- 1Centre for Reproductive Health, Queen’s Medical Research Institute, The University of Edinburgh, Edinburgh, United Kingdom
- 2Department of Environmental Immunology, UFZ-Helmholtz Centre for Environmental Research Leipzig-Halle, Leipzig, Germany
Natural killer (NK) cells are the predominant maternal uterine immune cell component, and they densely populate uterine mucosa to promote key changes in the post-ovulatory endometrium and in early pregnancy. It is broadly accepted that (a) immature, inactive endometrial NK (eNK) cells in the pre-ovulatory endometrium become activated and transition into decidual NK (dNK) cells in the secretory stage, peri-implantation endometrium, and continue to mature into early pregnancy; and (b) that secretory-stage and early pregnancy dNK cells promote uterine vascular growth and mediate trophoblast invasion, but do not exert their killing function. However, this may be an overly simplistic view. Evidence of specific dNK functional killer roles, as well as opposing effects of dNK cells on the uterine vasculature before and after conception, indicates the presence of a transitory secretory-stage dNK cell (s-dNK) phenotype with a unique angiodevelopmental profile during the peri-implantation period, that is that is functionally distinct from the angiomodulatory dNK cells that promote vessel destabilisation and vascular cell apoptosis to facilitate uterine vascular changes in early pregnancy. It is possible that abnormal activation and differentiation into the proposed transitory s-dNK phenotype may have implications in uterine pathologies ranging from infertility to cancer, as well as downstream effects on dNK cell differentiation in early pregnancy. Further, dysregulated transition into the angiomodulatory dNK phenotype in early pregnancy will likely have potential repercussions for adverse pregnancy outcomes, since impaired dNK function is associated with several obstetric complications. A comprehensive understanding of the uterine NK cell temporal differentiation pathway may therefore have important translational potential due to likely NK phenotypic functional implications in a range of reproductive, obstetric, and gynaecological pathologies.
Introduction
The uterine mucosa undergoes dynamic cyclical tissue breakdown, regeneration and remodelling, leading to a carefully timed and defined period during which an embryo is able to attach and invade into a receptive uterus (1, 2). In the human endometrium, decidualisation is a dynamic, multistep progression of events, which begins in the secretory stage of the uterine cycle (luteal phase of the menstrual cycle) in response to rising ovarian steroid hormones (progesterone and estradiol) produced by the corpus luteum following ovulation, and is marked by the differentiation of fibroblast-like endometrial stromal cells (ESCs) into large, secretory, ‘decidual’ cells. Implantation of a conceptus takes place in the mid-secretory endometrium during a transient embryo-receptive period known as the ‘window of implantation’ (3). The induction of this receptive endometrial phenotype is reliant on an acute pro-inflammatory decidualisation initiation phase that leads to secretory and phenotypic changes in the uterine epithelium, and is also associated with the accumulation of maternal leukocytes in the endometrium and angiogenic growth of the spiral arteries (the terminating branches of the uterine arteries) in preparation for implantation (3, 4). Decidualisation is maintained after implantation, mainly under the influence of progesterone, and is required for the maintenance of pregnancy (3, 5, 6). In this review, we will focus on events in the human uterus, while referring, where appropriate, to relevant evidence from mouse models.
Innate lymphoid cells (ILCs; lymphocytes that do not express diverse antigen receptors) are the most prevalent leukocytes populating the uterine mucosa during the menstrual cycle and in early pregnancy. ILC subsets are categorised according to their cytokine profiles and transcription factor expression: ILC1s express T-bet and secrete IFN-γ; ILC2s express GATA-3 and secrete IL-5, IL-9 and IL-13; and ILC3s, which include a lymphoid tissue inducer-like subset, are defined by their expression of RORγt and secrete IL-17 and IL-22. Natural killer (NK) cells are known as key members of the ILC family, although it remains to be clarified whether tissue-resident NK cells in the uterine mucosa constitute a unique NK cell population or denote a population that includes ILC1s and ILC3s (7, 8). NK cells represent the predominant maternal immune cell component in the secretory stage endometrium and comprise up to 70% of leukocytes in first trimester decidua: they promote critical changes in the uterine microenvironment both during the ‘window of implantation’ and in early pregnancy (9–11), and uterine NK numbers subsequently diminish from mid-gestation to term (12).
Inactive NK cells proliferate and become activated in the decidualising secretory-stage endometrium, and then further expand in pregnancy (13, 14). Recent evidence also suggests that the uterine NK niche may be replenished from the circulation (15). While it was historically believed that the same NK cells that are activated during the secretory stage, expand and continue in pregnancy, evidence from several studies over the last decade indicate progressive NK cell differentiation within the uterine mucosa in response to the local environment during both endometrial regeneration and pregnancy (2, 15–17). However, it is still widely accepted that, despite their name, NK cells in the uterine mucosa do not kill, but are pro-angiogenic (18–22). Activated uterine NK cells do, nevertheless, live up to their name, and induce apoptosis of distinct cellular populations pre- and post-conception, to promote implantation, placentation and uterine vascular remodelling, thus ensuring pregnancy success (9, 10), despite producing several immunosuppressive molecules that may contribute to the establishment of maternal-fetal immune tolerance (23). Uterine NK cells are predominantly CD56brightCD16– (as opposed to peripheral blood NK cells, which are largely CD56dim/–CD16bright), and once activated in the secretory stage, produce cytotoxic proteins and thus have cytolytic capacity (21, 24), but do not cause cytotoxicity of healthy placental cells (22). Further, while secretory-stage dNK cells do indeed stimulate growth and development of the spiral arteries to ensure an adequately vascularised endometrium for implantation (20, 25), dNK cells in early pregnancy bestow destabilising and pro-apoptotic effects on vascular cells to initiate the spiral artery remodelling process requisite for increased vascular provision to the developing fetus (10, 11, 26–30). At this time, uterine NK cells also promote trophoblast invasion, which is when specialised placental-derived cells (trophoblasts) invade the decidua towards the spiral arteries in order to remodel them (10, 31). Further, pre- and post-conception uterine NK cells display different secretory profiles that support distinct uterine vascular processes (25, 26, 29). Thus, uterine NK cells exhibit divergent immune profiles and functions before and after pregnancy, that are both indirectly and directly influenced by the temporal endocrine adaptions within the local uterine tissue environment (9–11, 26, 29, 32–35). Indeed, a recent murine study investigating the early pregnancy uterine transcriptome has demonstrated that endocrine and paracrine regulators of immune responses and different cellular behaviours exhibit differential region-specific expression patterns within the uterus (36), further emphasising the contributory role of the local uterine microenvironment in mediating cellular phenotypic and functional profiles. Here, we describe the disparate functional profiles of NK cells in the uterine mucosa during the ‘window of implantation’ and in early pregnancy. These divergent NK characteristics indicate a pivotal but transitory angiodevelopmental uterine NK cell phenotype that also implements endometrial reconstruction in the peri-implantation endometrium; which is functionally distinct from the equally essential, angiomodulatory uterine NK cell phenotype in early pregnancy that induces vessel structure destablisation, vascular cell apoptosis and trophoblast invasion, to facilitate uterine vascular remodelling and placentation for successful pregnancy outcomes (Figure 1).
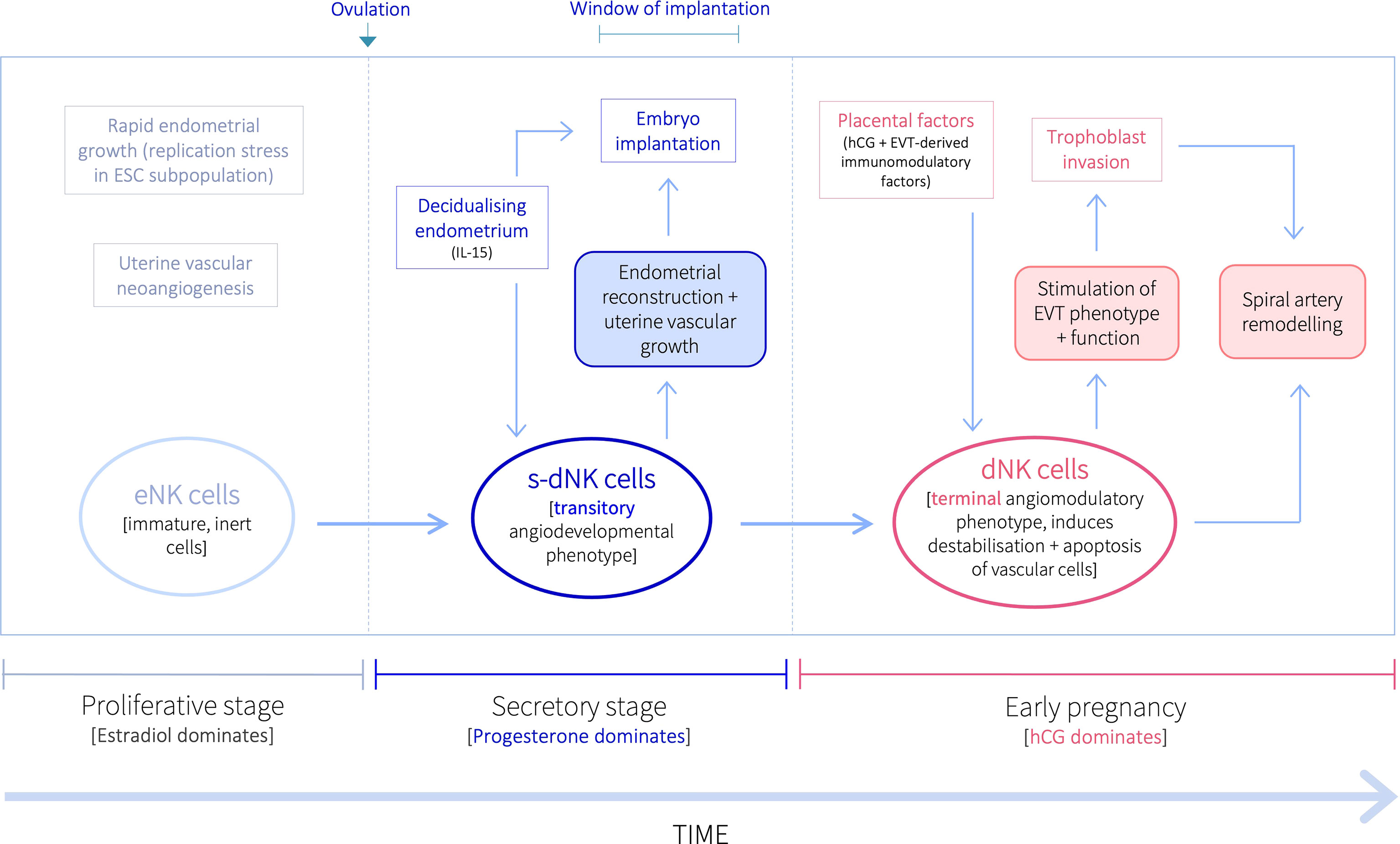
Figure 1 Proposed temporal natural killer cell differentiation pathway within the uterine mucosa. Following ovulation, progesterone upregulates the production of IL-15 by ESCs in the secretory stage endometrium decidualisation progresses. IL-15 stimulates the proliferation, activation, and maturation of inert eNK cells into a transitory angiodevelopmental s-dNK phenotype that induces uterine vascular growth and mediates endometrial reconstruction to facilitate embryo implantation. Following implantation, placental-derived hCG drives further NK proliferation, and EVT-derived immunomodulatory factors promote their differentiation into a terminal angiomodulatory dNK phenotype, with destabilising and pro-apoptotic effects on vascular cells and pro-invasive effects on EVT, to initiate the uterine vascular changes crucial for successful pregnancy outcomes.
Timing of NK Cell Proliferation in the Uterine Mucosa: Secretory Stage vs. Early Pregnancy Expansion
Temporally and physiologically distinct vascular processes during the secretory and early pregnancy are necessary to provide an adequate oxygen and nutrient supply to the placental unit, to meet the demands of a rapidly developing fetus. Successful implantation and establishment of pregnancy rely on uterine vascular development and expansion, whereby decidual capillaries and spiral arteries undergo vascular growth and development, in response to coordinated progesterone and estradiol secretion (20, 37, 38). Subsequently, in early pregnancy, the uterine spiral arteries must be remodelled into large-diameter, high-flow vessels with low resistance, facilitating an approximately 10-fold increase in blood supply into the intervillous space for placental uptake (39). These disparate vascular processes correspond to two separate bursts of uterine NK cell proliferation that are mediated by temporal endocrine and immunological adaptations within the uterine environment: there is a significant increase in number during the peri-implantation period in the secretory stage endometrium, followed by a further increase in early pregnancy (35, 37, 40).
Rapid proliferation of uterine NK cells is concurrent with secretory-stage ESC decidual transformation and growth of the uterine vasculature. Under the action of ovarian steroid hormones, an acute pro-inflammatory ESC response leads to the production of adhesion molecules in the overlying epithelium, which renders the uterus transiently receptive for embryo implantation (18, 41). During this time, the NK cells within the uterine mucosa undergo post-ovulatory phenotypic changes, and display distinctive gene and protein expression profiles, although neither the progesterone receptor (PR) nor estrogen receptors (ERs) are expressed by human uterine mucosal NK cells (32, 42, 43). Instead, the previously inert endometrial NK (eNK) cells are activated by ESC-derived interleukin 15 (IL-15) (35, 42): the mid-secretory endometrium begins to express high levels of IL-15, which is upregulated by progesterone as decidualisation progresses, and promotes their proliferation, activation, and maturation into decidual NK (dNK) cells (44–49). If there is no fertilisation, the corpus luteum will regress, resulting in progesterone (and estradiol) withdrawal, and in turn, IL-15 withdrawal. This leads to death of the activated dNK cells and subsequent decline of dNK-derived soluble factor products that maintain vascular integrity, thereby contributing to spiral artery constriction and endometrial ischemia, and ultimately causing ESC cell death and menses (40, 41, 50, 51). If pregnancy ensues, the uterine microenvironment remains abundantly rich in IL-15 throughout the first trimester of pregnancy, until placentation and uterine vascular remodelling are complete (33, 34). Subsequently, IL-15 levels decline, corresponding to waning dNK numbers from mid-gestation to term (12, 34).
The post-ovulatory dNK cell proliferative surge and activation is explained by increased IL-15 levels in the peri-implantation endometrium. However, the further dramatic dNK cell expansion in early pregnancy remained unexplained until it was demonstrated that extensive post-implantation dNK proliferation can be induced by human chorionic gonadotropin (hCG), one of the earliest proteins secreted by trophoblast cells (35). hCG is a heterodimeric glycoprotein with an identical alpha subunit to luteinizing hormone (LH), and hence binds the LH receptor (LHR); and both mature hCG and LH are modified by N-linked carbohydrate side chains that are important for the stability and assembly of the proteins, although the carbohydrate side chains between the two proteins are not identical (52, 53). Thus, while NK cells in uterine mucosa do not express the LHR, they do express the mannose receptor (MR; also known as CD206), a cell surface lectin that binds glycoproteins with N-linked carbohydrate side chains (54). The uterine NK cell MR can therefore recognise carbohydrate moieties in glycosylated proteins. As such, hCG-carbohydrate-side chain recognition by dNK-expressed MR may induce non-canonical hCG signalling to stimulate dNK proliferation in early pregnancy (35). However, since hCG and LH do not share identical N-linked carbohydrate side chains (53), the pre-ovulatory LH surge (which induces ovulation) is likely unable to promote eNK activation and maturation into dNK cells, as indicated by the lack of uterine NK proliferation reported upon LH stimulation in vitro (35). This is also consistent with the significant rise in secretory-stage uterine NK number occurring several days after ovulation, when decidualisation begins. The second surge in dNK cell number, which occurs during early pregnancy and placentation, corresponds to the exponentially rising hCG levels that peak at around 10 weeks of gestation (55); levels then decline until approximately 16th week of gestation and remain relatively constant thereafter until term (55), consistent with declining dNK numbers from mid-gestation to term (12). Notably, failing pregnancies have been associated with lower daily rates of increase in hCG, with low circulating hCG concentrations predictive of pregnancy loss (56). Interestingly, a unique dNK population has been identified in the decidua of multigravid women, termed pregnancy-trained decidual NKs (PTdNKs), whose activation may foster proper placentation, demonstrating the trained memory function of dNK cells in enhancing placentation (57).
NK Cells in the Secretory Stage Uterine Mucosa
Endometrial neoangiogenesis and rapid endometrial growth take place during the estradiol-mediated proliferative phase of the uterine cycle to regenerate the endometrium after menstruation; and following ovulation, rising levels of progesterone and estradiol initiate the decidualisation process during secretory stage of the uterine cycle (9, 37, 58). Decidualisation is characterised by differentiation of ESCs into specialised decidual cells, NK cell accumulation within the uterine mucosa, uterine vascular growth, and local edema (3, 4). A sufficiently decidualised stroma, with increased vascularisation of the endometrium, is essential for invasion of the blastocyst and embryo implantation (37). Successful implantation in humans also requires a receptive epithelium for initial attachment of the conceptus, which is reliant on a pro-inflammatory endometrial environment (59–64). The presence of NK cells in the endometrium prior to conception is unique to humans, and the functional profiles of secretory-stage dNK cells demonstrate their prominent roles in mediating endometrial reconstruction, promoting growth and development of the uterine vasculature, as well as contributing to an inflammatory endometrial phenotype that is essential for implantation (65).
Decidual transformation is not static, but a dynamic process that begins after ovulation, during the secretory stage of the uterine cycle. It encompasses three critical transitory phases: an acute pro-inflammatory decidualisation initiation phase, which subsequently transitions to an anti-inflammatory secretory phase during which time embryo implantation takes place, followed by a final resolution phase. The transient pro-inflammatory decidualisation initiation response that is crucial for the induction of endometrial receptivity, is accompanied by a surge of free radical production, and the secretion of various cytokines, growth factors and angiogenic factors (4, 61, 66, 67). However, the rapid pre-ovulatory endometrial proliferation (post-menstruation endometrial regeneration) prompts replication stress in a subpopulation of ESCs that are consequently unable to differentiate into specialised decidual cells during the decidualisation initiation phase, but instead undergo acute cellular senescence (a tightly coordinated biological process implicated in embryo development, wound healing and tissue repair) (9). These senescent ESCs, in turn, secrete a host of inflammatory mediators that signal to induce the production of epithelial adhesion molecules involved in endometrial receptivity. Acute decidual senescence, through its stimulation of an acute pro-inflammatory decidual phenotype, thus contributes to the induction of key receptivity gene expression in the overlying endometrial epithelium (9, 61), and may therefore be fundamental to the acquisition of a receptive endometrial phenotype. Concomitant systematic clearance of acutely senescent decidual cells, regulates endometrial reconstruction (rejuvenation and remodelling of ESCs) that is necessary ensure a mature decidual cell environment for embryo implantation. Secretory stage dNK cells are pivotal to this process, as they target and eliminate senescent cells through granule exocytosis. Senescent decidual ESC clearance by midsecretory-stage dNK cells, coincident with the ‘window of implantation’, thereby exerts a role in maintaining tissue homeostasis from cycle to cycle, and may directly assist embryo implantation in the presence of a conceptus (9). In addition, secretory stage dNK cells also release prokineticin 1 during the ‘window of implantation’, which regulates the expression of several implantation-related genes, and has therefore been proposed as a marker of endometrial receptivity (68, 69).
Peri-implantation growth and development of the spiral arteries (branching, elongation and vascular maturation) increases the uterine vascular surface area in preparation for pregnancy, and is prerequisite for implantation and placentation to proceed (37, 70, 71). Secretory-stage dNK cells produce a range of angiogenic mediators including vascular endothelial growth factor (VEGF)-A, VEGF-C, placental growth factor (PlGF), and angiopoietins 1 and 2 (25). The VEGF family constitutes seven distinct proteins, which include VEGF-A, VEGF-C and PlGF. VEGF-A is a key regulator of decidual angiogenesis and the maintenance of vascular integrity, and when genetically ablated in mice, causes embryonic lethality (20, 25, 72). VEGF-C has been characterised as a selective growth factor for lymphatic vessels and was found to affect the migration and proliferation of endothelial cells (25). VEGF signaling is mediated via interactions with two structurally related tyrosine kinase receptors, VEGFR-1/fms-like tyrosine kinase I (Flt-1) and VEGFR-2 (Flk-1) (73). Upregulation of VEGF-A and its cognate receptors that act on the uterine microvasculature during the secretory stage, may lead to increased vascularity and blood flow, in turn, enhancing the vascular conditions for implantation (74). PlGF is also heavily expressed in human pregnancies, binds to both Flt-1 and Flk-1, and promotes the further expression of angiogenic factors such as VEGF-A, basic fibroblast growth factor, platelet-derived growth factor beta, matrix metalloproteinases (MMPs) (20, 75). The release of VEGF and PlGF can be further triggered by NK-cell activating receptors NKp30 and NKp44, both of which are expressed by IL-15-activated dNK cells, while inert pre-ovulatory eNK cells lack NKp30 and NKp44 expression (13, 31). These receptors are also upregulated by prolactin (48), levels of which rise in the late secretory stage and are high throughout pregnancy (49). Angiopoietins can also work in collaboration with VEGFs, to control vascular growth and integrity, and can influence the stability of spiral arteries (25). Thus, peri-implantation dNK cells in the secretory stage uterine mucosa have an angiodevelopmental secretome, playing a central role in supporting growth and elongation of the uterine vasculature for subsequent remodelling into enlarged uteroplacental vessels (25, 70).
The profound changes that take place in the secretory stage endometrium are not only important for implantation success, but aberrant decidual transformation can cause endometrial functional inadequacy, which has been implicated in infertility/reproductive disorders, gynaecological disorders, female reproductive tract cancers, and several obstetric complications (4, 28, 62, 76–88). dNK cell functions undoubtedly play fundamental roles during the secretory stage, and any impairment of the immune-endocrine network (including NK cellular functions) at this time could lead to primary reproductive failure, or uterine pathologies such as endometriosis (characterised by the ectopic growth of endometrial tissue) and its associated infertility (69, 89). Further, phenotypic and functional impairment of uterine NK cells (rendering them unable to exert their classical anti-tumour actions) has been demonstrated in endometrial cancer, likely resulting from a dysregulated and imbalanced endocrine-immune network (19, 90).
Abnormal dNK cell activation and differentiation during the secretory stage can also directly interfere with implantation (69). Depending on the extent of dNK functional dysregulation, this may cause implantation failure, or contribute to anomalous elongation of the ‘window of implantation’ to permit abnormal/delayed embryos to implant, and may also lead to downstream repercussions along the continuum of disorders that have their origins in implantation and placental development (including recurrent miscarriage, pre-eclampsia, intrauterine growth restriction and preterm birth). Indeed, recurrent miscarriage has been associated with a significantly altered secretory-stage dNK phenotype compared to fertile women (91). While some studies show no differences in percentage of total secretory-stage dNK cell numbers detected in women with recurrent miscarriage compared to those without, others report increased dNK levels in the endometrium of women with recurrent miscarriage, with further differences in women with primary versus secondary pregnancy loss (91–95). The lack of consensus of these studies indicates a distinct lack of predictive value of secretory-stage dNK numbers for subsequent pregnancy outcome. Secretory-stage dNK phenotypic and functional studies may therefore be more informative.
NK Cells in the Early Pregnancy Uterine Mucosa
The production of pro-angiogenic factors is fundamental for appropriate vascular development in the peri-implantation uterus and in the very early stages of pregnancy. However, while peri-implantation dNK cells demonstrate vascular constructive functions and have a pro-angiogenic profile with no apoptotic effects on vascular cells (20, 25), anti-angiogenic effects may be required as pregnancy progresses, to permit the physiological change in spiral arteries to take place (26, 29). Spiral arteries consist of several outer layers of vascular smooth muscle cells (VSMC) and an inner layer of endothelial cells (ECs); and spiral artery remodelling involves removal of VSMCs and the replacement of ECs with extravillous trophoblasts (EVTs), which are specialised placental cells arising from the trophectoderm-derived cytotrophoblast. Following implantation, the trophectoderm (the outer layer of the blastocyst) gives rise to the cytotrophoblast, which differentiates along either a villous or an extravillous pathway: villous cytotrophoblasts give rise to the placental villi, across which maternal-fetal gas and nutrient exchange take place; and EVTs migrate and invade through the decidua towards the spiral arteries in order to remodel them. Insufficient spiral artery remodelling and impaired trophoblast invasion have been associated with a range of obstetric complications, recurrent miscarriage, late spontaneous abortion, including pre-eclampsia, intrauterine growth restriction (IUGR), preterm birth, and placental abruption (collectively referred to as the ‘great obstetric syndromes’) (96–101).
Spiral artery remodelling takes place in two stages: an EVT-independent, followed by an EVT-dependent stage. The initial EVT-independent stage of this physiological change in the spiral arteries include VSMC hypertrophy, disorganisation and dedifferentiation, EC activation and vacuolisation, as well as breaks in the VSMC and EC layers: these events take place in the presence of leukocytes, ahead of trophoblast invasion (30, 102–104). Indeed, histological studies of first trimester decidua have detected early apoptotic changes in vascular cells of the spiral artery in the presence of maternal leukocytes but not EVT (103, 104), and VSMC dedifferentiation (30). Further, dNK cells isolated from 9–14-week decidual tissue (from elective termination of normal pregnancies) induced caspase-dependent apoptotic changes in VSMCs and ECs via a Fas signalling pathway, while dNK cells isolated from abnormally remodelling pregnancies (with high resistance to blood flow, and therefore at higher risk of developing pre-eclampsia) produced significantly lower levels of pro-apoptotic factors and failed to induce vascular cell apoptosis (10). In addition, dNK cells isolated from normally remodelling first-trimester pregnancies were found to secrete factors that induced endothelial activation and the disruption of endothelial integrity, thus further contributing to the early vascular changes during spiral artery remodelling; whereas dNK cell-secreted factors from abnormally remodelling pregnancies did not promote EC activation and destabilisation (11). Finally, dNK secretion of angiogenic growth factor levels during pregnancy decreases with increasing gestational age (29), and notably, elevated angiogenic factor secretion by dNK cells has been demonstrated in abnormally remodelling pregnancies (26). These lines of evidence demonstrate the essential roles that dNK cells play in initiating vascular remodelling of the maternal uterine spiral arteries (10, 11, 30, 39, 103); and EVT subsequently complete the remodelling process instigated by the dNK cells, to create uteroplacental vessels that provide the fetal blood supply (77, 102, 103, 105). Indeed, anomalous NK cell receptor gene expression has been linked to aberrant remodelling of the uterine vasculature and the primary stage of several human gestational pathologies, and compromised functional capacity of dNK cells in early pregnancy has been associated with recurrent miscarriage and pre-eclampsia, and other disorders that implicate defective spiral artery remodelling (106–110).
In addition to their direct effects on vascular remodelling, dNK cells in early pregnancy also produce a large repertoire of cytokines, growth factors and proteases that can promote EVT motility and invasion (10, 31). For example, dNK cells produce interleukin (IL)-8 and interferon γ-induced protein (IP)-10, while EVTs express their receptors, CXCR-1 and CXCR-3, respectively, which promotes EVT recruitment and migration to the spiral arteries (31). Further, in vitro studies demonstrated that dNK cells were able to promote the invasive behaviour of EVTs through a hepatocyte growth factor-mediated mechanism, which was found to be impaired in dNK cells isolated from abnormally remodelling pregnancies at higher risk of developing pre-eclampsia, which also secreted lower levels of several factors with known stimulatory effects on trophoblasts (urokinase plasminogen activator, heparin-binding epidermal growth factor, CXCL16, IL-1β and IL-8) (10). Additionally, as well as directly promoting EVT invasion, dNK cells also influence the differentiation of EVT into an invasive phenotype via their secretion of cytokines and chemokines that can alter the EVT adhesion molecule repertoire, whilst also promoting EVT survival (111–114). However, abnormally remodelling pregnancies demonstrate impaired dNK chemoattraction for EVT, as well as a reduced ability to promote EVT differentiation (115). It is noteworthy to add that dNK production of proteases (e.g. matrix metalloproteinases or urokinase plasminogen activator), as well as factors that mediate the upregulation of proteases (IL-1β, IL-6, IL-8, IL-10, leptin and TNF-α), can promote the breakdown of the elastic fibres at the basal sections of decidual spiral arteries and confer changes in the extracellular matrix, to support EVT invasion (116–120). Additionally, dNK-derived VEGF-C may also generate protective effects by conferring EVT resistance to cytotoxicity, as well as stimulating the assembly of EVT into networks of tube-like structures (18, 121). Thus, while dNK promote these EVT phenotypic and functional characteristics that are requisite for successful trophoblast invasion (including EVT survival, differentiation, migration, motility and invasion); EVT, as they migrate and invade through the decidua, influence dNK phenotype and functions, in addition to the placental induction of dNK proliferation through non-canonical hCG signalling. These dNK-trophoblast interactions in early pregnancy therefore represent an interdependent relationship through reciprocal actions, as well as via several functional similarities to promote vascular remodelling (27).
dNK cells can interact with invading EVT through their expression of human leukocyte antigen (HLA)-binding cell-surface receptors and that bind to HLA molecules expressed by EVT. For example, EVT-derived membrane-bound HLA-G and its soluble form (sHLA-G; secreted by EVT) have been shown to stimulate NK cell proliferation and cytokine stimulation, to promote uterine vascular modelling (122, 123). Additional actions of the homodimer form of the sHLA-G, via binding to KIR2DL4 (one of the killer-cell immunoglobulin (Ig)-like receptors expressed by NK cells), increases dNK secretion of IL-6, IL-8 and TNF-α (124). Upregulation of these cytokines may directly contribute to spiral artery remodelling, as well as having stimulatory effects on trophoblast invasion (11, 31, 111, 112, 120, 125, 126). Further, specific dNK-trophoblast interactions through dNK KIR and fetal EVT HLA-C are of particular importance, as certain KIR–HLA-C combinations have been associated with an increased risk of pre-eclampsia, recurrent miscarriage and IUGR (108–110). The repertoire of KIRs is heterogeneous, with two haplotypes: A and B. The A haplotype has seven KIR loci with only one activating receptor, KIR2SD4; however, despite being an activating receptor, the most common allele of KIR2SD4 has a deletion, resulting in individuals with two A haplotypes having no activating receptors. The B haplotypes are characterised by the incidence of extra loci that are not present on the A haplotype, consisting of activating receptors KIR2SD1, 2, 3, 5 and KIR3SD1, and the inhibitory receptors KIR2DL2 and KIR2DL5. HLA-C expresses both maternal and paternal antigens, resulting in two allotypes, HLA-C1 and HLA-C2 (109, 127). In pregnancies where dNK cells with the AA KIR genotype (lacking most or all of the activating receptors) are paired with EVT expressing the HLA-C2 allotype, there is a high risk of pre-eclampsia development (109). Conversely, dNK cells expressing activating KIRs enhance placentation and confer protection against reproductive and obstetric disorders (108, 128, 129), and altered dNK interactions with fetal HLA-C and HLA-G have been observed in abnormally remodelling pregnancies, which may compromise several components of the spiral artery remodelling process (130).
As stated previously, there is broad consent that NK cells in the uterine mucosa do not kill. However, we have described here the killing functions of dNK cells found both in the secretory stage endometrium and in early pregnancy. Further, accumulating evidence indicates that dNK cells can also kill infected cells in the presence of viruses (131–133). Indeed, in vitro studies demonstrated that dNK cells are able to invade trophoblast organ culture, co-localise with placental cells, degranulate and kill virally infected trophoblasts, as well as selectively kill intracellular bacteria in EVT without killing the trophoblast (132–134).
Peri-Implantation vs. Early Pregnancy dNK Cells: Phenotype and Function
The divergent functional profiles of dNK cells before and after conception demonstrate that the temporal endocrine and immunological fluctuations in the uterine microenvironment strongly influence dNK phenotype. Timely differentiation into appropriate dNK phenotypes within the uterine mucosa during the secretory stage and in early pregnancy are crucial for endometrial reconstruction, implantation, and successful pregnancy outcomes. Indeed, we have described here the numerous reproductive and obstetric disorders, including recurrent implantation failure, recurrent miscarriage, pre-eclampsia, IUGR and preterm birth, that have been associated with the absence of appropriate NK cell activation and function (10, 11, 20, 26, 106–110, 115, 130). Further, impaired dNK functional capacity has also been indicated in several uterine pathologies (19, 69, 89, 90). We therefore propose (i) a transitory angiodevelopmental secretory-stage dNK (s-dNK) cell phenotype during the ‘window of implantation’, whose phenotype and functional roles are fundamental for uterine vascular developmental growth and endometrial reconstruction, under indirect progesterone control. This is followed by an immunomodulatory shift in early pregnancy, stimulated by hCG and other placental-derived immunomodulatory factors, into (ii) a terminal angiomodulatory dNK phenotype with destabilising and pro-apoptotic effects on vascular cells, and pro-invasive effects on EVT, to initiate and support uterine vascular remodelling into enlarged uteroplacental vessels (Figure 1).
Concluding Remarks
It is probable that untimely or abnormal activation and differentiation into the transient angiodevelopmental s-dNK phenotype is implicated in a wide range of reproductive, gynaecological, and obstetric disorders; and disruption of further differentiation in early pregnancy into the appropriate angiomodulatory dNK phenotype with pro-apoptotic effects on vascular cells and pro-invasive effects on EVT, will also have serious implications in several adverse pregnancy outcomes. A comprehensive understanding of the stepwise NK cell temporal differentiation pathway within the uterine mucosa could therefore have substantial translational potential due to the important functional roles of s-dNK and dNK cells in normal vs. complicated pregnancies, as well as in a spectrum of uterine pathologies (10, 11, 49), and warrants further investigation.
Author Contributions
RF developed the scope and focus of the review. RF and AZ wrote the article. All authors approved the submitted version.
Conflict of Interest
The authors declare that the research was conducted in the absence of any commercial or financial relationships that could be construed as a potential conflict of interest.
Publisher’s Note
All claims expressed in this article are solely those of the authors and do not necessarily represent those of their affiliated organizations, or those of the publisher, the editors and the reviewers. Any product that may be evaluated in this article, or claim that may be made by its manufacturer, is not guaranteed or endorsed by the publisher.
Acknowledgments
RF was supported by a Medical Research Council Centre Grant (project reference MR/N022556/1).
References
1. Norwitz ER, Schust DJ, Fisher SJ. Implantation and the Survival of Early Pregnancy. New Engl J Med (2001) 345(19):1400–8. doi: 10.1056/NEJMra000763
2. Kopcow HD, Eriksson M, Mselle TF, Damrauer SM, Wira CR, Sentman CL, et al. Human Decidual NK Cells From Gravid Uteri and NK Cells From Cycling Endometrium Are Distinct NK Cell Subsets. Placenta (2010) 31(4):334–8. doi: 10.1016/j.placenta.2010.01.003
3. Gellersen B, Brosens IA, Brosens JJ. Decidualization of the Human Endometrium: Mechanisms, Functions, and Clinical Perspectives. Semin Reprod Med (2007) 25(6):445–53. doi: 10.1055/s-2007-991042
4. Gellersen B, Brosens JJ. Cyclic Decidualization of the Human Endometrium in Reproductive Health and Failure. Endocr Rev (2014) 35(6):851–905. doi: 10.1210/er.2014-1045
5. Salamonsen LA, Nie G, Hannan NJ, Dimitriadis E. Society for Reproductive Biology Founders' Lecture 2009. Preparing Fertile Soil: The Importance of Endometrial Receptivity. Reprod Fertil Dev (2009) 21(7):923–34. doi: 10.1071/RD09145
6. Fraser R, Smith R, Lin CJ. A 3D Endometrial Organotypic Model Simulating the Acute Inflammatory Decidualisation Initiation Phase With Epithelial Induction of the Key Endometrial Receptivity Marker, Integrin αvβ3. Hum Reprod Open (2021) 2021(4):hoab034. doi: 10.1093/hropen/hoab034
7. Vazquez J, Chasman DA, Lopez GE, Tyler CT, Ong IM, Stanic AK. Transcriptional and Functional Programming of Decidual Innate Lymphoid Cells. Front Immunol (2019) 10:3065. doi: 10.3389/fimmu.2019.03065
8. Huhn O, Zhao X, Esposito L, Moffett A, Colucci F, Sharkey AM. How Do Uterine Natural Killer and Innate Lymphoid Cells Contribute to Successful Pregnancy? Front Immunol (2021) 12:607669. doi: 10.3389/fimmu.2021.607669
9. Brighton PJ, Maruyama Y, Fishwick K, Vrljicak P, Tewary S, Fujihara R, et al. Clearance of Senescent Decidual Cells by Uterine Natural Killer Cells in Cycling Human Endometrium. Elife (2017) 6:e31274. doi: 10.7554/eLife.31274
10. Fraser R, Whitley GS, Johnstone AP, Host AJ, Sebire NJ, Thilaganathan B, et al. Impaired Decidual Natural Killer Cell Regulation of Vascular Remodelling in Early Human Pregnancies With High Uterine Artery Resistance. J Pathol (2012) 228(3):322–32. doi: 10.1002/path.4057
11. Fraser R, Whitley GS, Thilaganathan B, Cartwright JE. Decidual Natural Killer Cells Regulate Vessel Stability: Implications for Impaired Spiral Artery Remodelling. J Reprod Immunol (2015) 110:54–60. doi: 10.1016/j.jri.2015.04.003
12. Williams PJ, Searle RF, Robson SC, Innes BA, Bulmer JN. Decidual Leucocyte Populations in Early to Late Gestation Normal Human Pregnancy. J Reprod Immunol (2009) 82(1):24–31. doi: 10.1016/j.jri.2009.08.001
13. Manaster I, Mizrahi S, Goldman-Wohl D, Sela HY, Stern-Ginossar N, Lankry D, et al. Endometrial NK Cells are Special Immature Cells That Await Pregnancy. J Immunol (2008) 181(3):1869–76. doi: 10.4049/jimmunol.181.3.1869
14. Kaneko N, Kuo HH, Boucau J, Farmer JR, Allard-Chamard H, Mahajan VS, et al. Loss of Bcl-6-Expressing T Follicular Helper Cells and Germinal Centers in COVID-19. Cell (2020) 183(1):143–57.e13. doi: 10.1016/j.cell.2020.08.025
15. Strunz B, Bister J, Jönsson H, Filipovic I, Crona-Guterstam Y, Kvedaraite E, et al. Continuous Human Uterine NK Cell Differentiation in Response to Endometrial Regeneration and Pregnancy. Sci Immunol (2021) 6(56):eabb7800. doi: 10.1126/sciimmunol.abb7800
16. Huhn O, Ivarsson MA, Gardner L, Hollinshead M, Stinchcombe JC, Chen P, et al. Distinctive Phenotypes and Functions of Innate Lymphoid Cells in Human Decidua During Early Pregnancy. Nat Commun (2020) 11(1):381. doi: 10.1038/s41467-019-14123-z
17. Vento-Tormo R, Efremova M, Botting RA, Turco MY, Vento-Tormo M, Meyer KB, et al. Single-Cell Reconstruction of the Early Maternal-Fetal Interface in Humans. Nature (2018) 563(7731):347–53. doi: 10.1038/s41586-018-0698-6
18. Kalkunte S, Chichester CO, Gotsch F, Sentman CL, Romero R, Sharma S. Evolution of Non-Cytotoxic Uterine Natural Killer Cells. Am J Reprod Immunol (2008) 59(5):425–32. doi: 10.1111/j.1600-0897.2008.00595.x
19. Wang F, Qualls AE, Marques-Fernandez L, Colucci F. Biology and Pathology of the Uterine Microenvironment and its Natural Killer Cells. Cell Mol Immunol (2021) 18(9):2101–13. doi: 10.1038/s41423-021-00739-z
20. Rätsep MT, Felker AM, Kay VR, Tolusso L, Hofmann AP, Croy BA. Uterine Natural Killer Cells: Supervisors of Vasculature Construction in Early Decidua Basalis. Reproduction (2015) 149(2):R91–102. doi: 10.1530/REP-14-0271
21. Tabiasco J, Rabot M, Aguerre-Girr M, El Costa H, Berrebi A, Parant O, et al. Human Decidual NK Cells: Unique Phenotype and Functional Properties – a Review. Placenta (2006) 27 (Suppl A):S34–9. doi: 10.1016/j.placenta.2006.01.009
22. Le Bouteiller P, Tabiasco J. Killers Become Builders During Pregnancy. Nat Med (2006) 12(9):991–2. doi: 10.1038/nm0906-991
23. Koopman LA, Kopcow HD, Rybalov B, Boyson JE, Orange JS, Schatz F, et al. Human Decidual Natural Killer Cells are a Unique NK Cell Subset With Immunomodulatory Potential. J Exp Med (2003) 198(8):1201–12. doi: 10.1084/jem.20030305
24. El Costa H, Tabiasco J, Berrebi A, Parant O, Aguerre-Girr M, Piccinni MP, et al. Effector Functions of Human Decidual NK Cells in Healthy Early Pregnancy Are Dependent on the Specific Engagement of Natural Cytotoxicity Receptors. J Reprod Immunol (2009) 82(2):142–7. doi: 10.1016/j.jri.2009.06.123
25. Li XF, Charnock-Jones DS, Zhang E, Hiby S, Malik S, Day K, et al. Angiogenic Growth Factor Messenger Ribonucleic Acids in Uterine Natural Killer Cells. J Clin Endocrinol Metab (2001) 86(4):1823–34. doi: 10.1210/jc.86.4.1823
26. Wallace AE, Fraser R, Gurung S, Goulwara SS, Whitley GS, Johnstone AP, et al. Increased Angiogenic Factor Secretion by Decidual Natural Killer Cells From Pregnancies With High Uterine Artery Resistance Alters Trophoblast Function. Hum Reprod (2014) 29(4):652–60. doi: 10.1093/humrep/deu017
27. Wallace AE, Fraser R, Cartwright JE. Extravillous Trophoblast and Decidual Natural Killer Cells: A Remodelling Partnership. Hum Reprod Update (2012) 18(4):458–71. doi: 10.1093/humupd/dms015
28. Cartwright JE, Fraser R, Leslie K, Wallace AE, James JL. Remodelling at the Maternal-Fetal Interface: Relevance to Human Pregnancy Disorders. Reprod (Cambridge England) (2010) 140(6):803–13. doi: 10.1530/REP-10-0294
29. Lash GE, Schiessl B, Kirkley M, Innes BA, Cooper A, Searle RF, et al. Expression of Angiogenic Growth Factors by Uterine Natural Killer Cells During Early Pregnancy. J Leukoc Biol (2006) 80(3):572–80. doi: 10.1189/jlb.0406250
30. Ma Y, Yu X, Zhang L, Liu J, Shao X, Li YX, et al. Uterine Decidual Niche Modulates the Progressive Dedifferentiation of Spiral Artery Vascular Smooth Muscle Cells During Human Pregnancy†. Biol Reprod (2021) 104(3):624–37. doi: 10.1093/biolre/ioaa208
31. Hanna J, Goldman-Wohl D, Hamani Y, Avraham I, Greenfield C, Natanson-Yaron S, et al. Decidual NK Cells Regulate Key Developmental Processes at the Human Fetal-Maternal Interface. Nat Med (2006) 12(9):1065–74. doi: 10.1038/nm1452
32. King A, Gardner L, Loke YW. Evaluation of Oestrogen and Progesterone Receptor Expression in Uterine Mucosal Lymphocytes. Hum Reprod (1996) 11(5):1079–82. doi: 10.1093/oxfordjournals.humrep.a019300
33. Verma S, Hiby SE, Loke YW, King A. Human Decidual Natural Killer Cells Express the Receptor for and Respond to the Cytokine Interleukin 15. Biol Reprod (2000) 62(4):959–68. doi: 10.1095/biolreprod62.4.959
34. Gordon SM. Interleukin-15 in Outcomes of Pregnancy. Int J Mol Sci (2021) 22(20):11094–107. doi: 10.3390/ijms222011094
35. Kane N, Kelly R, Saunders PT, Critchley HO. Proliferation of Uterine Natural Killer Cells is Induced by Human Chorionic Gonadotropin and Mediated via the Mannose Receptor. Endocrinology (2009) 150(6):2882–8. doi: 10.1210/en.2008-1309
36. Li R, Wang TY, Xu X, Emery O, Yi M, Wu SP, et al. Spatial Transcriptomic Profiles of Mouse Uterine Microenvironments at Pregnancy Day 7.5. Biol Reprod (2022) 00,1–17. doi: 10.1093/biolre/ioac061
37. Torry DS, Leavenworth J, Chang M, Maheshwari V, Groesch K, Ball ER, et al. Angiogenesis in Implantation. J Assist Reprod Genet (2007) 24(7):303–15. doi: 10.1007/s10815-007-9152-7
38. Demir R, Yaba A, Huppertz B. Vasculogenesis and Angiogenesis in the Endometrium During Menstrual Cycle and Implantation. Acta Histochem (2010) 112(3):203–14. doi: 10.1016/j.acthis.2009.04.004
39. Robson A, Harris LK, Innes BA, Lash GE, Aljunaidy MM, Aplin JD, et al. Uterine Natural Killer Cells Initiate Spiral Artery Remodeling in Human Pregnancy. FASEB J (2012) 26(12):4876–85. doi: 10.1096/fj.12-210310
40. Mahajan D, Sharma NR, Kancharla S, Kolli P, Tripathy A, Sharma AK, et al. Role of Natural Killer Cells During Pregnancy and Related Complications. Biomolecules (2022) 12(1):68–85. doi: 10.3390/biom12010068
41. King A. Uterine Leukocytes and Decidualization. Hum Reprod Update (2000) 6(1):28–36. doi: 10.1093/humupd/6.1.28
42. Manaster I, Mandelboim O. The Unique Properties of Human NK Cells in the Uterine Mucosa. Placenta (2008) 29(Suppl A):S60–6. doi: 10.1016/j.placenta.2007.10.006
43. Manaster I, Mandelboim O. The Unique Properties of Uterine NK Cells. Am J Reprod Immunol (2010) 63(6):434–44. doi: 10.1111/j.1600-0897.2009.00794.x
44. Moffett-King A. Natural Killer Cells and Pregnancy. Nat Rev Immunol (2002) 2(9):656–63. doi: 10.1038/nri886
45. Gubbay O, Critchley HO, Bowen JM, King A, Jabbour HN. Prolactin Induces ERK Phosphorylation in Epithelial and CD56(+) Natural Killer Cells of the Human Endometrium. J Clin Endocrinol Metab (2002) 87(5):2329–35. doi: 10.1210/jcem.87.5.8515
46. Dunn CL, Critchley HO, Kelly RW. IL-15 Regulation in Human Endometrial Stromal Cells. J Clin Endocrinol Metab (2002) 87(4):1898–901. doi: 10.1210/jcem.87.4.8539
47. Sun R, Li AL, Wei HM, Tian ZG. Expression of Prolactin Receptor and Response to Prolactin Stimulation of Human NK Cell Lines. Cell Res (2004) 14(1):67–73. doi: 10.1038/sj.cr.7290204
48. Mavoungou E, Bouyou-Akotet MK, Kremsner PG. Effects of Prolactin and Cortisol on Natural Killer (NK) Cell Surface Expression and Function of Human Natural Cytotoxicity Receptors (NKp46, NKp44 and Nkp30). Clin Exp Immunol (2005) 139(2):287–96. doi: 10.1111/j.1365-2249.2004.02686.x
49. Dosiou C, Giudice LC. Natural Killer Cells in Pregnancy and Recurrent Pregnancy Loss: Endocrine and Immunologic Perspectives. Endocr Rev (2005) 26(1):44–62. doi: 10.1210/er.2003-0021
50. Espinoza J, Romero R, Mee Kim Y, Kusanovic JP, Hassan S, Erez O, et al. Normal and Abnormal Transformation of the Spiral Arteries During Pregnancy. J Perinat Med (2006) 34(6):447–58. doi: 10.1515/JPM.2006.089
51. Igarashi T, Konno R, Okamoto S, Moriya T, Satoh S, Yajima A. Involvement of Granule-Mediated Apoptosis in the Cyclic Changes of the Normal Human Endometrium. Tohoku J Exp Med (2001) 193(1):13–25. doi: 10.1620/tjem.193.13
52. Matzuk MM, Boime I. The Role of the Asparagine-Linked Oligosaccharides of the Alpha Subunit in the Secretion and Assembly of Human Chorionic Gonadotrophin. J Cell Biol (1988) 106(4):1049–59. doi: 10.1083/jcb.106.4.1049
53. Taylor ME. Structure and Function of the Macrophage Mannose Receptor. Results Probl Cell Differ (2001) 33:105–21. doi: 10.1007/978-3-540-46410-5_6
54. East L, Isacke CM. The Mannose Receptor Family. Biochim Biophys Acta (2002) 1572(2-3):364–86. doi: 10.1016/S0304-4165(02)00319-7
55. Cole LA. Immunoassay of Human Chorionic Gonadotropin, its Free Subunits, and Metabolites. Clin Chem (1997) 43(12):2233–43. doi: 10.1093/clinchem/43.12.2233
56. Sugantha SE, Webster S, Sundar E, Lenton EA. Predictive Value of Plasma Human Chorionic Gonadotrophin Following Assisted Conception Treatment. Hum Reprod (2000) 15(2):469–73. doi: 10.1093/humrep/15.2.469
57. Gamliel M, Goldman-Wohl D, Isaacson B, Gur C, Stein N, Yamin R, et al. Trained Memory of Human Uterine NK Cells Enhances Their Function in Subsequent Pregnancies. Immunity (2018) 48(5):951–62.e5. doi: 10.1016/j.immuni.2018.03.030
58. Okada H, Tsuzuki T, Murata H. Decidualization of the Human Endometrium. Reprod Med Biol (2018) 17(3):220–7. doi: 10.1002/rmb2.12088
59. Achache H, Revel A. Endometrial Receptivity Markers, the Journey to Successful Embryo Implantation. Hum Reprod Update (2006) 12(6):731–46. doi: 10.1093/humupd/dml004
60. Quenby S. Implantation Involves an Initial Pro-Inflammatory Response. Fertil Steril (2020) 114(2):288–9. doi: 10.1016/j.fertnstert.2020.05.039
61. Salker MS, Nautiyal J, Steel JH, Webster Z, Sucurovic S, Nicou M, et al. Disordered IL-33/ST2 Activation in Decidualizing Stromal Cells Prolongs Uterine Receptivity in Women With Recurrent Pregnancy Loss. PLoS One (2012) 7(12):e52252. doi: 10.1371/journal.pone.0052252
62. Lessey BA. Assessment of Endometrial Receptivity. Fertil Steril (2011) 96(3):522–9. doi: 10.1016/j.fertnstert.2011.07.1095
63. Lessey BA. Adhesion Molecules and Implantation. J Reprod Immunol (2002) 55(1-2):101–12. doi: 10.1016/S0165-0378(01)00139-5
64. Apparao KB, Murray MJ, Fritz MA, Meyer WR, Chambers AF, Truong PR, et al. Osteopontin and its Receptor Alphavbeta(3) Integrin are Coexpressed in the Human Endometrium During the Menstrual Cycle But Regulated Differentially. J Clin Endocrinol Metab (2001) 86(10):4991–5000. doi: 10.1210/jcem.86.10.7906
65. Zhang S, Lin H, Kong S, Wang S, Wang H, Wang H, et al. Physiological and Molecular Determinants of Embryo Implantation. Mol Aspects Med (2013) 34(5):939–80. doi: 10.1016/j.mam.2012.12.011
66. Brosens JJ, Gellersen B. The Uterus Under Hormonal Control–Cycling for Life. Mol Cell Endocrinol (2012) 358(2):145. doi: 10.1016/j.mce.2012.02.023
67. Al-Sabbagh M, Fusi L, Higham J, Lee Y, Lei K, Hanyaloglu AC, et al. NADPH Oxidase-Derived Reactive Oxygen Species Mediate Decidualization of Human Endometrial Stromal Cells in Response to Cyclic AMP Signaling. Endocrinology (2011) 152(2):730–40. doi: 10.1210/en.2010-0899
68. Evans J, Catalano RD, Morgan K, Critchley HO, Millar RP, Jabbour HN. Prokineticin 1 Signaling and Gene Regulation in Early Human Pregnancy. Endocrinology (2008) 149(6):2877–87. doi: 10.1210/en.2007-1633
69. Zhang X, Wei H. Role of Decidual Natural Killer Cells in Human Pregnancy and Related Pregnancy Complications. Front Immunol (2021) 12:728291. doi: 10.3389/fimmu.2021.728291
70. Fournier SB, D'Errico JN, Stapleton PA. Uterine Vascular Control Preconception and During Pregnancy. Compr Physiol (2021) 11(3):1871–93. doi: 10.1002/cphy.c190015
71. Rogers PA, Abberton KM. Endometrial Arteriogenesis: Vascular Smooth Muscle Cell Proliferation and Differentiation During the Menstrual Cycle and Changes Associated With Endometrial Bleeding Disorders. Microscopy Res Technique (2003) 60(4):412–9. doi: 10.1002/jemt.10279
72. Chinoy MR, Graybill MM, Miller SA, Lang CM, Kauffman GL. Angiopoietin-1 and VEGF in Vascular Development and Angiogenesis in Hypoplastic Lungs. Am J Physiol Lung Cell Mol Physiol (2002) 283(1):L60–6. doi: 10.1152/ajplung.00317.2001
73. Shibuya M. Tyrosine Kinase Receptor Flt/VEGFR Family: Its Characterization Related to Angiogenesis and Cancer. Genes Cancer (2010) 1(11):1119–23. doi: 10.1177/1947601910392987
74. Wen L, Chen LH, Li HY, Chang SP, Liao CY, Tsui KH, et al. Roles of Estrogen and Progesterone in Endometrial Hemodynamics and Vascular Endothelial Growth Factor Production. J Chin Med Assoc (2009) 72(4):188–93. doi: 10.1016/S1726-4901(09)70052-8
75. Ratajczak MZ, Ratajczak J, Machalinski B, Majka M, Marlicz W, Carter A, et al. Role of Vascular Endothelial Growth Factor (VEGF) and Placenta-Derived Growth Factor (PlGF) in Regulating Human Haemopoietic Cell Growth. Br J Haematol (1998) 103(4):969–79. doi: 10.1046/j.1365-2141.1998.01076.x
76. Makieva S, Giacomini E, Ottolina J, Sanchez AM, Papaleo E, Viganò P. Inside the Endometrial Cell Signaling Subway: Mind the Gap(s). Int J Mol Sci (2018) 19(9):2477. doi: 10.3390/ijms19092477
77. Norwitz ER. Defective Implantation and Placentation: Laying the Blueprint for Pregnancy Complications. Reprod Biomed Online (2006) 13(4):591–9. doi: 10.1016/S1472-6483(10)60649-9
78. Rabaglino MB, Post Uiterweer ED, Jeyabalan A, Hogge WA, Conrad KP. Bioinformatics Approach Reveals Evidence for Impaired Endometrial Maturation Before and During Early Pregnancy in Women Who Developed Preeclampsia. Hypertension (Dallas Tex 1979) (2015) 65(2):421–9. doi: 10.1161/HYPERTENSIONAHA.114.04481
79. Conrad KP, Rabaglino MB, Post Uiterweer ED. Emerging Role for Dysregulated Decidualization in the Genesis of Preeclampsia. Placenta (2017) 60:119–29. doi: 10.1016/j.placenta.2017.06.005
80. Tan SY, Hang F, Purvarshi G, Li MQ, Meng DH, Huang LL. Decreased Endometrial Vascularity and Receptivity in Unexplained Recurrent Miscarriage Patients During Midluteal and Early Pregnancy Phases. Taiwanese J Obstet Gynecol (2015) 54(5):522–6. doi: 10.1016/j.tjog.2014.10.008
81. Timeva T, Shterev A, Kyurkchiev S. Recurrent Implantation Failure: The Role of the Endometrium. J Reprod Infertil (2014) 15(4):173–83.
82. Patel BG, Lessey BA. Clinical Assessment and Management of the Endometrium in Recurrent Early Pregnancy Loss. Semin Reprod Med (2011) 29(6):491–506. doi: 10.1055/s-0031-1293203
83. Strowitzki T, Germeyer A, Popovici R, von Wolff M. The Human Endometrium as a Fertility-Determining Factor. Hum Reprod Update (2006) 12(5):617–30. doi: 10.1093/humupd/dml033
84. Franasiak JM, Holoch KJ, Yuan L, Schammel DP, Young SL, Lessey BA. Prospective Assessment of Midsecretory Endometrial Leukemia Inhibitor Factor Expression Versus Alphanubeta3 Testing in Women With Unexplained Infertility. Fertil Steril (2014) 101(6):1724–31. doi: 10.1016/j.fertnstert.2014.02.027
85. Donaghay M, Lessey BA. Uterine Receptivity: Alterations Associated With Benign Gynecological Disease. Semin Reprod Med (2007) 25(6):461–75. doi: 10.1055/s-2007-991044
86. Lessey BA. Implantation Defects in Infertile Women With Endometriosis. Ann New York Acad Sci (2002) 955:265–80396–406. doi: 10.1111/j.1749-6632.2002.tb02787.x
87. Lessey BA, Castelbaum AJ, Sawin SW, Buck CA, Schinnar R, Bilker W, et al. Aberrant Integrin Expression in the Endometrium of Women With Endometriosis. J Clin Endocrinol Metab (1994) 79(2):643–9. doi: 10.1210/jcem.79.2.7519194
88. Yuan X, Saravelos SH, Wang Q, Xu Y, Li TC, Zhou C. Endometrial Thickness as a Predictor of Pregnancy Outcomes in 10787 Fresh IVF-ICSI Cycles. Reprod BioMed Online (2016) 33(2):197–205. doi: 10.1016/j.rbmo.2016.05.002
89. Thiruchelvam U, Wingfield M, O'Farrelly C. Natural Killer Cells: Key Players in Endometriosis. Am J Reprod Immunol (2015) 74(4):291–301. doi: 10.1111/aji.12408
90. Degos C, Heinemann M, Barrou J, Boucherit N, Lambaudie E, Savina A, et al. Endometrial Tumor Microenvironment Alters Human NK Cell Recruitment, and Resident NK Cell Phenotype and Function. Front Immunol (2019) 10:877. doi: 10.3389/fimmu.2019.00877
91. Lachapelle MH, Miron P, Hemmings R, Roy DC. Endometrial T, B, and NK Cells in Patients With Recurrent Spontaneous Abortion. Altered Profile and Pregnancy Outcome. J Immunol (1996) 156(10):4027–34.
92. Shimada S, Kato EH, Morikawa M, Iwabuchi K, Nishida R, Kishi R, et al. No Difference in Natural Killer or Natural Killer T-Cell Population, But Aberrant T-Helper Cell Population in the Endometrium of Women With Repeated Miscarriage. Hum Reprod (2004) 19(4):1018–24. doi: 10.1093/humrep/deh159
93. Michimata T, Ogasawara MS, Tsuda H, Suzumori K, Aoki K, Sakai M, et al. Distributions of Endometrial NK Cells, B Cells, T Cells, and Th2/Tc2 Cells Fail to Predict Pregnancy Outcome Following Recurrent Abortion. Am J Reprod Immunol (2002) 47(4):196–202. doi: 10.1034/j.1600-0897.2002.01048.x
94. Woon EV, Greer O, Shah N, Nikolaou D, Johnson M, Male V. Number and Function of Uterine Natural Killer Cells in Recurrent Miscarriage and Implantation Failure: A Systematic Review and Meta-Analysis. Hum Reprod Update (2022) 1–35. doi: 10.1093/humupd/dmac006
95. Strobel L, Vomstein K, Kyvelidou C, Hofer-Tollinger S, Feil K, Kuon R-J, et al. Different Background: Natural Killer Cell Profiles in Secondary Versus Primary Recurrent Pregnancy Loss. J Clin Med (2021) 10(2):194. doi: 10.3390/jcm10020194
96. Brosens IA, Robertson WB, Dixon HG. The Role of the Spiral Arteries in the Pathogenesis of Preeclampsia. Obstet Gynecol Annu (1972) 1:177–91.
97. Brosens IA, Robertson WB, Dixon HG. The Role of the Spiral Arteries in the Pathogenesis of Pre-Eclampsia. J Pathol (1970) 101(4):Pvi.
98. Pijnenborg R, Anthony J, Davey DA, Rees A, Tiltman A, Vercruysse L, et al. Placental Bed Spiral Arteries in the Hypertensive Disorders of Pregnancy. Br J Obstet Gynaecol (1991) 98(7):648–55. doi: 10.1111/j.1471-0528.1991.tb13450.x
99. Meekins JW, Pijnenborg R, Hanssens M, McFadyen IR, van Asshe A. A Study of Placental Bed Spiral Arteries and Trophoblast Invasion in Normal and Severe Pre-Eclamptic Pregnancies. Br J Obstet Gynaecol (1994) 101(8):669–74. doi: 10.1111/j.1471-0528.1994.tb13182.x
100. Sebire NJ, Backos M, Goldin RD, Regan L. Placental Massive Perivillous Fibrin Deposition Associated With Antiphospholipid Antibody Syndrome. BJOG an Int J Obstet Gynaecol (2002) 109(5):570–3. doi: 10.1111/j.1471-0528.2002.00077.x
101. Brosens I, Pijnenborg R, Vercruysse L, Romero R. The “Great Obstetrical Syndromes” Are Associated With Disorders of Deep Placentation. Am J Obstet Gynecol (2011) 204(3):193–201. doi: 10.1016/j.ajog.2010.08.009
102. Craven CM, Morgan T, Ward K. Decidual Spiral Artery Remodelling Begins Before Cellular Interaction With Cytotrophoblasts. Placenta (1998) 19(4):241–52. doi: 10.1016/S0143-4004(98)90055-8
103. Smith SD, Dunk CE, Aplin JD, Harris LK, Jones RL. Evidence for Immune Cell Involvement in Decidual Spiral Arteriole Remodeling in Early Human Pregnancy. Am J Pathol (2009) 174(5):1959–71. doi: 10.2353/ajpath.2009.080995
104. Hazan AD, Smith SD, Jones RL, Whittle W, Lye SJ, Dunk CE. Vascular-Leukocyte Interactions: Mechanisms of Human Decidual Spiral Artery Remodeling In Vitro. Am J Pathol (2010) 177(2):1017–30. doi: 10.2353/ajpath.2010.091105
105. Pijnenborg R, Vercruysse L, Hanssens M. The Uterine Spiral Arteries in Human Pregnancy: Facts and Controversies. Placenta (2006) 27(9-10):939–58. doi: 10.1016/j.placenta.2005.12.006
106. Moffett A, Chazara O, Colucci F, Johnson MH. Variation of Maternal KIR and Fetal HLA-C Genes in Reproductive Failure: Too Early for Clinical Intervention. Reprod Biomed Online (2016) 33(6):763–9. doi: 10.1016/j.rbmo.2016.08.019
107. Fukui A, Funamizu A, Yokota M, Yamada K, Nakamua R, Fukuhara R, et al. Uterine and Circulating Natural Killer Cells and Their Roles in Women With Recurrent Pregnancy Loss, Implantation Failure and Preeclampsia. J Reprod Immunol (2011) 90(1):105–10. doi: 10.1016/j.jri.2011.04.006
108. Hiby SE, Apps R, Sharkey AM, Farrell LE, Gardner L, Mulder A, et al. Maternal Activating KIRs Protect Against Human Reproductive Failure Mediated by Fetal HLA-C2. J Clin Invest (2010) 120(11):4102–10. doi: 10.1172/JCI43998
109. Hiby SE, Walker JJ, O'Shaughnessy KM, Redman CW, Carrington M, Trowsdale J, et al. Combinations of Maternal KIR and Fetal HLA-C Genes Influence the Risk of Preeclampsia and Reproductive Success. J Exp Med (2004) 200(8):957–65. doi: 10.1084/jem.20041214
110. Hiby SE, Apps R, Chazara O, Farrell LE, Magnus P, Trogstad L, et al. Maternal KIR in Combination With Paternal HLA-C2 Regulate Human Birth Weight. J Immunol (2014) 192(11):5069–73. doi: 10.4049/jimmunol.1400577
111. De Oliveira LG, Lash GE, Murray-Dunning C, Bulmer JN, Innes BA, Searle RF, et al. Role of Interleukin 8 in Uterine Natural Killer Cell Regulation of Extravillous Trophoblast Cell Invasion. Placenta (2010) 31(7):595–601. doi: 10.1016/j.placenta.2010.04.012
112. Jovanović M, Vićovac L. Interleukin-6 Stimulates Cell Migration, Invasion and Integrin Expression in HTR-8/SVneo Cell Line. Placenta (2009) 30(4):320–8. doi: 10.1016/j.placenta.2009.01.013
113. Knöfler M. Critical Growth Factors and Signalling Pathways Controlling Human Trophoblast Invasion. Int J Dev Biol (2010) 54(2-3):269–80. doi: 10.1387/ijdb.082769mk
114. Hannan NJ, Jones RL, White CA, Salamonsen LA. The Chemokines, CX3CL1, CCL14, and CCL4, Promote Human Trophoblast Migration at the Feto-Maternal Interface. Biol Reprod (2006) 74(5):896–904. doi: 10.1095/biolreprod.105.045518
115. Wallace AE, Host AJ, Whitley GS, Cartwright JE. Decidual Natural Killer Cell Interactions With Trophoblasts Are Impaired in Pregnancies at Increased Risk of Preeclampsia. Am J Pathol (2013) 183(6):1853–61. doi: 10.1016/j.ajpath.2013.08.023
116. Choudhury RH, Dunk CE, Lye SJ, Harris LK, Aplin JD, Jones RL. Decidual Leucocytes Infiltrating Human Spiral Arterioles are Rich Source of Matrix Metalloproteinases and Degrade Extracellular Matrix In Vitro and in Situ. Am J Reprod Immunol (2019) 81(1):e13054. doi: 10.1111/aji.13054
117. Harris LK. IFPA Gabor Than Award Lecture: Transformation of the Spiral Arteries in Human Pregnancy: Key Events in the Remodelling Timeline. Placenta (2011) 32 Suppl 2:S154–8. doi: 10.1016/j.placenta.2010.11.018
118. Knöfler M, Simmons DG, Lash GE, Harris LK, Armant DR. Regulation of Trophoblast Invasion - A Workshop Report. Placenta (2008) 29(Suppl A):S26–8. doi: 10.1016/j.placenta.2007.11.008
119. Harris LK, Aplin JD. Vascular Remodeling and Extracellular Matrix Breakdown in the Uterine Spiral Arteries During Pregnancy. Reprod Sci (Thousand Oaks Calif) (2007) 14(8 Suppl):28–34. doi: 10.1177/1933719107309588
120. Jovanović M, Stefanoska I, Radojcić L, Vićovac L. Interleukin-8 (CXCL8) Stimulates Trophoblast Cell Migration and Invasion by Increasing Levels of Matrix Metalloproteinase (MMP)2 and MMP9 and Integrins Alpha5 and Beta1. Reprod (Cambridge England) (2010) 139(4):789–98. doi: 10.1530/REP-09-0341
121. Hu Y, Eastabrook G, Tan R, MacCalman CD, Dutz JP, von Dadelszen P. Decidual NK Cell-Derived Conditioned Medium Enhances Capillary Tube and Network Organization in an Extravillous Cytotrophoblast Cell Line. Placenta (2010) 31(3):213–21. doi: 10.1016/j.placenta.2009.12.011
122. van der Meer A, Lukassen HG, van Cranenbroek B, Weiss EH, Braat DD, van Lierop MJ, et al. Soluble HLA-G Promotes Th1-Type Cytokine Production by Cytokine-Activated Uterine and Peripheral Natural Killer Cells. Mol Hum Reproduct (2007) 13(2):123–33. doi: 10.1093/molehr/gal100
123. van der Meer A, Lukassen HG, van Lierop MJ, Wijnands F, Mosselman S, Braat DD, et al. Membrane-Bound HLA-G Activates Proliferation and Interferon-Gamma Production by Uterine Natural Killer Cells. Mol Hum Reproduct (2004) 10(3):189–95. doi: 10.1093/molehr/gah032
124. Li C, Houser BL, Nicotra ML, Strominger JL. HLA-G Homodimer-Induced Cytokine Secretion Through HLA-G Receptors on Human Decidual Macrophages and Natural Killer Cells. Proc Natl Acad Sci USA (2009) 106(14):5767–72. doi: 10.1073/pnas.0901173106
125. Meisser A, Cameo P, Islami D, Campana A, Bischof P. Effects of Interleukin-6 (IL-6) on Cytotrophoblastic Cells. Mol Hum Reproduct (1999) 5(11):1055–8. doi: 10.1093/molehr/5.11.1055
126. Champion H, Innes BA, Robson SC, Lash GE, Bulmer JN. Effects of Interleukin-6 on Extravillous Trophoblast Invasion in Early Human Pregnancy. Mol Hum Reproduct (2012) 18(8):391–400. doi: 10.1093/molehr/gas010
127. Mandelboim O, Reyburn HT, Valés-Gómez M, Pazmany L, Colonna M, Borsellino G, et al. Protection From Lysis by Natural Killer Cells of Group 1 and 2 Specificity Is Mediated by Residue 80 in Human Histocompatibility Leukocyte Antigen C Alleles and Also Occurs With Empty Major Histocompatibility Complex Molecules. J Exp Med (1996) 184(3):913–22. doi: 10.1084/jem.184.3.913
128. Kennedy PR, Chazara O, Gardner L, Ivarsson MA, Farrell LE, Xiong S, et al. Activating KIR2DS4 Is Expressed by Uterine NK Cells and Contributes to Successful Pregnancy. J Immunol (2016) 197(11):4292–300. doi: 10.4049/jimmunol.1601279
129. Xiong S, Sharkey AM, Kennedy PR, Gardner L, Farrell LE, Chazara O, et al. Maternal Uterine NK Cell-Activating Receptor KIR2DS1 Enhances Placentation. J Clin Invest (2013) 123(10):4264–72. doi: 10.1172/JCI68991
130. Wallace AE, Whitley GS, Thilaganathan B, Cartwright JE. Decidual Natural Killer Cell Receptor Expression is Altered in Pregnancies With Impaired Vascular Remodeling and a Higher Risk of Pre-Eclampsia. J Leukoc Biol (2015) 97(1):79–86. doi: 10.1189/jlb.2A0614-282R
131. Le Bouteiller P, Siewiera J, Casart Y, Aguerre-Girr M, El Costa H, Berrebi A, et al. The Human Decidual NK-Cell Response to Virus Infection: What can We Learn From Circulating NK Lymphocytes? J Reprod Immunol (2011) 88(2):170–5. doi: 10.1016/j.jri.2010.12.005
132. Siewiera J, El Costa H, Tabiasco J, Berrebi A, Cartron G, Le Bouteiller P, et al. Human Cytomegalovirus Infection Elicits New Decidual Natural Killer Cell Effector Functions. PLoS Pathog (2013) 9(4):e1003257. doi: 10.1371/journal.ppat.1003257
133. Sen Santara S, Crespo ÂC, Mulik S, Ovies C, Boulenouar S, Strominger JL, et al. Decidual NK Cells Kill Zika Virus-Infected Trophoblasts. Proc Natl Acad Sci USA (2021) 118(47):e2115410118. doi: 10.1073/pnas.2115410118
Keywords: decidual natural killer (dNK) cells, differentiation, secretory stage endometrium, progesterone, human chorionic gonadotropin, uterine vascular growth, spiral artery remodelling, immune regulation
Citation: Fraser R and Zenclussen AC (2022) Killer Timing: The Temporal Uterine Natural Killer Cell Differentiation Pathway and Implications for Female Reproductive Health. Front. Endocrinol. 13:904744. doi: 10.3389/fendo.2022.904744
Received: 25 March 2022; Accepted: 16 May 2022;
Published: 27 June 2022.
Edited by:
Benoit Pourcet, Université de Lille, FranceReviewed by:
Susan Laird, Sheffield Hallam University, United KingdomJohn D. Aplin, The University of Manchester, United Kingdom
Copyright © 2022 Fraser and Zenclussen. This is an open-access article distributed under the terms of the Creative Commons Attribution License (CC BY). The use, distribution or reproduction in other forums is permitted, provided the original author(s) and the copyright owner(s) are credited and that the original publication in this journal is cited, in accordance with accepted academic practice. No use, distribution or reproduction is permitted which does not comply with these terms.
*Correspondence: Rupsha Fraser, rupsha.fraser@ed.ac.uk