- 1Laboratory of Metabolic Research, Istituto Auxologico Italiano, Istituto di Ricovero e Cura a Carattere Scientifico (IRCCS), San Giuseppe Hospital, Piancavallo, Verbania, Italy
- 2Reference Center for Prader Willi Syndrome, Research Institute, Bambino Gesù Children’s Hospital, Rome, Italy
- 3Division of Endocrinology, Department of Translational Medicine, University of Piemonte Orientale, Novara, Italy
- 4Division of Auxology, Istituto Auxologico Italiano, IRCCS, San Giuseppe Hospital, Piancavallo, Verbania, Italy
- 5Department of Clinical Sciences and Community Health, University of Milan, Milan, Italy
Irisin is a myokine involved in the browning of white adipose tissue and regulation of energy expenditure, glucose homeostasis and insulin sensitivity. Debated evidence exists on the metabolic role played by irisin in children with overweight or obesity, while few information exist in children with Prader Willi Syndrome (PWS), a condition genetically prone to obesity. Here we assessed serum irisin in relation to the metabolic profile and body composition in children and adolescents with and without PWS. In 25 PWS subjects [age 6.6-17.8y; body mass index standard deviation score (BMI SDS) 2.5 ± 0.3] and 25 age, and BMI-matched controls (age 6.8-18.0y; BMI SDS, 2.8 ± 0.1) we assessed irisin levels and metabolic profile inclusive of oral glucose tolerance test (OGTT), and body composition by dual-energy X-ray absorptiometry (DXA). In PWS, we recorded lower levels of fat-free mass (FFM) (p <0.05), fasting (p<0.0001) and 2h post-OGTT insulin (p<0.05) and lower insulin resistance as expressed by homeostatic model of insulin resistance (HOMA-IR) (p<0.0001). Irisin levels were significantly lower in PWS group than in controls with common obesity (p<0.05). In univariate correlation analysis, positive associations linked irisin to insulin OGTT0 (p<0.05), insulin OGTT120 (p<0.005), HOMA-IR (p<0.05) and fasting C-peptide (p<0.05). In stepwise multivariable regression analysis, irisin levels were independently predicted by insulin OGTT120. These results suggest a link between irisin levels and insulin sensitivity in two divergent models of obesity.
Introduction
Prader-Willi Syndrome (PWS) is a rare genetic disease caused by the lack of expression of paternal genes on chromosome 15q11.2-q13 (1) and it represents one of the most common forms of genetic obesity. The estimated incidence rate is 1 in 25,000 live births (2). Three main genetic mechanism have been detected in PWS: interstitial deletion of the proximal long arm of chromosome 15 (15q11-q13)(DEL15), maternal uniparental disomy of chromosome 15 (UPD15) and imprinting defects (3).
PWS is a complex multisystem disorder, characterized by infantile lethargy and muscle hypotonia followed by hyperphagia and excess weight gain during early child-hood, mildly dysmorphic acro-facial features, kyphoscoliosis, developmental delay with learning and behavioral problems, and a variable number of hypothalamo-pituitary disorders comprising impaired GH secretion with short stature, hypogonadism, hypothyroidism (1, 2, 4, 5). A typical feature of PWS is a lack of satiety due to hypothalamic dysfunction, which generates obsessive craving for food approximately by the age of 2 years and progresses to development of severe obesity in a large proportion of patients unless overeating is promptly controlled by caregivers (6).
With respect to subjects with common obesity, obese children with PWS display distinct phenotypic and metabolic features (1), consisting of higher fat mass (FM), lower fat-free mass (FFM) and impaired muscle function (7, 8). Despite this adverse phenotype, fasting insulin levels and insulin resistance are comparatively lower at all ages in obese patients with PWS (9–11). Furthermore, non-obese children with PWS showed lower insulin and glucose levels than their obese counterpart (12). When plotted against results obtained in non-PWS controls, reasons for higher insulin sensitivity in PWS include a modest accumulation of visceral fat after adjustment for total adiposity (13), an disproportionately elevated ghrelin concentration for the degree of obesity (8, 14), high levels of adiponectin (10) and an impaired GH secretion (15).
In the past few years, attention has focused on irisin, a myokine involved in the cross-talk between muscle and adipose tissue (16, 17) that has been proposed to play a role in the pathophysiology of obesity. Originally described in mice by Böstrom et al. (16), irisin is a 112 amino acid cleavage product of fibronectin type III domain-containing protein 5 (FDNC5), that was shown to stimulate the “browning” of white adipocytes, thus acting to increasing total body energy expenditure, reducing body weight, and reducing insulin resistance (16, 18). While irisin was first identified as a myokine secreted in response to exercise, recent studies in rodents and humans demonstrated that irisin is also expressed and secreted by white adipose tissue (WAT) (17), and there is emerging evidence that irisin could also act as an adipokine (17, 19, 20). As such, in the adult population circulating irisin is positively associated with body weight (21–24) and several measures of adiposity (22, 23), as well as muscle mass (20–22).
On the contrary, scanty and debated information exists on the role played by irisin in relation to pediatric obesity (25). While studies found a positive correlation between irisin and body mass index (BMI), waist circumference (WC) and fat-free mass (26–28), others documented a negative or a lack of correlation between irisin and adiposity measures (29–31). Conceivably, at this stage divergences may depend on variability in hormonal activity, muscle development and body fat accumulation in growing children (32, 33).
With regard to irisin and glucose metabolism, its role is not completely understood both in children and adults (18, 25). Irisin facilitates glucose uptake by skeletal muscle and adipose tissue (by increasing the expression of GLUT4) and improves hepatic glucose metabolism, increasing glycogenesis and reducing gluconeogenesis (18). Also, it contributes to β-islet cell survival and function (34). In obese children, irisin concentrations have been correlated to glucose and insulin levels as well as insulin resistance (27, 28,31, 35). Intriguingly, a cross-sectional study in obese children with and without insulin resistance recorded no difference in serum irisin levels (36), while a negative correlation was observed in healthy children between fasting glucose and irisin levels (37).
In PWS, circulating irisin level have only been assessed in a few studies (20, 38, 39). A previous study from our group in adult obese subjects with PWS reported significantly lower irisin levels in PWS than in controls with common obesity, while being similar to values recorded in lean subjects (20). In a recent study encompassing children and adults, Faienza et al. showed that serum irisin levels didnot differ between PWS and normal weight subjects but, interestingly, the authors found significantly lower irisin levels both in pediatric and adult PWS subjects carrying DEL15 as compared to controls (40).
Given this background, our study aimed to explore circulating irisin in relation to the metabolic profile and body composition in obese children and adolescents with and without PWS. Secondary aim of the study was to assess the predictors of irisin levels in our cohorts.
Materials and Methods
Patients
This study enrolled 50 patients, consisting of 25 PWS children and adolescents (16 M/9 F; age, 6.6-17.8y; BMI SDS, 2.5 ± 0.3) and 25 age, gender and BMI-matched control subjects (11 M/14 F; age, 6.8-18.0y; BMI SDS, 2.8 ± 0.1). PWS subjects were referred to the Center for Prader-Willi Syndrome of the Bambino Gesù Children’s Hospital in Rome, Italy. All PWS individuals received a diagnosis based on typical syndromic features confirmed by molecular genetic studies of chromosome 15, including 15q11-q13 deletion in 15 (8 males and 7 females) and UPD15 in the remaining 10 patients (8 males and 2 females).
With respect to hormone replacement therapy, 16 patients with PWS were treated with growth hormone, while 1 PWS patient and one control subject were treated with levothyroxine. For all study participants, exclusion criteria included previously known liver disease, kidney failure, autoimmune diseases, uncontrolled hypothyroidism and/or diabetes mellitus, chronic exposure to anti-inflammatory steroids. Alcohol consumption was investigated, and none was an alcohol drinker. The study design was conformed to the ethical guidelines of the Declaration of Helsinki (1975) and was firstly approved by the Ethical Research Committees of the Bambino Gesù Children’s Hospital (protocol 2092/2020 approved 25 march 2020).
Written informed consent was obtained from all participants by their parents, and from patients, when appropriate. The study protocol was conformed to the guidelines of the European Convention on Human Rights and Biomedicine concerning biomedical research.
Body Measurements
Weight and height were measured to the nearest 0.1 kg and 0.1 cm, respectively, using standard methods. Waist circumference (WC) was measured midway between the lowest rib and the top of the iliac crest after expiration; hip measurements were taken at the greatest circumference around the nates. BMI was calculated as weight in kg divided by the square of the height in meters and expressed as standard deviation score, and BMI SDS was calculated according to BMI reference tables (41). The BMI cut-of point of ≥ 2 SDS was used to define obesity (41). Pubertal development was assessed according to Tanner’s criteria (42). A dual-energy X-ray absorptiometry using a Hologic QDR Discovery, and the APEX-system software version 13.3 (Hologic Bedford, MA) with fan beam in array mode was performed by the same operator. Quality control scans were performed daily using a simulated L1-4 lumbar spine phantom. The measurements were performed by using standard positioning techniques. Total body scans were obtained to estimate Fat Mass (FM%), Fat Free Mass (FFM%) expressed as percentage of total body weight (total body less head, with the skull excluded from analysisis). Trunk Fat (TFM), and FFM/FM ratios were also calculated. Coefficient of variation were between 0.3285 to 1.038%.
Metabolic Studies
Glucose homeostasis was evaluated by fasting glucose levels, oral glucose tolerance test (OGTT)-derived glucose and insulin levels at time 0 and 120 min, and glycated hemoglobin (HbA1c) levels in all subjects. Glucose tolerance was assessed according to ADA guidelines for children and adolescents (43). Insulin resistance was calculated by the homeostatic model of insulin resistance (HOMA-IR) index: insulin (mIU/L) ×[glucose (mmol/L)/22.5] (44). Blood samples were drawn at 08.00 a.m. under 12 hours fasting conditions then vials were centrifuged, and sera were stored at − 80°C until requested. Serum irisin levels were assessed using a commercially available human ELISA kit EK-067–29 (Phoenix Pharmaceutics, Inc, Burlingame, CA, USA) in accordance with the manufacturer’s instructions. This ELISA is specific for human irisin, and quality controls were included in all ELISA measurements with the results falling within the expected range. All samples were analyzed in duplicate. Intra-assay and inter-assay coefficients of variation (CV) of irisin immunoassays were less than 10% and 15% respectively, and minimum detectable concentration was 1.5 ng/mL. Serum leptin concentrations were quantified using a commercially available ELISA kit (Mediagnost GmbH, Reutlingen, Germany) with overall inter- and intra-assay CVs of 6.8–8.3% and 5.5–6.9% respectively.
Serum adiponectin levels were determined by an enzyme linked immunosorbent assay (DRG Instruments GmbH, Marburg, Germany), the detection limit was 1.56–100 ng/ml, sensitivity was 0.2 ng/ml, inter- and intra-assay CV was 2.4–8.4 and 0.9–7.4%, respectively.
Routine laboratory data included levels of aspartate aminotransferase (AST), alanine aminotransferase (ALT), gamma-glutamyl transpeptidase (GGT), glucose, total cholesterol (CHO), high-density (HDL) and low-density lipoprotein (LDL) cholesterol, triglycerides (TG) and HbA1c, measured by enzymatic methods (Roche Diagnostics, Mannheim, Germany). Levels of insulin were measured using a Cobas Integra 800 Autoanalyzer (Roche Diagnostics, Indianapolis, IN, USA). A two-site, solid-phase chemiluminescent immunometric assay or competitive immunoassay (Immulite 2000 Analyzer; DPC, Los Angeles, CA) was used to determine C-peptide levels.
Statistical Analysis
Statistical analysis was performed using SPSS version 21 (Somers, NY, USA). Values are expressed as means ± standard error mean (SEM). Data points not normally distributed obtained by the Shapiro–Wilk test were log-transformed to improve the symmetry and homoscedasticity of the distribution. For comparative analysis, ANOVA between the 2 groups was used. Pearson’s correlation analysis was used to identify significant associations between variables of interest. Stepwise multivariable regression analysis was used to evaluate the independent association of irisin with metabolic, anthropometric or biochemical parameters.
Two multilinear models were built which included the obese phenotype (common obesity = 0; PWS = 1) in association with parameters of body composition and metabolism (model 1: group, BMI SDs; Glucose OGTT0, Glucose OGTT120, Insulin OGGT0, Insulin OGTT120 C-Peptide, HOMA-IR; leptin, adiponectin; model 2: group, BMI SDS, Glucose OGTT0, Glucose OGTT120, Insulin OGTT0, C-Peptide, HOMA-IR, leptin adiponectin; with the exclusion of predictor from the previous model: Insulin OGTT120). β coefficients and significance values obtained from the regression models are reported. A p value < 0.05 was considered as statistically significant.
Results
A summary of anthropometric and biochemical data is reported in Tables 1, 2. BMI standard deviation score (SDS) values were comparable between the two groups and ranged, collectively, between 1.1 and 5.7. Within the PWS group, 14 out of 25 were obese (BMI > 2 SDS) and, overall, 15 were prepubertal and 10 were postpubertal. Among controls, 22 out of 25 were obese and, overall, 14 were prepubertal and 11 were postpubertal. No difference in age and gender distribution were observed between groups.
Following OGTT, abnormal glucose metabolism was detected in 2 children with PWS (8%) and 4 controls (16%): impaired fasting glucose was found in 1 subject of the control group and no patient with PWS while impaired glucose tolerance was found in 2 patients with PWS and 3 controls. No cases of type 2 diabetes mellitus (T2DM) were diagnosed in either group.
Anthropometric parameters differed between groups, and subjects with PWS showed lower values of FFM (p <0.05), WC (p <0.005) and waist-to-hip ratio (p<0.05) with respect to controls.
Children and adolescents with PWS harbored lower insulin OGTT0 (p <0.0001) and insulin OGTT120 levels (p<0.05) than controls. PWS group also showed lower fasting C-peptide levels (p<0.0001), lower insulin resistance expressed as HOMA-IR (p<0.0001) and higher adiponectin levels (p<0.005) compared to controls (Table 2). There were no differences in lipids and liver enzymes between populations.
Analysis of circulating irisin showed measurable levels in all cases. As illustrated in Figure 1, the distribution of irisin levels was somewhat more dispersed around the mean in patients with PWS than controls, with the former exhibiting lower concentrations of irisin. When only obese subjects were compared, mean circulating irisin was also significantly lower in the subgroups of obese PWS patients that in obese controls (21.8 ± 1.6 vs 25.9 ± 1.1 ng/ml, p<0.05). In more detailed analysis, irisin levels of PWS patients with DEL15 were significantly reduced compared with controls with common obesity (21.8 ± 0.8 ng/ml and 25.8 ± 1.1 ng/ml, p=0.018) while PWS patients with UPD15 did not show significant differences compared with controls (23.3 ± 1.0 ng/ml and 25.7 8 ± 1.1 ng/ml, p=0.2).
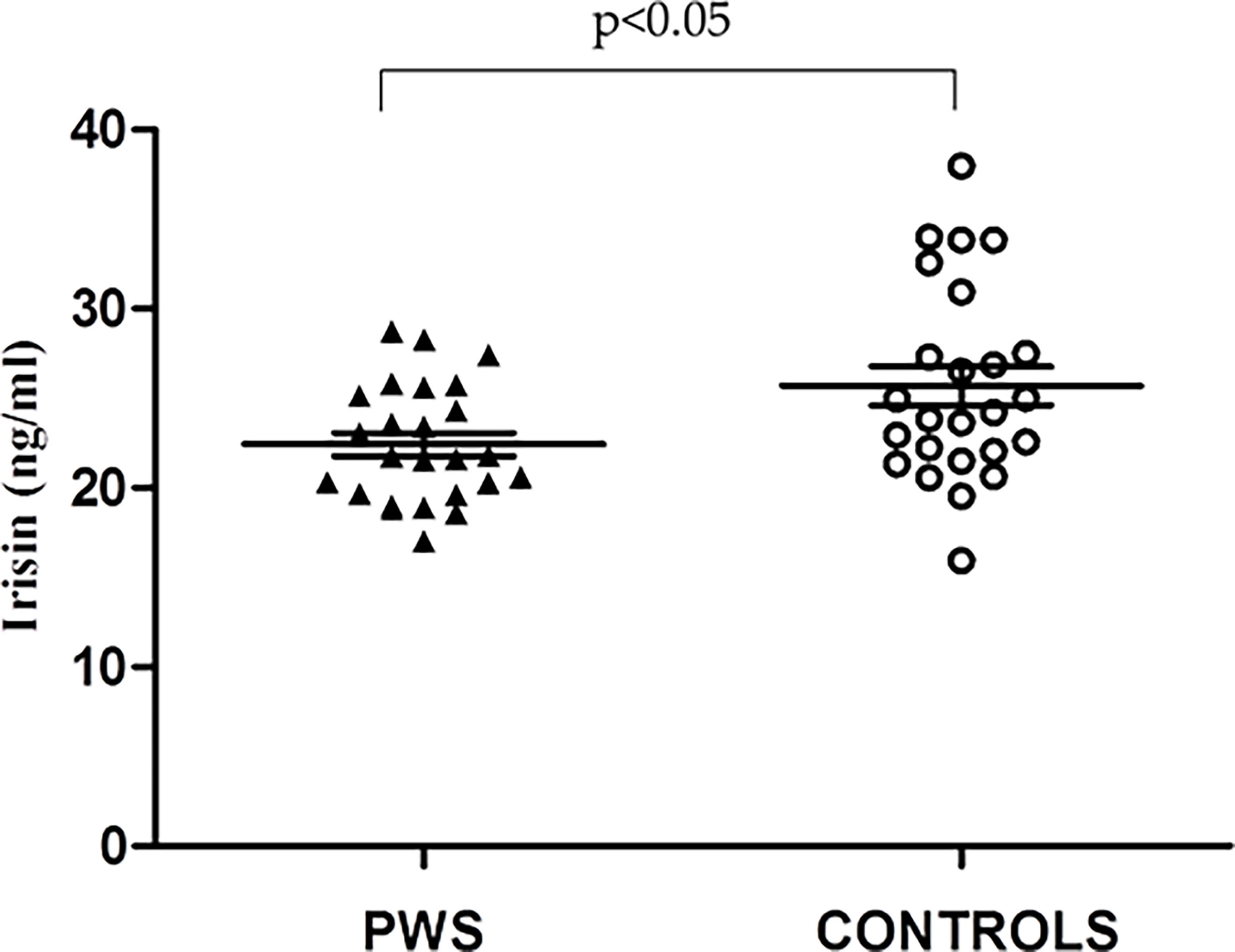
Figure 1 Individual values of circulating irisin levels obtained in Prader Willi Syndrome (PWS) patient (black triangles) and controls (white circles). Lines represent mean ± standard error of the mean (SEM) values.
In gender-based analysis, irisin levels did not differ between males and females in PWS (22.08 ± 0.8 and 23.07 ± 1.1 ng/ml, p =0.5) and controls (27.1 ± 2.4 and 24.0 ± 1.4 ng/ml p=0.07). Analysis by pubertal stage showed no difference in irisin levels between prepubertal and postpubertalsubjects both in the PWS (22.1 ± 0.8 vs 22.9 ± 1.1, p=0.5) and control group (26.4 ± 1.5 vs 24.9 ± 1.1, p=0.5).
Correlation analysis in separate groups showed no significant association between irisin and the study variables. In merged datasets (Table 3), positive associations linked irisin to PWS status, insulin OGTT0 and insulin OGTT120 levels, HOMA-IR and fasting C-peptide levels.
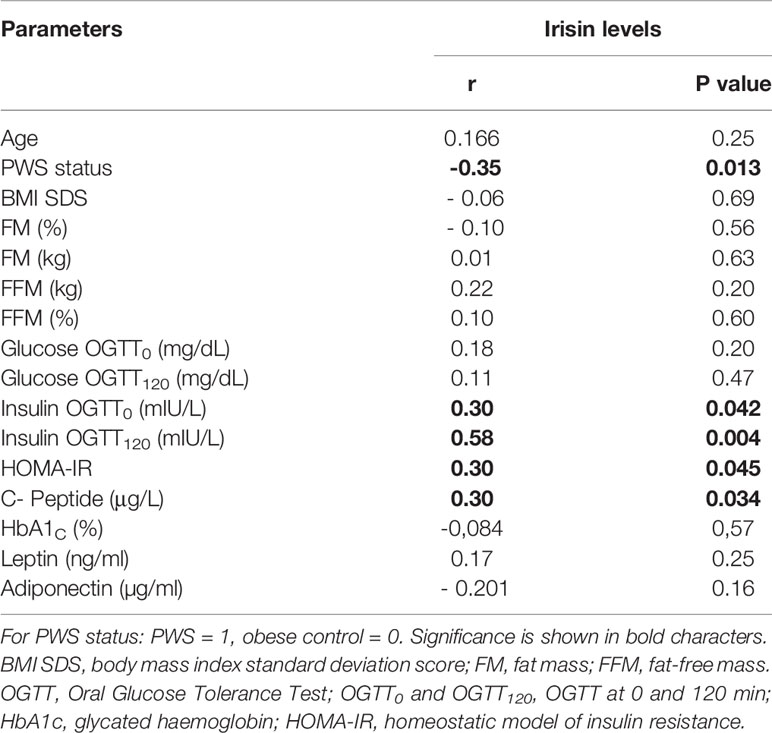
Table 3 Pearson’s correlation analysis between irisin levels and anthropometric and biochemical parameters in the study populations as a whole.
After controlling for age, sex and BMI SDS, correlation remained significant between irisin and insulin OGTT0 (r=0.345, p<0.05), insulin OGTT120 (r=0.622, p<0.005), HOMA-IR (r= 0.338, p<0.05) and C-peptide (r=0.325, p<0.05). When controlled for groups (PWS and non-PWS), only the correlation between irisin and insulin OGTT120 was maintained (r = 0.45, p= 0.041).
Stepwise multivariable regression analysis was performed in the two groups as a whole, and a number of models were explored to analyze the predictors of irisin levels in the study populations. As shown in Table 4, analysis documented that irisin levels were only predicted by insulin OGTT120 levels (β =0.58, p=0.005). After its removal from the regression equation, the PWS group acted as independent negative predictor of irisin levels (β =-0.35, p=0.025). No variable entered the regression equation in analyses on separate groups.
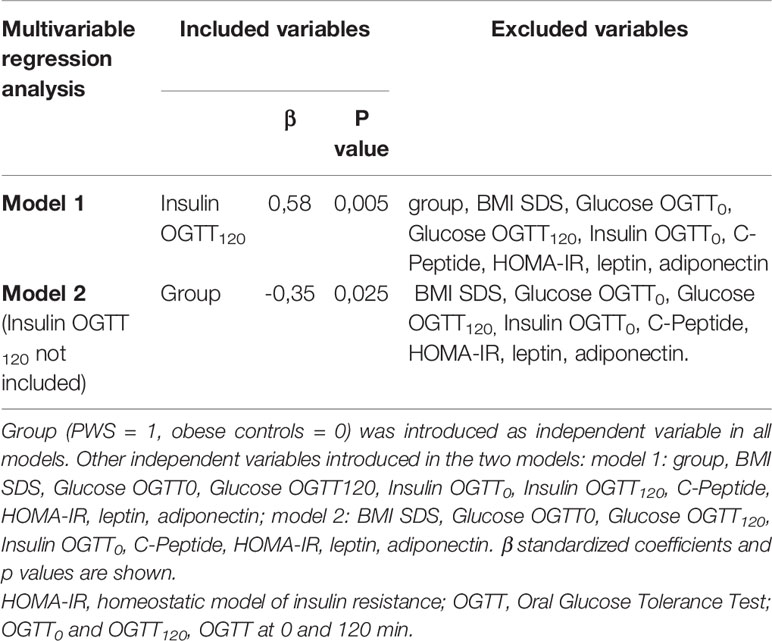
Table 4 Regression coefficients derived from the stepwise multivariable regression analysis conducted in the PWS subjects and obese controls as a whole on irisin as the dependent variable.
Discussion
The present study analyzed circulating irisin levels in children and adolescents with and without PWS in relation to metabolic profile and body composition. Current results show that irisin levels are lower in PWS than in controls independent of BMI, and that a strong association links irisin to measures of insulin resistance, particularly insulin OGTT120 levels.
It is well known that clinical features of PWS include muscle hypotonia, endocrine disturbances, childhood-onset obesity, and peripheral adiposity (4).
Although this adverse phenotype, obese children and adolescents with PWS have a milder metabolic derangement compared to their BMI-matched controls (11, 45, 46). In particular, PWS children show lower insulin and C-peptide levels, lower insulin resistance, as well as higher adiponectin and HDL cholesterol levels (47). Our study confirmed these peculiarities: in patients with PWS, in fact, we found significantly lower fasting and post-OGTT insulin levels, C-peptide and HOMA-IR together with higher levels of adiponectin with respect to controls matched for BMI SDS, confirming better insulin sensitivity. In addition, HDL cholesterol levels in PWS patients appear to be slightly higher, also not significantly, than in controls.
Irisin, a myokine that induces the browning of WAT and regulates the transcription of thermogenic genes (16), has recently attracted attention for its potential role in obesity and metabolic syndrome via regulation of WAT accumulation and body weight control (48).
In children, the direction of the relationship between irisin levels and obesity is debated (25), with some authors reporting unaltered or even decreased irisin levels (28, 48), and others showing higher irisin levels in obese children as compared with healthy controls (28, 35, 49). Potential reasons for such discrepancy involve the role of peripubertal development on the interplay between fat mass, muscle, and fat/muscle mass ratio (25), as well as the potential for irisin to be secreted as a myokine in condition of healthy body weight (16, 50, 51) and as an adipokine in condition of fat accumulation (17, 20, 23).
In PWS, irisin has been primarily studied in adults and its levels have been reported to be lower than in BMI-matched obese controls, while being similar to those observed in normal weight subjects (20, 38, 39). In our population, we found that obese PWS children had lower irisin levels than controls with common obesity, a finding that parallels our previous results in PWS adults (20). Although our study confirmed significant differences in lean mass even in young PWS subjects as compared to their control counterpart, the lack of relationship between irisin and lean mass suggests the negligible role for the latter to explain differences in circulating irisin between groups. In a gender based analysis, irisin concentrations did not appear to differ between genders both in PWS and controls. While previous studies in children found higher irisin levels in girls, either lean or obese, than boys (22, 30), this difference has not been confirmed in subsequent studies in overweight or obese children (26, 35, 37). Analysis by the pubertal stage showed no difference in irisin levels between prepubertal and postpubertal children with PWS and in controls, which agrees with results from previous studies (28, 52). In particular, pediatric PWS with DEL15 have significantly reduced levels of irisin compared with controls with common obesity. Our results obtained in a young and homogenous populations, confirm previous finding by Faienza at al., who highlighted a potential role for PWS genotype, particularly DEL15 genotype, in decreasing irisin levels significantly in comparison to controls (40). Also, it is to be noted that a previous study showed that different genetic subtypes in PWS had also different endocrine characteristics, for instance growth hormone secretion (15).
Noteworthy, irisin partakes in the regulation of glucose homeostasis and insulin sensitivity by promoting glucose uptake and glycogenolysis, and by reducing gluconeogenesis (18, 34). In children and adolescents, many but not all authors described positive associations between irisin and insulin or glucose levels, and insulin resistance (26,31, 35, 53). In PWS and non-PWS children, our results support evidence of a significant positive association between circulating irisin level and several measures of insulin metabolism, including levels of levels and post-OGTT insulin, fasting peptide-C, and HOMA-IR and expand to the pediatric age previous observations obtained in adults with PWS (20). Insulin OGGT120 was the only predictor of irisin levels in the groups as a whole, suggesting a tight and independent association between irisin and post-absorptive insulin secretion. Noticeably, this relationship remained significant even after adjustment for potential confounders such as age, gender, and BMI, confirming previous similar observations (54). Based on evidence that irisin acts as an insulin-sensitizing hormone, facilitates liver and muscle glucose metabolism, and promotes β-cell survival (55), it is possible that irisin reflects a compensatory response to insulin resistance and initial deterioration of glucose tolerance. As such, divergences in irisin between PWS and non-PWS subjects reflect differences in insulin sensitivity, as confirmed by divergences in levels of adiponectin, an adipokine known for its insulin-sensitizing effects (10). Further, Reinher and coworkers reported higher irisin level in obese children with impaired glucose tolerance compared to obese children with normal glucose tolerance and normal-weight children (31). This implies that the lower irisin levels seen in PWS reflects their healthier glucose homeostasis as compared to obese controls, while the association documented between irisin and post-OGTT insulin levels could reflect its association with hyperinsulineamia and insulin resistance. Alternatively, Sesti et al. hypothesized that a decrease in insulin clearance could be the mechanism underlying higher irisin levels in individuals with increasing post-OGTT insulin levels (54), in the attempt to reduce β-cell stress due to increasing insulin resistance (53). Whether these mechanisms are responsible for current observations in children with and without PWS warrant further investigation. Together, we hypothesize that irisin is an active participant in the regulation of insulin resistance rather than an innocent by-stander. It is conceivable that irisin secretion increases in obesity to maximize energy usage and glucose homeostasis, so as to achieve metabolic balance and compensate for the underlying irisin resistance (18, 56).
Some limitations should be acknowledged in our study. First, it was based on cross-sectional analysis and so it is not possible to provide information on changes in irisin levels possibly linked to modifications of clinical parameters such as weight and metabolic balance, therefore, no conclusion regarding cause–effect relationships can be made. The HOMA model is only an assessment of insulin resistance. Clamp studies are actually the “gold standard” for analyzing insulin resistance. However, validation studies demonstrated a good correlation between HOMA and clamp techniques in young participants (57).
Notwithstanding these limitations, this study enrolled a consistent sample of PWS children that were extensively characterized in terms of anthropometric, clinical and metabolic profiling. Also, irisin assessment was carried out with what is currently considered as the best available irisin immunoassay (18).
Together, our findings suggest that circulating irisin levels are lower in PWS compared to BMI-matched children possibly due to differences in body composition and insulin resistance. The potential influence of a genetic component associated with PWS cannot be, however, entirely excluded and needs further assessment.
Data Availability Statement
The raw data supporting the conclusions of this article will be made available by the authors, without undue reservation.
Ethics Statement
The studies involving human participants were reviewed and approved by Ethical Research Committees of the Bambino Gesù Children’s Hospital. Written informed consent to participate in this study was provided by the participants’ legal guardian/next of kin.
Author Contributions
Conceptualization, SM and PM; methodology, SM, RV, AlC, and SB; formal analysis, SM and PM; investigation, SM and DF; resources, DF, AnC, and MS; data curation, DF, SB, AlC; writing—original draft preparation, SM; writing—review and editing, DF, CM, MS, GG, GA, AnC, and PM; supervision, PM and MS. All authors have read and agreed to the published version of the manuscript.
Funding
Research funded by the Italian Ministry of Health.
Conflict of Interest
The authors declare that the research was conducted in the absence of any commercial or financial relationships that could be construed as a potential conflict of interest.
Publisher’s Note
All claims expressed in this article are solely those of the authors and do not necessarily represent those of their affiliated organizations, or those of the publisher, the editors and the reviewers. Any product that may be evaluated in this article, or claim that may be made by its manufacturer, is not guaranteed or endorsed by the publisher.
References
1. Cassidy SB, Driscoll DJ. Prader-Willi Syndrome. Eur J Hum Genet (2009) 17(1):3–13. doi: 10.1038/ejhg.2008.165
2. Tan Q, Orsso CE, Deehan EC, Triador L, Field CJ, Tun HM, et al. Current and Emerging Therapies for Managing Hyperphagia and Obesity in Prader-Willi Syndrome: A Narrative Review. Obes Rev (2020) 21(5):e12992. doi: 10.1111/obr.12992
3. Butler MG, Hartin SN, Hossain WA, Manzardo AM, Kimonis V, Dykens E, et al. Molecular Genetic Classification in Prader-Willi Syndrome: A Multisite Cohort Study. J Med Genet (2019) 56(3):149–53. doi: 10.1136/jmedgenet-2018-105301
4. Angulo MA, Butler MG, Cataletto ME. Prader-Willi Syndrome: A Review of Clinical, Genetic, and Endocrine Findings. J Endocrinol Invest (2015) 38(12):1249–63. doi: 10.1007/s40618-015-0312-9
5. Muscogiuri G, Barrea L, Faggiano F, Maiorino MI, Parrillo M, Pugliese G, et al. Obesity in Prader-Willi Syndrome: Physiopathological Mechanisms, Nutritional and Pharmacological Approaches. J Endocrinol Invest (2021) 44(10):2057–70. doi: 10.1007/s40618-021-01574-9
6. Tauber M, Hoybye C. Endocrine Disorders in Prader-Willi Syndrome: A Model to Understand and Treat Hypothalamic Dysfunction. Lancet Diabetes Endocrinol (2021) 9(4):235–46. doi: 10.1016/S2213-8587(21)00002-4
7. Reus L, Zwarts M, van Vlimmeren LA, Willemsen MA, Otten BJ, Nijhuis-van der Sanden MWG. Motor Problems in Prader-Willi Syndrome: A Systematic Review on Body Composition and Neuromuscular Functioning. Neurosci Biobehav Rev (2011) 35(3):956–69. doi: 10.1016/j.neubiorev.2010.10.015
8. Irizarry KA, Miller M, Freemark M, Haqq AM. Prader Willi Syndrome: Genetics, Metabolomics, Hormonal Function, and New Approaches to Therapy. Adv Pediatr (2016) 63(1):47–77. doi: 10.1016/j.yapd.2016.04.005
9. Haqq AM, Muehlbauer MJ, Newgard CB, Grambow S, Freemark M. The Metabolic Phenotype of Prader-Willi Syndrome (PWS) in Childhood: Heightened Insulin Sensitivity Relative to Body Mass Index. J Clin Endocrinol Metab (2011) 96(1):E225–232. doi: 10.1210/jc.2010-1733
10. Lacroix D, Moutel S, Coupaye M, Huvenne H, Faucher P, Pelloux V, et al. Metabolic and Adipose Tissue Signatures in Adults With Prader-Willi Syndrome: A Model of Extreme Adiposity. J Clin Endocrinol Metab (2015) 100(3):850–9. doi: 10.1210/jc.2014-3127
11. Crinò A, Grugni G. Update on Diabetes Mellitus and Glucose Metabolism Alterations in Prader-Willi Syndrome. Curr Diabetes Rep (2020) 20(2):7. doi: 10.1007/s11892-020-1284-5
12. Brambilla P, Crinò A, Bedogni G, Bosio L, Cappa M, Corrias A, et al. Metabolic Syndrome in Children With Prader-Willi Syndrome: The Effect of Obesity. Nutr Metab Cardiovasc Dis (2011) 21(4):269–76. doi: 10.1016/j.numecd.2009.10.004
13. Goldstone AP, Thomas EL, Brynes AE, Bell JD, Frost G, Saeed N, et al. Visceral Adipose Tissue and Metabolic Complications of Obesity are Reduced in Prader-Willi Syndrome Female Adults: Evidence for Novel Influences on Body Fat Distribution. J Clin Endocrinol Metab (2001) 86(9):4330–8. doi: 10.1210/jcem.86.9.7814
14. Tauber M, Coupaye M, Diene G, Molinas C, Valette M, Beauloye V. Prader-Willi Syndrome: A Model for Understanding the Ghrelin System. J Neuroendocrinol (2019) 31(7):e12728. doi: 10.1111/jne.12728
15. Grugni G, Marzullo P. Diagnosis and Treatment of GH Deficiency in Prader-Willi Syndrome. Best Pract Res Clin Endocrinol Metab (2016) 30(6):785–94. doi: 10.1016/j.beem.2016.11.003
16. Boström P, Wu J, Jedrychowski MP, Korde A, Ye L, Lo JC, et al. A PGC1-α-Dependent Myokine That Drives Brown-Fat-Like Development of White Fat and Thermogenesis. Nature (2012) 481(7382):463–8. doi: 10.1038/nature10777
17. Roca-Rivada A, Castelao C, Senin LL, Landrove MO, Baltar J, Belén Crujeiras A, et al. FNDC5/irisin is Not Only a Myokine But Also an Adipokine. PloS One (2013) 8(4):e60563. doi: 10.1371/journal.pone.0060563
18. Perakakis N, Triantafyllou GA, Fernández-Real JM, Huh JY, Park KH, Seufert J, et al. Physiology and Role of Irisin in Glucose Homeostasis. Nat Rev Endocrinol (2017) 13(6):324–37. doi: 10.1038/nrendo.2016.221
19. Rodríguez A, Becerril S, Ezquerro S, Méndez-Giménez L, Frühbeck G. Crosstalk Between Adipokines and Myokines in Fat Browning. Acta Physiol (Oxf) (2017) 219(2):362–81. doi: 10.1111/apha.12686
20. Mai S, Grugni G, Mele C, Vietti R, Vigna L, Sartorio A, et al. Irisin Levels in Genetic and Essential Obesity: Clues for a Potential Dual Role. Sci Rep (2020) 10(1):1020. doi: 10.1038/s41598-020-57855-5
21. Huh JY, Panagiotou G, Mougios V, Brinkoetter M, Vamvini MT, Schneider BE, et al. FNDC5 and Irisin in Humans: I. Predictors of Circulating Concentrations in Serum and Plasma and II. mRNA Expression and Circulating Concentrations in Response to Weight Loss and Exercise. Metab Clin Exp (2012) 61(12):1725–38. doi: 10.1016/j.metabol.2012.09.002
22. Löffler D, Müller U, Scheuermann K, Friebe D, Gesing J, Bielitz J, et al. Serum Irisin Levels are Regulated by Acute Strenuous Exercise. J Clin Endocrinol Metab (2015) 100(4):1289–99. doi: 10.1210/jc.2014-2932
23. Pardo M, Crujeiras AB, Amil M, Aguera Z, Jiménez-Murcia S, Baños R, et al. Association of Irisin With Fat Mass, Resting Energy Expenditure, and Daily Activity in Conditions of Extreme Body Mass Index. Int J Endocrinol (2014) 2014:857270. doi: 10.1155/2014/857270
24. Leung WKC, Yu AP, Lai CWK, Siu PM. Association of Markers of Proinflammatory Phenotype and Beige Adipogenesis With Metabolic Syndrome in Chinese Centrally Obese Adults. J Diabetes Res (2018) 2018:8956509. doi: 10.1155/2018/8956509
25. Elizondo-Montemayor L, Mendoza-Lara G, Gutierrez-DelBosque G, Peschard-Franco M, Nieblas B, Garcia-Rivas G. Relationship of Circulating Irisin With Body Composition, Physical Activity, and Cardiovascular and Metabolic Disorders in the Pediatric Population. Int J Mol Sci (2018) 19(12):E3727. doi: 10.3390/ijms19123727
26. Palacios-González B, Vadillo-Ortega F, Polo-Oteyza E, Sánchez T, Ancira-Moreno M, Romero-Hidalgo S, et al. Irisin Levels Before and After Physical Activity Among School-Age Children With Different BMI: A Direct Relation With Leptin. Obes (Silver Spring) (2015) 23(4):729–32. doi: 10.1002/oby.21029
27. Binay Ç, Paketçi C, Güzel S, Samancı N. Serum Irisin and Oxytocin Levels as Predictors of Metabolic Parameters in Obese Children. J Clin Res Pediatr Endocrinol (2017) 9(2):124–31. doi: 10.4274/jcrpe.3963
28. Jang HB, Kim HJ, Kang JH, Park SI, Park KH, Lee HJ. Association of Circulating Irisin Levels With Metabolic and Metabolite Profiles of Korean Adolescents. Metabolism (2017) 73:100–8. doi: 10.1016/j.metabol.2017.05.007
29. Shim YS, Kang MJ, Yang S, Hwang IT. Irisin is a Biomarker for Metabolic Syndrome in Prepubertal Children. Endocr J (2018) 65(1):23–31. doi: 10.1507/endocrj.EJ17-0260
30. Blüher S, Panagiotou G, Petroff D, Markert J, Wagner A, Klemm T, et al. Effects of a 1-Year Exercise and Lifestyle Intervention on Irisin, Adipokines, and Inflammatory Markers in Obese Children. Obes (Silver Spring) (2014) 22(7):1701–8. doi: 10.1002/oby.20739
31. Reinehr T, Elfers C, Lass N, Roth CL. Irisin and its Relation to Insulin Resistance and Puberty in Obese Children: A Longitudinal Analysis. J Clin Endocrinol Metab (2015) 100(5):2123–30. doi: 10.1210/jc.2015-1208
32. Moran A, Jacobs DR, Steinberger J, Hong CP, Prineas R, Luepker R, et al. Insulin Resistance During Puberty: Results From Clamp Studies in 357 Children. Diabetes (1999) 48(10):2039–44. doi: 10.2337/diabetes.48.10.2039
33. Guo B, Wu Q, Gong J, Xiao Z, Tang Y, Shang J, et al. Gender Difference in Body Fat for Healthy Chinese Children and Adolescents. Child Obes (2016) 12(2):144–54. doi: 10.1089/chi.2015.0139
34. Liu S, Du F, Li X, Wang M, Duan R, Zhang J, et al. Effects and Underlying Mechanisms of Irisin on the Proliferation and Apoptosis of Pancreatic β Cells. PloS One (2017) 12(4):e0175498. doi: 10.1371/journal.pone.0175498
35. Çatlı G, Küme T, Tuhan HÜ, Anık A, Çalan ÖG, Böber E, et al. Relation of Serum Irisin Level With Metabolic and Antropometric Parameters in Obese Children. J Diabetes Complications (2016) 30(8):1560–5. doi: 10.1016/j.jdiacomp.2016.07.019
36. Nigro E, Scudiero O, Ludovica Monaco M, Polito R, Schettino P, Grandone A, et al. Adiponectin Profile and Irisin Expression in Italian Obese Children: Association With Insulin-Resistance. Cytokine (2017) 94:8–13. doi: 10.1016/j.cyto.2016.12.018
37. Al-Daghri NM, Alkharfy KM, Rahman S, Amer OE, Vinodson B, Sabico S, et al. Irisin as a Predictor of Glucose Metabolism in Children: Sexually Dimorphic Effects. Eur J Clin Invest (2014) 44(2):119–24. doi: 10.1111/eci.12196
38. Hirsch HJ, Gross I, Pollak Y, Eldar-Geva T, Gross-Tsur V. Irisin and the Metabolic Phenotype of Adults With Prader-Willi Syndrome. PloS One (2015) 10(9):e0136864. doi: 10.1371/journal.pone.0136864
39. Hirsch HJ, Gross-Tsur V, Sabag Y, Nice S, Genstil L, Benarroch F, et al. Myokine Levels After Resistance Exercise in Young Adults With Prader-Willi Syndrome (PWS). Am J Med Genet A. (2020) 182(1):115–21. doi: 10.1002/ajmg.a.61391
40. Faienza MF, Brunetti G, Grugni G, Fintini D, Convertino A, Pignataro P, et al. The Genetic Background and Vitamin D Supplementation can Affect Irisin Levels in Prader-Willi Syndrome. J Endocrinol Invest (2021) 44(10):2261–71. doi: 10.1007/s40618-021-01533-4
41. Cole TJ, Bellizzi MC, Flegal KM, Dietz WH. Establishing a Standard Definition for Child Overweight and Obesity Worldwide: International Survey. BMJ (2000) 320(7244):1240–3. doi: 10.1136/bmj.320.7244.1240
42. Tanner JM, Whitehouse RH. Clinical Longitudinal Standards for Height, Weight, Height Velocity, Weight Velocity, and Stages of Puberty. Arch Dis Child (1976) 51(3):170–9. doi: 10.1136/adc.51.3.170
43. Arslanian S, Bacha F, Grey M, Marcus MD, White NH, Zeitler P. Evaluation and Management of Youth-Onset Type 2 Diabetes: A Position Statement by the American Diabetes Association. Diabetes Care (2018) 41(12):2648–68. doi: 10.2337/dci18-0052
44. Matthews DR, Hosker JP, Rudenski AS, Naylor BA, Treacher DF, Turner RC. Homeostasis Model Assessment: Insulin Resistance and Beta-Cell Function From Fasting Plasma Glucose and Insulin Concentrations in Man. Diabetologia (1985) 28(7):412–9. doi: 10.1007/BF00280883
45. Schuster DP, Osei K, Zipf WB. Characterization of Alterations in Glucose and Insulin Metabolism in Prader-Willi Subjects. Metabolism (1996) 45(12):1514–20. doi: 10.1016/S0026-0495(96)90181-X
46. McAlister KL, Fisher KL, Dumont-Driscoll MC, Rubin DA. The Relationship Between Metabolic Syndrome, Cytokines and Physical Activity in Obese Youth With and Without Prader-Willi Syndrome. J Pediatr Endocrinol Metab (2018) 31(8):837–45. doi: 10.1515/jpem-2017-0539
47. Mele C, Crinò A, Fintini D, Mai S, Convertino A, Bocchini S, et al. Angiopoietin-Like 8 (ANGPTL8) as a Potential Predictor of NAFLD in Paediatric Patients With Prader-Willi Syndrome. J Endocrinol Invest (2020) 44(7):1447–56. doi: 10.1007/s40618-020-01444-w
48. Waseem R, Shamsi A, Mohammad T, Hassan MI, Kazim SN, Chaudhary AA, et al. FNDC5/Irisin: Physiology and Pathophysiology. Molecules (2022) 27(3):1118. doi: 10.3390/molecules27031118
49. Dokumacioglu E, Iskender H, Sahin A, Erturk EY, Kaynar O. Serum Levels of Nesfatin-1 and Irisin in Obese Children. Eur Cytokine Netw (2020) 31(1):39–43. doi: 10.1684/ecn.2020.0444
50. Hofmann T, Elbelt U, Stengel A. Irisin as a Muscle-Derived Hormone Stimulating Thermogenesis–a Critical Update. Peptides (2014) 54:89–100. doi: 10.1016/j.peptides.2014.01.016
51. Zhang R, Fu T, Zhao X, Qiu Y, Hu X, Shi H, et al. Association of Circulating Irisin Levels With Adiposity and Glucose Metabolic Profiles in a Middle-Aged Chinese Population: A Cross-Sectional Study. Diabetes Metab Syndr Obes (2020) 13:4105–12. doi: 10.2147/DMSO.S275878
52. Taş D, Akman Öden A, Akgül S, Metin ZE, Pınar A, Kanbur N. The Effect of Pubertal Stage on the Concentrations of the Novel Adipomyokine, Irisin, in Male Adolescents. J Clin Res Pediatr Endocrinol (2020) 12(2):168–74. doi: 10.4274/jcrpe.galenos.2019.2019.0119
53. Mittelman SD, Van Citters GW, Kim SP, Davis DA, Dea MK, Hamilton-Wessler M, et al. Longitudinal Compensation for Fat-Induced Insulin Resistance Includes Reduced Insulin Clearance and Enhanced Beta-Cell Response. Diabetes (2000) 49(12):2116–25. doi: 10.2337/diabetes.49.12.2116
54. Sesti G, Andreozzi F, Fiorentino TV, Mannino GC, Sciacqua A, Marini MA, et al. High Circulating Irisin Levels are Associated With Insulin Resistance and Vascular Atherosclerosis in a Cohort of Nondiabetic Adult Subjects. Acta Diabetol (2014) 51(5):705–13. doi: 10.1007/s00592-014-0576-0
55. Marrano N, Biondi G, Borrelli A, Cignarelli A, Perrini S, Laviola L, et al. Irisin and Incretin Hormones: Similarities, Differences, and Implications in Type 2 Diabetes and Obesity. Biomolecules (2021) 11(2):286. doi: 10.3390/biom11020286
56. Arhire LI, Mihalache L, Covasa M. Irisin: A Hope in Understanding and Managing Obesity and Metabolic Syndrome. Front Endocrinol (Lausanne) (2019) 10:524. doi: 10.3389/fendo.2019.00524
Keywords: irisin, PWS, obesity, glucose metabolism, children, adolescents
Citation: Mai S, Fintini D, Mele C, Convertino A, Bocchini S, Grugni G, Aimaretti G, Vietti R, Scacchi M, Crinò A and Marzullo P (2022) Circulating Irisin in Children and Adolescents With Prader-Willi Syndrome: Relation With Glucose Metabolism. Front. Endocrinol. 13:918467. doi: 10.3389/fendo.2022.918467
Received: 12 April 2022; Accepted: 09 May 2022;
Published: 14 June 2022.
Edited by:
Marwan El Ghoch, Beirut Arab University, LebanonReviewed by:
Giuseppe Petito, University of Campania Luigi Vanvitelli, ItalyAndrea P. Rossi, Ca’ Foncello Hospital, Italy
Copyright © 2022 Mai, Fintini, Mele, Convertino, Bocchini, Grugni, Aimaretti, Vietti, Scacchi, Crinò and Marzullo. This is an open-access article distributed under the terms of the Creative Commons Attribution License (CC BY). The use, distribution or reproduction in other forums is permitted, provided the original author(s) and the copyright owner(s) are credited and that the original publication in this journal is cited, in accordance with accepted academic practice. No use, distribution or reproduction is permitted which does not comply with these terms.
*Correspondence: Stefania Mai, s.mai@auxologico.it