- Department of Gastroenterology, The First Affiliated Hospital, Zhejiang University School of Medicine, Hangzhou, China
Background and objective: Endoscopic bariatric and metabolic therapies (EBMTs) are emerging minimally invasive therapeutic options for obesity and its related complications, including non-alcoholic fatty liver disease (NAFLD). This study aimed to evaluate the effects of EBMTs on NALFD in patients with obesity.
Methods: Four databases were searched until Nov 2021. Randomized controlled trials (RCTs) and observational studies reporting liver-related outcomes following Food and Drug Administration (FDA)-approved and non-FDA-approved EBMTs were included. Liver parameters, metabolic parameters, and weight loss were evaluated. Risk of bias was assessed using the “risk of bias” tool in the Cochrane Collaboration for RCTs and the Methodological Index for Non-Randomized Studies criteria for observational studies.
Results: Thirty-three studies with 1710 individuals were included. Regarding the effects of EBMTs on liver fibrosis, a significant decline of NAFLD Fibrosis Score, but not transient elastography-detected liver stiffness or Fibrosis-4 Index, was observed. EBMTs significantly improved liver steatosis (control attenuation parameter and Hepatic Steatosis Index), NAFLD Activity Score, and Homeostasis Model Assessment of Insulin Resistance. EBMTs reduced serum levels of alanine transaminase, aspartate aminotransferase, and gamma-glutamyl transpeptidase considerably. Moreover, EBMTs had reducing effects on the serum levels of triglycerides and total cholesterol as well as body weight.
Conclusions: Our meta-analysis suggested that EBMTs could ameliorate NAFLD based on the evidence of improved liver steatosis, liver function, and insulin resistance. Large-scale, prospective, long-term studies are warranted to clarify the role of EBMTs in patients with different stages of NAFLD.
1 Introduction
Non-alcoholic fatty liver disease (NAFLD) is the most common cause of chronic liver disease worldwide, affecting up to 25% of the general population (1, 2). Furthermore, ~25% of NAFLD patients can develop non-alcoholic steatohepatitis (NASH), which may progress to fibrosis and cirrhosis (3). Obesity and insulin resistance represent the most important risk factors for NAFLD development (1). Although some emerging drugs such as sodium-glucose cotransporter 2 (SGLT2) inhibitors have shown benefits for NAFLD (4), approved pharmacotherapy for NAFLD is lacking. The backbone of NAFLD management is lifestyle modification through diet and exercise (5). Unfortunately, lifestyle interventions rarely permit total body weight loss (TBWL) of 7–10% to improve steatosis and liver biochemistry, or TBWL >10% to improve fibrosis (6). Recent studies have shown the benefit of bariatric surgery on the histologic and serologic features of NAFLD/NASH (7). Nevertheless, as with any surgical procedure, bariatric surgery is expensive and associated with potential health risks, especially in patients with end-stage liver disease. Therefore, novel, safe, and efficacious therapies for NAFLD/NASH are needed urgently.
In recent years, endoscopic bariatric and metabolic therapies (EBMTs) have emerged as less invasive methods targeted to achieve comparable results to bariatric surgery for obesity and its metabolic comorbidities at a more affordable cost and a lower risk of complications (8). Currently, six bariatric and metabolic-endoscopy devices have been approved by the US Food and Drug Administration (FDA): Orbera Intragastric Balloon System (IGB; Apollo Endosurgery, Austin, TX, USA), Obalon Balloon System (Obalon Therapeutics, Carlsbad, CA, USA), transpyloric shuttle (TPS; BAROnova, San Carlos, CA, USA), OverStitch Endoscopic Suturing System (Apollo Endosurgery) for endoscopic sleeve gastroplasty (ESG), incisionless operating platform (IOP; USGI Medical, San Clemente, CA, USA) for primary obesity surgery endoluminal (POSE), and AspireAssist (Aspire Bariatrics, King of Prussia, PA, USA) for aspiration therapy (AT). Some EBMTs are currently in clinical trials, including other types of IGB, such as the Spatz Adjustable Balloon System and ReShape Duo Balloon, and small intestinal EBMTs, including duodenal jejunal bypass liner (DJBL), duodenal mucosal resurfacing (DMR), and incisionless magnetic anastomosis system (IMAS). In addition to the effects of significant reduction in body weight (8), an increasing number of studies have reported EBMTs to have positive effects on NAFLD, including liver function, steatosis, and fibrosis (9–11). However, the small sample size limited the statistical robustness of the results. Besides, most of the studies evaluated only a few NAFLD biomarkers.
We undertook a systematic review and meta-analysis for comprehensive assessment of the therapeutic effects of EBMTs for NAFLD. We explored the potential role of EBMTs (IGB, ESG, POSE, AT, DJBL, and DMR) for NAFLD therapy by evaluating liver fibrosis, steatosis, NAFLD Activity Score (NAS), liver volume and liver function. Changes in insulin resistance, lipid profile, and weight loss were also reported.
2 Methods
2.1 Protocol and Guidance
This study was carried out in accordance with Preferred Reporting Items for Systematic Reviews and Meta-Analyses guideline (12). The study protocol is registered with PROSPERO (CRD42021247382).
2.2 Search Strategy
Searches were developed and conducted by an experienced research librarian and lead author (MR) in the databases MEDLINE, EMBASE, Web of Science, and Cochrane Central Register of Controlled Trials (CENTRAL) from inception to Nov 2021. We also reviewed the references of identified trials for additional relevant literature. Unpublished “gray” literature and ongoing trials were also searched. The full search strategy is presented in Supplementary Appendix 1.
2.3 Eligibility Criteria and Study Selection
Randomized controlled trials (RCTs) and observational studies aiming to evaluate EBMTs in obese/NAFLD/type-2 diabetes mellitus (T2DM) patients which reported liver-related outcomes (e.g., liver fibrosis, steatosis, and liver function) were eligible for inclusion. Eight types of bariatric and metabolic endoscopy were included with no restriction on language: IGB, TPS, ESG, POSE, AT, DJBL, DMR, and IMAS. Included studies were required to report outcomes at ≥3 months and be undertaken for patients ≥18 years of age. For comparative studies, relevant data were selected for inclusion.
The exclusion criteria were: (i) review articles, case reports/series, editorials, and comments; (ii) patients with cirrhosis intending to undergo liver transplantation; (iii) studies with fewer than five participants. In case of overlap between articles reporting on the same cohort, we included the study with the largest cohort or the most recent study.
Retrieved studies were imported into Endnote™ X9 (Clarative Analytics, Philadelphia, PA, USA), and duplicate hits were deleted. Two researchers (MR and XZ) screened articles independently by title and abstract. Subsequently, potentially eligible studies were checked in full text for inclusion by the same authors. Disagreements were solved by consensus.
2.4 Data Abstraction
Two independent reviewers (MR and JY) extracted data from each study using a predesigned form for data extraction. The data we sought were: (i) study characteristics (first author, year of publication, study design, country); (ii) characteristics of study participants at baseline (disease type, total number of participants, age, sex, body mass index [BMI]); (iii) intervention characteristics (intervention type, follow-up duration); (iv) clinical outcomes.
2.5 Assessment of Study Quality
RCTs were assessed for methodologic quality using the “risk of bias” tool within CENTRAL (13). Two independent reviewers (MR and MY) assessed each study for risk of bias. The overall risk of bias was considered to be “high” if ≥1 domain was rated as a high risk of bias. A RCT was considered to have a “low” risk of bias if all domains were considered to be at low risk. Otherwise, the trial was considered as having an “unclear” risk of bias. For observational studies, we assessed the risk of bias using Methodological Index for Non-Randomized Studies (MINORS) criteria (14). Each domain was scored 0, 1, or 2, with a maximum sum of 16 for noncomparative studies, and 24 for comparative studies.
2.6 Data Synthesis and Statistical Analyses
The meta-analysis was undertaken using a random-effects model. Continuous outcomes were analyzed using the mean difference (MD) and associated 95% confidence intervals (CIs). Primary outcomes were liver fibrosis (transient elastography-detected liver stiffness, NAFLD Fibrosis Score [NFS], and Fibrosis-4 Index [FIB-4]), liver steatosis (control attenuation parameter [CAP] and Hepatic Steatosis Index [HSI]), NAS, liver volume, and level of liver enzymes (alanine transaminase [ALT], aspartate aminotransferase [AST], and gamma-glutamyl transpeptidase [GGT]). Secondary outcomes were Homeostasis Model Assessment of Insulin Resistance (HOMA-IR), weight loss (body weight, BMI, TBWL, excess weight loss [EWL]), as well as levels of glycated hemoglobin A1c (HbA1c), and the lipid profile (triglycerides [TG], total cholesterol [TC], high-density lipoprotein-cholesterol [HDL-C], low-density lipoprotein-cholesterol [LDL-C]).
We assessed clinical heterogeneity based on the protocol and methodology of the included studies. Statistical heterogeneity was investigated using the I2 test: I2 <25% denoted “low” heterogeneity; I2 = 25–50% denoted “moderate” heterogeneity; I2 >50% denoted “high” heterogeneity. To identify the potential source of bias, subgroup analysis and meta-regression analysis were carried out, if possible. Meta-regression analysis was performed under random effects model using the Restricted Maximum Likelihood (REML) method by including baseline BMI, TBWL, and duration of the follow-up as covariates. We also undertook sensitivity analysis by removing a specific study from the meta-analysis. Funnel plot and Egger’s regression asymmetry test were used to evaluate publication bias. Significance was established at a two-sided α-level of 0.05. Statistical analyses were carried out with Stata 16.0 (Stata Corporation, College Station, TX, USA).
3 Results
3.1 Study Selection
Our search of databases identified 1648 records, of which 542 were duplicates. Two additional records were identified by a manual search. Among these articles, 1023 were excluded following screening of the title/abstract, and an additional 52 articles were excluded after full-text review. Finally, 33 studies remained eligible for this systematic review and meta-analysis, of which 26 were peer-reviewed published studies and seven were conference abstracts (Figure 1).
3.2 Study Characteristics
A total of 1710 participants were enrolled in the included studies. Study characteristics are summarized in Table 1. Sixteen studies evaluated IGB, among which one RCT (21) compared IGB vs. sham; three observational studies compared IGB vs. no IGB (22), cognitive-behavioral therapy (24), and lifestyle modification (25), respectively; the remaining 12 studies (9, 15–20, 23, 26–28, 44) were observational noncomparative studies. One prospective observational study (29) compared ESG vs. IGB. Two observational noncomparative studies (10, 30) evaluated ESG. One prospective noncomparative study assessed POSE (31). Two RCTs (32, 33) compared AT vs. lifestyle counseling. Eight observational noncomparative studies (11, 34–40, 45, 46) evaluated DJBL. Two prospective noncomparative studies (41, 42) and one RCT (43) compared DMR vs. sham. With regard to TPS and IMAS, no study fulfilled the inclusion criteria.
Studies were conducted in Europe (n = 16), north America (n = 6), Asia (n = 5), Australia (n = 2), and south America (n = 1); the remaining three international multicenter studies were conducted in Europe and America. When broken down by disease type, there were fifteen studies for obese patients, eight for obese T2DM patients, seven for obese NAFLD patients, and three for T2DM patients. The age and BMI at the time of the procedure varied from 31.5 years to 58 years and 30.3 kg/m2 to 65 kg/m2, respectively. The median duration of follow-up was 6 (range, 6–24) months.
3.3 Liver Fibrosis
The effects of EBMTs on liver fibrosis were evaluated by transient elastography-detected liver stiffness (three studies, 91 participants), NFS (three studies, 152 participants), FIB-4 (three studies, 49 participants), and AST-to-platelet ratio index (APRI) (one study, 21 participants). EBMTs were associated with a significant decrease in NFS (MD −0.58 [95%CI −0.97 to −0.20]) (Figure 2A). Nevertheless, a non-significant difference was observed between EBMTs and transient elastography-detected liver stiffness (MD −6.39 kPa [95%CI −13.73 to 0.96]) (Figure 2B) or FIB-4 (MD −0.28 [95%CI −0.63 to 0.07]) (Figure 2C). One study (9) reported that IGB reduced APRI significantly by 0.73 (P = 0.005) and the magnetic resonance elastography-detected liver stiffness value by 0.3 kPa (P = 0.03).
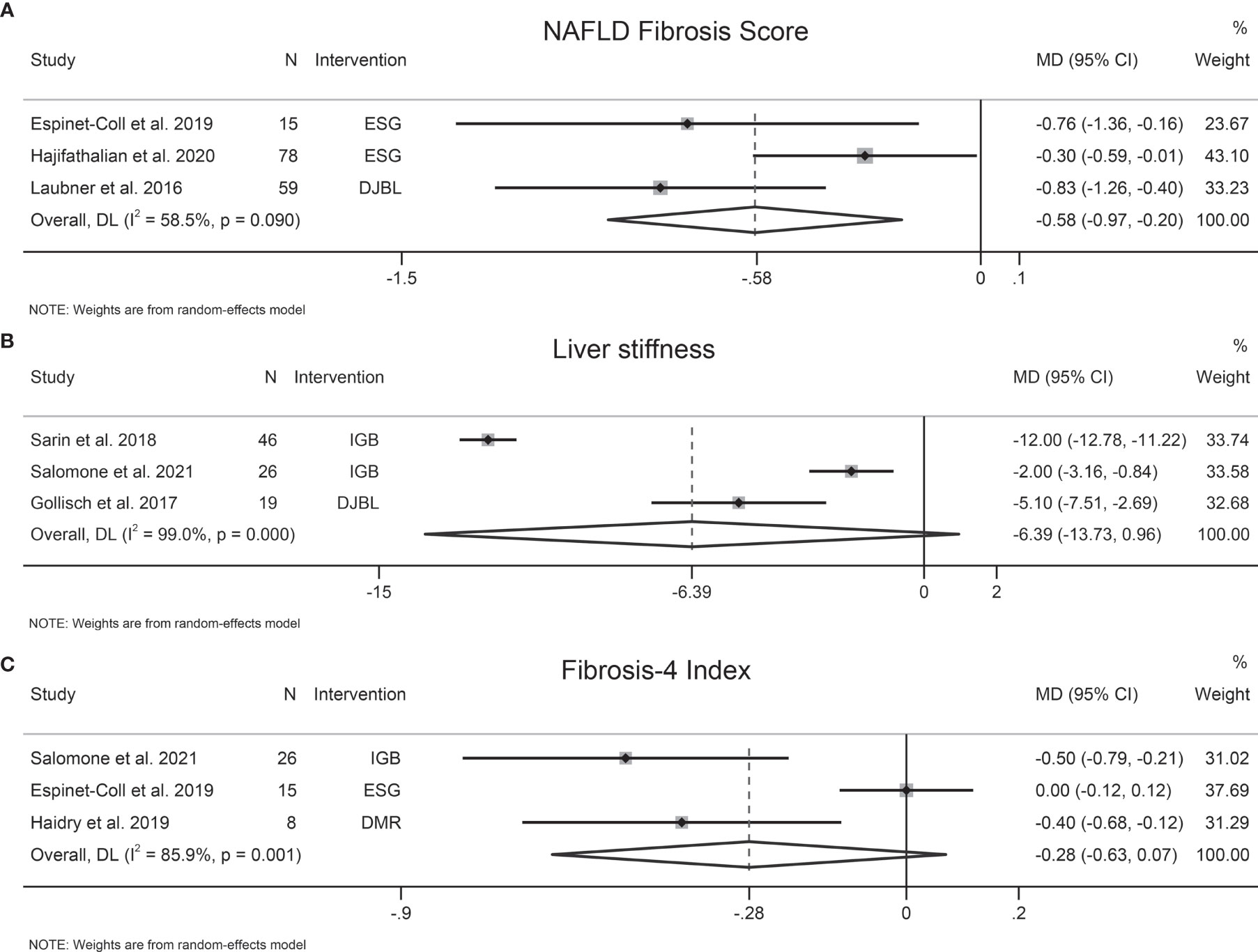
Figure 2 Forest plot of the effects of bariatric and metabolic endoscopy on liver fibrosis. (A) NAFLD Fibrosis Score (NFS), (B) transient elastography-detected liver stiffness, (C) Fibrosis-4 Index (FIB-4).
Heterogeneity was high in terms of transient elastography-detected liver stiffness, NFS, and FIB-4, at I2 = 99%, 58.5%, and 85.9%, respectively. Sensitivity analysis showed that the results of our meta-analysis were stable. We did not evaluate publication bias due to the small number of trials.
3.4 Liver Steatosis
The effects of EBMTs on liver steatosis were assessed by CAP (four studies, 83 participants), HSI (two studies, 93 participants), magnetic resonance imaging-proton density fat fraction (MRI-PDFF) (one study, 48 participants), and Fatty Liver Index (FLI) (one study, 15 participants). EBMTs were associated with a significant decrease in CAP (MD −53.76 dB/m [95%CI −73.04 to −34.47]) (Figure 3A) and HSI (MD −5.25 [95%CI −8.3 to −2.11]) (Figure 3B). However, Mingrone et al. found a non-significant relationship between DMR therapy and liver MRI-PDFF value (P = 0.096) (43). Similarly, another study showed that FLI did not change significantly following ESG (P = 0.280) (29).
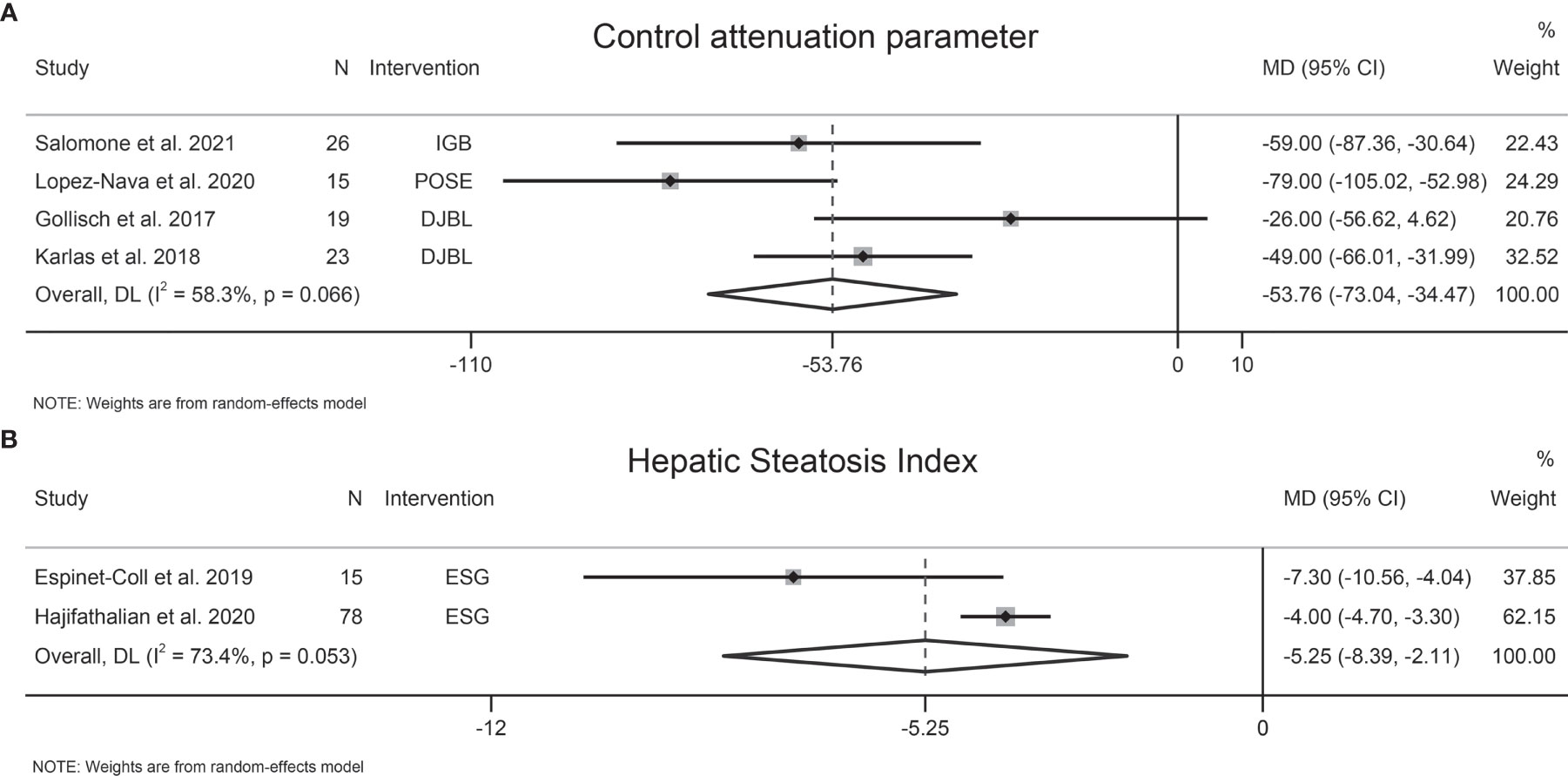
Figure 3 Forest plot of the effects of bariatric and metabolic endoscopy on liver steatosis. (A) control attenuation parameters (CAP), (B) Hepatic Steatosis Index (HSI).
Heterogeneity was high regarding CAP and HSI, at I2 = 58.3% and 73.4%, respectively. Sensitivity analysis showed that the results of our meta-analysis were stable. Publication bias was not assessed due to the small number of studies.
3.5 NAFLD Activity Score
Two studies involving 28 participants evaluated the effects of EBMTs on NAS, and both studies evaluated IGB. Following IGB, NAS was reduced significantly (MD −3 [95%CI −3.27 to −2.73]) (Figure 4A). Substantial heterogeneity was not found (I2 = 0.0%). The number of included studies was insufficient to carry out the Egger test.
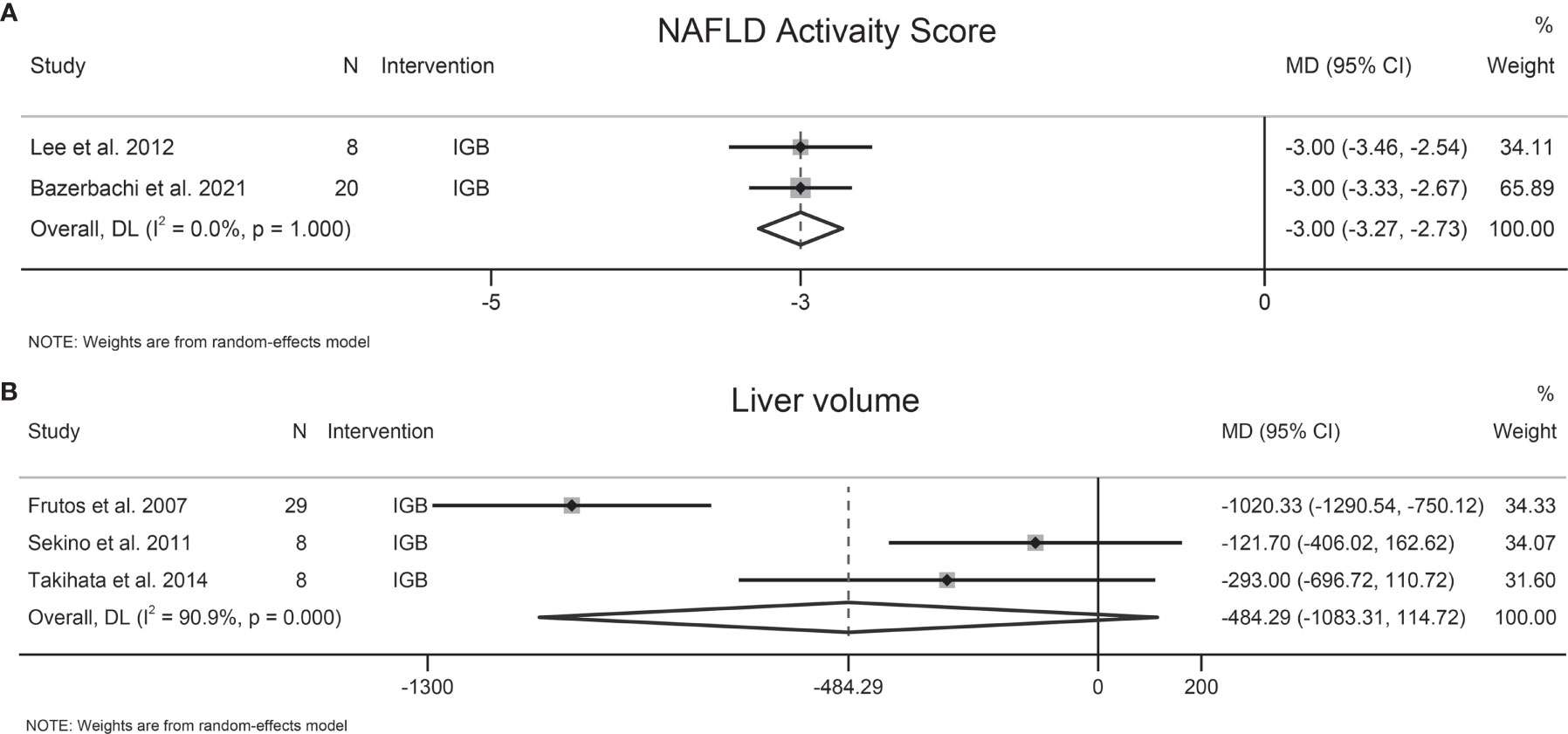
Figure 4 Forest plot of change in (A) NAFLD Activity Score (NAS) and (B) liver volume following bariatric and metabolic endoscopy.
3.6 Liver Volume
Three studies involving 45 patients explored the effect of EBMTs on liver volume, all of which evaluated IGB. Following IGB, a significant change of liver volume was not found (MD −484.29 cm3 [95%CI −1083.31 to 114.72]) (Figure 4B). Heterogeneity was high: I2 = 90.9%. Sensitivity analysis showed that the results of our meta-analysis were stable. A test of publication bias was not done due to the small number of trials.
3.7 Liver Enzymes
3.7.1 ALT
Twenty-eight studies involving 1365 participants reported the effects of EBMTs on the serum level of ALT. Overall, EBMTs were associated with significant reduction of the ALT level (MD −12.44 U/L [95%CI −14.70 to −10.19]) (Figure 5). Categorization into subgroups depending on intervention type revealed that all types of EBMTs could reduce the ALT level significantly (IGB: MD −16.09 U/L [95%CI −20.66 to −11.52], 14 studies, 758 participants; ESG: MD: −6.03 U/L [95%CI −8.83 to −3.24], three studies, 185 participants; AT: MD −7.76 U/L [95%CI −9.04 to −6.49], two studies, 121 participants; DJBL: MD −13.78 U/L [95%CI −16.72 to −10.84], six studies, 200 participants; DMR: MD −10.12 U/L [95%CI −14.58 to −5.66], two studies, 65 participants).
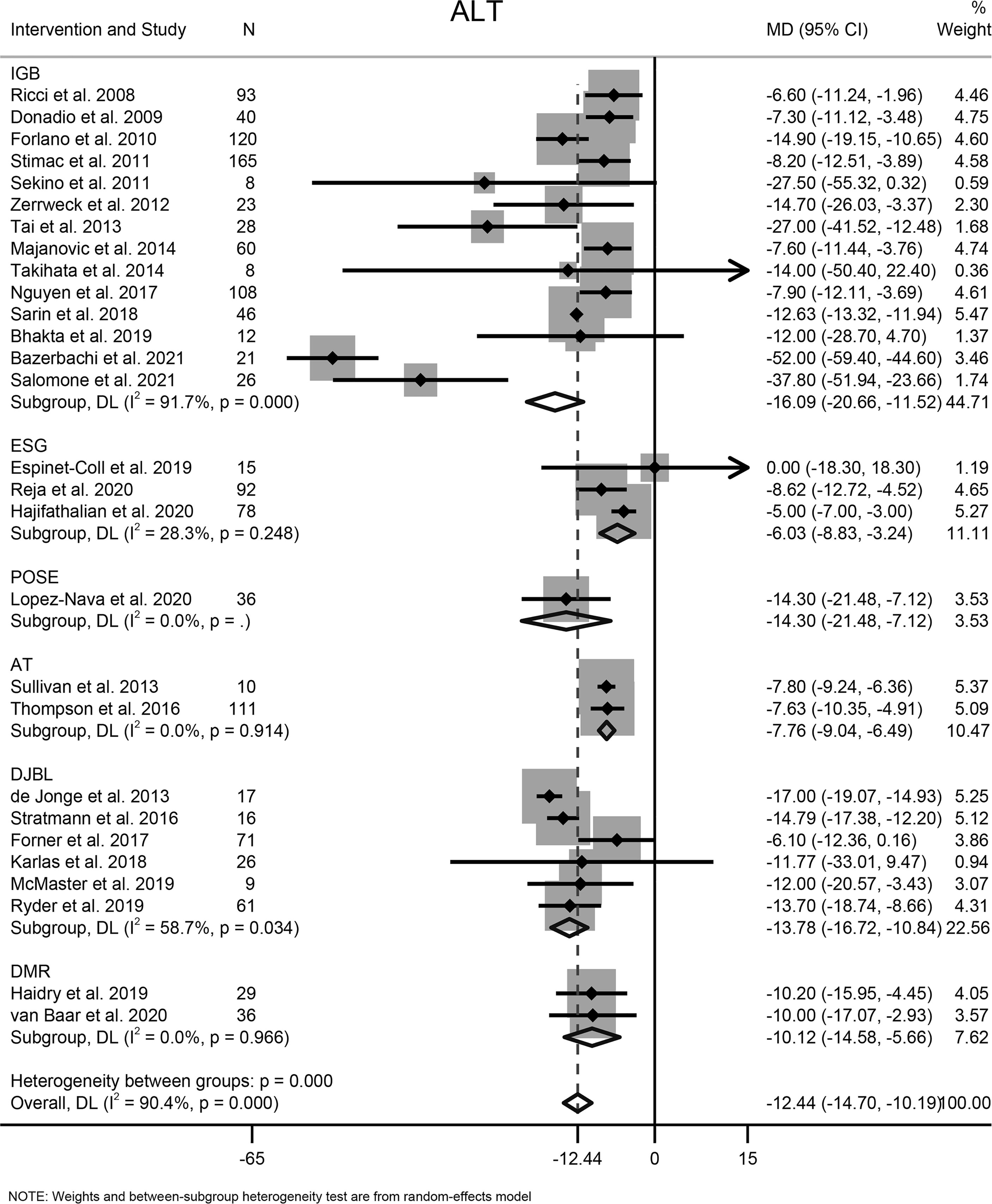
Figure 5 Forest plot of change in level of alanine transaminase following bariatric and metabolic endoscopy with subgroup analysis based on intervention type. ALT, alanine transaminase.
High heterogeneity among the studies was identified (I2 = 90.4%), so subgroup analysis, meta-regression, and sensitivity analysis were carried out. Subgroup analysis was conducted according to the intervention type, disease type, study design, BMI at baseline (<40, ≥40 kg/m2), and ALT level at baseline (<40, ≥40 and <60, ≥60 U/L). The ALT level at baseline was suspected of being a source of heterogeneity. On meta-regression analysis, the ALT level at baseline was a significant predictor for the magnitude of change in the ALT level (β = −0.66 [95%CI −0.80 to −0.51], P < 0.001). Sensitivity analysis demonstrated the stability of our meta-analysis. The funnel plot was symmetrical (Figure 8A) and Egger test P value was not significant (P = 0.762).
3.7.2 AST
The effects of EBMTs on the serum level of AST were assessed in 15 studies (494 participants). Overall, EBMTs significantly lowered the serum AST level (MD −7.88 U/L [95%CI −11.11 to −4.64]) (Figure 6). All types of EBMTs except DJBL (MD −4.33 U/L [95%CI −9.14 to 0.47], three studies, 104 participants) were associated with a reduction in the AST level (IGB: MD −15.52 U/L [95%CI −25.88 to 5.25], seven studies, 147 participants; ESG: MD −2.80 U/L [95%CI −4.26 to −1.34], two studies, 93 participants; AT: MD −2.71 U/L [95%CI −4.14 to −1.28], two studies, 121 participants).
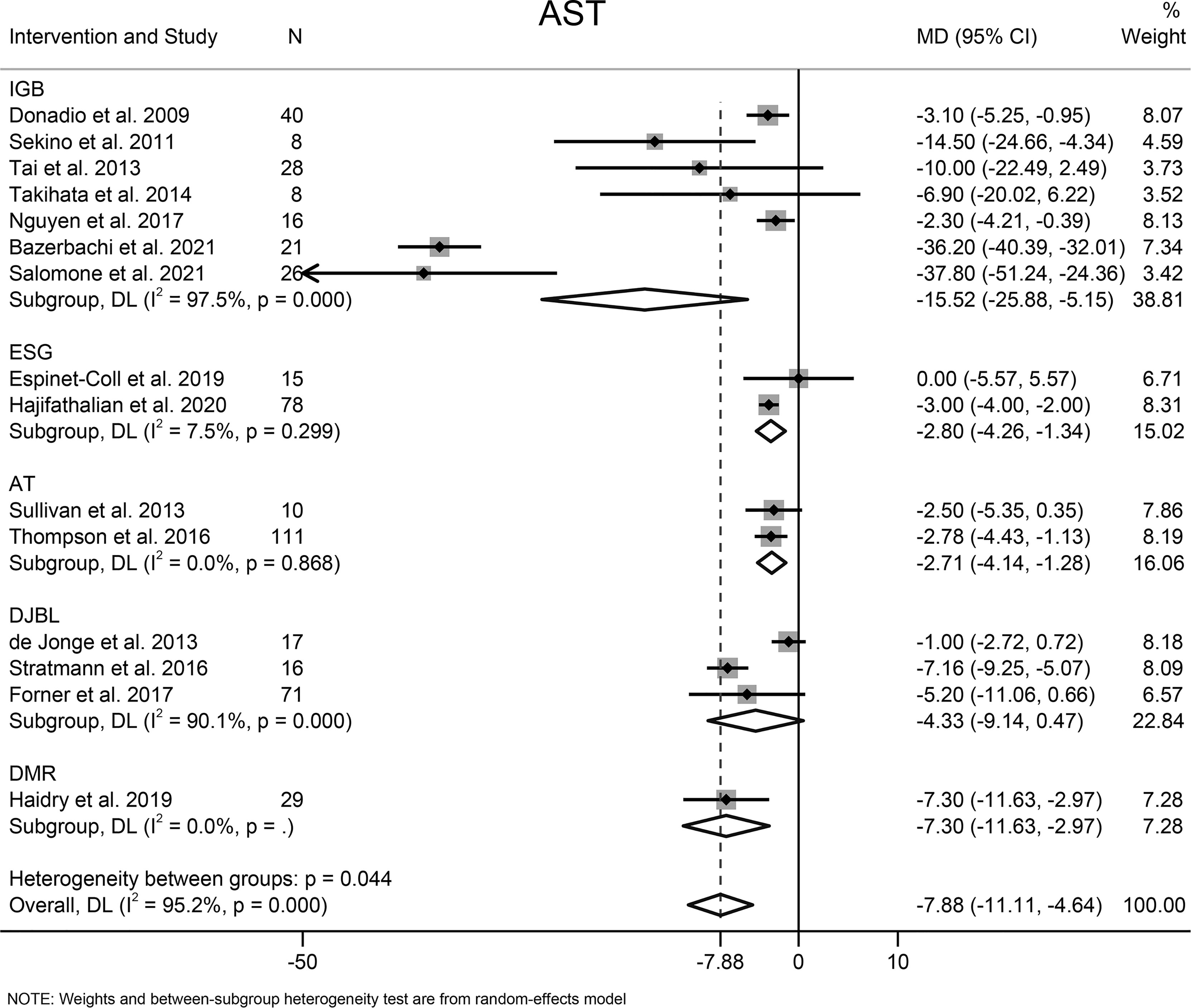
Figure 6 Forest plot of change in level of aspartate aminotransferase following bariatric and metabolic endoscopy with subgroup analysis based on intervention type. AST, aspartate aminotransferase.
The heterogeneity was high (I2 = 95.2%), so subgroup analysis, meta-regression analysis, and sensitivity analysis were conducted. Subgroup analysis was done according to the intervention type, disease type, study design, BMI at baseline (<40, ≥40 kg/m2), and AST level at baseline (<30, ≥30 and <60, ≥60 U/L). The AST level at baseline was suspected of being the source of heterogeneity. Further meta-regression analysis revealed that the AST level at baseline was a significant predictor for the magnitude of change in the AST level (β = −0.74, [95%CI −1.02 to −0.45], P < 0.001). Through sensitivity analysis, we found that the study by Bazerbachi and colleagues (9) was likely to be the main source of heterogeneity. After excluding that study, the heterogeneity decreased to 77.8%, and the mean difference in the AST level changed to −4.21 U/L [95%CI −5.86 to −2.56]. Though the funnel plot seemed to be visually asymmetrical (Figure 8B), no significant publication bias was detected by Egger test (P = 0.095).
3.7.3 GGT
Fourteen studies with 744 participants evaluated the effects of EBMTs on the serum GGT level. Overall, bariatric metabolic endoscopy could reduce the serum level of GGT significantly (MD: −12.07 U/L [95%CI −15.79 to −8.35]) (Figure 7). IGB (MD −9.88 U/L [95%CI −13.12 to −6.65]) and DJBL (MD −17.14 U/L [95%CI: −25.41 to −8.87]) were associated with a significantly decreased GGT level.
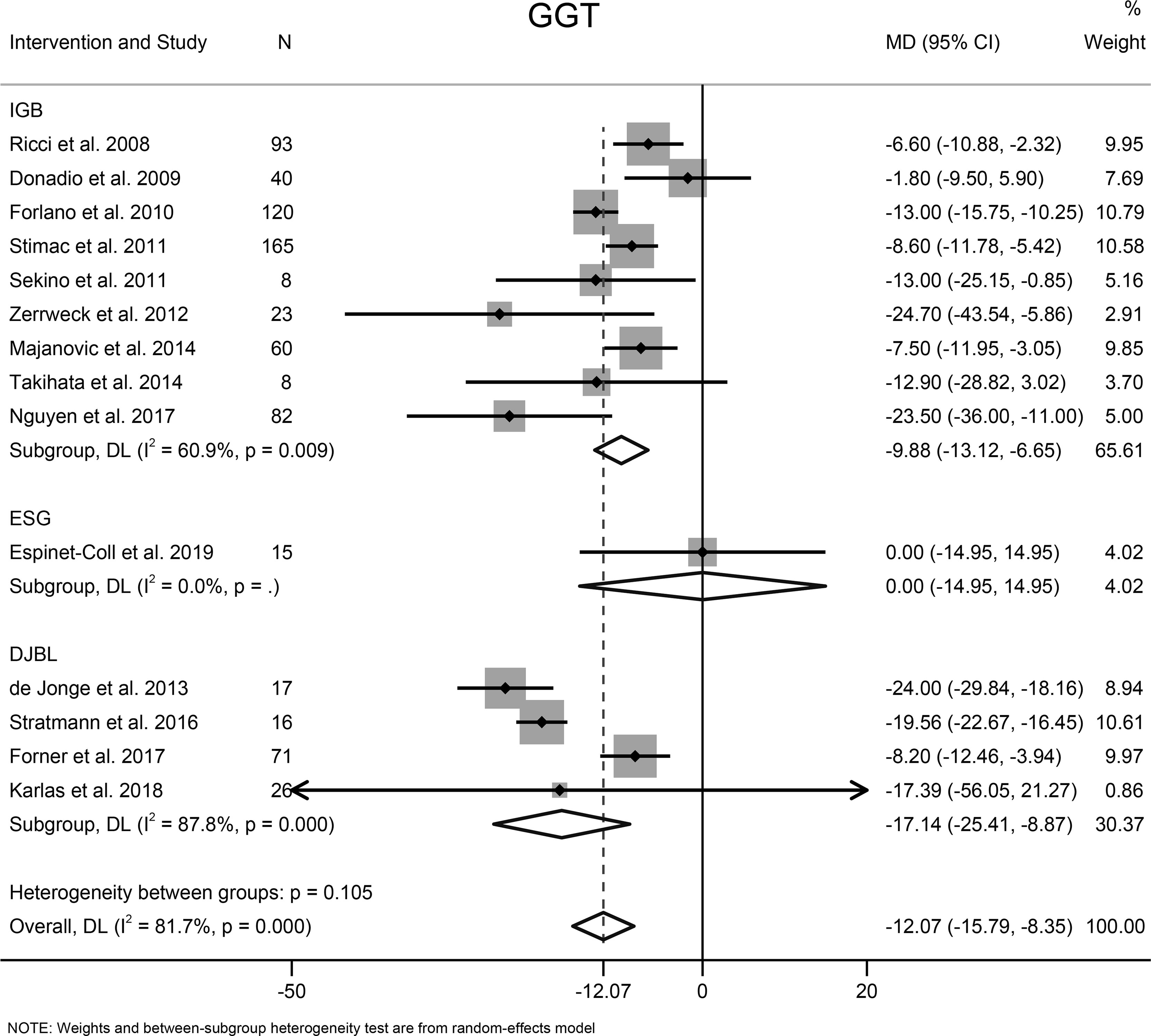
Figure 7 Forest plot of change in level of gamma-glutamyl transpeptidase following bariatric and metabolic endoscopy with subgroup analysis based on intervention type. GGT, gamma-glutamyl transpeptidase.
The heterogeneity test for the included studies revealed that the heterogeneity among the studies was high (I2 = 81.7%). Subgroup analysis was conducted according to the intervention type, disease type, study design, BMI at baseline (<40, ≥40 kg/m2), and GGT level at baseline (<35, ≥35 and <60, ≥60 U/L). The AST level at baseline was suspected of being a source of heterogeneity. Meta-regression analysis showed that the GGT level at baseline was a significant predictor for the magnitude of change in the GGT level (β = −0.47 [95%CI −0.70 to −0.25, P < 0.001). Sensitivity analysis suggested that the results were stable. The funnel plot was symmetrical (Figure 8C), and Egger test indicated no significant publication bias (P = 0.988).
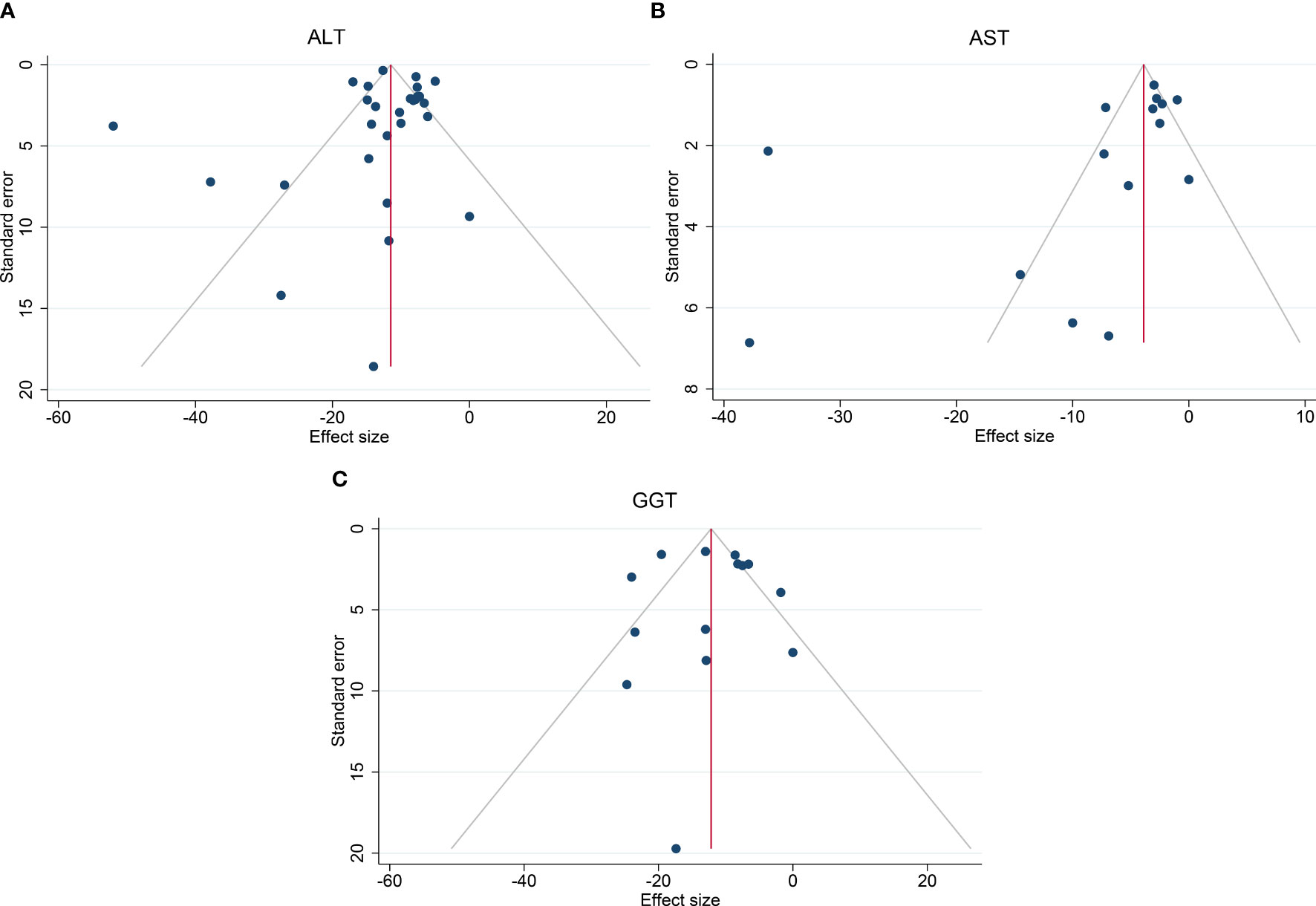
Figure 8 Funnel plots to assess publication bias. (A) Funnel plot of ALT, (B) Funnel plot of AST, (C) Funnel plot of GGT. ALT, alanine transaminase; AST, aspartate aminotransferase; GGT, gamma-glutamyl transpeptidase.
3.8 Insulin Resistance
Nine studies involving 399 patients reported the effects of EBMTs on HOMA-IR. Following EBMTs, HOMA-IR was decreased significantly (MD −1.9 [95%CI −2.49 to −1.30]) (Supplementary Figure 1A), suggesting improved insulin resistance.
Nineteen studies involving 693 patients reported the effects of EBMTs on the HbA1c level, among which six-each evaluated IGB and DJBL, three evaluated DMR, and two-each evaluated ESG and AT. The HbA1c level was reduced significantly (MD −0.74 [95%CI −1.01 to −0.48]) (Supplementary Figure 1B) following EBMTs, which indicated improved glucose homeostasis.
3.9 Lipid Profile
Eighteen studies reported on the lipid profile in blood. EBMTs were associated with a significantly reduced serum level of TG (MD −0.33 mmol/L [95%CI −0.43 to −0.22], 14 studies, 709 participants) (Supplementary Figure 2A) and TC (MD −0.21 mmol/L [95%CI −0.32 to −0.09], 13 studies, 671 participants) (Supplementary Figure 2B). However, a significant change was not found in terms of the serum level of HDL-C (MD 0.03 mmol/L [95%CI −0.01 to 0.07], 14 studies, 582 participants) (Supplementary Figure 2C) or LDL-C (MD −0.08 mmol/L [95%CI −0.22 to 0.05], 11 studies, 483 participants) (Supplementary Figure 2D).
3.10 Weight Loss
All 33 studies reported weight loss-related outcomes. EBMTs reduced body weight (MD −12.18 kg [95%CI −15.42 to −8.93], 22 studies, 1224 participants) (Supplementary Figure 3A) and BMI (MD −4.33 kg/m2 [95%CI −5.12 to −3.54], 23 studies, 1213 participants) (Supplementary Figure 3B) significantly. Similarly, significant TBWL (MD 13.61 [95%CI 11.26 to 15.97], seven studies, 366 participants) (Supplementary Figure 3C) and EWL (MD 31.14 [95%CI 21.72 to 40.56], eight studies, 458 participants) (Supplementary Figure 3D) were observed following EBMTs.
3.11 Adverse Events
Regarding the safety of EBMTs, twenty-one studies provided information about adverse events, but the reporting was inconsistent and fragmented (Supplementary Table 1). Common adverse events include nausea, vomiting, abdominal pain, etc. The incidence of serious adverse events ranged from 0% to 19% across the individual studies.
3.12 Assessment of Risk of Bias
The risk-of-bias tool within CENTRAL was used to assess the risk of bias of RCTs (Supplementary Table 2). Two RCTs (32, 33) were evaluated as high risk, and two (21, 43) as unclear. All four RCTs had an unclear risk of bias of outcome. Both studies for AT (32, 33) were at a high risk in the domain of “absence of masking of participants”. The study by Lee and coworkers (21) had an unclear risk of bias for random-sequence generation. We used MINORS criteria to assess the quality of observational studies (Supplementary Table 3). The scores were 19 or 20 for comparative studies and ranged from 9 to 13 for noncomparative studies. For the included observational studies, a high risk of bias was found in the domain of “prospective calculation of the study size”, “prospective collection of data”, and “loss to follow-up”. An unclear risk of bias was found for “unbiased assessment of the study end point”. There was unclear and high risk of bias for the domain of “inclusion of consecutive patients”. Assessment of the risk of bias was not done in conference abstracts due to insufficient information.
4 Discussion
This meta-analysis explored the potential role of EBMTs for NAFLD by evaluating liver parameters, metabolic parameters, and weight loss. According to our meta-analysis, EBMTs reduced the serum level of ALT, AST, and GGT considerably. Further meta-regression analysis revealed that the magnitude of reduction of the level of liver enzymes was positively correlated with the liver-enzyme level at baseline. Liver steatosis (CAP and HSI), NAS, and insulin resistance improved following EBMTs. With regard to the effects of EBMTs on liver fibrosis, our meta-analysis indicated a significant decline of NFS, but not transient elastography-detected liver stiffness or FIB-4. Moreover, EBMTs had reducing effects on the serum levels of TG and TC as well as body weight.
Recently, Jirapinyo and colleagues (47) carried out a meta-analysis to explore the effects of FDA-approved EBMTs on NAFLD. Significant improvement of liver fibrosis and other NAFLD surrogates (e.g., ALT level, hepatic steatosis, histologic NAS) were observed following EBMTs. In addition, metabolic parameters (insulin resistance and waist circumference) were improved significantly. Based on those results, Jirapinyo and coworkers suggested that EBMTs might ameliorate NAFLD. However, non-FDA-approved EBMTs were not included in their meta-analysis.
For more comprehensive evaluation of the role of EBMTs in NAFLD, we undertook a meta-analysis including FDA-approved EBMTs and non-FDA-approved small intestinal EBMTs such as DJBL and DMR. Our results are mostly consistent with the findings of Jirapinyo and colleagues. Importantly, we found that non-FDA-approved small intestinal EBMTs showed comparable effects with those of FDA-approved devices in terms of improvement in liver parameters.
In our meta-analysis, liver fibrosis was evaluated by non-invasive biomarkers, such as liver stiffness, NFS (48), and FIB-4 (49). EMBTs could decrease NFS significantly, but a significant change in liver stiffness or FIB-4 was not observed. All four studies reporting liver stiffness showed a significant decrease in liver stiffness, which was inconsistent with the results of our meta-analysis. The small study size, diverse disease types, and diverse intervention types may account for this difference. Although these non-invasive biomarkers had high accuracy for detecting clinically significant liver fibrosis, liver biopsy remains the standard for NAFLD diagnosis (50). Two studies have reported the effects of EBMTs on the histologic liver fibrosis. A randomized sham-controlled trial in Singapore evaluated the efficacy of the BioEnterics intragastric balloon (BIB) in ameliorating histology-confirmed NASH (21): a significant change in the fibrosis score was not observed in the BIB group compared with that in the sham group (P = 0.303) (21). More recently, an open-labeled prospective study in the USA with 20 NASH patients showed that histologic fibrosis improved in 3/20 patients, remained unchanged in 12/20 patients, and worsened in 5/20 patients, following IGB (9). Future large-scale, randomized controlled clinical trials exploring the effects of different types of EBMTs on histologic fibrosis features are warranted.
When broken down into different types of EBMTs, we found that IGB (the most well-established EBMT available) could improve the level of liver enzymes and histologic NAS significantly, data which are in line with other studies (51, 52). A recent meta-analysis demonstrated that the beneficial effects of swallowable IGB on liver enzymes and insulin resistance were no worse than those of endoscopic IGB (52). As safe and efficacious procedures, the potential utility of IGB for NAFLD has been mentioned in the clinical practice guidelines for NAFLD set by the Asian Pacific Association. Future studies are needed to clarify the long-term efficacy of IGB for NAFLD/NASH patients as well as the safety of IGB in patients with end-stage liver disease.
The potential mechanisms of EBMTs for NAFLD treatment are not clear, but are likely to be related to aspects dependent and independent of weight loss. Insulin resistance is pivotal for NAFLD progression (1). Weight loss could lead to an improvement of insulin resistance in skeletal muscle, thereby improving NAFLD. Weight loss-independent mechanisms differ among different types of EBMTs, in which gut hormones may play a crucial role (53). It has been reported that IGB therapy reduced plasma ghrelin levels (54) and increased levels of sirtuin-1 (55) (a well-known regulator of energy homeostasis and metabolism). Following DJBL implantation, increased fasting and postprandial glucagon-like peptide-1 (GLP-1) levels have been observed (34, 56). GLP-1 can stimulate glucose-dependent insulin secretion, thereby reducing postprandial hyperglycemia. Moreover, alteration of the gut microbiota was observed when DJBL was in situ, and returned to baseline levels after removal of the device, which may account for the beneficial effects of DJBL for NAFLD (57).
There was significant heterogeneity among the included studies. We tried to search for possible sources of heterogeneity through subgroup analysis, meta-regression analysis, and sensitivity analysis: only the liver-enzyme levels at baseline were found to be a potential source of heterogeneity. We speculate that two main factors may account for such high heterogeneity: (i) disease types varied from obesity, T2DM, to NAFLD/NASH, and different disease phenotypes may respond differently to a specific therapy; (ii) the diversity of interventions may have led to heterogeneity. Importantly, the magnitude of changes in the levels of liver enzymes was positively corelated with the liver-enzyme level at baseline, indicating that EBMTs could control liver-enzyme levels within the normal range irrespective of NAFLD severity.
Our meta-analysis had five main limitations. First, most of clinical studies in this field are observational studies, which do not allow establishment of firm conclusions. Second, the sample sizes of the included studies were relatively small. Third, most of the included studies focused on the effects of EBMTs in obese cases and/or T2DM patients, and reported changes in liver parameters; only a few studies were done in NAFLD/NASH patients. Further studies are warranted to clarify the safety and efficacy of EBMTs in individuals with different phenotypes of NAFLD. Moreover, the median follow-up time was only 6 months, which did not allow the evaluation of long-term outcomes. Finally, liver histology, the “gold standard” for the diagnosis of NAFLD, was not evaluated in most of the included studies. Therefore, future studies considering the histologic features of NAFLD/NASH are needed.
Despite the limitations stated above, our systematic review and meta-analysis provides the most comprehensive evaluation of the potential role of EBMTs in NAFLD. Eight types of intervention were included in our meta-analysis. Besides, a wide variety of NAFLD biomarkers, including liver histology, non-invasive parameters assessing liver steatosis and fibrosis, metabolic parameters, and weight loss, were evaluated.
5 Conclusions
Based on limited evidence, our meta-analysis suggested that EBMTs could ameliorate NAFLD by improving steatosis, NAS, insulin resistance, as well as reducing serum levels of liver enzymes, triglycerides and total cholesterol. EBMTs may bridge a critical gap between less efficacious pharmacologic/behavioral strategies and efficacious but invasive bariatric surgical procedures in NAFLD treatment. Future large-scale trials with rigorous methods are warranted to further clarify the short-term/long-term efficacy and safety of EBMTs for patients with different stages of NAFLD.
Data Availability Statement
The original contributions presented in the study are included in the article/Supplementary Material. Further inquiries can be directed to the corresponding author.
Author Contributions
Study concept and design, MR and FJ. Written of the protocol, MR. Database searches and study selection, MR and XZ. Risk of bias assessment, MR and MY. Meta-analysis and drafting of the manuscript, MR. Critical revision of the manuscript for important intellectual content, XZ and FJ. Administrative, technical, or material support, YZ, FM, and JY. Study supervision, FJ. All authors have made a significant contribution to this study and have approved the final manuscript.
Funding
The work was supported in part by a grant from the Key Research and Development Program of Zhejiang Province (2019C03031).
Conflict of Interest
The authors declare that the research was conducted in the absence of any commercial or financial relationships that could be construed as a potential conflict of interest.
Publisher’s Note
All claims expressed in this article are solely those of the authors and do not necessarily represent those of their affiliated organizations, or those of the publisher, the editors and the reviewers. Any product that may be evaluated in this article, or claim that may be made by its manufacturer, is not guaranteed or endorsed by the publisher.
Supplementary Material
The Supplementary Material for this article can be found online at: https://www.frontiersin.org/articles/10.3389/fendo.2022.931519/full#supplementary-material
References
1. Younossi ZM. Non-Alcoholic Fatty Liver Disease - A Global Public Health Perspective. J Hepatol (2019) 70(3):531–44. doi: 10.1016/j.jhep.2018.10.033
2. Hashem A, Shastri Y, Al Otaibi M, Buchel E, Saleh H, Ahmad R, et al. Expert Opinion on the Management of Non-Alcoholic Fatty Liver Disease (Nafld) in the Middle East With a Focus on the Use of Silymarin. Gastroenterol Insights (2021) 12(2):155–65. doi: 10.3390/gastroent12020014
3. Estes C, Razavi H, Loomba R, Younossi Z, Sanyal AJ. Modeling the Epidemic of Nonalcoholic Fatty Liver Disease Demonstrates an Exponential Increase in Burden of Disease. Hepatology (2018) 67(1):123–33. doi: 10.1002/hep.29466
4. Goya T, Imoto K, Tashiro S, Aoyagi T, Takahashi M, Kurokawa M, et al. The Efficacy of Tofogliflozin on Metabolic Dysfunction-Associated Fatty Liver Disease. Gastroenterol Insights (2022) 13(1):20–6. doi: 10.3390/gastroent13010003
5. Konerman MA, Jones JC, Harrison SA. Pharmacotherapy for Nash: Current and Emerging. J Hepatol (2018) 68(2):362–75. doi: 10.1016/j.jhep.2017.10.015
6. Vilar-Gomez E, Martinez-Perez Y, Calzadilla-Bertot L, Torres-Gonzalez A, Gra-Oramas B, Gonzalez-Fabian L, et al. Weight Loss Through Lifestyle Modification Significantly Reduces Features of Nonalcoholic Steatohepatitis. Gastroenterology (2015) 149(2):367–78.e5;quize14-5. doi: 10.1053/j.gastro.2015.04.005
7. Lassailly G, Caiazzo R, Ntandja-Wandji LC, Gnemmi V, Baud G, Verkindt H, et al. Bariatric Surgery Provides Long-Term Resolution of Nonalcoholic Steatohepatitis and Regression of Fibrosis. Gastroenterology (2020) 159(4):1290–301.e5. doi: 10.1053/j.gastro.2020.06.006
8. Jung SH, Yoon JH, Choi HS, Nam SJ, Kim KO, Kim DH, et al. Comparative Efficacy of Bariatric Endoscopic Procedures in the Treatment of Morbid Obesity: A Systematic Review and Network Meta-Analysis. Endoscopy (2020) 52(11):940–54. doi: 10.1055/a-1149-1862
9. Bazerbachi F, Vargas EJ, Rizk M, Maselli DB, Mounajjed T, Venkatesh SK, et al. Intragastric Balloon Placement Induces Significant Metabolic and Histologic Improvement in Patients With Nonalcoholic Steatohepatitis. Clin Gastroenterol Hepatol (2021) 19(1):146–54.e4. doi: 10.1016/j.cgh.2020.04.068
10. Hajifathalian K, Mehta A, Ang B, Skaf D, Shah SL, Saumoy M, et al. Improvement in Insulin Resistance and Estimated Hepatic Steatosis and Fibrosis After Endoscopic Sleeve Gastroplasty. Gastrointest Endosc (2021) 93(5):1110–8. doi: 10.1016/j.gie.2020.08.023
11. Ryder REJ, Irwin SP, Burbridge W, Gandhi H, Bashir T, Allden RA, et al. The United Kingdom's First Nhs Endobarrier Service for Advanced Diabesity: 1-Year Outcomes for All 62 Treated Patients. Brit J Diabetes (2019) 19(2):110–7. doi: 10.15277/bjd.2019.226
12. Liberati A, Altman DG, Tetzlaff J, Mulrow C, Gotzsche PC, Ioannidis JP, et al. The Prisma Statement for Reporting Systematic Reviews and Meta-Analyses of Studies That Evaluate Healthcare Interventions: Explanation and Elaboration. BMJ (2009) 339:b2700. doi: 10.1136/bmj.b2700
13. Higgins JP, Altman DG, Gotzsche PC, Juni P, Moher D, Oxman AD, et al. The Cochrane Collaboration's Tool for Assessing Risk of Bias in Randomised Trials. BMJ (2011) 343:d5928. doi: 10.1136/bmj.d5928
14. Slim K, Nini E, Forestier D, Kwiatkowski F, Panis Y, Chipponi J. Methodological Index for Non-Randomized Studies (Minors): Development and Validation of a New Instrument. ANZ J Surg (2003) 73(9):712–6. doi: 10.1046/j.1445-2197.2003.02748.x
15. Frutos MD, Morales MD, Lujan J, Hernandez Q, Valero G, Parrilla P. Intragastric Balloon Reduces Liver Volume in Super-Obese Patients, Facilitating Subsequent Laparoscopic Gastric Bypass. Obes Surg (2007) 17(2):150–4. doi: 10.1007/s11695-007-9040-3
16. Ricci G, Bersani G, Rossi A, Pigo F, De Fabritiis G, Alvisi V. Bariatric Therapy With Intragastric Balloon Improves Liver Dysfunction and Insulin Resistance in Obese Patients. Obes Surg (2008) 18(11):1438–42. doi: 10.1007/s11695-008-9487-x
17. Donadio F, Sburlati LF, Masserini B, Lunati EM, Lattuada E, Zappa MA, et al. Metabolic Parameters After Bioenterics Intragastric Balloon Placement in Obese Patients. J Endocrinol Invest (2009) 32(2):165–8. doi: 10.1007/BF03345708
18. Forlano R, Ippolito AM, Iacobellis A, Merla A, Valvano MR, Niro G, et al. Effect of the Bioenterics Intragastric Balloon on Weight, Insulin Resistance, and Liver Steatosis in Obese Patients. Gastrointest Endosc (2010) 71(6):927–33. doi: 10.1016/j.gie.2009.06.036
19. Sekino Y, Imajo K, Sakai E, Uchiyama T, Iida H, Endo H, et al. Time-Course of Changes of Visceral Fat Area, Liver Volume and Liver Fat Area During Intragastric Balloon Therapy in Japanese Super-Obese Patients. Intern Med (2011) 50(21):2449–55. doi: 10.2169/internalmedicine.50.5672
20. Stimac D, Majanovic SK, Turk T, Kezele B, Licul V, Orlic ZC. Intragastric Balloon Treatment for Obesity: Results of a Large Single Center Prospective Study. Obes Surg (2011) 21(5):551–5. doi: 10.1007/s11695-010-0310-0
21. Lee YM, Low HC, Lim LG, Dan YY, Aung MO, Cheng CL, et al. Intragastric Balloon Significantly Improves Nonalcoholic Fatty Liver Disease Activity Score in Obese Patients With Nonalcoholic Steatohepatitis: A Pilot Study. Gastrointest Endosc (2012) 76(4):756–60. doi: 10.1016/j.gie.2012.05.023
22. Zerrweck C, Maunoury V, Caiazzo R, Branche J, Dezfoulian G, Bulois P, et al. Preoperative Weight Loss With Intragastric Balloon Decreases the Risk of Significant Adverse Outcomes of Laparoscopic Gastric Bypass in Super-Super Obese Patients. Obes Surg (2012) 22(5):777–82. doi: 10.1007/s11695-011-0571-2
23. Tai CM, Lin HY, Yen YC, Huang CK, Hsu WL, Huang YW, et al. Effectiveness of Intragastric Balloon Treatment for Obese Patients: One-Year Follow-Up After Balloon Removal. Obes Surg (2013) 23(12):2068–74. doi: 10.1007/s11695-013-1027-7
24. Majanovic SK, Ruzic A, Bulian AP, Licul V, Orlic ZC, Stimac D. Comparative Study of Intragastric Balloon and Cognitive-Behavioral Approach for Non-Morbid Obesity. Hepatogastroenterology (2014) 61(132):937–41. doi: 10.5754/hge14146
25. Takihata M, Nakamura A, Aoki K, Kimura M, Sekino Y, Inamori M, et al. Comparison of Intragastric Balloon Therapy and Intensive Lifestyle Modification Therapy With Respect to Weight Reduction and Abdominal Fat Distribution in Super-Obese Japanese Patients. Obes Res Clin Pract (2014) 8(4):e331–8. doi: 10.1016/j.orcp.2013.07.002
26. Nguyen V, Li J, Gan J, Cordero P, Ray S, Solis-Cuevas A, et al. Outcomes Following Serial Intragastric Balloon Therapy for Obesity and Nonalcoholic Fatty Liver Disease in a Single Centre. Can J Gastroenterol Hepatol (2017) 2017:4697194. doi: 10.1155/2017/4697194
27. Bhakta D, Popov V, Whitsett M. The Impact of Endoscopic Bariatric Therapies on Metabolic Outcomes and Nonalcoholic Fatty Liver Disease in Veterans. Surg Obes Related Dis (2019) 15(10):S219. doi: 10.1016/j.soard.2019.08.466
28. Salomone F, Currenti W, Magri G, Boskoski I, Zelber-Sagi S, Galvano F. Effects of Intragastric Balloon in Patients With Nonalcoholic Fatty Liver Disease and Advanced Fibrosis. Liver Int (2021) 41(9):2112–6. doi: 10.1111/liv.14917
29. Espinet Coll E, Vila Lolo C, Diaz Galan P, Gomez Valero JA, Bacchiddu S, Quintana Tomas C, et al. Bariatric and Metabolic Endoscopy in the Handling of Fatty Liver Disease. A New Emerging Approach? Rev Esp Enferm Dig (2019) 111(4):283–93. doi: 10.17235/reed.2019.5949/2018
30. Reja D, Shahid HM, Tyberg A, Bareket R, John ES, Patel S, et al. Weight and Metabolic Outcomes Associated With Endoscopic Sleeve Gastroplasty: A Multicenter Study. Gastrointestinal Endoscopy (2020) 91(6):Ab220–Ab. doi: 10.1016/j.gie.2020.03.1747
31. Lopez-Nava G, Turro R, Maselli DB, Bautista-Castano I, Matar R, Asokkumar R, et al. Primary Obesity Surgery Endoluminal 2 (Pose2): An International Multicenter Prospective Trial. Gastrointestinal Endoscopy (2020) 91(6):Ab60–Ab. doi: 10.1016/j.gie.2020.03.584
32. Sullivan S, Stein R, Jonnalagadda S, Mullady D, Edmundowicz S. Aspiration Therapy Leads to Weight Loss in Obese Subjects: A Pilot Study. Gastroenterology (2013) 145(6):1245–52.e1-5. doi: 10.1053/j.gastro.2013.08.056
33. Thompson CC, Abu Dayyeh BK, Kushner R, Sullivan S, Schorr AB, Amaro A, et al. Percutaneous Gastrostomy Device for the Treatment of Class Ii and Class Iii Obesity: Results of a Randomized Controlled Trial. Am J Gastroenterol (2017) 112(3):447–57. doi: 10.1038/ajg.2016.500
34. de Jonge C, Rensen SS, Koek GH, Joosten MF, Buurman WA, Bouvy ND, et al. Endoscopic Duodenal-Jejunal Bypass Liner Rapidly Improves Plasma Parameters of Nonalcoholic Fatty Liver Disease. Clin Gastroenterol Hepatol (2013) 11(11):1517–20. doi: 10.1016/j.cgh.2013.07.029
35. Laubner K, Nicolaus L, Bettinger D, Neumann-Haefelin C, Perakakis N, Thimme R, et al. Duodenal-Jejunal Bypass Liner Reduces Nonalcoholic Fatty Liver Disease (Nafld) in Obese Patients With Type 2 Diabetes (T2dm). Diabetes (2016) 65:A507–A.
36. Stratmann B, Krepak Y, Schiffer E, Jarick I, Hauber M, Lee-Barkey YH, et al. Beneficial Metabolic Effects of Duodenal Jejunal Bypass Liner for the Treatment of Adipose Patients With Type 2 Diabetes Mellitus: Analysis of Responders and Non-Responders. Horm Metab Res (2016) 48(10):630–7. doi: 10.1055/s-0042-115175
37. Forner PM, Ramacciotti T, Farey JE, Lord RV. Safety and Effectiveness of an Endoscopically Placed Duodenal-Jejunal Bypass Device (Endobarrier(R)): Outcomes in 114 Patients. Obes Surg (2017) 27(12):3306–13. doi: 10.1007/s11695-017-2939-4
38. Gollisch KS, Lindhorst A, Raddatz D. Endobarrier Gastrointestinal Liner in Type 2 Diabetic Patients Improves Liver Fibrosis as Assessed by Liver Elastography. Exp Clin Endocrinol Diabetes (2017) 125(2):116–21. doi: 10.1055/s-0042-118961
39. Karlas T, Feisthammel J, Schutz T, Bluher M, Lichtinghagen R, Keim V, et al. Impact of Duodenal-Jejunal Bypass Liner on Non-Alcoholic Fatty Liver Disease. J Hepatol (2018) 68:S829–S. doi: 10.1016/S0168-8278(18)31933-0
40. McMaster JJ, Shanahan ER, Do A, Rich G, Veronique C, Macdonald GA, et al. Weight Loss and Metabolic Improvements One Year After Treatment With a Duodenal-Jejunal Bypass Sleeve (Endobarrier (R)). Gastroenterology (2019) 156(6):S1170–S1. doi: 10.1016/S0016-5085(19)39895-6
41. Haidry RJ, van Baar AC, Galvao Neto MP, Rajagopalan H, Caplan J, Levin PS, et al. Duodenal Mucosal Resurfacing: Proof-Of-Concept, Procedural Development, and Initial Implementation in the Clinical Setting. Gastrointest Endosc (2019) 90(4):673–81.e2. doi: 10.1016/j.gie.2019.03.024
42. van Baar ACG, Holleman F, Crenier L, Haidry R, Magee C, Hopkins D, et al. Endoscopic Duodenal Mucosal Resurfacing for the Treatment of Type 2 Diabetes Mellitus: One Year Results From the First International, Open-Label, Prospective, Multicentre Study. Gut (2020) 69(2):295–303. doi: 10.1136/gutjnl-2019-318349
43. Mingrone G, van Baar AC, Deviere J, Hopkins D, Moura E, Cercato C, et al. Safety and Efficacy of Hydrothermal Duodenal Mucosal Resurfacing in Patients With Type 2 Diabetes: The Randomised, Double-Blind, Sham-Controlled, Multicentre Revita-2 Feasibility Trial. Gut (2021) 71(2):254–64. doi: 10.1136/gutjnl-2020-323608
44. Sarin SK, Anand L, Bhardwaj A, Jamwal KD, Maiwall R, Choudhury AK, et al. Intragastric Balloon in Compensated Nash (Non Alcoholic Steato Hepatitis) Cirrhotics - an Observational Study. Hepatology (2018) 68:977a–8a.
45. Quezada N, Munoz R, Morelli C, Turiel D, Hernandez J, Pimentel F, et al. Safety and Efficacy of the Endoscopic Duodenal-Jejunal Bypass Liner Prototype in Severe or Morbidly Obese Subjects Implanted for Up to 3 Years. Surg Endosc (2018) 32(1):260–7. doi: 10.1007/s00464-017-5672-0
46. Betzel B, Cooiman MI, Aarts EO, Janssen IMC, Wahab PJ, Groenen MJM, et al. Clinical Follow-Up on Weight Loss, Glycemic Control, and Safety Aspects of 24 Months of Duodenal-Jejunal Bypass Liner Implantation. Surg Endosc (2020) 34(1):209–15. doi: 10.1007/s00464-019-06752-8
47. Jirapinyo P, McCarty TR, Dolan RD, Shah R, Thompson CC. Effect of Endoscopic Bariatric and Metabolic Therapies on Nonalcoholic Fatty Liver Disease: A Systematic Review and Meta-Analysis. Clin Gastroenterol Hepatol (2021) 20(3):511–24. doi: 10.1016/j.cgh.2021.03.017
48. Angulo P, Hui JM, Marchesini G, Bugianesi E, George J, Farrell GC, et al. The Nafld Fibrosis Score: A Noninvasive System That Identifies Liver Fibrosis in Patients With Nafld. Hepatology (2007) 45(4):846–54. doi: 10.1002/hep.21496
49. Sterling RK, Lissen E, Clumeck N, Sola R, Correa MC, Montaner J, et al. Development of a Simple Noninvasive Index to Predict Significant Fibrosis in Patients With Hiv/Hcv Coinfection. Hepatology (2006) 43(6):1317–25. doi: 10.1002/hep.21178
50. Wong VW, Adams LA, de Ledinghen V, Wong GL, Sookoian S. Noninvasive Biomarkers in Nafld and Nash - Current Progress and Future Promise. Nat Rev Gastroenterol Hepatol (2018) 15(8):461–78. doi: 10.1038/s41575-018-0014-9
51. Chandan S, Mohan BP, Khan SR, Facciorusso A, Ramai D, Kassab LL, et al. Efficacy and Safety of Intragastric Balloon (Igb) in Non-Alcoholic Fatty Liver Disease (Nafld): A Comprehensive Review and Meta-Analysis. Obes Surg (2021) 31(3):1271–9. doi: 10.1007/s11695-020-05084-0
52. Zou ZY, Zeng J, Ren TY, Shi YW, Yang RX, Fan JG. Efficacy of Intragastric Balloons in the Markers of Metabolic Dysfunction-Associated Fatty Liver Disease: Results From Meta-Analyses. J Clin Transl Hepatol (2021) 9(3):353–63. doi: 10.14218/JCTH.2020.00183
53. Finelli C, Padula MC, Martelli G, Tarantino G. Could the Improvement of Obesity-Related Co-Morbidities Depend on Modified Gut Hormones Secretion? World J Gastroenterol (2014) 20(44):16649–64. doi: 10.3748/wjg.v20.i44.16649
54. Mion F, Napoleon B, Roman S, Malvoisin E, Trepo F, Pujol B, et al. Effects of Intragastric Balloon on Gastric Emptying and Plasma Ghrelin Levels in Non-Morbid Obese Patients. Obes Surg (2005) 15(4):510–6. doi: 10.1381/0960892053723411
55. Mariani S, Fiore D, Persichetti A, Basciani S, Lubrano C, Poggiogalle E, et al. Circulating Sirt1 Increases After Intragastric Balloon Fat Loss in Obese Patients. Obes Surg (2016) 26(6):1215–20. doi: 10.1007/s11695-015-1859-4
56. Shuang J, Zhang Y, Ma L, Tan X, Huang J, Wang X, et al. Relief of Diabetes by Duodenal-Jejunal Bypass Sleeve Implantation in the High-Fat Diet and Streptozotocin-Induced Diabetic Rat Model Is Associated With an Increase in Glp-1 Levels and the Number of Glp-1-Positive Cells. Exp Ther Med (2015) 10(4):1355–63. doi: 10.3892/etm.2015.2669
Keywords: endoscopy, non-alcoholic fatty liver disease (NAFLD), bariatrics, liver function tests, meta-analysis
Citation: Ren M, Zhou X, Zhang Y, Mo F, Yang J, Yu M and Ji F (2022) Effects of Bariatric Endoscopy on Non-Alcoholic Fatty Liver Disease: A Comprehensive Systematic Review and Meta-Analysis. Front. Endocrinol. 13:931519. doi: 10.3389/fendo.2022.931519
Received: 29 April 2022; Accepted: 11 May 2022;
Published: 17 June 2022.
Edited by:
Stefano Ballestri, Local Health Unit of Modena, ItalyReviewed by:
Giovanni Tarantino, University of Naples Federico II, ItalyGeraldine Ooi, Monash University, Australia
Copyright © 2022 Ren, Zhou, Zhang, Mo, Yang, Yu and Ji. This is an open-access article distributed under the terms of the Creative Commons Attribution License (CC BY). The use, distribution or reproduction in other forums is permitted, provided the original author(s) and the copyright owner(s) are credited and that the original publication in this journal is cited, in accordance with accepted academic practice. No use, distribution or reproduction is permitted which does not comply with these terms.
*Correspondence: Feng Ji, amlmZW5nQHpqdS5lZHUuY24=