- 1Department of Ophthalmology, The Second Affiliated Hospital of Fujian Medical University, Quanzhou, China
- 2The School of Medical Technology and Engineering, Fujian Medical University, Fuzhou, China
- 3Centre of Neurological and Metabolic Research, The Second Affiliated Hospital of Fujian Medical University, Quanzhou, China
- 4Group of Neuroendocrinology, Garvan Institute of Medical Research, Sydney, NSW, Australia
Diabetic retinopathy (DR) is the leakage and obstruction of retinal microvessels caused by chronic progressive diabetes that leads to a series of fundus lesions. If not treated or controlled, it will affect vision and even cause blindness. DR is caused by a variety of factors, and its pathogenesis is complex. Pericyte-related diseases are considered to be an important factor for DR in many pathogeneses, which can lead to DR development through direct or indirect mechanisms, but the specific mechanism remains unclear. Exosomes are small vesicles of 40–100 nm. Most cells can produce exosomes. They mediate intercellular communication by transporting microRNAs (miRNAs), proteins, mRNAs, DNA, or lipids to target cells. In humans, intermittent hypoxia has been reported to alter circulating excretory carriers, increase endothelial cell permeability, and promote dysfunction in vivo. Therefore, we believe that the changes in circulating exocrine secretion caused by hypoxia in DR may be involved in its progress. This article examines the possible roles of miRNAs, proteins, and DNA in DR occurrence and development and discusses their possible mechanisms and therapy. This may help to provide basic proof for the use of exocrine hormones to cure DR.
1 Introduction
Microvascular complications are one of the most common complications of diabetes mellitus. Persistent high blood sugar levels can harm a range of organs, including the heart, kidneys, and retina. The most common complications of diabetes are microvascular complications, particularly diabetic nephropathy (DN) and retinopathy (1). Diabetic retinopathy (DR) is chronic retinopathy that causes leakage and blockage of retinal blood vessels, which can result in a range of fundus lesions, including microangiomas, hard exudates, cotton spots, neovascularization, vitreous hyperplasia, macular edema, and retinal detachment, among others. In recent years, with the advancement of medical technology, particularly the emergence of antiangiogenic drugs, there have been more options for DR treatment; however, it remains an important cause of impaired vision and even blindness.
The etiology of DR is associated with several morphological alterations, including pericyte loss, basement membrane thickening, increased vascular permeability, and microaneurysms. Pericytes and endothelial cells (ECs) are the most common retinal vascular cells. Communication between these two cell types is important for microvascular stabilization and remodeling. Pericyte loss is an early pathological feature of DR and frequently occurs in diabetic patients and animals (2). These two cell types share the same basement membrane on the vessel wall. There is communication between them due to the discontinuity of the basement membrane. ECs and pericytes communicate through the gap junctions between PEG sockets with other paracrine signaling factors, including growth factors, secreted cytokines, and extracellular secretions (2–5). Because pericytes are an abundant cell type in microvessels, their dysfunction can result in a variety of vascular-related diseases, including stroke and renal infarction, among others. Studies have shown that diseases associated with the diabetic retina are closely associated with pericytes. For example, pericytes create cPWWP2A (circ RNAs - PWWP2A), which is subsequently transferred to ECs via exosomes (5). CPWWP2A silencing can exacerbate diabetic retinal microvascular damage and dysfunction, such as pericyte loss, acellular capillary microaneurysm, vascular leakage, and inflammation (5).
Exosomes refer to small membrane vesicles containing complex RNAs and proteins. Under normal and pathological conditions, many cells secrete exosomes. They are primarily sourced in the multivesicles formed by the invagination of intracellular lysosomal granules, and the outer membranes of the multivesicles are released into the extracellular matrix following fusion with the cell membrane. Exosomes are considered to be membrane vesicles that are secreted exclusively and play a role in intercellular communication. Studies have shown that exosomes can maintain stable blood sugar levels in the body through multiple mechanisms and slow the development of diabetes and the associated microvessel formation, reducing the progression of this diabetic complication. However, the specific mechanism of exosome action remains unclear (1, 2). Many biological effects of exosomes are expressed by microRNA (miRNA), and miRNAs regulate different pathological alterations during DR, which include cell proliferation, apoptosis, inflammation responses, microcirculation impairments, oxidative stress, and cellular death by controlling the key molecules, particularly vascular endothelial growth factor (VEGF) (6). Therefore, miRNA antagonists or mimics as a novel class of drugs could be potentially helpful to control the occurrence and progression of pathological changes during DR.
This article examines the role and possible mechanisms of DR and the occurrence of exosomes and their possible use in its treatment. To reverse the chronic consequences of DR, we might use exosome substances to treat this disease, for example, miRNA-21 and miR-200a-3p.
2 Diabetic retinopathy and pericytes
DR is characterized by a severe deterioration of the retinal microvasculature, resulting in hypoperfusion, increased capillary permeability, abnormal proliferation of retinal blood vessels, and ultimately even blindness (7). DR can be classified into two types according to fundus changes: non-proliferative and proliferative phases (7, 8). The non-proliferative phase is confined to the retina; blood vessels undergo microaneurysms and bleeding and display vascular instability, macular edema, basement membrane thickening, and vascular degeneration (9). By comparison, the proliferative phase is characterized by neovascularization. New blood vessels are prone to rupture, which may eventually lead to retinal bleeding and detachment (8).
Although DR is primarily considered to be a disease caused by decreased EC function, there is significant evidence from animal studies that its pathogenesis begins with pericyte loss. Studies of diabetic complications in humans have shown that pericyte exfoliation in DR is associated with microvascular lesion development, including microaneurysms, acellular capillaries, vascular distortion, increased permeability, and capillary perfusion (4). The early pericyte loss is rapidly accompanied by EC loss and capillary network collapse, resulting in reduced retinal blood flow. It is proposed that the initial pericyte loss is driven by angpt-2 (10). The regeneration and plasticity of pericytes allow possible treatment of diseases associated with vascular malnutrition, including muscular dystrophy, ischemic stroke, and DR. To facilitate intercellular communication, the tight binding of pericytes and ECs occurs through direct contact, with ion exchange by gap junctions such as connexin43 (11) and the exchange of other paracrine molecules such as cathepsin D (12) and sphingosine 1-phosphate (13). Pericytes may serve as targets to treat microvascular diseases such as diabetic pathological angiogenesis and complications (14).
3 Effects of exosomes on diabetic retinopathy
Exosomes are released into the extracellular space from many cell types. These exosomes are broadly distributed in the body fluids. In recent years, mRNA and miRNA have been identified in vitro, which can be absorbed by nearby or distant cells, and regulate the receptor cells, thus playing a role in the occurrence and development of related diseases.
3.1 Exosomes
3.1.1 Concept and classification of exosomes
Exosomes currently refers specifically to discoid vesicles with a diameter of 40–100 nm. They are common membrane-bound nanovesicles which transport proteins, lipids, DNA, mRNA, and miRNA among other biomolecules. They are initially formed by endocytosis. Above all, internalization of the cell membrane produces endosomes. Subsequently, many small vesicles are formed in the inner body through the invaginated part of the inner body membrane. Such endosomes are termed multivesicular bodies (MVBs). Finally, MVBs fuse with the cell membrane, releasing endosome vesicles outside the cell as exosomes. Exosomes are produced by cells via exocytosis and are taken up by target cells. They transport substances and messages between cells through the circulation of body fluids. Therefore, exosomes play a role in different physiological and pathological processes in the human body (15, 16). Many biological effects of exosomes are expressed through miRNAs. miRNAs are a class of endogenous short non-coding single-stranded RNA molecules of 19–23 nucleotides in length which are from genome regions that do not code for proteins (17). They can be found in human fluids in a stable state, according to increasing data. Extracellular miRNAs can be loaded into high-density lipoprotein or bound by argonaute-2 protein outside vesicles, in addition to being packed into exosomes or microvesicles. All three mechanisms protect miRNAs against degradation and ensure their long-term stability (16). miRNAs negatively regulate the expression levels of target genes and confer characteristic changes on them, playing a regulatory role in almost all cellular processes. When miRNAs are analyzed as exosome miRNAs rather than intracellular miRNAs, researchers discovered a new role in certain cases, being exported inside extracellular vesicles, with Toll-like receptor (TLR)–binding miRNA released by cells from injured or stressed tissues able to reach the endosomal compartment and propagate inflammatory signals in distant recipient cells (17). Exosomal miR-21 and miR-29a were initially revealed to have the ability to attach to TLRs and activate immune cells, in addition to their traditional action of targeting miRNA (18). The quantity and composition of secreted miRNAs vary between diseased and healthy individuals (16, 19–21). To date, hundreds of miRNAs have been found in eye tissue, which may become a new biomarker for the early diagnosis of non-invasive ocular diseases (22).
3.1.2 Regulation of exosomes secretion
Exosomes are primarily derived from multivesicles formed by the invagination of lysosomal granules in cells, and the outer membranes of multivesicular vesicles are released into the extracellular environment after fusion with the cell surface. Although many cells are able to secrete exosomes under normal or abnormal conditions, under pathological conditions, exosome secretions may increase or their content may change.
On the one hand, it has been found that the RAB family (a member of the RAS oncogene family) of small GTPase proteins controls different steps of vesicle transport in cells (23), such as vesicle budding, mobility of vesicles and organelle interaction through the cytoskeleton, and the junction of vesicles with target chambers to form membrane fusion (24). Since the first proteomic study, endosomal-related members of this family have been identified in exosomes (25). For example, RAB-11 has been implicated in the control of TfR and Hsc70 released from exosomes in K562 cells (26).
On the other hand, in some studies, cellular stress enhanced exosome release (27–29). For example, studies found that radiotherapy-induced cellular senescence is associated with a significant rise in the release of exosome-like microvesicles. In premature aging, this new secretory phenotype depends on p53 activation. Radiation therapy can induce increased DNA damage, such as p53-dependent vesicle increase (28). At present, the specific mechanism of increased secretion caused by cellular stress is unclear, but the increased secretion may act on adjacent cells, leading to pathological changes in these cells.
3.2 Regulation of endothelial function
The two main cellular components of retinal microvessels are pericytes and ECs. The formation, maturation, and stabilization of microvessels require the interaction of these two cell types. Endothelial dysfunction is among the risk variables for DR development. Studies have shown that pericytes activated by the hypoxia-inducible factor (HIF) pathway can secrete exosomes under hypoxia and can regulate EC migration, germination, and angiogenesis (30). In addition to those from pericytes, exosomes from neurons, glial cells, ECs, and the circulation can modulate EC integrity and intercellular cross-talk in the neurovascular unit under physiological and pathological conditions (31–33). Gong et al. found that exosomes from mesenchymal stem cells (MSCs) were found to facilitate the transfer of miRNA from MSCs to human umbilical vein ECs (HUVECs) and promote angiogenesis. Their findings demonstrated that MSCs secrete exosomes containing proteins, cytokines, and chemicals that promote HUVEC-mediated tubular formation, increase the bud number of HUVEC spheroids, and attract ECs and promote their proliferation (34). Zhu et al. found that retinal astrocytes may release exosomes to transmit autophagy-inducing signals and regulate EC proliferation and migration; thus, they participate in the occurrence and development of retinal vascular-related diseases (35).
Endothelial-mesenchymal transition (EndMT) has been found to contribute to pathological fibrosis in proliferative DR (PDR). Gu et al. discovered that miR-202-5p secreted by retinal pigment epithelial cells (ARPE) can act as an important mediator of intercellular cross-talk and transfer miR-202-5p via the TGF/Smad pathway to inhibit EndMT (36). Cao et al. reported that MSC-derived exosomal SNHG7 can inhibit EndMT and tube formation by human retinal microvascular ECs (HRMECs) stimulated by high glucose (HG) by interacting with the miR-34a-5p/XBP1 signaling pathway, providing a viable treatment approach for DR therapy (37).
3.3 Smooth muscle cell proliferation and differentiation
Vascular smooth muscle cells (VSMCs) are a special cell type with abnormal plasticity in response to environmental stressors. Because abnormally increased VSMC proliferation is associated with a variety of vascular disorders, controlling its phenotype may have important implications for delaying DR development. In DR development caused by microvascular diseases caused by microcirculation disorders, hypoxia frequently occurs. Hypoxia promotes VSMC growth. New research suggests that miRNAs are key regulators of the VSMC hypoxia response. Previous studies have shown that miR-1260b is among the more upregulated hypoxia-related amines in VSMCs (38). GDF11-Smad–dependent signaling mediated by miR-1260b is an important signaling method for VSMC proliferation, and hypoxia controls this axis, which promotes aberrant VSMC proliferation (39). The new findings demonstrated that miR-1260b downregulation reduces VSMC proliferation. Consequently, hypoxia-induced increased miR-1260b expression may stimulate VSMC proliferation (39). Research has also shown that HG can induce VSMC calcification/aging, which in turn leads to diabetes-related vascular calcification/aging. Studies have shown that Notch3 is abundant in HG-stimulated HUVECs (HG-HUVEC-Exo). In addition, Notch3 expression in VSMCs was clearly increased in HG-stimulated HUVECs compared with the HG-stimulated HUVEC treatment group. When Notch3 inhibitors act in vivo to inhibit Notch3 expression, the capacity of HG-stimulated HUVECs to stimulate calcification/senescence of VSMCs is reduced (40).
Therefore, miR-1260b downregulation can inhibit VSMC proliferation and Notch3 expression, which may consequently delay VSMC calcification/aging and aid in the treatment of DR.
3.4 Macrophage activation
Inflammation is the central component of the pathogenesis of diabetes and metabolic syndrome, particularly in the development of complications. DR is considered a vascular and neurodegenerative disease that develops after periods of inadequate blood glucose control. Retinal microvascular disease is the early pathogenesis, which is caused by low-level, persistent leukocyte activation, resulting in recurrent capillary blockage and gradual retinal-depleting ischemia. At the molecular level, macrophage-restricted protein tyrosine phosphatase 1B (PTP1B) is a critical moderator of metabolic syndrome inflammation involving insulin resistance. PTP1B imbalance may underlie retinal microangiopathy (41). Hyperglycemia in patients with diabetes leads to an increased generation of reactive oxygen species (ROS) and the accumulation of advanced glycation or lipid oxidation end products (AGE and ALE, respectively), affecting the physiological functions of the retina (42). AGE and AGE receptor (RAGE) interaction induces a pro-inflammatory phenotype in microglia, resulting in an enhanced release of inflammatory cytokines (TNF-α and IL-6) (43, 44). Elevated TNF-α and IL-1β levels are consistent with increased intraretinal neovascularization in DR and increased microvascular degeneration in ischemic retinopathy (45, 46). Hyperglycemia could activate VEGF expression, and HIF-1 is translocated to the extracellular signal–regulated kinase (ERK)1/2–nuclear factor κB (NF-κB) signaling pathway in the nucleus and microglia (47, 48). VEGF overexpression contributes to retinal neovascularization, whereas translocation of HIF-1 increases the transcription of angiogenesis-related genes (49).
3.5 Platelet activation
Vascular fibrosis is the main pathological feature of the proliferative stage of DR. However, the molecular mechanism of its occurrence remains unclear. Connective tissue growth factor (CTGF), a main fibrotic factor, is highly expressed in DR and plays a key role in retinal endothelial membrane thickening (50). The study showed that exosomes of platelet plasma in diabetic rats (DM-PRP-Exos) considerably elevated Müller cell growth and metastasis compared with exosomes of platelet plasma from normal control rats (Nor-PRP-Exos). The above results suggest that platelet plasma secretion–induced fibrogenesis may be triggered by activating the phosphoinositide-3 kinase-serine/threonine kinase (PI3K/Akt) signaling pathway (51). Therefore, under hyperglycemia, platelets are stimulated to produce PRP-Exos, which activate the PI3K/Akt signaling pathway (52).
Platelet plasma exosomes (PRP-Exos) mediate hyperglycemia-induced retinal endothelial damage via upregulating the TLR4 signaling pathway. They can transfer platelet cytokines, alter protein expression, and cause retinal endothelial dysfunction and early DR. A study found that the PRP-Exo levels in the circulation of diabetic rats were significantly increased. At the same time, it was further proved that HG effectively enhances the capacity of platelets to produce PRP-Exos in vitro. It was shown that PRP-Exos can promote TLR4 expression and that of its downstream proteins MyD88, p-NF-κB/P65, and NF-κB/P65 and activate the TLR4 pathway (53). Accumulating evidence suggests that TLR4 has a vital function in regulating retinal homeostasis and is engaged in DR progression (54). The new study found that CXCL10 may activate the TLR4 pathway in vitro. CXCL10 blockade can downregulate the TLR4 signaling pathway and diminish PRP-Exo–induced retinal inflammation. These results suggested that CXCL10 appears to be a key regulator of PRP-Exo–derived retinal endothelial damage in DR (53). Consequently, inhibiting the TLR4 signaling pathway provides a new therapeutic idea for reducing the early vascular DR complications induced by PRP-Exo.
3.6 In summary
As described above, circulating exosome action on various related cells leads to DR. Figure 1 shows the effect of exosomes on cells associated with DR.
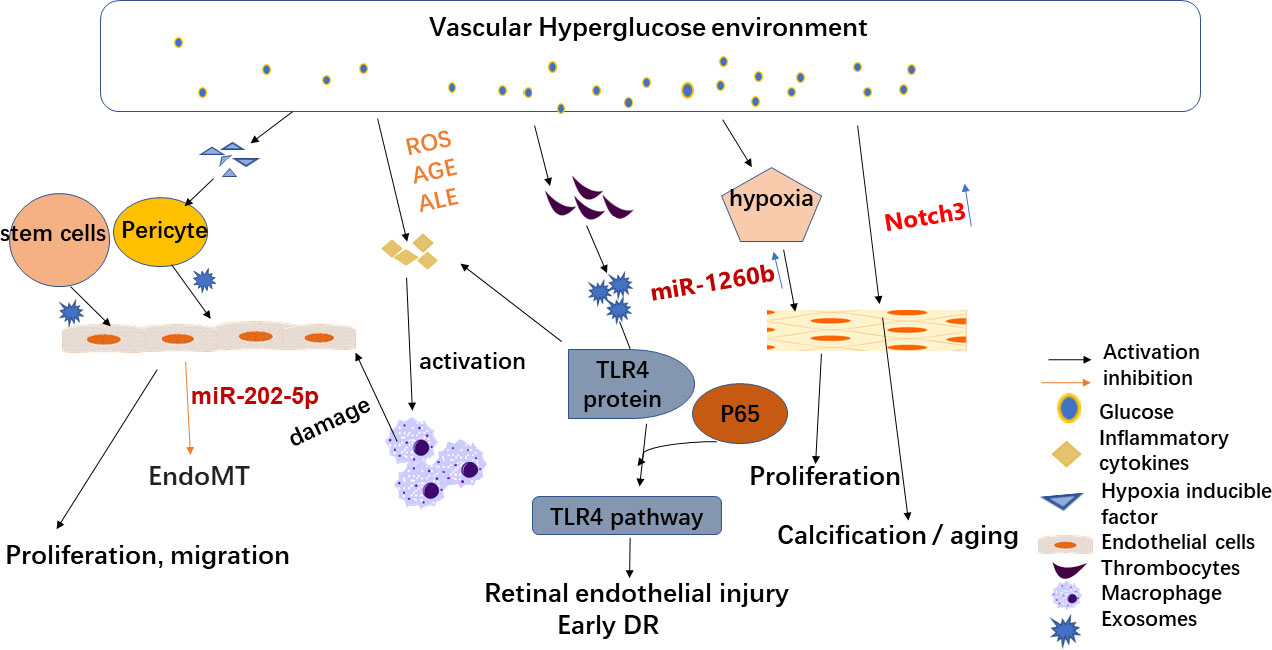
Figure 1 Effects of exosomes on diabetic retinopathy-related cells. High-glucose environment and hypoxia alter circulating exosomes and play a key role in DR development. Therefore, stem cell exosomes may be an effective treatment for DR. Hypoxia-induced increased secretion of hypoxia-inducible factors can lead to endothelial dysfunction (ED) and promote endothelial cell permeability in vitro. Hypoxia also leads to changes in circulating exosomes (such as miR-1260b), resulting in the proliferation of vascular smooth muscle cells. A high-glucose microenvironment also leads to a greater generation of reactive oxygen species (ROS) and the build-up of advanced glycation or lipid oxidation end products (AGE or ALE), which in turn leads to inflammatory increased cytokines (TNF-α, the secretion of IL-6) and thus to inflammation and exacerbates the harm of DR. PRP-Exos participate in early DR formation via mediating hyperglycemia-induced retinal endothelial damage by upregulating the TLR4 signaling pathway.
4 Effects of exosomes on pericytes
Long-term hyperglycemia causes DR, a frequent microvascular condition. Intercommunication of pericytes and ECs is critical for maintaining vascular homeostasis and remodeling. Hypoxia was found to upregulate circEhmt1 production in pericytes, which can subsequently be transported to ECs by exosomes. In addition, circEhmt1 overexpression has a protective effect against HG-induced EC damage in vitro. Mechanistically, circEhmt1, which is strongly expressed in pericyte nuclei, upregulates NFIA (transcription factor) levels and inhibits NLRP3-mediated inflammatory bodies (55).
Diabetes-related stress enhanced cPWWP2A expression in pericytes but had no effect on ECs, according to the findings. Pericytes create cPWWP2A, which is delivered to ECs through exosomes. In vitro studies demonstrated that cPWWP2A directly regulates pericyte biology, whereas EC biology is directly regulated by cPWWP2A-carrying exosomes.
CPWWP2A acts as an endogenous miR-579 sponge, isolating and inhibiting the activity of miR-579. Diabetes-induced retinal vascular dysfunction was reduced in vivo when cPWWP2A overexpression or miR-579 suppression was used. Furthermore, suppressing cPWWP2A or overexpressing miR-579 can exacerbate microvascular dysfunction by inhibiting the cPWWP2A-mediated signaling pathway. This study suggests that intervention at the level of cPWWP2A or miR-579 expression could provide an opportunity for the treatment of diabetic microvascular complications (2).
Exosomes reduce pericyte migration by downregulating NF-κB p65 signaling, thereby maintaining the function of the blood spinal cord barrier (BSCB). Studies have found that bone marrow MSC–derived extracellular vesicles prevent pericyte migration by inhibiting the activation of the NF-κB signaling pathway. This improves the integrity of the BSCB (56).
Pericyte activation is a key pathogenic characteristic of interstitial fibrosis (RIF). It was found that MSC-derived exosomes deliver the miR-34c-5p by inhibiting core focusing (CF) to reduce cellular activation and help exosomal miR-34c-5p enter pericytes through the RIFcd81–epidermal growth factor receptor (EGFR) ligand receptor complex. The findings of this study offer a potential therapy option for renal fibrosis (57).
5 Possible mechanisms of exosome-mediated diabetic retinopathy
Although studies have demonstrated exosome involvement in DR progression, including multiple cascades and interconnections, the specific processes remain unknown. The mechanisms associated with exosomes and DR are mainly hypoxia, inflammation, and stress intensification. As mentioned above, exosomes are extensively involved in DR development and progression. Next, we will attempt to explain the signaling pathways and molecular mechanisms by which exosomes, mainly through miRNAs, may be involved in DR development and progression.
5.1 TGF-β–mediated pathways
TGF-β and TGF-β–mediated signaling pathways play an exacerbating role in DR pathogenesis (58). Further research has revealed that they are essential regulators of cell growth and mid-differentiation. For example, as key inducers of tissue fibrosis, they can promote fibroblast proliferation, ultimately leading to tissue fibrosis (59, 60). Lou et al. reported that the miR-21 expression level in the retina of rats with DR was considerably higher than the normal rats, implying that abnormal retinal miR-21 expression may participate in DR pathogenesis. Moreover, the analysis results of this study showed that TGF-β signaling pathway inhibitors greatly reduced the effect of miR-21 in DR in rats and improved ocular hemodynamics in these animals. This suggests that miR-21 controls the TGF-β signaling pathway involved in the pathogenesis of DR. Therefore, TGF-β signaling pathway regulation by miR-21 affects hemodynamics in rats with PDR (61). Li et al. found that inhibiting the TGF-β2/Smad pathway increased miR-200a-3p, which prevented DR development. It was also found that miR-200a-3p was significantly downregulated in both ARPE-19 cells and retinal tissues of rats with DR after HG treatment, whereas TGF-β2 expression was upregulated. Subsequently, miR-200a-3p overexpression greatly accelerated cell proliferation, decreased apoptosis, and reduced the level of secreted inflammatory cytokines and VEGF in HG-injured ARPE-19 cells. MiR-200a-3p overexpression attenuated HG-induced damage in ARPE-19 cells, decreased the secretion of inflammatory cytokines, and downregulated VEGF expression through inactivation of the TGF-β2/Smad pathway. In vivo, miR-200a-3p upregulation improved retinal angiogenesis and inflammation in DR in rats, thus providing a novel target for DR therapy. However, it is unclear how miR-200a-3p expression was upregulated in vivo (62).
5.2 PI3K/Akt signaling pathway
The PI3K/Akt signaling pathway is a downstream signal of a variety of cell-surface receptors that regulate cell proliferation, survival, and death (63). Zhang et al. discovered that miR-183 was markedly upregulated in a rat model of DR with activation of the PI3K/Akt/VEGF signaling pathway. It was observed that miR-183 expression was upregulated and BTG1 expression was downregulated in retinal tissue in DR in rats. MiR-183 overexpression activated the PI3K/Akt/VEGF signaling pathway to inhibit BTG1 and promote EC proliferation but inhibit apoptosis. According to the findings, miR-183 inhibition could inhibit vascular EC proliferation and angiogenesis by downregulating BTG1 and inactivating the PI3K/Akt/VEGF signaling pathway (64).
It is known that, in PDR, miR-21 expression is increased and can promote retinal pigment epithelial cell proliferation and migration (65). Lu et al. found that miR-21 may be a target for DR therapy because it has the potential to block DR. Downregulation of miR-21 disrupts the survival of retinal vascular ECs (RVECs), inducing apoptosis of these cells, and attenuates angiogenesis by inhibiting the PI3K/Akt/VEGF signaling pathway and upregulating phosphatase and tensin homolog (PTEN). The results indicated that miR-21 overexpression may activate the PI3K/Akt/VEGF signaling pathway by inhibiting PTEN expression, thereby stimulating RVEC activity and angiogenesis in DR in rats, suggesting that miR-21 may be a target of DR treatment (66).
According to Wang et al., HG decreased the relative miR-199a-3p expression level in HRMECs and apre-19 cells but increased VEGF expression. Upregulation of miR-199a-3p not only significantly alleviated HG-induced cell proliferation and migration but also significantly inhibited the PI3K/Akt signaling pathway and HG-induced angiogenesis. MiR-199a-3p upregulation can control the PI3K/Akt pathway by suppressing VEGF and promoting HG-induced angiogenesis in HRMECs (67).
5.3 p38 MAPK signaling pathway
The MAPK signaling pathway is present in many cells, delivering extracellular stimuli that elicit biological responses. The P38 signal transduction pathway (MAPK pathway) regulates a wide range of biological functions (68). Li et al. found that miR-141-3p inhibited retinal angiogenesis in glaucoma mice by preventing activation of the docking protein 5 (DOK5)–mediated MAPK signaling pathway. The DOK5 gene was repressed by miR-141-3p, which activated the MAPK pathway. The findings revealed that miR-141-3p reduced the proliferation and angiogenesis of retinal vascular epithelial cells and promoted RGC apoptosis (69). Chen et al. found that MSC-derived exosomes prevented hypoxia-induced cell death by carrying miR-21 and inhibiting p38 MAPK signaling (70). MSC exosomes may aid patients with diabetes, according to their findings. Dai et al. reported that baicalin (BAI) inhibited the activation of the NF-κB and p38 MAPK pathways by upregulating miR-145 and had a protective effect on HG-induced injury of human retinal pigment epithelial cells (71).
5.4 NF-κB pathway
NF-κB functions primarily in biological processes, including the inflammatory response and immunity. It was demonstrated to be activated in the diabetic retina in numerous studies, its activation increasing capillary apoptosis (72), which is a precursor to the development of DR characteristics. The activity of the NF-κB signaling pathway has been reported to be enhanced in diabetic rat studies. In turn, NF-κB p65 expression upregulation increases ROS production, which leads to microaneurysms, retinal neovascularization, and vitreous hemorrhage in diabetic rats, and promotes DR progression (73, 74).
According to Li et al., the number of pericytes in retinal capillaries of miR-874 mimics-treated DR in rats rose, whereas EC proliferation was reduced. MiR-874 inhibitor exacerbates DR in diabetic rats after treatment. The results showed that miR-874 overexpression suppressed NF-κB signaling pathway expression and alleviated DR in diabetic rats (75). Li et al. demonstrated low miR-486-3p expression and high TLR4 and NF-κB expression in HG-treated Müller cells. TLR4 is the action site of miR-486-3p. In HG-treated Müller cells, miR-486-3p upregulation or TLR4 downregulation prevented oxidative damage, inflammation, and apoptosis while promoting proliferation. This study highlighted that the protective role of exosomal-induced miR-486-3p upregulation in DR mice is by TLR4/NF-κB axis inhibition (76).
Hui et al. found that miR-145 was downregulated in HG-treated retinal microvascular ECs (RECs), while miR-145 overexpression suppressed enhanced TLR4 expression and NF-κB p65 nuclear translocation in HG-treated RECs. More importantly, miR-145 overexpression reduced REC apoptosis, oxidative stress, and inflammatory cytokine release in HG environments. These findings revealed that miR-145 may exert antioxidant and anti-inflammatory effects in DR (54). Ye and Steinle reported decreased miR-146a expression in human RECs cultured with HG. Overexpression of miR-146a with a miR-146a mimic reduced TLR4/NF-κB and TNF expression in RECs induced by HG. Overexpression of miR-146a in RECs reduced MyD88-dependent and -independent signaling under HG conditions. The results showed that miR-146a suppressed TLR4/NF-κB and TNF-α, the potential site of action for reducing REC inflammation (77).
5.5 Summary
Exosomes have multiple mechanisms of action in DR, but the specific mechanisms remain unclear. However, exosomes might have a critical role in this process and its treatment. Figure 2 shows the possible signaling pathways and molecular mechanisms involving miRNA in DR formation and development. Table 1 shows exosome-derived proteins and RNA involved in DR.
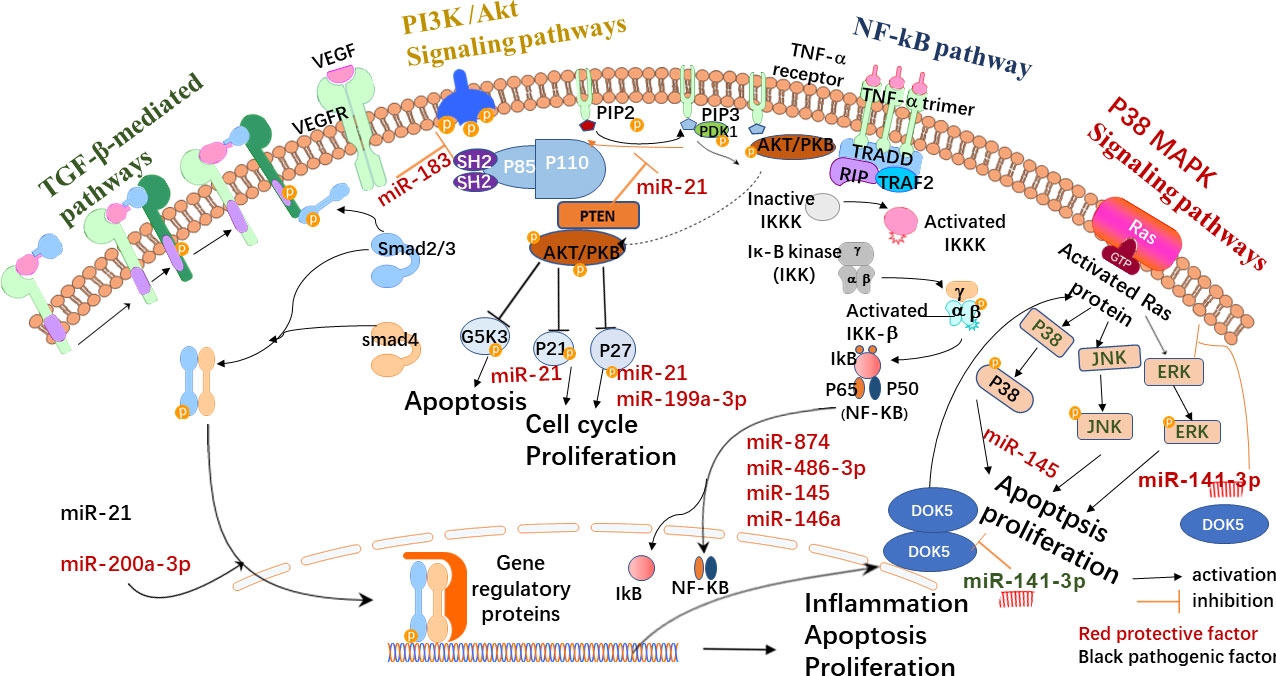
Figure 2 Possible exosome-mediated signaling pathways in DR. Exosomes are synthesized in various cells. Under physiological or pathological conditions, their carriers may change and participate in the formation of the collective pathological state. They may activate TGF-β, the signaling pathway that mediates the hemodynamics of individuals with PDR. By blocking TGF-β, the activation of the signaling pathway promotes cell proliferation and reduces apoptosis and the inflammatory response. Exosomes (such as miR-141-3p) may also inhibit retinal neovascularization by preventing the activation of the p38/MAPK signaling pathway. Exosomes can also be detected by the PI3K/Akt signaling pathway, which can induce vascular endothelial cell proliferation while preventing cell apoptosis, as well as cell proliferation and migration of the retinal pigment epithelium cell. The stimulation of the signaling system may also aid in the reduction of HG-induced cell proliferation, migration, and angiogenesis. In diabetic rats, the activity of the NF-κB signaling pathway rose dramatically, and inhibiting the NF-κB signaling pathway can reduce inflammation, apoptosis, and oxidative stress.
6 Exosomes and diabetes-related cardiovascular and cerebrovascular events
The vascular complications of diabetes include macrovascular and microvascular complications. In recent years, many studies have proved that miRNA has a good therapeutic effect in the treatment not only of DR but also of other complications of diabetes. There is increasing evidence that exosomes change in the blood of patients with diabetes and are implicated in the progression of diabetes, including microvascular complications, inflammation, and changes in coagulation (78, 79). Next, we briefly introduce the role of miRNA in the treatment of other complications of diabetes. Figure 3 shows the exosomes related to cardiovascular and cerebrovascular risk events, such as DN, myocardial infarction (MI), and stroke.
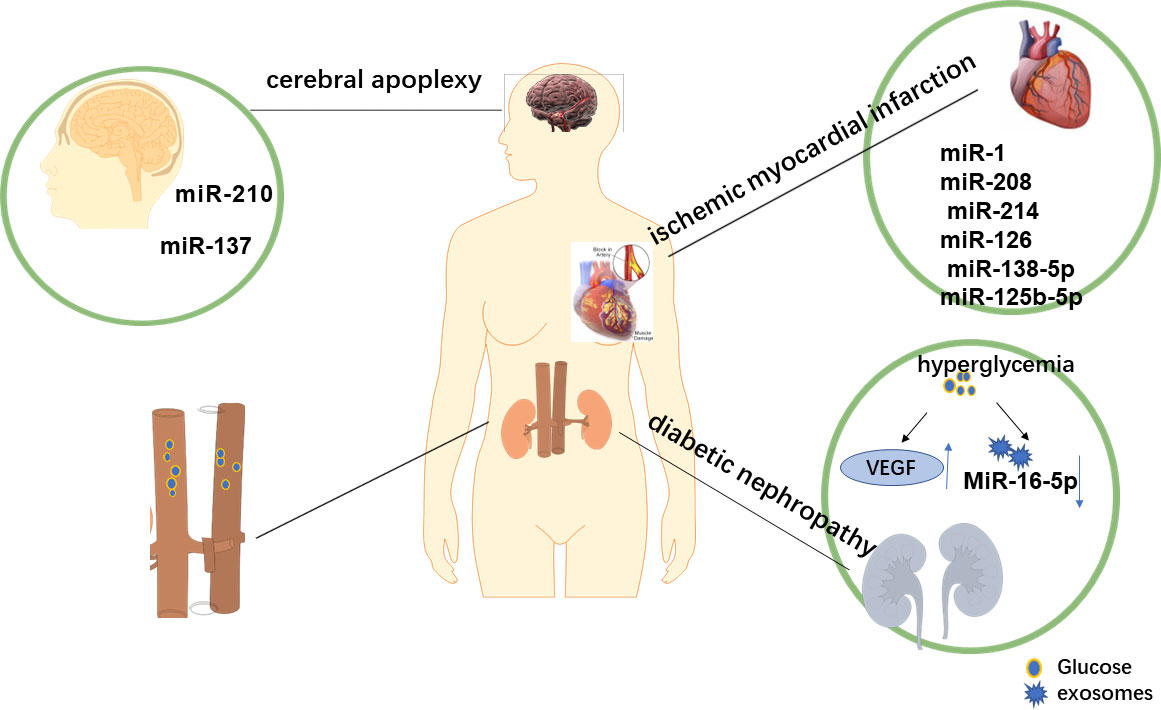
Figure 3 Exocrine-related complications of diabetes. During the development t of diabetes mellitus, changes in the microenvironment also lead to alterations in exocrine bodies, which are involved in cardiovascular and cerebrovascular risk events, such as diabetic nephropathy, myocardial infarction, and stroke. Ever-increasing evidence suggests that the level of exosomes in the blood of patients with diabetes is elevated and is involved in the pathophysiology of diabetes-related diseases. Therefore, we concluded that the overexpression and downregulation of specific miRNA might be a new method to treat diabetes and its related complications.
6.1 Diabetic nephropathy
It was found that rapamycin (mTOR) is a core component of cell growth signaling, and, when its activity is enhanced, it can promote protein translation and autophagy. Autophagy protects against renal injury induced by hyperglycemia (80). Ebrahim et al. found that BMSC-derived exosomes enhanced autophagy via blocking the mTOR signaling pathway in a model of DR. In addition, they found that when using MSC-derived exosomes to treat mice with DN, the histological morphology of the kidneys was restored and fibrosis markers were reduced (81).
The development of DN is also associated with podocyte injury (82). VEGF produced by podocytes is not beneficial in treating DN (83). MiR-16-5p could reduce VEGF expression. Hyperglycemia reduces podocyte miR-16-5p production and stimulates VEGF release. Following miR-16-5p overexpression in human embryonic stem cells, exosomes might deliver it to HG-treated podocytes, reducing the degree of podocyte apoptosis and expressing VEGF, thereby delaying the occurrence and development of DN (84).
6.2 Ischemic myocardial infarction
MI is a major cause of mortality in all cardiovascular diseases. Ischemia and reperfusion damage is an inevitable adverse reaction after MI. There is presently no effective treatment to reduce the damage to the heart from MI. Recent research has shown that miRNAs in the heart and circulation are markedly altered after MI (85). For example, Cheng et al. found significantly increased miR-1 and miR-208 levels in the urine of patients with acute MI and in the circulating blood of rats after acute MI (86). Mao et al. found that KLF3-AS1 mediates Sirt1 expression by serving as a ceRNA to sponge miR-138-5p, thereby regulating cardiomyocyte pyroptosis and MI progression (87). Furthermore, miR-125b-5p inhibited p53 and BAK1 production, which reduced apoptosis. In addition, increased miR-125b-5p expression in macrophages alleviated hypoxia/reperfusion-induced cellular damage. Enhanced miR-125b-5p production in the myocardium significantly reduced the size of the MI (88, 89). The findings of Zhu et al. showed a unique method, whereby cell-free hypo-exo promotes ischemic heart repair via anti-apoptotic miR-125b-5p (89). The role of exosomes in MI is increasingly recognized, but the mechanisms involved and their role in improving cardiac function remain unclear (90).
6.3 Stroke
Reflex mechanisms are engaged to protect cerebral perfusion in early hemorrhagic strokes, such as intracerebral hemorrhage and subarachnoid hemorrhage, but the corresponding secondary injury and malfunction can lead to cerebral ischemia, hypoxia, and ultimately neuronal cell death (91). Recent evidence suggests that exosomes may perform various functions in brain repair and as biomarkers for stroke (92). In a previous research, exosome extraction from MSCs was reported to ameliorate specific brain tissue damage in an experimental animal model (93). Exosomes generated by endothelial progenitor cells (EPCs) have been shown to protect ECs from hypoxia/reoxidation injury, which is partially due to the role of miR-210 (94). According to Liu et al., miR-137 upregulation promoted EPC proliferation and angiogenesis in mice with ischemic stroke by the Notch pathway (95). Taken together, exosomes are implicated in the occurrence and progression of stroke through various mechanisms, providing new ideas for stroke treatment.
7 Conclusion and prospects
In recent years, exosomes have been studied as a new biological entity engaged in intercellular communication in a variety of physiological and pathological processes. Ongoing technological and experimental advances have the potential to uncover cellular and molecular mechanisms of intercellular communication, organ homeostasis, and disease, enhancing our ability to use these mechanisms as therapeutic and diagnostic tools. DR increases the risk for blindness in those with diabetes. Previous studies have found that exosomes may play a role in both the pathogenesis and treatment of DR. Earlier studies have found that circulatory miRNAs are differentially expressed in subjects with diabetes (96), suggesting that miRNAs could be used as new biomarkers for detecting or predicting the overall progression of the disease, as well as the progression of retinopathy from mild to sight-threatening (97–99). For example, miR-221, an antiangiogenic miRNA found in blood as a biomarker for DR in individuals with T2DM and PDR, was found to be implicated in the physiopathology of T2DM and macrovascular problems (100–102). RNA sequencing has established the potential biomarkers let-7a and miR-151 in serum for early-stage and late-stage DR in patients with T2DM. The previous study has discovered that using multiple miRNAs and anti-miRNAs in a combinational therapy is a unique method that involves targeting numerous pathways with different drugs and may provide a synergistic angiostatic effect that can help to prevent DR pathologic angiogenesis problems. Overexpression of miR-216a, for example, protected against HRMEC damage in DR by inhibiting the NOS2/JAK/STAT axis in the DR rat retina (103). Simultaneously, miRNA-29b-3p increases HRMEC apoptosis in DR by inhibiting SIRT1 (104). Upregulation of miR-203a-3p, which targets VEGFA and HIF-1, may decrease retinal neovascularization in the oxygen-induced retinopathy rat model (105). In DR development, the involvement of exosomes and the role of pericytes have separately been widely studied. However, limited studies have investigated the role of pericyte-related exosomes in the occurrence and development of DR, particularly its biological mechanism. Consequently, future studies should focus on the effect of exocytosis on pericytes and thus its potential role in DR. The use of stem cell exosomes for the treatment of DR requires further basic and clinical research.
In conclusion, exosomes, particularly their miRNAs, are involved in the pathophysiological process of DR and establish multilevel connections. Exosomes have broad application prospects in the treatment and prognostic evaluation of DR.
Author contributions
S-rN is responsible for writing the manuscript. J-mH is responsible for data collection. YH and SL are mainly responsible for reviewing and revising the article. All authors contributed to the article and approved the submitted version.
Funding
This work was supported by the Science and Technology Bureau of Quanzhou (grant number 2020CT003 and 2021N036S).
Conflict of interest
The authors declare that the research was conducted in the absence of any commercial or financial relationships that could be construed as a potential conflict of interest.
Publisher’s note
All claims expressed in this article are solely those of the authors and do not necessarily represent those of their affiliated organizations, or those of the publisher, the editors and the reviewers. Any product that may be evaluated in this article, or claim that may be made by its manufacturer, is not guaranteed or endorsed by the publisher.
Abbreviations
DR, diabetic retinopathy; miRNA, microRNA; ECs, endothelial cells; MVBs, multivesicular bodies; miRNAs, microRNAs; NC RNA, noncoding RNA; MSCs, mesenchymal stem cells; HRMECs, human retinal microvascular endothelial cells; HG, high glucose; HUVECs, human umbilical vein endothelial cells; EndMT, endothelial mesenchymal transformation; VSMCs, vascular smooth muscle cells; HUVEC-Exo, human umbilical vein endothelial cell exosome; ROS, reactive oxygen species; AGE, advanced glycation end products; ALE, advanced lipid oxidation end products; RAGE, AGE receptor; CTGF, connective tissue growth factor; PRP-Exos, exosomes derived from platelet-rich plasma; CXCL10, CXCchemokineligand-10; BSCB, Blood spinal cord barrier; BMSC-EVs, Extracellular vesicles derived from bone marrow mesenchymal stem cells; TGF-β 2, transforming growth factor-β 2; VEGF, vascular endothelial growth factor; REC, retinal microvascular endothelial cells; DKD, development of diabetic nephropathy; IR, ischemia and reperfusion; MI, myocardial infarction; EPCs, endothelial progenitor cells.
References
1. Cui X, Zhu L, Zhai R, Zhang B, Zhang F. Mesenchymal stem cell-derived exosomes: a promising vector in treatment for diabetes and its microvascular complications. Am J Transl Med (2021) 13(5):3942–53.
2. Liu C, Ge HM, Liu BH, Dong R, Shan K, Chen X, et al. Targeting pericyte-endothelial cell crosstalk by circular RNA-cPWWP2A inhibition aggravates diabetes-induced microvascular dysfunction. Proc Natl Acad Sci U.S.A. (2019) 116(15):7455–64. doi: 10.1073/pnas.1814874116
3. van Dijk CG, Nieuweboer FE, Pei JY, Xu YJ, Burgisser P, van Mulligen E, et al. The complex mural cell: pericyte function in health and disease. Int J Cardiol (2015) 190:75–89. doi: 10.1016/j.ijcard.2015.03.258
4. Arboleda-Velasquez JF, Valdez CN, Marko CK, D’Amore PA. From pathobiology to the targeting of pericytes for the treatment of diabetic retinopathy. Curr Diabetes Rep (2015) 15(2):573. doi: 10.1007/s11892-014-0573-2
5. Huang H. Pericyte-endothelial interactions in the retinal microvasculature. Int J Mol Sci (2020) 21(19):7413. doi: 10.3390/ijms21197413
6. Akbari Kordkheyli V, Amir Mishan M, Khonakdar Tarsi A, Mahrooz A, Rezaei Kanavi M, Hafezi-Moghadam A, et al. MicroRNAs may provide new strategies in the treatment and diagnosis of diabetic retinopathy: Importance of VEGF. Iran J Basic Med Sci (2021) 24(3):267–79. doi: 10.22038/ijbms.2021.52164.11807
7. Hammes HP, Feng Y, Pfister F, Brownlee M. Diabetic retinopathy: targeting vasoregression. Diabetes (2011) 60(1):9–16. doi: 10.2337/db10-0454
8. Winkler EA, Bell RD, Zlokovic BV. Central nervous system pericytes in health and disease. Nat Neurosci (2011) 14(11):1398–405. doi: 10.1038/nn.2946
10. Hammes H, Lin J, Wagner P, Feng Y, Vom Hagen F, Krzizok T, et al. Angiopoietin-2 causes pericyte dropout in the normal retina: evidence for involvement in diabetic retinopathy. J Clin Med (2004) 53(4):1104–10. doi: 10.2337/diabetes.53.4.1104
11. Li AF, Sato T, Haimovici R, Okamoto T, Roy S. High glucose alters connexin 43 expression and gap junction intercellular communication activity in retinal pericytes. Invest Ophthalmol Vis Sci (2003) 44(12):5376–82. doi: 10.1167/iovs.03-0360
12. Monickaraj F, McGuire P, Das A. Cathepsin d plays a role in endothelial-pericyte interactions during alteration of the blood-retinal barrier in diabetic retinopathy. FASEB J (2018) 32(5):2539–48. doi: 10.1096/fj.201700781RR
13. McGuire PG, Rangasamy S, Maestas J, Das A. Pericyte-derived sphingosine 1-phosphate induces the expression of adhesion proteins and modulates the retinal endothelial cell barrier. Arterioscler Thromb Vasc Biol (2011) 31(12):e107–115. doi: 10.1161/ATVBAHA.111.235408
14. Caporali A, Martello A, Miscianinov V, Maselli D, Vono R, Spinetti G. Contribution of pericyte paracrine regulation of the endothelium to angiogenesis. Pharmacol Ther (2017) 171:56–64. doi: 10.1016/j.pharmthera.2016.10.001
15. He C, Zheng S, Luo Y, Wang B. Exosome theranostics: Biology and translational medicine. Theranostics (2018) 8(1):237–55. doi: 10.7150/thno.21945
16. Zhang J, Li S, Li L, Li M, Guo C, Yao J, et al. Exosome and exosomal microRNA: trafficking, sorting, and function. Genomics Proteomics Bioinf (2015) 13(1):17–24. doi: 10.1016/j.gpb.2015.02.001
17. Bosch S, Young NA, Mignot G, Bach JM. Epigenetic mechanisms in immune disease: The significance of toll-like receptor-binding extracellular vesicle-encapsulated microRNA. Front Genet (2020) 11:578335. doi: 10.3389/fgene.2020.578335
18. Fabbri M, Paone A, Calore F, Galli R, Gaudio E, Santhanam R, et al. MicroRNAs bind to toll-like receptors to induce prometastatic inflammatory response. Proc Natl Acad Sci U.S.A. (2012) 109(31):E2110–2116. doi: 10.1073/pnas.1209414109
19. Ambros V. The functions of animal microRNAs. Nature (2004) 431(7006):350–5. doi: 10.1038/nature02871
20. Doench JG, Sharp PA. Specificity of microRNA target selection in translational repression. Genes Dev (2004) 18(5):504–11. doi: 10.1101/gad.1184404
21. Sevignani C, Calin GA, Siracusa LD, Croce CM. Mammalian microRNAs: a small world for fine-tuning gene expression. Mamm Genome (2006) 17(3):189–202. doi: 10.1007/s00335-005-0066-3
22. Guo R, Shen W, Su C, Jiang S, Wang J. Relationship between the pathogenesis of glaucoma and miRNA. Ophthalmic Res (2017) 57(3):194–9. doi: 10.1159/000450957
23. Kowal J, Tkach M, Thery C. Biogenesis and secretion of exosomes. Curr Opin Cell Biol (2014) 29:116–25. doi: 10.1016/j.ceb.2014.05.004
24. Stenmark H. Rab GTPases as coordinators of vesicle traffic. Nat Rev Mol Cell Biol (2009) 10(8):513–25. doi: 10.1038/nrm2728
25. Thery C, Boussac M, Veron P, Ricciardi-Castagnoli P, Raposo G, Garin J, et al. Proteomic analysis of dendritic cell-derived exosomes: a secreted subcellular compartment distinct from apoptotic vesicles. J Immunol (2001) 166(12):7309–18. doi: 10.4049/jimmunol.166.12.7309
26. Savina A, Fader CM, Damiani MT, Colombo MI. Rab11 promotes docking and fusion of multivesicular bodies in a calcium-dependent manner. Traffic (2005) 6(2):131–43. doi: 10.1111/j.1600-0854.2004.00257.x
27. Kanemoto S, Nitani R, Murakami T, Kaneko M, Asada R, Matsuhisa K, et al. Multivesicular body formation enhancement and exosome release during endoplasmic reticulum stress. Biochem Biophys Res Commun (2016) 480(2):166–72. doi: 10.1016/j.bbrc.2016.10.019
28. Lehmann BD, Paine MS, Brooks AM, McCubrey JA, Renegar RH, Wang R, et al. Senescence-associated exosome release from human prostate cancer cells. Cancer Res (2008) 68(19):7864–71. doi: 10.1158/0008-5472.CAN-07-6538
29. Beer L, Zimmermann M, Mitterbauer A, Ellinger A, Gruber F, Narzt MS, et al. Analysis of the secretome of apoptotic peripheral blood mononuclear cells: Impact of released proteins and exosomes for tissue regeneration. Sci Rep (2015) 5:16662. doi: 10.1038/srep16662
30. Mayo JN, Bearden SE. Driving the hypoxia-inducible pathway in human pericytes promotes vascular density in an exosome-dependent manner. Microcirculation (2015) 22(8):711–23. doi: 10.1111/micc.12227
31. Zagrean AM, Hermann DM, Opris I, Zagrean L, Popa-Wagner A. Multicellular crosstalk between exosomes and the neurovascular unit after cerebral ischemia. therapeutic implications. Front Neurosci (2018) 12:811. doi: 10.3389/fnins.2018.00811
32. Huang C, Fisher KP, Hammer SS, Navitskaya S, Blanchard GJ, Busik JV. Plasma exosomes contribute to microvascular damage in diabetic retinopathy by activating the classical complement pathway. Diabetes (2018) 67(8):1639–49. doi: 10.2337/db17-1587
33. Xu B, Zhang Y, Du XF, Li J, Zi HX, Bu JW, et al. Neurons secrete miR-132-containing exosomes to regulate brain vascular integrity. Cell Res (2017) 27(7):882–97. doi: 10.1038/cr.2017.62
34. Gong M, Yu B, Wang J, Wang Y, Liu M, Paul C, et al. Mesenchymal stem cells release exosomes that transfer miRNAs to endothelial cells and promote angiogenesis. Oncotarget (2017) 8(28):45200–12. doi: 10.18632/oncotarget.16778
35. Zhu L, Zang J, Liu B, Yu G, Hao L, Liu L, et al. Oxidative stress-induced RAC autophagy can improve the HUVEC functions by releasing exosomes. J Cell Physiol (2020) 235(10):7392–409. doi: 10.1002/jcp.29641
36. Gu S, Liu Y, Zou J, Wang W, Wei T, Wang X, et al. Retinal pigment epithelial cells secrete miR-202-5p-containing exosomes to protect against proliferative diabetic retinopathy. Exp Eye Res (2020) 201:108271. doi: 10.1016/j.exer.2020.108271
37. Cao X, Xue LD, Di Y, Li T, Tian YJ, Song Y. MSC-derived exosomal lncRNA SNHG7 suppresses endothelial-mesenchymal transition and tube formation in diabetic retinopathy via miR-34a-5p/XBP1 axis. Life Sci (2021) 272:119232. doi: 10.1016/j.lfs.2021.119232
38. Lee J, Heo J, Kang H. miR-92b-3p-TSC1 axis is critical for mTOR signaling-mediated vascular smooth muscle cell proliferation induced by hypoxia. Cell Death Differ (2019) 26(9):1782–95. doi: 10.1038/s41418-018-0243-z
39. Seong M, Kang H. Hypoxia-induced miR-1260b regulates vascular smooth muscle cell proliferation by targeting GDF11. BMB Rep (2020) 53(4):206–11. doi: 10.5483/BMBRep.2020.53.4.136
40. Lin X, Li S, Wang YJ, Wang Y, Zhong JY, He JY, et al. Exosomal Notch3 from high glucose-stimulated endothelial cells regulates vascular smooth muscle cells calcification/aging. Life Sci (2019) 232:116582. doi: 10.1016/j.lfs.2019.116582
41. Forrester JV, Kuffova L, Delibegovic M. The role of inflammation in diabetic retinopathy. Front Immunol (2020) 11:583687. doi: 10.3389/fimmu.2020.583687
42. Sharma Y, Saxena S, Mishra A, Saxena A, Natu SM. Advanced glycation end products and diabetic retinopathy. J Ocul Biol Dis Infor (2012) 5(3-4):63–9. doi: 10.1007/s12177-013-9104-7
43. Chen J, Sun Z, Jin M, Tu Y, Wang S, Yang X, et al. Inhibition of AGEs/RAGE/Rho/ROCK pathway suppresses non-specific neuroinflammation by regulating BV2 microglial M1/M2 polarization through the NF-kappaB pathway. J Neuroimmunol (2017) 305:108–14. doi: 10.1016/j.jneuroim.2017.02.010
44. Subedi L, Lee JH, Gaire BP, Kim SY. Sulforaphane inhibits MGO-AGE-Mediated neuroinflammation by suppressing NF-kappaB, MAPK, and AGE-RAGE signaling pathways in microglial cells. Antioxidants (Basel) (2020) 9(9):792. doi: 10.3390/antiox9090792
45. Rivera JC, Sitaras N, Noueihed B, Hamel D, Madaan A, Zhou T, et al. Microglia and interleukin-1beta in ischemic retinopathy elicit microvascular degeneration through neuronal semaphorin-3A. Arterioscler Thromb Vasc Biol (2013) 33(8):1881–91. doi: 10.1161/ATVBAHA.113.301331
46. Yoshida S, Yoshida A, Ishibashi T. Induction of IL-8, MCP-1, and bFGF by TNF-alpha in retinal glial cells: implications for retinal neovascularization during post-ischemic inflammation. Graefes Arch Clin Exp Ophthalmol (2004) 242(5):409–13. doi: 10.1007/s00417-004-0874-2
47. Zhang T, Ouyang H, Mei X, Lu B, Yu Z, Chen K, et al. Erianin alleviates diabetic retinopathy by reducing retinal inflammation initiated by microglial cells via inhibiting hyperglycemia-mediated ERK1/2-NF-kappaB signaling pathway. FASEB J (2019) 33(11):11776–90. doi: 10.1096/fj.201802614RRR
48. Yu Z, Zhang T, Gong C, Sheng Y, Lu B, Zhou L, et al. Erianin inhibits high glucose-induced retinal angiogenesis via blocking ERK1/2-regulated HIF-1alpha-VEGF/VEGFR2 signaling pathway. Sci Rep (2016) 6:34306. doi: 10.1038/srep34306
49. Hachana S, Pouliot M, Couture R, Vaucher E. Diabetes-induced inflammation and vascular alterations in the goto-kakizaki rat retina. Curr Eye Res (2020) 45(8):965–74. doi: 10.1080/02713683.2020.1712730
50. Kang GY, Bang JY, Choi AJ, Yoon J, Lee WC, Choi S, et al. Exosomal proteins in the aqueous humor as novel biomarkers in patients with neovascular age-related macular degeneration. J Proteome Res (2014) 13(2):581–95. doi: 10.1021/pr400751k
51. Jin X, Zhao W, Zhou P, Niu T. YAP knockdown inhibits proliferation and induces apoptosis of human prostate cancer DU145 cells. Mol Med Rep (2018) 17(3):3783–8. doi: 10.3892/mmr.2017.8352
52. Zhang W, Jiang H, Kong Y. Exosomes derived from platelet-rich plasma activate YAP and promote the fibrogenic activity of Muller cells via the PI3K/Akt pathway. Exp Eye Res (2020) 193:107973. doi: 10.1016/j.exer.2020.107973
53. Zhang W, Dong X, Wang T, Kong Y. Exosomes derived from platelet-rich plasma mediate hyperglycemia-induced retinal endothelial injury via targeting the TLR4 signaling pathway. Exp Eye Res (2019) 189:107813. doi: 10.1016/j.exer.2019.107813
54. Hui Y, Yin Y. MicroRNA-145 attenuates high glucose-induced oxidative stress and inflammation in retinal endothelial cells through regulating TLR4/NF-kappaB signaling. Life Sci (2018) 207:212–8. doi: 10.1016/j.lfs.2018.06.005
55. Ye L, Guo H, Wang Y, Peng Y, Zhang Y, Li S, et al. Exosomal circEhmt1 released from hypoxia-pretreated pericytes regulates high glucose-induced microvascular dysfunction via the NFIA/NLRP3 pathway. Oxid Med Cell Longev (2021) 2021:8833098. doi: 10.1155/2021/8833098
56. Lu Y, Zhou Y, Zhang R, Wen L, Wu K, Li Y, et al. Bone mesenchymal stem cell-derived extracellular vesicles promote recovery following spinal cord injury via improvement of the integrity of the blood-spinal cord barrier. Front Neurosci (2019) 13:209. doi: 10.3389/fnins.2019.00209
57. Hu X, Shen N, Liu A, Wang W, Zhang L, Sui Z, et al. Bone marrow mesenchymal stem cell-derived exosomal miR-34c-5p ameliorates RIF by inhibiting the core fucosylation of multiple proteins. Mol Ther (2022) 30(2):763–81. doi: 10.1016/j.ymthe.2021.10.012
58. Kim DJ, Kang JM, Park SH, Kwon HK, Song SJ, Moon H, et al. Diabetes aggravates post-ischaemic renal fibrosis through persistent activation of TGF-beta1 and shh signalling. Sci Rep (2017) 7(1):16782. doi: 10.1038/s41598-017-16977-z
59. Kilari S, Yang B, Sharma A, McCall DL, Misra SJ. Increased transforming growth factor beta (TGF-β) and pSMAD3 signaling in a murine model for contrast induced kidney injury. Sci Rep (2018) 8(1):1–12. doi: 10.1038/s41598-018-24340-z
60. van der Velden JL, Wagner DE, Lahue KG, Abdalla ST, Lam YW, Weiss DJ, et al. TGF-beta1-induced deposition of provisional extracellular matrix by tracheal basal cells promotes epithelial-to-mesenchymal transition in a c-jun NH2-terminal kinase-1-dependent manner. Am J Physiol Lung Cell Mol Physiol (2018) 314(6):L984–97. doi: 10.1152/ajplung.00053.2017
61. Lou H, Wang S, Guo T, Yang Y. Role of miR-21 in rats with proliferative diabetic retinopathy via TGF-β signaling pathway. Eur Rev Med Pharmacol Sci (2019) 23(3 Suppl):9–16. doi: 10.26355/eurrev_201908_18621
62. Xue L, Xiong C, Li J, Ren Y, Zhang L, Jiao K, et al. miR-200-3p suppresses cell proliferation and reduces apoptosis in diabetic retinopathy via blocking the TGF-β2/Smad pathway. Biosci Rep (2020) 40(11):BSR20201545. doi: 10.1042/BSR20201545
63. Fruman DA, Chiu H, Hopkins BD, Bagrodia S, Cantley LC, Abraham RT. The PI3K pathway in human disease. Cell (2017) 170(4):605–35. doi: 10.1016/j.cell.2017.07.029
64. Zhang Z-Z, Qin X-H, Zhang J. Metabolism. MicroRNA-183 inhibition exerts suppressive effects on diabetic retinopathy by inactivating BTG1-mediated PI3K/Akt/VEGF signaling pathway. Am J Physiol Endocrinol Metab (2019) 316(6):E1050–60. doi: 10.1152/ajpendo.00444.2018
65. Usui-Ouchi A, Ouchi Y, Kiyokawa M, Sakuma T, Ito R, Ebihara N. Upregulation of mir-21 levels in the vitreous humor is associated with development of proliferative vitreoretinal disease. PloS One (2016) 11(6):e0158043. doi: 10.1371/journal.pone.0158043
66. Lu JM, Zhang ZZ, Ma X, Fang SF, Qin XH. Repression of microRNA-21 inhibits retinal vascular endothelial cell growth and angiogenesis via PTEN dependent-PI3K/Akt/VEGF signaling pathway in diabetic retinopathy. Exp Eye Res (2020) 190:107886. doi: 10.1016/j.exer.2019.107886
67. Wang L, Liu W, Huang XJE. MicroRNA-199a-3p inhibits angiogenesis by targeting the VEGF/PI3K/AKT signalling pathway in an in vitro model of diabetic retinopathy. Pathol M (2020) 116:104488. doi: 10.1016/j.yexmp.2020.104488
68. Qi R, Liu H, Wang Q, Wang J, Yang F, Long D, et al. Expressions and regulatory effects of P38/ERK/JNK mapks in the adipogenic trans-differentiation of C2C12 myoblasts. Cell Physiol Biochem (2017) 44(6):2467–75. doi: 10.1159/000486169
69. Zhang LQ, Cui H, Yu YB, Shi HQ, Zhou Y, Liu MJ. MicroRNA-141-3p inhibits retinal neovascularization and retinal ganglion cell apoptosis in glaucoma mice through the inactivation of docking protein 5-dependent mitogen-activated protein kinase signaling pathway. J Cell Physiol (2019) 234(6):8873–87. doi: 10.1002/jcp.27549
70. Chen J, Chen J, Cheng Y, Fu Y, Zhao H, Tang M, et al. Mesenchymal stem cell-derived exosomes protect beta cells against hypoxia-induced apoptosis via miR-21 by alleviating ER stress and inhibiting p38 MAPK phosphorylation. Stem Cell Res Ther (2020) 11(1):97. doi: 10.1186/s13287-020-01610-0
71. Dai C, Jiang S, Chu C, Xin M, Song X, Zhao B. Baicalin protects human retinal pigment epithelial cell lines against high glucose-induced cell injury by up-regulation of microRNA-145. Exp Mol Pathol (2019) 106:123–30. doi: 10.1016/j.yexmp.2019.01.002
72. Antzelevitch C. Genetic, molecular and cellular mechanisms underlying the J wave syndromes. Circ J (2012) 76(5):1054–65. doi: 10.1253/circj.CJ-12-0284
73. Chen F, Zhang H-Q, Zhu J, Liu K-Y, Cheng H, Li G-L, et al. Puerarin enhances superoxide dismutase activity and inhibits RAGE and VEGF expression in retinas of STZ–induced early diabetic rats. Asian Pacific J Trop Med (2012) 5(11):891–6. doi: 10.1016/S1995-7645(12)60166-7
74. Yin Y, Chen F, Wang W, Wang H, Zhang X. Resolvin D1 inhibits inflammatory response in STZ-induced diabetic retinopathy rats: Possible involvement of NLRP3 inflammasome and NF-κB signaling pathway. Mol Vis (2017) 23:242.
75. Li R, Yuan H, Zhao T, Yan Y, Liu Z, Cai J, et al. miR-874 ameliorates retinopathy in diabetic rats by NF-kappaB signaling pathway. Adv Clin Exp Med (2021) 30(4):421–30. doi: 10.17219/acem/130602
76. Li W, Jin L, Cui Y, Nie A, Xie N, Liang G. Bone marrow mesenchymal stem cells-induced exosomal microRNA-486-3p protects against diabetic retinopathy through TLR4/NF-kappaB axis repression. J Endocrinol Invest (2021) 44(6):1193–207. doi: 10.1007/s40618-020-01405-3
77. Ye EA, Steinle JJ. miR-146a attenuates inflammatory pathways mediated by TLR4/NF-kappaB and TNFalpha to protect primary human retinal microvascular endothelial cells grown in high glucose. Mediators Inflammation (2016) 2016:3958453. doi: 10.1155/2016/3958453
78. Freeman D, Noren Hooten N, Eitan E, Green J, Mode N, Bodogai M, et al. Altered extracellular vesicle concentration, cargo, and function in diabetes. Diabetes (2018) 67(11):2377–88. doi: 10.2337/db17-1308
79. O'Neill S, Bohl M, Gregersen S, Hermansen K, O'Driscoll L. Blood-based biomarkers for metabolic syndrome. Trends Endocrinol Metab (2016) 27(6):363–74. doi: 10.1016/j.tem.2016.03.012
80. De Rechter S, Decuypere JP, Ivanova E, van den Heuvel LP, De Smedt H, Levtchenko E, et al. Autophagy in renal diseases. Pediatr Nephrol (2016) 31(5):737–52. doi: 10.1007/s00467-015-3134-2
81. Ebrahim N, Ahmed IA, Hussien NI, Dessouky AA, Farid AS, Elshazly AM, et al. Mesenchymal stem cell-derived exosomes ameliorated diabetic nephropathy by autophagy induction through the mTOR signaling pathway. Cells (2018) 7(12):556. doi: 10.3390/cells7120226
82. Zhang X, Song Z, Guo Y, Zhou M. The novel role of TRPC6 in vitamin d ameliorating podocyte injury in STZ-induced diabetic rats. Mol Cell Biochem (2015) 399(1-2):155–65. doi: 10.1007/s11010-014-2242-9
83. Bus P, Scharpfenecker M, van der Wilk P, Wolterbeek R, Bruijn JA, Baelde HJ. The VEGF-a inhibitor sFLT-1 improves renal function by reducing endothelial activation and inflammation in a mouse model of type 1 diabetes. Diabetologia (2017) 60(9):1813–21. doi: 10.1007/s00125-017-4322-3
84. Duan YR, Chen BP, Chen F, Yang SX, Zhu CY, Ma YL, et al. Exosomal microRNA-16-5p from human urine-derived stem cells ameliorates diabetic nephropathy through protection of podocyte. J Cell Mol Med (2021) 25(23):10798–813. doi: 10.1111/jcmm.14558
85. Sahoo S, Losordo DW. Exosomes and cardiac repair after myocardial infarction. Circ Res (2014) 114(2):333–44. doi: 10.1161/CIRCRESAHA.114.300639
86. Cheng Y, Wang X, Yang J, Duan X, Yao Y, Shi X, et al. A translational study of urine miRNAs in acute myocardial infarction. J Mol Cell Cardiol (2012) 53(5):668–76. doi: 10.1016/j.yjmcc.2012.08.010
87. Mao Q, Liang XL, Zhang CL, Pang YH, Lu YX. LncRNA KLF3-AS1 in human mesenchymal stem cell-derived exosomes ameliorates pyroptosis of cardiomyocytes and myocardial infarction through miR-138-5p/Sirt1 axis. Stem Cell Res Ther (2019) 10(1):393. doi: 10.1186/s13287-019-1522-4
88. Wang X, Ha T, Zou J, Ren D, Liu L, Zhang X, et al. MicroRNA-125b protects against myocardial ischaemia/reperfusion injury via targeting p53-mediated apoptotic signalling and TRAF6. Cardiovasc Res (2014) 102(3):385–95. doi: 10.1093/cvr/cvu044
89. Zhu LP, Tian T, Wang JY, He JN, Chen T, Pan M, et al. Hypoxia-elicited mesenchymal stem cell-derived exosomes facilitates cardiac repair through miR-125b-mediated prevention of cell death in myocardial infarction. Theranostics (2018) 8(22):6163–77. doi: 10.7150/thno.28021
90. Davidson SM, Padro T, Bollini S, Vilahur G, Duncker DJ, Evans PC, et al. Progress in cardiac research: from rebooting cardiac regeneration to a complete cell atlas of the heart. Cardiovasc Res (2021) 117(10):2161–74. doi: 10.1093/cvr/cvab200
91. Xiao M, Li Q, Feng H, Zhang L, Chen Y. Neural vascular mechanism for the cerebral blood flow autoregulation after hemorrhagic stroke. Neural Plast (2017) 2017:5819514. doi: 10.1155/2017/5819514
92. Otero-Ortega L, Laso-Garcia F, Gomez-de Frutos M, Fuentes B, Diekhorst L, Diez-Tejedor E, et al. Role of exosomes as a treatment and potential biomarker for stroke. Transl Stroke Res (2019) 10(3):241–9. doi: 10.1007/s12975-018-0654-7
93. Otero-Ortega L, Gomez de Frutos MC, Laso-Garcia F, Rodriguez-Frutos B, Medina-Gutierrez E, Lopez JA, et al. Exosomes promote restoration after an experimental animal model of intracerebral hemorrhage. J Cereb Blood Flow Metab (2018) 38(5):767–79. doi: 10.1177/0271678X17708917
94. Ma X, Wang J, Li J, Ma C, Chen S, Lei W, et al. Loading MiR-210 in endothelial progenitor cells derived exosomes boosts their beneficial effects on Hypoxia/Reoxygeneation-injured human endothelial cells via protecting mitochondrial function. Cell Physiol Biochem (2018) 46(2):664–75. doi: 10.1159/000488635
95. Liu XL, Wang G, Song W, Yang WX, Hua J, Lyu L. microRNA-137 promotes endothelial progenitor cell proliferation and angiogenesis in cerebral ischemic stroke mice by targeting NR4A2 through the notch pathway. J Cell Physiol (2018) 233(7):5255–66. doi: 10.1002/jcp.26312
96. Zampetaki A, Kiechl S, Drozdov I, Willeit P, Mayr U, Prokopi M, et al. Plasma microRNA profiling reveals loss of endothelial miR-126 and other microRNAs in type 2 diabetes. Circ Res (2010) 107(6):810–7. doi: 10.1161/CIRCRESAHA.110.226357
97. Jimenez-Lucena R, Camargo A, Alcala-Diaz JF, Romero-Baldonado C, Luque RM, van Ommen B, et al. A plasma circulating miRNAs profile predicts type 2 diabetes mellitus and prediabetes: from the CORDIOPREV study. Exp Mol Med (2018) 50(12):1–12. doi: 10.1038/s12276-018-0194-y
98. Jimenez-Lucena R, Rangel-Zuniga OA, Alcala-Diaz JF, Lopez-Moreno J, Roncero-Ramos I, Molina-Abril H, et al. Circulating miRNAs as predictive biomarkers of type 2 diabetes mellitus development in coronary heart disease patients from the CORDIOPREV study. Mol Ther Nucleic Acids (2018) 12:146–57. doi: 10.1016/j.omtn.2018.05.002
99. Barutta F, Bellini S, Mastrocola R, Bruno G, Gruden G. MicroRNA and microvascular complications of diabetes. Int J Endocrinol (2018) 2018:6890501. doi: 10.1155/2018/6890501
100. Martinez B, Peplow PV. MicroRNAs as biomarkers of diabetic retinopathy and disease progression. Neural Regener Res (2019) 14(11):1858–69. doi: 10.4103/1673-5374.259602
101. Mammadzada P, Bayle J, Gudmundsson J, Kvanta A, Andre H. Identification of diagnostic and prognostic microRNAs for recurrent vitreous hemorrhage in patients with proliferative diabetic retinopathy. J Clin Med (2019) 8(12):2217. doi: 10.3390/jcm8122217
102. Chen S, Yuan M, Liu Y, Zhao X, Lian P, Chen Y, et al. Landscape of microRNA in the aqueous humour of proliferative diabetic retinopathy as assessed by next-generation sequencing. Clin Exp Ophthalmol (2019) 47(7):925–36. doi: 10.1111/ceo.13554
103. Liu Y, Xiao J, Zhao Y, Zhao C, Yang Q, Du X, et al. microRNA-216a protects against human retinal microvascular endothelial cell injury in diabetic retinopathy by suppressing the NOS2/JAK/STAT axis. Exp Mol Pathol (2020) 115:104445. doi: 10.1016/j.yexmp.2020.104445
104. Zeng Y, Cui Z, Liu J, Chen J, Tang S. MicroRNA-29b-3p promotes human retinal microvascular endothelial cell apoptosis via blocking SIRT1 in diabetic retinopathy. Front Physiol (2019) 10:1621. doi: 10.3389/fphys.2019.01621
Keywords: Diabetic retinopathy, pericyte, microRNA, exosomes, treatment
Citation: Niu S-r, Hu J-m, Lin S and Hong Y (2022) Research progress on exosomes/microRNAs in the treatment of diabetic retinopathy. Front. Endocrinol. 13:935244. doi: 10.3389/fendo.2022.935244
Received: 03 May 2022; Accepted: 11 July 2022;
Published: 09 August 2022.
Edited by:
Michele Lanza, University of Campania Luigi Vanvitelli, ItalyReviewed by:
Bertrand Kaeffer, Institut National de recherche pour l’agriculture, l’alimentation et l’environnement (INRAE), FranceEleni Anastasiadou, Sapienza University of Rome, Italy
Copyright © 2022 Niu, Hu, Lin and Hong. This is an open-access article distributed under the terms of the Creative Commons Attribution License (CC BY). The use, distribution or reproduction in other forums is permitted, provided the original author(s) and the copyright owner(s) are credited and that the original publication in this journal is cited, in accordance with accepted academic practice. No use, distribution or reproduction is permitted which does not comply with these terms.
*Correspondence: Shu Lin, c2h1bGluMTk1NkAxMjYuY29t; Yu Hong, MTU5ODU5OTgwMDhAMTYzLmNvbQ==
†ORCID: Shu Lin, orcid.org/0000-0002-4239-2028