- 1Department of Cardiology, The First Affiliated Hospital of Nanjing Medical University, Jiangsu Province Hospital, Nanjing, China
- 2Department of Cardiology, Affiliated Suzhou Hospital of Nanjing Medical University, Suzhou Municipal Hospital, Suzhou, China
Background: Ethylene oxide (EO) has been shown to associate with increased cardiovascular risk. This study aimed to explore the relationship and its meditating factors between EO exposure and the major cardiovascular risk factor of obesity among the general adult population.
Methods: Cross-sectional data of 3,220 participants from National Health and Nutritional Examination Survey (NHANES) 2013–2016 were enrolled. Obesity was defined as body mass index (BMI) ≥30 kg/m2, and abdominal obesity was defined as waist circumference (WC) ≥102 cm in men and ≥88 cm in women. The association among hemoglobin adduct of EO (HbEO), inflammatory biomarkers, and obesity was evaluated using restricted cubic splines and the multivariable linear regression model. Mediation analysis was used to further assess their association.
Results: The increased quartiles of HbEO were inversely associated with BMI and WC [Q1 vs. Q4, BMI: β = −2.98 (−3.74, −2.22), WC: β = −6.50 (−8.60, −4.39); all p for trend < 0.05], and were inversely associated with obesity after full adjustment [obesity: OR = 0.43 (0.31, 0.58), abdominal obesity: OR = 0.42 (0.27, 0.65); all p for trend < 0.05]. The levels of alkaline phosphatase, white blood cells, lymphocytes, and neutrophils were also positively associated with BMI and WC (all p < 0.05). Mediation analysis showed that exposure of EO not only had a negative direct effect on BMI and WC, but also generated an inverse indirect effect.
Conclusions: Current findings showed an inverse association between HbEO and obesity, and suggested that systemic inflammation may not be their only mediator. Additional research is required to explore the underlying link of EO and system metabolism.
Introduction
As a metabolite of ethylene, ethylene oxide (EO) is a ubiquitous compound in the environment (1). EO is a widely used industrial chemical for manufacturing various daily products such as antifreeze, polyester, detergents, adhesives, textiles, solvents, and pesticides. Exposure to EO in the general population may be through application of sterilized medical equipment (2), fumigation of food and cosmetics (3), and inhalation of contaminated air, tobacco smoke, and/or vehicle exhaust (4, 5). Exposure to EO raises various health issues. EO is a highly reactive organic compound causing the alkylation of DNA, RNA, and proteins (1). Previous large prospective general population studies have shown that the exposure to EO is associated with an increased risk of cancer and has been classified as a Group 1 human carcinogen by the International Agency for Research on Cancer (6). In addition, studies have also suggested that chronic EO exposure may be correlated (7, 8) with the systemic inflammatory response.
The use of EO sterilization in personal protective equipment (PPE) plays an important role in the COVID-19 pandemic. Notably, the EO residue in regulatory compliance single-use sterile medical devices, products, and PPEs (for example, the residual EO on face masks <10 μg/g) should be overall safe. However, the wide application of PPE potentially increased the exposure to EO, which leads to substantial confusion about chronic EO exposures for the general population (9, 10). Furthermore, the chronic exposure to EO is also associated with various cardiovascular risk factors such as smokers, serum lipid levels, and diabetes (1, 11–14). In our previous study, EO is associated with dyslipidemia and that might be mediated by the systemic inflammation (15). While the above evidence supports that the environmental pollutants such as EO may have an adverse effect on the cardiovascular system (16), only limited studies have explored such associations and the potential inflammatory mechanism.
Studies have also shown a significant association between exposure to a variety of environmental chemicals and obesity in humans (17). Meanwhile, the worldwide epidemic of overweight and obesity continues to be a major challenge in chronic disease prevention. Obesity leads to significant adverse health effects due to its association with metabolic disturbances and is a major cardiovascular risk factor (18, 19). Obesity increases cardiovascular risk through lipid abnormalities. The development of insulin resistance, hepatic fatty acid metabolic disturbance, intravascular lipolysis, adipose tissue dysfunctionality, and chronic inflammation is also closely related to obesity, metabolic syndrome, and dyslipidemia (20–22).
However, the relationship between EO and obesity remained unclear. Herein, our study hypothesized that the serum level of EO adduct hemoglobin (HbEO) might be associated with obesity, which is mediated by inflammation. The objective of the current study is to demonstrate the association of HbEO levels with body mass parameters and obesity, and the mediating role of systemic inflammatory biomarkers in the general population.
Methods
Study participants
This cross-sectional epidemiologic study used publicly available data from the National Health and Nutrition Examination Survey (NHANES) conducted by the US National Center for Health Statistics (NCHS). Authors have not been involved in the collection and production of the database in use. The NHANES survey represents the noninstitutionalized civilian population in the US. All participants provided signed informed consent in accordance with the protocol approved by the ethics review board of NCHS. The detailed obtained ethics approval and written informed consent are available at https://www.cdc.gov/nchs/nhanes/irba98.htm. The survey design, methods, and data protocols are publicly available at https://www.cdc.gov/nchs/nhanes/about_nhanes.htm.
Among NHANES 2013–2016, including three survey cycles in the total sample, there were 3,220 participants enrolled in the analysis. Participants without blood EO, underage participants, those with missing data on weight and waist indexes, and those who are pregnant were excluded (Figure 1).
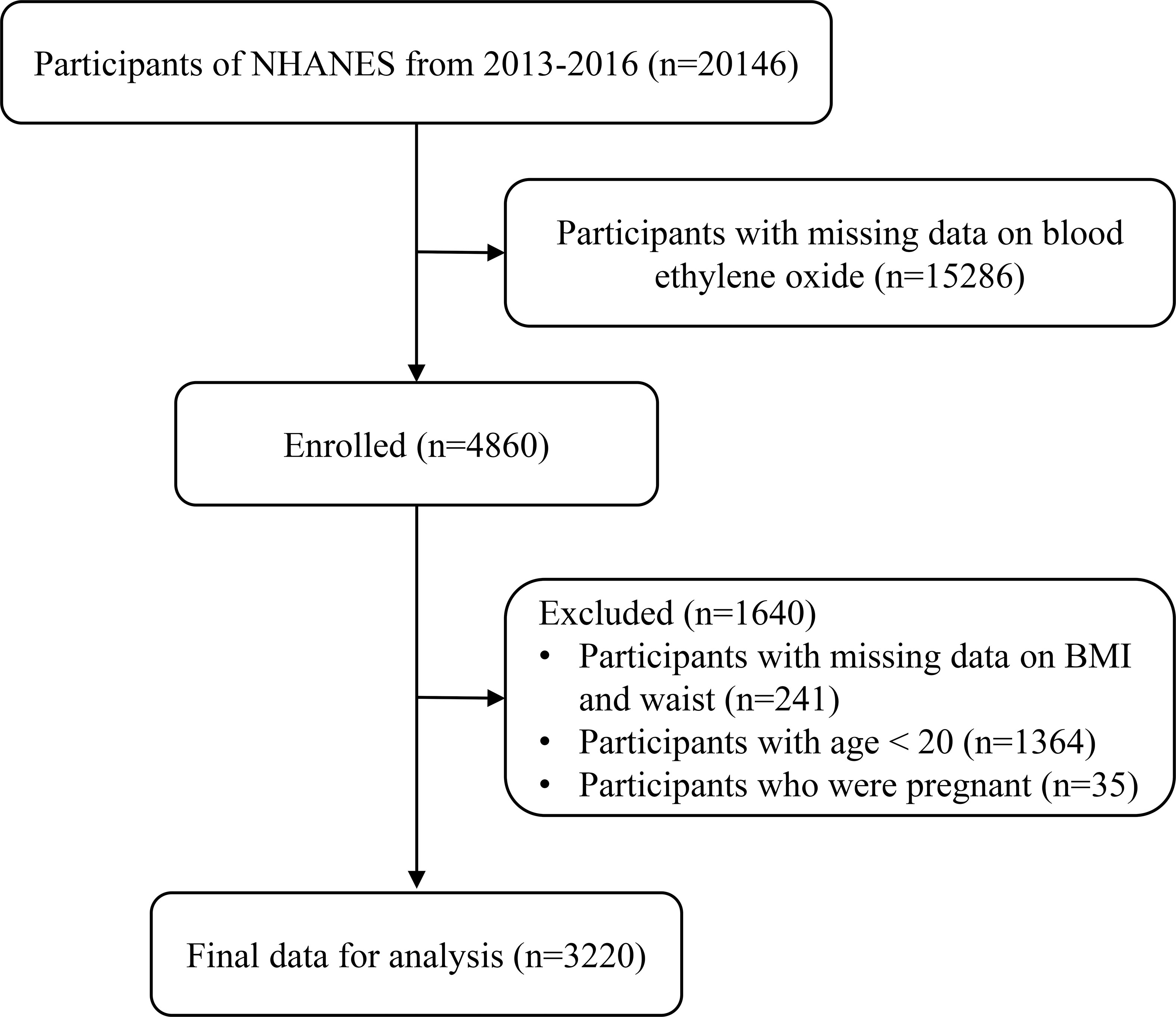
Figure 1 Study population flowchart. NHANES, National Health and Nutrition Examination Survey; BMI, body mass index.
Anthropometric measures
Weight, height, and waist circumference (WC) were measured at the Mobile Examination Centers (MECs) using the standard methodology by using the standardized procedure manual. Body mass index (BMI) is calculated by dividing body weight in kilograms by height in meters squared (kg/m2). Obesity was defined as BMI at or above 30 kg/m2. While WC adds critical information along with BMI, WC was measured with a tape measure at the uppermost lateral border of the hip crest. WC ≥102 cm in men and ≥88 cm in women were considered abdominal obesity (20).
Blood ethylene oxide levels
Hemoglobin adduct of ethylene oxide (HbEO) has been shown to be a highly sensitive method for determining exposure to EO (23).
Blood samples were obtained in the morning from participants who had fasted for at least 9 h in MECs. Washed-packed red blood cell specimens were processed and stored at −30°C until preset batches of samples were supplied to technicians for analysis on a regular basis. Human whole blood or erythrocyte levels were determined to assess HbEO. The reaction products with the N-terminal valine of the hemoglobin protein chains (N-[2-carbamoyl ethyl] valine and N-[2-hydroxycarbamoyl-ethyl] valine EO adducts) were measured using the modified Edman reaction by high-performance liquid chromatography coupled with tandem mass spectrometry (HPLC-MS/MS) with the corresponding commercial assay kit (Tech Diagnostics, Anaheim, CA). For analytes with analytical results less than the lower limit of detection (12.9 pmol/g Hb), the values were replaced with the lower limit of detection divided by the square root of two (LLOD/sqrt (2)). The method for HbEO measurement is provided at https://wwwn.cdc.gov/Nchs/Nhanes/2015-2016/ETHOX_I.htm.
Covariates
NHANES uses a complex sampling design and constructs sample weights to produce nationally representative data. Sampling weights are used to produce correct population estimates because each sample person does not have an equal probability of selection. Survey weights are constructed for each 2-year cycle, which considers non-response, over-sampling, post-stratification, and sampling error. Details for sampling weight are found at https://wwwn.cdc.gov/nchs/nhanes/tutorials/module3.aspx.
The demographic characteristics including age, sex, educational level, race, income status, smoking status, alcohol consumption, sedentary time, daily energy intake, and past medical history (hypertension and diabetes mellitus) were obtained during the family interview using a standardized questionnaire.
Educational levels were classified into three categories: below high school, high school, and above high school. Race and ethnicity were classified as follows: Mexican American, other Hispanic, non-Hispanic white, non-Hispanic black, and other race groups (including multiracial). The family poverty income ratio (PIR) was used to assess the economic status. Poverty is characterized as a family PIR of less than one. Alcohol users were identified as individuals who consumed at least 12 alcohol drinks in a single calendar year. A smoker was defined as someone who had smoked at least 100 cigarettes throughout the course of his or her lifetime. The number of minutes of sedentary behavior on a typical day was assessed by interviewing participants. Lipid profiles (total cholesterol [TC] and high-density lipoprotein cholesterol [HDL-C]) and inflammation markers (including alkaline phosphatase [ALP], white blood cell count [WBC], neutrophil count [NEU], and lymphocyte count [LYM]) in the corresponding cycles were obtained from the laboratory data.
Statistical analysis
Weighting was performed prior to the analysis to adjust for sample frame based on independent population. Examination sample weights, which account for the differential probabilities of selection, nonresponse, and noncoverage, were incorporated into the estimation process using the “survey” package in R studio.
To verify the normal distribution of continuous variables, a Kolmogorov–Smirnov statistical test was performed. Continuous variables are presented as the means [standard deviations (SDs)] with normal distribution or medians with interquartile ranges (IQRs, Q1–Q3) with non-normal distribution. Categorical variables are presented as numbers (%). HbEO levels were log2-transformed to normalize the distributions. To investigate the dose–response curves between HbEO levels and the anthropometric parameters, restricted cubic splines with knots were used at the 5th, 35th, 65th, and 95th percentiles of HbEO level distribution. HbEO was divided into quartiles (Q) with the lowest quartile serving as the reference group to further evaluate the relationships with inflammatory markers, and serum lipid profiles using multivariable linear regression models. Model 1 was unadjusted. Model 2 was adjusted for age, sex, education level, and race. Model 3 was the same as Model 2 with additional adjustments for poverty, smoker, alcohol user, energy intake levels, sedentary time, TC, HDL-C, diabetes, and hypertension.
To determine the extent to which the relationship between HbEO levels and anthropometric parameters was mediated by inflammation (ALP, WBC, NEY, and LYM), causal mediation analyses were applied to estimate the direct effect (DE, mediator to outcome), indirect effect (IE, exposure to mediator), and total effect (TE, exposure to outcome). The 1,000 bootstrapping was used for significance testing, and the analyses were adjusted for the same covariates in Model 3 (24, 25).
The statistical analyses were performed by SPSS (version 24.0; IBM) and R software (version 3.6.0; The R Foundation for Statistical Computing). Two-sided p < 0.05 was considered statistically significant.
Results
Baseline characteristics
Table 1 demonstrated the characteristics of the study population. The study included 2,721 participants, 49.2% of whom are male with a median age of 47 years. For the prevalence of the obese-related lifestyle and risk factors, there were 31.2% of participants with hypertension, 10.4% with diabetes, 14.9% in poverty, 43.9% with smoking habits, 77.2% were alcohol users, and 49.3% with sedentary time >6 h. The median BMI in the overall population was 28.0 (24.5, 32.7) kg/m2, and the median WC was 99.0 (88.2, 109.7) cm. A total of 1,262 (38.9%) individuals were considered in the obese category, and 1,863 (58.8%) were in the abdominal obesity category. The median HbEO level in the studied population was 39.7 (21.8, 55.7) pmol/g·Hb. Median TC was 188.0 (162.0, 214.0) mg/dl and median HDL-C was 51.0 (42.0, 64.0) mg/dl.
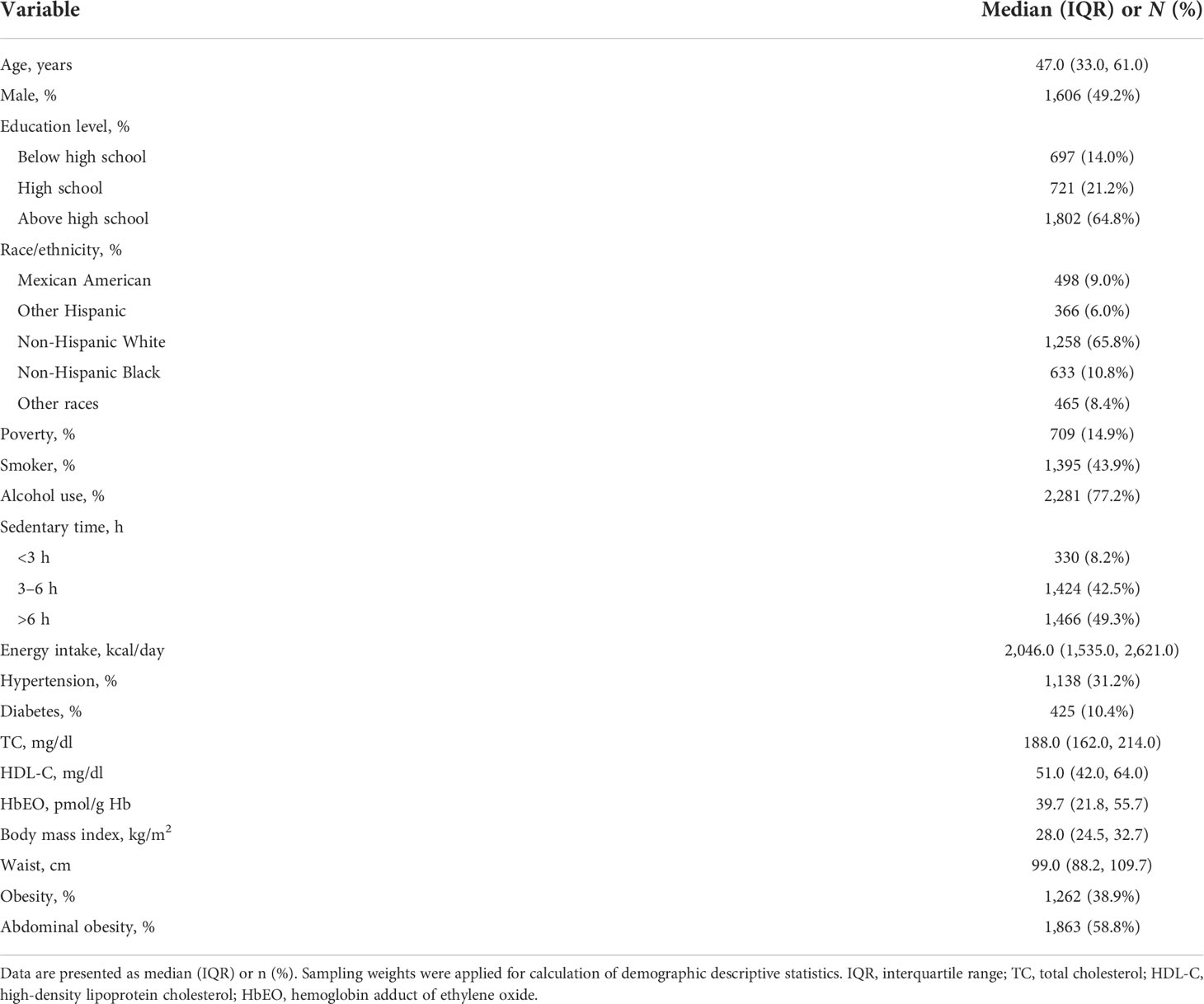
Table 1 Survey-weighted, sociodemographic, and health status characteristics of adult NHANES 2013–2016 participants with available blood ethylene oxide (n = 2,721).
Association between HbEO and anthropometric parameters
The relationships between the HbEO level and anthropometric parameters (BMI and WC) in both continuous and categorical analyses are presented in Table 2. The continuous analysis showed that log2-transformed HbEO had a significant inverse association with BMI and WC regardless of adjustment (p < 0.001).
Compared with the lowest quartile, the adjusted β (95% CI) in the highest quartile was −2.98 (−3.74, −2.22) for BMI and −6.50 (−8.60, −4.39) for WC after full adjustment (Model 3, both p < 0.001). Also regardless of the adjustment, when compared with the lowest quantiles of HbEO, it remained a significant trend in both BMI (p for trend <0.001) and WC (p for trend <0.05).
In addition, the cubic spline curve (including log2-transformed HbEO and four blood lipids as continuous variables) also yielded inverse trends similar to the above results (Figure 2A: BMI; Figure 2B: WC). HbEO exhibited a linear association with BMI and WC (p for nonlinearity >0.05).
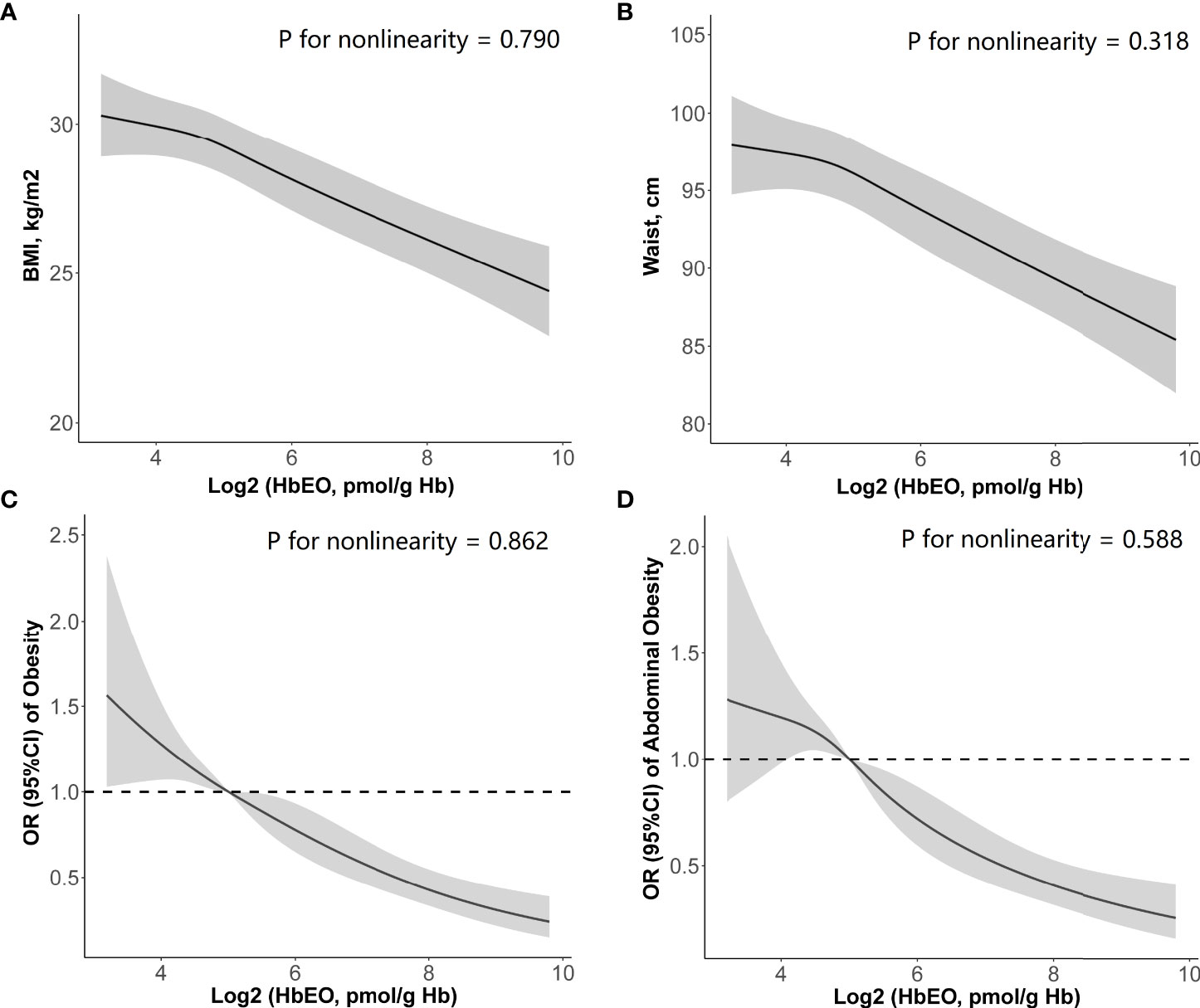
Figure 2 Restricted cubic spline (RCS) plots of the association of HbEO levels with (A) body mass index, (B) waist circumference, (C) prevalence of obesity, and (D) prevalence of abdominal obesity. Adjusted for age, sex, education level, race, poverty, smoker, alcohol user, energy intake levels, sedentary time, total cholesterol, high-density lipoprotein cholesterol, diabetes, and hypertension. HbEO, hemoglobin adduct of ethylene oxide; BMI, body mass index.
Association between HbEO and obesity
The associations between HbEO and the prevalence of obesity (both BMI-defined obesity and WC-defined abdominal obesity) are shown in Table 3. The continuous analysis showed that HbEO also had a significant inverse association with obesity regardless of adjustment (p < 0.01).
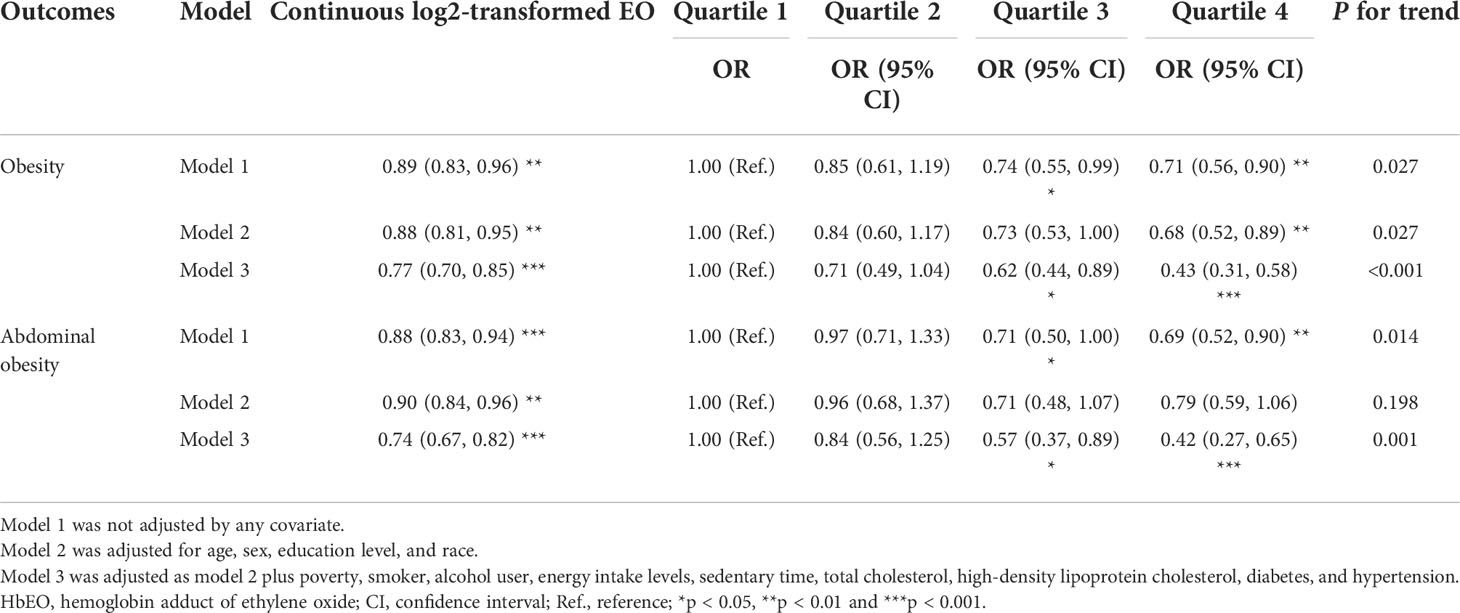
Table 3 Multiple logistic regression associations of HbEO with obesity and abdominal obesity in adults.
After adjustment of a series of covariates, HbEO was significantly and inversely associated with obesity (p for trend <0.0001). Compared with the lowest quartile, the multivariate odds ratios (ORs) and 95% confident intervals (95% CIs) across the increasing quartiles of HbEO declined as 0.71 (0.49, 1.04), 0.62 (0.44, 0.89), and 0.43 (0.31, 0.58) (Model 3: p for trend <0.001). Similar association between HbEO and abdominal obesity was also found across the increasing quartiles compared with the reference quartile in the fully adjusted model [Model 3: OR = 0.84 (0.56, 1.25), 0.57 (0.37, 0.89), and 0.42 (0.27, 0.65); p for trend = 0.001]. In the restricted cubic spline curve, HbEO also had a negative correlation and linear association with obesity (p for nonlinearity = 0.862, Figure 2C) and abdominal obesity (p for nonlinearity = 0.588, Figure 2D).
Mediation effect of systemic inflammation between HbEO and anthropometric parameters
There were significant correlations between the inflammatory markers (ALP, WBC, NEY, and LYM) and anthropometric parameters (BMI and WC) in multivariate linear regression models (all p < 0.01, Table 4). In addition, HbEO was significantly associated with inflammation markers (all p for trend < 0.05, Table S1).
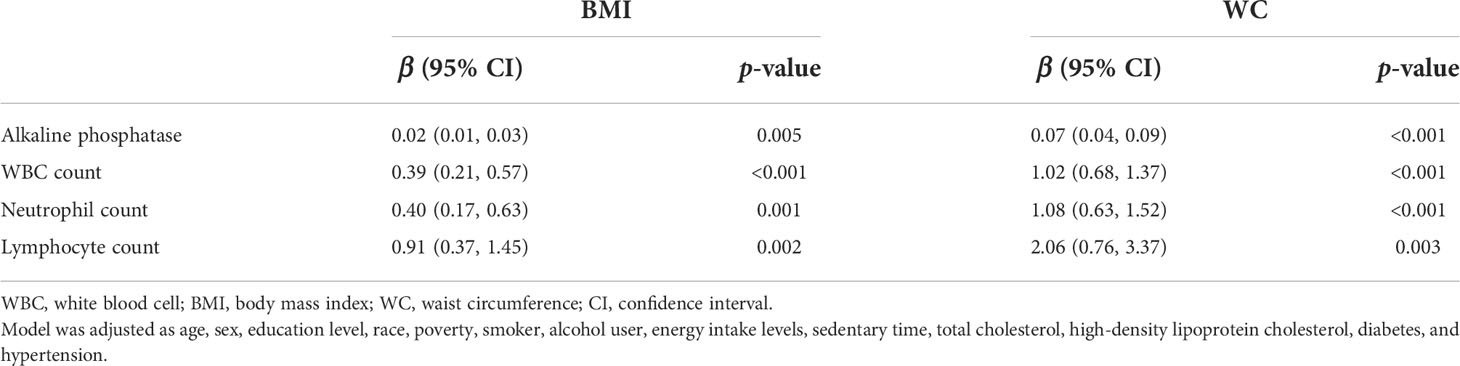
Table 4 Multiple linear regression associations of inflammatory markers with anthropometric parameters in adults.
Therefore, further mediation analysis to explore such a relationship showed that these inflammatory markers significantly mediated the associations between HbEO and BMI, and the proportions were 3.80%, 18.89%, 14.6%, and 8.86%, respectively (all p < 0.05). Similarly, inflammatory markers mediated the correlation between HbEO and WC at 5.70%, 21.40%, 16.80%, and 9.26% (all p < 0.001), respectively. Of note, the direct and indirect effects demonstrated opposite signs, which indicates that a suppression effect exists (Table 5).
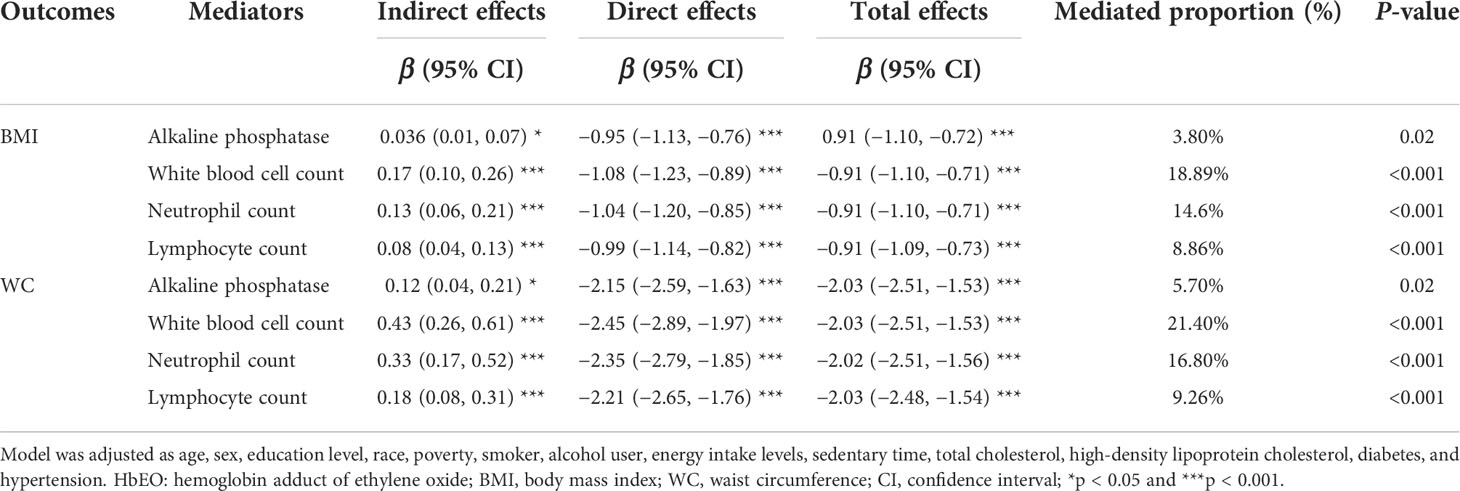
Table 5 The mediation effects of inflammatory markers on the association of log2-transformed HbEO with anthropometric parameters in adults.
Subgroup analysis
The results of the subgroup analyses to explore the interaction of these factors (age, sex, smoking, energy intake, sedentary time, hypertension, and diabetes) with EO-related obesity can be seen in Tables S2 and S3.
Notably, the inverse association of HbEO with obesity and abdominal obesity did not differ between smokers and nonsmokers (p for interaction = 0.770). On the other hand, a significant inverse association remained in hypertension and diabetes subgroups across the increasing quartiles of HbEO (p for trend < 0.05). The comorbidity of hypertension showed a significant difference in abdominal obesity, and the comorbidity of diabetes showed a significant difference in both obesity and abdominal obesity (p for interaction < 0.05).
Discussion
The present study demonstrated the association of HbEO with BMI and WC based on a US national-scale general population. We found that the level of HbEO was linearly and inversely associated with BMI and WC, which was further supported in the regression analysis for the risk of obesity. In addition, HbEO was positively associated with the inflammation markers. This relationship between HbEO and anthropometric parameters might be mediated by multiple mechanisms not expecting systemic inflammation.
Previous studies demonstrated that exposure to EO was shown to be related to inflammation (14, 26, 27). EO could cause various adverse health impacts regardless of the exposure route. Growing evidence has demonstrated that the exposure to EO was associated with the major risk factors and also the increased risk of cardiovascular diseases. Although the potential mechanism of EO-related CVD risk remained unknown, oxidative stress, insulin resistance, and inflammation might be triggered by the direct effect and intermediate product of EO (28). Also, previous studies showed that human exposure to these reactive electrophilic chemicals, such as acrylamide and EO, could contribute to human cancer or be associated with angina, heart attack, and total cardiovascular disease, and may contribute to CVD via inflammatory reactions and abnormal fatty acid metabolism (hypo-HDL-C and hypertriglyceride) (13, 14, 29). While both inflammation and dyslipidemia are important inducers of CVD (30–32), current results consisted of the previous studies that the increased HbEO was positively associated with various inflammatory parameters, suggesting that inflammation does contribute to this aspect.
On the other hand, obesity is a well-established risk factor for CVDs, which may also be associated with chronic systemic inflammation. Of note, WC is an indicator of visceral body fat associated with cardiometabolic disease and CVD, and a high WC may even unmask a higher CVD risk in individuals with normal weight (33, 34). Overweight and obesity recapitulate many features of the inflammatory processes (35). This obesity-related persistent inflammation could lead to endothelial dysfunction and atherosclerosis via the mediators released by adipose tissues (36). However, from the perspective of EO exposure and obesity, the current results firstly showed an inverse association between HbEO and risk of obesity (both overall and abdominal obesity) in adults, which were counterintuitive to a certain extent. To further explore these findings, further mediation analysis of their overlap mechanism—systemic inflammation between HbEO and anthropometric parameters—showed that suppression effects were presented in this general population, which suggested that the mechanisms of this phenomenon might extend beyond the magnitude of body weight alone.
While inverse trends in subgroups remained in these two populations, the differences in the associations between HbEO and the prevalence of abdominal obesity in hypertension and overall obesity in diabetes are consistent with these associations previously reported. Previous studies in patients with type 2 diabetes mellitus have demonstrated the phenomenon of the obesity paradox whereby higher BMI in the overweight population is associated with a lower risk of mortality (37), and patients with HTN are often associated with abdominal obesity due to the lipo-metabolism abnormalities (38, 39), which suggested that there might be a common pathway and metabolism disturbance to chronic exposure of EO, while EO exposure through smoking is the major resource in the general population and adult tobacco smokers tend to have lower BMIs and unhealthier diets relative to nonsmokers due to the increased insulin resistance, central fat accumulation, and aberrant lipolysis (1, 40, 41). Although the inverse trend remained in the smoking group, which is in line with our understanding, the current results did not differ between smokers and nonsmokers in the subgroup analysis. One explanation for the lack of a correlation in our study may be that tobacco consumption was self-reported and qualitative.
Previous studies reported that exposure to certain chemicals was associated with weight loss via leptin, adiponectin hormones, and the thermogenesis pathway, and may imply loss of body reserves and activation of catabolic pathways, increased catabolic cytokines leading to cardiomyocyte apoptosis and inflammation, with widespread harm (42–44). There were studies showing that the participation of EO in the pluronic modification of leptin could overcome leptin resistance at the level of the blood–brain barrier (45–47). These results indicated that chronic exposure to EO causes alterations in systemic metabolism as well as inflammation, lipolysis, and insulin resistance. In our previous study, HbEO is closely linked to serum lipid profiles (positively correlated with TC, TG, and LDL-C, and negatively correlated with HDL-C), suggesting possible dyslipidemia (15). The above mechanisms have a certain contribution to the inverse association of EO exposure derived from adduct data, which provides evidence to explore the possible mechanisms of EO-related increased CVD risk. More research is needed to determine whether the metabolic disruption and the weight changes that differ in different body components (such as gaining fat and losing muscle) are related to such CVD risk.
In summary, the exposure to EO in the general population was inversely associated with obesity and related anthropometric parameters (BMI and WC), while HbEO was positively associated with various inflammatory parameters and the mediation analysis showed a suppression effect. This implies that the EO-related CVD may not be simply explained as inflammation and dyslipidemia.
The current study has several limitations that should be considered. First, the main limitation is the cross-sectional design, and some data were self-reported by participants. Associations between exposure and parameters may change due to lifestyle factors (such as occupation), which should not be considered as direct causality and requires further validation in prospective settings. Second, as with any epidemiological analysis, our findings may be influenced by unmeasured confounding variables. Further analysis needs to consider both fat mass and dynamics of persistent organic pollutants. Data accounting for the exposure-controlled variable and related biomarkers of EO were insufficient. Lastly, multiple measurements of HbEO levels should be included in future studies to explore the cumulative effect.
Conclusion
The current study demonstrated that higher HbEO levels were associated with an inverse trend of obesity risk and BMIs in the general adult population. Systemic inflammation may not fully account for the observed relationship between EO exposure and obesity. Further prospective and mechanistic studies are needed to validate and explore the phenomenon in the future.
Data availability statement
The datasets presented in this study can be found in online repositories. The names of the repository/repositories and accession number(s) can be found in the article/Supplementary Material.
Ethics statement
The studies involving human participants were reviewed and approved by the ethics review board of NCHS research. The patients/participants provided their written informed consent to participate in this study.
Author contributions
IC: Conceptualization, Methodology, Writing—original draft, and Finalization. XZ: Software, Formal analysis, and Visualization. QZ: Investigation and Methodology. ML: Investigation and Methodology. SL: Data curation. ZZ: Data curation. WY: Supervision. YZ: Project administration. HZ: Supervision and Project administration. XL: Validation and Writing—reviewing and editing. All authors contributed to the article and approved the submitted version.
Acknowledgments
We appreciate the National Center for Health Statistics (NCHS) of the Centers for Disease Control (CDC) and Prevention and all participants who enrolled in the NHANES. The author thank Dr. Yanxiu Li for the administration supports.
Conflict of interest
The authors declare that the research was conducted in the absence of any commercial or financial relationships that could be construed as a potential conflict of interest.
Publisher’s note
All claims expressed in this article are solely those of the authors and do not necessarily represent those of their affiliated organizations, or those of the publisher, the editors and the reviewers. Any product that may be evaluated in this article, or claim that may be made by its manufacturer, is not guaranteed or endorsed by the publisher.
Supplementary material
The Supplementary Material for this article can be found online at: https://www.frontiersin.org/articles/10.3389/fendo.2022.926971/full#supplementary-material
References
1. Kirman CR, Li AA, Sheehan PJ, Bus JS, Lewis RC, Hays SM. Ethylene oxide review: Characterization of total exposure via endogenous and exogenous pathways and their implications to risk assessment and risk management. J Toxicol Environ Health B Crit Rev (2021) 24(1):1–29. doi: 10.1080/10937404.2020.1852988
2. Mendes GC, Brandão TR, Silva CL. Ethylene oxide sterilization of medical devices: A review. Am J Infect Control (2007) 35(9):574–81. doi: 10.1016/j.ajic.2006.10.014
3. Kolman A, Chovanec M, Osterman-Golkar S. Genotoxic effects of ethylene oxide, propylene oxide and epichlorohydrin in humans: Update review (1990-2001). Mutat Res (2002) 512(2-3):173–94. doi: 10.1016/s1383-5742(02)00067-4
4. Bono R, Vincenti M, Saglia U, Pignata C, Russo R, Gilli G. Tobacco smoke and formation of n-(2-hydroxyethyl)valine in human hemoglobin. Arch Environ Health (2002) 57(5):416–21. doi: 10.1080/00039890209601430
5. Szwiec E, Friedman L, Buchanan S. Levels of ethylene oxide biomarker in an exposed residential community. Int J Environ Res Public Health (2020) 17(22):8646. doi: 10.3390/ijerph17228646
6. Vincent MJ, Kozal JS, Thompson WJ, Maier A, Dotson GS, Best EA, et al. Ethylene oxide: Cancer evidence integration and dose-response implications. Dose Response (2019) 17(4):1559325819888317. doi: 10.1177/1559325819888317
7. Kirman CR, Sweeney LM, Teta MJ, Sielken RL, Valdez-Flores C, Albertini RJ, et al. Addressing nonlinearity in the exposure-response relationship for a genotoxic carcinogen: Cancer potency estimates for ethylene oxide. Risk Anal (2004) 24(5):1165–83. doi: 10.1111/j.0272-4332.2004.00517.x
8. Rusyn I, Asakura S, Li Y, Kosyk O, Koc H, Nakamura J, et al. Effects of ethylene oxide and ethylene inhalation on DNA adducts, apurinic/apyrimidinic sites and expression of base excision DNA repair genes in rat brain, spleen, and liver. DNA Repair (Amst) (2005) 4(10):1099–110. doi: 10.1016/j.dnarep.2005.05.009
9. Funatsu K, Kiminami H, Abe Y, Carpenter JF. Impact of ethylene oxide sterilization of polymer-based prefilled syringes on chemical degradation of a model therapeutic protein during storage. J Pharm Sci (2019) 108(1):770–4. doi: 10.1016/j.xphs.2018.09.029
10. Dias FN, Ishii M, Nogaroto SL, Piccini B, Penna TC. Sterilization of medical devices by ethylene oxide, determination of the dissipation of residues, and use of green fluorescent protein as an indicator of process control. J BioMed Mater Res B Appl Biomater (2009) 91(2):626–30. doi: 10.1002/jbm.b.31438
11. Ghosh M, Godderis L. Genotoxicity of ethylene oxide: A review of micronucleus assay results in human population. Mutat Res Rev Mutat Res (2016) 770(Pt A):84–91. doi: 10.1016/j.mrrev.2016.05.002
12. Shahab L, Goniewicz ML, Blount BC, Brown J, McNeill A, Alwis KU, et al. Nicotine, carcinogen, and toxin exposure in long-term e-cigarette and nicotine replacement therapy users: A cross-sectional study. Ann Intern Med (2017) 166(6):390–400. doi: 10.7326/M16-1107
13. Guo J, Wan Z, Cui G, Pan A, Liu G. Association of exposure to ethylene oxide with risk of diabetes mellitus: results from NHANES 2013-2016. Environ Sci Pollut Res Int (2021) 28(48):68551–9. doi: 10.1007/s11356-021-15444-7
14. Zeng G, Zhang Q, Wang X, Wu KH. Association between blood ethylene oxide levels and the risk of cardiovascular diseases in the general population. Environ Sci Pollut Res Int (2021) 28(45):64921–8. doi: 10.1007/s11356-021-15572-0
15. Zhu X, Kong X, Chen M, Shi S, Cheang I, Zhu Q, et al. Blood ethylene oxide, systemic inflammation, and serum lipid profiles: Results from NHANES 2013-2016. Chemosphere (2022) 299:134336. doi: 10.1016/j.chemosphere.2022.134336
16. Bhatnagar A. Cardiovascular pathophysiology of environmental pollutants. Am J Physiol Heart Circ Physiol (2004) 286(2):H479–85. doi: 10.1152/ajpheart.00817.2003
17. Darbre PD. Endocrine disruptors and obesity. Curr Obes Rep (2017) 6(1):18–27. doi: 10.1007/s13679-017-0240-4
18. Meldrum DR, Morris MA, Gambone JC. Obesity pandemic: Causes, consequences, and solutions-but do we have the will? Fertil Steril (2017) 107(4):833–9. doi: 10.1016/j.fertnstert.2017.02.104
19. Clark M, Hoenig M. Metabolic effects of obesity and its interaction with endocrine diseases. Vet Clin North Am Small Anim Pract (2016) 46(5):797–815. doi: 10.1016/j.cvsm.2016.04.004
20. Powell-Wiley TM, Poirier P, Burke LE, Després JP, Gordon-Larsen P, Lavie CJ, et al. American Heart association council on lifestyle and cardiometabolic health; council on cardiovascular and stroke nursing; council on clinical cardiology; council on epidemiology and prevention; and stroke council. obesity and cardiovascular disease: A scientific statement from the American heart association. Circulation (2021) 143(21):e984–e1010. doi: 10.1161/CIR.0000000000000973
21. Klop B, Elte JW, Cabezas MC. Dyslipidemia in obesity: Mechanisms and potential targets. Nutrients (2013) 5(4):1218–40. doi: 10.3390/nu5041218
22. Stolarczyk E. Adipose tissue inflammation in obesity: A metabolic or immune response? Curr Opin Pharmacol (2017) 37:35–40. doi: 10.1016/j.coph.2017.08.006
23. Kawai T, Autieri MV, Scalia R. Adipose tissue inflammation and metabolic dysfunction in obesity. Am J Physiol Cell Physiol (2021) 320(3):C375–91. doi: 10.1152/ajpcell.00379.2020
24. Yang M, Frame T, Tse C, Vesper HW. High-throughput, simultaneous quantitation of hemoglobin adducts of acrylamide, glycidamide, and ethylene oxide using UHPLC-MS/MS. J Chromatogr B Analyt Technol BioMed Life Sci (2018) 1086:197–205. doi: 10.1016/j.jchromb.2018.03.048
25. VanderWeele TJ. Mediation analysis: A practitioner's guide. Annu Rev Public Health (2016) 37:17–32. doi: 10.1146/annurev-publhealth-032315-021402
26. MacKinnon DP, Krull JL, Lockwood CM. Equivalence of the mediation, confounding and suppression effect. Prev Sci (2000) 1(4):173–81. doi: 10.1023/a:1026595011371
27. Lynch DW, Lewis TR, Moorman WJ, Burg JR, Groth DH, Khan A, et al. Carcinogenic and toxicologic effects of inhaled ethylene oxide and propylene oxide in F344 rats. Toxicol Appl Pharmacol (1984) 76(1):69–84. doi: 10.1016/0041-008x(84)90030-9
28. Ulrich CE, Geil RG, Tyler TR, Kennedy GL Jr, Birnbaum HA. Two-week aerosol inhalation study in rats of ethylene oxide/propylene oxide copolymers. Drug Chem Toxicol (1992) 15(1):15–31. doi: 10.3109/01480549209035170
29. Somade OT, Ajayi BO, Adeyi OE, Adeshina AA, Adekoya MO, Abdulhameed RO. Oxidative stress-mediated induction of pulmonary oncogenes, inflammatory, and apoptotic markers following time-course exposure to ethylene glycol monomethyl ether in rats. Metabol Open (2020) 9:100075. doi: 10.1016/j.metop.2020.100075
30. Mori K, Inoue N, Fujishiro K, Kikuchi M, Chiba S. Biochemical changes in rat erythrocytes caused by ethylene oxide exposure. Fundam Appl Toxicol (1990) 15(3):441–7. doi: 10.1016/0272-0590(90)90030-n
31. Wellen KE, Hotamisligil GS. Inflammation, stress, and diabetes. J Clin Invest (2005) 115(5):1111–9. doi: 10.1172/JCI25102
32. Genkel VV, Shaposhnik II. Conceptualization of heterogeneity of chronic diseases and atherosclerosis as a pathway to precision medicine: Endophenotype, endotype, and residual cardiovascular risk. Int J Chronic Dis (2020) 2020:5950813. doi: 10.1155/2020/5950813
33. Greenfield DM, Snowden JA. Cardiovascular diseases and metabolic syndrome. In: Carreras E, Dufour C, Mohty M, Kröger N, editors. The EBMT handbook: Hematopoietic stem cell transplantation and cellular therapies, 7th ed. Cham (CH: Springer (2019).
34. Piché ME, Poirier P, Lemieux I, Després JP. Overview of epidemiology and contribution of obesity and body fat distribution to cardiovascular disease: An update. Prog Cardiovasc Dis (2018) 61(2):103–13. doi: 10.1016/j.pcad.2018.06.004
35. Sahakyan KR, Somers VK, Rodriguez-Escudero JP, Hodge DO, Carter RE, Sochor O, et al. Normal-weight central obesity: Implications for total and cardiovascular mortality. Ann Intern Med (2015) 163(11):827–35. doi: 10.7326/M14-2525
36. Rocha VZ, Libby P. Obesity, inflammation, and atherosclerosis. Nat Rev Cardiol (2009) 6(6):399–409. doi: 10.1038/nrcardio.2009.55
37. Gravina G, Ferrari F, Nebbiai G. The obesity paradox and diabetes. Eat Weight Disord (2021) 26(4):1057–68. doi: 10.1007/s40519-020-01015-1
38. Sangrós FJ, Torrecilla J, Giráldez-García C, Carrillo L, Mancera J, Mur T, et al. Association of general and abdominal obesity with hypertension, dyslipidemia and prediabetes in the PREDAPS study. Rev Esp Cardiol (Engl Ed) (2018) 71(3):170–7. doi: 10.1016/j.rec.2017.04.035
39. Chen SH, Chen SC, Lai YP, Chen PH, Yeh KY. Abdominal obesity and hypertension are correlated with health-related quality of life in Taiwanese adults with metabolic syndrome. BMJ Open Diabetes Res Care (2020) 8(1):e000947. doi: 10.1136/bmjdrc-2019-000947
40. Handali S, Rezaei M. Arsenic and weight loss: At a crossroad between lipogenesis and lipolysis. J Trace Elem Med Biol (2021) 68:126836. doi: 10.1016/j.jtemb.2021.126836
41. Yu XY, Song P, Zou MH. Obesity paradox and smoking gun: A mystery of statistical confounding? Circ Res (2018) 122(12):1642–4. doi: 10.1161/CIRCRESAHA.118.312897
42. Kim MS, Kim WJ, Khera AV, Kim JY, Yon DK, Lee SW, et al. Association between adiposity and cardiovascular outcomes: an umbrella review and meta-analysis of observational and mendelian randomization studies. Eur Heart J (2021) 42(34):3388–403. doi: 10.1093/eurheartj/ehab454
43. Grundlingh J, Dargan PI, El-Zanfaly M, Wood DM. 2,4-dinitrophenol (DNP): a weight loss agent with significant acute toxicity and risk of death. J Med Toxicol (2011) 7(3):205–12. doi: 10.1007/s13181-011-0162-6
44. Ferrer B, Prince LM, Tinkov AA, Santamaria A, Farina M, Rocha JB, et al. Chronic exposure to methylmercury enhances the anorexigenic effects of leptin in C57BL/6J male mice. Food Chem Toxicol (2021) 147:111924. doi: 10.1016/j.fct.2020.111924
45. Pulvers K, Emami AS, Nollen NL, Romero DR, Strong DR, Benowitz NL, et al. Tobacco consumption and toxicant exposure of cigarette smokers using electronic cigarettes. Nicotine Tob Res (2018) 20(2):206–14. doi: 10.1093/ntr/ntw333
46. Price TO, Farr SA, Yi X, Vinogradov S, Batrakova E, Banks WA, et al. Transport across the blood-brain barrier of pluronic leptin. J Pharmacol Exp Ther (2010) 333(1):253–63. doi: 10.1124/jpet.109.158147
Keywords: ethylene oxide (EO), obesity, abdominal obesity, inflammation, NHANES, epidemiology
Citation: Cheang I, Zhu X, Zhu Q, Li M, Liao S, Zuo Z, Yao W, Zhou Y, Zhang H and Li X (2022) Inverse association between blood ethylene oxide levels and obesity in the general population: NHANES 2013–2016. Front. Endocrinol. 13:926971. doi: 10.3389/fendo.2022.926971
Received: 23 April 2022; Accepted: 18 August 2022;
Published: 12 September 2022.
Edited by:
Yuanyuan Wang, Monash University, AustraliaReviewed by:
Shengxu Li, Children’s Hospitals and Clinics of Minnesota, United StatesR. Deepa, Public Health Foundation of India, India
Copyright © 2022 Cheang, Zhu, Zhu, Li, Liao, Zuo, Yao, Zhou, Zhang and Li. This is an open-access article distributed under the terms of the Creative Commons Attribution License (CC BY). The use, distribution or reproduction in other forums is permitted, provided the original author(s) and the copyright owner(s) are credited and that the original publication in this journal is cited, in accordance with accepted academic practice. No use, distribution or reproduction is permitted which does not comply with these terms.
*Correspondence: Xinli Li, xinli3267@njmu.edu.cn
†These authors have contributed equally to this work and share first authorship