- 1Chinese Academy of Science (CAS) Key Laboratory of Tropical Marine Bio-resources and Ecology (LMB), Guangdong Provincial Key Laboratory of Applied Marine Biology, South China Sea Institute of Oceanology, Chinese Academy of Sciences, Guangzhou, China
- 2Southern Marine Science and Engineering Guangdong Laboratory (Guangzhou), Guangzhou, China
- 3University of Chinese Academy of Sciences, Beijing, China
Neurohypophysial hormones regulate the reproductive behavior of teleosts; however, their role in the gestation and parturition of ovoviviparous fishes with male pregnancy (syngnathids) remains to be demonstrated. In the present study, the complementary DNA (cDNA) sequences of arginine vasotocin (VT) and isotocin (IT) from the lined seahorse (Hippocampus erectus) were cloned and identified. We observed that the mature core peptides of seahorse VT and IT were conserved among teleosts. In the phylogenic tree, seahorse VT and IT were clustered independently with teleost VT and IT. The tissue distribution patterns of VT and IT were similar, and both were highly expressed in the brain, gills, and gonads. Interestingly, they were also expressed to some extent in the brood pouch. In situ hybridization revealed that VT and IT messenger RNA (mRNA) signals in the brain were mainly located in the preoptic area region of the hypothalamus. Intraperitoneal administration of the VT core peptide to pregnant seahorses induced premature parturition, stimulated gonadotropin release, increased serum estrogen levels, and decreased prolactin secretion. Moreover, VT injection upregulated the mRNA expression of the membrane estrogen receptor in the brood pouch. In summary, neurohypophysial hormones promote premature parturition by regulating estrogen synthesis through the hypothalamus–pituitary–gonad axis.
Introduction
Neurohypophysial hormones are hypothalamic neuropeptides transported to the posterior pituitary and function as the regulators of vertebrate reproduction. In mammals, neurohypophysial hormones, including oxytocin (OT) and arginine vasopressin (AVP), are primarily involved in uterine contraction, parturition, and lactation (1). OT and AVP only differ in two amino acids, which arose from a gene duplication event during evolution (2). In teleosts, isotocin (IT) is homologous to the mammalian OT whereas arginine vasotocin (VT) is homologous to AVP (3, 4). VT and IT are nonapeptide neurohormones that are primarily synthesized in the neurons of the hypothalamus. They play central roles in fish physiology and behavior, including reproduction, metabolism, osmoregulation, and social behavior (5, 6).
The administration of nonapeptide hormones affects the sexual behaviors and parturition of teleosts (7, 8). IT affects the ovulation of oviparous fishes, such as killifish (Fundulus heteroclitus), seurukan fish (Osteochilus vittatus), and Hoven’s carp (Leptobarbus hoevenii) (9–11). Similarly, VT injections stimulate the courtship behavior of the male white perch (Morone americana) (12) and influence the aggressive behavior of the rainbow trout (13). Moreover, VT can modulate steroidogenic shift and control serum E2 levels to stimulate oocyte maturation in female catfish (Heteropneustes fossilis) (14). In ovoviviparous fishes, the administration of IT and VT induces premature parturition in the guppy (Poecilia reticulata) and topminnow (Gambusia affinis) (15).
Syngnathids (pipefish and seahorse) are ovoviviparous fishes with male pregnancy and complex reproductive behavior (16). Syngnathid females transfer eggs into the brood pouch, which is a special structure on the ventral surface of males. Then, the eggs are fertilized and developed in the brood pouch until hatching. The brood pouch is similar to the mammalian placenta, and it can provide nutrition and oxygen to the embryos (17, 18). However, the endocrinological mechanism underlying the seahorse reproductive behavior, including male pregnancy and parturition, is still unknown.
In this study, we characterized the neurohypophysial hormones (VT and IT) of the lined seahorse (Hippocampus erectus) and detected their expression patterns in adult tissues. We also analyzed the locations of VT and IT genes in the brain. Finally, VT was injected in vivo to evaluate its functional role in the parturition of pregnant seahorses, its effects on serum sex steroid hormones, and the expression profiles of regulatory genes in the hypothalamus–pituitary–gonad (HPG) axis.
Materials and methods
Experimental fish and tissue sampling
Cultured adult lined seahorses (H. erectus) (6 months old) were collected from the Zhangzhou Fisheries Center, Fujian Province, China. Seahorses were maintained in seawater tanks at 26 ± 0.5°C, 26 ± 2% salinity, and a 12:12-h day-light cycle. The seahorses were fed twice daily with frozen Mysis shrimp and reared for 2 weeks before the experiments. The lined seahorses were anesthetized with 0.05% MS-222 (Sigma, St. Louis, MO, USA) and dissected. Tissues were collected, frozen in liquid nitrogen, and stored at an -80°C freezer until RNA extraction. All animal experiments were conducted in accordance with the guidelines and approval of the Animal Research and Ethics Committee of the Chinese Academy of Sciences.
Cloning of the seahorse VT and IT genes
Total RNA was isolated from the brain using a TRIzol reagent (Invitrogen, Carlsbad, CA, USA), according to the manufacturer’s instructions. Total RNA (1 μg) was reverse-transcribed using the ReverTra Ace-α highly efficient reverse transcription kit (Toyobo, Osaka, Japan). Based on the brain transcriptome and genome data of the lined seahorse (19, 20), the full-length cDNA sequences of seahorse VT and IT were obtained using the rapid amplification of cDNA ends (RACE). The 5′-UTR and 3′-UTR ends were amplified using a SMARTer RACE 5′/3′ kit (Clontech, Palo Alto, CA, USA). The PCR primers used are listed in Table 1. Target fragments were subcloned into a PMD18-T vector (Takara, Dalian, China). The positive clones of target fragments were sequenced by Tsingke BioTech (Beijing, China).
Phylogenetic and synteny analysis of seahorse vasotocin and isotocin
The cDNA sequences of seahorse VT and IT were translated into amino acid sequences by using Expasy (http://web.expasy.org/protparam). Multiple sequence alignments were performed using the DNAMAN software. The signal peptide was predicted using the SignalP 5.0 server (http://www.cbs.dtu.dk/services/SignalP/). A phylogenetic tree was constructed with Mega 6.0 software (21) using the neighbor-joining method (bootstrap = 1,000). A synteny analysis of VT and IT genes was performed by comparing the gene arrangements of the VT and IT gene loci in zebrafish, platyfish, tilapia, medaka, fugu, tiger-tailed seahorse, and lined seahorse. The gene loci of these teleosts were identified using the genome data from Ensembl (http://www.ensembl.org).
Tissue distribution of seahorse vasotocin and isotocin
Total RNA was isolated from various tissues of male seahorses (n=3), including the brain regions (telencephalon, optic tectum, cerebellum, hypothalamus, and pituitary), liver, gill, intestine, kidney, muscle, heart, gonad, and brood pouch. Reverse transcription PCR was performed using 1 μg of RNA isolated from each tissue. PCR conditions were as follows: predenaturation at 94°C for 3 min; 30 cycles at 94°C for 15 s, 55°C for 15 s, 72°C for 30 s, and extension at 72°C for 10 min; and cooling to 4°C. The housekeeping gene (β-actin) was used as a positive control.
RNA in situ hybridization
The fragments of VT and IT genes (528 and 589 bp, respectively) were subcloned into the pGEM-T easy vector. Plasmid DNA containing the VT and IT inserts was extracted using the E.Z.N.A Plasmid DNA Mini kit (Omega, Norcross, GA, USA). Sense and antisense riboprobes were synthesized from SalI- and NcoI-linearized pGEM-T easy plasmids using the DIG RNA Labeling kit (Roche, Mannheim, Germany). The brain samples of lined seahorse were fixed in 4% paraformaldehyde overnight at 4°C. The fixed brains were then dehydrated in 20% sucrose and embedded in an optimal cutting temperature (OCT) compound (Sakura, Tokyo, Japan). Cross-sections (10 μm), including those of the hypothalamus area, were collected on 3-aminopropylysilane-coated slides. Collected sections were digested with proteinase K (1 μg/ml) at 37°C for 20 min. The slices were prehybridized with hybridization buffer without probe at 58°C for 1 h and hybridized with DIG-labeled probes (0.5 μg/ml) in hybridization buffer at 58°C overnight in a sealed humidity chamber. After hybridization, slides were washed in 2× saline-sodium citrate (SSC) buffer and 0.1× SSC buffer for 30 min at 58°C. The slides were then blocked with 2% normal sheep serum and incubated with an alkaline phosphatase–conjugated anti-DIG antibody (Roche; 1:1,000 dilution). The color change reaction was conducted using an nitroblue tetrazolium (NBT)/5-bromo-4-chloro-3-indolye phosphate (BCIP) stock solution (Roche, Basel, Switzerland), and the reaction was stopped using tap water.
In vivo effects of vasotocin on the parturition of pregnant seahorses
To evaluate the functional role of VT in parturition, pregnant seahorses were injected with [Arg8] vasotocin acetate salt (VT, V7009; Sigma). The pregnant seahorses (6 months old) were anesthetized and injected intraperitoneally with VT (high dose: 500 ng/g; low dose: 50 ng/g) and saline (n = 6) as the sham control. Seahorses from each group were sampled at 6-h postinjection. The pituitary and brood pouch were dissected and frozen in liquid nitrogen until RNA extraction. Blood samples were collected from the tail vessels at 6-h postinjection. Serum samples were separated by centrifugation at 3,000 × g for 30 min at 4°C and stored at -20°C. The serum levels of estrogen and testosterone were determined via an enzyme-linked immunosorbent assay (ELISA) kit (Cayman Chemical Company, Ann Arbor, MI, USA) (Estradiol ELISA kit no. 501890 and Testosterone ELISA kit no. 582701) according to the manufacturer’s protocol (22). Approximately 5 μl of serum sample was diluted in 45 μl of ELISA buffer to detect the concentration of estrogen and testosterone using the standard samples as a reference. The mRNA expression profiles of gonadotropins, follicle-stimulating hormone (FSHβ), luteinizing hormone (LHβ), and prolactin (PRL) in the pituitary were analyzed.
Quantitative real-time PCR
The expression levels of all the tested genes were measured using the qRT-PCR methodology described previously (22). The primers used for the qRT-PCR assays are listed in Table 1. The template was amplified at 94°C for 1 min, followed by 40 cycles at 94°C for 15 s, 50−55°C for 15 s, and extension at 72°C for 20 s. The housekeeping gene β-actin was used as the reference gene. The relative abundance of each target gene was evaluated using the 2−ΔΔCt method (23).
Statistical analysis
Data were shown as the mean ± standard error of the mean (SEM). Significant differences were evaluated by one-way analysis of variance (ANOVA), followed by Duncan’s multiple range tests using Prism 6.0 (GraphPad Software, San Diego, CA, USA). Differences between groups with p < 0.05 were deemed significant.
Results
Characterization of seahorse VT and IT genes
The lined seahorse VT cDNA sequence (GenBank Accession No. MW490038) had an Open Reading Frame (ORF) of 477 bp, encoding for a protein of 158 amino acids (aa) (Figure 1A). The VT precursor had a signal peptide of 24 aa, a 9-aa hormone moiety (CYIQNCPRG), an enzymatic processing site [Gly-Lys-Arg (GKR)], and an 88-aa neurophysin. The IT precursor cDNA (GenBank Accession No. MW490039) had an ORF of 471 bp, encoding for a protein of 156 aa (Figure 1B). The IT precursor had a signal peptide of 19 aa, a hormone moiety of 9 aa (CYIQNCPLG), an enzymatic processing site, a 95-aa neurophysin, and a 30-aa copeptin region with a leucine-rich core segment. Homology analysis showed that seahorse VT had the highest sequence identity with the VT sequences of medaka (77.8%) and clownfish (77.8%) and that the IT sequence was highly similar to that of clownfish (80.1%) and flounder (78.8%) (Table 2).
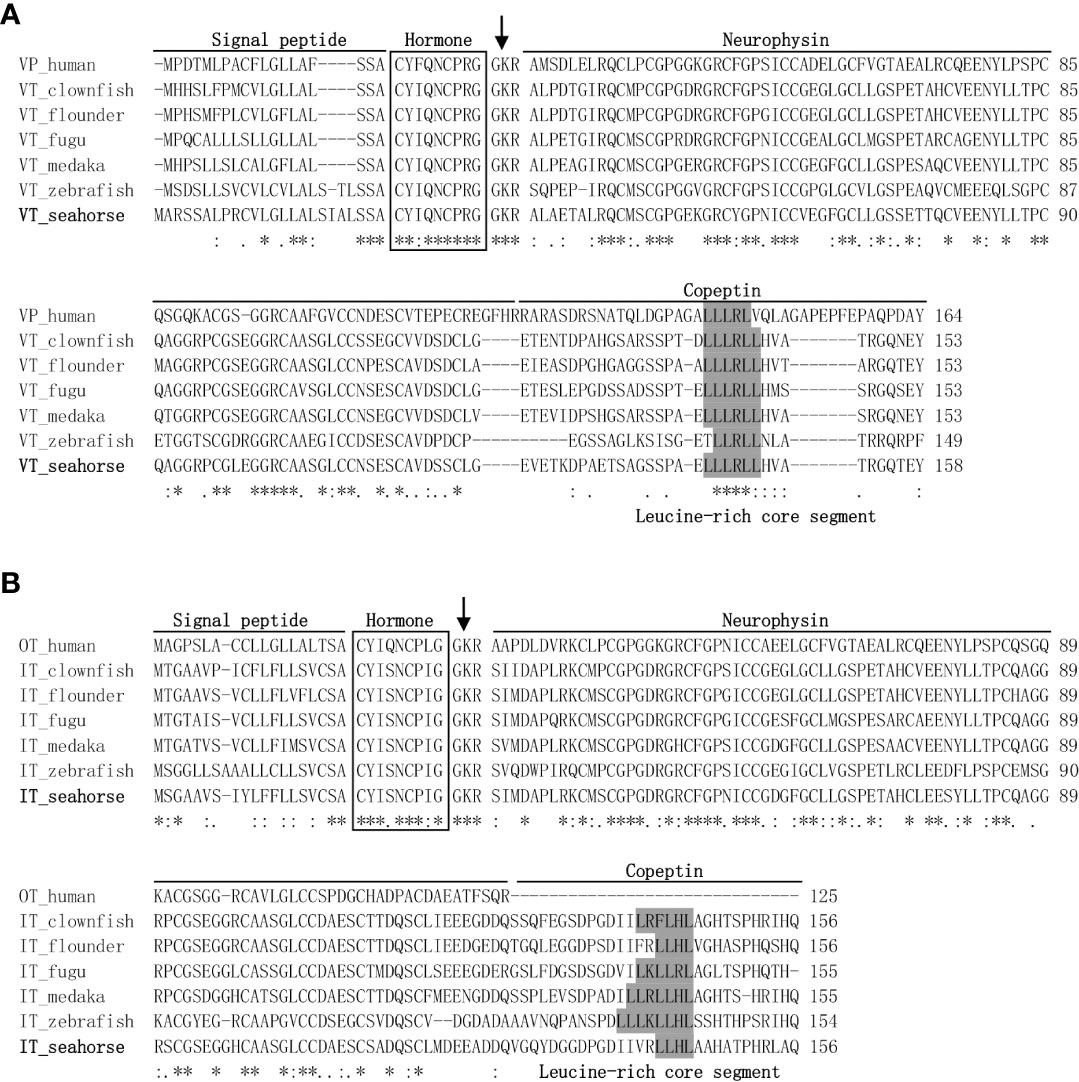
Figure 1 Genetic structure and multiple sequence alignments of vasotocin (VT) (A) and isotocin (IT) (B). The mature hormone peptides are boxed, and the GKR cleavage site was marked with an arrow. Genetic structures, including a signal peptide, neurophysin, and copeptin parts, were lined out. GenBank accession numbers for teleost VT and IT proteins are listed in Table 2. Symbol asterisks means that the amino acid residues in that column are identical in the alignment.
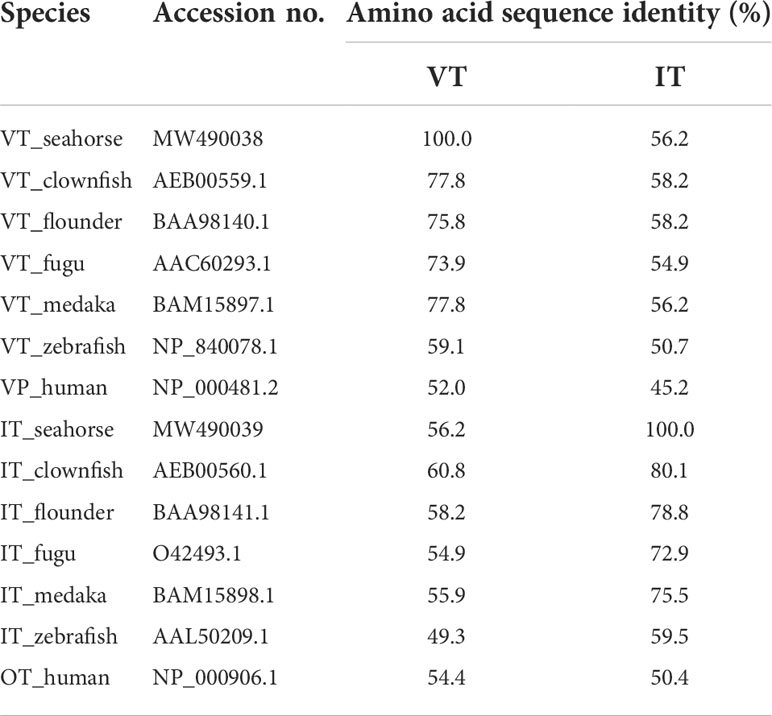
Table 2 Similarities of amino acid sequences of the neurohypophysial hormone between the lined seahorse and other teleosts.
Phylogeny and synteny analyses of seahorse vasotocin and isotocin
The phylogenetic tree revealed that the neurohypophysial hormones were clustered into two separate clades: VT and IT clades. Seahorse VT were clustered together with other teleost VT precursors, while seahorse IT were clustered with other teleost IT precursors (Figure 2). Human vasopressin (VP) and OT were used as outgroups. A synteny analysis of VT and IT showed that the organization and orientation of these genes in the loci were highly conserved among teleost species (Figure S1). The synteny of VT and IT in seahorse was the same as that in other teleosts, except for zebrafish, in which VT and IT were located on two separate chromosomes.
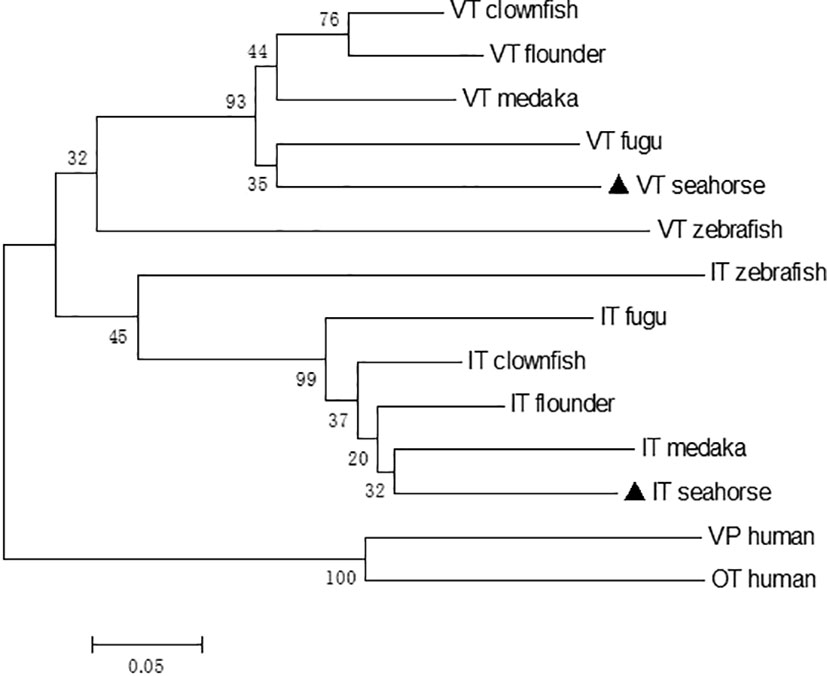
Figure 2 Neighbor-joining phylogenetic tree of VT and IT and their homologous sequences in vertebrates. The numbers indicate the bootstrap values of 1,000 replicates.
Tissue distribution of seahorse vasotocin and isotocin
The tissue distribution of seahorse VT and IT was determined using semiquantitative reverse transcription PCR (Figure 3). Both VT and IT mRNAs were widely expressed in the central nervous system and peripheral tissues and were highly expressed in the hypothalamus, gills, and gonads. Interestingly, these genes were also expressed in the brood pouch to some extent.
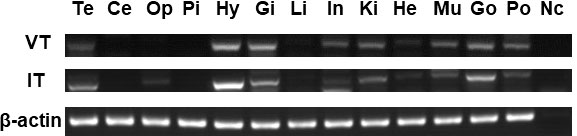
Figure 3 Analysis of VT and IT expression patterns in various tissues of male seahorses by reverse transcription PCR. The brain regions: Te, telencephalon; Ce, cerebellum; Op, optic tectum; Pi, pituitary; and Hy, hypothalamus. The peripheral tissues: Gi, gill; Li, liver; In, intestine; Ki, kidney; He, heart; Mu, muscle; Go, gonad; Po, brood pouch. Nc, negative control.
Localization of vasotocin and isotocin in the brain
The functions of lined seahorse VT and IT were evaluated based on their relative expression patterns. Therefore, the localization of the cells expressing VT and IT mRNAs was detected in the lined seahorse brain (hypothalamus) via in situ hybridization. Both VT and IT mRNAs were located in the preoptic area (POA) region of the hypothalamus (Figure 4). VT mRNA was mainly expressed in the central area of the POA region, whereas IT mRNA was expressed in the edge area of the POA region.
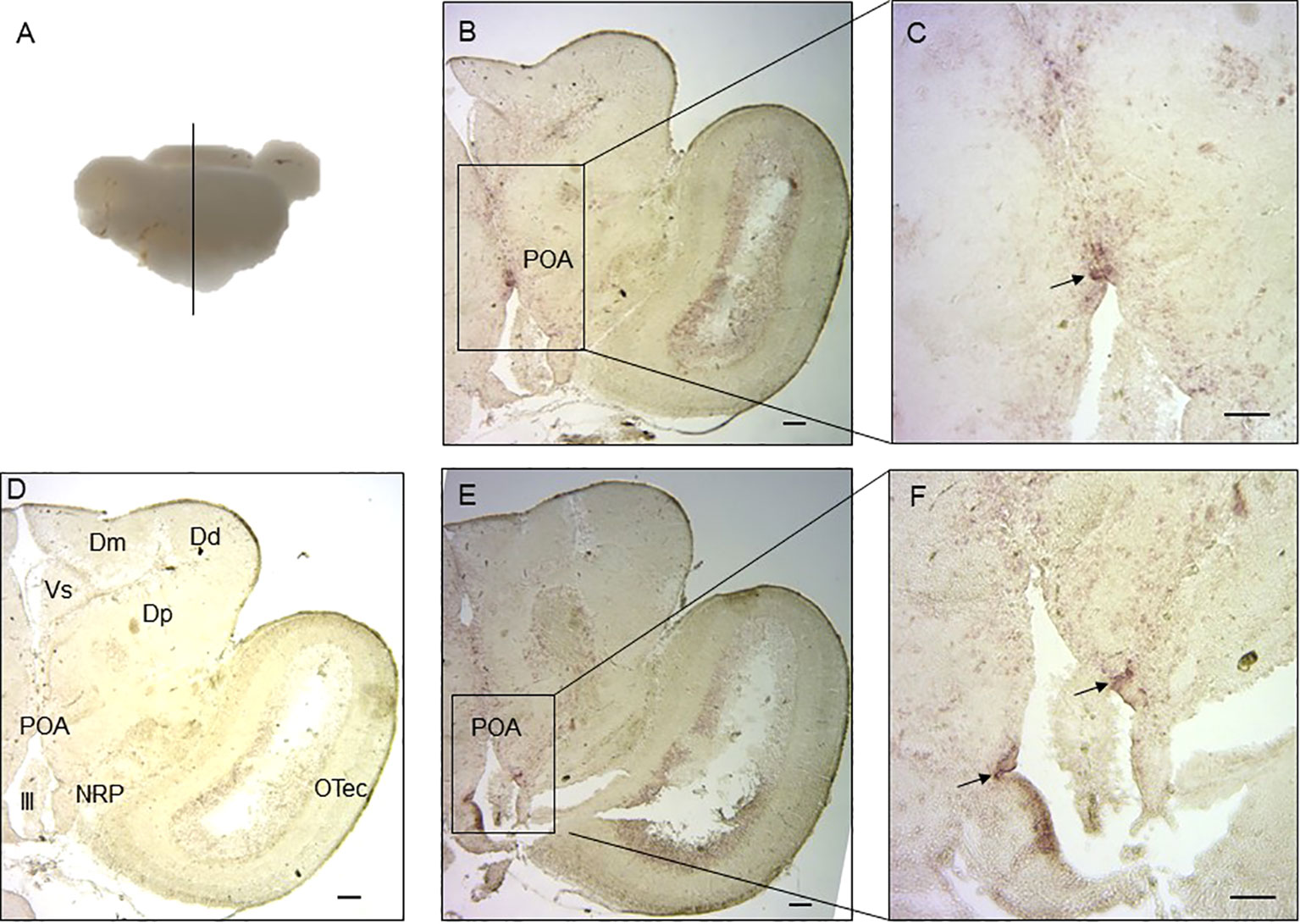
Figure 4 Localization of cells expressing VT and IT mRNAs in the lined seahorse brain. (A) Schematic diagram indicating the position of the coronal section and brain slides selected in the following. (B) In situ hybridization with an antisense VT mRNA probe showing the midbrain coronal section. (C) Enlarged part of the hypothalamus region using a VT antisense probe. (D) In situ hybridization with a sense VT probe within hypothalamus and midbrain regions of the lined seahorse. The brain regions are marked out in the micrograph. (E). In situ hybridization with an antisense IT mRNA probe showing the midbrain coronal section. (F) Enlarged part of the hypothalamus region using and IT antisense probe. OTec, optic tectum; Dd, dorsal part of dorsal telencephalon; Dp, posterior part of dorsal telencephalon; Dm, medial part of dorsal telencephalon; Vs, supracommissural nucleus of ventral telencephalon; POA, preoptic area; NRP, the nucleus of posterior recess; III, the third ventricle. Scale bars = 200 μm.
Effects of vasotocin administration on the parturition of pregnant seahorses
After VT injection, serum estrogen levels increased remarkably, whereas serum testosterone levels did not change significantly (Figure 5A). The pregnant seahorses continued swinging their body to perform parturition behavior to let the seahorse larvae hatch out from the brood pouch. In addition, premature seahorse larvae (induced by high and low doses of VT) still had large yolk sacs and were shorter than the larvae that hatched normally (Figure 5B, Table 3). FSHβ and LHβ mRNA levels increased significantly, whereas PRL mRNA levels decreased significantly in the pituitary of pregnant seahorses. Moreover, the mRNA expression of the membrane estrogen receptor [G protein-coupled estrogen receptor (GPER)] in the brood pouch significantly increased after a high-dose VT injection, whereas ERα and ERβ mRNAs showed no significant changes (Figure 6).
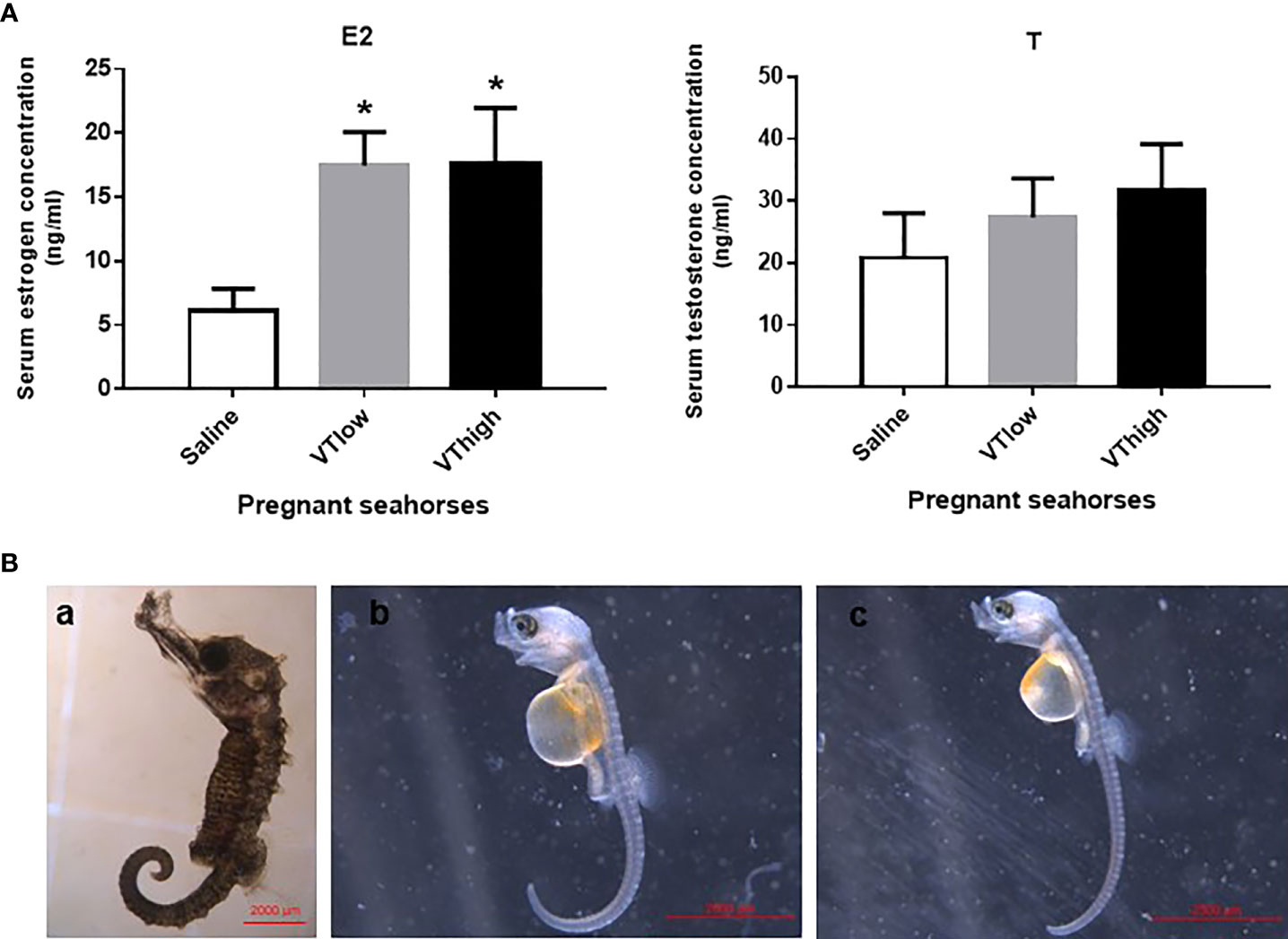
Figure 5 Serum estrogen and testosterone levels after VT injection (A). Seahorse larvae injected with low (B-b) and high doses (B-c) of VT compared to normal hatched seahorse larvae (B-a). Data are presented as mean ± SEM. Asterisks indicate significant differences between different treatments (p < 0.05).
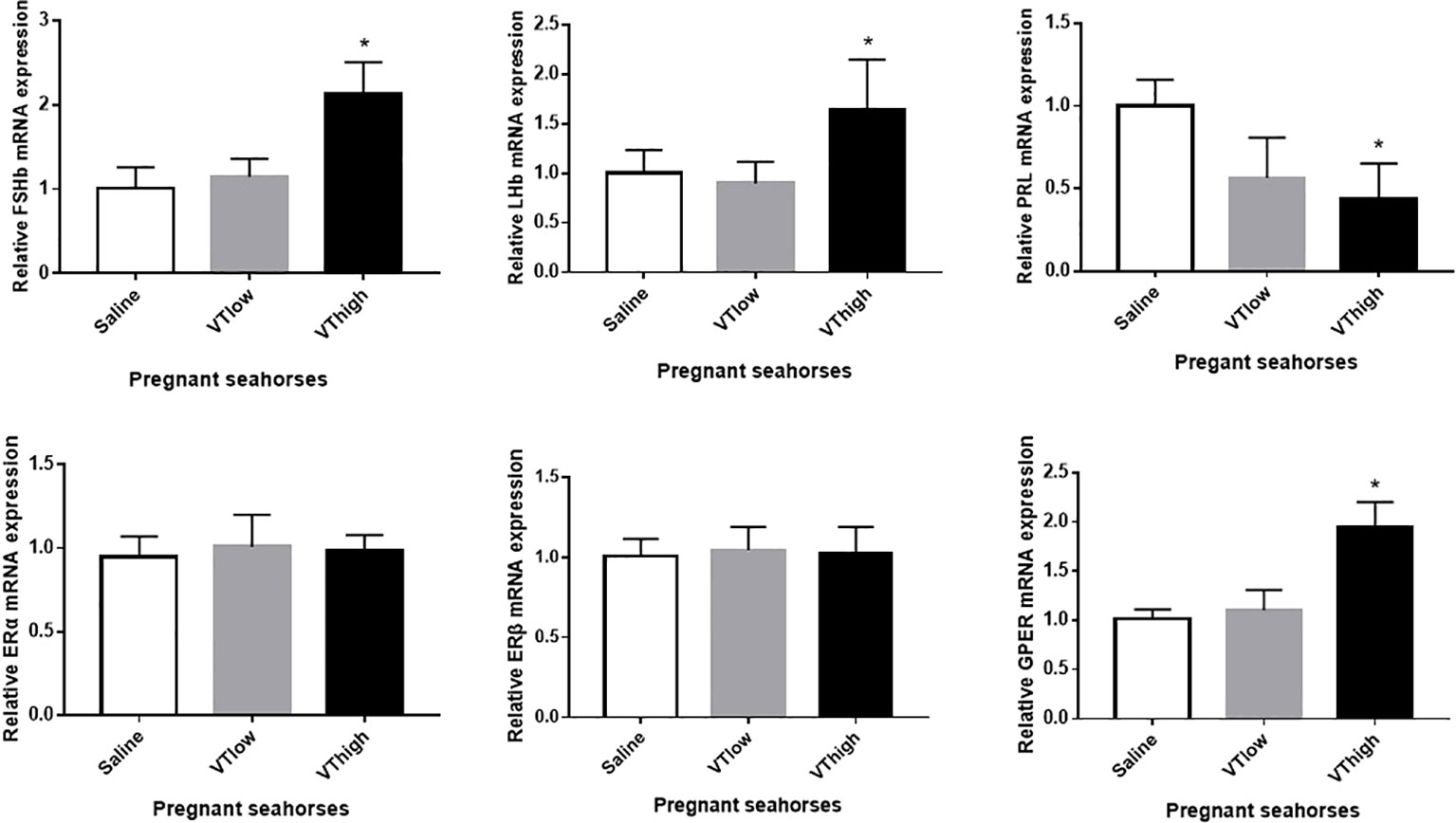
Figure 6 Effects of the VT core peptide injection on the expression of FSHβ, LHβ, and PRL genes in the pituitary and ERα, ERβ, and GPER genes in the brood pouch of pregnant lined seahorses. The mRNA expression levels were determined using qRT-PCR. Data are presented as mean ± SEM. Asterisks indicate significant differences between different treatments (p < 0.05).
Discussion
In the present study, the cDNAs of the neurohypophysial hormones VT and IT were cloned and characterized in the lined seahorse. Sequence analysis revealed that the mature peptides of VT and IT were highly conserved among the teleost species. In addition to the signal and mature peptides, the neurohypophysial nonapeptide precursor had three domains: GKR, neurophysin, and copeptin (2, 24). The GKR domain, which is involved in the cleavage and amidation of the hormone, was conserved. However, the N-terminus of neurophysin was divergent whereas the rest was conserved. The domains of neurohypophysial hormones in teleosts are identical (3). Phylogeny analysis revealed that seahorse VT was clustered with other teleost VTs, and seahorse IT was clustered with other teleost ITs independently. Synteny analysis showed that VT and IT gene loci were conserved among teleosts. These results showed that the cloned seahorse VT and IT genes are orthologous to those of other teleosts.
Reverse transcription PCR showed that both VT and IT mRNAs are highly expressed in the hypothalamus, gills, and gonads of the lined seahorse, similar to the findings in other teleosts (25, 26). Seahorse VT mRNA was expressed much more widely than IT mRNA, suggesting that VT plays more diverse roles in various tissues than IT. The high expression in sexual tissues suggests that VT and IT play important roles in regulating reproductive behavior. In addition, the expression in the gills suggests that VT and IT are involved in osmoregulation (5).
In situ hybridization showed that seahorse VT and IT were mainly expressed in the cells of the POA region in the hypothalamus. This result is consistent with the findings in the orange-spotted grouper (27), bluehead wrasse (7), and weakly electric fish (28). Most studies in teleosts have suggested that VT and IT expression in the brain is limited to the hypothalamus, especially in the POA region, which is the center of sex-related genes. Surprisingly, VT mRNA is expressed in the dorsal and medial telencephalic regions of the cichlid fish (Astatotilapia burtoni) (29). These results indicate that the neurohypophysial hormones regulate sexual behavior through interactions with other key genes involved in reproductive behavior.
VT plays a role in regulating the sexual behavior of both male and female fishes. VT controls oocyte maturation and ovulation in female catfish by stimulating estrogen secretion and inhibiting progestin steroids (14). In male zebrafish, VT stimulates courtship behavior and reproductive physiology (30). In addition, VT plays a role in regulating aggressive behavior in mudskippers (31). In ovoviviparous fishes, VT can induce premature parturition in the topminnow (G. affinis) and guppy (P. reticulata) (15). VT can also induce premature parturition in ovoviviparous fishes with male pregnancy, including in lined seahorses. We observed that after VT injection, pregnant seahorses performed parturition and continuously shook their bodies to let the seahorse fries hatch out from the brood pouch. Sex steroids, including estrogen, play an important role in regulating sex-role reversal behavior (32). In lined seahorses, serum estrogen levels increase during pregnancy and decrease during the post-pregnancy stage (33). In the present study, the mRNA levels of GPER increased significantly after VT injection. This finding is in agreement with the postparturition upregulation of GPER in big-belly seahorses (34). These findings demonstrate that sex steroids, such as estrogen, play important roles in the parturition of pregnant seahorses. Moreover, VT induces parturition in pregnant seahorses and brood pouch compression, a process that may be mediated by GPER.
In the lined seahorse, VT induces estrogen secretion by stimulating gonadotropin (FSHβ and LHβ) synthesis during the parturition stage. This finding suggests that VT regulates reproductive physiology through the HPG axis in lined seahorses. VT also downregulated the PRL mRNA expression during parturition in lined seahorses. PRL maintains the brood pouch in a proper condition to support embryonic development in seahorses. In addition, PRL hormone levels are higher in the mating stage than in the parturition stage (16). These results indicate that VT is involved in the premature parturition of seahorses by upregulating estrogen secretion and downregulating PRL synthesis. Taken together, VT regulates the fish reproductive behavior via the HPG axis, in which the VT-stimulated gonadotropin synthesis and inhibited prolactin secretion in the pituitary regulate the estrogen secretion.
The premature release of embryos might be a stress response in pregnant seahorses. Insufficient nutrition, characterized by limited food consumption for a relatively long period, might promote premature parturition to release the embryos and keep them alive through VT stimulation. Studies have shown that VT is involved in the stress response; VT neurons innervate the corticotropic cells of the pituitary and influence adrenocorticotropin, which is secreted from the interrenal (5, 35). Pregnant seahorses may upregulate VT hormones in response to stress when suffering from nutritional deficiencies.
In conclusion, the present study reported the physiological role of neurohypophysial hormones in the reproductive behavior of ovoviviparous fishes with male pregnancy. Moreover, we demonstrated their roles in regulating parturition through the HPG axis. These findings suggest that VT regulates parturition behavior by upregulating serum estrogen levels. In addition, VT induces parturition through the membrane estrogen receptor (GPER). Our results shed light on the endocrinological mechanism of neurohypophysial hormones in regulating the reproductive behavior of teleosts.
Data availability statement
The original contributions presented in the study are included in the article/Supplementary Material. Further inquiries can be directed to the corresponding author.
Ethics statement
The animal study was reviewed and approved by the Animal research and Ethics Committees of the Chinese Academy of Sciences.
Author contributions
HZ and QL designed the research. HZ and YL carried out the experiment. HZ, YL, and GQ analyzed the experiment data. QL provided lab space and equipment. All authors contributed to the article and approved the submitted version.
Funding
The present study was supported by the Key Special Project for Introduced Talents Team of Southern Marine Science and Engineering Guangdong Laboratory (Guangzhou) (GML2019ZD0401, GML2019ZD0407), the Strategic Priority Research Program of the Chinese Academy of Sciences (No. XDA19060301), the National Science Foundation of China (No. 41976116, 41825013), the Youth Innovation Promotion Association CAS (2019337), and Guangdong Basic and Applied Basic Research Foundation (2021A1515011393) the Special Foundation for National Science and Technology Basic Research Program of China (2018FY100100), Science and Technology Planning Project of Guangdong Province, China (2020B1212060058).
Conflict of interest
The authors declare that the research was conducted in the absence of any commercial or financial relationships that could be construed as a potential conflict of interest.
Publisher’s note
All claims expressed in this article are solely those of the authors and do not necessarily represent those of their affiliated organizations, or those of the publisher, the editors and the reviewers. Any product that may be evaluated in this article, or claim that may be made by its manufacturer, is not guaranteed or endorsed by the publisher.
Supplementary material
The Supplementary Material for this article can be found online at: https://www.frontiersin.org/articles/10.3389/fendo.2022.923234/full#supplementary-material
Supplementary Figure 1 | Conserved synteny for the genomic region comprising VT and IT gene loci in teleosts. The gene organization and orientation in the genomic region containing the VT and IT genes were obtained from the Ensembl genome browser (http://www.ensembl.org).
References
1. Baribeau DA, Anagnostou E. Oxytocin and vasopressin: Linking pituitary neuropeptides and their receptors to social neurocircuits. Front Neurosci (2015) 9:335. doi: 10.3389/fnins.2015.00335
2. Gwee PC, Tay BH, Brenner S, Venkatesh B. Characterization of the neurohypophysial hormone gene loci in elephant shark and the Japanese lamprey: Origin of the vertebrate neurohypophysial hormone genes. BMC Evol Biol (2009) 9:47. doi: 10.1186/1471-2148-9-47
3. Banerjee P, Chaube R, Joy KP. Molecular cloning, sequencing and tissue expression of vasotocin and isotocin precursor genes from ostariophysian catfishes: Phylogeny and evolutionary considerations in teleosts. Front Neurosci (2015) 9:166. doi: 10.3389/fnins.2015.00166
4. Urano A, Ando H. Diversity of the hypothalamo-neurohypophysial system and its hormonal genes. Gen Comp Endocrinol (2011) 170:41–56. doi: 10.1016/j.ygcen.2010.09.016
5. Balment RJ, Lu W, Weybourne E, Warne JM. Arginine vasotocin a key hormone in fish physiology and behaviour: A review with insights from mammalian models. Gen Comp Endocrinol (2006) 147:9–16. doi: 10.1016/j.ygcen.2005.12.022
6. Thompson RR, Walton JC. Peptide effects on social behavior: Effects of vasotocin and isotocin on social approach behavior in Male goldfish (Carassius auratus). Behav Neurosci (2004) 118:620–6. doi: 10.1037/0735-7044.118.3.620
7. Godwin J, Thompson R. Nonapeptides and social behavior in fishes. Hormones Behav (2012) 61:230–8. doi: 10.1016/j.yhbeh.2011.12.016
8. Munakata A, Kobayashi M. Endocrine control of sexual behavior in teleost fish. Gen Comp Endocrinol (2010) 165:456–68. doi: 10.1016/j.ygcen.2009.04.011
9. Lyu LK, Li JS, Wang XJ, Yao YJ, Li JF, Li Y, et al. Arg-vasotocin directly activates isotocin receptors and induces COX2 expression in ovoviviparous guppies. Front Endocrinol (Lausanne) (2021) 12:617580. doi: 10.3389/fendo.2021.617580
10. Muchlisin ZA, Arfandi G, Adlim M, Fadli N, Sugianto S. Induced spawning of seurukan fish, Osteochilus vittatus (Pisces: Cyprinidae) using ovaprim, oxytocin and chicken pituitary gland extracts. Aacl Bioflux (2014) 7:412–8.
11. Pickford GE, Strecker EL. The spawning reflex response of the killifish, Fundulus heteroclitus: Isotocin is relatively inactive in comparison with arginine vasotocin. Gen Comp Endocrinol (1977) 32:132–7. doi: 10.1016/0016-6480(77)90143-5
12. Salek SJ, Sullivan CV, Godwin J. Arginine vasotocin effects on courtship behavior in Male white perch (Morone americana). Behav Brain Res (2002) 133:177–83. doi: 10.1016/S0166-4328(02)00003-7
13. Backstrom T, Winberg S. Arginine-vasotocin influence on aggressive behavior and dominance in rainbow trout. Physiol Behav (2009) 96:470–5. doi: 10.1016/j.physbeh.2008.11.013
14. Joy KP, Chaube R. Vasotocin–a new player in the control of oocyte maturation and ovulation in fish. Gen Comp Endocrinol (2015) 221:54–63. doi: 10.1016/j.ygcen.2015.02.013
15. Venkatesh B, Tan CH, Lam TJ. Prostaglandins and teleost neurohypophyseal hormones induce premature parturition in the guppy, Poecilia reticulata. Gen Comp Endocrinol (1992) 87:28–32. doi: 10.1016/0016-6480(92)90146-B
16. Scobell SK, MacKenzie DS. Reproductive endocrinology of syngnathidae. J Fish Biol (2011) 78:1662–80. doi: 10.1111/j.1095-8649.2011.02994.x
17. Stolting KN, Wilson AB. Male Pregnancy in seahorses and pipefish: Beyond the mammalian model. BioEssays News Rev molecular Cell Dev Biol (2007) 29:884–96. doi: 10.1002/bies.20626
18. Whittington CM, Friesen CR. The evolution and physiology of Male pregnancy in syngnathid fishes. Biol Rev Cambridge Philos Soc (2020) 95:1252–72. doi: 10.1111/brv.12607
19. Lin Q, Fan S, Zhang Y, Xu M, Zhang H, Yang Y, et al. The seahorse genome and the evolution of its specialized morphology. Nature (2016) 540:395–9. doi: 10.1038/nature20595
20. Lin Q, Qiu Y, Gu R, Xu M, Li J, Bian C, et al. Draft genome of the lined seahorse, Hippocampus erectus. GigaScience (2017) 6:1–6. doi: 10.1093/gigascience/gix030
21. Tamura K, Stecher G, Peterson D, Filipski A, Kumar S. MEGA6: Molecular evolutionary genetics analysis version 6.0. Mol Biol Evol (2013) 30:2725–9. doi: 10.1093/molbev/mst197
22. Zhang H, Zhang B, Qin G, Li S, Lin Q. The roles of the kisspeptin system in the reproductive physiology of the lined seahorse (Hippocampus erectus), an ovoviviparous fish with Male pregnancy. Front Neurosci (2018) 12:940. doi: 10.3389/fnins.2018.00940
23. Livak KJ, Schmittgen TD. Analysis of relative gene expression data using real-time quantitative PCR and the 2(T)(-delta delta c) method. Methods (2001) 25:402–8. doi: 10.1006/meth.2001.1262
24. Banerjee P, Chaube R, Joy KP. Molecular cloning and characterisation of an isotocin paralogue ([V8] isotocin) in catfishes (Superorder ostariophysi): Origin traced likely to the fish-specific whole genome duplication. J Neuroendocrinol (2018) 30:e12647. doi: 10.1111/jne.12647
25. Godwin J, Sawby R, Warner RR, Crews D, Grober MS. Hypothalamic arginine vasotocin mRNA abundance variation across sexes and with sex change in a coral reef fish. Brain Behav Evol (2000) 55:77–84. doi: 10.1159/000006643
26. Ramallo MR, Grober M, Canepa MM, Morandini L, Pandolfi M. Arginine-vasotocin expression and participation in reproduction and social behavior in males of the cichlid fish Cichlasoma dimerus. Gen Comp Endocrinol (2012) 179:221–31. doi: 10.1016/j.ygcen.2012.08.015
27. Nagarajan G, Aruna A, Chang CF. Neuropeptide arginine vasotocin positively affects neurosteroidogenesis in the early brain of grouper, Epinephelus coioides. J Neuroendocrinol (2015) 27:718–36. doi: 10.1111/jne.12298
28. Pouso P, Cabana A, Goodson JL, Silva A. Preoptic area activation and vasotocin involvement in the reproductive behavior of a weakly pulse-type electric fish, Brachyhypopomus gauderio. Front Integr Neurosci (2019) 13:37. doi: 10.3389/fnint.2019.00037
29. Rodriguez-Santiago M, Nguyen J, Winton LS, Weitekamp CA, Hofmann HA. Arginine vasotocin preprohormone is expressed in surprising regions of the teleost forebrain. Front Endocrinol (2017) 8. doi: 10.3389/fendo.2017.00195
30. Altmieme Z, Jubouri M, Touma K, Cote G, Fonseca M, Julian T, et al. A reproductive role for the nonapeptides vasotocin and isotocin in Male zebrafish (Danio rerio). Comp Biochem Phys B (2019) 238:110333. doi: 10.1016/j.cbpb.2019.110333
31. Kagawa N, Nishiyama Y, Kato K, Takahashi H, Kobayashi Y, Sakamoto H, et al. Potential roles of arginine-vasotocin in the regulation of aggressive behavior in the mudskipper (Periophthalmus modestus). Gen Comp Endocrinol (2013) 194:257–63. doi: 10.1016/j.ygcen.2013.09.023
32. Eens M, Pinxten R. Sex-role reversal in vertebrates: Behavioural and endocrinological accounts. Behav Processes (2000) 51:135–47. doi: 10.1016/S0376-6357(00)00124-8
33. Lin T, Liu X, Xiao D, Zhang D. Plasma levels of immune factors and sex steroids in the Male seahorse Hippocampus erectus during a breeding cycle. Fish Physiol Biochem (2017) 43:889–99. doi: 10.1007/s10695-017-0343-6
34. Whittington CM, Griffith OW, Qi W, Thompson MB, Wilson AB. Seahorse brood pouch transcriptome reveals common genes associated with vertebrate pregnancy. Mol Biol Evol (2015) 32:3114–31. doi: 10.1093/molbev/msv177
Keywords: seahorse, vasotocin, male pregnancy, parturition, reproductive behavior
Citation: Zhang H, Liu Y, Qin G and Lin Q (2022) Identification of neurohypophysial hormones and the role of VT in the parturition of pregnant seahorses (Hippocampus erectus). Front. Endocrinol. 13:923234. doi: 10.3389/fendo.2022.923234
Received: 19 April 2022; Accepted: 06 July 2022;
Published: 29 July 2022.
Edited by:
Bin Wang, Yellow Sea Fisheries Research Institute (CAFS), ChinaReviewed by:
Paula Gabriela Vissio, University of Buenos Aires, ArgentinaAzadeh Hatef, University of Saskatchewan, Canada
Copyright © 2022 Zhang, Liu, Qin and Lin. This is an open-access article distributed under the terms of the Creative Commons Attribution License (CC BY). The use, distribution or reproduction in other forums is permitted, provided the original author(s) and the copyright owner(s) are credited and that the original publication in this journal is cited, in accordance with accepted academic practice. No use, distribution or reproduction is permitted which does not comply with these terms.
*Correspondence: Qiang Lin, bGlucWlhbmdAc2NzaW8uYWMuY24=