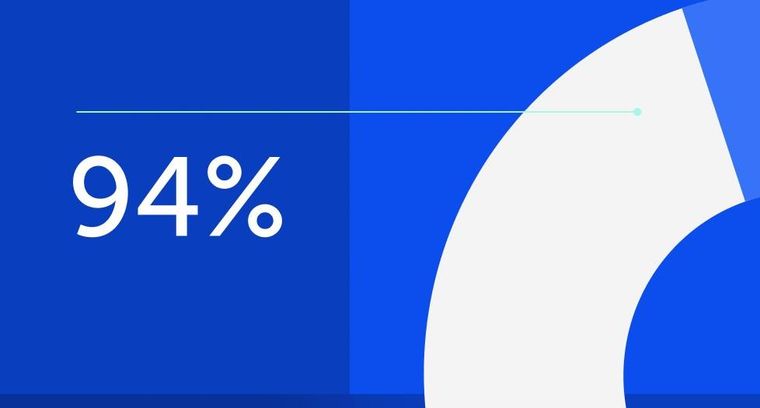
94% of researchers rate our articles as excellent or good
Learn more about the work of our research integrity team to safeguard the quality of each article we publish.
Find out more
REVIEW article
Front. Endocrinol., 09 August 2022
Sec. Developmental Endocrinology
Volume 13 - 2022 | https://doi.org/10.3389/fendo.2022.941029
This article is part of the Research TopicPreterm Birth and Placental PathologyView all 5 articles
The fetal membranes healing is a complex and dynamic process of replacing devitalized and missing cellular structures and tissue layers. Multiple cells and extracellular matrices, and cell differentiation, migration and proliferation may participate in restoring the integrity of damaged tissue, however this process still remains unclear. Therefore, there is a need to identify and integrate new ideas and methods to design a more effective dressing to accelerate fetal membrane healing. This review explores the function and role of galectins in the inflammatory, epithelial mesenchymal transition, proliferative migration, and remodeling phases of fetal membrane healing. In conclusion, the preliminary findings are promising. Research on amnion regeneration is expected to provide insight into potential treatment strategies for premature rupture of membranes.
Premature rupture of the fetal membranes (PROM) is frequently linked to poor postnatal outcomes (1, 2). The membranes may separate and result in tissue injury and amniotic fluid loss (3). The fetal membranes consist of the amnion and chorionic villus (4, 5). The amnion layer is extremely elastic, as it supports fetal development stretches and stresses as well as amniotic fluid buildup throughout pregnancy (6). The chorion layer, which is thicker and stiffer than the amnion, slips over the amnion layer (7). The amnion exhibits striking regenerative potential in few but exciting clinical trials. Furthermore, preclinical wound healing of the fetal membrane has been already reported by the in vitro experiments (amniotic epithelial cells, puncture model of the fetal membrane) (8, 9) and the in vivo system (mice, rabbits) (9, 10), as described in Table 1. However, the key molecular mechanism of fetal membranes to heal wounds after spontaneous or iatrogenic membrane rupture still remains unknown. Previous researches have demonstrated that galectins are a key player in the membrane healing process such as the healing of skin and cornea (11, 12), nonetheless, although galectins are overexpressed on fetal membranes in patients with PROM (13, 14), their function in fetal membrane healing is still unidentified. In this review, we will present the current knowledge on fetal membrane wound healing and discuss the possible role galectins may play in for the development of clinical solution to improve healing of the fetal membranes.
Generally, the amnion consists of two types of cells: superficial epithelial cells and inferior mesenchymal cells. As is reported, the interstitial collagen (types I, III and V) is produced by amniotic mesenchymal cells to maintain the mechanical integrity (15). In some cases, such as amniocentesis, the ruptured membranes could spontaneously “reseal” (16, 17). Remarkably, rats were the first used to make histological investigations of the healing process in embryonic membranes. Sopher (18) demonstrated that puncturing rat gestational sacs on day 15 of pregnancy with a 21-gauge needle resulted in the proliferation of amnion mesenchymal cells within 24 hours. Similarly, via both primary human amnion cells in culture and a aseptic puncture model in mice, Mogami (9) demonstrated that tiny rupture of fetal membrane in the second trimester of pregnancy (0.47 mm) healed successfully in the mice model. Furthermore, after undergoing collagen transplantation to treat spontaneous PPROM patients at 16.5 weeks of pregnancy, the patients successfully delivered boys with an Apgar score of 6-7 at 30 weeks and 3 days, and the boys grew up to be healthy and active adults (19). Despite this, only a few explants and the interactions between the membranes and the sealant were investigated in vivo throughout the complete gestational age. Since the healing of cultured fetal membrane explants may take a long period, in vitro tissue explants become increasingly sick with time, which may limit their ability to repair (20).
Wound healing is a process that restores damaged skin to maintain tissue homeostasis. Adult epidermal wound healing is divided into four main stages: hemostasis, inflammation, proliferation and migration, and regression, remodeling and healing. (21) Fetal tissue healing is much simpler compared to adult tissue (21), the process of amnion membrane tissue rupture is not accompanied by vascular damage, granulation tissue is usually not formed, and inflammation is suppressed to a minimum. The healing of fetal membranes may be initiated by rupture signal activation involved in the recruitment of inflammatory cells (22), while the vascular damage in the chorionic villus may also contribute to injury responses in the fetal membranes (23). In a mouse model of sterile PROM, macrophages are recruited to the site of injury. A well-tissue local inflammatory response induces epithelial mesenchymal transition of amniotic epithelial cells, which accelerates cell migration and healing of the amnion (24). Therefore, it is hypothesized that the healing of fetal membranes (especially healing of amnion membrane) may consist of four stages: ① inflammation, ② epithelial mesenchymal transition EMT, ③ proliferation and migration (mostly migration) (20), and ④ remodeling (it is difficult for fetal membrane to heal completely without normal intervention). It is critical at this stage in the repair of the fetal membrane to increase the potential for epithelial cell migration across the wound.
Galectins, which are carbohydrate-binding proteins that bind to complex carbohydrates on the cell surface and in the extracellular matrix, can decipher this information and govern the interaction between cells or between cells and the ECM (25–27). Over the last few decades, galectins have been identified as crucial for implantation and pregnancy maintenance (28, 29). In a growing number of studies, their role in trophoblast cell function and placental development has been revealed (30). In addition, evidence show that they play key roles in the control of fetal-maternal immunological tolerance and angiogenesis (31). Furthermore, it has also been recognized for its important function in healing. We intend to synthesize current understanding about galectins in the healing of fetal membrane in this review. Galectin family members may activate diverse signal transduction pathways and govern different biological processes, depending on their position within the cell. In the maternal-fetal interface, it is known that 9 subtypes of galectins are substantially expressed, such as galectin-1、-3、-7、-8、-9、-13、-14、-15、-16 (32). Studies demonstrating that galectins are involved in a variety of key cellular responses, including cell-cell and cell ECM interactions, immunological tolerance, and the anti-regulation of the inflammatory response (33–35). Galectin-1 is highly involved in the regulation of the immune response. The presence of significant galectin-1 on the surface of placental mesenchymal stromal cells derived from chorionic villi in early pregnancy, as well as the presence of this galectin in exosomes, opens up a new avenue for galectin-1 to function as a key signal molecule in the placental secretory group (36). Evidence suggests that galectin-1 can exist in integrin subunits, as well as interact with ECM glycoproteins such as fetal fibronectin and laminin, which may have an effect on cell invasion (37). In line with this, human amniotic epithelium expresses galectin-1, galectin-3, galectin-7 and galectin-8, and galectin-3 is significantly increased in the amniotic epithelium of patients with chorioamnionitis (14). The theory of the healing progress may improve the prognosis of premature rupture of membranes.
As can be seen in Figure 1, the inflammatory response caused by fetal membrane injury provides the possibility of initiation of healing of the fetal membrane (38, 39). In wound healing such as epidermal neutrophils are among the first leukocytes recruited during inflammation, migrating from the damaged vascular system. Monocytes are usually recruited to wounds later than neutrophils; once there, they differentiate into macrophages, which remove debris as well as dying neutrophils by phagocytosis (40). However, studies have observed that only macrophages accumulate around aseptically ruptured amniotic membranes. In contrast to the typical epidermal wound healing process in adults, migration of neutrophils was rarely observed (9). This may be related to the aseptic nature of the absence of infection and inflammatory stimuli. M2-dominant macrophages are recruited from the amniotic fluid to the site of injury and release cytokines such as TNF-α and IL-1β to promote healing of the fetal membranes (9). Therefore, M2 macrophages may play a central role in the inflammatory process of fetal membranes.
Figure 1 Inflammatory phase of fetal/amnion membrane healing. The inflammatory response caused by fetal membrane injury provides the possibility to initiate the healing of fetal membranes. (A) The fetal membranes are ruptured by external infection or mechanical injury; (B) Monocytes are first recruited from amniotic fluid to the site of injury and transformed into macrophages; (C) Galectins stimulate monocytes to enhance the expression levels of Akt, PI3K and PPAR-γ, and turn on the M2 polarization of macrophages; (D) M2-type macrophages release TNF-α, IL-1β and other cytokines to promote fetal membrane healing. M2-type macrophages release cytokines such as TNF-α and IL-1β to promote the healing of amnion membranes. akt, protein kinase B; PI3K, phosphatidylinositol-3 kinase; PPAR-γ, peroxisome proliferator-activated receptor-gamma; TNF-α, tumor necrosis factor-α; IL- 1β, interleukin-1β.
Among all family members, galectin-1, galectin-3 and galectin-9 are highly expressed in immune cells. Peritoneal macrophages from galectin-3-deficient mice showed higher levels of apoptosis compared to cells from wild-type mice, suggesting that galectins may also protect macrophages from cell death (41). Galectin-1 does not affect macrophage survival and also stimulates the ERK1/2 signaling pathway to regulate macrophage activity by promoting arginase activity (42) and PGE2 production (43, 44). In addition, galectin-1 has been shown to promote M2 macrophage phenotypic polarization, tissue repair and regeneration (45). In the presence of galectin-3 inhibitors, M2 markers (mannose receptors) are downregulated and M1 markers (iNOS) are upregulated on RAW264.7 macrophages, and this phenotypic skew suggests that galectin-3 promotes macrophage polarization toward the M2 phenotype (46). THP-1 monocytes co-cultured with human-galectin-9-expressing porcine kidney epithelial cells turned on the M2 differentiation program while decreasing the M1 differentiation program by enhancing the phosphorylation levels of Akt and PI3K as well as the expression level of PPAR-γ (47). LPS regulates macrophage M1/M2 subtype polarization through the galectin-9/Tim-3 signaling pathway, and upregulation of galectin-9 polarizes macrophages to M2 (48). Galectins can be found to promote macrophage survival and stimulate macrophage M2 phenotype polarization to generate an inflammatory response and initiate fetal membrane healing. These findings suggest that galectins could be selected as a potential therapeutic target for fetal membrane rupture to modulate the immune system and promote tissue regeneration.
As illustrated in Figure 2, expression of inflammatory factors after fetal membrane rupture can promote the development of epithelial-mesenchymal transition. Interleukins, TNF-α, and IFN-γ are able to induce EMT and the ability of epithelial cells to transform into myo- mesenchymal stem cells through different signaling pathways. In pPROM mice, waveform protein-positive cells can be observed scattered in the epithelial layer of the ruptured mouse amnion, indicating the occurrence of EMT in vivo. EMT is known to accelerate cell migration and thus wound closure.
Figure 2 Epithelial-mesenchymal transition phase of fetal/amnion membrane healing. Expression of inflammatory factors after amnion membrane rupture can promote epithelial- mesenchymal transition, and EMT can accelerate cell migration, thus accelerating wound closure. (A–C) Galectins stimulate amnion epithelial cells through the Hedgehog signal pathway, TGF-β1/Smad signal pathway, MAPK JNK/p38 signal pathway, FAK / PI3K / AKT / mTOR signal pathway and NF-κB signal pathway stimulated the transformation of amnion epithelial cells into amnion mesenchymal cells. EMT, epithelial-mesenchymal transition.
Despite few research on the participation of the galectin family in EMT, the studies in tumor cells may provide possibility of its involvement in fetal membrane EMT. In galectin-1 overexpressing suppressor T cells (TS cells), galectin-1 promotes EMT by upregulating the expression of N-cadherin and downregulating the expression of E-cadherin, inducing TS cell migration and invasion (49). In gastric cancer fibroblasts (CAF), galectin-1 stimulated high expression by upregulating β1-integrin (50) and induced EMT through the Hedgehog signaling pathway (51), which promoted migration and invasion of cancer cells. Activation of TGF-β by galectin-3 induces EMT in lung cancer A549 cells (52). In fibroblasts, galectin-1 induced EMT through the TGF-β1/Smad signaling pathway thereby promoting invasion and metastasis of gastric cancer cells (53). Galectin-1 induces epithelial mesenchymal transition (EMT) in human ovarian cancer cells through activation of the MAPK JNK/p38 signaling pathway (54). In human prostate cancer transplants, galectin-4 binding to receptor tyrosine kinases activated the expression of phosphorylated ERK, phosphorylated Akt and Twist and decreased E-cadherin expression, thereby promoting EMT (55). Similarly, in upper urothelial migratory cell carcinoma, galectin-1 promoted EMT by activating the FAK/PI3K/AKT/mTOR pathway significantly reduces MMP-2 and MMP-9 levels and promotes EMT (56). Furthermore, in human pancreatic cancer, galectin-1 triggers EMT through NF-κB transcriptional regulation and induces significant overexpression of invasion and migration-related genes including MMP1, S100A7, and ankyrin-3, thus playing a major metastatic invasive role (57). Taken together, we hypothesize that galectins can induce matrix metalloproteinase production through multiple signaling pathways thereby promoting cell migration and achieving wound healing.
Figure 3 shows a hypnosis that, after EMT occurs, it induces cell proliferation, synthesis of collagen, hyaluronic acid, etc., and extracellular matrix (ECM) formation. HAESCs promote wound healing by facilitating the migration and proliferation of keratinocytes via ERK, JNK and AKT signaling pathways (58). Growth factors are among the most important proteins for human cell proliferation. Proteins such as epidermal growth factor (EGF), transforming growth factor β1 (TGF-β1), hepatocyte growth factor (HGF), and basic fibroblast growth factor (bFGF) are structurally active in amniotic cells and promote amniotic cell growth (59). Intestinal epithelial cells lacking galectin-9 expression exhibit an attenuated proliferative response in the presence of regenerative stimuli (60). Galectin-1 can interact with cell surface growth factors, stimulate their phosphorylation, and affect the proliferation of umbilical vein endothelial cells (61). In addition, Galectin-1 was found affecting the development of mouse trophoblast stem cells in vitro and promoting the expression of the Matrix metalloproteinase (MMP) and the TGF-β1 (49). In epithelial cells, galectin-7 regulates the proliferation and differentiation of keratin-forming cells through the JNK1-miR-203-p63 pathway (62). On the other hand, there is evidence that galectin-3 regulates the Smad2/3 signaling pathway through interaction with TGF-β1 protein, which in turn regulates the proliferation and migration of human pulmonary artery smooth muscle cells. Moreover, TGF-β1 and galectin-3 can mutually regulate the expression levels of proteins and mRNAs (63). Studies have showed that active collagen synthesis, matrix remodeling occurs in the healing membrane after a major rupture of the fetal membrane (9). Glycan modification may affect proliferative signaling due to transcriptional or epigenetic regulation of glycan-modifying enzymes and altered expression of glycan-binding proteins (64). Galectin-3 deficiency in Mst1-TG mice leads to dysregulation of signaling related to extracellular matrix remodeling and collagen formation (65). Epithelial cell proliferation is significantly decreased, collagen-I accumulation is decreased, and alpha smooth muscle actin expression is downregulated in galectin-9-deficient mice. Galectin-9 promotes extracellular matrix synthesis and collagen accumulation via TGF-β-induced MAPK/ERK, TAK1/JNK, and PI3K/AKT signaling pathways (66). Galectin-8 stimulates TGF-β1 via β1-integrin/FAK pathways to enhance type I collagen, fibronectin FN and connective tissue growth factor CTGF protein levels in human gingival fibroblasts (67).
Figure 3 Migratory proliferative phase of fetal/amnion membrane healing. (A, B) Galectins regulate cells migration by interacting with MMPs; (C, D) Galectins regulate cells proliferation by interacting with EGF, TGF-β1, HGF, bFGF and other proteins. Abbreviations: EMT, epithelial-mesenchymal transition; MMPs, matrix metalloproteinases; EGF, epidermal growth factor ; TGF-β1, transforming growth-factor-β1; HGF, hepatocyte growth factor; bFGF, basic fibroblast growth factor.
Studies have shown that cells closest to the wound preferentially migrate, while cells far from the wound preferentially proliferate (68). Galectins significantly affect this marker by regulating metastasis-related events. Indeed, galectin-3 has been identified as a glycoprotein associated with cell motility (8). Galectin-3 binds to the cell adhesion glycoprotein CD146 to promote cytokine secretion and mediated endothelial cell migration (69). On the other hand, galectin-3-silenced HTR-8/SVneo cells showed a significant reduction in migration and invasion, low-level expression of MMP-2 and MMP-9, and a massive reduction in integrin β1 (70). Microarray analysis showed that treatment with galectin-1 induced upregulation of genes encoding matrix metalloproteinases MMP-1, MMP-10, MMP-12 and tissue fibrinogen activator in dendritic cells, which was associated with increased migratory activity of these antigen-presenting cells (71). In addition, transfection of galectin-4cDNA into placental trophoblast cells induced enhanced Akt phosphorylation upregulated the expression of MMP-9 and N-Cadherin, promoting trophoblast migration and invasion (72).
In contrast, during the remodeling phase, as inflammation gradually decreases, the proliferative process is downregulated to avoid excessive tissue proliferation and is gradually replaced by the initiation of collagen rearrangement (38, 39). During the remodeling phase, most of the endothelial cells, macrophages and fibroblasts undergo apoptosis and human amniotic mesenchymal cells (AMC) undergo MET to re-differentiate into amniocytes and form the fetal membrane tissue together with the rearranged collagen and extracellular matrix. Amniocytes dynamically switch between epithelial and mesenchymal states to maintain amniotic membrane integrity and repair membrane damage, as well as to respond to inflammation and mechanical injury to protect the fetus until delivery (8, 73). During this process, collagen is rearranged by the action of matrix metalloproteinases, gradually forming an ordered matrix structure, and the major component changes from type III to type I collagen. However, the role of galectins in amniotic tissue remodeling has not been studied yet.
Studies of the role of galectins in wound healing have revealed that galectin-3 promotes re-epithelialization of corneal, intestinal and skin wounds; galectin-7 promotes re-epithelialization of corneal, skin, kidney and uterine wounds; and galectin-2 and galectin-4 promote re-epithelialization of intestinal wounds. Galectin-3 is present on the cell surface, within ECM, and in the cytoplasm. Galectin-3 influences cell-matrix interaction by binding to the ECM and cell surface glycosylated counter receptors (e.g., growth factor receptors, integrins, certain isoforms of laminin, fibronectin and vitronectin). Furthermore, Galectin-3 in the nucleus of cells may influence cell-matrix interactions indirectly by its effect on the expression of well-known cell adhesion molecules (e.g., α6β1 and α4β7 integrins) and cytokines (e.g., IL-1) (12). Galectin-3 is present in high density at sites of corneal epithelial cell-matrix adhesion (74), an ideal placement for influence on cell-matrix interactions and cell migration. In a recent study, using galectin3−/− mice and cells isolated from these mice, Liu et al. (75)demonstrated that the absence of galectin-3 impairs keratinocyte migration and skin wound re-epithelialization. Interestingly, in this study, the promigratory function of the lectin was attributed to cytosolic galectin-3, and, therefore, is likely to be carbohydrate independent. Lagana et al. (76) demonstrated that galectin-3 interactions with N-acetylglucosaminyltransferase V (GnT-V)-modified N-glycans on mammary carcinoma cell surface support α5β1 integrin activation and cell motility. Noorjahan et al. (77) have demonstrated that galectin-3 by interacting with GnT-V-modified complex N-glycans, activates α3β1-integrin-Rac1 signaling to induce formation of lamellipodia in epithelial cells, and, this in turn, promotes cell migration and re-epithelialization of wounds.
We hypothesize that the amnion is capable of healing based on previous research. Wound management agents should actively regulate the fetal membrane (especially healing of amnion membrane) phase and several strategies exist that can support the maintaining of fetal membrane structural integrity. In this review, we summarize the role of galectins in fetal/amniotic membrane healing. During the various stages of amniotic membrane healing, galectins positively influence amniotic membrane wound healing through various activities, including macrophage polarization, cell proliferation migration, collagen synthesis, and ECM remodeling. In vivo and in vitro data suggest that galectins can aid amniotic membrane healing by modulating inflammation and supporting cell proliferation. However, additional data from human systemic studies are needed to fully elucidate the mechanism of action of galectins and support their efficacy in fetal membrane healing.
D-ZC and D-XX conceived the central idea. J-LC screened the literature and wrote the initial draft of the study. The remaining authors contributed to refining the ideas, carrying out additional analyses, and finalizing this study. All authors contributed to editorial changes in the manuscript. All authors read and approved the final manuscript.
This research was funded by Development and Demonstration Program of Wuxi (grant No. N20192004), Key Research and Development Program of Jiangsu Province (grant No. BE2015617), and Anhui Provincial Education Department Scientific Research Project (Postgraduate Innovation Research and Practice Program of Anhui Medical University)(YJS20210285).
The authors declare that the research was conducted in the absence of any commercial or financial relationships that could be construed as a potential conflict of interest.
All claims expressed in this article are solely those of the authors and do not necessarily represent those of their affiliated organizations, or those of the publisher, the editors and the reviewers. Any product that may be evaluated in this article, or claim that may be made by its manufacturer, is not guaranteed or endorsed by the publisher.
1. Goeschen K, Mesrogli M, Rosswog U, Schneider J. [Premature rupture of fetal membranes near term: wait it out or intervene?]. Z Geburtshilfe Perinatol (1989) 193(1):16–23.
2. Rudelstorfer R, Gruber W, Janisch H. [Premature rupture of the fetal membranes and threatened premature labor–fetal outcome in conservative management]. Gynakol Rundsch (1989) 29 Suppl 2:137–40.
3. Menon R, Richardson LS. Preterm prelabor rupture of the membranes: A disease of the fetal membranes. Semin Perinatol (2017) 41(7):409–19. doi: 10.1053/j.semperi.2017.07.012
4. Meinert M, Eriksen GV, Petersen AC, Helmig RB, Laurent C, Uldbjerg N, et al. Proteoglycans and hyaluronan in human fetal membranes. Am J Obstet Gynecol (2001) 184(4):679–85. doi: 10.1067/mob.2001.110294
5. Passloer HJ. Problems and practical application of fibrin gluing in early premature rupture of the fetal membranes. 4 clinical single cases using various procedures. Geburtshilfe Frauenheilkd (1989) 49(12):1067–9. doi: 10.1055/s-2008-1036289
6. Menon R, Moore JJ. Fetal membranes, not a mere appendage of the placenta, but a critical part of the fetal-maternal interface controlling parturition. Obstet Gynecol Clin North Am (2020) 47(1):147–62. doi: 10.1016/j.ogc.2019.10.004
7. Calvin SE, Oyen ML. Microstructure and mechanics of the chorioamnion membrane with an emphasis on fracture properties. Ann N Y Acad Sci (2007) 1101:166–85. doi: 10.1196/annals.1389.009
8. Richardson L, Menon R. Proliferative, migratory, and transition properties reveal metastate of human amnion cells. Am J Pathol (2018) 188(9):2004–15. doi: 10.1016/j.ajpath.2018.05.019
9. Mogami H, Hari Kishore A, Akgul Y, Word RA. Healing of preterm ruptured fetal membranes. Sci Rep (2017) 7(1):13139. doi: 10.1038/s41598-017-13296-1
10. Kivelio A, Dekoninck P, Perrini M, Brubaker CE, Messersmith PB, Mazza E, et al. Mussel mimetic tissue adhesive for fetal membrane repair: initial in vivo investigation in rabbits. Eur J Obstet Gynecol Reprod Biol (2013) 171(2):240–5. doi: 10.1016/j.ejogrb.2013.09.003
11. Dvorankova B, Szabo P, Lacina L, Gal P, Uhrova J, Zima T, et al. Human galectins induce conversion of dermal fibroblasts into myofibroblasts and production of extracellular matrix: potential application in tissue engineering and wound repair. Cells Tissues Organs (2011) 194(6):469–80. doi: 10.1159/000324864
12. Panjwani N. Role of galectins in re-epithelialization of wounds. Ann Transl Med (2014) 2(9):89. doi: 10.3978/j.issn.2305-5839.2014.09.09
13. Kolanowska DG, Swietlicki A, Drews K, Seremak-Mrozikiewicz A. The role of galectins in obstetrics with particular emphasis on premature preterm rupture of membranes. Ginekol Pol (2021) 92(10):731–4. doi: 10.5603/GP.a2021.0187
14. Stefanoska I, Tadić J, Vilotić A, Jovanović Krivokuća M, Abu Rabi T, Vićovac L. Histological chorioamnionitis in preterm prelabor rupture of the membranes is associated with increased expression of galectin-3 by amniotic epithelium. J Matern Fetal Neonatal Med (2017) 30(18):2232–6. doi: 10.1080/14767058.2016.1243100
15. Parry S, Strauss JF. Premature rupture of the fetal membranes. N Engl J Med (1998) 338(10):663–70. doi: 10.1056/nejm199803053381006
16. Johnson JW, Egerman RS, Moorhead J. Cases with ruptured membranes that "reseal". Am J Obstet Gynecol (1990) 163(3):1024–30; discussion 1030-1022. doi: 10.1016/0002-9378(90)91117-u
17. Borgida AF, Mills AA, Feldman DM, Rodis JF, Egan JF. Outcome of pregnancies complicated by ruptured membranes after genetic amniocentesis. Am J Obstet Gynecol (2000) 183(4):937–9. doi: 10.1067/mob.2000.108872
18. Sopher D. The response of rat fetal membranes to injury. Ann R Coll Surg Engl (1972) 51(4):240–9.
19. Mogami H, Kishore AH, Word RA. Collagen type 1 accelerates healing of ruptured fetal membranes. Sci Rep (2018) 8(1):696. doi: 10.1038/s41598-017-18787-9
20. Devlieger R, Gratacos E, Wu J, Verbist L, Pijnenborg R, Deprest JA. An organ-culture for in vitro evaluation of fetal membrane healing capacity. Eur J Obstet Gynecol Reprod Biol (2000) 92(1):145–50. doi: 10.1016/s0301-2115(00)00439-5
21. Subramaniam T, Fauzi MB, Lokanathan Y, Law JX. The role of calcium in wound healing. Int J Mol Sci (2021) 22(12):6486. doi: 10.3390/ijms22126486
22. Cordeiro JV, Jacinto A. The role of transcription-independent damage signals in the initiation of epithelial wound healing. Nat Rev Mol Cell Biol (2013) 14(4):249–62. doi: 10.1038/nrm3541
23. Regan JK, Kannan PS, Kemp MW, Kramer BW, Newnham JP, Jobe AH, et al. Damage-associated molecular pattern and fetal membrane vascular injury and collagen disorganization in lipopolysaccharide-induced intra-amniotic inflammation in fetal sheep. Reprod Sci (2016) 23(1):69–80. doi: 10.1177/1933719115594014
24. Mogami H, Word RA. Healing mechanism of ruptured fetal membrane. Front Physiol (2020) 11:623. doi: 10.3389/fphys.2020.00623
25. Barondes SH, Castronovo V, Cooper DN, Cummings RD, Drickamer K, Feizi T, et al. Galectins: a family of animal beta-galactoside-binding lectins. Cell (1994) 76(4):597–8. doi: 10.1016/0092-8674(94)90498-7
26. Barondes SH, Cooper DN, Gitt MA, Leffler H. Galectins. structure and function of a large family of animal lectins. J Biol Chem (1994) 269(33):20807–10.
27. Kasai K, Hirabayashi J. Galectins: a family of animal lectins that decipher glycocodes. J Biochem (1996) 119(1):1–8. doi: 10.1093/oxfordjournals.jbchem.a021192
28. Terness P, Kallikourdis M, Betz AG, Rabinovich GA, Saito S, Clark DA. Tolerance signaling molecules and pregnancy: IDO, galectins, and the renaissance of regulatory T cells. Am J Reprod Immunol (2007) 58(3):238–54. doi: 10.1111/j.1600-0897.2007.00510.x
29. Than NG, Romero R, Kim CJ, McGowen MR, Papp Z, Wildman DE. Galectins: guardians of eutherian pregnancy at the maternal-fetal interface. Trends Endocrinol Metab (2012) 23(1):23–31. doi: 10.1016/j.tem.2011.09.003
30. Blidner AG, Rabinovich GA. 'Sweetening' pregnancy: galectins at the fetomaternal interface. Am J Reprod Immunol (2013) 69(4):369–82. doi: 10.1111/aji.12090
31. Than NG, Romero R, Balogh A, Karpati E, Mastrolia SA, Staretz-Chacham O, et al. Galectins: Double-edged swords in the cross-roads of pregnancy complications and female reproductive tract inflammation and neoplasia. J Pathol Transl Med (2015) 49(3):181–208. doi: 10.4132/jptm.2015.02.25
32. Ahmed H, Du SJ, O'Leary N, Vasta GR. Biochemical and molecular characterization of galectins from zebrafish (Danio rerio): notochord-specific expression of a prototype galectin during early embryogenesis. Glycobiology (2004) 14(3):219–32. doi: 10.1093/glycob/cwh032
33. Balogh A, Toth E, Romero R, Parej K, Csala D, Szenasi NL, et al. Placental galectins are key players in regulating the maternal adaptive immune response. Front Immunol (2019) 10:1240. doi: 10.3389/fimmu.2019.01240
34. Jovanovic Krivokuca M, Vilotic A, Nacka-Aleksic M, Pirkovic A, Cujic D, Legner J, et al. Galectins in early pregnancy and pregnancy-associated pathologies. Int J Mol Sci (2021) 23(1):69. doi: 10.3390/ijms23010069
35. Menkhorst E, Than NG, Jeschke U, Barrientos G, Szereday L, Dveksler G, et al. Medawar's PostEra: Galectins emerged as key players during fetal-maternal glycoimmune adaptation. Front Immunol (2021) 12:784473. doi: 10.3389/fimmu.2021.784473
36. Banfer S, Jacob R. Galectins in intra- and extracellular vesicles. Biomolecules (2020) 10(9):1232. doi: 10.3390/biom10091232
37. Camby I, Le Mercier M, Lefranc F, Kiss R. Galectin-1: a small protein with major functions. Glycobiology (2006) 16(11):137R–57R. doi: 10.1093/glycob/cwl025
38. Landén NX, Li D, Ståhle M. Transition from inflammation to proliferation: a critical step during wound healing. Cell Mol Life Sci (2016) 73(20):3861–85. doi: 10.1007/s00018-016-2268-0
39. Reinke JM, Sorg H. Wound repair and regeneration. Eur Surg Res (2012) 49(1):35–43. doi: 10.1159/000339613
40. Sonnemann KJ, Bement WM. Wound repair: toward understanding and integration of single-cell and multicellular wound responses. Annu Rev Cell Dev Biol (2011) 27:237–63. doi: 10.1146/annurev-cellbio-092910-154251
41. Hsu DK, Yang RY, Pan Z, Yu L, Salomon DR, Fung-Leung WP, et al. Targeted disruption of the galectin-3 gene results in attenuated peritoneal inflammatory responses. Am J Pathol (2000) 156(3):1073–83. doi: 10.1016/s0002-9440(10)64975-9
42. Correa SG, Sotomayor CE, Aoki MP, Maldonado CA, Rabinovich GA. Opposite effects of galectin-1 on alternative metabolic pathways of l-arginine in resident, inflammatory, and activated macrophages. Glycobiology (2003) 13(2):119–28. doi: 10.1093/glycob/cwg010
43. Rabinovich GA, Sotomayor CE, Riera CM, Bianco I, Correa SG. Evidence of a role for galectin-1 in acute inflammation. Eur J Immunol (2000) 30(5):1331–9. doi: 10.1002/(sici)1521-4141(200005)30:5<1331::Aid-immu1331>3.0.Co;2-h
44. Barrionuevo P, Beigier-Bompadre M, Ilarregui JM, Toscano MA, Bianco GA, Isturiz MA, et al. A novel function for galectin-1 at the crossroad of innate and adaptive immunity: galectin-1 regulates monocyte/macrophage physiology through a nonapoptotic ERK-dependent pathway. J Immunol (2007) 178(1):436–45. doi: 10.4049/jimmunol.178.1.436
45. Abebayehu D, Spence A, Boyan BD, Schwartz Z, Ryan JJ, McClure MJ. Galectin-1 promotes an M2 macrophage response to polydioxanone scaffolds. J BioMed Mater Res A (2017) 105(9):2562–71. doi: 10.1002/jbm.a.36113
46. Kianoush F, Nematollahi M, Waterfield JD, Brunette DM. Regulation of RAW264.7 macrophage polarization on smooth and rough surface topographies by galectin-3. J BioMed Mater Res A (2017) 105(9):2499–509. doi: 10.1002/jbm.a.36107
47. Jung SH, Hwang JH, Kim SE, Kim YK, Park HC, Lee HT. Human galectin-9 on the porcine cells affects the cytotoxic activity of M1-differentiated THP-1 cells through inducing a shift in M2-differentiated THP-1 cells. Xenotransplantation (2017) 24(4):e12305. doi: 10.1111/xen.12305
48. Zhang W, Zhang Y, Fang Q. Effect of galectin-9/Tim-3 pathway on the polarization of M1/M2 subtype in murine macrophages induced by lipopolysaccharide. Zhonghua Wei Zhong Bing Ji Jiu Yi Xue (2018) 30(9):836–41. doi: 10.3760/cma.j.issn.2095-4352.2018.09.004
49. You JL, Wang W, Tang MY, Ye YH, Liu AX, Zhu YM. A potential role of galectin-1 in promoting mouse trophoblast stem cell differentiation. Mol Cell Endocrinol (2018) 470:228–39. doi: 10.1016/j.mce.2017.11.003
50. He XJ, Tao HQ, Hu ZM, Ma YY, Xu J, Wang HJ, et al. Expression of galectin-1 in carcinoma-associated fibroblasts promotes gastric cancer cell invasion through upregulation of integrin β1. Cancer Sci (2014) 105(11):1402–10. doi: 10.1111/cas.12539
51. Chong Y, Tang D, Gao J, Jiang X, Xu C, Xiong Q, et al. Galectin-1 induces invasion and the epithelial-mesenchymal transition in human gastric cancer cells via non-canonical activation of the hedgehog signaling pathway. Oncotarget (2016) 7(50):83611–26. doi: 10.18632/oncotarget.13201
52. Wang YQ, Liu ZH, Shu J, Tang SE, Luo FY. Galectin3 regulates transforming growth factor-β-induced epithelial-mesenchymal. Zhongguo Yi Xue Ke Xue Yuan Xue Bao (2018) 40(2):219–24. doi: 10.3881/j.issn.1000-503X.2018.02.012
53. You X, Wu J, Zhao X, Jiang X, Tao W, Chen Z, et al. Fibroblastic galectin-1-fostered invasion and metastasis are mediated by TGF-β1-induced epithelial-mesenchymal transition in gastric cancer. Aging (Albany NY) (2021) 13(14):18464–81. doi: 10.18632/aging.203295
54. Zhu J, Zheng Y, Zhang H, Liu Y, Sun H, Zhang P. Galectin-1 induces metastasis and epithelial-mesenchymal transition (EMT) in human ovarian cancer cells via activation of the MAPK JNK/p38 signalling pathway. Am J Transl Res (2019) 11(6):3862–78.
55. Tsai CH, Tzeng SF, Chao TK, Tsai CY, Yang YC, Lee MT, et al. Metastatic progression of prostate cancer is mediated by autonomous binding of galectin-4-o-glycan to cancer cells. Cancer Res (2016) 76(19):5756–67. doi: 10.1158/0008-5472.Can-16-0641
56. Su YL, Luo HL, Huang CC, Liu TT, Huang EY, Sung MT, et al. Galectin-1 overexpression activates the fak/pi3k/akt/mtor pathway and is correlated with upper urinary urothelial carcinoma progression and survival. Cells (2020) 9(4):806. doi: 10.3390/cells9040806
57. Orozco CA, Martinez-Bosch N, Guerrero PE, Vinaixa J, Dalotto-Moreno T, Iglesias M, et al. Targeting galectin-1 inhibits pancreatic cancer progression by modulating tumor-stroma crosstalk. Proc Natl Acad Sci U.S.A. (2018) 115(16):E3769–e3778. doi: 10.1073/pnas.1722434115
58. Zhao B, Liu JQ, Zheng Z, Zhang J, Wang SY, Han SC, et al. Human amniotic epithelial stem cells promote wound healing by facilitating migration and proliferation of keratinocytes via ERK, JNK and AKT signaling pathways. Cell Tissue Res (2016) 365(1):85–99. doi: 10.1007/s00441-016-2366-1
59. Pogozhykh O, Hofmann N, Gryshkov O, von Kaisenberg C, Mueller M, Glasmacher B, et al. Repeated freezing procedures preserve structural and functional properties of amniotic membrane for application in ophthalmology. Int J Mol Sci (2020) 21(11)4029. doi: 10.3390/ijms21114029
60. Robinson BS, Saeedi B, Arthur CM, Owens J, Naudin C, Ahmed N, et al. Galectin-9 is a novel regulator of epithelial restitution. Am J Pathol (2020) 190(8):1657–66. doi: 10.1016/j.ajpath.2020.04.010
61. Tang D, Gao J, Wang S, Ye N, Chong Y, Huang Y, et al. Cancer-associated fibroblasts promote angiogenesis in gastric cancer through galectin-1 expression. Tumour Biol (2016) 37(2):1889–99. doi: 10.1007/s13277-015-3942-9
62. Chen HL, Chiang PC, Lo CH, Lo YH, Hsu DK, Chen HY, et al. Galectin-7 regulates keratinocyte proliferation and differentiation through JNK-miR-203-p63 signaling. J Invest Dermatol (2016) 136(1):182–91. doi: 10.1038/jid.2015.366
63. Cao N, Tang X, Gao R, Kong L, Zhang J, Qin W, et al. Galectin-3 participates in PASMC migration and proliferation by interacting with TGF-β1. Life Sci (2021) 274:119347. doi: 10.1016/j.lfs.2021.119347
64. Girotti MR, Salatino M, Dalotto-Moreno T, Rabinovich GA. Sweetening the hallmarks of cancer: Galectins as multifunctional mediators of tumor progression. J Exp Med (2020) 217(2):e20182041. doi: 10.1084/jem.20182041
65. Nguyen MN, Ziemann M, Kiriazis H, Su Y, Thomas Z, Lu Q, et al. Galectin-3 deficiency ameliorates fibrosis and remodeling in dilated cardiomyopathy mice with enhanced Mst1 signaling. Am J Physiol Heart Circ Physiol (2019) 316(1):H45–h60. doi: 10.1152/ajpheart.00609.2018
66. Hsu YA, Chang CY, Lan JL, Li JP, Lin HJ, Chen CS, et al. Amelioration of bleomycin-induced pulmonary fibrosis via TGF-β-induced smad and non-smad signaling pathways in galectin-9-deficient mice and fibroblast cells. J BioMed Sci (2020) 27(1):24. doi: 10.1186/s12929-020-0616-8
67. Smith PC, Metz C, de la Peña A, Oyanadel C, Avila P, Arancibia R, et al. Galectin-8 mediates fibrogenesis induced by cyclosporine in human gingival fibroblasts. J Periodontal Res (2020) 55(5):724–33. doi: 10.1111/jre.12761
68. Singer AJ, Clark RA. Cutaneous wound healing. N Engl J Med (1999) 341(10):738–46. doi: 10.1056/nejm199909023411006
69. Zhang Z, Miller MC, Xu X, Song C, Zhang F, Zheng Y, et al. NMR-based insight into galectin-3 binding to endothelial cell adhesion molecule CD146: Evidence for noncanonical interactions with the lectin's CRD β-sandwich f-face. Glycobiology (2019) 29(8):608–18. doi: 10.1093/glycob/cwz036
70. Bojić-Trbojević Ž, Jovanović Krivokuća M, Vilotić A, Kolundžić N, Stefanoska I, Zetterberg F, et al. Human trophoblast requires galectin-3 for cell migration and invasion. Sci Rep (2019) 9(1):2136. doi: 10.1038/s41598-018-38374-w
71. Fulcher JA, Hashimi ST, Levroney EL, Pang M, Gurney KB, Baum LG, et al. Galectin-1-matured human monocyte-derived dendritic cells have enhanced migration through extracellular matrix. J Immunol (2006) 177(1):216–26. doi: 10.4049/jimmunol.177.1.216
72. Wang M, Xu Y, Wang P, Xu Y, Jin P, Wu Z, et al. Galectin-14 promotes trophoblast migration and invasion by upregulating the expression of mmp-9 and n-cadherin. Front Cell Dev Biol (2021) 9:645658. doi: 10.3389/fcell.2021.645658
73. Richardson LS, Taylor RN, Menon R. Reversible EMT and MET mediate amnion remodeling during pregnancy and labor. Sci Signal (2020) 13(618):eaay1486. doi: 10.1126/scisignal.aay1486
74. Cao Z, Said N, Amin S, Wu HK, Bruce A, Garate M, et al. Galectins-3 and -7, but not galectin-1, play a role in re-epithelialization of wounds. J Biol Chem (2002) 277(44):42299–305. doi: 10.1074/jbc.M200981200
75. Liu W, Hsu DK, Chen HY, Yang RY, Carraway KL, Isseroff RR, et al. Galectin-3 regulates intracellular trafficking of EGFR through alix and promotes keratinocyte migration. J Invest Dermatol (2012) 132(12):2828–37. doi: 10.1038/jid.2012.211
76. Lagana A, Goetz JG, Cheung P, Raz A, Dennis JW, Nabi IR. Galectin binding to Mgat5-modified n-glycans regulates fibronectin matrix remodeling in tumor cells. Mol Cell Biol (2006) 26(8):3181–93. doi: 10.1128/mcb.26.8.3181-3193.2006
Keywords: amniotic epithelial cells, amniotic mesenchymal cells, galectin, macrophages, fetal membrane, wound healing
Citation: Chen J-L, Chen Y, Xu D-X and Chen D-Z (2022) Possible important roles of galectins in the healing of human fetal membranes. Front. Endocrinol. 13:941029. doi: 10.3389/fendo.2022.941029
Received: 11 May 2022; Accepted: 14 July 2022;
Published: 09 August 2022.
Edited by:
Lotte Elisabeth Van Der Meeren, Leiden University Medical Center, NetherlandsCopyright © 2022 Chen, Chen, Xu and Chen. This is an open-access article distributed under the terms of the Creative Commons Attribution License (CC BY). The use, distribution or reproduction in other forums is permitted, provided the original author(s) and the copyright owner(s) are credited and that the original publication in this journal is cited, in accordance with accepted academic practice. No use, distribution or reproduction is permitted which does not comply with these terms.
*Correspondence: Dao-Zhen Chen, Y2hlbmRhb3poZW5AMTYzLmNvbQ==; De-Xiang Xu, eHVkZXhAMTI2LmNvbQ==
†These authors have contributed equally to this work
Disclaimer: All claims expressed in this article are solely those of the authors and do not necessarily represent those of their affiliated organizations, or those of the publisher, the editors and the reviewers. Any product that may be evaluated in this article or claim that may be made by its manufacturer is not guaranteed or endorsed by the publisher.
Research integrity at Frontiers
Learn more about the work of our research integrity team to safeguard the quality of each article we publish.