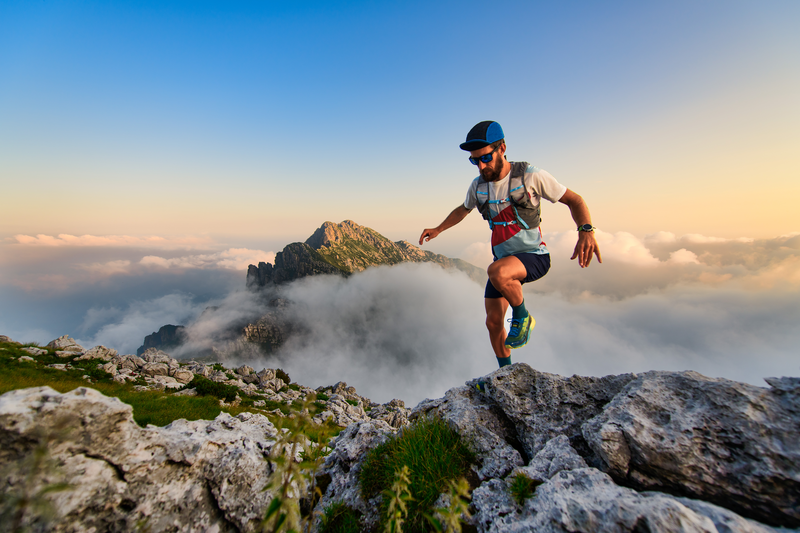
94% of researchers rate our articles as excellent or good
Learn more about the work of our research integrity team to safeguard the quality of each article we publish.
Find out more
ORIGINAL RESEARCH article
Front. Endocrinol. , 24 June 2022
Sec. Bone Research
Volume 13 - 2022 | https://doi.org/10.3389/fendo.2022.925848
This article is part of the Research Topic The Potential Effects and Mechanisms of Chinese Traditional Medicine on Bone Homeostasis and Remodeling, Volume II View all 7 articles
Postmenopausal Osteoporosis (PMOP) is the most prevalent primary osteoporosis, attributable to an imbalance in osteoblast and osteoclast activity. Modified You-Gui-Yin (MYGY), a traditional Chinese herbal formula, is able to effectively treat PMOP, while the critical components and pharmacological mechanisms of MYGY are still unclear. In this study, we aimed to investigate the therapeutic effects and underlying mechanisms of N-butanol extract of MYGY (MYGY-Nb) in ovariectomized (OVX)-induced osteoporosis mice. Histological staining and micro-computed tomography (μCT) analysis showed that MYGY-Nb was more effective in the suppression of OVX-induced bone loss than MYGY original formula. Subsequently, liquid chromatography and mass spectrometry analysis identified 16 critical compounds of MYGY-Nb and some of them are reported to affect osteoclast functions. Furthermore, in vivo and in vitro experiments demonstrated that MYGY-Nb significantly attenuated osteoclastogenesis by down-regulating RANKL-mediated NF-κB signaling. In conclusion, our study indicated that MYGY-Nb suppresses NF-κB signaling and osteoclast formation to mitigate bone loss in PMOP, implying that MYGY-Nb and its compounds are potential candidates for development of anti-PMOP drugs.
Osteoporosis is a skeletal disease characterized by reduced bone mass density and strength, increased skeletal fragility and fractures (1). As the population ages, the worldwide patients caused by osteoporosis might well reach 221 million by 2050 (2–4), and the medical and socioeconomic burden of osteoporosis, particularly postmenopausal osteoporosis (PMOP), will increase further (5). PMOP, mainly contributed by menopause – the cessation of ovarian function, is the most common primary osteoporosis. However, due to the cost and side effects of available drugs, most cases of PMOP have not been effectively treated so far (6). Therefore, the development of novel effective therapeutic agents with minimal side effects for the PMOP treatment is urgently needed (7).
Traditional Chinese medicine (TCM) has been used for thousands of years to treat various chronic diseases, especially osteoporosis (8–11). The Modified You-Gui-Yin (MYGY) is a well-known TCM formula, which is derived from You-Gui-Yin classic prescription recorded in Jing Yue Quan Shu written by Jingyue Zhang in the Ming Dynasty. The MYGY consists of ten herbs: Persicae semen, Cinnamomi cortex, Monkshood root, Eucommiae cortex, Lycii fructus, Dioscoreae rhizome, Carthami flos, Corni fructus, Glycyrrhizae radix and Preparata rehmanniae radix (Table 1), and has been widely applied to treat various chronic diseases with fewer side effects (12, 13). Our recent study has shown that MYGY-based therapies achieve significant clinical outcomes in PMOP management (14). In addition, several herbs of MYGY have been reported to ameliorate osteoporosis by inhibiting osteoclast activity, such as Glycyrrhizae radix, Dioscoreae rhizoma, Corni fructus (12, 15, 16). However, the underlying mechanisms and functional compounds of MYGY in the treatment of PMOP have not been fully elucidated, which limits the further development and application of MYGY.
In the process of bone reconstruction, the cumulative imbalance in the activity of osteoblasts and osteoclasts can lead to a decrease in bone mass, which is fundamental to the pathogenesis of PMOP (17). Of note, this imbalance is mainly due to the osteoclast reabsorption of bone in excess of osteoblasts production of mineralized extracellular matrix (18). Osteoclasts are produced by the differentiation of precursors from a monocyte/macrophage lineage that is derived from bone marrow hematopoietic stem cells (19). Thus, the prevention of these stem cells from differentiation of osteoclast is one of the most important strategies in PMOP treatment.
The survival, proliferation, differentiation and activation of osteoclasts require the presence of RANKL (receptor activator of nuclear factor kappa B ligand) and M-CSF (macrophage colony-stimulating factor) (20). In the process of osteoclastogenesis, RANKL interacts with RANK (receptor of RANKL) to induce the activation of NF-κB through the recruitment of adapter molecules such as TRAF6 (TNF receptor-associated factor 6), the degradation of IκB (the suppressor of NF-κB), and the phosphorylation of p65 (the subunit of NF-κB). These signaling cascades subsequently activate NFATc1 (nuclear factor of activated T cells, cytoplasmic 1), known as the primary transcription factor for osteoclastogenesis (21, 22). During the postmenopausal period, the expression of various inflammation inhibitors was found to decrease, but RANKL increased significantly, suggesting a critical role for RANKL in PMOP (23). And NF-κB signaling pathway participates in regulating the proliferation and function of osteoclasts (24–27). Thus, the suppression of osteoclast formation by targeting the RANKL-mediated NF-κB signaling pathway is probably a potential treatment strategy for osteoclastogenesis-related disorders, especially for PMOP.
In this study, we investigated the impact of N-butanol extract of MYGY (MYGY-Nb) on OVX mice and primary bone marrow-derived monocytes (BMMs). We found that MYGY-Nb possessed a stronger anti-osteoporosis effect with attenuating bone loss and osteoclast formation. Moreover, the mechanism by which MYGY-Nb suppressed osteoclastogenesis might be that MYGY-Nb inhibited RANKL-mediated NF-κB signaling. Taken together, our findings implicated that MYGY-Nb might be an effective medicine for treating PMOP.
All herbs of MYGY (Table 1) were provided by the First Affiliated Hospital of Zhejiang Chinese Medical University (Hangzhou, China). The N-butanol extract of MYGY was prepared as follows: Rou gui and Tao ren were mixed in a ratio of 1:2, soaked in 60% ethanol for 1 h, and then extracted 3 times by reflux method for 1.5 hours each. Other eight herbs including Fu zi, Du zhong, Gou qi, Shan yao, Hong hua, Shan zhu yu, Gan cao and Shu di huang were mixed in a1:3:2:2:2:1:2:1 ratio, steeped in 12 volumes of distilled water for 1 hour, and then extracted 3 times for 1.5 h each. Afterwards, the above two extracts were mixed and concentrated into MYGY extractum (3.4 g crude drug/mL). The above procedure is the preparation method of MYGY concentrate. After that, to extract its optimal anti-osteoporosis fraction, 95 ml MYGY extractum was dissolved into 300 ml distilled water, then, 300 ml N-butanol was mixed in equal proportion. After overnight emulsification and precipitation, the supernatant was collected and again extracted by the above method 3 times. Then, the supernatant was concentrated under reduced pressure until the N-butanol was completely evaporated to obtain the crude extract powder, namely MYGY-Nb. The MYGY-Nb powder was dissolved in 300ml sterile distilled water and stored at -20°C.
The MYGY-Nb powder was dissolved in methanol to 1 μg/mL for liquid chromatography analysis. Then a Waters Acquity UPLC BEH C18 column (100 mm×2.1 mm, 1.7 μm) was used to separate the compounds in MYGY-Nb with the mobile phase of acetonitrile (A)-0. 1% formic acid water (B), and the elution was gradient elution (0~7 min, 95% B; 7~15 min, 85% B; 15~18 min, 65% B; 18~23 min, 40% B; 23~27 min, 10% B; 27~29 min, 95% B) with volume flow rate of 0.3 mL/min, column temperature of 40°C and injection volume of 5 μL.
The MSE continuum mode of the SYNAPT G2-Si mass spectrometer was used to detect constituents of MYGY-Nb in the scan range of m/z 50~1500. In the ESI-/ESI+ modes, the capillary and cone well voltages were 3.0 kV and 40 V, respectively, and the argon flow rate was 0.15 mL/min. The cone well gas flow rate was 50 L/h at 120°C, and the desolvation flow rate was 800 L/h at 400°C. The 100 ng/mL solution of leucine-enkephalin (ESI-: m/z 555.261 5, ESI+: m/z 556.2771) was used as a calibration standard (Lock Spray TM) with a volume flow rate of 15 μL/min, and the instrument was calibrated with sodium formate for accurate mass determination. The UNIFI V1.8 software was used for data analysis.
10-week-old female C57BL/6J mice, supplied by Zhejiang Chinese Medical University Animal Center (Hangzhou, China), were maintained under standard feeding conditions with fresh water and food freely available. All mouse experiments were conducted in the pathogen-free laboratory of the Animal Center of Zhejiang Chinese Medical University (Hangzhou, China). Animals were randomly assigned into four groups (n = 6 in each group): (1) sham group, subjected to sham operation, (2) ovariectomy group (OVX), subjected to bilateral ovariectomy, (3) OVX+MYGY-Nb group, subjected to bilateral ovariectomy and then orally administrated MYGY-Nb extraction (20 mL/kg/day) for 12 weeks. (4) OVX+MYGY group, subjected to bilateral ovariectomy and then orally administrated MYGY extraction (20 mL/kg/day) for 12 weeks. The mice in other groups were addressed with the same dose of physiologic saline.
After the last administration, mice were sacrificed by pentobarbital sodium overdose, and then their femurs were isolated for μCT scanning (Skyscan1176, Belgium) at a 10 µm resolution. The three-dimensional (3D) structure reconstruction of the distal femurs was performed using the NRecon software. The bone mineral density (BMD), trabecular separation (Tb.Sp, mm), trabecular number (Tb.N, 1/mm), trabecular thickness (Tb.Th, mm) and bone volume fraction (BV/TV, %) were analyzed as described previously (28).
After μCT analysis, it was processed into 3-μm-thick paraffin sections of the femur as previously described (28). Subsequently, the sections were stained by Alcian blue hematoxylin/Orange G(ABH) for morphological analysis. The trabecular area (%) and lipid droplet area (%) of the region of the distal femur were calculated by OsteoMetrics software (Decatur, GA, USA). In IHC assays. the sections were incubated with 0.3% Triton X-100 for 10 min at room temperature and then treated with endogenous peroxidase blocker (ZSGB-BIO, PV-6001, Beijing, China) for 20 min. Subsequently, sections were incubated with primary antibodies of alkaline phosphatase (ALP, Arigo, ARG57422, 1:200), IKB alpha (IKBα, HuaBio, ET1603-6, 1:200), phosphorylated-p65(p-p65, Arigo, ARG51518,1:300), Nuclear factor of activated T-cells, cytoplasmic 1 (NFATc1, Novusbio, NB300-620, 1:300), and Receptor activator nuclear factor-κB (NF-κB) ligand (RANKL, Abcam, Ab45039, 1:500) overnight at 4°C, respectively. The next day, these sections were treated with a homologous secondary antibody for 20 min. The diaminobenzidine (DAB) solution (ZSGB-BIO, ZLI-9018, Beijing, China) was used to detect positive staining followed by hematoxylin re-staining. The quantification of positive staining was analyzed by using the software of Image-Pro Plus (Media Cybernetics, Silver Spring, USA).
Briefly, BMMs were separated from the femur and tibia of C57BL/6 J mice as previously described (29), and cultured in α minimum essential medium (α-MEM, Gibco, New York, USA) containing 10% fetal bovine serum (FBS, HyClone, Beijing, China), 1% streptomycin/penicillin (HyClone, Beijing, China) and 30 ng/mL M-CSF (R&D Systems, Minneapolis, MN, USA) in a humidified atmosphere at 37°C containing.
Cell Counting Kit-8 (CCK-8, Bioss, Beijing, China) assay was used to examine the viability of cells. The BMMs were seeded into 96-well plates at a density of 1 × 104 cells/well. After 24 h, BMMs were treated with 0, 10, and 100 μg/mL MYGY-Nb for 24, 48 or 72 h. Then the CCK8 solution was added to the culture medium and incubated at 37°C for 2 h. The absorbance was measured by BioTek Synergy HT spectrophotometer at absorbance was measured at 450 nm.
To examine osteoclast formation in vitro, BMMs were cultured for 6 days in 24-well plates (1 × 105 cells/well) with α-MEM containing 10% FBS, 1% streptomycin/penicillin, 30 ng/mL M-CSF, 50 ng/mL RANKL, and 0 or 10 μg/mL MYGY-Nb. And then, the cells were stained using the TRAP staining kit (Sigma-Aldrich, St. Louis, MO, USA). Osteoclasts (cells with more than three nuclei) were quantified by using Image J software (NIH, Bethesda, Maryland, USA).
The BMMs were cultured with 30 ng/mL M-CSF, 50 ng/mL RANKL and MYGY-Nb (0 or 10 μg/mL) for 4 days, and then mixed with RIPA buffer to extract total protein, the concentration of which was determined by bicinchoninic acid (BCA). Proteins were separated on 10% SDS-PAGE gels (20 μg/lane) and then transferred to PVDF membranes. The membranes were blocked with 5% skim milk for 1 h, followed by incubating with primary antibodies (IKBα, 1:1000 dilution, Cell Signaling Technology), (p65, 1:1000 dilution, Cell Signaling Technology), (p-p65, 1:1000 dilution, Cell Signaling Technology), (NFATc1, 1:1000 dilution, Novus Biologicals) and (β-actin, 1:10,000 dilution, Sigma-Aldrich) overnight at 4°C. After incubation with the corresponding secondary antibodies, proteins on the membrane were detected by the ultra ECL reagent kit (4A Biotech, Beijing, China) on an Image Quant LAS 4000 (EG, United States). The grey value was calculated by the software of Image J.
All data were analyzed using GraphPad Prism and presented in mean ± S.D. One-way ANOVA and Student’s t-test were performed to analyze the difference between groups. A value of P<0.05 was considered statistically significant.
To determine the effect of MYGY-Nb on PMOP, C57BL/6J mice were subjected to OVX surgery and then treated with MYGY original formula and MYGY-Nb (20 mL/kg/day) respectively for 12 weeks without any adverse effects. The μCT results showed a significant reduction in bone mass and changes of bone microarchitecture in OVX mice compared to controls (Figure 1A). After administration with MYGY original formula and MYGY-Nb, these OVX-induced abnormal symptoms were effectively alleviated, as illustrated by the increases in BMD, Tb.N, Tb.Th and BV/TV, as well as the decline in Tb.Sp (Figures 1B–F). Of note, MYGY-Nb was more effective in inhibiting bone loss than MYGY original formula (Figures 1A–F).
Figure 1 Changes of bone structure in OVX mice, MYGY original formula and MYGY-Nb administration mice. (A) Representative μCT images. Quantification of microstructural parameters including BMD (B), BV/TV (C), Tb. N (D), Tb. Th (E) and Tb. Sp (F). Scale bars: 50 μm. Data were presented as means ± S.D. *P < 0.05; **P < 0.01; ns: no significant difference; #P < 0.05. n = 6 in each group.
Additionally, ABH staining revealed that compared with sham mice, OVX mice displayed sparse trabeculae and massive adiposity accumulation (Figures 2A–C). As expected, in both MYGY original formula and MYGY-Nb administration groups, these OVX-induced effects were greatly inhibited, and MYGY-Nb showed a significantly better performance than MYGY original formula (Figures 2A–C).
Figure 2 MYGY-Nb and MYGY original formula protect against bone loss in OVX mice. (A) Alcian Blue Hematoxylin/Orange G staining of distal femur. Scale bars: 100 μm. (B) The area of trabecular bone (%). (C) The area of lipid droplets (%). Data were presented as means ± S.D. *P < 0.05; **P < 0.01; #P < 0.05. n = 6 in each group.
Therefore, we sought to identify the compounds of MYGY-Nb based on UHPLC-Q-TOF/MS analysis. Figure 3 showed the total ion chromatograms of MYGY-Nb in negative and positive ion modes. By comparison with the reference standard, retention time and published literatures, 16 main chemical constituents in MYGY-Nb were identified preliminarily (Table 2), among which Kynnipin glycosides, Rutin (Rutinoside)_1, Glycyrrhizin and Glycyrrhizic acid have shown the inhibiting functions in osteoclast formation (30–33).
Studies have shown that the main cause of PMOP is the disorder of bone metabolism contributed by an imbalance between osteoblastic bone formation and osteoclastic bone resorption (34). To determine whether MYGY-Nb regulates the formation of osteoblasts and osteoclasts, we performed TRAP histochemical staining and immunohistochemical staining of ALP. The histological results displayed that MYGY-Nb significantly suppressed OVX-induced increase in TRAP activities and decrease in ALP activities (Figures 4A, B), implying that MYGY-Nb prevents OVX-induced bone loss by regulating the formation of osteoclast and osteoblast. Surprisingly, these results are consistent with the effects of some compounds identified by UHPLC-Q-TOF/MS analysis.
Figure 4 MYGY-Nb treatment restores ALP and TRAP activity in OVX mice. (A) ALP immunohistochemical staining and quantification. (B) TRAP histochemical staining and quantification. Scale bars: 100 μm. Data were presented as means ± S.D. **P < 0.01; ***P < 0.001. n = 6 in each group.
Given that RANKL-induced NF-κB activation is required for osteoclast differentiation and function, we further investigated whether MYGY-Nb inhibits osteoclast formation through the RANKL-mediated NF-κB pathway in OVX-induced mice. By using IHC assay, we found that OVX mice showed a noticeable increase in expression of RANKL, p-p65 and NFATc1, and decrease in expression of IKBα. Nevertheless, after the administration of MYGY-Nb for 12 weeks, the changes of expression of these signaling molecules was inhibited (Figures 5A–D). Altogether, these data imply that MYGY-Nb might exert antiosteoporosis effects through the inhibition of RANKL-mediated NF-κB pathway.
Figure 5 MYGY-Nb inhibited osteoclast formation by suppressing the RANKL-mediated NF-κB pathway in OVX mice. Representative IHC images and quantifications of RANKL (A), IKBα (B), p-p65 (C) and NFATc1 (D). Scale bars: 100 μm. Data were presented as means ± S.D. **P < 0.01; ***P < 0.001. n = 6 in each group.
To validate the importance of MYGY-Nb in inhibition of osteoclast formation, we performed in vitro osteoclast differentiation using BMMs as osteoclast precursors. A CCK-8 assay was conducted to examine the viability of BMMs treated with MYGY-Nb. After treatment for 24, 48 and 72 h, MYGY-Nb showed no effects on BMMs at a dose of 1 and 10 μg/ml, whereas 100 μg/ml MYGY-Nb promoted cell proliferation (Figure 6A). Here, we used 10 μg/mL MYGY-Nb to treat BMMs in following osteoclastogenesis assays. After BMMs stimulated by RANKL with or without MYGY-Nb for 6 days, TRAP staining showed that MYGY-Nb led to an inhibition of RANKL-induced osteoclastogenesis (Figure 6B). Additionally, we detected the activity of NF-κB signaling pathway. The results indicated that MYGY-Nb significantly suppressed the RANKL-induced degradation of IκBα as well as upregulation of p-p65 and NFATc1 (Figures 6C–G). Together, these results indicate that MYGY-Nb inhibits RANKL-induced osteoclast formation by regulating NF-κB pathway.
Figure 6 The effect of MYGY-Nb on RANKL-induced osteoclast formation. (A) Cell viability of BMMs cultured with various concentrations of MYGY-Nb for 24 h, 48h and 72 h. (B) Formation and quantification of TRAP-positive cells from BMMs. (C–G) Effects of MYGY-Nb on the activation of the NF-κB signaling pathway in RANKL-induced BMMs. Data are presented as mean ± S.D. (n = 3), *P < 0.05; **P < 0.01; ***P < 0.001; ns, no significant difference, as compared to group treated with RANKL, n = 3 in each group.
In present study, we confirmed that MYGY-Nb is the optimal compound combination against PMOP. In OVX mice, MYGY-Nb effectively inhibits bone loss and osteoclastogenesis, as well as decreases the expression of RNAKL and NF-κB signaling genes. Subsequently, in vitro experiments further demonstrated that MYGY-Nb suppresses RANKL-induced osteoclast formation through the down-regulation of NF-κB signaling pathway. Altogether, these findings suggest that MYGY-Nb could target RANKL-mediated NF-κB pathway to attenuate osteoclast formation and bone loss.
PMOP is a most common primary osteoporosis (35). However, drugs commonly prescribed for treatment of PMOP have certain side effects, which limit their long-term use. TCM usually consists of several herbs that interact with each other to produce synergistic effects, which not only improve the efficacy but also may reduce the toxic side effects produced by single drugs (36, 37). It is well known that proper extraction of TCM formulas can optimize the synergy between effective compounds to avoid the overdose of ineffective compounds (38–40). MYGY is refined from the classic formula for kidney tonic, You-Gui-Yin, and has great anti-osteoporosis efficacy. In this study, we used N-butanol to extract MYGY and then explored its efficacy in OVX-induced osteoporosis mice. Surprisingly, ABH staining and μCT analysis both showed that MYGY-Nb was more effective in the inhibition of bone loss than the original MYGY formula, implying that the combination of compounds in MYGY-Nb has better therapeutic effects on PMOP.
In the pathogenesis of PMOP, over-activated osteoclast formation and excessive bone resorption are significant factors (23). Thus, the inhibition of osteoclast formation is an important strategy for PMOP treatment (41). In the present study, our data showed that MYGY-Nb significantly decreased the number of osteoclasts in OVX mice, which indicated that MYGY-Nb might regulate the process of osteoclastogenesis. The in vitro experiments further confirmed that MYGY-Nb significantly prevented RANKL-induced BMMs differentiation and suppressed the osteoclast formation. Then, we sought to clarify the combination of compounds in MYGY-Nb. UPLC/MS identified 16 major compounds of MYGY-Nb, in which kynylpinoside, rutinoside (Rutinos)_1, glycyrrhizin and glycyrrhetinic acid have been reported to have inhibitory effects on osteoclast formation (25–28). Our future studies are warranted to investigate the anti-osteoporosis efficacy of these compounds in more detail.
RANKL signaling is thought to be the major target of anti-resorptive agents that inhibit bone loss and osteoclast activation. RANKL-induced osteoclast differentiation activates NF-κB and NFATc1 (42–44). Under normal conditions, NF-κB protein is stored as an inactive form in the cytoplasm (the p50/p65 heterodimer associated with repressive IκBα) (45). Upon stimulation by RANKL, IκBα undergoes phosphorylation resulting in the masking of the nuclear localization signal on p65, which allows NF-κB to translocate to the nucleus. Previous studies have shown that the NF-κB inhibitor(-)-dehydroxymethylepoxyquinomycin blocks RANKL-induced osteoclast formation by down-regulating NFATc1 expression (46–49). In the present study, we found that MYGY-Nb suppressed OVX-induced expression of RANKL, p-p65 and NFATc1. In addition, MYGY-Nb also decreased the expression of IκBα, p-p65 and NFATc1 in RANKL-treated BMMs. Collectively, these data indicated that MYGY-Nb might play a key role in regulation of RANKL-mediated NF-κB signaling pathway. Thus, more precise genes of the NF-κB signaling pathway targeted by MYGY-Nb need to be explored in future studies.
In conclusion, our data reveal that MYGY-Nb exerts anti-osteoporotic efficacy by inhibiting osteoclast formation and reducing bone loss. Furthermore, we validated that the RANKL-mediated NF-κB signaling pathway plays an important role in the anti-osteoporosis efficacy of MYGY-Nb. Our findings provide evidence that MYGY-Nb is a promising therapeutic drug for PMOP.
The original contributions presented in the study are included in the article/supplementary material. Further inquiries can be directed to the corresponding authors.
The animal study was reviewed and approved by Animal Ethics Committee of Zhejiang Chinese Medical University.
Study conception and design: QZ, RX, and JC. Data acquisition: HL, WY, XW, SZ, MG, and PW. Data analysis and interpretation: XW, SZ, TX, HR, and HJ. Article drafting: QZ, RX, and HL. Critically revising important intellectual content: HQ, FY, and JC. Final approval of the version of the article to be published: HQ, FY, and JC.
This research has been partially supported by the Natural Science Foundation of China (Grant nos. 81904223), Hangzhou Medical and health science and Technology Project (B20200552).
The authors declare that the research was conducted in the absence of any commercial or financial relationships that could be construed as a potential conflict of interest.
All claims expressed in this article are solely those of the authors and do not necessarily represent those of their affiliated organizations, or those of the publisher, the editors and the reviewers. Any product that may be evaluated in this article, or claim that may be made by its manufacturer, is not guaranteed or endorsed by the publisher.
We appreciate the great help from the Public Platform of Medical Research Center, Academy of Chinese Medical Science, Zhejiang Chinese Medical University.
1. Jakob F, Genest F, Baron G, Stumpf U, Rudert M, Seefried L. [Regulation of Bone Metabolism in Osteoporosis: Novel Drugs for Osteoporosis in Development]. Unfallchirurg (2015) 118(11):925–32. doi: 10.1007/s00113-015-0085-9
2. Burge R, Dawson-Hughes B, Solomon DH, Wong JB, King A, Tosteson A. Incidence and Economic Burden of Osteoporosis-Related Fractures in the United State-2025. J Bone Miner Res (2007) 22(3):465–75. doi: 10.1359/jbmr.061113
3. Coughlan T, Dockery F. Osteoporosis and Fracture Risk in Older People. Clin Med (Lond) (2014) 14(2):187–91. doi: 10.7861/clinmedicine.14-2-187
4. Mukherjee K, Chattopadhyay N. Pharmacological Inhibition of Cathepsin K: A Promising Novel Approach for Postmenopausal Osteoporosis Therapy. Biochem Pharmacol (2016) 117:10–9. doi: 10.1016/j.bcp.2016.04.010
5. Rachner TD, Khosla S, Hofbauer LC. Osteoporosis: Now and the Future. Lancet (2011) 377(9773):1276–87. doi: 10.1016/S0140-6736(10)62349-5
6. Khosla S, Hofbauer LC. Osteoporosis Treatment: Recent Developments and Ongoing Challenges. Lancet Diabetes Endocrinol (2017) 5(11):898–907. doi: 10.1016/S2213-8587(17)30188-2
7. Cappola AR, Shoback DM. Osteoporosis Therapy in Postmenopausal Women With High Risk of Fracture. JAMA (2016) 316(7):715–6. doi: 10.1001/jama.2016.11032
8. Sasidharan S, Chen Y, Saravanan D, Sundram KM, Yoga Latha L. Extraction, Isolation and Characterization of Bioactive Compounds From Plants' Extracts. Afr J Tradit Complement Altern Med (2011) 8(1):1–10.
9. Zhao S, Baik OD, Choi YJ, Kim SM. Pretreatments for the Efficient Extraction of Bioactive Compounds From Plant-Based Biomaterials. Crit Rev Food Sci Nutr (2014) 54(10):1283–97. doi: 10.1080/10408398.2011.632698
10. Barnes J, McLachlan AJ, Sherwin CM, Enioutina EY. Herbal Medicines: Challenges in the Modern World. Part 1. Australia and New Zealand. Expert Rev Clin Pharmacol (2016) 9(7):905–15. doi: 10.1586/17512433.2016.1171712
11. Sammons HM, Gubarev MI, Krepkova LV, Bortnikova VV, Corrick F, Job KM, et al. Herbal Medicines: Challenges in the Modern World. Part 2. European Union and Russia. Expert Rev Clin Pharmacol (2016) 9(8):1117–27. doi: 10.1080/17512433.2016.1189326
12. Wang PE, Zhang L, Ying J, Jin X, Luo C, Xu S, et al. Bushenhuoxue Formula Attenuates Cartilage Degeneration in an Osteoarthritic Mouse Model Through TGF-Beta/MMP13 Signaling. J Transl Med (2018) 16(1):72. doi: 10.1186/s12967-018-1437-3
13. Xu HH, Li SM, Xu R, Fang L, Xu H, Tong PJ. Predication of the Underlying Mechanism of Bushenhuoxue Formula Acting on Knee Osteoarthritis via Network Pharmacology-Based Analyses Combined With Experimental Validation. J Ethnopharmacol (2020) 263:113217. doi: 10.1016/j.jep.2020.113217
14. Xu R, Zeng Q, Xia C, Chen J, Wang P, Zhao S, et al. Fractions of Shen-Sui-Tong-Zhi Formula Enhance Osteogenesis Via Activation of Beta-Catenin Signaling in Growth Plate Chondrocytes. Front Pharmacol (2021) 12:711004. doi: 10.3389/fphar.2021.711004
15. Kim JY, Kim YK, Choi MK, Oh J, Kwak HB, Kim JJ. Effect of Cornus Officinalis on Receptor Activator of Nuclear Factor-kappaB Ligand (RANKL)-Induced Osteoclast Differentiation. J Bone Metab (2012) 19(2):121–7. doi: 10.11005/jbm.2012.19.2.121
16. Lee SY, Lee KS, Yi SH, Kook SH, Lee JC. Acteoside Suppresses RANKL-Mediated Osteoclastogenesis by Inhibiting C-Fos Induction and NF-kappaB Pathway and Attenuating ROS Production. PloS One (2013) 8(12):e80873. doi: 10.1371/journal.pone.0080873
17. Warren JT, Zou W, Decker CE, Rohatgi N, Nelson CA, Fremont DH, et al. Correlating RANK Ligand/RANK Binding Kinetics With Osteoclast Formation and Function. J Cell Biochem (2015) 116(11):2476–83. doi: 10.1002/jcb.25191
18. Moriwaki S, Suzuki K, Muramatsu M, Nomura A, Inoue F, Into T, et al. Delphinidin, One of the Major Anthocyanidins, Prevents Bone Loss Through the Inhibition of Excessive Osteoclastogenesis in Osteoporosis Model Mice. PloS One (2014) 9(5):e97177. doi: 10.1371/journal.pone.0097177
19. Del Fattore A, Teti A, Rucci N. Osteoclast Receptors and Signaling. Arch Biochem Biophys (2008) 473(2):147–60. doi: 10.1016/j.abb.2008.01.011
20. Kim MH, Lim HJ, Bak SG, Park EJ, Jang HJ, Lee SW, et al. Eudebeiolide B Inhibits Osteoclastogenesis and Prevents Ovariectomy-Induced Bone Loss by Regulating RANKL-Induced NF-Kappab, C-Fos and Calcium Signaling. Pharm (Basel) (2020) 13(12):468. doi: 10.3390/ph13120468
21. Kikuta J, Ishii M. Osteoclast Migration, Differentiation and Function: Novel Therapeutic Targets for Rheumatic Diseases. Rheumatol (Oxf) (2013) 52(2):226–34. doi: 10.1093/rheumatology/kes259
22. Wei SJ, Zhang Q, Xiang YJ, Peng LY, Peng W, Ren Q, et al. Guizhi-Shaoyao-Zhimu Decoction Attenuates Bone Erosion in Rats That Have Collagen-Induced Arthritis via Modulating NF-kappaB Signalling to Suppress Osteoclastogenesis. Pharm Biol (2021) 59(1):262–74. doi: 10.1080/13880209.2021.1876100
23. Eghbali-Fatourechi G, Khosla S, Sanyal A, Boyle WJ, Lacey DL, Riggs BL. Role of RANK Ligand in Mediating Increased Bone Resorption in Early Postmenopausal Women. J Clin Invest (2003) 111(8):1221–30. doi: 10.1172/JCI17215
24. Krum SA, Chang J, Miranda-Carboni G, Wang CY. Novel Functions for NFkappaB: Inhibition of Bone Formation. Nat Rev Rheumatol (2010) 6(10):607–11. doi: 10.1038/nrrheum.2010.133
25. Li C, Yang Z, Li Z, Ma Y, Zhang L, Zheng C, et al. Maslinic Acid Suppresses Osteoclastogenesis and Prevents Ovariectomy-Induced Bone Loss by Regulating RANKL-Mediated NF-kappaB and MAPK Signaling Pathways. J Bone Miner Res (2011) 26(3):644–56. doi: 10.1002/jbmr.242
26. Zhong Z, Qian Z, Zhang X, Chen F, Ni S, Kang Z, et al. Tetrandrine Prevents Bone Loss in Ovariectomized Mice by Inhibiting RANKL-Induced Osteoclastogenesis. Front Pharmacol (2019) 10:1530. doi: 10.3389/fphar.2019.01530
27. Qian Z, Zhong Z, Ni S, Li D, Zhang F, Zhou Y, et al. Cytisine Attenuates Bone Loss of Ovariectomy Mouse by Preventing RANKL-Induced Osteoclastogenesis. J Cell Mol Med (2020) 24(17):10112–27. doi: 10.1111/jcmm.15622
28. Xia C, Wang P, Fang L, Ge Q, Zou Z, Dong R, et al. Activation of Beta-Catenin in Col2-Expressing Chondrocytes Leads to Osteoarthritis-Like Defects in Hip Joint. J Cell Physiol (2019) 234(10):18535–43. doi: 10.1002/jcp.28491
29. Wang C, Xiao F, Qu X, Zhai Z, Hu G, Chen X, et al. Sitagliptin, An Anti-Diabetic Drug, Suppresses Estrogen Deficiency-Induced OsteoporosisIn Vivo and Inhibits RANKL-Induced Osteoclast Formation and Bone Resorption In Vitro. Front Pharmacol (2017) 8:407. doi: 10.3389/fphar.2017.00407
30. Wang QL, Huo XC, Wang JH, Wang DP, Zhu QL, Liu B, et al. Rutin Prevents the Ovariectomy-Induced Osteoporosis in Rats. Eur Rev Med Pharmacol Sci (2017) 21(8):1911–7.
31. Chauhan S, Sharma A, Upadhyay NK, Singh G, Lal UR, Goyal R. In-Vitro Osteoblast Proliferation and in-Vivo Anti-Osteoporotic Activity of Bombax Ceiba With Quantification of Lupeol, Gallic Acid and Beta-Sitosterol by HPTLC and HPLC. BMC Complement Altern Med (2018) 18(1):233. doi: 10.1186/s12906-018-2299-1
32. Yin Z, Zhu W, Wu Q, Zhang Q, Guo S, Liu T, et al. Glycyrrhizic Acid Suppresses Osteoclast Differentiation and Postmenopausal Osteoporosis by Modulating the NF-Kappab, ERK, and JNK Signaling Pathways. Eur J Pharmacol (2019) 859:172550. doi: 10.1016/j.ejphar.2019.172550
33. Wu C, Wang N, Xu P, Wang X, Shou D, Zhu Y. Preparation and Application of Polyvinyl Alcohol-Decorated Cell Membrane Chromatography for Screening Anti-Osteoporosis Components From Liuwei Dihuang Decoction-Containing Serum. J Sep Sci (2020) 43(11):2105–14. doi: 10.1002/jssc.201901203
34. Manolagas SC, Jilka RL. Bone Marrow, Cytokines, and Bone Remodeling. Emerging Insights Into the Pathophysiology of Osteoporosis. N Engl J Med (1995) 332(5):305–11. doi: 10.1056/NEJM199502023320506
35. Andreopoulou P, Bockman RS. Management of Postmenopausal Osteoporosis. Annu Rev Med (2015) 66:329–42. doi: 10.1146/annurev-med-070313-022841
36. Gao Z, Lu Y, Halmurat U, Jing J, Xu D. Study of Osteoporosis Treatment Principles Used Historically by Ancient Physicians in Chinese Medicine. Chin J Integr Med (2013) 19(11):862–8. doi: 10.1007/s11655-013-1328-z
37. Jin R, Lin ZJ, Xue CM, Zhang B. An Improved Association-Mining Research for Exploring Chinese Herbal Property Theory: Based on Data of the Shennong's Classic of Materia Medica. J Integr Med (2013) 11(5):352–65. doi: 10.3736/jintegrmed2013051
38. Jiang WY. Therapeutic Wisdom in Traditional Chinese Medicine: A Perspective From Modern Science. Trends Pharmacol Sci (2005) 26(11):558–63. doi: 10.1016/j.tips.2005.09.006
39. Wang XL, Wang NL, Zhang Y, Gao H, Pang WY, Wong MS, et al. Effects of Eleven Flavonoids From the Osteoprotective Fraction of Drynaria Fortunei (KUNZE) J. SM. @ on Osteoblastic Proliferation Using an Osteoblast-Like Cell Line. Chem Pharm Bull (Tokyo) (2008) 56(1):46–51. doi: 10.1248/cpb.56.46
40. Zhou J, Zhou T, Jiang M, Wang X, Liu Q, Zhan Z, et al. Research Progress on Synergistic Anti-Tumor Mechanisms of Compounds in Traditional Chinese Medicine. J Tradit Chin Med (2014) 34(1):100–5. doi: 10.1016/s0254-6272(14)60062-5
41. Lewiecki EM. New Targets for Intervention in the Treatment of Postmenopausal Osteoporosis. Nat Rev Rheumatol (2011) 7(11):631–8. doi: 10.1038/nrrheum.2011.130
42. Shinohara M, Takayanagi H. Novel Osteoclast Signaling Mechanisms. Curr Osteoporos Rep (2007) 5(2):67–72. doi: 10.1007/s11914-007-0005-1
43. de la Rica L, Garcia-Gomez A, Comet NR, Rodriguez-Ubreva J, Ciudad L, Vento-Tormo R, et al. NF-kappaB-Direct Activation of microRNAs With Repressive Effects on Monocyte-Specific Genes is Critical for Osteoclast Differentiation. Genome Biol (2015) 16:2. doi: 10.1186/s13059-014-0561-5
44. Hu H, Li C, Zhang H, Wu G, Huang Y. Role of Vasodilator-Stimulated Phosphoprotein in RANKL-Differentiated Murine Macrophage RAW264.7 Cells: Modulation of NF-Kappab, C-Fos and NFATc1 Transcription Factors. Exp Ther Med (2021) 21(5):412. doi: 10.3892/etm.2021.9856
45. Ghosh S, Karin M. Missing Pieces in the NF-kappaB Puzzle. Cell (2002) 109 Suppl:S81–96. doi: 10.1016/s0092-8674(02)00703-1
46. Takatsuna H, Asagiri M, Kubota T, Oka K, Osada T, Sugiyama C, et al. Inhibition of RANKL-Induced Osteoclastogenesis by (-)-DHMEQ, a Novel NF-kappaB Inhibitor, Through Downregulation of Nfatc1. J Bone Miner Res (2005) 20(4):653–62. doi: 10.1359/JBMR.041213
47. Park JH, Lee NK, Lee SY. Current Understanding of RANK Signaling in Osteoclast Differentiation and Maturation. Mol Cells (2017) 40(10):706–13. doi: 10.14348/molcells.2017.0225
48. Zhang Y, Xu S, Li K, Tan K, Liang K, Wang J, et al. Mtorc1 Inhibits NF-Kappab/NFATc1 Signaling and Prevents Osteoclast Precursor Differentiation, In Vitro and In Mice. J Bone Miner Res (2017) 32(9):1829–40. doi: 10.1002/jbmr.3172
Keywords: N-butanol extraction, Modified You-Gui-Yin, postmenopausal osteoporosis, osteoclast formation, NF-κB pathway
Citation: Zeng Q, Xu R, Ling H, Zhao S, Wang X, Yuan W, Gu M, Xu T, Wang P, Ruan H, Jin H, Qu H, Ye F and Chen J (2022) N-Butanol Extract of Modified You-Gui-Yin Attenuates Osteoclastogenesis and Ameliorates Osteoporosis by Inhibiting RANKL-Mediated NF-κB Signaling. Front. Endocrinol. 13:925848. doi: 10.3389/fendo.2022.925848
Received: 22 April 2022; Accepted: 23 May 2022;
Published: 24 June 2022.
Edited by:
Ling-Feng Zeng, Guangdong Provincial Hospital of Chinese Medicine, ChinaReviewed by:
Bing Shu, Shanghai University of Traditional Chinese Medicine, ChinaCopyright © 2022 Zeng, Xu, Ling, Zhao, Wang, Yuan, Gu, Xu, Wang, Ruan, Jin, Qu, Ye and Chen. This is an open-access article distributed under the terms of the Creative Commons Attribution License (CC BY). The use, distribution or reproduction in other forums is permitted, provided the original author(s) and the copyright owner(s) are credited and that the original publication in this journal is cited, in accordance with accepted academic practice. No use, distribution or reproduction is permitted which does not comply with these terms.
*Correspondence: Jiali Chen, Y2hlbmppYWxpMTU4NUAxNjMuY29t; Fusheng Ye, eWVmdXNoZW5nMDUyMEAxNjMuY29t; Hangbo Qu, cXVoYjEyMjJAMTYzLmNvbQ==
†These authors have contributed equally to this work
Disclaimer: All claims expressed in this article are solely those of the authors and do not necessarily represent those of their affiliated organizations, or those of the publisher, the editors and the reviewers. Any product that may be evaluated in this article or claim that may be made by its manufacturer is not guaranteed or endorsed by the publisher.
Research integrity at Frontiers
Learn more about the work of our research integrity team to safeguard the quality of each article we publish.