- College of Science, Institute of Biology, University of the Philippines, Diliman, Quezon City, Philippines
Sexual dimorphisms can be seen in many organisms with some exhibiting subtle differences while some can be very evident. The difference between male and female can be seen on the morphological level such as discrepancies in body mass, presence of body hair in distinct places, or through the presence of specific reproductive structures. It is known that the development of the reproductive structures is governed by hormone signaling, most commonly explained through the actions of androgen signaling. The developmental program of the male and female external genitalia involves a common anlage, the genital tubercle or GT, that later on develop into a penis and clitoris, respectively. Androgen signaling involvement can be seen in the different tissues in the GT that express Androgen receptor and the different genes that are regulated by androgen in the mesenchyme and endoderm component of the GT. Muscles are also known to be responsive to androgen signaling with male and female muscles exhibiting different capabilities. However, the occurrence of sexual dimorphism in muscle development is unclear. In this minireview, a summary on the role of androgen in the sexually dimorphic development of the genital tubercle was provided. This was used as a framework on analyzing the different mechanism employed by androgen signaling to regulate the sexual dimorphism in muscle development.
Introduction
Biological differences are evident in extant species to date, accounting for great diversity in physiology, reproductive strategies, anatomy, and morphology. Initially, dimorphic organs have similar precursor structures that undergo the same early developmental program which includes mitotic growth and cellular differentiation. This will then be followed by a stage of divergent developmental process which usually involves sex hormones, androgens and estrogens, which leads to sexual dimorphism. This sexual dimorphism is important in establishing male- and female-specific organs to ensure greater reproductive success. Among the apparent manifestations of sexual dimorphism can be determined morphologically in terms of size, shape, colors, and development of appendages (1). In many mammalian species such as elephants, sea lions, and kangaroos, adult males are larger than females. Neck crests are also prominent in male bulls and stallions due to greater muscle build-up in the head, neck, and shoulder regions as compared to their female counterparts and has been attributed to androgen-facilitated muscle deposition patterns (2). However, the developmental program underlying sexual dimorphisms are not yet fully understood.
One of the most notable sexually dimorphic structures in mice are the features of the external genitalia (ExG) and the anogenital distance (AGD) in the perineum, area between the anus and genitals (3). Male mice have longer and more pronounced ExG and AGD as compared to female mice. Some distinguishing features of the ExG between the males and females include the presence of erectile tissue (diffused in clitoris), organ size (larger for the penis), bone (larger for the penis), cartilage (absent in the clitoris), surface spines (absent in the clitoris), urethral position (completely within the penis for males), and cross-sectional shape (U-shaped for the clitoris and circular for the penis) (4). The penis and clitoris of the ExG both develop from the ambisexual genital tubercle (GT), from embryonic day 11 or E 11, and become sex-specific at E 16 in mice (5). The longer AGD is used in sexing mice pups as early as E16.5. The perineum region is longer in male mice which may be attributed to the presence of well-developed bulbocavernosus/levator ani (BC/LA) muscle complex (3).
Androgen signaling has been identified as the primary factor that establishes male characteristics. The ligands for androgen signaling are testosterone (T) and dihydrotestosterone (DHT). T is the primary circulating androgen that is secreted by the Leydig cells of the testis while DHT is a product of the conversion of T via 5a-reductase in several reproductive tissues (6). The androgen receptor (Ar) is composed of different functional domains: N-terminal domain, DNA-binding domain, hinge region and C-terminal or ligand binding domain (7). Androgen signaling is initiated by the binding of androgens, (T) or dihydrotestosterone (DHT) to androgen receptor resulting to the dimerization of Ar and its nuclear translocation. The Ar can bind to the DNA of target genes through androgen response elements (ARE) (8). Ar is known to regulate transcription of different genes and more recently, non-genomic functions of AR have been evident (ADD 2 here).
The developmental program of the ExG formation has been explained through the dominant effect of androgen signaling. Muscle development and physiology is known to be responsive to the effect of testosterone. The understanding of the masculinization process of the external genitalia and AGD might help in identifying the occurrence of sexually dimorphic muscle development.
The study of sexual dimorphism in the external genitalia
The development of the ExG starts with a common anlage known as the genital tubercle or GT (Figure 1) which will later develop into the penis and clitoris. The GT is composed of different tissues originating from the ectoderm, endoderm, and mesoderm. The epidermis of the GT is mainly comprised of ectodermal cells while the urethral tube is of endodermal origin. The mesenchyme of the GT is derived from the pericloacal mesenchyme which is primarily from the mesoderm. Each of these tissue types contribute to the development of the GT and towards a sexual dimorphic structure of a penis or clitoris. One of the most notable structure that distinguish the male and female GT is the fusion of urethral folds. Failure of urethral folds fusion leads to hypospadias, a human penile condition wherein the urethral opening is not located at the tip of the penis. This condition can be replicated in mice when androgen signaling is disrupted during the stage of urethral tube fusion (9, 10). Additionally, exclusive dysregulation of signaling in the ectoderm, endoderm,or mesenchyme exhibits a hypospadias-like phenotype, in varying degree, due to the failure in the proper fusion of the urethral folds (10–13). This shows that each of this tissue contributes to GT masculinization.
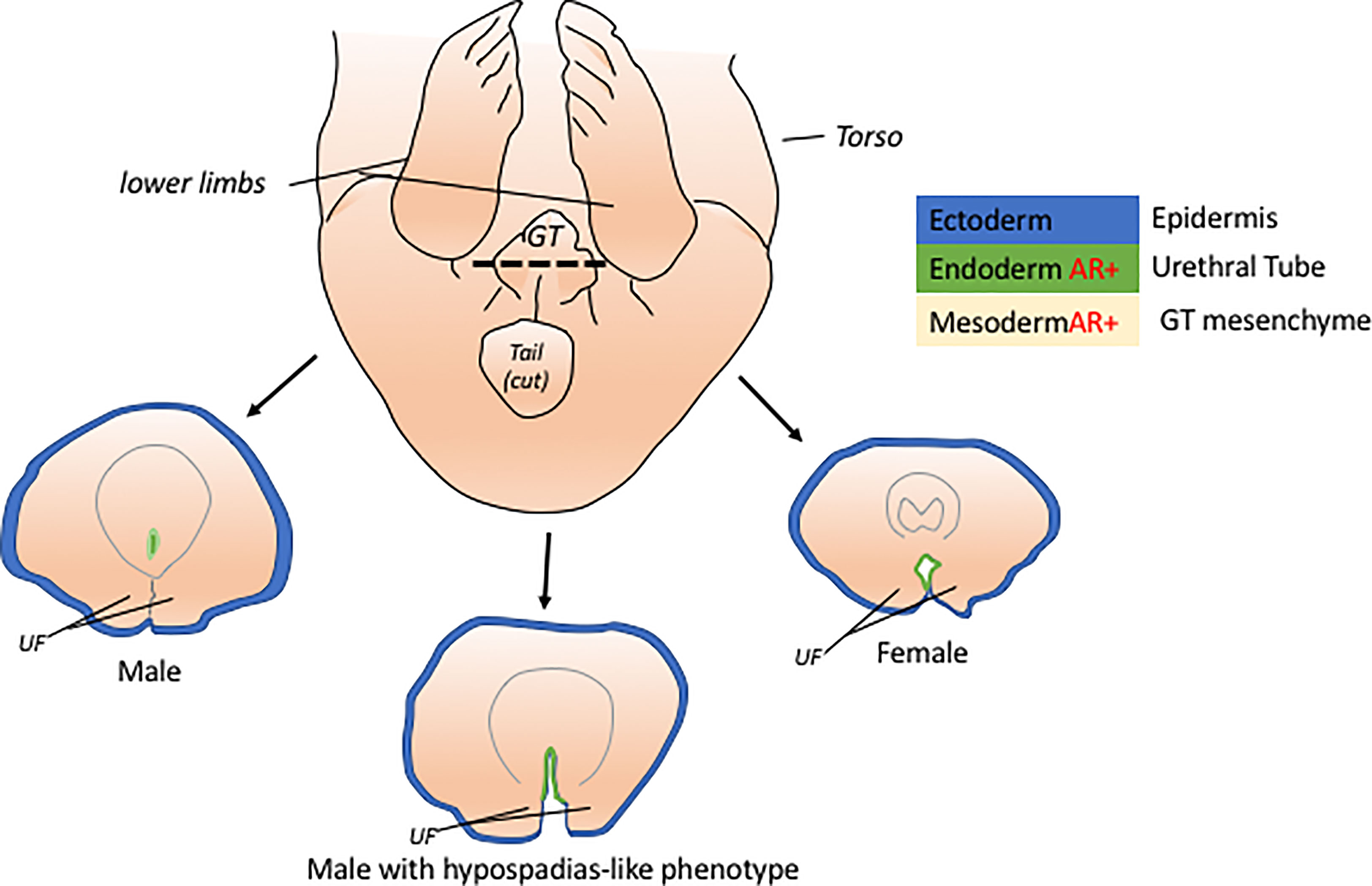
Figure 1 The genital tubercle or GT is the common anlage of the penis and clitoris. The GT has cells from 3 embryonic layers, ectoderm, mesoderm, and endoderm. At early embryonic stage, the male GT is distinguished from female GT through the fusion of the urethral folds (UF) which can be viewed histologically through a cross-section of the GT (dotted line). The masculinization of the male GT is attributed to the active androgen signaling in the endoderm and mesoderm tissues of the GT. Unfused UF in males, hypospadias-like phenotype, are due to disruptions in the signaling of the endoderm and mesoderm of the GT.
Androgen signaling has been a consistent variable in the study of a masculinized GT. Androgen receptor (AR) expression is observed in the mesenchyme of the urethral folds and the urethral tube while its expression in the ectoderm is not detected (10, 12). Only the mesenchyme -specific knock-out (KO) of AR results to hypoplasia of the urethral folds. This indicates that the influence of androgen signaling during male-specific GT development may depend on which AR-expressing tissue is most important in the masculinization process (ADD 10 or 12). A microarray analysis comparing the GT ventral mesenchyme and urethra of male and female shows some genes that are differentially expressed at the onset of sexually dimorphic features. Cyp1b1, Fkbp51 (FK506 binding protein 5) and Mafb (v-maf masculoaponeurotic fibrosarcoma oncogene family protein B) are differentially expressed in male and female GTs (14). Mafb is more prominently expressed in the male GT mesenchyme than in females. Additionally, exogenous exposure to androgen induced Mafb expression in females while ArKO (Androgen receptor knockout) mice downregulated its expression. Interestingly, androgen exposure in the absence of Mafb resulted to failed masculinization of the urethra and Mafb KO mice exhibited defective urethra which is similar to hypospadias (10). This implies that androgen is essential in inducing and regulating Mafb action in the masculinization of the urethral folds. Another signaling pathway that is influenced by androgen signaling is the Wnt signaling. Beta-catenin (B-cat), a downstream effector of Wnt-signaling, is found to be highly expressed in the male ventral mesenchyme. Such expression pattern can be replicated in the female through the administration of testosterone propionate, a testosterone analogue. KO of Ar and B-cat in the urethral ventral mesenchyme both leads to hypospadias-like phenotype (12).
From the studies of GT development mentioned above, there are some investigative strategies that can be used as a framework in identifying sexually dimorphic muscle formation. First, it is important to identify which cell or tissues are necessary for the proper sexually dimorphic development of tissues or organs. And also, which of these tissues express Ar and which tissue-specific Ar deletion results to drastic changes in phenotype. Second, all the above-mentioned studies include comparison between male and female structures and differential gene expression patterns. This method is necessary to elucidate which developmental mechanism is necessary for general development of structures and which is necessary for male- or female- specific developmental program. It is important that identified mechanism may be used to manipulate the female GT development which will result to structures or expression pattern that will resemble that of the male, or vice versa. And lastly, genes that are differentially expressed between male and females need to be validated on how androgen signaling might influence its expression pattern. This can be done by using different hormonal modulation experiments (see Diff review paper) and analyze how gene expression patterns are affected.
Muscle development
Muscle development starts with the specification of a somite region known as dermomyotome which leads to primary myotome formation. The myotome contains muscle cells or myoblasts that migrate to different parts of the body to serve as muscle precursor cells for the rib and back muscles, body walls, limbs and tongue. This process occurs between E9 – E12 in mice (15). During this time, the development of the muscles is not observed to be androgen-dependent nor is it different between male and female embryos.
The regulation of myogenesis is dependent on the expression of members of the basic helix-loop-helix domain-containing myogenic regulatory factors (MRFs) (Figure 2A). MRFs include myogenic factor 5 (Myf5) and 6 (Myf6), myogenic differentiation 1 (MyoD), and myogenin (Myog) (16). Myf5 and MyoD are suggested to act redundantly and upstream of both Myf6 and Myog. Myf5 mutants exhibit initial delayed muscle development which was rescued once MyoD begins to be expressed resulting in an overall normal myogenesis (17, 18). Similarly, MyoD mutants possess normal skeletal muscle development with a notable persistent expression of Myf5, which is normally down-regulated after day 14 of gestation (18). This suggests that some functional redundancy between MyoD and Myf5 exists. Myog null mutation results in normal initiation of myogenesis but defects in myocyte differentiation and myotube formation were observed (19, 20). These MRFs act downstream of the paired domain and homeobox-containing transcription factors paired box gene 3 (Pax3) and 7 (Pax7) (21, 22). Pax3 mutants display failure in the proper specification of the dermamyotome and myotome compartments of the somite which may lead to defective body wall musculature development (23). Pax 7 could substitute for some of the function of Pax3 in somite development but not the functions on myogenic specification (24). The primary role of Pax7 is suggested to be implicated with the specification of myogenic satellite cells (22). The satellite cells contribute to the development, growth and repair of muscles. During development and muscle regeneration, the satellite cells are activated and start to express MyoD (Figure 2A). Activated satellite cells become the myoblasts and undergo proliferation. Post-mitotic myoblasts will further differentiate and express Myog or Myf6. These transcription factors will regulate the terminal differentiation of myocytes and myotube formation (25, 26). The regulatory mechanism of myogenesis is considered as one of the active areas of research and more findings are being accumulated that could specify the exact hierarchy and players during muscle development.
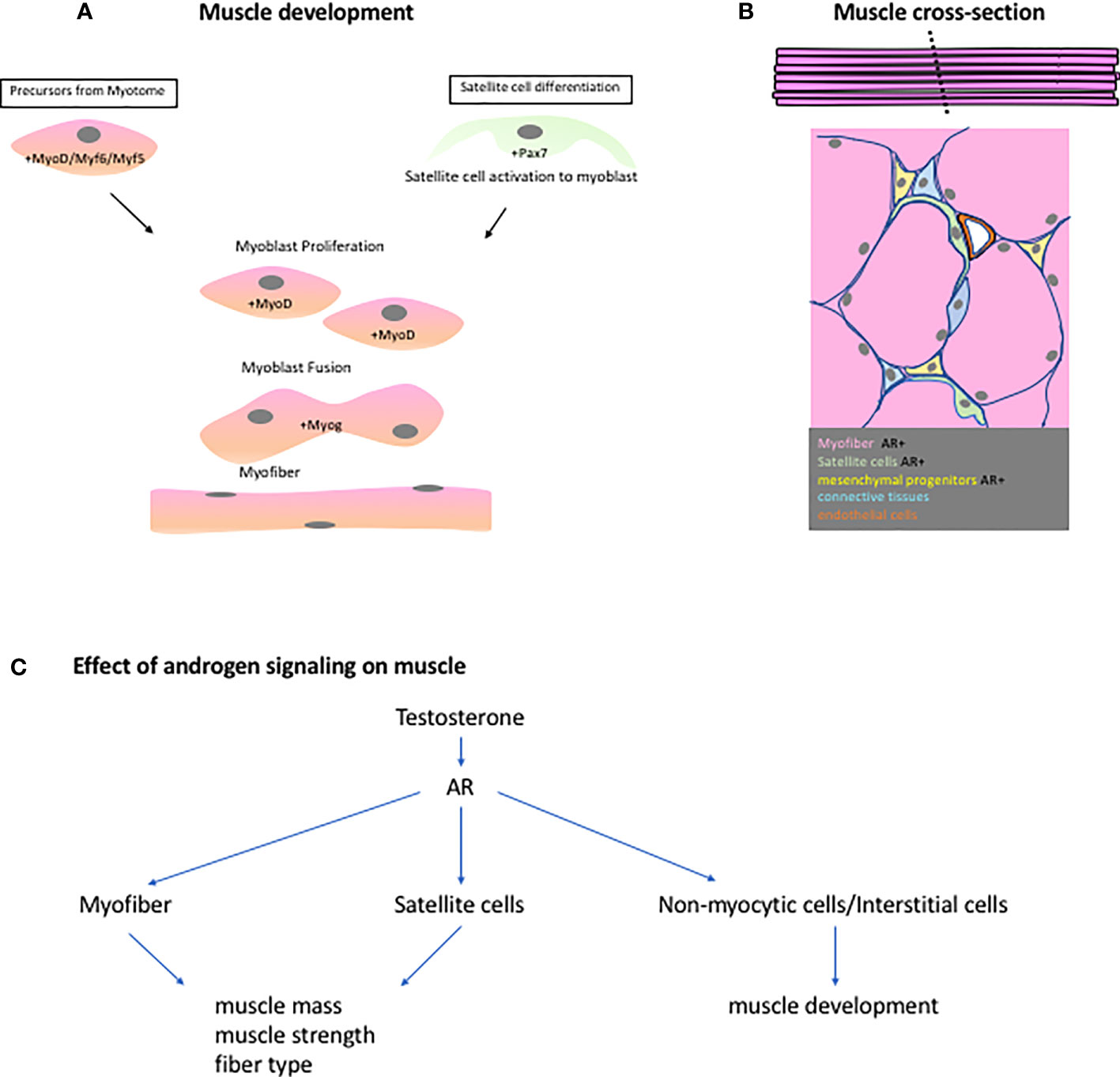
Figure 2 (A) Muscle embryonic development is highly regulated by the MRFs (Myf5, Myf6,MyoD and Myog). Precusor myoblast from the myotome travel to different parts of the body to establish the different muscle groups. These precursors or myoblast, expressing MyoD, will proliferate, fuse, and differentiate to form syncytial myofibers. During regeneration, the resident stem cells of the muscles, satellite cells, are activated and begin to express MyoD. It will then follow the same steps as in the embryonic muscle development. (B) A cross-section of the muscle shows the different cell population found in the muscles. Some of these cells express AR and are instrumental to the androgen-responsive nature of some muscle groups. (C) A scheme showing the effects of androgen signaling based on ArKO studies on different muscle groups.
Androgen effects on muscle development
The most recognized effect of androgens on muscles is studied through physiological changes on athletes taking testosterone (T) and other related steroids. It was discovered that intramuscular injection of T results in an increase in the net protein synthesis followed by enlargement of the muscle fibers (27). The mechanism of hypertrophy is explained through the concepts of nuclear domain and ceiling theory. A muscle fiber contains numerous myonuclei that sustain protein synthesis over a finite number of cytoplasm (nuclear domain). Upon T treatment, the protein synthesis becomes too high to be sustained by one myonuclei (ceiling theory) hence promoting myonuclear accretion. This process will create an enlargement of the muscle fiber leading to muscle hypertrophy (28–30). This could possibly be attributed to the ability of testosterone, through the androgen receptor, to increase the expression of Myog and mTOR and decrease the expression of myostatin, a negative regulator of muscle mass (31). Additionally, it has been reported that androgen can regulate the expression of polyamine biosynthesis genes such as Odc1 and Amd1. This may also mediate the anabolic actions of androgens on muscle mass (32).
To understand the effect of androgen on the sexual dimorphism in muscle development, the points discussed in studying sexual dimorphism in GT can be used as perspective for summarizing the role of androgen on muscles. First is to identify the type of tissues that are necessary for muscle development and how Ar is expressed and utilized in those cells. A summary of this can be seen in Supplementary Table 1. The effect of androgen signaling is very diverse since the muscle cell population is also composed of different types of cells (Figure 2B, C). A cross section of a skeletal muscle will reveal the presence of different cell such as myofibers, satellite cells, connective tissues, pericytes, endothelial cells, and other mesenchymal cells.
Satellite cells (SC) are the resident stem cells of the muscles and testosterone treatment results in the increase of SC number in humans, rats and pigs leading to muscle hyperthrophy (33–36). SC are described as targets of androgen actions since Ar expression is evident on SC in the LA muscle (37). In a study using depletion of satellite cells and testosterone treatment, it was discovered that muscle hypertrophy occurred in testosterone-treated female mice regardless if the mice were depleted with SC (Pax7CreER/CreER+;Rosa26DTA/DTA) or with the normal number of SC (38). This was further confirmed when satellite cell-specific AR KO (Pax7CreERT2+;ARflox)female mice exhibited increased mass upon testosterone treatment (39). In the same study, a myofiber-specific ArKO or mArKO (Arflox/flox;HSA-Cre) was observed to have increased muscle mass upon testosterone treatment while there was a loss in grip strength. This indicates that muscle mass is not controlled by AR in the myofiber or satellite cell but muscle strength is directly affected by androgen signaling. An RNA seq of the mARKO suggests that glucose/amino acid metabolism and muscle sarcomeric gene regulation, such as Mylk4, is regulated by androgen signaling (39).
Aside from satellite cells, a population of uncommitted progenitor cells of mesenchymal (40) and adipocytes origin (41) acts as reservoir of satellite cells during muscle regeneration or hypertrophy. Ar deletion targeted to mesenchymal stem cells resulted in upreglation of key adipogenic transcription factors such as Peroxisome proliferator-activated receptor gamma (PPARγ) and CCAAT/enhancer binding protein alpha (C/EBPα) (42). Additionally, testosterone treatment of CH3 10T1/2 cells result in the upregulation of myogenic differentiation markers such as MyoD and myosin heavy chain II (MyHC) and downregulation of markers for adipogenic differentiation such as PPARγ and C/EBPα (43). These studies suggest that androgen can shift the commitment of cells from adipocytes or vascular fate to myogenic cells (44). Other cells found in the interstitial compartment such as connective tissues may play a role in regulation of muscle development. A ligament known as gubernaculum which connects the gonad to the future inguinal canal is known to work with the cremaster muscle in the proper descent of the testis. Gubernaculum-specific Ar deletion resulted in increased Pax7 expression and decreased expression of skeletal muscle actin suggesting defective development of the cremaster muscle. However, cremaster muscle-specific Ar deletion did not show any observable phenotypes. The authors suggested that the deficiency in AR results in the loss of Ar-induced paracrine signals from the gubernacular cells to the myoblasts (45).
Interestingly, another non-myocytic cell is found to be necessary for the sexually dimorphic development of muscles in the perineum, the BC/LA muscle. The BC is a striated muscle complex inserted into the urethral bulb or bulb of the penis (46, 47) which possesses a posterior extension which is the LA. The functions of these muscles in mouse have not yet been completely studied but equivalent structures in human are suggested to be involved for sustaining erection (BC) and defecation (LA) (48–51). BC/LA complex is known to be sensitive to androgen signaling (52) which is evident in the table, BC/LA is the muscle complex that is mostly affected by muscle-specific AR deletion. A study on the embryonic development of the BC revealed that the Ar expressed in the mesenchyme surrounding the BC muscle cells are more important for masculinized BC and not the Ar in the myoblast (53). The study mentioned a possible paracrine regulation from non-myocytic cells to myoblast that controls proliferation of myoblast leading to a bigger BC in male. Such well-developed BC in male was feminized after mesenchyme-specific Ar deletion.
The second point in studying the sexually dimorphic organ is to identify the inherent difference between male and female muscle development. There are a few studies that compare the development of muscles between male and female or even to compare the difference in muscle markers or gene players in the normal development of sexually dimorphic muscles. The formation of BC and LA are more prominent in male than in female and ArKO mouse exhibits defective formation in such muscles (46, 51). A study discovered that there are more satellite cells in male LA as compared to female and prenatal exposure of female to exogenous testosterone analogue resulted in the increase of satellite cells and increased LA muscle mass. Additionally, a study observed that the formation of BC in male and female was similar until E.16.5 when the myoblast number in males become more prominent than in the female. ArKO in the mesenchyme of the BC resulted into feminized BC muscles (53). In a later stage (E17), the BC/LA of the female undergoes higher apoptosis rate which leads to an underdeveloped perineum muscle in female (51). These studies show that examining development of BC/LA muscles at embryonic stage,while comparing male and female, can provide a clear picture on the role of androgen in the differential muscle mass of male and female BC/LA.
The male and female difference in the development of other muscle groups has not been studied at embryonic stages or whether a sensitive time window of the sexually dimorphism of muscle exists. Many publications show the control of androgen on varying processes of myogenesis (CITE here) but not on the inherent difference between male and female muscle properties. There are some reports of differences in myogenic properties, and its dependency on androgen signaling between males and females that were observed in adult mice. It was identified that global-KO of Ar affected only the muscle mass of male mice and not that of the female. A reduction in the muscle mass of the tibialis anterior (TA) extensor digitorum longus (EDL), gastronomicus (GAST), and soleus (SOL) was evident in male ArKO mice and the same muscles were unaffected in female ARKO (54). Furthermore, muscle contractile strength was also reduced in the EDL of male ARKO, becoming more similar to that of the female. There are studies suggesting that the type of fibers between male and females are inherently different from each other. Muscle fiber types is distinguished by the (MyHC) expressed in the fiber and is correlated with the morphology and abundance of glycolytic enzymes. This translates into contractile velocity of the muscles with type IIB having a higher relative velocity than IIA (55) (Resnicow 2010). In mouse masseter, or muscles of mastication, the female has more Type IIA fibers (expressing MyHC-IIA) while male exhibit more type IIB fibers. The expression of MyHC-IIA is higher in males in the SOL and TA but in the plantaris, IIA is higher in females than in males (56). Another study reported that Mylk4 is differentially expressed in fast-type muscles of male and female mice. Mylk4 is a gene implicated with increased muscle strength and it was found to be more expressed in male fat-type muscles compared to female. Additionally, Mylk4 expression can be stimulated by androgen treatment (39).
The third perspective on GT development, confirm the androgen-responsive nature of the genes that were found to be inherently different between male and females, is more difficult to be applied in this review. There are numerous studies measuring the androgen responsiveness of varying key players on myogenesis and muscle physiology,which can be found elsewhere (57–59). These reports are extremely helpful in understanding the possible use of androgens on muscle dystrophy and muscle regeneration. These may be used as initial candidates in understanding the inherent difference in male and female muscles. This will require examining the role of these genes or processes in the natural setting of male and female muscle development and growth.
Conclusion
In terms of musculature, are males and females created equal? The investigation on the sexual dimorphism of the muscles could utilize the perspectives used in discovering the formation of a gender-specific ExG. It would be helpful to identify what are the unique properties of male and female muscles and identify whether these properties are responsive to androgen. It is also important to recognize the different tissues or cells involved in the development and/or physiology of muscles. Each cell type might contribute to the muscle growth through autocrine and paracrine fashion. However, the muscle, as an organ, has its own complexity different from the ExG. Muscles have different muscle fiber types, with each muscle complex comprised of different ratio of such fiber types. These contributes to the differential androgen responsiveness/dependency of numerous muscle groups. Unlike the ExG, muscles can regenerate and such process, its fruition and implementation, adds another vantage point in understanding androgen`s influence. Muscles has many other properties that goes beyond structure such as muscle strength, contractile velocity, and endurance which cannot be easily distinguished as dimorphic or not. This makes the study of muscle development dynamic and,dare I say, exciting.
Author contributions
The author confirms being the sole contributor of this work and has approved it for publication.
Conflict of interest
The author declares that the research was conducted in the absence of any commercial or financial relationships that could be construed as a potential conflict of interest.
Publisher’s note
All claims expressed in this article are solely those of the authors and do not necessarily represent those of their affiliated organizations, or those of the publisher, the editors and the reviewers. Any product that may be evaluated in this article, or claim that may be made by its manufacturer, is not guaranteed or endorsed by the publisher.
Supplementary material
The Supplementary Material for this article can be found online at: https://www.frontiersin.org/articles/10.3389/fendo.2022.940229/full#supplementary-material
Supplementary Table 1 | A summary of studies detailing the effect of androgen on muscle as mediated by different cell population found in the muscles.
References
1. Ralls K, Mesnick S. Sexual dimorphisms. In: Perrin W, Bernd W, Thewissen JGM, editors. Encyclopedia of marine mammals, 2nd ed. Cambridge, Massachusetts: Academic Press (2009). p. 1005–11.
2. McPherson FJ, Chenoweth PJ. Mammalian sexual dimorphism. Anim Reprod Sci (2012) 131(3-4):109–22. doi: 10.1016/j.anireprosci.2012.02.007
3. Ipulan LA, Suzuki K, Matsushita S, Suzuki H, Okazawa M, Jacinto S, et al. Development of the external genitalia and their sexual dimorphic regulation in mice. Sex Dev (2014) 8(5):297–310. doi: 10.1159/000357932
4. Weiss DA, Rodriguez E, Cunha T, Menshenina J, Barcellos D, Chan LY, et al. Morphology of the external genitalia of the adult Male and female mice as an endpoint of sex differentiation. Mol Cell Endocrinol (2012) 354(1-2):94–102. doi: 10.1016/j.mce.2011.08.009
5. Yamada G, Satoh Y, Baskin LS, Cunha GR. Cellular and molecular mechanisms of development of the external genitalia. Differentiation (2003) 71(8):445–60. doi: 10.1046/j.1432-0436.2003.7108001.x
6. Mooradian AD, Morley JE, Korenman SG. Biological actions of androgens. Endocr Rev (1987) 8(1):1–28. doi: 10.1210/edrv-8-1-1
7. Evans RM. The steroid and thyroid hormone receptor superfamily. Science (1988) 240(4854):889–95. doi: 10.1126/science.3283939
8. Freedman LP. Anatomy of the steroid receptor zinc finger region. Endocr Rev (1992) 13(2):129–45. doi: 10.1210/edrv-13-2-129
9. Ipulan LA, Raga D, Suzuki K, Murashima A, Matsumaru D, Cunha G, et al. Investigation of sexual dimorphisms through mouse models and Hormone/Hormone-disruptor treatments. Differentiation (2016) 91(4-5):78–89. doi: 10.1016/j.diff.2015.11.001
10. Suzuki K, Numata T, Suzuki H, Raga DD, Ipulan LA, Yokoyama C, et al. Sexually dimorphic expression of mafb regulates masculinization of the embryonic urethral formation. Proc Natl Acad Sci USA (2014) 111(46):16407–12. doi: 10.1073/pnas.1413273111
11. Mazahery AR, Suzuki K, Nagafuchi A, Miyajima M, Nakagata N, Orvis GD, et al. Functional analysis of ectodermal β-catenin during external genitalia formation. Congenit Anom (Kyoto) (2013) 53(1):34–41. doi: 10.1111/cga.12001
12. Miyagawa S, Satoh Y, Haraguchi R, Suzuki K, Iguchi T, Taketo MM, et al. Genetic interactions of the androgen and wnt/beta-catenin pathways for the masculinization of external genitalia. Mol Endocrinol (2009) 23(6):871–80. doi: 10.1210/me.2008-0478
13. Miyagawa S, Moon A, Haraguchi R, Inoue C, Harada M, Nakahara C, et al. Dosage-dependent hedgehog signals integrated with wnt/beta-catenin signaling regulate external genitalia formation as an appendicular program. Development (2009) 136(23):3969–78. doi: 10.1242/dev.039438
14. Nishida H, Miyagawa S, Matsumaru D, Wada Y, Satoh Y, Ogino Y, et al. Gene expression analyses on embryonic external genitalia: Identification of regulatory genes possibly involved in masculinization processes. Congenit Anom (Kyoto) (2008) 48(2):63–7. doi: 10.1111/j.1741-4520.2008.00180.x
15. Braun T, Gautel M. Transcriptional mechanisms regulating skeletal muscle differentiation, growth and homeostasis. Nat Rev Mol Cell Biol (2011) 12(6):349–61. doi: 10.1038/nrm3118
16. Bryson-Richardson RJ, Currie PD. The genetics of vertebrate myogenesis. Nat Rev Genet (2008) 9(8):632–46. doi: 10.1038/nrg2369
17. Braun T, Rudnicki MA, Arnold HH, Jaenisch R. Targeted inactivation of the muscle regulatory gene myf-5 results in abnormal rib development and perinatal death. Cell (1992) 71(3):369–82. doi: 10.1016/0092-8674(92)90507-9
18. Rudnicki MA, Schnegelsberg PN, Stead RH, Braun T, Arnold HH, Jaenisch R. MyoD or myf-5 is required for the formation of skeletal muscle. Cell (1993) 75(7):1351–9. doi: 10.1016/0092-8674(93)90621-V
19. Hasty P, Bradley A, Morris JH, Edmondson DG, Venuti JM, Olson EN, et al. Muscle deficiency and neonatal death in mice with a targeted mutation in the myogenin gene. Nature (1993) 364(6437):501–6. doi: 10.1038/364501a0
20. Nabeshima Y, Hanaoka K, Hayasaka M, Esumi E, Li S, Nonaka I. Myogenin gene disruption results in perinatal lethality because of severe muscle defect. Nature (1993) 364(6437):532–5. doi: 10.1038/364532a0
21. Maroto M, Reshef R, Münsterberg AE, Koester S, Goulding M, Lassar AB. Ectopic pax-3 activates MyoD and myf-5 expression in embryonic mesoderm and neural tissue. Cell (1997) 89(1):139–48. doi: 10.1016/S0092-8674(00)80190-7
22. Seale P, Sabourin LA, Girgis-Gabardo A, Mansouri A, Gruss P, Rudnicki MA. Pax7 is required for the specification of myogenic satellite cells. Cell (2000) 102(6):777–86. doi: 10.1016/S0092-8674(00)00066-0
23. Tremblay P, Dietrich S, Mericskay M, Schubert FR, Li Z, Paulin D. A crucial role for Pax3 in the development of the hypaxial musculature and the long-range migration of muscle precursors. Dev Biol (1998) 203(1):49–61. doi: 10.1006/dbio.1998.9041
24. Relaix F, Rocancourt D, Mansouri A, Buckingham M. Divergent functions of murine Pax3 and Pax7 in limb muscle development. Genes Dev (2004) 18(9):1088–105. doi: 10.1101/gad.301004
25. Chargé SB, Rudnicki MA. Cellular and molecular regulation of muscle regeneration. Physiol Rev (2004) 84(1):209–38. doi: 10.1152/physrev.00019.2003
26. Chen Y, Zajac JD, MacLean HE. Androgen regulation of satellite cell function. J Endocrinol (2005) 186(1):21–31. doi: 10.1677/joe.1.05976
27. Ferrando AA, Tipton KD, Doyle D, Phillips SM, Cortiella J, Wolfe RR. Testosterone injection stimulates net protein synthesis but not tissue amino acid transport. Am J Physiol (1998) 275(5 Pt 1):E864–71. doi: 10.1152/ajpendo.1998.275.5.E864
28. Kadi F, Schjerling P, Andersen LL, Charifi N, Madsen JL, Christensen LR, et al. The effects of heavy resistance training and detraining on satellite cells in human skeletal muscles. J Physiol (2004) 558(Pt 3):1005–12. doi: 10.1113/jphysiol.2004.065904
29. Kadi F, Bonnerud P, Eriksson A, Thornell LE. The expression of androgen receptors in human neck and limb muscles: Effects of training and self-administration of androgenic-anabolic steroids. Histochem Cell Biol (2000) 113(1):25–9. doi: 10.1007/s004180050003
30. Kadi F, Eriksson A, Holmner S, Thornell LE. Effects of anabolic steroids on the muscle cells of strength-trained athletes. Med Sci Sports Exerc (1999) 31(11):1528–34. doi: 10.1097/00005768-199911000-00006
31. Deane CS, Hughes DC, Sculthorpe N, Lewis MP, Stewart CE, Sharples AP. Impaired hypertrophy in myoblasts is improved with testosterone administration. J Steroid Biochem Mol Biol (2013) 138:152–61. doi: 10.1016/j.jsbmb.2013.05.005
32. Lee NK, Skinner JP, Zajac JD, MacLean HE. Ornithine decarboxylase is upregulated by the androgen receptor in skeletal muscle and regulates myoblast proliferation. Am J Physiol Endocrinol Metab (2011) 301(1):E172–9. doi: 10.1152/ajpendo.00094.2011
33. Sinha-Hikim I, Taylor WE, Gonzalez-Cadavid NF, Zheng W, Bhasin S. Androgen receptor in human skeletal muscle and cultured muscle satellite cells: Up-regulation by androgen treatment. J Clin Endocrinol Metab (2004) 89(10):5245–55. doi: 10.1210/jc.2004-0084
34. Joubert Y, Tobin C, Lebart MC. Testosterone-induced masculinization of the rat levator ani muscle during puberty. Dev Biol (1994) 162(1):104–10. doi: 10.1006/dbio.1994.1070
35. Joubert Y, Tobin C. Satellite cell proliferation and increase in the number of myonuclei induced by testosterone in the levator ani muscle of the adult female rat. Dev Biol (1989) 131(2):550–7. doi: 10.1016/S0012-1606(89)80025-9
36. Mulvaney DR, Marple DN, Merkel RA. Proliferation of skeletal muscle satellite cells after castration and administration of testosterone propionate. Proc Soc Exp Biol Med (1988) 188(1):40–5. doi: 10.3181/00379727-188-42704
37. Swift-Gallant A, Monks DA. Androgen receptor expression in satellite cells of the neonatal levator ani of the rat. Dev Neurobiol (2013) 73(6):448–54. doi: 10.1002/dneu.22066
38. Englund DA, Peck BD, Murach KA, Neal AC, Caldwell HA, McCarthy JJ, et al. Resident muscle stem cells are not required for testosterone-induced skeletal muscle hypertrophy. Am J Physiol Cell Physiol (2019) 317(4):C719–C24. doi: 10.1152/ajpcell.00260.2019
39. Sakakibara I, Yanagihara Y, Himori K, Yamada T, Sakai H, Sawada Y, et al. Myofiber androgen receptor increases muscle strength mediated by a skeletal muscle splicing variant of Mylk4. iScience (2021) 24(4):102303. doi: 10.1016/j.isci.2021.102303
40. Grounds MD, White JD, Rosenthal N, Bogoyevitch MA. The role of stem cells in skeletal and cardiac muscle repair. J Histochem Cytochem (2002) 50(5):589–610. doi: 10.1177/002215540205000501
41. Jankowski RJ, Deasy BM, Huard J. Muscle-derived stem cells. Gene Ther (2002) 9(10):642–7. doi: 10.1038/sj.gt.3301719
42. Semirale AA, Zhang XW, Wiren KM. Body composition changes and inhibition of fat development In vivo implicates androgen in regulation of stem cell lineage allocation. J Cell Biochem (2011) 112(7):1773–86. doi: 10.1002/jcb.23098
43. Singh R, Artaza JN, Taylor WE, Gonzalez-Cadavid NF, Bhasin S. Androgens stimulate myogenic differentiation and inhibit adipogenesis in C3H 10T1/2 pluripotent cells through an androgen receptor-mediated pathway. Endocrinology (2003) 144(11):5081–8. doi: 10.1210/en.2003-0741
44. LaBarge MA, Blau HM. Biological progression from adult bone marrow to mononucleate muscle stem cell to multinucleate muscle fiber in response to injury. Cell (2002) 111(4):589–601. doi: 10.1016/S0092-8674(02)01078-4
45. Kaftanovskaya EM, Huang Z, Barbara AM, De Gendt K, Verhoeven G, Gorlov IP, et al. Cryptorchidism in mice with an androgen receptor ablation in gubernaculum testis. Mol Endocrinol (2012) 26(4):598–607. doi: 10.1210/me.2011-1283
46. Yiou R, Delmas V, Carmeliet P, Gherardi RK, Barlovatz-Meimon G, Chopin DK, et al. The pathophysiology of pelvic floor disorders: Evidence from a histomorphologic study of the perineum and a mouse model of rectal prolapse. J Anat (2001) 199(Pt 5):599–607. doi: 10.1046/j.1469-7580.2001.19950599.x
47. Zhang SW, Bai YZ, Zhang SC, Wang DJ, Zhang T, Zhang D, et al. Embryonic development of the striated muscle complex in rats with anorectal malformations. J Pediatr Surg (2008) 43(8):1452–8. doi: 10.1016/j.jpedsurg.2008.02.059
48. Sachs BD. Role of striated penile muscles in penile reflexes, copulation, and induction of pregnancy in the rat. J Reprod Fertil (1982) 66(2):433–43. doi: 10.1530/jrf.0.0660433
49. Karacan I, Aslan C, Hirshkowitz M. Erectile mechanisms in man. Science (1983) 220(4601):1080–2. doi: 10.1126/science.6844930
50. Wallach SJ, Hart BL. The role of the striated penile muscles of the Male rat in seminal plug dislodgement and deposition. Physiol Behav (1983) 31(6):815–21. doi: 10.1016/0031-9384(83)90278-0
51. Jacob DA, Ray T, Bengston CL, Lindsten T, Wu J, Thompson CB, et al. The role of cell death in sexually dimorphic muscle development: Male-specific muscles are retained in female Bax/Bak knockout mice. Dev Neurobiol (2008) 68(11):1303–14. doi: 10.1002/dneu.20658
52. MacLean HE, Chiu WS, Notini AJ, Axell AM, Davey RA, McManus JF, et al. Impaired skeletal muscle development and function in Male, but not female, genomic androgen receptor knockout mice. FASEB J (2008) 22(8):2676–89. doi: 10.1096/fj.08-105726
53. Ipulan LA, Suzuki K, Sakamoto Y, Murashima A, Imai Y, Omori A, et al. Nonmyocytic androgen receptor regulates the sexually dimorphic development of the embryonic bulbocavernosus muscle. Endocrinology (2014) 155(7):2467–79. doi: 10.1210/en.2014-1008
54. Ophoff J, Van Proeyen K, Callewaert F, De Gendt K, De Bock K, Vanden Bosch A, et al. Androgen signaling in myocytes contributes to the maintenance of muscle mass and fiber type regulation but not to muscle strength or fatigue. Endocrinology (2009) 150(8):3558–66. doi: 10.1210/en.2008-1509
55. Resnicow DI, Deacon JC, Warrick HM, Spudich JA, Leinwand LA. Functional diversity among a family of human skeletal muscle myosin motors. Proc Natl Acad Sci U S A (2010) 107(3):1053–8. doi: 10.1073/pnas.0913527107
56. Haizlip KM, Harrison BC, Leinwand LA. Sex-based differences in skeletal muscle kinetics and fiber-type composition. Physiol (Bethesda) (2015) 30(1):30–9. doi: 10.1152/physiol.00024.2014
57. Lee NK, MacLean HE. Polyamines, androgens, and skeletal muscle hypertrophy. J Cell Physiol (2011) 226(6):1453–60. doi: 10.1002/jcp.22569
58. Dubois V, Laurent M, Boonen S, Vanderschueren D, Claessens F. Androgens and skeletal muscle: Cellular and molecular action mechanisms underlying the anabolic actions. Cell Mol Life Sci (2012) 69(10):1651–67. doi: 10.1007/s00018-011-0883-3
Keywords: androgen, muscle, external genitalia, sexual dimorphism, developmental mechanism
Citation: Ipulan-Colet LA (2022) Sexual dimorphism through androgen signaling; from external genitalia to muscles. Front. Endocrinol. 13:940229. doi: 10.3389/fendo.2022.940229
Received: 10 May 2022; Accepted: 28 June 2022;
Published: 27 July 2022.
Edited by:
Rodolfo A. Rey, Hospital de Niños Ricardo Gutiérrez, ArgentinaReviewed by:
Richard R. Behringer, University of Texas MD Anderson Cancer Center Houston, United StatesCopyright © 2022 Ipulan-Colet. This is an open-access article distributed under the terms of the Creative Commons Attribution License (CC BY). The use, distribution or reproduction in other forums is permitted, provided the original author(s) and the copyright owner(s) are credited and that the original publication in this journal is cited, in accordance with accepted academic practice. No use, distribution or reproduction is permitted which does not comply with these terms.
*Correspondence: Lerrie Ann Ipulan-Colet, bGRpcHVsYW5jb2xldEB1cC5lZHUucGg=