 10.1002/jbmr.1602
96. Bolland MJ, Grey A. A comparison of adverse event and fracture efficacy data for strontium ranelate in regulatory documents and the publication record. BMJ Open (2014) 4(10):e005787. doi: 10.1136/bmjopen-2014-005787
97. Thouverey C, Caverzasio J. Suppression of p38alpha MAPK signaling in osteoblast lineage cells impairs bone anabolic action of parathyroid hormone. J Bone Miner Res (2016) 31(5):985–93. doi: 10.1002/jbmr.2762
98. Hauser B, Alonso N, Riches PL. Review of current real-world experience with teriparatide as treatment of osteoporosis in different patient groups. J Clin Med (2021) 10(7):1403. doi: 10.3390/jcm10071403
99. Noble JA, McKenna MJ, Crowley RK. Should denosumab treatment for osteoporosis be continued indefinitely? Ther Adv Endocrinol Metab (2021) 12:20420188211010052. doi: 10.1177/20420188211010052
100. Kim B, Cho YJ, Lim W. Osteoporosis therapies and their mechanisms of action (Review). Exp Ther Med (2021) 22(6):1379. doi: 10.3892/etm.2021.10815
101. Brown JP, Engelke K, Keaveny TM, Chines A, Chapurlat R, Foldes AJ, et al. Romosozumab improves lumbar spine bone mass and bone strength parameters relative to alendronate in postmenopausal women: results from the active-controlled fracture study in postmenopausal women with osteoporosis at high risk (ARCH) trial. J Bone Miner Res (2021) 36(11):2139–52. doi: 10.1002/jbmr.4409
102. Chavassieux P, Chapurlat R, Portero-Muzy N, Roux JP, Garcia P, Brown JP, et al. Bone-forming and antiresorptive effects of romosozumab in postmenopausal women with osteoporosis: Bone histomorphometry and microcomputed tomography analysis after 2 and 12 months of treatment. J Bone Miner Res (2019) 34(9):1597–608. doi: 10.1002/jbmr.3735
Keywords: circRNA, miRNA, osteoporosis, bone development, treatment
Citation: Gao M, Zhang Z, Sun J, Li B and Li Y (2022) The roles of circRNA–miRNA–mRNA networks in the development and treatment of osteoporosis. Front. Endocrinol. 13:945310. doi: 10.3389/fendo.2022.945310
Received: 16 May 2022; Accepted: 01 July 2022;
Published: 05 August 2022.
Edited by:
Zhiyong Hou, Third Hospital of Hebei Medical University, ChinaReviewed by:
Xun Sun, Tianjin Hospital, ChinaChangfeng Lu, Zhejiang University School of Medicine, China
Copyright © 2022 Gao, Zhang, Sun, Li and Li. This is an open-access article distributed under the terms of the Creative Commons Attribution License (CC BY). The use, distribution or reproduction in other forums is permitted, provided the original author(s) and the copyright owner(s) are credited and that the original publication in this journal is cited, in accordance with accepted academic practice. No use, distribution or reproduction is permitted which does not comply with these terms.
*Correspondence: Yuan Li, yuanli@sdu.edu.cn; Bo Li, libo_career@163.com