- 1Department of Molecular Medicine, Atomic Bomb Disease Institute and Nagasaki University Graduate School of Biomedical Sciences, Nagasaki, Japan
- 2Department of General Medicine, Nagasaki University Graduate School of Biomedical Sciences, Nagasaki, Japan
- 3Division of Cancer Biology, National Cancer Center Research Institute, Tokyo, Japan
Mitochondria-eating protein (MIEAP) is a molecule important for non-canonical mitophagy and thought to be a tumor suppressor. Our previous study found that MIEAP expression is defective in thyroid oncocytomas, irrespective of being benign or malignant, and also in non-oncocytic thyroid cancers. Thyroid oncocytomas are composed of large polygonal cells with eosinophilic cytoplasm that is rich in abnormal mitochondria. Thus, our data indicate that, together with increased mitochondrial biogenesis that compensates for the dysfunction of the mitochondria, MIEAP plays a critical role in the accumulation of mitochondria in thyroid oncocytic tumors, whereas a defective MIEAP expression alone is not sufficient for mitochondrial accumulation in non-oncocytic cancers with normal mitochondria. To clarify whether MIEAP is a tumor suppressor in the thyroids and whether MIEAP knockout (KO) alone is sufficient for the oncocytic phenotype and also to extend our effort toward canonical mitophagy (a selective autophagy), we here conducted mouse studies using genetically engineered mice. BrafCA/wt mice developed thyroid cancers 1 year after intrathyroidal injection of adenovirus expressing Cre, while cancer development was observed at 6 months in adenovirus-Cre-injected BrafCA/wt;MieapKO/KO and BrafCA/wt;Atg5flox/flox mice [where autophagy-related 5 (ATG5) is a component of autophagic machinery], although KO of either molecule alone was not sufficient for cancer development. These data demonstrate that MIEAP or ATG5 KO accelerated thyroid cancer development. However, cancers in adenovirus-Cre-injected BrafCA/wt;MieapKO/KO and BrafCA/wt;Atg5flox/flox mice were not oncocytic. In conclusion, we here show that MIEAP and ATG5 are both tumor suppressors in thyroid carcinogenesis, but as we have anticipated from our previous data, KO of either molecule does not confer the oncocytic phenotype to BRAFV600E-positive thyroid cancers. The combination of disruptive mitochondrial function and impaired mitochondrial quality control may be necessary to establish a mouse model of thyroid oncocytoma.
Introduction
Thyroid oncocytic tumors (also called eosinophilic or oxyphilic tumors) are characterized by large polygonal cells with eosinophilic cytoplasm (1). These tumors contain abnormally enlarged mitochondria in excess and frequently have mutations in mitochondrial DNA (mtDNA) encoding complex I of the electron transport chain (2). Therefore, the mitochondria accumulated are dysfunctional.
Although it has previously been reported that mitochondrial accumulation is due to compensated increase in mitochondrial biogenesis (3–6), abnormal mitochondria are usually eliminated by a mitochondrial quality control system. Mitophagy, a type of selective autophagy, is included in this system (hereafter called “canonical mitophagy”). A thyroid oncocytic carcinoma cell line XTC.UC1 (7) has been found to have a loss–of–function mutation (V380L) in PARK2, causing defective canonical mitophagy (8). However, the same mutation exists only in one of seven oncocytic cell carcinomas, and no mutations have been found in genes coding the components of canonical mitophagy machinery in thyroid oncocytic carcinomas (9–11).
Recently, another mitochondrial quality control system has been found, in which mitochondria–eating protein (MIEAP) plays an indispensable role (called “non–canonical mitophagy”) (12). The expression of MIEAP has been reported to be suppressed by the methylation of its promoter in a fraction of colon, breast, and gastric cancers (13–16). Gastric cancer cell lines with defective MEAP expression are more invasive in a hypoxic condition than those with intact MIEAP expression (17), and gastric and breast cancer cell lines overexpressing MIEAP die through apoptosis (14, 15). Breast cancer patients with a higher MIEAP expression have a better prognosis than those with a lower expression (16). Furthermore, in a mouse model, ApcMin/+,MieapKO/KO mice show more aggressive intestinal lesions than ApcMin/+ mice (18). Thus, MIEAP is now thought to be a tumor suppressor.
We have recently found that MIEAP was expressed in human thyroid non–oncocytic (conventional) follicular adenomas (FAs), but not in oncocytic FAs and conventional and oncocytic carcinomas (19), and therefore postulated that loss of MIEAP expression and a compensatory increase in mitochondria biogenesis induce the accumulation of mitochondria in thyroid oncocytic adenomas and cancers, however, in thyroid conventional cancers, the mitochondria are not accumulated despite defective MIEAP expression because of no compensatory increase in mitochondrial biogenesis. Functionally, in XTC.UC1 cells in which the expression of MIEAP is also suppressed, exogenously induced MIEAP reduced the amounts of abnormal mitochondria, as indicated by decreased reactive oxygen species levels, mtDNA/nuclear DNA ratios, and cytoplasmic acidification.
To further explore the roles of MIEAP in the oncocytic phenotype of thyroid tumors and in the carcinogenesis of thyroid cancers in general (that is, both oncocytic and conventional cancers) as a tumor suppressor, we utilized a mouse model of BRAFV600E–positive thyroid cancer that we have previously established. In this model, BrafCA mice developed thyroid cancers 1 year after intrathyroidal injection of adenovirus expressing Cre DNA recombinase (20). MIEAP–defective BrafCA mice were generated (BrafCA/wt,MieapKO/KO), and their thyroid tumorigenicity and oncocytic phenotype were compared with those in BrafCA/wt and MieapKO/KO mice. Moreover, to extend our effort toward the study on the role of canonical autophagy in thyroid carcinogenesis, the conditional autophagy–related 5 (ATG5)–defective (Atg5flox/flox) mice were also employed and used to generate BrafCA/wt,Atg5flox/flox mice.
Materials and methods
Generation of BrafCA/wt,MieapKO/KO and BrafCA/wt,Atg5flox/flox mice
BrafCA (B6.129P2(Cg)–Braftm1Mmcm/J, stock# 017837) mice (21), having Cre–activating BrafV600E, were obtained from Jackson Laboratory (Bar Harbor, ME, USA). MieapKO mice have previously been generated (18). Atg5flox mice, in which exon 3 of the Atg5 gene is flanked by two loxP sequences, have originally been generated by N. Mizushima (22) and were provided through the RIKEN Bioresource Center (Tsukuba, Japan, http://en.brc.riken.jp/).
BrafCA/wt mice were crossed with MieapKO/KO or Atg5flox/flox, generating BrafCA/wt,MieapKO/wt or BrafCA/wt,Atg5flox/wt mice, which were subsequently backcrossed to MieapKO/KO or Atg5flox/flox mice to produce BrafCA/wt,MieapKO/KO or BrafCA/wt,Atg5flox/flox mice, respectively. Upon intrathyroidal Cre DNA recombinase expression (that is, injection of Ad–TgP–Cre (23) in our case, see below), the thyroids of these mice express BRAFV600E and lose ATG5 expression.
Experimental designs
Surgery and injection of adenovirus into the thyroid left lobes of 4– to 6–week–old mice were performed as previously described (20, 23). The adenovirus used in this study was Ad–TgP–Cre, in which Cre DNA recombinase is expressed under the control of a thyroid–specific thyroglobulin (Tg) promoter (23). A total of 3–4 × 109 adenovirus particles/mouse were injected. Ad–TgP–Cre–injected BrafCA/wt and Atg5flox/flox mice were designated as Brafthyr–V600E and Atg5thyr–KO/KO mice, respectively. The male–to–female mice ratio was approximately 1:1 in all the experimental groups. After 6 and 12 months, mice were anesthetized with isoflurane, blood was collected via cardiac tap, and mice were euthanized by cervical dislocation. For histological examinations, the thyroids were removed from all mice.
Animal care and all experimental procedures were performed in accordance with the Guideline for Animal Experimentation of Nagasaki University with the approval of the Institutional Animal Care and Use Committee (permission number: 1803191437). All surgeries were performed under isoflurane anesthesia, and every effort was made to minimize suffering.
Hematoxylin and eosin staining and immunohistochemistry
Tissues were fixed in 10% neutral–buffered formalin and then embedded in paraffin. Sections (4 μm thick) were prepared and stained with hematoxylin and eosin (H&E) or immunostained with primary antibodies: rabbit monoclonal anti–Tg (ab156008, Abcam, Cambridge, UK, dilution of 1:250), rabbit monoclonal anti–Ki–67 (ab66155, Abcam, dilution of 1:100), rabbit polyclonal anti–tumor suppressor P53–binding protein 1 (53BP1) (A300–272A, Bethyl Laboratories, Montgomery, TX, USA, dilution of 1:200), rabbit monoclonal anti–MIEAP (ab180154, Abcam, dilution of 1:400), and rabbit polyclonal anti–TOMM20 (translocase of outer mitochondrial membrane 20) (11802–1–AP, Proteintech, Tokyo, Japan, dilution of 1:250). Immunohistochemistry was performed with an ImmPRESS® HRP horse anti–rabbit IgG PLUS Polymer Kit, Peroxidase (MP–7801, Vector Laboratories, Burlingame, CA, USA) for Tg and Ki–67 and the 488–conjugated goat polyclonal anti–rabbit IgG (A11008, Life Technologies, Tokyo, Japan, dilution of 1:200) for 53BP1, MIEAP, and TOMM20. Slides were analyzed using an All–in–One BZ–9000 Fluorescence Microscope (Keyence, Osaka, Japan), and the fluorescent intensity was quantified using a BZ–II Analyzer (Keyence). At least 100 cells were evaluated in each sample to measure the fluorescent intensities of TOMM20 and the number of Ki–67 and 53BP1 foci.
Evaluation of apoptosis
TUNEL staining was performed with an Apop–tag™ Fluorescein Direct in situ Apoptosis Detection Kit (Merck Millipore, Darmstadt, Germany). The slides were embedded with a VECTASHIELD Mounting Medium containing 4′,6–diamidino–2–phenylindole (Vector Laboratories, Burlingame, CA, USA) and analyzed using an All–in–One BZ–9000 Fluorescence Microscope. At least 100 cells were evaluated in each sample to determine the percentages of TUNEL–positive cells.
Evaluation of the prognostic significance of MIEAP and ATG5
The prognostic values of MIEAP and ATG5 expression levels were evaluated with The Human Protein Atlas (https://www.proteinatlas.org/), which is connected to The Cancer Genome Atlas (TCGA) dataset (https://www.cancer.gov/about–nci/organization/ccg/research/structural–genomics/tcga).
Statistical analyses
All data were expressed as means ± or SE and analyzed for significant differences using the Student’s t–test. A P–value of less than 0.05 was considered statistically significant.
Results
To investigate the role of MIEAP and ATG5 in thyroid carcinogenesis, we prepared Brafthyr–V600E, MieapKO/KO, Atg5thyr–KO/KO, Brafthyr–V600E,MieapKO/KO, and Brafthyr–V600E,Atg5thyr–KO/KO mice. Mice were subjected to intrathyroidal injection of Ad–TgP–Cre at 4 to 6 weeks and sacrificed at 6 and 12 months. Macroscopically, the thyroids of MieapKO/KO and Atg5thyr–KO/KO mice were normal at 6 and 12 months, and those of most of Brafthyr–V600E mice were enlarged at 12, but not 6, months as we have previously reported (20). In Brafthyr–V600E,MieapKO/KO and Brafthyr–V600E,Atg5thyr–KO/KO mice, thyroid enlargements were detected at not only 12 but also 6 months (Table 1). The representative pictures of the thyroids in non–injected and injected Brafthyr–V600E,MieapKO/KO mice at 12 months are shown in Figure 1A. The thyroid weights of each mouse group are shown in Figure 1B. There was no difference in the thyroid left lobe weights among the control, Brafthyr–V600E,MieapKO/KO, and Brafthyr–V600E,Atg5thyr–KO/KO mice at 6 months, but the injected left lobes were significantly heavier in Brafthyr–V600E and Brafthyr–V600E,Mieap–KO/KO mice than the control at 12 months (Figure 1B). The injected left lobes of Brafthyr–V600E,Atg5thyr–KO/KO mice tended to be only slightly (but not significantly) heavier than the control lobes.
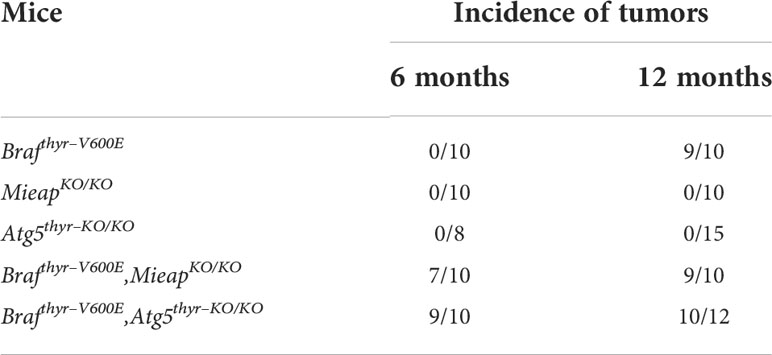
Table 1 Summary of incidences of thyroid tumors in Brafthyr–V600E, MieapKO/KO, Atg5thyr–KO/KO, Brafthyr–V600E,MieapKO/KO, and Brafthyr–V600E,Atg5thyr–KO/KO mice.
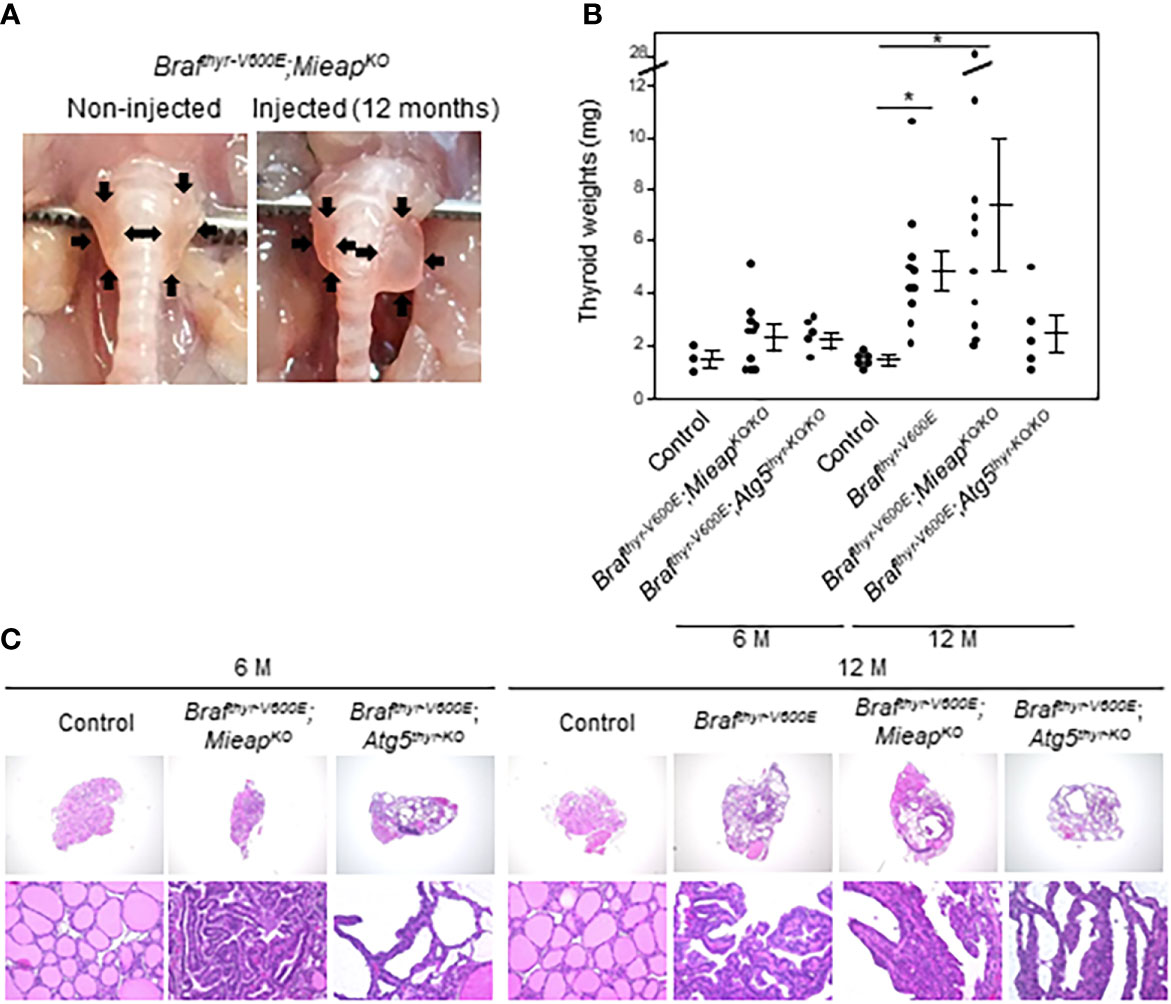
Figure 1 Gross appearance (A), thyroid left lobe weights (B), and histology (C) in control, Brafthyr–V600E, Brafthyr–V600E,MieapKO, and Brafthyr–V600E,Atg5thyr–KO/KO mice. Mice were sacrificed at 6 and 12 months. The thyroid glands were removed, and thyroid weights and histology were determined as described in Materials and methods. Data are shown as black circles for individual mice and as means ± SE. *P < 0.05. M, months. The original magnifications were ×40 and ×400.
In H&E staining, all the enlarged thyroid left lobes of Brafthyr–V600E mice at 12 months and of Brafthyr–V600E,MieapKO/KO and Brafthyr–V600E,Atg5thyr–V600E mice at 6 and 12 months comparably showed papillary growth of atypical follicular cells exhibiting hyperchromatic irregular–shaped nuclei and intranuclear cytoplasmic inclusions and follicular and nestic growth of atypical follicular cells with severe nuclear atypia as we have previously detected in Brafthyr–V600E mice (20) (Figure 1C and data not shown). Again, the thyroids of MieapKO/KO and Atg5thyr–KO/KO mice were normal at 6 and 12 months. Thus, Brafthyr–V600E,MieapKO/KO and Brafthyr–V600E,Atg5thyr–KO/KO mice developed thyroid cancers earlier than Brafthyr–V600E mice.
The thyroid tissues obtained at 12 months were examined in detail. All the cancers in the three groups (Brafthyr–V600E, Brafthyr–V600E,MieapKO/KO, and Brafthyr–V600E,Atg5thyr–KO/KO mice) showed comparable Tg expression levels with heterogenous expression patterns (Figure 2), indicating that the differentiation status is similar in these groups. The percentages of Ki–67–positive and TUNEL–positive cells, i.e., proliferating and apoptotic cells, respectively, were significantly higher in tumors of the three groups than those of the control (Figures 3A–C). The percentages of TUNEL–positive cells in the tumors of Brafthyr–V600E,MieapKO/KO mice were significantly higher than those of Brafthyr–V600E mice. We also evaluated ROS–induced genomic damages by 53BP1 staining as ROS markers because it has been reported that abnormal mitochondria produce intracellular reactive oxygen species (ROS) (19, 24). 53BP1 accumulates at the DNA double–strand break sites (25). The number of 53BP1 foci was higher in the tumors of the three groups than that of the control (Figures 3A, D), and this number in Brafthyr–V600E,MieapKO/KO mice was significantly higher than that in Brafthyr–V600E mice. Knockout (KO) of MIEAP appears to induce higher ROS than that of ATG5, causing higher 53BP1 foci and also presumably higher apoptotic cells. Finally, BRAFV600E and ATG5 KO did not affect MIEAP expression (Figure 3A).
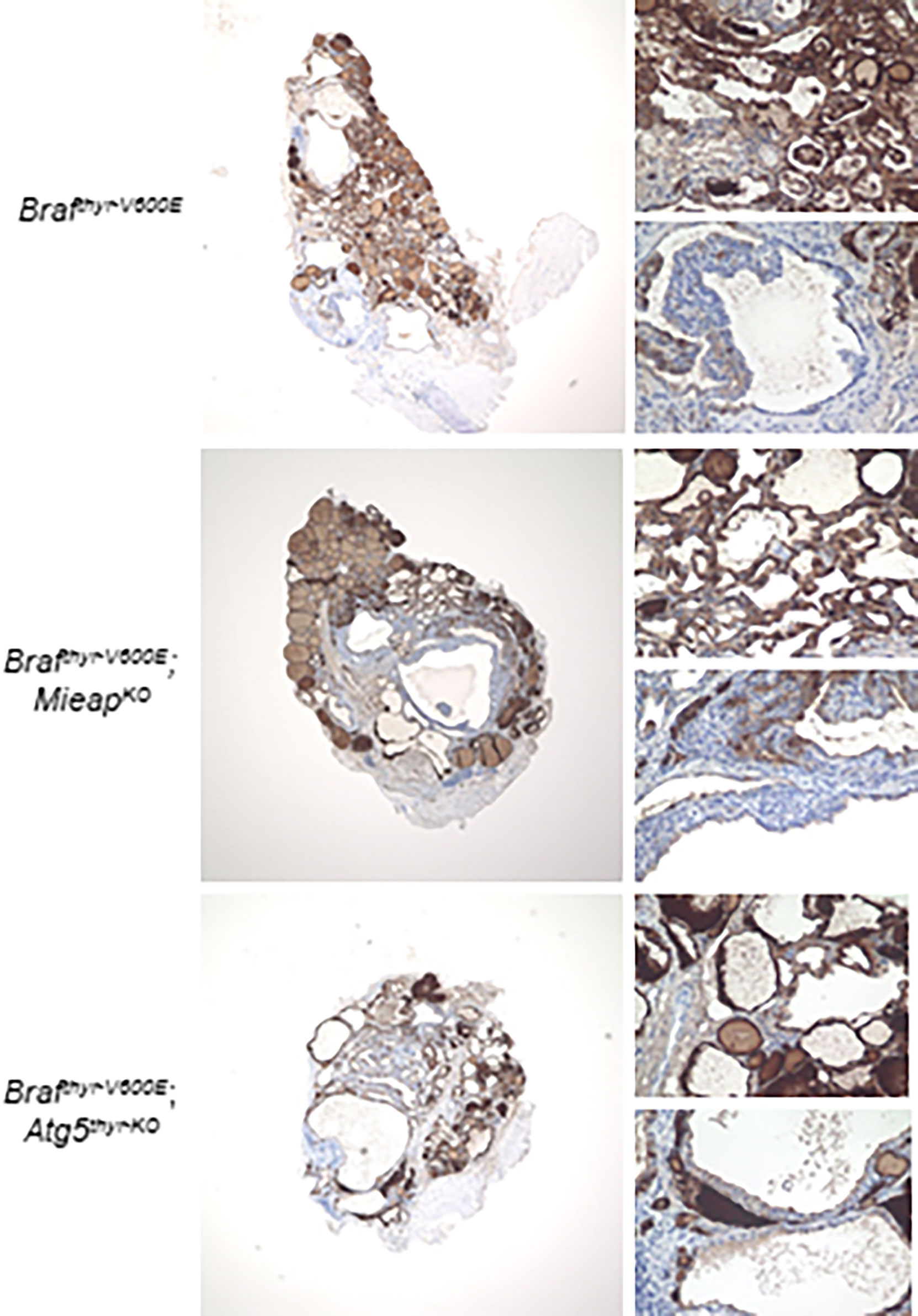
Figure 2 Immunohistochemistry (IHC) staining for thyroglobulin (Tg) expression in the Brafthyr–V600E, Brafthyr–V600E,MieapKO/KO, and Brafthyr–V600E,Atg5thyr–KO/KO mice at 12 months. The upper– and lower–right panels in each tissue depict normal and defective Tg expression areas, respectively. The thyroid glands obtained in Figure 1 were used for Tg staining as described in Materials and methods. The original magnifications were ×40 and ×400.
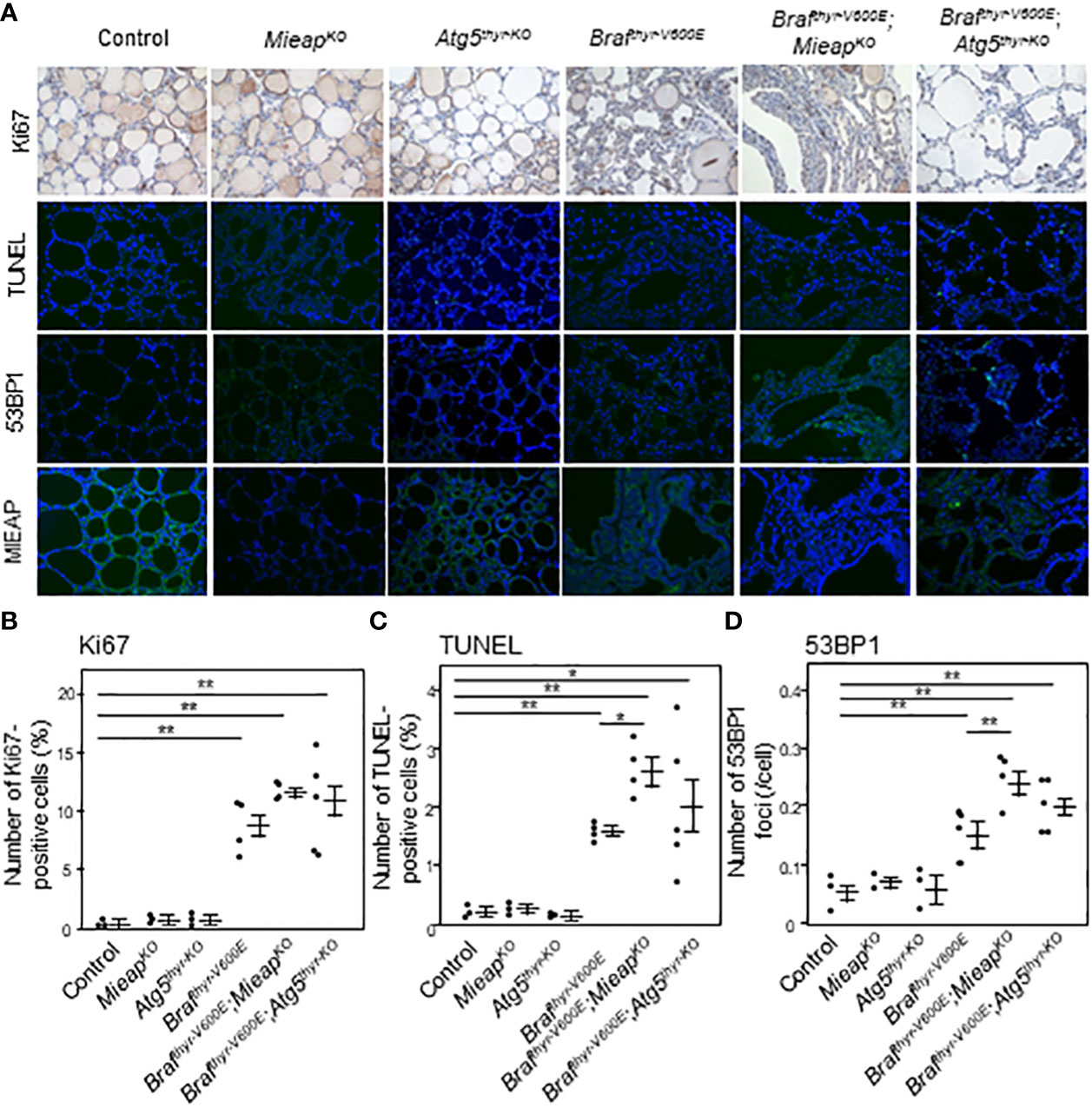
Figure 3 IHC staining for Ki67, TUNEL, 53BP1, and MIEAP expression (A) and quantification of Ki67–positive cells (B), TUNEL–positive cells (C), and 53BP1 foci (D) in the thyroids from control, MieapKO/KO, Atg5thyr–KO/KO, Brafthyr–V600E, Brafthyr–V600E,MieapKO/KO, and Brafthyr–V600E, Atg5thyr–KO/KO mice at 12 months. The thyroid tissues obtained in Figure 1 were used for IHC as described in Materials and methods. The original magnifications were ×400. Data are shown as black circles for individual mice and as means ± SE. *P < 0.05, **P < 0.01. M, months.
MIEAP and ATG5 play a role in mitochondrial quality control (i.e., elimination of aging, unhealthy mitochondria) as previously reported (24, 26). Therefore, the cytoplasmic accumulation of mitochondria was evaluated by immunostaining with TOMM20. Although the expression was highly heterogenous, the average expression levels were comparable in the three groups (Figures 4A, B). Thus, a loss of non–canonical mitophagy (MIEAP KO) or of canonical mitophagy/autophagy (ATG5 KO) by itself is not sufficient for mitochondrial accumulation, that is, the oncocytic phenotype.
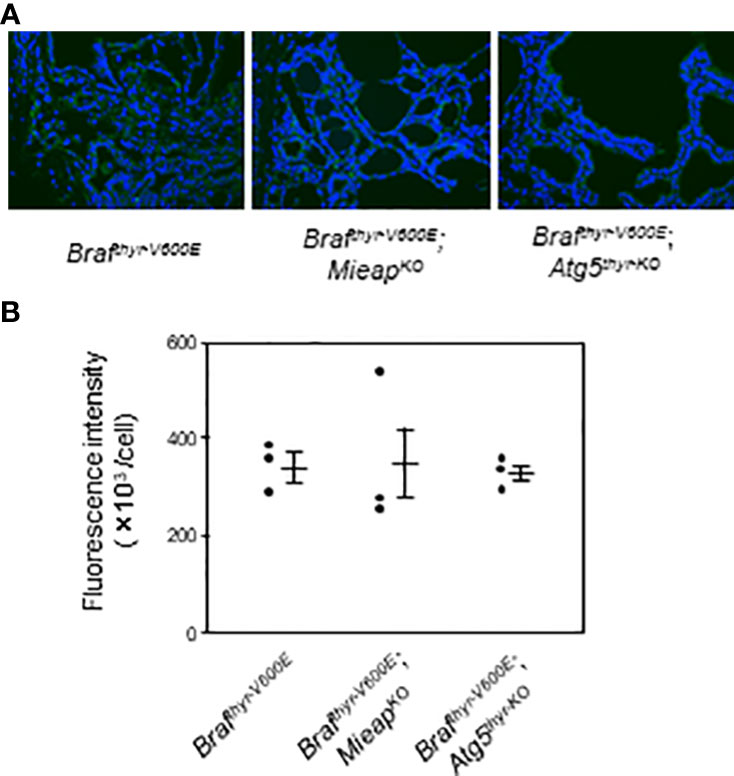
Figure 4 IHC staining for TOMM20 expression (A) and quantification of fluorescence intensities (B) in the thyroids from Brafthyr–V600E, Brafthyr–V600E,MieapKO/KO, and Brafthyr–V600E, Atg5thyr–KO/KO mice. The thyroid tissues obtained in Figure 1 were used for IF as described in Materials and methods. The original magnifications were ×400. Data are shown as black circles for individual mice and as means ± SE. M, months.
Finally, prognostic analysis of MIEAP and ATG5 expression levels using the Human Protein Atlas and TCGA dataset revealed that these two molecules are not prognostic in papillary thyroid cancers (PTCs) (data not shown).
Discussion
A mitochondrial quality control system, which eliminates abnormal/unhealthy mitochondria, includes canonical mitophagy (a selective type of autophagy) and non–canonical mitophagy. We first examined whether MIEAP, a molecule involved in non–canonical mitophagy, and ATG5, a component of autophagic machinery and involved in canonical mitophagy, are tumor suppressors in thyroid carcinogenesis using genetically engineered mice and found that thyroid cancer development was accelerated in Brafthyr–V600E,Atg5thyr–KO/KO and Brafthyr–V600E,MieapKO/KO mice compared with Brafthyr–V600E mice. These results demonstrate that both ATG5 and MIEAP are indeed tumor suppressors and are consistent with previous studies showing an acceleration of development of lung tumors in lox–stop–lox–KrasG12D,Atg5flox/flox or 7flox/flox and lox–stop–lox–BrafV600E,Atg7flox/flox mice intranasally delivered with adenovirus expressing Cre (26–28) and of colon cancers in ApcMin,MieapKO/KO mice (18). Although the components of the autophagic machinery have multiple functions [including non–selective and selective autophagy and also autophagy–independent functions (29)], mitophagy, a common function of ATG5 and MIEAP, appears to play a role in their tumor–suppressive function. Impairment of mitophagy causes the accumulation of aging, abnormal/unhealthy mitochondria. In this regard, Smith et al. (30) showed accelerated tumor formation with enhanced cell proliferation and reduced apoptosis in a mouse model of colorectal cancers with Lgr5–creER,Apcflox/flox,PolgAmut/mut mice (PolgAmut/mut mice are a model of accelerated mtDNA mutagenesis) compared with Lgr5–creER,Apcflox/flox mice. Most of the tumors in the former were complex I–deficient. Furthermore, the growth of adenoma organoids from Lgr5–creER,Apcflox/flox mice was enhanced by metformin, a complex I inhibitor, confirming that the pharmacological inhibition of complex I can also enhance colon adenoma organoid growth.
In human studies, heteroplasmic germline mtDNA mutations causing complex I dysfunction have shown to shift to homoplasmy in a nasopharyngeal oncocytoma, indicating a selective growth advantage of complex I mutations (31). Omics studies [including genomic (nuclear and mitochondrial) and metabolic landscapes] in renal oncocytoma have indicated that mtDNA mutations are early genetic events, and thereby driver mutations (32). Human colorectal adenoma and adenocarcinomas have been reported to have decreased levels or absence of OXPHOS subunit expression and higher complex I mutations compared to normal tissues (30). In colorectal epithelia, mtDNA mutations causing an OXPHOS defect increase with aging and are enriched during the colorectal tumorigenesis process, suggesting the metabolic benefits of mtDNA mutations in colorectal carcinogenesis. All these data also suggest the important role of mtDNA mutations causing mitochondrial functional defect for tumorigenic process and emphasize the tumor–suppressive function of normal mitochondria.
However, no tumor development in the thyroids of MieapKO/KO and Atg5thyr–KO/KO mice in this study and also in the lungs or colons in the above studies (adenovirus expressing Cre–treated Atg5/7flox/flox and MieapKO/KO mice) suggests that MIEAP or ATG5/7 KO alone is not sufficient for tumor initiation in the thyroids, lungs, and colons. In contrast, benign liver tumor development has been demonstrated in systematic mosaic Atg5KO/KO and liver–specific Atg7KO/KO mice (33, 34). Thus, whether the defective expression of certain proteins involved in mitochondrial quality control causing mitochondrial dysfunction can by itself drive cellular transformation or not may be dependent on the tissues examined.
Thyroid cancers developed in Brafthyr–V600E, Brafthyr–V600E,Atg5thyr–KO/KO, and Brafthyr–V600E,MieapKO/KO mice are similar in terms of the degree of cell proliferation and differentiated status, but tumor sizes and ROS levels indicated by 53BP1 foci were the highest in Brafthyr–V600E,MieapKO/KO mice. Thus, mitochondrial damage appear to be higher in Brafthyr–V600E,MieapKO/KO than Brafthyr–V600E,Atg5thyr–KO/KO mice, suggesting that the role of MIEAP in mitochondrial quality control may be more crucial than that of ATG5.
In addition, we have reported that the expression of MIEAP is suppressed in thyroid conventional cancers in humans (19). However, thyroid cancers in Brafthyr–V600E mice in this study preserved MIEAP expression. The mechanism(s) for defective MIEAP in human thyroid non–oncocytic cancers, although unknown, may be specific for humans.
The second purpose of this study was to see whether MIEAP or ATG5 KO causes the oncocytic phenotype in BRAFV600E–derived thyroid cancers. As mentioned in the Introduction, we have previously postulated that defective MIEAP expression and increased mitochondrial biogenesis which compensates impaired mitochondrial function are necessary for mitochondrial accumulation (19). Therefore, it is reasonable that the amounts of mitochondria were not increased in thyroid cancers in Brafthyr–V600E,Atg5thyr–KO/KO and Brafthyr–V600E,MieapKO/KO mice in this study. In contrast, previous studies with mouse models of oncogene–driven cancers have shown that oncogene–derived adenomas did not progress to cancers and were instead diverted to indolent benign oncocytic tumors, indicating the essentiality of autophagy for the progression of benign tumors to a more malignant state (see ref (33). for a review). For example, in the lung cancer models of Cre–activatable KrasG12D or BrafV600E, the conditional KO of ATG5 or ATG7 accelerated tumor initiation as mentioned above, which were, however, diverted to benign oncocytoma (26–28). Thus, the conversion of lung adenoma to benign oncocytoma by inhibiting autophagy is considered a potential anticancer strategy. Accumulation of deformed and dysfunctional mitochondria was also observed in liver adenomas in liver–specific Atg7KO/KO mice mentioned above (34, 35). Again, whether defective mitochondrial function alone can cause the oncocytic phenotype or not may be dependent on the tissues examined.
It should be noted here that, clinically, oncocytomas are usually benign in many tissues, with the thyroid being an exception (36). We could not confirm this difference in this study because thyroid tumors developed in Brafthyr–V600E,Atg5thyr–KO/KO and Brafthyr–V600E,MieapKO/KO mice were not oncocytic. We need a further study with Brafthyr–V600E,Atg5thyr–KO/KO and Brafthyr–V600E,MieapKO/KO mice with mtDNA mutations.
In conclusion, we here show that MIEAP or ATG5 KO accelerates the development of thyroid cancers in a mouse model of BRAFV600E–mediated thyroid cancer. Although MIEAP or ATG5 KO did not alter the cell proliferation rate or differentiation status in mice and their expression levels are not related to prognosis in human PTCs, our results suggest that these molecules are tumor suppressors. As mentioned above, MIEAP expression is defective not only in oncocytic thyroid tumors but also in conventional thyroid cancers in which BRAFV600E is the main driver mutation, and our results indicate that mitophagy is critical for suppressing carcinogenesis in thyroid cancers in general. However, KO of either molecule alone was not sufficient for the oncocytic phenotype of BRAFV600E–mediated thyroid cancers. To establish a mouse model of thyroid oncocytoma, a combination of disruptive mitochondrial function and impaired mitochondrial quality control will be necessary.
Data availability statement
The original contributions presented in the study are included in the article/supplementary material. Further inquiries can be directed to the corresponding author.
Ethics statement
This study was reviewed and approved by The Institutional Animal Care and Use Committee at Nagasaki University.
Author contributions
Conception and design: YN and HA. Administrative support: YN. Provision of study materials: YaN. Collection and assembly of data: KH, TK, and MS. Data analysis and interpretation: KH and YN. All authors contributed to the article and approved the submitted version.
Funding
This work was supported in part by a Grants–in–Aid for Scientific Research (19K090282) from the Ministry of Education, Culture, Sports, Science and Technology, Tokyo, Japan.
Conflict of interest
The authors declare that the research was conducted in the absence of any commercial or financial relationships that could be construed as a potential conflict of interest.
Publisher’s note
All claims expressed in this article are solely those of the authors and do not necessarily represent those of their affiliated organizations, or those of the publisher, the editors and the reviewers. Any product that may be evaluated in this article, or claim that may be made by its manufacturer, is not guaranteed or endorsed by the publisher.
Abbreviations
ATG, autophagy–related; FA, follicular adenoma; KO, knockout; MIEAP, mitochondria–eating protein; mtDNA, mitochondrial DNA; ROS, reactive oxygen species; Tg, thyroglobulin; TOMM20, translocase of outer mitochondrial membrane 20; 53BP1, tumor suppressor p53–binding protein 1.
References
1. Maximo V, Lima J, Prazeres H, Soares P, Sobrinho–Simoes M. The biology and the genetics of hurthle cell tumors of the thyroid. Endocr Relat Cancer (2012) 19(4):R131–47. doi: 10.1530/ERC-11-0354
2. Porcelli AM, Ghelli A, Ceccarelli C, Lang M, Cenacchi G, Capristo M, et al. The genetic and metabolic signature of oncocytic transformation implicates HIF1alpha destabilization. Hum Mol Genet (2010) 19(6):1019–32. doi: 10.1093/hmg/ddp566
3. Savagner F, Chevrollier A, Loiseau D, Morgan C, Reynier P, Clark O, et al. Mitochondrial activity in XTC. UC1 Cells derived Thyroid oncocytoma Thyroid (2001) 11(4):327–33. doi: 10.1089/10507250152039055
4. Savagner F, Franc B, Guyetant S, Rodien P, Reynier P, Malthiery Y. Defective mitochondrial ATP synthesis in oxyphilic thyroid tumors. J Clin Endocrinol Metab (2001) 86(10):4920–5. doi: 10.1210/jcem.86.10.7894
5. Savagner F, Mirebeau D, Jacques C, Guyetant S, Morgan C, Franc B, et al. PGC–1–related coactivator and targets are upregulated in thyroid oncocytoma. Biochem Biophys Res Commun (2003) 310(3):779–84. doi: 10.1016/j.bbrc.2003.09.076
6. Baris O, Savagner F, Nasser V, Loriod B, Granjeaud S, Guyetant S, et al. Transcriptional profiling reveals coordinated up–regulation of oxidative metabolism genes in thyroid oncocytic tumors. J Clin Endocrinol Metab (2004) 89(2):994–1005. doi: 10.1210/jc.2003-031238
7. Zielke A, Tezelman S, Jossart GH, Wong M, Siperstein AE, Duh QY, et al. Establishment of a highly differentiated thyroid cancer cell line of hurthle cell origin. Thyroid. (1998) 8(6):475–83. doi: 10.1089/thy.1998.8.475
8. Lee J, Ham S, Lee MH, Kim SJ, Park JH, Lee SE, et al. Dysregulation of parkin–mediated mitophagy in thyroid hurthle cell tumors. Carcinogenesis. (2015) 36(11):1407–18. doi: 10.1093/carcin/bgv122
9. Ganly I, Makarov V, Deraje S, Dong Y, Reznik E, Seshan V, et al. Integrated genomic analysis of hurthle cell cancer reveals oncogenic drivers, recurrent mitochondrial mutations, and unique chromosomal landscapes. Cancer Cell (2018) 34(2):256–70.e5. doi: 10.1016/j.ccell.2018.07.002
10. Ganly I, Ricarte Filho J, Eng S, Ghossein R, Morris LG, Liang Y, et al. Genomic dissection of hurthle cell carcinoma reveals a unique class of thyroid malignancy. J Clin Endocrinol Metab (2013) 98(5):E962–72. doi: 10.1210/jc.2012-3539
11. Gopal RK, Kubler K, Calvo SE, Polak P, Livitz D, Rosebrock D, et al. Widespread chromosomal losses and mitochondrial DNA alterations as genetic drivers in hurthle cell carcinoma. Cancer Cell (2018) 34(2):242–55.e5. doi: 10.1016/j.ccell.2018.06.013
12. Nakamura Y, Arakawa H. Discovery of mieap–regulated mitochondrial quality control as a new function of tumor suppressor p53. Cancer Sci (2017) 108(5):809–17. doi: 10.1111/cas.13208
13. Kamino H, Nakamura Y, Tsuneki M, Sano H, Miyamoto Y, Kitamura N, et al. Mieap–regulated mitochondrial quality control is frequently inactivated in human colorectal cancer. Oncogenesis. (2016) 4:e181. doi: 10.1038/oncsis.2015.43
14. Gaowa S, Futamura M, Tsuneki M, Kamino H, Tajima JY, Mori R, et al. Possible role of p53/Mieap–regulated mitochondrial quality control as a tumor suppressor in human breast cancer. Cancer Sci (2018) 109(12):3910–20. doi: 10.1111/cas.13824
15. Sano H, Futamura M, Gaowa S, Kamino H, Nakamura Y, Yamaguchi K, et al. p53/Mieap–regulated mitochondrial quality control plays an important role as a tumor suppressor in gastric and esophageal cancers. Biochem Biophys Res Commun (2020) 529(3):582–9. doi: 10.1016/j.bbrc.2020.05.168
16. Futamura M, Tokumaru Y, Takabe K, Arakawa H, Asano Y, Mori R, et al. MIEAP, a p53–downstream gene, is associated with suppression of breast cancer cell proliferation and better survival. Am J Cancer Res (2021) 11(12):6060–73.
17. Okuyama K, Kitajima Y, Egawa N, Kitagawa H, Ito K, Aishima S, et al. Mieap–induced accumulation of lysosomes within mitochondria (MALM) regulates gastric cancer cell invasion under hypoxia by suppressing reactive oxygen species accumulation. Sci Rep (2019) 9(1):2822. doi: 10.1038/s41598-019-39563-x
18. Tsuneki M, Nakamura Y, Kinjo T, Nakanishi R, Arakawa H. Mieap suppresses murine intestinal tumor via its mitochondrial quality control. Sci Rep (2015) 5:12472. doi: 10.1038/srep12472
19. Mussazhanova Z, Shimamura M, Kurashige T, Ito M, Nakashima M, Nagayama Y. Causative role for defective expression of mitochondria–eating protein in accumulation of mitochondria in thyroid oncocytic cell tumors. Cancer Sci (2020) 111(8):2814–23. doi: 10.1111/cas.14501
20. Shimamura M, Shibusawa N, Kurashige T, Mussazhanova Z, Matsuzaki H, Nakashima M, et al. Mouse models of sporadic thyroid cancer derived from BRAFV600E alone or in combination with PTEN haploinsufficiency under physiologic TSH levels. PloS One (2018) 13(8):e0201365. doi: 10.1371/journal.pone.0201365
21. Dankort D, Filenova E, Collado M, Serrano M, Jones K, McMahon M. A new mouse model to explore the initiation, progression, and therapy of BRAFV600E–induced lung tumors. Genes Dev (2007) 21(4):379–84. doi: 10.1101/gad.1516407
22. Hara T, Nakamura K, Matsui M, Yamamoto A, Nakahara Y, Suzuki–Migishima R, et al. Suppression of basal autophagy in neural cells causes neurodegenerative disease in mice. Nature. (2006) 441(7095):885–9. doi: 10.1038/nature04724
23. Shimamura M, Nakahara M, Orim F, Kurashige T, Mitsutake N, Nakashima M, et al. Postnatal expression of BRAFV600E does not induce thyroid cancer in mouse models of thyroid papillary carcinoma. Endocrinology. (2013) 154(11):4423–30. doi: 10.1210/en.2013-1174
24. Kitamura N, Nakamura Y, Miyamoto Y, Miyamoto T, Kabu K, Yoshida M, et al. Mieap, a p53–inducible protein, controls mitochondrial quality by repairing or eliminating unhealthy mitochondria. PloS One (2011) 6(1):e16060. doi: 10.1371/journal.pone.0016060
25. Ward IM, Minn K, Jorda KG, Chen J. Accumulation of checkpoint protein 53BP1 at DNA breaks involves its binding to phosphorylated histone H2AX. J Biol Chem (2003) 278(22):19579–82. doi: 10.1074/jbc.C300117200
26. Strohecker AM, Guo JY, Karsli–Uzunbas G, Price SM, Chen GJ, Mathew R, et al. Autophagy sustains mitochondrial glutamine metabolism and growth of BrafV600E–driven lung tumors. Cancer Discovery (2013) 3(11):1272–85. doi: 10.1158/2159-8290.CD-13-0397
27. Guo JY, Karsli–Uzunbas G, Mathew R, Aisner SC, Kamphorst JJ, Strohecker AM, et al. Autophagy suppresses progression of K–ras–induced lung tumors to oncocytomas and maintains lipid homeostasis. Genes Dev (2013) 27(13):1447–61. doi: 10.1101/gad.219642.113
28. Rao S, Tortola L, Perlot T, Wirnsberger G, Novatchkova M, Nitsch R, et al. A dual role for autophagy in a murine model of lung cancer. Nat Commun (2014) 5:3056. doi: 10.1038/ncomms4056
29. Galluzzi L, Green DR. Autophagy–independent functions of the autophagy machinery. Cell. (2019) 177(7):1682–99. doi: 10.1016/j.cell.2019.05.026
30. Smith AL, Whitehall JC, Bradshaw C, Gay D, Robertson F, Blain AP, et al. Age–associated mitochondrial DNA mutations cause metabolic remodelling that contributes to accelerated intestinal tumorigenesis. Nat Cancer (2020) 1(10):976–89. doi: 10.1038/s43018-020-00112-5
31. Gasparre G, Iommarini L, Porcelli AM, Lang M, Ferri GG, Kurelac I, et al. An inherited mitochondrial DNA disruptive mutation shifts to homoplasmy in oncocytic tumor cells. Hum Mutat (2009) 30(3):391–6. doi: 10.1002/humu.20870
32. Gopal RK, Calvo SE, Shih AR, Chaves FL, McGuone D, Mick E, et al. Early loss of mitochondrial complex I and rewiring of glutathione metabolism in renal oncocytoma. Proc Nat Acad Sci USA (2018) 115(27):E6283–e90. doi: 10.1073/pnas.1711888115
33. Rebecca VW, Amaravadi RK. Emerging strategies to effectively target autophagy in cancer. Oncogene. (2016) 35(1):1–11. doi: 10.1038/onc.2015.99
34. Takamura A, Komatsu M, Hara T, Sakamoto A, Kishi C, Waguri S, et al. Autophagy–deficient mice develop multiple liver tumors. Genes Dev (2011) 25(8):795–800. doi: 10.1101/gad.2016211
35. Inami Y, Waguri S, Sakamoto A, Kouno T, Nakada K, Hino O, et al. Persistent activation of Nrf2 through p62 in hepatocellular carcinoma cells. J Cell Biol (2011) 193(2):275–84. doi: 10.1083/jcb.201102031
Keywords: thyroid cancer, ATG5, MIEAP, oncocytoma, mitochondria
Citation: Hamada K, Kurashige T, Shimamura M, Arakawa H, Nakamura Y and Nagayama Y (2022) MIEAP and ATG5 are tumor suppressors in a mouse model of BRAFV600E-positive thyroid cancer. Front. Endocrinol. 13:932754. doi: 10.3389/fendo.2022.932754
Received: 30 April 2022; Accepted: 22 August 2022;
Published: 15 September 2022.
Edited by:
Jeffrey A Knauf, Cleveland Clinic, United StatesReviewed by:
Eijun Nishihara, Kuma Hospital, JapanIñigo Landa, Brigham and Women’s Hospital and Harvard Medical School, United States
Copyright © 2022 Hamada, Kurashige, Shimamura, Arakawa, Nakamura and Nagayama. This is an open-access article distributed under the terms of the Creative Commons Attribution License (CC BY). The use, distribution or reproduction in other forums is permitted, provided the original author(s) and the copyright owner(s) are credited and that the original publication in this journal is cited, in accordance with accepted academic practice. No use, distribution or reproduction is permitted which does not comply with these terms.
*Correspondence: Yuji Nagayama, bmFnYXlhbWFAbmFnYXNha2ktdS5hYy5qcA==