- 1Section of Endocrinology and Investigative Medicine, Imperial College London, London, United Kingdom
- 2Department of Endocrinology, Imperial College Healthcare National Health Service (NHS) Trust, London, United Kingdom
The neuropeptide kisspeptin is now well-established as the master regulator of the mammalian reproductive axis. Beyond the hypothalamus, kisspeptin and its cognate receptor are also extensively distributed in extra-hypothalamic brain regions. An expanding pool of animal and human data demonstrates that kisspeptin sits within an extensive neuroanatomical and functional framework through which it can integrate a range of internal and external cues with appropriate neuroendocrine and behavioural responses. In keeping with this, recent studies reveal wide-reaching effects of kisspeptin on key behaviours such as olfactory-mediated partner preference, sexual motivation, copulatory behaviour, bonding, mood, and emotions. In this review, we provide a comprehensive update on the current animal and human literature highlighting the far-reaching behaviour and mood-altering roles of kisspeptin. A comprehensive understanding of this important area in kisspeptin biology is key to the escalating development of kisspeptin-based therapies for common reproductive and related psychological and psychosexual disorders.
Introduction
From an evolutionary perspective, the reproductive system is the most fundamental biological system obligate for species survival. In view of this, the behavioural strategies to facilitate reproduction must be highly efficient and meticulously coordinated (1). In many species, the timing of reproductive activity is coordinated by the integration of internal physiological events and behavioural factors (2). Indeed, in most sexually reproducing species, reproductive behaviour occurs when fertilisation is most likely to happen and is otherwise suppressed (3). Moreover, several species like humans have evolved to gain reward and satisfaction from sex and its precursors (sexual desire and arousal) (4). Taken together, it is therefore conceivable that reproductive behaviour, mood and emotions are mediated by similar hormonal mechanisms, in a presumed drive towards successful reproduction. Although oestrogen and testosterone impact reproductive behaviour circuits (5), emerging data supports the key roles for upstream factors, particularly kisspeptin which is the focus of this review.
The reproductive neuropeptide kisspeptin is a well-established orchestrator of reproductive hormone secretion, acting at the apex of the hypothalamic-pituitary-gonadal (HPG) reproductive axis (6, 7). Emerging data from animal models and human studies reveals that outside of the classical reproductive axis, kisspeptin also modulates reproductive behaviour, mood and emotions, in part at least through its extensive distribution in the limbic system (8–11). In this review, we provide a comprehensive update on the current animal and human literature highlighting the far-reaching behaviour and mood-altering effects of kisspeptin. A comprehensive understanding of this important area in kisspeptin biology is key to the escalating development of kisspeptin-based therapies for common reproductive and related psychological and psychosexual disorders. In addition, we discuss the most recent data examining kisspeptin’s effects on copulatory behaviour and mood in rodents, as well as a broad range of contemporary human evidence, thereby providing an update to the field. Furthermore, we separate out the evidence into pre-clinical animal studies and human studies to permit fuller methodological and cross-species appraisal of the data.
Methods
We performed a literature review and identified relevant publications by means of a series of PubMed searches for English-language articles. The search terms were (“kisspeptin” OR “KISS1” OR “KISS1R” OR “GPR54”) AND (“behaviour” OR “sex” OR “olfaction/olfactory” OR “audition/auditory” OR “partner/mate preference” OR “lordosis” OR “erection” OR “mood” OR “emotion” OR “depression” OR “anxiety” OR “fear). Relevant data were subsequently extracted from the identified publications, and secondary data sources identified therein. To ensure inclusion of the most up-to-date data, searches were performed up until 25th April 2022.
Kisspeptin: An Overview
Encoded by the KISS1 gene in humans (Kiss1 in non-humans), the kisspeptins are a family of structurally related peptides derived from a common 145-amino acid precursor (10, 11). Although debate exists as to what extent each peptide is naturally present, cleavage gives rise to four circulating fragments: kisspeptin-54, -14, -13 and -10, with the suffix conveying the amino acid length. These four kisspeptin isoforms share a common C-terminal decapeptide sequence (equivalent to kisspeptin-10), conferring the minimum amino acid sequence required for biological activity at a seven transmembrane G protein-coupled receptor, the kisspeptin receptor (encoded by KISS1R humans/Kiss1r non-humans) (8, 10, 11).
Following its initial discovery as a human malignant melanoma metastasis-suppressor gene by Danny Welch and colleagues in Hershey Pennsylvania (from where its name originates based on the local chocolate sweets) (12), evidence emerged identifying kisspeptin-signalling as essential for reproductive health. Humans with inactivating mutations of KISS1 or KISS1R display a phenotype of hypogonadotropic hypogonadism (6, 7), whereas activating mutations of KISS1 or KISS1R causes central precocious puberty (13). Subsequent physiological studies have been key in defining kisspeptin’s role within the reproductive axis: kisspeptin sits at the apex of the HPG axis stimulating endogenous gonadotropin-releasing hormone (GnRH) release, thereby resulting in release of gonadotropins [luteinizing hormone (LH) and follicle stimulating hormone (FSH)] from the anterior pituitary and subsequent sex steroid release from the gonads (14).
Beyond mammalian species, the kisspeptin system is also found in non-mammalian vertebrates. Indeed, in 2004 the ortholog of the kisspeptin receptor (Kiss2r) was first isolated in a teleost species (Nile tilapia), providing the initial evidence of a kisspeptin system in fish (15). Unlike rodents and humans, more than one form of kisspeptin and kisspeptin receptor paralogs have been identified in many fish species. For instance, zebrafish possess two kisspeptin genes (Kiss1 and Kiss2) and two corresponding cognate receptor genes (Kiss1r and Kiss2r) (16). While both Kiss1 and Kiss1r are co-expressed in the ventral habenula, Kiss2 expressing neurons reside in the hypothalamic preoptic area and are implicated in gonadotropin secretion (17, 18). However, it is worth noting that several gene mutation studies in zebrafish and medaka reveal that the reproductive role of kisspeptin may be dispensable (19–22), with further studies warranted to examine the possible redundancy of kisspeptin-signalling in reproduction in different species of fish [reviewed extensively and recently in (23)].
Patterns of Kisspeptin-Signalling
A series of distribution studies have been instrumental in improving our knowledge of central and peripheral kisspeptin expression, thereby providing an anatomical framework for the pleotropic roles of kisspeptin-signalling.
Central Kisspeptin Expression
Hypothalamic Expression
Kisspeptin is expressed in two anatomically discrete hypothalamic nuclei in rodents: the arcuate nucleus (ARC) and the anteroventral periventricular nucleus (AVPV) (24–26). Of note, most kisspeptin neurons in the ARC co-express neurokinin B and dynorphin, which are termed the kisspeptin/neurokinin B/dynorphin (KNDy) neurons (27). The two equivalent regions of kisspeptin expression in the human hypothalamus have been mapped to the infundibular nucleus (corresponding to the rodent ARC) and the rostral preoptic area, with both neuronal populations projecting to GnRH neurons in the mediobasal hypothalamus (28, 29). Interestingly, the limited degree to which these human populations mirror the rodent populations in terms of neurokinin B and dynorphin co-expression suggests species variation (30–32).
Extra-Hypothalamic Expression
Kisspeptin-immunoreactive fibres have been identified in the rodent medial amygdala (MeA), bed nucleus of the stria terminalis, paraventricular thalamus and periaqueductal grey (26), as well as Kiss1r mRNA detected in the amygdala, frontal cortex, hippocampus, midbrain, pons, striatum and thalamus (8). Moreover, the highest density of kisspeptin receptor expression in the mouse central nervous system can be found in the dentate gyrus of the hippocampus (33). Turning to humans, KISS1 and KISS1R mRNA are widely detected outside the hypothalamus, including the amygdala, caudate nucleus, cingulate gyrus, globus pallidus, hippocampus, medial frontal gyrus, nucleus accumbens, parahippocampal gyrus, putamen, striatum, substantia nigra, superior frontal gyrus and thalamus, as determined by reverse transcription polymerase chain reaction (9, 10). From a functional perspective, these brain structures constitute key limbic and paralimbic regions, which have important functions in controlling mood and behaviour (34) and alludes to kisspeptin’s involvement in these important domains.
Peripheral Kisspeptin Expression
In rodents, Kiss1 and Kiss1r mRNA has been identified in peripheral regions, including the liver, intestine (particularly the caecum) and bone (8, 35, 36). Moreover, abundant Kiss1 and Kiss1r expression is detected in peripheral reproductive organs in rodents, including ovary (35), uterus (37) and testes (38). Regarding humans, KISS1 mRNA expression is principally found in the placenta and testis, as well as moderate expression levels in the liver, pancreas, small intestine (9, 11). Moreover, notable KISS1R mRNA expression is present in the placenta and pancreas, with lower expression levels in organs such as adipose tissue, kidney, liver, small intestine, spleen, stomach, testis and bone (9–11, 39).
Reproductive Behaviour
Reproductive behaviour constitutes a complex chain of behavioural adaptations and strategies related to the ultimate production of offspring. It includes the identification of suitable mating partners, courtship, and sexual behaviour, as well as bonding (2). A wealth of literature implicates kisspeptin-signalling in all aspects of reproductive behaviour from rodents through to humans.
Olfaction
Olfaction underpins many important mating behaviours in mammals through the detection of chemosignals, also known as pheromones. The accessory olfactory bulb is the primary centre for detecting and processing information from chemosignals in animals (40).
Preclinical Animal Studies
Using immunofluorescence histochemistry in adult male rats, kisspeptin neurons have been visualised in the mitral cell layer of the accessory olfactory bulb (41). Furthermore, these kisspeptin fibres connect bi-directionally with kisspeptin neuronal fibres in the MeA which in turn, make connections with the preoptic area of the hypothalamus (41). The existence of this neuro-anatomical framework suggests that kisspeptin is a potential integrator of olfactory pheromonal cues with the HPG axis.
To provide functional relevance for the neuroanatomical connections between the olfactory system and kisspeptin populations, animal models have examined the influence of opposite-sex olfactory stimuli on kisspeptin neuronal activity. In ovariectomised female rats, increased kisspeptin activity is observed in the AVPV in response to male (but not female) odours, with associated augmentation of the LH surge (42). Similarly, female mice show activation in AVPV kisspeptin neurons on exposure to male (but not female) urinary pheromones (43). Sexual dimorphism is demonstrated with male mice exhibiting no changes in AVPV or arcuate kisspeptin activity when exposed to female odours (44). Instead, a two-fold rise in the number of c-Fos positive MeA kisspeptin neurons is observed with a concomitant rise in LH release (44).
Olfactory signals are also vital in sheep and goats, whereby the introduction of a stimulus male can override the normal suppressive effects of oestradiol in anoestrous females. Termed the “ram effect”, this sociosexual stimulus is known to be mediated by male pheromones detected by the female (45). In ovariectomised goats, exposure to a sexually mature male is associated with a significant rise in arcuate kisspeptin neuronal activity with concurrent LH pulse generation (46). Similarly, when anoestrous ewes are exposed to a novel male, increased c-Fos activity is seen in arcuate kisspeptin neurons with associated increases in LH amplitude and pulse frequency, an effect which is blocked by pre-administration of a kisspeptin antagonist (47).
Human Studies
Using functional neuroimaging [functional Magnetic Resonance Imaging (fMRI)], a recent study demonstrated that peripheral kisspeptin administration enhances limbic brain activity in healthy heterosexual men when exposed to an established feminine scent (Chanel No5) (48). In response to this feminine olfactory stimulus, brain activation was seen in key limbic regions involved in olfactory processing, hedonic valuation of olfactory stimuli and sexual arousal (49). Notably in this study, kisspeptin did not enhance brain activity in control motor areas, indicating specificity of kisspeptin’s effects in olfactory and limbic circuits governing sexual behaviour on exposure to a feminine olfactory stimulus (48).
Taken together, these findings provide insight into the roles of kisspeptin as a key integrator of sexually relevant olfactory stimuli with limbic pathways and the HPG axis in several species including humans (Summarised in Figure 1).
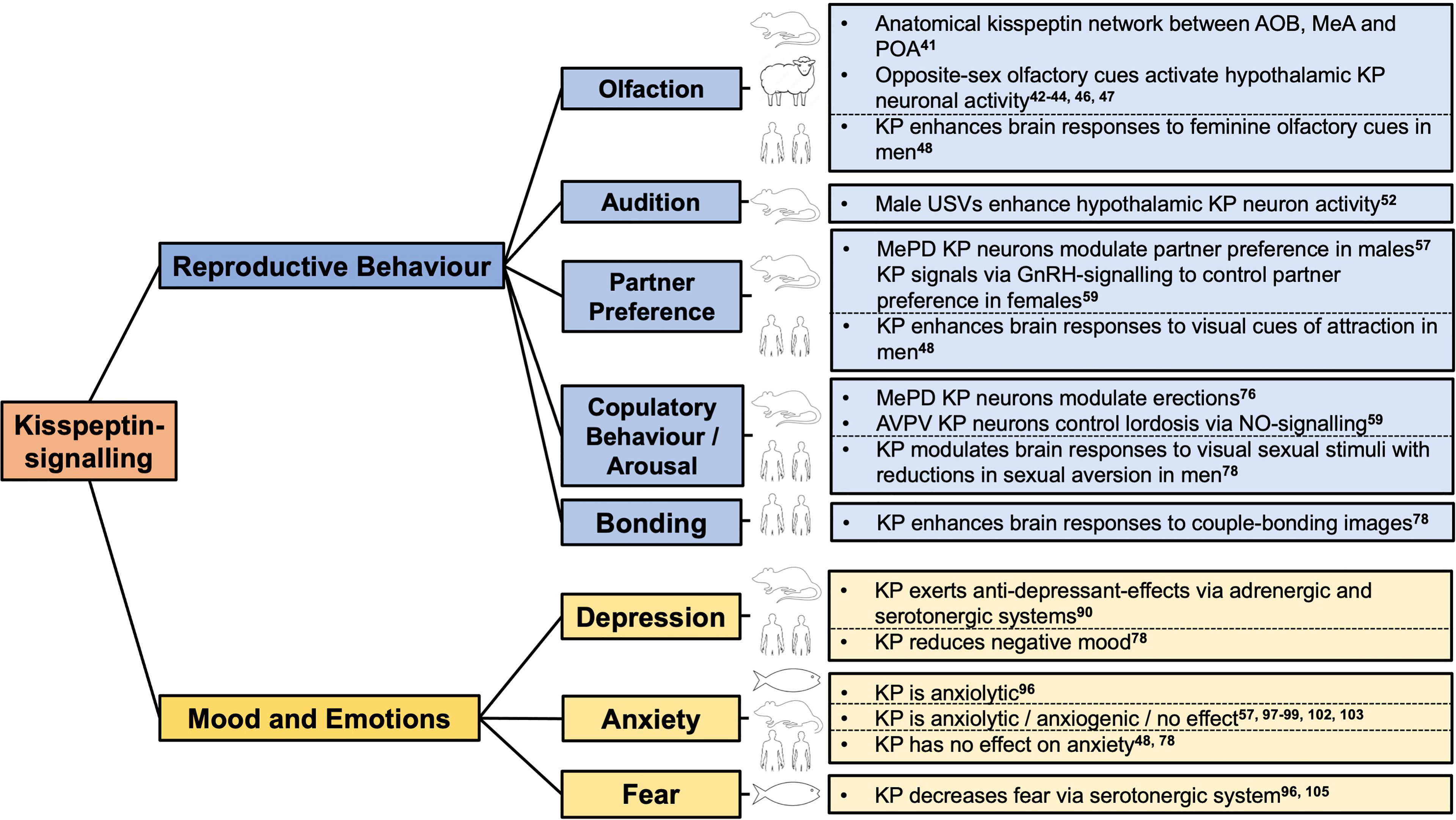
Figure 1 Kisspeptin-signalling has neuromodulatory effects on reproductive behaviour, mood and emotions in zebrafish to humans. AOB, accessory olfactory bulb; AVPV, anteroventral periventricular nucleus; GnRH, gonadotropin releasing hormone; KP, kisspeptin; MeA, medial amygdala; MePD, posterodorsal subnucleus of the medial amygdala; POA, preoptic area; USVs, ultrasonic vocalisations.
Audition
Beyond olfaction, acoustic signals are crucial for sexual communication, mate selection and ultimately coordinating reproduction (50). Curiously, abundant kisspeptin receptor expression is present in the rodent dorsal cochlear nucleus (33), which may reveal a plausible role for kisspeptin-signalling in auditory processing.
Preclinical Animal Studies
In rodents, the presence of a receptive female results in the male emitting song-like ultrasonic vocalisations (USV) to convey information about his motivational state and enhance female sexual approach (51). In terms of reproductive outcomes, a positive relationship between the number of female deliveries and the number of USV syllables emitted by male mice has been observed, revealing that breeding pairs in which males can emit USVs more frequently have more offspring (52). In keeping with this, female mice show more approach behaviour towards vocalising males than devocalised males (achieved by inferior laryngeal nerve sectioning). In a further experiment, female mice were placed in a soundproof chamber in a soundproof room and exposed to an audio file repeatedly for 20 minutes which consisted of either male mice USVs or control noise. Using this experimental paradigm, ARC kisspeptin neuronal activity (determined using dual-label immunocytochemistry with pCREB) significantly increased following exposure to male USVs, whereas neuronal activity in the AVPV was unaffected. Moreover, a moderate positive correlation was identified between ARC kisspeptin neuronal activity and the duration of female searching behaviour during the first five minutes of the USV playback, suggesting that the female’s approaching behaviour towards USVs of male mice relates to the activation of kisspeptin neurons (52). Taken together, this highlights that kisspeptin has the property to integrate vocal signals during sexual interaction with the central reproductive hormonal axis in rodents (Summarised in Figure 1). Whether vocal signals modulate the downstream release of reproductive hormones remains to be studied.
Human Studies
It has been shown that acoustic signals in humans can independently influence the perceived attractiveness of an individual (53). Indeed, clinical studies reveal that women’s preferences for men’s voices peaks around the time of ovulation (54), highlighting the possible involvement of reproductive hormones in the processing of auditory cues of attraction in humans. To date, there have been no human studies assessing the role of kisspeptin in auditory cues of attraction but would be an interesting avenue for future work based on the aforementioned nonhuman data.
Sexual Partner Preference and Sexual Motivation
In mammalian reproduction, sexually active males seek female conspecifics, while oestrous females approach males, with this sex-specific behaviour termed sexual partner preference (55). An increasing body of work implicates kisspeptin-signalling in the neuroendocrine modulation of sexual partner preference.
Preclinical Animal Studies
In a landmark study in 2007 which triggered much of the recent interest in kisspeptin and behaviour, whereas wildtype male mice spend >70% of their investigatory time with female stimulus animals, gonad intact Kiss1r knockout (KO) male mice exhibit no preference for either sex and spend an equivalent investigatory time with male and female conspecifics, despite normosmia (56). Of note, testosterone replacement in these mutant mice fails to restore normal partner preference (56), highlighting a key role for the kisspeptin receptor specifically in controlling sexual partner preference. Similarly, using the chemogenetic DREADDs (Designer Receptor Exclusively Activated by Designer Drugs) approach to selectively stimulate kisspeptin neurons in the posterodorsal subnucleus of the MeA (MePD), doubles the time male mice spend investigating an oestrous female over another gonadally-intact male (57).
Moreover, a very recent study examined the effects of kisspeptin on sexual motivation and its dependence on testosterone levels in male rodents (58). In this study, behavioural effects with an oestrous female were evaluated following intranasal administration of a GnRH analogue (buserelin), intraperitoneal kisspeptin or intranasal kisspeptin. Intranasal buserelin increased circulating testosterone levels but did not affect behavioural measures of sexual incentive motivation, whereas intraperitoneal kisspeptin increased both circulating testosterone and sexual motivation. Notably, intranasal kisspeptin also increased sexual motivation, despite not affecting circulating testosterone levels (58). Taken together, these data provide interesting insight for GnRH/testosterone-independent kisspeptin-effects to stimulate sexual motivation in male rodents.
A similar critical role for kisspeptin-signalling in regulating sexual partner preference in female rodents has been observed in a key elegant study. Ovariectomised and hormone-primed Kiss1 KO mice fail to display normal male-directed preference, whereas a single peripheral injection of kisspeptin resolves this deficit (59). Interestingly, selective viral ablation of AVPV kisspeptin neurons also results in female mice failing to display any male-direct preference, which again resolves following a single peripheral injection of kisspeptin (59), indicating site-specificity of the AVPV for kisspeptins control of mate preference in female mice. Furthermore, exploiting a transgenic GnRH deficient mouse model (which progressively loses GnRH expression during adulthood) results in female mice displaying female rather than male-directed preference, which normalises following a single peripheral injection of GnRH (but not kisspeptin) (59). This indicates that kisspeptin signals through GnRH neurons to specifically mediate sexual partner preference.
Human Studies
Visual cues are a fundamental aspect of human sexual attraction, with facial beauty known to provide a symbol of sexual potential and reproductive fitness (60, 61). Moreover, evidence reveals that prefrontal areas, in particular the medial pre-frontal cortex (mPFC), are heavily involved in the human perception of beauty (62–64). Consistent with this, in a recent study of healthy heterosexual men, peripheral kisspeptin administration increased activity in the mPFC and superior frontal gyrus (a further aesthetic brain region), in response to female faces (48). It is interesting that in this study, significant correlations were observed between kisspeptin-enhanced brain activity and relevant psychometric parameters. For instance, men with lower baseline sexual quality of life scores displayed higher brain responses to kisspeptin in the anterior cingulate cortex and insula when viewing less attractive female faces (48). From a functional perspective, these areas are implicated in sexual arousal (65), facial attraction (63, 66) and motivation towards reward (67, 68). Therefore, kisspeptin’s enhancement of these brain regions on viewing less attractive female faces may serve to strengthen feelings of reward, attraction and incentive motivation in individuals experiencing lower sexual quality of life, ultimately to encourage reproduction at a population level.
Copulatory Behaviour and Arousal
In female mammals, sexual receptivity (as a proxy for sexual motivation) relates to behavioural strategies required for fertile copulation with a male. It comprises the adoption of a posture which facilitates intravaginal ejaculation to occur, termed lordosis (69). In a sexually receptive female rodent, lordosis is characterised by dorsal flexion of the back and an immobile posture in response to stimulation by male mounting (70). Similarly, male sexual and copulatory behaviours include a succession of behavioural responses, which in rodents includes mounting, thrusting and intromitting behaviours before reaching ejaculation (71).
Preclinical Animal Studies
The MeA is a key brain region involved in sexual behaviour. Lesioning of the MeA in rats suppresses male copulatory behaviours such as erections and intromissions (72, 73). Although androgen receptors are present in the MeA (74), direct administration of androgens does not cause spontaneous erections (75), suggesting that additional factors are required to stimulate this physiological pathway. In keeping with this, microinjections of kisspeptin directly into the MeA of male rats dose-dependently stimulates ex-copula erections, an effect blocked by pre-treatment with a kisspeptin receptor antagonist (76). Furthermore, when kisspeptin is infused into the intracerebroventricular space, no erections are observed (despite comparable rises in circulating LH), demonstrating site-specificity of the MeA for kisspeptin’s effects on rodent erections and crucially suggests its role in this aspect of sexual behaviour is independent of downstream GnRH, LH or testosterone (76).
Regarding female rodents, in Kiss1 KO female mice, there is significant impairment of lordosis behaviour, which is rescued by a single peripheral injection of kisspeptin (59). Peripheral and direct intracerebroventricular injection of kisspeptin are also able to induce the lordosis reflex in wildtype adult females, highlighting the ability of peripheral kisspeptin to cross into the brain (59). A population of kisspeptin neurons in the rostral periventricular area of the third ventricle (RP3V) appear to have a central role in this behaviour as selective ablation of RP3V neurons leads to profound deficits in lordosis, whereas optogenetic stimulation of these neurons activates lordosis behaviour (59). A particularly important observation in this study was that kisspeptin’s effects on lordosis are GnRH-independent as GnRH is not able to induce lordosis behaviour in Kiss1 knockout females (59). Indeed, tract-tracing reveals that RP3V kisspeptin neurons project to another population of kisspeptin cells in the ventrolateral part of the ventromedial hypothalamus, which express neuronal nitric oxide synthase (nNOS) (59). Peripheral kisspeptin administration does not stimulate lordosis in nNOS knockout females, however, co-administration of kisspeptin with a nitric oxide donor is able to restore lordosis behaviour (59). More recently, the same group showed that direct injection of kisspeptin into the ventromedial hypothalamus stimulates lordosis, whereas direct injection of an nNOS inhibitor decreases lordosis (77). By contrast, no effect on lordosis is observed when kisspeptin is injected into the paraventricular nucleus (77). Taken together, this suggests that kisspeptin action via nitric oxide signalling in the ventromedial hypothalamus is essential for sexual behaviour in female mice.
Human Studies
In healthy heterosexual men, peripheral administration of kisspeptin enhances limbic brain activity (as determined by fMRI) in response to visual sexual stimuli, including the anterior and posterior cingulate and amygdala (78). Given that desire for sexual stimulation is an important component of the human sexual response (79), it is intriguing that in this study the greater kisspeptin enhanced limbic brain activity (including in the putamen) to sexual images, the less aversion to sex healthy men displayed (determined using behavioural tests). Moreover, in response to sexual images, kisspeptin was observed to activate limbic structures (including the hippocampus) more in men with lower baseline reward behavioural scores, which is highly relevant given that human sexual behaviour is closely associated with pleasure and reward. Of note, very recent data reveals that using the same administration protocol, kisspeptin does not affect brain responses to visual food stimuli or appetite parameters in healthy young men, assessed by fMRI and psychometric tests respectively (80), indicating that kisspeptin’s effects on limbic brain regions are specific to sexual and emotional stimuli. Furthermore, this latter recent study suggests that the effects of kisspeptin on human metabolism may be predominantly driven by changes in energy expenditure (81).
In addition to modulating human brain processing during stimulatory tasks, kisspeptin’s effects on resting brain connectivity have also been explored. Resting brain connectivity constitutes an important element of human behaviour (82) with the default mode network being the principle of these (83). In a subsequent fMRI analysis of healthy heterosexual men, peripheral administration of kisspeptin was observed to modulate the default mode network, which correlated with enhanced limbic brain activity in response to visual sexual images (84). Moreover, kisspeptin’s modulation of this network was greater in men with less reward drive and correlated with reduced sexual aversion (84). To date, there have been no human studies of kisspeptin’s effects on female sexual behaviour.
Collectively, experimental evidence reveals an important neuromodulatory role for kisspeptin-signalling in copulatory and sexual behaviours in rodents. In addition, clinical studies illustrate the emerging influence of kisspeptin in human sexual and emotional brain processing. Given these interesting findings, studies in patients with psychosexual dysfunction to translate these findings for patient benefit, are eagerly awaited (Summarised in Figure 1).
Bonding Behaviour
Attachment and emotional union with a partner are important components in mammalian reproduction (85). To date, kisspeptins role in bonding behaviour has only been explored in humans. Using fMRI, peripheral administration of kisspeptin to healthy men has been shown to enhance limbic brain activity, in response to non-sexual couple-bonding images (78). Specifically, this was observed to occur in the thalamus and globus pallidus, which are brain regions implicated in romantic love (86, 87), as well as the amygdala, also implicated in bonding (87). From a functional perspective, kisspeptin’s enhanced activation of the amygdala correlated with improvements in positive mood (78), implicating kisspeptin in the processing of bonding stimuli, which is important in driving reproduction from a behavioural level.
Mood and Emotions
Mood and emotions are important prerequisites for optimal reproductive health. In fact, mood disorders in humans are frequently associated with low sexual desire (88) and decreased fertility rates (89). In view of the intricate crosstalk between reproduction, mood and emotions, it is plausible that they are regulated by similar hormonal mechanisms.
Depression
Preclinical Animal Studies
Kisspeptins antidepressant-like effects have been identified in male mice using a modified forced swimming paradigm (90). During this test, rodents are placed into a container filled with water and assessed for active (swimming and climbing) and passive (immobility) behaviours, suggestive of anti-depressant and depressant effects, respectively (91). This study revealed that intracerebroventricular delivery of kisspeptin increased antidepressant-like behaviours (climbing and swimming times), whilst reducing the duration of immobility (90). Regarding interplay with downstream systems, pre-treatment with a nonselective α-adrenergic, α2-adrenergic or nonselective 5-HT2 serotonergic receptor blocker abolished kisspeptins anti-depressive actions, whereas pre-treatment with a cholinergic, dopaminergic or GABAergic receptor blocker had no effect on kisspeptins actions. Therefore, the results from these receptor blockade experiments indicate that kisspeptin can interplay with downstream adrenergic and serotonergic systems to bring about antidepressant-like effects (90).
Given the interaction between kisspeptin and the serotonergic system, it is interesting to consider the brain regions involved in eliciting the antidepressant-like effects. In a recent study, adult male rats received four weeks of intraperitoneal escitalopram (92), a widely prescribed selective serotonin reuptake inhibitor for mood disorders, which increases serotonin activity in the brain by limiting its reabsorption. This experimental paradigm significantly upregulated Kiss1 mRNA expression in the amygdala (272% increase compared to saline treated rats). Furthermore, Kiss1r mRNA was highly increased in the hypothalamus, hippocampus and cerebellum by 170%, 177% and 131%, respectively (92). Indeed, these findings are congruent with a previous study demonstrating that intra-cerebroventricular injections of serotonin hydrochloride to male rats significantly enhances hypothalamic kisspeptin expression and resultant circulating LH concentrations (93). Collectively, these data garner further evidence for the interplay between kisspeptin and the serotonergic system in eliciting antidepressant actions, as well as evidence for the putative brain regions involved.
Human Studies
In healthy young men peripheral administration of kisspeptin does not appear to affect positive mood during psychometric testing, whereas administration does reduce negative mood (78), in keeping with the aforementioned antidepressant-like effects in rodents (90). From a mechanistic perspective, brain responses (determined using fMRI) in this study revealed that kisspeptin administration enhanced frontal brain activity in response to participants specifically viewing negative images (e.g. pictures of car crash scenes) (78). This is especially pertinent given that frontal brain activity is important in human negative-mood regulation (94). Taken together, these studies in rodents and humans reveal antidepressant-like effects from kisspeptin-signalling (Summarised in Figure 1). An exciting direction for future clinical investigation will be determining whether kisspeptin’s positive stimulatory effects can be recapitulated in patients with mood disorders.
Anxiety
Preclinical Animal Studies
Similar to mood, anxiety and reproductive health are closely related, with anxiety an established trigger for sexual dysfunction (95). However, unlike the experimental evidence revealing an unambiguous and positive modulatory effect for kisspeptin-signalling in depression, its influence on anxiety-like behaviour remains to be fully clarified.
Intracranial administration of kisspeptin to zebrafish increases the number of top-to-bottom transitions during a novel tank diving test, indicating stimulatory exploratory behaviour and attenuated anxiety (96). Moreover, this was associated with a significant increase in c-fos mRNA expression in the ventral habenula (96), an evolutionarily conserved structure in the brain of vertebrates implicated in emotional decision-making (16). Regarding rodents, selectively stimulating MePD kisspeptin neurons in male mice using the pharmacosynthetic DREADDs approach has been observed to enhance social interaction between the test mouse and a same sex juvenile conspecific during a 5-minute study period by >50% (57). Using this experimental approach, selectively stimulating kisspeptin neurons in MePD also increases exploratory time in the open arms of an elevated plus maze (EPM) test almost 15-fold, indicating an anxiolytic effect (57).
Comparatively, other studies suggest anxiety-like behaviour related to kisspeptin. In male rats, central administration of kisspeptin dose-dependently reduces the number of entries into and the time spent in the open arms an EPM test, whilst inducing hyperthermia, spontaneous locomotor activity and dose-dependently increasing basal corticosterone levels, suggesting anxiogenic-like kisspeptin effects (97). Furthermore, anxiety-associated behaviour has been examined by generating a Kiss1r-deleted murine model, whereby the kisspeptin receptor was globally deleted, but rescued selectively in GnRH neurons, to ensure normal testosterone levels (98). This resulted in male mice spending twice as long in the open arms of an EPM (without affecting performance in the open field test), which may indicate that intact kisspeptin-signalling enhances anxiogenic responses to heights (98). Moreover, a recent study investigated whether central administration of the octapeptide fragment kisspeptin-8 influences anxiety responses in rodents (99). In this study, adult male rats displayed anxiogenic effects as suggested by decreased time in the open arm of an EPM and increased corticosterone levels (99). While kisspeptin can bind and activate neuropeptide FF receptors (100), which have been implicated in anxiety (101), administration of kisspeptin-8 in this study increased LH levels, suggesting kisspeptin receptor activation (99).
In contrast, further experiments suggest that kisspeptin has no modulatory effects on anxiety responses in rodents. In male rats, intraperitoneal administration of kisspeptin does not affect levels of plasma adrenocorticotropic hormone and corticosterone under basal or stress-induced conditions (achieved by 30-minutes of restraint in a plastic rodent restraint tube) (102). Similarly, central administration of kisspeptin to male rats has been observed not to influence stress-related behaviours, including locomotive, grooming and sleeping behaviour (103).
Human Studies
Congruent with the latter rodent data, peripheral administration of kisspeptin in two separate clinical studies has been observed not to affect psychometric measures of anxiety or circulating cortisol levels in healthy men (48, 78).
Taken together, this indicates that depending on the species examined, the route of kisspeptin administration and the experimental model employed, kisspeptin-signalling may have an anxiolytic (57, 96), anxiogenic (97–99) or have no effects on anxiety (48, 78, 102, 103) (Summarised in Figure 1). Further studies (particularly in rodents where results are conflicting) are therefore required to clarify this contradictory area in kisspeptin biology. However, human data is currently reassuring given the future potential for kisspeptin-based therapeutics.
Fear
Fear is associated with impaired reproductive performance (104), triggering studies examining the influence of kisspeptin-signalling in modulating fear.
Preclinical Animal Studies
In zebrafish, both Kiss1 and Kiss1r are co-expressed in the ventral habenula (17, 18). From this key evolutionary conserved brain structure, Kiss1 and Kiss1r neuronal projections are sent to the median raphe (105), which resides near serotonergic neurons (106). By means of an alarm-substance-evoked fear paradigm in zebrafish, intracranial administration of Kiss1 (Kiss1-15), but not Kiss2 (Kiss2-10), has been observed to reduce erratic movements and freezing behaviour (i.e, behavioural parameters indicative of fear) and dose-dependently increase serotonin-related gene expression (pet-1 and slc6a4a mRNA levels), demonstrating that habenula kisspeptin modulates the serotonergic system to attenuate fear (96). Moreover, pharmacological blockade using methysergide (a 5-HT1A and 5-HT2 receptor antagonist) blocks the effect of intracranial kisspeptin administration to reduce fear (105). Taken together, these data highlight that interplay between kisspeptin-signalling and the serotonin system (via 5-HT1A and 5-HT2 receptors) decreases the fear response in zebrafish (Summarised in Figure 1). In view of these findings, further studies are warranted to examine the fear-altering effects of kisspeptin in non-piscine species, such as humans where no studies have currently been performed as yet.
Interplay with Downstream Systems to Modulate Behaviour
Given the central role of kisspeptin-signalling in governing the aforementioned essential behaviours required for successful reproduction, it is interesting to consider the possible mechanisms. Kisspeptin may be directly acting on kisspeptin receptors expressed in key limbic and paralimbic brain structures (8–11) to regulate behaviour or indirectly through downstream reproductive signals, although as discussed above there is robust experimental evidence of roles independent of these downstream reproductive signals (such as GnRH, LH and gonadal steroids). In addition, kisspeptin also interacts with a plethora of additional downstream neuroendocrine systems as discussed below.
Preclinical Animal Studies
In the rodent amygdala, fluorescence immunochemistry reveals that kisspeptin neurons reside in close apposition and receive putative innervation from vasopressinergic and dopaminergic neurons (41). Furthermore, MeA kisspeptin neurons are a mixed population in which 71% express markers for GABAergic (Vgat) and 29% for glutamatergic (Vglut2) neurotransmission in male mice (44). In view of the established functions these neurotransmitter systems have in regulating behaviour (107–109), could suggest their interplay with kisspeptin-signalling to bring about these behavioural effects.
Regarding female rodents, whereas sexual partner preference depends on downstream GnRH-signalling (59), interplay with NO in the ventromedial hypothalamus is vital for kisspeptins role in regulating the key reproductive behaviour of lordosis (77). Moreover, kisspeptin induces antidepressant-like actions in rodents through adrenergic and serotonergic systems (90). Consistent with this, kisspeptin neurons in the habenula modulates the serotonergic system to suppress the fear response in zebrafish (96, 105).
Human Studies
Until recently, there was no data regarding kisspeptin’s interplay with downstream neuropeptide or neurotransmitters outside of the reproductive hormonal axis in humans. Of note, a range of animal models reveal that kisspeptin interacts with the key inhibitory neurotransmitter gamma-aminobutyric acid (GABA) (110–114). In keeping with this, a recent study employed proton magnetic resonance spectroscopy to examine the in vivo effects of kisspeptin administration on central GABA levels in the human brain (115). Using this approach, peripheral kisspeptin administration resulted in a 15% decrease in total endogenous GABA concentrations in the anterior cingulate cortex of healthy men (115). This is particularly relevant given that a comparable magnitude of GABA change has previously been reported in psychological studies with functional significance (116, 117).
Collectively, these data reveal that while the mood and behavioural effects of kisspeptin may be the direct action of kisspeptin on its receptor, they may also be the result of interplay between kisspeptin and these additional downstream neuropeptides and neurotransmitter systems with kisspeptin serving as the overall conductor. Further studies examining other downstream neuroendocrine systems of interest are warranted in humans, particularly given the recent in vivo data provided by proton magnetic resonance spectroscopy.
Conclusion
Reproduction and the related behavioural strategies that are required to produce offspring are intricately linked processes. Indeed, disturbances in these behaviours can have detrimental effects on reproduction, suggesting coordination through similar hormonal mechanisms. Furthering our knowledge in this regard has major clinical significance, given the prevalence of psychosexual dysfunction (118) and the associated detrimental effects on quality of life, interpersonal relationships and fertility (88, 119, 120).
The reproductive neuropeptide kisspeptin sits at the apex of the HPG axis, where it has important roles in controlling downstream reproductive hormone secretion (6, 7). Beyond the hypothalamus, kisspeptin and its receptor are located throughout the rodent and human limbic systems (8–11), providing an anatomical framework for the important modulatory roles kisspeptin-signalling plays in reproductive behaviour. As discussed throughout this review, data from animal models reveals stimulatory effects on all aspects from olfactory-mediated sexual partner preference and copulatory behaviours, through to controlling mood and emotions in a presumed drive towards reproduction. Crucially, current limitations in the studies of kisspeptin-signalling in behavioural modulation need to be acknowledged. For instance, kisspeptin can potentially modulate activity in several brain regions based on its gene and receptor expression pattern as well as human functional MRI findings above. However, the importance of distinct kisspeptin populations beyond the studied hypothalamus, amygdala and hippocampus (such as the thalamus) with regards to behavioural roles remain to be clarified in animal models. Indeed, the application of novel chemogenetic (such as DREADDs) or optogenetic techniques to selectively stimulate or inhibit kisspeptin neuronal populations (44, 57) will allow investigation of endogenous kisspeptin-signalling in these unexplored brain regions in future. This is scientifically important as although human functional MRI studies have identified several brain regions involved in behaviour following kisspeptin administration, the precise neuronal subtypes (e.g. kisspeptin, GABA, dopamine etc) cannot be identified by fMRI studies. However, recent proton magnetic spectroscopy studies (for GABA) have provided some recent human data in this regard (115). An additional limitation of the behavioural studies so far is that certain behaviours have been investigated exclusively in one sex (e.g., anxiety in male animals/humans, or human sexual behaviour as yet only in men), therefore additional studies are warranted to examine for sexual dimorphisms.
Building on the animal literature and from a translational perspective, modern functional neuroimaging techniques (such as fMRI and spectroscopy) have been indispensable to investigate the role of kisspeptin in human sexual and emotional brain processing. Given these findings, studies examining kisspeptin in patients with psychosexual and mood disorders are much needed to improve our knowledge of the neuromodulatory effects of kisspeptin in health and disease. To this end, combined with the available updated literature, these data would provide fundamental mechanistic and pharmacological insight for the neurophysiological actions of kisspeptin and unlock kisspeptin as a therapeutic target for the management of reproductive, and related psychological and psychosexual disorders.
Author Contributions
EM and LY, researched the material and wrote the first draft. EM and LY, joint first authors. WD and AC, co-corresponding and senior authors. All authors contributed to the article and approved the submitted version.
Funding
This article presents independent research funded by the Medical Research Council (MRC) and supported by National Institute for Health Research (NIHR) Imperial Biomedical Research Centre and NIHR Clinical Research Facility. The views expressed are those of the authors and not necessarily those of the MRC, NIHR or the Department of Health.
EM and LY are funded by MRC Clinical Research Training Fellowships (MR/T006242/1 and MR/R000484/1), AA by an NIHR Clinician Scientist Fellowship (CS-2018-18-ST2–002), WD by an NIHR Research Professorship (NIHR RP-2014-05-001) and NIHR Senior Investigator Award, and AC by the National Health Service.
Conflict of Interest
The authors declare that the research was conducted in the absence of any commercial or financial relationships that could be construed as a potential conflict of interest.
Publisher’s Note
All claims expressed in this article are solely those of the authors and do not necessarily represent those of their affiliated organizations, or those of the publisher, the editors and the reviewers. Any product that may be evaluated in this article, or claim that may be made by its manufacturer, is not guaranteed or endorsed by the publisher.
References
1. Schiller CE, Johnson SL, Abate AC, Schmidt PJ, Rubinow DR. Reproductive Steroid Regulation of Mood and Behavior. Compr Physiol (2016) 6:1135–60. doi: 10.1002/cphy.c150014
2. Snoeren EMS. Female Reproductive Behavior. Curr Topics Behav Neurosci (2019) 43:1–44. doi: 10.1007/7854_2018_68
3. Lenschow C, Lima SQ. In the Mood for Sex: Neural Circuits for Reproduction. Curr Opin Neurobiol (2020) 60:155–68. doi: 10.1016/j.conb.2019.12.001
4. Berridge KC, Kringelbach ML. Affective Neuroscience of Pleasure: Reward in Humans and Animals. Psychopharmacol (Berl) (2008) 199:457–80. doi: 10.1007/s00213-008-1099-6
5. Jennings KJ, de Lecea L. Neural and Hormonal Control of Sexual Behavior. Endocrinology (2020) 161:bqaa150. doi: 10.1210/endocr/bqaa150
6. de Roux N, Genin E, Carel J-C, Matsuda F, Chaussain J-L, Milgrom E. Hypogonadotropic Hypogonadism Due to Loss of Function of the KiSS1-Derived Peptide Receptor Gpr54. Proc Natl Acad Sci (2003) 100:10972–6. doi: 10.1073/pnas.1834399100
7. Seminara SB, Messager S, Chatzidaki EE, Thresher RR, Acierno JS, Shagoury JK, et al. The GPR54 Gene as a Regulator of Puberty. N Engl J Med (2003) 349:1614–27. doi: 10.1056/NEJMoa035322
8. Lee DK, Nguyen T, O’Neill GP, Cheng R, Liu Y, Howard AD, et al. Discovery of a Receptor Related to the Galanin Receptors. FEBS Lett (1999) 446:103–7. doi: 10.1016/S0014-5793(99)00009-5
9. Muir AI, Chamberlain L, Elshourbagy NA, Michalovich D, Moore DJ, Calamari A, et al. AXOR12, a Novel Human G Protein-Coupled Receptor, Activated by the Peptide KiSS-1. J Biol Chem (2001) 276:28969–75. doi: 10.1074/jbc.M102743200
10. Kotani M, Detheux M, Vandenbogaerde A, Communi D, Vanderwinden JM, le Poul E, et al. The Metastasis Suppressor Gene KiSS-1 Encodes Kisspeptins, the Natural Ligands of the Orphan G Protein-Coupled Receptor Gpr54. J Biol Chem (2001) 276:34631–6. doi: 10.1074/jbc.M104847200
11. Ohtaki T, Shintani Y, Honda S, Matsumoto H, Hori A, Kanehashi K, et al. Metastasis Suppressor Gene KiSS-1 Encodes Peptide Ligand of a G-Protein-Coupled Receptor. Nature (2001) 411:613–7. doi: 10.1038/35079135
12. Lee JH, Miele ME, Hicks DJ, Phillips KK, Trent JM, Weissman BE, et al. KiSS-1, a Novel Human Malignant Melanoma Metastasis-Suppressor Gene. J Natl Cancer Inst (1996) 88:1731–7. doi: 10.1093/jnci/88.23.1731
13. Teles MG, Bianco SDC, Brito VN, Trarbach EB, Kuohung W, Xu S, et al. A GPR54-Activating Mutation in a Patient With Central Precocious Puberty. N Engl J Med (2008) 358:709–15. doi: 10.1056/NEJMoa073443
14. Pinilla L, Aguilar E, Dieguez C, Millar RP, Tena-Sempere M. Kisspeptins and Reproduction: Physiological Roles and Regulatory Mechanisms. Physiol Rev (2012) 92:1235–316. doi: 10.1152/physrev.00037.2010
15. Parhar IS, Ogawa S, Sakuma Y. Laser-Captured Single Digoxigenin-Labeled Neurons of Gonadotropin-Releasing Hormone Types Reveal a Novel G Protein-Coupled Receptor (Gpr54) During Maturation in Cichlid Fish. Endocrinology (2004) 145:3613–8. doi: 10.1210/en.2004-0395
16. Ogawa S, Parhar IS. Functions of Habenula in Reproduction and Socio-Reproductive Behaviours. Front Neuroendocrinol (2022) 64:100964. doi: 10.1016/j.yfrne.2021.100964
17. Kitahashi T, Ogawa S, Parhar IS. Cloning and Expression of Kiss2 in the Zebrafish and Medaka. Endocrinology (2009) 150:821–31. doi: 10.1210/en.2008-0940
18. Ogawa S, Parhar IS. Biological Significance of Kisspeptin-Kiss 1 Receptor Signaling in the Habenula of Teleost Species. Front Endocrinol (2018) 9:222. doi: 10.3389/fendo.2018.00222
19. Tang H, Liu Y, Luo D, Ogawa S, Yin Y, Li S, et al. The Kiss/Kissr Systems are Dispensable for Zebrafish Reproduction: Evidence From Gene Knockout Studies. Endocrinology (2015) 156:589–99. doi: 10.1210/en.2014-1204
20. Liu Y, Tang H, Xie R, Li S, Liu X, Lin H, et al. Genetic Evidence for Multifactorial Control of the Reproductive Axis in Zebrafish. Endocrinology (2017) 158:604–11. doi: 10.1210/en.2016-1540
21. Nakajo M, Kanda S, Karigo T, Takahashi A, Akazome Y, Uenoyama Y, et al. Evolutionally Conserved Function of Kisspeptin Neuronal System Is Nonreproductive Regulation as Revealed by Nonmammalian Study. Endocrinology (2018) 159:163–83. doi: 10.1210/en.2017-00808
22. Etzion T, Zmora N, Zohar Y, Levavi-Sivan B, Golan M, Gothilf Y. Ectopic Over Expression of Kiss1 may Compensate for the Loss of Kiss2. Gen Comp Endocrinol (2020) 295:113523. doi: 10.1016/j.ygcen.2020.113523
23. Sivalingam M, Parhar IS. Hypothalamic Kisspeptin and Kisspeptin Receptors: Species Variation in Reproduction and Reproductive Behaviours. Front Neuroendocrinol (2022) 64:100951. doi: 10.1016/j.yfrne.2021.100951
24. Gottsch ML, Cunningham MJ, Smith JT, Popa SM, Acohido B v, Crowley WF, et al. A Role for Kisspeptins in the Regulation of Gonadotropin Secretion in the Mouse. Endocrinology (2004) 145:4073–7. doi: 10.1210/en.2004-0431
25. Mikkelsen JD, Simonneaux V. The Neuroanatomy of the Kisspeptin System in the Mammalian Brain. Pept (NY) (2009) 30:26–33. doi: 10.1016/j.peptides.2008.09.004
26. Clarkson J, Tassigny Xd’Anglemontde, Colledge WH, Caraty A, Herbison AE. Distribution of Kisspeptin Neurones in the Adult Female Mouse Brain. J Neuroendocrinol (2009) 21:673–82. doi: 10.1111/j.1365-2826.2009.01892.x
27. Goodman RL, Lehman MN, Smith JT, Coolen LM, de Oliveira CVR, Jafarzadehshirazi MR, et al. Kisspeptin Neurons in the Arcuate Nucleus of the Ewe Express Both Dynorphin A and Neurokinin B. Endocrinology (2007) 148:5752–60. doi: 10.1210/en.2007-0961
28. Rometo AM, Krajewski SJ, Voytko ML, Rance NE. Hypertrophy and Increased Kisspeptin Gene Expression in the Hypothalamic Infundibular Nucleus of Postmenopausal Women and Ovariectomized Monkeys. J Clin Endocrinol Metab (2007) 92:2744–50. doi: 10.1210/jc.2007-0553
29. Hrabovszky E, Molnár CS, Sipos MT, Vida B, Ciofi P, Borsay BA, et al. Sexual Dimorphism of Kisspeptin and Neurokinin B Immunoreactive Neurons in the Infundibular Nucleus of Aged Men and Women. Front Endocrinol (2011) 2:80. doi: 10.3389/fendo.2011.00080
30. Rumpler É, Skrapits K, Takács S, Göcz B, Trinh SH, Rácz G, et al. Characterization of Kisspeptin Neurons in the Human Rostral Hypothalamus. Neuroendocrinology (2021) 111:249–62. doi: 10.1159/000507891
31. Molnár CS, Vida B, Sipos MT, Ciofi P, Borsay BÁ, Rácz K, et al. Morphological Evidence for Enhanced Kisspeptin and Neurokinin B Signaling in the Infundibular Nucleus of the Aging Man. Endocrinology (2012) 153:5428–39. doi: 10.1210/en.2012-1739
32. Hrabovszky E, Sipos MT, Molnár CS, Ciofi P, Borsay BÁ, Gergely P, et al. Low Degree of Overlap Between Kisspeptin, Neurokinin B, and Dynorphin Immunoreactivities in the Infundibular Nucleus of Young Male Human Subjects Challenges the KNDy Neuron Concept. Endocrinology (2012) 153:4978–89. doi: 10.1210/en.2012-1545
33. Herbison AE, de Tassigny XDA, Doran J, Colledge WH. Distribution and Postnatal Development of Gpr54 Gene Expression in Mouse Brain and Gonadotropin-Releasing Hormone Neurons. Endocrinology (2010) 151:312–21. doi: 10.1210/en.2009-0552
34. Yang L, Comninos AN, Dhillo WS. Intrinsic Links Among Sex, Emotion, and Reproduction. Cell Mol Life Sci (2018) 75:2197–210. doi: 10.1007/s00018-018-2802-3
35. Terao Y, Kumano S, Takatsu Y, Hattori M, Nishimura A, Ohtaki T, et al. Expression of KiSS-1, a Metastasis Suppressor Gene, in Trophoblast Giant Cells of the Rat Placenta. Biochim Biophys Acta (BBA) - Gene Struct Expression (2004) 1678:102–10. doi: 10.1016/j.bbaexp.2004.02.005
36. Son HE, Kim KM, Kim EJ, Jang WG. Kisspeptin-10 (KP-10) Stimulates Osteoblast Differentiation Through GPR54-Mediated Regulation of BMP2 Expression and Activation. Sci Rep (2018) 8:2134. doi: 10.1038/s41598-018-20571-2
37. Zhang P, Tang M, Zhong T, Lin Y, Zong T, Zhong C, et al. Expression and Function of Kisspeptin During Mouse Decidualization. PLoS One (2014) 9:e97647. doi: 10.1371/journal.pone.0097647
38. Mei H, Doran J, Kyle V, Yeo SH, Colledge WH. Does Kisspeptin Signaling Have a Role in the Testes? Front Endocrinol (2013) 4:198. doi: 10.3389/fendo.2013.00198
39. Comninos AN, Hansen MS, Courtney A, Choudhury S, Yang L, Mills EG, et al. Acute Effects of Kisspeptin Administration on Bone Metabolism in Healthy Men. J Clin Endocrinol Metab (2022) 107:1529–40. doi: 10.1210/clinem/dgac117
40. Parkes AS, Bruce HM. Olfactory Stimuli in Mammalian Reproduction. Science (1961) 134:1049–54. doi: 10.1126/science.134.3485.1049
41. Pineda R, Plaisier F, Millar RP, Ludwig M. Amygdala Kisspeptin Neurons: Putative Mediators of Olfactory Control of the Gonadotropic Axis. Neuroendocrinology (2017) 104:223–8. doi: 10.1159/000445895
42. Watanabe Y, Ikegami K, Ishigaki R, Ieda N, Uenoyama Y, Maeda KI, et al. Enhancement of the Luteinising Hormone Surge by Male Olfactory Signals Is Associated With Anteroventral Periventricular Kiss1 Cell Activation in Female Rats. J Neuroendocrinol (2017) 29:e12505. doi: 10.1111/jne.12505
43. Bakker J, Pierman S, González-Martínez D. Effects of Aromatase Mutation (ArKO) on the Sexual Differentiation of Kisspeptin Neuronal Numbers and Their Activation by Same Versus Opposite Sex Urinary Pheromones. Horm Behav (2010) 57:390–5. doi: 10.1016/j.yhbeh.2009.11.005
44. Aggarwal S, Tang C, Sing K, Kim HW, Millar RP, Tello JA. Medial Amygdala Kiss1 Neurons Mediate Female Pheromone Stimulation of Luteinizing Hormone in Male Mice. Neuroendocrinology (2019) 108:172–89. doi: 10.1159/000496106
45. Fabre-Nys C, Kendrick KM, Scaramuzzi RJ. The “Ram Effect”: New Insights Into Neural Modulation of the Gonadotropic Axis by Male Odors and Socio-Sexual Interactions. Front Neurosci (2015) 9:111. doi: 10.3389/fnins.2015.00111
46. Murata K, Wakabayashi Y, Sakamoto K, Tanaka T, Takeuchi Y, Mori Y, et al. Effects of Brief Exposure of Male Pheromone on Multiple-Unit Activity at Close Proximity to Kisspeptin Neurons in the Goat Arcuate Nucleus. J Reprod Dev (2011) 57:10–70. doi: 10.1262/jrd.10-070E
47. de Bond JAP, Li Q, Millar RP, Clarke IJ, Smith JT. Kisspeptin Signaling Is Required for the Luteinizing Hormone Response in Anestrous Ewes Following the Introduction of Males. PLoS One (2013) 8:e57972. doi: 10.1371/journal.pone.0057972
48. Yang L, Demetriou L, Wall MB, Mills EGA, Zargaran D, Sykes M, et al. Kisspeptin Enhances Brain Responses to Olfactory and Visual Cues of Attraction in Men. JCI Insight (2020) 5:e133633. doi: 10.1172/jci.insight.133633
49. Fjaeldstad A, Fernandes HM, van Hartevelt TJ, Gleesborg C, Møller A, Ovesen T, et al. Brain Fingerprints of Olfaction: A Novel Structural Method for Assessing Olfactory Cortical Networks in Health and Disease. Sci Rep (2017) 7:1–13. doi: 10.1038/srep42534
50. Charlton BD, Martin-Wintle MS, Owen MA, Zhang H, Swaisgood RR. Vocal Behaviour Predicts Mating Success in Giant Pandas. R Soc Open Sci (2018) 5:181323. doi: 10.1098/rsos.181323
51. Dombret C, Capela D, Poissenot K, Parmentier C, Bergsten E, Pionneau C, et al. Neural Mechanisms Underlying the Disruption of Male Courtship Behavior by Adult Exposure to Di(2-Ethylhexyl) Phthalate in Mice. Environ Health Perspect (2017) 125:087001. doi: 10.1289/EHP1443
52. Asaba A, Osakada T, Touhara K, Kato M, Mogi K, Kikusui T. Male Mice Ultrasonic Vocalizations Enhance Female Sexual Approach and Hypothalamic Kisspeptin Neuron Activity. Horm Behav (2017) 94:53–60. doi: 10.1016/j.yhbeh.2017.06.006
53. Groyecka A, Pisanski K, Sorokowska A, Havlícek J, Karwowski M, Puts D, et al. Attractiveness Is Multimodal: Beauty Is Also in the Nose and Ear of the Beholder. Front Psychol (2017) 8:778. doi: 10.3389/fpsyg.2017.00778
54. Pisanski K, Hahn AC, Fisher CI, DeBruine LM, Feinberg DR, Jones BC. Changes in Salivary Estradiol Predict Changes in Women’s Preferences for Vocal Masculinity. Horm Behav (2014) 66:493–7. doi: 10.1016/j.yhbeh.2014.07.006
55. Kondo Y, Hayashi H. Neural and Hormonal Basis of Opposite-Sex Preference by Chemosensory Signals. Int J Mol Sci (2021) 22:8311. doi: 10.3390/ijms22158311
56. Kauffman AS, Park JH, McPhie-Lalmansingh AA, Gottsch ML, Bodo C, Hohmann JG, et al. The Kisspeptin Receptor GPR54 Is Required for Sexual Differentiation of the Brain and Behavior. J Neurosci (2007) 27:8826–35. doi: 10.1523/JNEUROSCI.2099-07.2007
57. Adekunbi DA, Li XF, Lass G, Shetty K, Adegoke OA, Yeo SH, et al. Kisspeptin Neurones in the Posterodorsal Medial Amygdala Modulate Sexual Partner Preference and Anxiety in Male Mice. J Neuroendocrinol (2018) 30:e12572. doi: 10.1111/jne.12572
58. Magarramova LA, Tissen IY, Blazhenko AA, Lebedev AA, Loskutov SI, Proshin SN. Kisspeptin is Testosterone Independent Regulator of Sexual Motivation in Male Rats. J Exp Biol Agric Sci (2022) 10:131–4. doi: 10.18006/2022.10(1).131.134
59. Hellier V, Brock O, Candlish M, Desroziers E, Aoki M, Mayer C, et al. Female Sexual Behavior in Mice is Controlled by Kisspeptin Neurons. Nat Commun (2018) 9:400. doi: 10.1038/s41467-017-02797-2
60. Perrett DI, Lee KJ, Penton-Voak I, Rowland D, Yoshikawa S, Burt DM, et al. Effects of Sexual Dimorphism on Facial Attractiveness. Nature (1998) 6696:884–7. doi: 10.1038/29772
61. Penton-Voak IS, Perrett DI, Castles DL, Kobayashi T, Burt DM, Murray LK, et al. Menstrual Cycle Alters Face Preference. Nature (1999) 399:741–2. doi: 10.1038/21557
62. Aharon I, Etcoff N, Ariely D, Chabris CF, O’Connor E, Breiter HC. Beautiful Faces Have Variable Reward Value: fMRI and Behavioral Evidence. Neuron (2001) 32:537–51. doi: 10.1016/S0896-6273(01)00491-3
63. O’Doherty J, Winston J, Critchley H, Perrett D, Burt DM, Dolan RJ. Beauty in a Smile: The Role of Medial Orbitofrontal Cortex in Facial Attractiveness. Neuropsychologia (2003) 41:147–55. doi: 10.1016/S0028-3932(02)00145-8
64. Cela-Conde CJ, Marty G, Maestu F, Ortiz T, Munar E, Fernandez A, et al. Activation of the Prefrontal Cortex in the Human Visual Aesthetic Perception. Proc Natl Acad Sci (2004) 101:6321–5. doi: 10.1073/pnas.0401427101
65. Arnow BA, Desmond JE, Banner L, Glover GH, Solomon A, Polan ML, et al. Brain Activation During Sexual Arousal in Healthy Heterosexual Males. Brain (2002) 125:1014–23. doi: 10.1093/brain/awf108
66. Winston JS, O’Doherty J, Kilner JM, Perrett DI, Dolan RJ, O’Doherty J, et al. Brain Systems for Assessing Facial Attractiveness. Neuropsychologia (2007) 45:195–206. doi: 10.1016/j.neuropsychologia.2006.05.009
67. Holroyd CB, Yeung N. Motivation of Extended Behaviors by Anterior Cingulate Cortex. Trends Cognit Sci (2012) 16:122–8. doi: 10.1016/j.tics.2011.12.008
68. Murty VP, LaBar KS, Adcock RA. Distinct Medial Temporal Networks Encode Surprise During Motivation by Reward Versus Punishment. Neurobiol Learn Mem (2016) 134:55–64. doi: 10.1016/j.nlm.2016.01.018
69. Tsukahara S, Kanaya M, Yamanouchi K. Neuroanatomy and Sex Differences of the Lordosis-Inhibiting System in the Lateral Septum. Front Neurosci (2014) 8:299. doi: 10.3389/fnins.2014.00299
70. Guarraci FA, Frohardt RJ. “What a Girl Wants”: What Can We Learn From Animal Models of Female Sexual Motivation? Front Behav Neurosci (2019) 13:216. doi: 10.3389/fnbeh.2019.00216
71. Hull EM, Rodríguez-Manzo G. Male Sexual Behavior. In: Hormones, Brain and Behavior, 3rd ed. Netherlands: Elsevier (2016). p. 1–57.
72. Kondo Y, Arai Y. Functional Association Between the Medial Amygdala and the Medial Preoptic Area in Regulation of Mating Behavior in the Male Rat. Physiol Behav (1995) 57:69–73. doi: 10.1016/0031-9384(94)00205-J
73. Kondo Y, Sachs BD, Sakuma Y. Importance of the Medial Amygdala in Rat Penile Erection Evoked by Remote Stimuli From Estrous Females. Behav Brain Res (1997) 88:153–60. doi: 10.1016/S0166-4328(97)02287-0
74. Cooke BM, Breedlove SM, Jordan CL. Both Estrogen Receptors and Androgen Receptors Contribute to Testosterone-Induced Changes in the Morphology of the Medial Amygdala and Sexual Arousal in Male Rats. Horm Behav (2003) 43:336–46. doi: 10.1016/S0018-506X(02)00047-8
75. Bialy M, Sachs BD. Androgen Implants in Medial Amygdala Briefly Maintain Noncontact Erection in Castrated Male Rats. Horm Behav (2002) 42:345–55. doi: 10.1006/hbeh.2002.1821
76. Gresham R, Li S, Adekunbi DA, Hu M, Li XF, TO’Byrne K. Kisspeptin in the Medial Amygdala and Sexual Behavior in Male Rats. Neurosci Lett (2016) 627:13–7. doi: 10.1016/j.neulet.2016.05.042
77. Bentefour Y, Bakker J. Kisspeptin Signaling and nNOS Neurons in the VMHvl Modulate Lordosis Behavior But Not Mate Preference in Female Mice. Neuropharmacology (2021) 198:108762. doi: 10.1016/j.neuropharm.2021.108762
78. Comninos AN, Wall MB, Demetriou L, Shah AJ, Clarke SA, Narayanaswamy S, et al. Kisspeptin Modulates Sexual and Emotional Brain Processing in Humans. J Clin Invest (2017) 127:709–19. doi: 10.1172/JCI89519
79. Kaplan HS. New Sex Therapy - Active Treatment of Sexual Dysfunction. 1st edition, New York: Routledge. (1974) p. 1–560.
80. Yang L, Demetriou L, Wall MB, Mills EG, Wing VC, Thurston L, et al. The Effects of Kisspeptin on Brain Response to Food Images and Psychometric Parameters of Appetite in Healthy Men. J Clin Endocrinol Metab (2020) 106:e1837–48. doi: 10.1210/clinem/dgaa746
81. Mills EG, Izzi-Engbeaya C, Abbara A, Comninos AN, Dhillo WS. Functions of Galanin, Spexin and Kisspeptin in Metabolism, Mood and Behaviour. Nat Rev Endocrinol (2021) 17:97–113. doi: 10.1038/s41574-020-00438-1
82. Fox MD, Raichle ME. Spontaneous Fluctuations in Brain Activity Observed With Functional Magnetic Resonance Imaging. Nat Rev Neurosci (2007) 8:700–11. doi: 10.1038/nrn2201
83. Raichle ME, MacLeod AM, Snyder AZ, Powers WJ, Gusnard DA, Shulman GL. A Default Mode of Brain Function. Proc Natl Acad Sci USA (2001) 98:676–82. doi: 10.1073/pnas.98.2.676
84. Comninos AN, Demetriou L, Wall MB, Shah AJ, Clarke SA, Narayanaswamy S, et al. Modulations of Human Resting Brain Connectivity by Kisspeptin Enhance Sexual and Emotional Functions. JCI Insight (2018) 3:e121958. doi: 10.1172/jci.insight.121958
85. Fisher HE. Lust, Attraction, and Attachment in Mammalian Reproduction. Hum Nat (1998) 9:23–52. doi: 10.1007/s12110-998-1010-5
86. Acevedo BP, Aron A, Fisher HE, Brown LL. Neural Correlates of Long-Term Intense Romantic Love. Soc Cogn Affect Neurosci (2012) 7:145–59. doi: 10.1093/scan/nsq092
87. Young LJ, Wang Z. The Neurobiology of Pair Bonding. Nat Neurosci (2004) 7:1048–54. doi: 10.1038/nn1327
88. Pastuszak AW, Badhiwala N, Lipshultz LI, Khera M. Depression is Correlated With the Psychological and Physical Aspects of Sexual Dysfunction in Men. Int J Impot Res (2013) 25:194–9. doi: 10.1038/ijir.2013.4
89. Williams KE, Marsh WK, Rasgon NI. Mood Disorders and Fertility in Women: A Critical Review of the Literature and Implications for Future Research. Hum Reprod Update (2007) 13:607–16. doi: 10.1093/humupd/dmm019
90. Tanaka M, Csabafi K, Telegdy G. Neurotransmissions of Antidepressant-Like Effects of Kisspeptin-13. Regul Pept (2013) 180:1–4. doi: 10.1016/j.regpep.2012.08.017
91. Ramaker MJ, Dulawa SC. Identifying Fast-Onset Antidepressants Using Rodent Models. Mol Psychiatry (2017) 22:656–65. doi: 10.1038/mp.2017.36
92. Pałasz A, Piwowarczyk-Nowak A, Suszka-Świtek A, Bogus K, Filipczyk Ł, della VA, et al. Escitalopram as a Modulator of Proopiomelanocortin, Kisspeptin, Kiss1R and MCHR1 Gene Expressions in the Male Rat Brain. Mol Biol Rep (2020) 47:8273–8. doi: 10.1007/s11033-020-05806-8
93. Mahmoudi F, Gollo KH. Influences of Serotonin Hydrochloride on Adiponectin, Ghrelin and KiSS1 Genes Expression. Galen Med J (2020) 9:e1767. doi: 10.31661/gmj.v9i0.1767
94. Silvers JA, Wager TD, Weber J, Ochsner KN. The Neural Bases of Uninstructed Negative Emotion Modulation. Soc Cogn Affect Neurosci (2015) 10:10–8. doi: 10.1093/scan/nsu016
95. Velurajah R, Brunckhorst O, Waqar M, McMullen I, Ahmed K. Erectile Dysfunction in Patients With Anxiety Disorders: A Systematic Review. Int J Impot Res (2021) 34:177–86. doi: 10.1038/s41443-020-00405-4
96. Ogawa S, Nathan FM, Parhar IS. Habenular Kisspeptin Modulates Fear in the Zebrafish. Proc Natl Acad Sci (2014) 111:3841–6. doi: 10.1073/pnas.1314184111
97. Csabafi K, Jászberényi M, Bagosi Z, Lipták N, Telegdy G. Effects of Kisspeptin-13 on the Hypothalamic-Pituitary-Adrenal Axis, Thermoregulation, Anxiety and Locomotor Activity in Rats. Behav Brain Res (2013) 241:56–61. doi: 10.1016/j.bbr.2012.11.039
98. Delmas S, Porteous R, Bergin DH, Herbison AE. Altered Aspects of Anxiety-Related Behavior in Kisspeptin Receptor-Deleted Male Mice. Sci Rep (2018) 8:2794. doi: 10.1038/s41598-018-21042-4
99. Ibos KE, Bodnár É, Bagosi Z, Bozsó Z, Tóth G, Szabó G, et al. Kisspeptin-8 Induces Anxiety-Like Behavior and Hypolocomotion by Activating the HPA Axis and Increasing GABA Release in the Nucleus Accumbens in Rats. Biomedicines (2021) 9:1–22. doi: 10.3390/biomedicines9020112
100. Oishi S, Misu R, Tomita K, Setsuda S, Masuda R, Ohno H, et al. Activation of Neuropeptide FF Receptors by Kisspeptin Receptor Ligands. ACS Med Chem Lett (2011) 2:53–7. doi: 10.1021/ml1002053
101. Lin YT, Chen JC. Neuropeptide FF Modulates Neuroendocrine and Energy Homeostasis Through Hypothalamic Signaling. Chin J Physiol (2019) 62:47–52. doi: 10.4103/CJP.CJP_23_19
102. Rao YS, Mott NN, Pak TR. Effects of Kisspeptin on Parameters of the HPA Axis. Endocrine (2011) 39:220–8. doi: 10.1007/s12020-011-9439-4
103. Thomson EL, Patterson M, Murphy KG, Smith KL, Dhillo WS, Todd JF, et al. Central and Peripheral Administration of Kisspeptin-10 Stimulates the Hypothalamic-Pituitary-Gonadal Axis. J Neuroendocrinol (2004) 16:850–8. doi: 10.1111/j.1365-2826.2004.01240.x
104. Kongsted AG. Stress and Fear as Possible Mediators of Reproduction Problems in Group Housed Sows: A Review. Acta Agric Scand - Section A: Anim Sci (2004) 54:58–66. doi: 10.1080/09064700410032031
105. Nathan FM, Ogawa S, Parhar IS. Kisspeptin1 Modulates Odorant-Evoked Fear Response via Two Serotonin Receptor Subtypes (5-HT1A and 5-HT2) in Zebrafish. J Neurochem (2015) 133:870–8. doi: 10.1111/jnc.13105
106. Lillesaar C, Tannhäuser B, Stigloher C, Kremmer E, Bally-Cuif L. The Serotonergic Phenotype is Acquired by Converging Genetic Mechanisms Within the Zebrafish Central Nervous System. Dev Dyn (2007) 236:1072–84. doi: 10.1002/dvdy.21095
107. Insel TR. The Challenge of Translation in Social Neuroscience: A Review of Oxytocin, Vasopressin, and Affiliative Behavior. Neuron (2010) 65:768–79. doi: 10.1016/j.neuron.2010.03.005
108. Bromberg-Martin ES, Matsumoto M, Hikosaka O. Dopamine in Motivational Control: Rewarding, Aversive, and Alerting. Neuron (2010) 68:815–34. doi: 10.1016/j.neuron.2010.11.022
109. Johnson CS, Hong W, Micevych PE. Posterodorsal Medial Amygdala Regulation of Female Social Behavior: GABA Versus Glutamate Projections. J Neurosci (2021) 41:8790–800. doi: 10.1523/JNEUROSCI.1103-21.2021
110. Neal-Perry G, Lebesgue D, Lederman M, Shu J, Zeevalk GD, Etgen AM. The Excitatory Peptide Kisspeptin Restores the Luteinizing Hormone Surge and Modulates Amino Acid Neurotransmission in the Medial Preoptic Area of Middle-Aged Rats. Endocrinology (2009) 150:3699–708. doi: 10.1210/en.2008-1667
111. Defazio RA, Elias CF, Moenter SM. Gabaergic Transmission to Kisspeptin Neurons is Differentially Regulated by Time of Day and Estradiol in Female Mice. J Neurosci (2014) 34:16296–308. doi: 10.1523/JNEUROSCI.3057-14.2014
112. Li XF, Hu MH, Hanley BP, Lin YS, Poston L, Lightman SL, et al. The Posterodorsal Medial Amygdala Regulates the Timing of Puberty Onset in Female Rats. Endocrinology (2015) 156:3725–36. doi: 10.1210/en.2015-1366
113. Adekunbi DA, Li XF, Li S, Adegoke OA, Iranloye BO, Morakinyo AO, et al. Role of Amygdala Kisspeptin in Pubertal Timing in Female Rats. PLoS One (2017) 12:e0183596. doi: 10.1371/journal.pone.0183596
114. di Giorgio NP, Semaan SJ, Kim J, P v López, Bettler B, Libertun C, et al. Impaired GABAB Receptor Signaling Dramatically Up-Regulates Kiss1 Expression Selectively in Nonhypothalamic Brain Regions of Adult But Not Prepubertal Mice. Endocrinology (2014) 155:1033–44. doi: 10.1210/en.2013-1573
115. Comninos AN, Yang L, O’Callaghan J, Mills EG, Wall MB, Demetriou L, et al. Kisspeptin Modulates Gamma-Aminobutyric Acid Levels in the Human Brain. Psychoneuroendocrinology (2021) 129:105244. doi: 10.1016/j.psyneuen.2021.105244
116. Cleve M, Gussew A, Reichenbach JR. In Vivo Detection of Acute Pain-Induced Changes of GABA+ and Glx in the Human Brain by Using Functional 1h MEGA-PRESS MR Spectroscopy. Neuroimage (2015) 105:67–75. doi: 10.1016/j.neuroimage.2014.10.042
117. Bollmann S, Ghisleni C, Poil SS, Martin E, Ball J, Eich-Höchli D, et al. Developmental Changes in Gamma-Aminobutyric Acid Levels in Attention-Deficit/Hyperactivity Disorder. Trans Psychiatry (2015) 5:e589. doi: 10.1038/tp.2015.79
118. Mitchell KR, Mercer CH, Ploubidis GB, Jones KG, Datta J, Field N, et al. Sexual Function in Britain: Findings From the Third National Survey of Sexual Attitudes and Lifestyles (Natsal-3). Lancet (2013) 382:1817–29. doi: 10.1016/S0140-6736(13)62366-1
119. Berger MH, Messore M, Pastuszak AW, Ramasamy R. Association Between Infertility and Sexual Dysfunction in Men and Women. Sex Med Rev (2016) 4:353–65. doi: 10.1016/j.sxmr.2016.05.002
Keywords: kisspeptin, KISS1, behaviour, sex, mood, emotions
Citation: Mills EG, Yang L, Abbara A, Dhillo WS and Comninos AN (2022) Current Perspectives on Kisspeptins Role in Behaviour. Front. Endocrinol. 13:928143. doi: 10.3389/fendo.2022.928143
Received: 25 April 2022; Accepted: 11 May 2022;
Published: 09 June 2022.
Edited by:
Takayoshi Ubuka, Cancer Medical Service, JapanReviewed by:
Kevin T. O’Byrne, King’s College London, United KingdomSatoshi Ogawa, Monash University, Malaysia
Copyright © 2022 Mills, Yang, Abbara, Dhillo and Comninos. This is an open-access article distributed under the terms of the Creative Commons Attribution License (CC BY). The use, distribution or reproduction in other forums is permitted, provided the original author(s) and the copyright owner(s) are credited and that the original publication in this journal is cited, in accordance with accepted academic practice. No use, distribution or reproduction is permitted which does not comply with these terms.
*Correspondence: Waljit S. Dhillo, dy5kaGlsbG9AaW1wZXJpYWwuYWMudWs=; Alexander N. Comninos, YS5jb21uaW5vc0BpbXBlcmlhbC5hYy51aw==
†ORCID: Waljit S. Dhillo, https://orcid.org/0000-0001-5950-4316
Alexander N. Comninos, https://orcid.org/0000-0002-7104-2297
‡These authors share first authorship
§These authors share senior authorship