- 1Department of Nephrology, The Third Affiliated Hospital of Chongqing Medical University (Gener Hospital), Chongqing, China
- 2Department of Nephrology, Chongqing Kaizhou District People’s Hospital of Chongqing, Chongqing, China
Metabolic syndrome (MetS) includes visceral obesity, hyperglycemia, dyslipidemia, and hypertension. The prevalence of MetS is 20-25%, which is an important risk factor for chronic kidney disease (CKD). MetS causes effects on renal pathophysiology, including glomerular hyperfiltration, RAAS, microalbuminuria, profibrotic factors and podocyte injury. This review compares several criteria of MetS and analyzes their differences. MetS and the pathogenesis of CKD includes insulin resistance, obesity, dyslipidemia, inflammation, oxidative stress, and endothelial dysfunction. The intervention of MetS-related renal damage is the focus of this article and includes controlling body weight, hypertension, hyperglycemia, and hyperlipidemia, requiring all components to meet the criteria. In addition, interventions such as endoplasmic reticulum stress, oxidative stress, gut microbiota, body metabolism, appetite inhibition, podocyte apoptosis, and mesenchymal stem cells are reviewed.
1 Introduction
MetS called “Syndrome X” at first, it refers to a pathological state of metabolic disorders of proteins, fats, carbohydrates, and other substances in humans. It is including hypertension, hyperlipidemia, hyperuricemia, hyperglycemia, central obesity and insulin resistance. MetS is a common risk factor for the morbidity and mortality of cardiovascular events such as myocardial infarction, stroke, sudden cardiac death and thrombosis (1–6), it is also an important cause of new-onset CKD and progression of CKD. With the increasing prevalence of MetS, its effects on the kidneys have attracted an increasing amount of attention from nephrologists. The prevalence of MetS and MetS-related renal injury are also different due to different races, lifestyles, and MetS diagnostic criteria. Therefore, we will summarize the epidemiology, pathogenesis, diagnosis and treatment progress of MetS-related renal injury.
2 MetS Definition and Prevalence
There is no uniform diagnostic standard for MetS in the world, and the World Health Organization (WHO, 1998) (7), National Cholesterol Education/Adult Treatment Panel III (NCEP-ATPIII,2001) (8), Modified NCEP-ATP III,2010 (9), American Heart Association (AHA, 2005) (10), International Diabetes Federation (IDF, 2005) (11), and the Chinese Diabetes Society (CDS,2020) (12) definitions are currently used. Components of the several diagnostic criteria include central adiposity, an impaired glucose tolerance, waist circumference (WC); blood pressure, high density lipoprotein, triglycerides (TG), and serum glucose. The WHO believes that the basic condition for diagnosing MetS is an abnormal glucose metabolism, diabetes, or insulin resistance (IR). The IDF believes central adiposity is a prerequisite for the diagnosis of MetS. Due to the different focus of several diagnostic criteria, the incidence of MetS in the same group is different.Therefore, to unify the diagnostic criteria of MetS, IDF, American Heart Association/National Heart, Lung, and Blood Institute (AHA/NHLBI), and other institutions published the MetS Joint Interim Statement (JIS) criterion (13). This standard no longer regards WC as a necessary condition for the diagnosis of MetS. The JIS criterion for defining MetS includes: (1) raised fasting blood glucose (FBG) or the use of hypoglycemic drugs; (2) increased blood pressure or the use of antihypertensive drugs; (3) increased plasma TG or the use of hypolipidemic drugs; (4) a decreased HDL-C; and (5) the Chinese criterion for central obesity (or visceral obesity) with a waist circumference of >85 cm for men and >80 cm for women. MetS was diagnosed if three or more of the above criteria were met (13). The diagnostic criteria of the China Diabetes Association (CDA) MetS of the Chinese Medical Association has a high similarity and coincidence rate with the JIS and NCEP-ATPIII (Kappa values are 0.730 and 0.774, respectively) (14). Table 1 shows the common definitions of MetS.
It is estimated that the worldwide prevalence of MetS is 20 – 25% (15), but there are great differences in the prevalence of MetS in various countries. According to the literature, the lowest prevalence of MetS patients is only 6.1% and the highest is 55.6%. This may be due to different gender, age, racial, eating habits, education, medical security, nature of work, and living environment. From 1980 to 2012, the prevalence of MetS in the United States increased to 35% (16), however, the prevalence of MetS in the United States has been gradually decreasing in recent years. The data released by the National Health and Nutrition Examination (NHANES) in 2020 showed that the incidence rate of MetS was 24% in men and 22% in women (17). A meta-analysis from China showed that the prevalence of MetS was 24.5% between 2007 and 2015. By sex, the prevalence was 19.2% in men and 27.0% in females. The older the age, the higher the prevalence of MetS. The prevalence of MetS in aged ≥ 60 was 2.33 times higher than those in aged 15-39 (32.4% vs. 13.9%). The prevalence of MetS was 1.3 times higher in individuals living in urban areas than those living in rural areas (24.9% vs. 19.2%) (18). A joint survey study between the United States and China showed that the prevalence of MetS in the two countries was 36.6-37.3% and 23.0%, respectively. The highest prevalence of MetS is in aged 40-50 (19). MetS is also common in South Asian countries, including Afghanistan, Bangladesh, India, Maldives, Nepal, Pakistan, and Sri Lanka. The lowest prevalence of MetS was only 8.6%, and the highest was 46.1% (20). All were in South Asian, using the ATP III diagnostic criteria, Pakistani males had a higher prevalence of MetS (55.6% vs. 45.9%) (21), while Indian females had a 1.30-fold higher prevalence of MetS (50.9% vs. 39.2%) (22). From 2009 to 2013, the prevalence of MetS in Japan was 14.6% (648/4446). By sex, the prevalence was 20.6% in males and 6.1% in females (23). It can be seen that the prevalence of MetS is greatly affected by dietary habits, medical insurance, gender and age, especially postmenopausal women have a higher prevalence of MetS.
3 Association Between MetS and CKD
MetS and CKD are causal and influence each other. A large number of studies have confirmed that MetS can lead to changes in renal structure and function, such as a decreased glomerular filtration rate (GFR) and increased urinary microalbumin (24–27). A meta-analysis showed that the risk of CKD in MetS was 1.34 times higher than those without MetS (28). Another meta-analysis showed that MetS increased the risk of CKD by 50% (29). Many studies found that each component of MetS was associated with CKD. The more components there were, the higher the risk of CKD (odds ratio, 1.96; 95%: 1.71,2.34) (26, 29). However, a few reports have shown a nonsignificant association, which may have been related to the different diagnostic criteria used for MetS (30). Similarly, due to impaired renal function, microenvironment changes and disorder of glucose and lipid metabolism in patients with CKD, the incidence of MetS in patients with CKD is significantly higher than that in the general population. With the progress of CKD, the incidence of MetS gradually increases (31). A study in Thailand found that the prevalence of MetS in patients with CKD was 71.3%, and the prevalence of met in patients with ckd3a to 5 was 70.1%, 72.3%, 73.4% and 72.7% respectively, which was significantly higher than that in patients without CKD (32).
Recently, after using the “MetS score” and the “MetS factor” to refine MetS and its components, it was found that regardless of gender and racial, the higher the score of MetS, the higher prevalence of CKD, including GFR decline and microalbuminuria increase (33–35). When each component of MetS was evaluated separately, it was found that hypertension and increased LDL metabolism were associated with microalbuminuria and estimated glomerular filtration rate (eGFR), hyperglycemia and hypertriglyceridemia were associated with microalbuminuria (36). Sixty percent of the study population had MetS in CKD3-4. After more than 2 years of follow-up, CKD stage 3-4 patients with MetS had a 1.33 times increased risk of progression to ESRD. Among the components of MetS, hyperlipidemia and elevated blood pressure are more likely lead to the progression of CKD to ESRD; Low HDL cholesterol increases the risk of death; Impaired glucose metabolism is an important risk factor for ESRD and death (37).
4 The Pathogenesis of MetS-Related Renal Injury
The pathogenesis of MetS-related renal damage is complex, including insulin resistance, obesity, hypertension, dyslipidemia, inflammation, oxidative stress, and endothelial dysfunction. The pathogenesis of MetS-related kidney injury is shown in Figure 1.
4.1 Insulin Resistance
Insulin resistance is essential factor of MetS, it is able to induce sodium retention and vascular endothelium vasoconstriction by antinatriuresis, which leads to RAAS activation and renal tubular lipid accumulation finally. This may be the important cause of MetS-related renal damage caused by insulin resistance (38, 39). Insulin resistance-related renal injury also includes transforming growth factor-β1 (TGF-β1), which is increased in the adipocytes of obese patients with insulin resistance and is responsible for the proliferation of mesangial cells and ultimately CKD, a potent initiator of disease (40–43). The sterol regulatory element binding protein-1 (SREBP-1) increases lipid droplet deposition in renal tubular cells and interstitial extracellular mechanisms (44–46), leading to tubular atrophy and interstitial fibrosis. Insulin like growth factor-1 (IGF-1) and dedifferentiation of vascular smooth muscle cells can induce connected tissue growth factor (CTGF), thus promoting renal tubular fibrosis Hyperglycemia in rat mesangial cells inhibits the degradation of the extracellular matrix by metalloproteinase 9, resulting in mesangial extracellular matrix proliferation and fibrosis (47–50). In addition, insulin resistance damages the microvessels of patients, especially the fundus, kidneys, muscles and cardiac arteries, and eventually damages the corresponding target organs (51).
4.2 Obesity
Hemodynamic changes, abnormal lipid metabolism, and dysregulations of the hormone response are the primary pathogeneses of obesity-Related Glomerulopathy (ORG) (52). A study found that aldosterone levels were significantly elevated in obese patients, hypertension and high WC were positively correlated with the level of aldosterone and negatively correlated with HDL (53). Activation of the RAAS and enhanced sympathetic activity in obese patients lead to increased levels of aldosterone, which reflexively lead to increased renal tubular reabsorption of sodium salts, resulting in water and sodium retention (54). The activation of RAAS in obese patients leads to hemodynamic changes such as increased of GFR and renal plasma flow (RPF), which causes glomerular hyperfiltration, compensated glomerulomegaly, segmental sclerosis, and promoting the progression of MetS-related renal damage (55–57). Hormone regulation disorder and ectopic lipid deposition in obese patients can directly or indirectly affect the structure and function of renal intrinsic cells (52). Hyperinsulinemia promotes the secretion of leptin by adipocytes, and leptin levels are significantly elevated in MetS patients (58, 59). Increased leptin secretion in MetS patients leads to kidney damage through the following two pathways, including (1) promoting the expression of TGF-β1 in renal parenchymal cells, increasing the production of type IV collagen, leading to tubular atrophy, interstitial fibrosis and glomerulosclerosis, (2) Leptin causes sodium reabsorption, leading to changes in renal hemodynamics (60, 61).
4.3 Hypertension
Hypertension is an important condition for the diagnosis of Mets. Hypertension in MetS patients is closely related to plasma aldosterone levels and sympathetic nerve activity (53). First, visceral adipocytes secrete a large number of substances such as angiotensinogen, which leads to the activation of RAAS (62–64). Second, the accumulation of excessive fat in and around the kidneys of patients with MetS compresses renal parenchymal cells, resulting in impaired pressure natriuresis (65–68). Thirdly, other hormone secretion disorders in MetS patients (including increased leptin, reduced adiponectin, etc.) lead to increased sympathetic nerve activity in the body (69–71), which are important reasons for hypertension in MetS patients. For a long time, the changes in renal structure and function caused by hypertension cannot be ignored, and it is also one of the important secondary causes of ESRD. Hypertension primarily causes kidney damage due to ischemia. Ischemia causes renal tubular, renal vascular, and glomerular damage, primarily renal tubular damage (72, 73). Ischemia can also increase the synthesis and secretion of angiotensin II (ANG II), which can further constrict blood vessels and lead to the proliferation of renal parenchymal cells, damaging the kidney through hemodynamic and non-hemodynamic effects (74).
4.4 Another Pathogenesis
During MetS, adiponectin, leptin, chemerin, resistin, IL-6, and tumor necrosis factor α(TNF-α) and other adipokines are abnormally secreted and released, or dysfunctional, which induces oxidative stress, endothelial dysfunction, inflammatory effects, and increased sympathetic activity, and finally lead to changes in renal function and structure (75–79). Furthermore, during Mets, adipose tissue secretion of pro-inflammatory factors increased, including macrophage chemoattractant protein-1 (MCP-1), macrophage migration inhibitory factor, chemokine ligand 5, and macrophage colony-stimulating factor. These pro-inflammatory factors can lead to inflammatory response, resulting in proteinuria and impaired renal function (80–82). The production of reactive oxygen species (ROS) in renal tissue increases due to inflammatory cell infiltration. ROS may cause damage to proximal tubules by interfering with renal tubular ion transport by altering renal and hemodynamics. ROS can also induce TGF-β1 and fibrinolysis through the activation of the nuclear factor-κ light chain enhancer and nicotinamide adenine dinucleotide phosphate oxidase (NADPH) pathways of activated B cells. The expression of pro-fibrotic molecules, such as zymogen activator inhibitor 1, thus aggravates the progress of renal fibrosis (83).IR is an independently risk factor in many diseases, it affects renal podocytes. Podocyte foot loss causes partial shedding of the glomerular filtration barrier (GFB), resulting in macromolecular leakage and proteinuria (84, 85). The adipose tissue secretes all components of the RAAS. During Mets, excessive activation of RAAS will lead to increased renal volume load and hyperfiltration, thus damaging the GFB, including endothelial cells, basement membrane, especially the podocytes (67, 86). In obese patients, a large number of lipid droplets can be found in renal innate cells, especially in podocytes. The deposition of lipid droplets leads to the depletion of renal cell energy, and ultimately the apoptosis of intrinsic renal cells, resulting in CKD and even ESRD (85, 87, 88).
5 Diagnosis of MetS-Related Renal Injury
MetS-related kidney disease is renal impairment in patients with MetS that includes glomerular hyperfiltration, eGFR < 60/mL/min per 1.73 m2, proteinuria and/or microalbuminuria, renal tubular dysfunction, ultrasound abnormalities (increased intra-renal resistive indices) (89), and histopathological abnormalities (90). Of course, MetS with CKD is not exactly MetS-related kidney disease. In addition, there is consistent evidence in the literature regarding the association between MetS and kidney stones (91, 92).
Several components of MetS may affect the kidney, or they may be combined to damage the kidney. Therefore, the damage of MetS to the renal tissue structure is also diverse. Kidney pathological characteristics in patients with MetS include glomerulomegaly, podocytopathy, mesangial cell and matrix proliferation, GBM thickening, global sclerosis, segmental sclerosis, tubular atrophy, interstitial fibrosis, and renal vascular injuries (arterial sclerosis and hyalinosis) (93–97). Kidney pathological characteristics for patients with MetS is shown in Figure 2 (98).
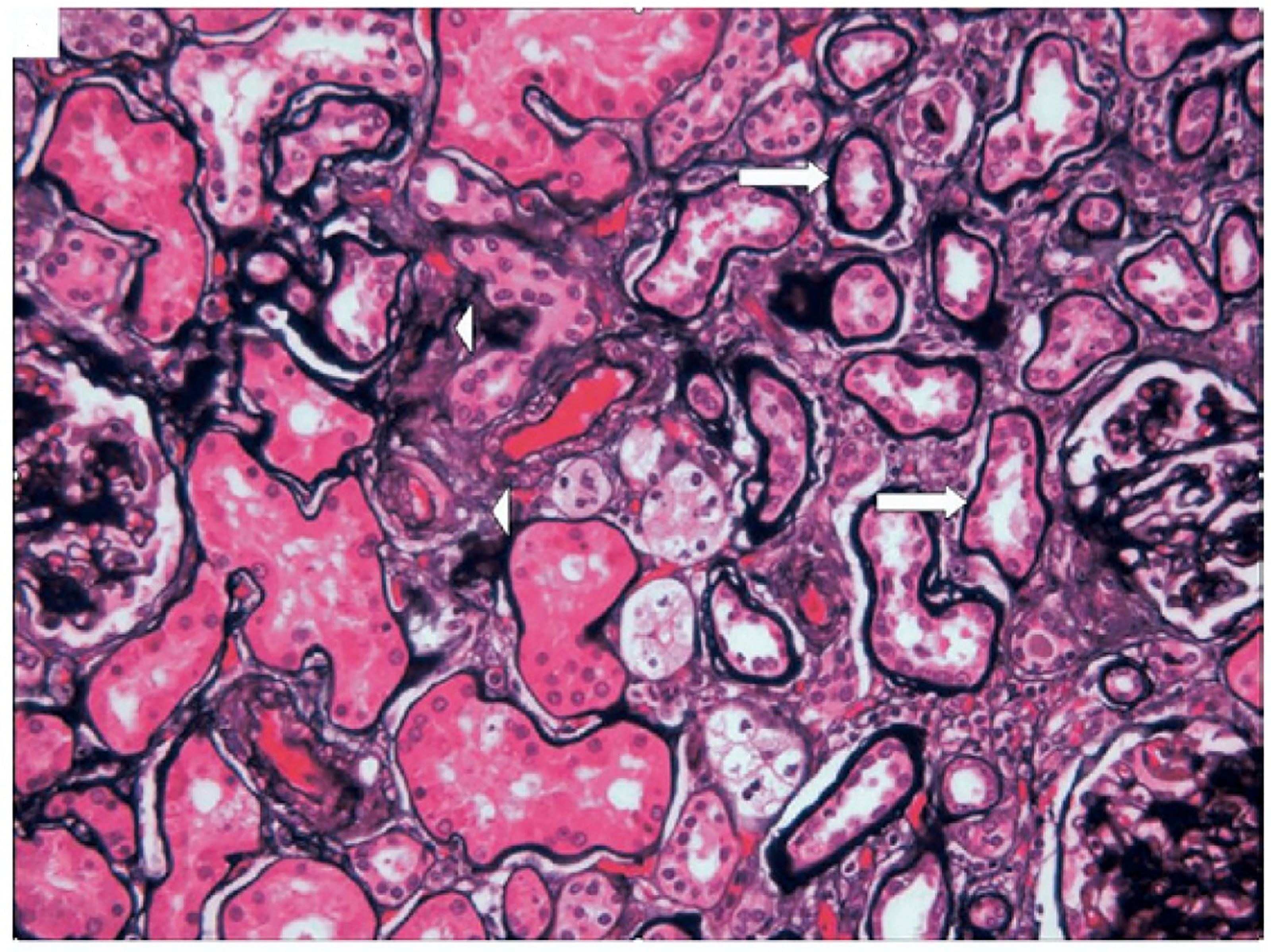
Figure 2 Kidney pathological characteristics for patients with MetS. Tubular atrophy (arrows) and interstitial fibrosis (arrowheads), PASM. This picture is from Mariam P et al., 2009 (98).
Recent studies have found that some new biomarkers are significantly altered in the blood and urine of MetS patients, such as growth differentiation factor -11 (GDF-11). GDF- 11 is an important endocrine factor involved in the metabolic process of the body (99, 100), was found to be negatively correlated with body mass index and WC of MetS patients (101, 102). Growth differentiation factor 15 (GDF-15) is an endocrine factor involved in metabolism, and GDF-15 levels have been found to be significantly increased in elderly MetS patients and has been independently correlated with MetS (103, 104). A study from the Japanese found that urinary A-megalin is associated with the clustering number of MetS traits including hyperhomocysteinemia (105, 106). At present, Urinary podocyte-derived EVs (pEVs) is widely recognized as a specific biomarker for podocyte injury, and studies have found that it is significantly increased in secondary podocytes, such as early diabetic nephropathy, hypertensive nephropathy, and eclampsia (107–109). An animal study found that the urine pEVs of MetS model pigs increased significantly, Meanwhile, kidney histology confirmed the existence of podocyte and mitochondrial damage in MetS pigs. Therefore, urinary pEVs may be an early biomarker for MetS-related kidney injury (88). Whether the above indicators can be used as markers for the diagnosis of MetS-related nephropathy requires further study.
6 Management of MetS-Related Kidney Disease
A study found that patients with uncontrolled MetS had a 3.28 times higher risk of rapid decline in renal function than those who were controlled (110). Therefore, early treatment of MetS is beneficial to prevent and delay the progression of kidney disease. Specific measures include lifestyle change, managing weight control, hypertension, hyperlipidemia, and abnormal blood sugar. If each component of MetS is treated, each component can meet the standard. In recent years, studies have found that intervention targeting gut microbiota, oxidative stress, and inflammatory responses, and stem cell transplantation may help to alleviate Mets related nephropathy.
6.1 Lifestyle Interventions
Lifestyle interventions have always been important means of control MetS, including changing dietary patterns, focusing on a veggie–fruit–grains dietary pattern (111), adhering to aerobic exercise, a diet rich in medium-chain fatty acids and short-chain fatty acids, reasonable sleep, smoking cessation, and avoiding excessive intake of coffee. A study found that excessive coffee consumption (≥3 cups/day) increased the incidence of MetS by 1.5 times (112).
6.2 Weight Loss
Lifestyle interventions such as dietary restriction, change dietary Patterns, aerobic exercise, a diet rich in medium-chain fatty acids and short-chain fatty acids, reasonable sleep, and smoking cessation have always been important means of weight control. Studies have found that after dietary restriction or dietary restriction combined with aerobic exercise, serum creatinine and albuminuria decreased, GFR increased, renal hemodynamics improved, and the risk of kidney stone formation reduced with weight loss (81, 113–115). A study from Taiwan showed that compared with other dietary patterns, MetS patients with veggie–fruit–grains dietary pattern had better parameters of kidney function, including lower serum creatinine, blood urea nitrogen, and serum uric acid levels, and higher eGFR (111). A study of bariatric surgery in adolescents showed that eGFR increased by an average of 26ml/min/1.73m2 three years after surgery. Participants with albuminuria at baseline had improved significantly after operation (116). Similarly, after 6 years of follow-up for weight loss by gastric bypass, it was found that the patient’s metabolic-related indicators were well controlled, cardiovascular events were significantly reduced, and the risk of moderate to severe kidney disease was reduced by 45% (117). However, the existing literature demonstrated that despite its perioperative risks and short-term complications, surgical weight loss has a better long-term prognosis for cardiovascular and renal disease (116, 118–121).
6.3 Antihypertensive Therapy
Patients with hypertension should first adhere to a salt restriction diet. The second is the application of antihypertensive drugs. It is recommended to use angiotensin converting enzyme inhibitor (ACEI) and angiotensin receptor antagonist (ARB) to control hypertension in MetS patients, and this is beneficial to reduce Renin Angiotensin Aldosterone System(RAAS) activation, relieve glomerular hyperfiltration, and reduce proteinuria and hyperuricemia (122).RAAS blocker combined with exercise training can better reduce the hypertension, urine albumin-to-creatinine ratio, and serum creatinine of MetS patients (39, 123). Losartan can stably reduce blood pressure in MetS patients, while maintaining the normal circadian rhythm of blood pressure and achieve renal protection (124).
Animal studies have found that long-term administration of telmisartan can reduce the release of leptin from adipose tissue, thereby reducing proteinuria (125). Calcium channel blocker drugs, benidipine can also reduce the mean arterial pressure and resistance of the renal arterioles in MetS patients, and the urinary protein excretion rate is reduced by 1.5 times (126).
6.4 Lipids Adjustment
Statins have very solid evidence in controlling hyperlipidemia, stabilizing atherosclerosis, and reducing the risk of cardiovascular disease, and have become the first-line drugs for hyperlipidemia in MetS patients (127). Fibrates are also effective in hyperlipidemia, especially in the control of atherosclerotic dyslipidemia (128). In addition, the anti-inflammatory, antioxidant, antithrombotic, anti-fibrotic, and endothelial cell function improvement effects of statins can reduce proteinuria in patients with MetS and delay the progression of kidney disease (129). A study found that the benefit of cardiovascular protection in patients with MetS treated with statins was significantly higher than that without MetS. The eGFR in the MetS increased by 13.7mi/min/1.73m2, which was 2.36 times higher than that in MetS patients without statins (130). In MetS patients with CKD, it is safe to control hyperlipidemia with statins or fibrates. Is the combination of the two drugs safe for MetS with a variety of dyslipidemia? A study found that in MetS patients with CKD treated with low-dose statins combined with fibrate still have high safety and can significantly reduce proteinuria (131). Studies have found that the expression of 11beta-Hydroxysteroid dehydrogenase type 1 (11β-HSD1) is significantly increased in obesity and glucose and lipid metabolism disorders (132). 11β-HSD1 inhibitors can improve the lipid metabolism disorder in MetS (133–135).
6.5 Glucose Control
Metformin, pioglitazone, dipeptidyl peptidase-4 (DPP-4) inhibitors, glucagon-like peptide 1 (GLP-1) receptor agonists, and the sodium-glucose cotransporter 2 (SGLT2) inhibitor have been studied in patients with MetS. Metformin has good safety in the treatment of hyperglycemia and can enhance insulin sensitivity at the same time. It is the best solution for the treatment of MetS glucose metabolism disorder in children (136). Thiazolidinedione increases insulin sensitivity, improves IR and glycemic control, and also significantly reduces blood pressure, increases high-density lipoprotein (HDL), improves endothelial cell function and fibrinolytic activity, and reduces inflammation.
DPP4 inhibitors not only have definite hypoglycemic effect, but also play a protective role in cardiovascular, kidney and important target organs through the following mechanisms. Including regulating the levels of adenosine monophosphate-activated protein kinase and adiponectin levels in MetS mice, reducing inflammatory response and fatty liver (137), DPP-4/incretin axis reducing cardiovascular events (138), and improving hyperglycemia induced vascular lesions through endothelium-dependent Manner (139).
In recent years, GLP-1 receptor agonists have been used more and more widely. They not only exert the hypoglycemic effect, but also do not increase the risk of hypoglycemia. At the same time, they also have the effects of reducing weight, controlling hyperlipidemia, hypertension, and reducing inflammatory reaction, so as to prevent cardiovascular events (140). Liraglutide is a widely used GLP-1 receptor agonist, which has definite efficacy in reducing plaque formation and anti-atherosclerotic action (141).
Similarly, in addition to controlling hyperglycemia, SGLT2 also plays a role in reducing weight loss, controlling hypertension, increasing urinary sodium excretion and reducing edema by reducing sympathetic activity, improving insulin resistance (142), regulating renal sodium and urate transport and excretion in MetS patients (143, 144). It has a definite protective effect on cardiovascular and renal function in type 2 diabetes and MetS patients.
6.6 Chinese Herbal Medicines
In recent years, many Chinese herbal medicines and their extracts have achieved significant efficacy in the treatment of MetS and MetS- related kidney damage. They mainly improve components of the MetS and protect the kidneys by exerting anti-endoplasmic reticulum stress, antioxidant and anti-inflammatory activities.
Endoplasmic reticulum stress is an important pathogenesis of MetS kidney damage. Berberine can reduce urinary microalbumin, the body mass index, and postprandial blood glucose and triglyceride levels in MetS patients by regulating glucose and lipid metabolism, endoplasmic reticulum stress, inflammatory factors, insulin resistance, oxidative stress, and intestinal microbiota (145–148).
Osthol and Hibiscus sabdariffa L (HSL) have significant antioxidant effects. The mechanisms include inhibiting ketohexokinase activity and regulating adipogenesis; reducing oxidative stress by activating nuclear factor-erythroid 2-related factor (Nrf2) (149); promoting an increase in the enzymatic and non-enzymatic antioxidant systems, leading to a reduction in oxygen species (150). Ultimately, it promotes renal repair and delay renal progression in MetS patients.
Pycnogenol is an extract of pine bark, which is rich in bioactive substances such as procyanidins and catechins, has strong anti-inflammatory activities (151), affects endothelial function and reduces blood pressure (152). A randomized controlled study of ramipril alone and ramipril combined with pycnogenol in the treatment of MetS found that the combined treatment group decreased urinary albumin more significantly than the control group, and the renal cortical blood flow rate and renal function improved more significantly (123).
6.7 Other Treatments
6.7.1 Probiotics
Gut microbiota can interfere with host metabolism, and the taxonomic species or abundance of gut microbes are affected by dietary patterns, lifestyles, and drugs. A study found changes in gut microbial taxonomic species in obese patients (153), and gut microbial-derived metabolites may induce subclinical inflammatory processes in MetS patients, leading to target organ damage (154).. Studies have found that probiotics have great benefits for obese and MetS patients by improving the body’s inflammatory state and reducing homocysteine and blood glucose levels (155). A randomized controlled study of patients with MetS found that the severity of MetS was significantly reduced after probiotic supplementation (156). Whether probiotics have a protective effect on MetS-related renal damage needs further study.
6.7.2 Glycine
Glycine is a non-essential amino acid in the human body, but it participates in the important process of metabolism, as well as the process of immune regulation and anti-inflammatory. A study found that plasma the glycine level in MetS patients were lower than that in healthy. Glycine supplementation can improve a variety of clinical symptoms of MetS, such as abnormal glucose metabolism, overweight, hypertension, and hyperlipidemia (157). A study of 60 patients with MetS found that daily supplementation glycine (15 g/day) for 3 months, significantly reduced oxidative stress responses in the treatment group, including superoxide dismutase-specific activity and thiobarbituric acid reactive substances. The patient’s hypertension was significantly improved (158).
6.7.3 Stem Cell Therapy
Mesenchymal stem cells (MSCs) can improve the various disorders of MetS such as abdominal obesity, hyperglycemia, hypertriglyceridemia, and hypertension. MSCs promote renal injury in diabetic mice (159, 160). A superior source of MSCs is from the umbilical cord -MSCs, where the cells can be obtained conveniently, less invasively, and have excellent regenerative and immunosuppressive properties (161). However, whether stem cell therapy can improve the renal tissue structure and function of MetS related renal damage has not been studied.
7 Discussion
With improvements in living standards and changes in lifestyle, the prevalence of MetS is increasing annually. In the future, MetS-related renal damage will become one of the key diseases for CKD prevention and treatment. We should focus on prevention and pay attention to early comprehensive intervention by including the control of body weight, hypertension, hyperglycemia, and hyperlipidemia, requiring all components to meet the criteria. Recently, studies have demonstrated that many new intervention measures, such as anti-inflammatories, antioxidants, the regulation of intestinal microorganisms, inhibiting appetite, stem cell transplantation, and other treatment methods, have achieved preliminary results and are expected to become new treatment targets for MetS related renal injury. This will reduce the prevalence of MetS renal damage and improve the prognosis.
Author Contributions
LL, WT, xP, ET and ZW collected all the literature, carried out the analysis of data and outcome. LL mainly drafted the manuscript. JY revised and approved the final manuscript. Each author contributed important intellectual content during the drafting and revision of the manuscript. All the authors read and approved the final version of the manuscript to be published.
Funding
This work was supported by the National Natural Science Foundation of China (No. 81770682), the Basic and Frontier Research Program of Chongqing (cstc2017jcyjBX0014) and the Chongqing Talent Program Project (cstc2021ycjh-bgzxm0090).
Conflict of Interest
The authors declare that the research was conducted in the absence of any commercial or financial relationships that could be construed as a potential conflict of interest.
Publisher’s Note
All claims expressed in this article are solely those of the authors and do not necessarily represent those of their affiliated organizations, or those of the publisher, the editors and the reviewers. Any product that may be evaluated in this article, or claim that may be made by its manufacturer, is not guaranteed or endorsed by the publisher.
Abbreviations
MetS, Metabolic syndrome; RAAS, Renin Angiotensin Aldosterone System; GFR, glomerular filtration rate; eGFR, estimated glomerular filtration rate; CKD, chronic kidney disease; ESRD, end-stage renal disease; ACEI, angiotensin converting enzyme inhibitor; OS, oxygen species; Nrf2,erythroid 2-related factor; HSL, Hibiscus sabdariffa; HDL, high-density lipoprotein; DPP-4, dipeptidyl peptidase-4; SGLT2,sodium-glucose cotransporter 2; GLP-1,glucagon-like peptide 1; CVD, cardiovascular disease (CVD); ARB, angiotensin receptor antagonist; TMAO, trimethylamine N-oxide; LPS, lipopolysaccharide; MSCs, Mesenchymal stem cells; WHO, World Health Organization; NCEP-ATPIII, National Cholesterol Education/Adult Treatment Panel III; CDC, Chinese Diabetes Society; WC, waist circumference; TG, triglycerides; IR, insulin resistance; AHA/NHLBI, American Heart Association/National Heart, Lung, and Blood Institute; JIS, Joint Interim Statement; FBG, fasting blood glucose; CDA, China Diabetes Association; NHANES, National Health and Nutrition Examination; TGF-β1,transforming growth factor-β1; ORG,obesity-Related Glomerulopathy; RPF, renal plasma flow; ANG II, angiotensin II; TNF-α, tumor necrosis factor α; MCP-1, macrophage chemoattractant protein-1; ROS, reactive oxygen species; NADPH, nicotinamide adenine dinucleotide phosphate oxidase. GFB, glomerular filtration barrier; GDF-11, growth differentiation factor- 11; GDF-15, Growth differentiation factor 15; pEVs, podocyte-derived EVs; 11β-HSD11,11beta-Hydroxysteroid dehydrogenase type 1; MSCs, Mesenchymal stem cells.
References
1. Mente A, Yusuf S, Islam S, McQueen MJ, Tanomsup S, Onen CL, et al. INTERHEART Investigators. Metabolic Syndrome and Risk of Acute Myocardial Infarction a Case-Control Study of 26,903 Subjects From 52 Countries. J Am Coll Cardiol (2010) 55:2390–8. doi: 10.1016/j.jacc.2009.12.053
2. Li X, Li X, Lin H, Fu X, Lin W, Li M, et al. Metabolic Syndrome and Stroke: A Meta-Analysis of Prospective Cohort Studies. J Clin Neurosci (2017) 40:34–8. doi: 10.1016/j.jocn.2017.01.018
3. Stewart LK, Kline JA. Metabolic Syndrome Increases Risk of Venous Thromboembolism Recurrence After Acute Pulmonary Embolism. Ann Am Thorac Soc (2020) 17:821–8. doi: 10.1513/AnnalsATS.201907-518OC
4. Stewart LK, Beam DM, Casciani T, Cameron SJ, Kline JA. Effect of Metabolic Syndrome on Mean Pulmonary Arterial Pressures in Patients With Acute Pulmonary Embolism Treated With Catheter-Directed Thrombolysis. Int J Cardiol (2020) 302:138–42. doi: 10.1016/j.ijcard.2019.12.043
5. Nilsson PM, Laurent S, Cunha PG, Olsen MH, Rietzschel E, Franco OH, et al. Metabolic Syndrome, Arteries REsearch (MARE) Consortium. Characteristics of Healthy Vascular Ageing in Pooled Population-Based Cohort Studies: The Global Metabolic Syndrome and Artery Research Consortium. J Hypertens (2018) 36:2340–9. doi: 10.1097/HJH.0000000000001824
6. Tirandi A, Carbone F, Montecucco F, Liberale L. The Role of Metabolic Syndrome in Sudden Cardiac Death Risk: Recent Evidence and Future Directions. Eur J Clin Invest (2022) 2:e13693. doi: 10.1111/eci.13693
7. Takamiya T, Zaky WR, Edmundowics D, Kadowaki T, Ueshima H, Kuller LH, et al. World Health Organization-Defined Metabolic Syndrome is a Better Predictor of Coronary Calcium Than the Adult Treatment Panel III Criteria in American Men Aged 40–49 Years. Diabetes Care (2004) 12:2977–9. doi: 10.2337/diacare.27.12.2977
8. Expert Panel on Detection, Evaluation and Treatment of High Blood Cholesterol in Adults. Executive Summary of the Third Report of the National Cholesterol Education Program (NCEP) Adult Treatment Panel (ATP III). JAMA (2001) 19:2486–97. doi: 10.1001/jama.285.19.2486
9. Moy FM, Bulgiba A. The Modified NCEP ATP III Criteria Maybe Better Than the IDF Criteria in Diagnosing Metabolic Syndrome Among Malays in Kuala Lumpur. BMC Public Health (2010) 1:1–6. doi: 10.1186/1471-2458-10-678
10. Grundy SM, Cleeman JI, Daniels SR, Donato KA, Eckel RH, Franklin BA. Et Al: Diagnosis and Management of the Metabolic Syndrome: An American Heart Association/National Heart, Lung, and Blood Institute Scientific Statement. Circulation (2005) 112:2735–52. doi: 10.1161/CIRCULATIONAHA.105.169404
11. Ford ES. Prevalence of the Metabolic Syndrome Defined by the International Diabetes Federation Among Adults in the US. Diabetes Care (2005) 11:2745–9. doi: 10.2337/diacare.28.11.2745
12. Chinese Diabetes Society. Clinical Guidelines for Prevention and Treatment of Type 2 Diabetes Mellitus in the Elderly in China (2022 Edition). Zhonghua Nei Ke Za Zhi (2022) 1:12–50. doi: 10.3760/cma.j.cn112138-20211027-00751
13. Alberti KGMM, Eckel RH, Grundy SM, Zimmet PZ, Cleeman JI, Donato KA, et al. Harmonizing the Metabolic Syndrome: A Joint Interim Statement of the International Diabetes Federation Task Force on Epidemiology and Prevention; National Heart, Lung, and Blood Institute; American Heart Association; World Heart Federation; International Atherosclerosis Society; and International Association for the Study of Obesity. Circulation (2009) 120:1640–5. doi: 10.1161/CIRCULATIONAHA.109.192644
14. Xing Y, Xu S, Jia A, Cai J, Zhao M, Guo J, et al. Recommendations for Revision of Chinese Diagnostic Criteria for Metabolic Syndrome: A Nationwide Study. J Diabetes (2018) 3:232–9. doi: 10.1111/1753-0407.12578
15. Saklayen MG. The Global Epidemic of the Metabolic Syndrome. Curr Hypertens Rep (2018) 2:12. doi: 10.1007/s11906-018-0812-z
16. Xavier Moore J, Chaudhary N, Akinyemiju T. Metabolic Syndrome Prevalence by Race/Ethnicity and Sex in the United States, National Health and Nutrition Examination Survey, 1988-2012. Prev Chronic Dis (2017) 14:E24. doi: 10.5888/pcd14.160287
17. Hirode G, Wong RJ. Trends in the Prevalence of Metabolic Syndrome in the United States, 2011-2016. JAMA (2020) 24:2526-2528. doi: 10.1001/jama.2020.4501
18. Li R, Li W, Lun Z, Zhang H, Sun Z, Kanu JS, et al. Prevalence of Metabolic Syndrome in Mainland China: A Meta-Analysis of Published Studies. BMC Public Health (2016) 16:296. doi: 10.1186/s12889-016-2870-y
19. Zhang R, Sun J, Wang C, Wang X, Zhao P, Yuan Y, et al. The Racial Disparities in the Epidemic of Metabolic Syndrome With Increased Age: A Study From 28,049 Chinese and American Adults. Front Public Health (2022) 9:797183. doi: 10.3389/fpubh.2021.797183
20. Jayawardena R, Sooriyaarachchi P, Misra A. Abdominal Obesity and Metabolic Syndrome in South Asians: Prevention and Management. Expert Rev Endocrinol Metab (2021) 6:339–49. doi: 10.1080/17446651.2021.1982381
21. Iqbal Hydrie MZ, Shera AS, Fawwad A, Basit A, Hussain A. Prevalence of Metabolic Syndrome in Urban Pakistan (Karachi): Comparison of Newly Proposed International Diabetes Federation and Modified Adult Treatment Panel III Criteria. Metab Syndr Relat Disord (2009) 2:119–24. doi: 10.1089/met.2008.0055
22. Ravikiran M, Bhansali A, RaviKumar P, Bhansali S, Dutta P, Thakur JS, et al. Prevalence and Risk Factors of Metabolic Syndrome Among Asian Indians: A Community Survey. Diabetes Res Clin Pract (2010) 2:181–8. doi: 10.1016/j.diabres.2010.03.010
23. Morita A, Aiba N, Miyachi M, Watanabe S. The Associations of Eating Behavior and Dietary Intake With Metabolic Syndrome in Japanese: Saku Cohort Baseline Study. J Physiol Anthropol (2020) 1:40. doi: 10.1186/s40101-020-00250-w
24. Lucove J, Vupputuri S, Heiss G, North K, Russell M. Metabolic Syndrome and the Development of CKD in American Indians: The Strong Heart Study. Am J Kidney Dis (2008) 1:21–8. doi: 10.1053/j.ajkd.2007.09.014
25. Ming J, Xu S, Yang C, Gao B, Wan Y, Xing Y, et al. Metabolic Syndrome and Chronic Kidney Disease in General Chinese Adults: Results From the 2007–08 China National Diabetes and Metabolic Disorders Study. Clin Chim Acta (2014) 430:115–20. doi: 10.1016/j.cca.2014.01.004
26. Thomas G, Sehgal AR, Kashyap SR, Srinivas TR, Kirwan JP, Navaneethan SD. Metabolic Syndrome and Kidney Disease: A Systematic Review and Meta-Analysis. Clin J Am Soc Nephrol (2011) 10:2364–73. doi: 10.2215/CJN.02180311
27. Kawamoto R, Akase T, Ninomiya D, Kumagi T, Kikuchi A. Metabolic Syndrome is a Predictor of Decreased Renal Function Among Community-Dwelling Middle-Aged and Elderly Japanese. Int Urol Nephrol (2019) 12:2285–94. doi: 10.1007/s11255-019-02320-0
28. Wu N, Qin Y, Chen S, Yu C, Xu Y, Zhao J, et al. Association Between Metabolic Syndrome and Incident Chronic Kidney Disease Among Chinese: A Nation-Wide Cohort Study and Updated Meta-Analysis. Diabetes Metab Res Rev (2021) 7:e3437. doi: 10.1002/dmrr.3437
29. Alizadeh S, Ahmadi M, Ghorbani Nejad B, Djazayeri A, Shab-Bidar S. Metabolic Syndrome and its Components Are Associated With Increased Chronic Kidney Disease Risk: Evidence From a Meta-Analysis on 11 109 003 Participants From 66 Studies. Int J Clin Pract (2018) 23:e13201. doi: 10.1111/ijcp.13201
30. Kitiyakara C, Yamwong S, Cheepudomwit S, Domrongkitchaiporn S, Unkurapinun N, Pakpeankitvatana V, et al. The Metabolic Syndrome and Chronic Kidney Disease in a Southeast Asian Cohort. Kidney Int (2007) 7:693–700. doi: 10.1038/sj.ki.5002128
31. Ma A, Liu F, Wang C, Liang K, Yan F, Hou X, et al. Both Insulin Resistance and Metabolic Syndrome Accelerate the Progression of Chronic Kidney Disease Among Chinese Adults: Results From a 3-Year Follow-Up Study. Int Urol Nephrol (2018) 12:2239–44. doi: 10.1007/s11255-018-1934-6
32. Kittiskulnam P, Sripaiboonkij Thokanit N, Katavetin P, Susanthitaphong P, Srisawat N, Praditpornsilpa K, et al. The Magnitude of Obesity and Metabolic Syndrome Among Diabetic Chronic Kidney Disease Population: A Nationwide Study. PloS One (2018) 5:e0196332. doi: 10.1371/journal.pone.0196332
33. Wu M, Shu Y, Wang L, Song L, Chen S, Liu Y, et al. Metabolic Syndrome Severity Score and the Progression of CKD. Eur J Clin Invest (2022) 1:e13646. doi: 10.1111/eci.13646
34. DeBoer MD, Filipp SL, Musani SK, Sims M, Okusa MD, Gurka M. Metabolic Syndrome Severity and Risk of CKD and Worsened GFR: The Jackson Heart Study. Kidney Blood Press Res (2018) 2:555–67. doi: 10.1159/000488829
35. Pammer LM, Lamina C, Schultheiss UT, Kotsis F, Kollerits B, Stockmann H, et al. Association of the Metabolic Syndrome With Mortality and Major Adverse Cardiac Events: A Large Chronic Kidney Disease Cohort. J Intern Med (2021) 6:1219–32. doi: 10.1111/joim.13355
36. Ciardullo S, Ballabeni C, Trevisan R, Perseghin G. Metabolic Syndrome, and Not Obesity, Is Associated With Chronic Kidney Disease. Am J Nephrol (2021) 8:666–72. doi: 10.1159/000518111
37. Navaneethan SD, Schold JD, Kirwan JP, Arrigain S, Jolly SE, Poggio ED, et al. Metabolic Syndrome, ESRD, and Death in CKD. Clin J Am Soc Nephrol (2013) 6:945–52. doi: 10.2215/CJN.09870912
38. Lopes HF, Corrêa-Giannella ML, Consolim-Colombo FM, Egan BM. Visceral Adiposity Syndrome. Diabetol Metab Syndr (2016) 8:40. doi: 10.1186/s13098-016-0156-2
39. Ramirez-Jimenez M, Morales-Palomo F, Moreno-Cabañas A, Alvarez-Jimenez L, Ortega JF, Mora-Rodriguez R. Effects of Antihypertensive Medication and High-Intensity Interval Training in Hypertensive Metabolic Syndrome Individuals. Scand J Med Sci Sports (2021) 7:1411–9. doi: 10.1111/sms.13949
40. Correia-Costa L, Morato M, Sousa T, Cosme D, Tiago Guimarães J, Guerra A, et al. Urinary Fibrogenic Cytokines ET-1 and TGF-β1 Are Associated With Urinary Angiotensinogen Levels in Obese Children. Pediatr Nephrol (2016) 3:455–64. doi: 10.1007/s00467-015-3232-1
41. Soofi A, Wolf KI, Emont MP, Qi N, Martinez-Santibanez G, Grimley E, et al. The Kielin/Chordin-Like Protein (KCP) Attenuates High-Fat Diet-Induced Obesity and Metabolic Syndrome in Mice. J Biol Chem (2017) 22:9051–62. doi: 10.1074/jbc.M116.771428
42. Aihara K-I, Ikeda Y, Yagi S, Matsumoto T. Transforming Growth Factor-β1 as a Common Target Molecule for Development of Cardiovascular Diseases, Renal Insufficiency and Metabolic Syndrome. Cardiol Res Pract (2010) 28:2011. doi: 10.4061/2011/175381
43. Eid BG, Neamatallah T, Hanafy A, El-Bassossy HM, Binmahfouz L, Aldawsari HM, et al. Interference With Tgfβ1-Mediated Inflammation and Fibrosis Underlies Reno-Protective Effects of the CB1 Receptor Neutral Antagonists AM6545 and AM4113 in a Rat Model of Metabolic Syndrome. Molecules (2021) 4:866. doi: 10.3390/molecules26040866
44. Kagawa T, Kozai M, Masuda M, Harada N, Nakahashi O, Tajiri M, et al. Sterol Regulatory Element Binding Protein 1 Trans-Activates 25-Hydroxy Vitamin D 3 24-Hydroxylase Gene Expression in Renal Proximal Tubular Cells. Biochem Biophys Res Commun (2018) 2:275–82. doi: 10.1016/j.bbrc.2018.04.058
45. Bertolio R, Napoletano F, Mano M, Maurer-Stroh S, Fantuz M, Zannini A, et al. Sterol Regulatory Element Binding Protein 1 Couples Mechanical Cues and Lipid Metabolism. Nat Commun (2019) 1:1326. doi: 10.1038/s41467-019-09152-7
46. Li L, Yang J, Li F, Gao F, Zhu L, Hao J. FBXW7 Mediates High Glucose−Induced SREBP−1 Expression in Renal Tubular Cells of Diabetic Nephropathy Under PI3K/Akt Pathway Regulation. Mol Med Rep (2021) 4:233. doi: 10.3892/mmr.2021.11872
47. Aljaylani A, Fluitt M, Piselli A, Shepard BD, Tiwari S, Ecelbarger CM. Acid Loading Unmasks Glucose Homeostatic Instability in Proximal-Tubule-Targeted Insulin/Insulin-Like-Growth-Factor-1 Receptor Dual Knockout Mice. Cell Physiol Biochem (2020) 4:682–95. doi: 10.33594/000000248
48. Xi G, Xinchun S, Wai C, White MF, Clemmons DR. Hyperglycemia Induces Vascular Smooth Muscle Cell Dedifferentiation by Suppressing Insulin Receptor Substrate-1-Mediated P53/KLF4 Complex Stabilization. J Biol Chem (2019) 7:2407–21. doi: 10.1074/jbc.RA118.005398
49. Beneit N, Fernández-García CE, Martín-Ventura JL, Perdomo L, Escribano Ó, Michel JB, et al. Expression of Insulin Receptor (IR) A and B Isoforms, IGF-IR, and IR/IGF-IR Hybrid Receptors in Vascular Smooth Muscle Cells and Their Role in Cell Migration in Atherosclerosis. Cardiovasc Diabetol (2016) 15:161. doi: 10.1186/s12933-016-0477-3
50. Xi G, Wai C, White MF, Clemmons DR. Downregulation of Insulin Receptor Substrate 1 During Hyperglycemia Induces Vascular Smooth Muscle Cell Dedifferentiation. J Biol Chem (2017) 5:2009–20. doi: 10.1074/jbc.M116.758987
51. Owusu J, Barrett E. Early Microvascular Dysfunction: Is the Vasa Vasorum a “Missing Link” in Insulin Resistance and Atherosclerosis. Int J Mol Sci (2021) 14:7574. doi: 10.3390/ijms22147574
52. Yang S, Cao C, Deng T, Zhou Z. Obesity-Related Glomerulopathy: A Latent Change in Obesity Requiring More Attention. Kidney Blood Press Res (2020) 4:510–52. doi: 10.1159/000507784
53. Min SH, Kim SH, Jeong IK, Cho HC, Ok Jeong J, Hee Lee J, et al. Independent Association of Serum Aldosterone Level With Metabolic Syndrome and Insulin Resistance in Korean Adults. Korean Circ J (2018) 3:198–208. doi: 10.4070/kcj.2017.0200
54. Muñoz-Durango N, Fuentes CA, Castillo AE, González-Gómez LM, Vecchiola A, Fardella CE, et al. Role of the Renin-Angiotensin-Aldosterone System Beyond Blood Pressure Regulation: Molecular and Cellular Mechanisms Involved in End-Organ Damage During Arterial Hypertension. Int J Mol Sci (2016) 7:797. doi: 10.3390/ijms17070797
55. Helal I, Fick-Brosnahan GM, Schrier RW, Reed-Gitomer B, Schrier RW. Glomerular Hyperfiltration: Definitions, Mechanisms and Clinical Implications. Nat Rev Nephrol (2012) 5:293–300. doi: 10.1038/nrneph.2012.19
56. Remuzzi G, Perico N, Macia M, Piero R. The Role of Renin-Angiotensin-Aldosterone System in the Progression of Chronic Kidney Disease. Kidney Int Suppl (2002) 99:S57–65. doi: 10.1111/j.1523-1755.2005.09911
57. Sharma I, Liao Y, Zheng X, Kanwar YS. New Pandemic: Obesity and Associated Nephropathy. Front Med (Lausanne) (2021) 8:673556. doi: 10.3389/fmed.2021.673556
58. Zhang Y, Chua S Jr. Leptin Function and Regulation. Compr Physiol (2017) 1:351–69. doi: 10.1002/cphy.c160041
59. Vatier C, Jéru I, Fellahi S, Bastard JP, Vigouroux C. Leptin, Adiponectin, Lipodystrophic and Severe Insulin Resistance Syndromes. Ann Biol Clin (Paris) (2020) 3:261–4. doi: 10.1684/abc.2020.1551
60. Sikorska D, Grzymislawska M, Roszak M, Gulbicka P, Korybalska K, Witowski J. Simple Obesity and Renal Function. J Physiol Pharmacol (2017) 2:175–80.
61. Navarro Díaz M. Consequences of Morbid Obesity on the Kidney. Where are We Going? Clin Kidney J (2016) 6:782–7. doi: 10.1093/ckj/sfw094
62. Rasha F, Ramalingam L, Gollahon L, Layeequr Rahman R, Mizanoor Rahman S, Menikdiwela K, et al. Mechanisms Linking the Renin-Angiotensin System, Obesity, and Breast Cancer. Endocr Relat Cance (2019) 12:R653–72. doi: 10.1530/ERC-19-0314
63. Yvan-Charvet L, Quignard-Boulange A. Role of Adipose Tissue Renin-Angiotensin System in Metabolic and Inflammatory Diseases Associated With Obesity. Kidney Int (2011) 79:162–8. doi: 10.1038/ki.2010.391
64. Escasany E, Izquierdo-Lahuerta A, Medina-Gomez G. Underlying Mechanisms of Renal Lipotoxicity in Obesity. Nephron (2019) 1:28–32. doi: 10.1159/000494694
65. Seok Hui K, Kyu Hyang C, Jong Won P, Kyung Woo Y, Jun Young D. Association of Visceral Fat Area With Chronic Kidney Disease and Metabolic Syndrome Risk in the General Population: Analysis Using Multi-Frequency Bioimpedance. Kidney Blood Press Res (2015) 3:223–30. doi: 10.1159/000368498
66. Hall ME, do Carmo JM, da Silva AA, Juncos LA, Wang Z, Hall JE. Obesity, Hypertension, and Chronic Kidney Disease. Int J Nephrol Renovasc Dis (2014) 7:75–88. doi: 10.2147/IJNRD.S39739
67. Mende CW, Einhorn D. FATTY KIDNEY DISEASE: A NEW RENAL AND ENDOCRINE CLINICAL ENTITY? DESCRIBING THE ROLE OF THE KIDNEY IN OBESITY, METABOLIC SYNDROME, AND TYPE 2 DIABETES. Endocr Pract (2019) 8:854–8. doi: 10.4158/EP-2018-0568
68. Ferrara D, Montecucco F, Dallegri F, Carbone F. Impact of Different Ectopic Fat Depots on Cardiovascular and Metabolic Diseases. J Cell Physiol (2019) 12:21630–41. doi: 10.1002/jcp.28821
69. Gu P, Xu A. Interplay Between Adipose Tissue and Blood Vessels in Obesity and Vascular Dysfunction. Rev Endocr Metab Disord (2013) 1:49–58. doi: 10.1007/s11154-012-9230-8
70. Mair KM, Gaw R, MacLean MR. Obesity, Estrogens and Adipose Tissue Dysfunction Implications for Pulmonary Arterial Hypertension. Pulm Circ (2020) 3:2045894020952019. doi: 10.1177/2045894020952023
71. Hosseini A, Marjan Razavi B, Banach M, Hosseinzadeh H. Quercetin and Metabolic Syndrome: A Review. Phytother Res (2021) 10:5352–64. doi: 10.1002/ptr.7144
72. da Silva AA, do Carmo JM, Li X, Wang Z, Mouton AJ, Hall JE. Role of Hyperinsulinemia and Insulin Resistance in Hypertension: Metabolic Syndrome Revisited. Can J Cardiol (2020) 5:671–82. doi: 10.1016/j.cjca.2020.02.066
73. Simeoni M, Borrelli S, Garofalo C, Fuiano G, Esposito C, Comi A, et al. Atherosclerotic-Nephropathy: An Updated Narrative Review. J Nephrol (2021) 1:125–36. doi: 10.1007/s40620-020-00733-0
74. Katsimardou A, Imprialos K, Stavropoulos K, Sachinidis A, Doumas M, Athyros V. Hypertension in Metabolic Syndrome: Novel Insights. Curr Hypertens Rev (2020) 1:12–8. doi: 10.2174/1573402115666190415161813
75. Zhu Q, Scherer PE. Immunologic and Endocrine Functions of Adipose Tissue: Implications for Kidney Disease. Nat Rev Nephrol (2018) 14:105–20. doi: 10.1038/nrneph.2017.157
76. Cabandugama PK, Gardner MJ, Sowers JR. The Renin Angiotensin Aldosterone System in Obesity and Hypertension: Roles in the Cardiorenal Metabolic Syndrome. Med Clin North Am (2017) 1:129–37. doi: 10.1016/j.mcna.2016.08.009
77. Nasrallah MP, Ziyadeh FN. Overview of the Physiology and Pathophysiology of Leptin With Special Emphasis on Its Role in the Kidney. Semin Nephrol (2013) 1:54–65. doi: 10.1016/j.semnephrol.2012.12.005
78. Mao S, Fang L, Liu F, Jiang S, Wu L, Zhang J. Leptin and Chronic Kidney Diseases. Recept Signal Transduct Res (2018) 2:89–94. doi: 10.1080/10799893.2018.1431278
79. Esmaili S, Hemmati M, Karamian M. Physiological Role of Adiponectin in Different Tissues: A Review. Arch Physiol Biochem (2020) 1:67–73. doi: 10.1080/13813455.2018.1493606
80. Markova I, Miklankova D, Hüttl M, Kacer P, Skibova J, Kucera J, et al. The Effect of Lipotoxicity on Renal Dysfunction in a Nonobese Rat Model of Metabolic Syndrome: A Urinary Proteomic Approach. J Diabetes Res (2019) 6:8712979. doi: 10.1155/2019/8712979
81. Fu C-P, Sheu WH-H, Lee I-T, Lee W-J, Wang J-S, Liang K-W, et al. Weight Loss Reduces Serum Monocyte Chemoattractant Protein-1 Concentrations in Association With Improvements in Renal Injury in Obese Men With Metabolic Syndrome. Clin Chem Lab Med (2015) 4:623–9. doi: 10.1515/cclm-2014-0468
82. Leonardo Silveira Rossi J, Barbalho SM, Reverete de Araujo R, Dib Bechara M, Portero Sloan K, Sloan LA. Metabolic Syndrome and Cardiovascular Diseases: Going Beyond Traditional Risk Factors. Diabetes Metab Res Rev (2022) 3:e3502. doi: 10.1002/dmrr.3502
83. Lee H, Jose PA. Coordinated Contribution of NADPH Oxidase- and Mitochondria-Derived Reactive Oxygen Species in Metabolic Syndrome and Its Implication in Renal Dysfunction. Front Pharmacol (2021) 12:670076. doi: 10.3389/fphar.2021.670076
84. Welsh GI, Hale LJ, Eremina V, Jeansson M, Maezawa Y, Lennon R, et al. Insulin Signaling to the Glomerular Podocyte is Critical for Normal Kidney Function. Cell Metab (2010) 12:329–40. doi: 10.1016/j.cmet.2010.08.015
85. D’Agati VD, Chagnac A, de Vries APJ, Levi M, Porrini E, Herman-Edelstein M, et al. Obesity-Related Glomerulopathy: Clinical and Pathologic Characteristics and Pathogenesis. Nat Rev Nephrol (2016) 8:453–71. doi: 10.1038/nrneph.2016.75
86. Martin-Taboada M, Vila-Bedmar R, Medina-Gómez G. From Obesity to Chronic Kidney Disease: How Can Adipose Tissue Affect Renal Function? Nephron (2021) 6:609–13. doi: 10.1159/000515418
87. Miricescu D, Gabriela Balan D, Tulin A, Stiru O, Adela Vacaroiu I, Andrada Mihai D, et al. Impact of Adipose Tissue in Chronic Kidney Disease Development (Review). Exp Ther Med (2021) 5:539. doi: 10.3892/etm.2021.9969
88. Zhang L-H, Zhu X-Y, Eirin A, Aghajani Nargesi A, Woollard JR, Santelli A, et al. Early Podocyte Injury and Elevated Levels of Urinary Podocyte-Derived Extracellular Vesicles in Swine With Metabolic Syndrome: Role of Podocyte Mitochondria. Am J Physiol Renal Physiol (2019) 7:F12–22. doi: 10.1152/ajprenal.00399.2018
89. Bruno RM, Reesink KD, Ghiadoni L. Advances in the non-Invasive Assessment of Vascular Dysfunction in Metabolic Syndrome and Diabetes: Focus on Endothelium, Carotid Mechanics and Renal Vessels. Nutr Metab Cardiovasc Dis (2017) 2:121–8. doi: 10.1016/j.numecd.2016.09.004
90. Alexander MP, Patel TV, Farag YMK, Florez A, Rennke HG, Singh AK. Kidney Pathological Changes in Metabolic Syndrome: A Cross-Sectional Study. Am J Kidney Dis (2009) 53:751–9. doi: 10.1053/j.ajkd.2009.01.255
91. Chang CW, Ke HL, Lee JI, Lee YC, Jhan JH, Wang HS, et al. Metabolic Syndrome Increases the Risk of Kidney Stone Disease: A Cross-Sectional and Longitudinal Cohort Study. J Pers Med (2021) 11:1154. doi: 10.3390/jpm11111154
92. Qiu F, Xu Y, Ji X, Pu J, Zhou J, Huang. Incidence Y. And Correlation of Metabolic Syndrome and Kidney Stones in a Healthy Screening Population. Transl Androl Urol (2021) 9:3646–55. doi: 10.21037/tau-21-689
93. Trujillo J, Irasema Chirino Y, Martínez-Tagüeña N, Pedraza-Chaverri J. Renal Damage in the Metabolic Syndrome (MetSx): Disorders Implicated. Eur J Pharmacol (2018) 5:554–68. doi: 10.1016/j.ejphar.2017.11.032
94. Ohashi Y, Thomas G, Nurko S, Stephany B, Fatica R, Chiesa A, et al. Association of Metabolic Syndrome With Kidney Function and Histology in Living Kidney Donors. Am J Transplant (2013) 9:2342–51. doi: 10.1111/ajt.12369
95. Farahani RA, Afarideh M, Zhu X-Y, Tang H, Jordan KL, Saadiq IM, et al. Percutaneous Transluminal Renal Angioplasty Attenuates Poststenotic Kidney Mitochondrial Damage in Pigs With Renal Artery Stenosis and Metabolic Syndrome. J Cell Phyiol (2021) 5:4036–49. doi: 10.1002/jcp.30146
96. Braz Gonçalves L, Amante Miot H, Aparecida Custódio Domingues M, Claudino Oliveira C. Autopsy Patients With Obesity or Metabolic Syndrome as Basic Cause of Death: Are There Pathological Differences Between These Groups? Clin Med Insights Pathol (2018) 11:1179555718791575. doi: 10.1177/1179555718791575
97. Rodríguez-Rodríguez AE, Donate-Correa J, Luis-Lima S, Díaz-Martín L, Rodríguez-González C, Antonio Pérez-Pérez J, et al. Obesity and Metabolic Syndrome Induce Hyperfiltration, Glomerulomegaly, and Albuminuria in Obese Ovariectomized Female Mice and Obese Male Mice. Menopause (2021) 211:1296–306. doi: 10.1097/GME.0000000000001842
98. Alexander MP, Patel TV, Farag YM, Florez A, Rennke HG. Singh AK.Kidney Pathological Changes in Metabolic Syndrome: A Cross-Sectional Study. Am J Kidney Dis (2009) 5:751–9. doi: 10.1053/j.ajkd.2009.01.255
99. Lu B, Zhong J, Pan J, Yuan X, Ren M, Jiang L, et al. Gdf11 Gene Transfer Prevents High Fat Diet-Induced Obesity and Improves Metabolic Homeostasis in Obese and STZ-Induced Diabetic Mice. J Transl Med (2019) 1:422. doi: 10.1186/s12967-019-02166-1
100. Fife E, Kostka J, Kroc Ł, Guligowska A, Pigłowska M, Sołtysik B, et al. Relationship of Muscle Function to Circulating Myostatin, Follistatin and GDF11 in Older Women and Men. BMC Geriatr (2018) 1:200–9. doi: 10.1186/s12877-018-0888-y
101. Añón-Hidalgo J, Catalán V, Rodríguez A, Ramírez B, Silva C, Galofré JC, et al. Circulating GDF11 Levels Are Decreased With Age But are Unchanged With Obesity and Type 2 Diabetes. Aging (Albany NY) (2019) 6:1733–44. doi: 10.18632/aging.101865
102. Xu B, Huang Y, Zhang R, Tang M, He Z, Jin L, et al. Serum Growth Differentiation Factor 11 Is Closely Related to Metabolic Syndrome in a Chinese Cohort. J Diabetes Investig (2021) 2:234–43. doi: 10.1111/jdi.13337
103. Carballo-Casla A, García-Esquinas E, Buño-Soto A, Struijk EA, López-García E, Rodríguez-Artalejo F, et al. Metabolic Syndrome and Growth Differentiation Factor 15 in Older Adults. Geroscience (2021) 7:867-80. doi: 10.1007/s11357-021-00370-w
104. Lau ES, Paniagua SM, Zarbafian S, Hoffman U, Long MT, Hwang SJ, et al. Cardiovascular Biomarkers of Obesity and Overlap With Cardiometabolic Dysfunction. J Am Heart Assoc (2021) 14:e020215. doi: 10.1161/JAHA.120.020215
105. Kabasawa K, Hosojima M, Ito Y, Tanaka J, Hara M, Nakamura K, et al. Association of Metabolic Syndrome Traits With Urinary Biomarkers in Japanese Adults. Diabetol Metab Syndr (2022) 1:9. doi: 10.1186/s13098-021-00779-5
106. Moon H, Ko H-J, Kim A-S. Hyperhomocysteinemia Concurrent With Metabolic Syndrome Is Independently Associated With Chronic Kidney Disease Among Community-Dwelling Adults in an Urban Korean Population. Int J Environ Res Public Health (2020) 18:6810. doi: 10.3390/ijerph17186810
107. Burger D, Thibodeau J-F, Holterman CE, Burns KD, Touyz RM, Kennedy CRJ. Urinary Podocyte Microparticles Identify Prealbuminuric Diabetic Glomerular Injury. J Am Soc Nephrol (2014) 7:1401–7. doi: 10.1681/ASN.2013070763
108. Hyo Kwon S, Woollard JR, Saad A, Garovic VD, Zand L, Jordan KL, et al. Elevated Urinary Podocyte-Derived Extracellular Microvesicles in Renovascular Hypertensive Patients. Nephrol Dial Transplant (2017) 5:800–7. doi: 10.1093/ndt/gfw077
109. Gilani SI, Dolberg Anderson U, Jayachandran M, Weissgerber TL, Zand L, White WM, et al. Urinary Extracellular Vesicles of Podocyte Origin and Renal Injury in Preeclampsia. J Am Soc Nephrol (2017) 11:3363–72. doi: 10.1681/ASN.2016111202
110. Lin JH, Wu HC, Huang WH, Lu CL, Cheng MH, Wang HT, et al. Association Between Management of Metabolic Syndrome and Progression of Early-Stage Chronic Kidney Disease: An Observational Cohort Study. Ren Fail (2015) 1:29–36. doi: 10.3109/0886022X
111. Syauqy A, Hsu CY, Lee HA, Rau HH, Chao JC. Association Between Dietary Patterns and Kidney Function Parameters in Adults With Metabolic Syndrome: A Cross-Sectional Study. Nutrients (2020) 1:40. doi: 10.3390/nu13010040
112. Stutz B, Ahola AJ, Harjutsalo V, Forsblom C. Association Between Habitual Coffee Consumption and Metabolic Syndrome in Type 1 Diabetes. Nutr Metab Cardiovasc Dis (2018) 5:470–6. doi: 10.1016/j.numecd.2018.01.011
113. Straznicky NE, Grima MT, Lambert EA, Eikelis N, Dawood T, Lambert GW, et al. Exercise Augments Weight Loss Induced Improvement in Renal Function in Obese Metabolic Syndrome Individuals. J Hypertens (2011) 3:553–64. doi: 10.1097/HJH.0b013e3283418875
114. Sasaki Y, Kohjimoto Y, Iba A, Matsumura N, Hara I. Weight Loss Intervention Reduces the Risk of Kidney Stone Formation in a Rat Model of Metabolic Syndrome. Int J Urol (2015) 4:404–9. doi: 10.1111/iju.12691
115. Navaneethan SD, Yehnert H, Moustarah F, Schreiber MJ, Schauer PR, Beddhu S. Weight Loss Interventions in Chronic Kidney Disease: A Systematic Review and Meta-Analysis. Clin J Am Soc Nephrol (2009) 10:1565–74. doi: 10.2215/CJN.02250409
116. Nehus EJ, Khoury JC, Inge TH, Xiao N, Jenkins TM, Moxey-Mims MM, et al. Kidney Outcomes Three Years After Bariatric Surgery in Severely Obese Adolescents. Kidney Int (2017) 2:451–8. doi: 10.1016/j.kint.2016.09.031
117. Aminian A, Daigle CR, Romero-Talamás H, Kashyap SR, Kirwan JP, Brethauer SA, et al. Risk Prediction of Complications of Metabolic Syndrome Before and 6 Years After Gastric Bypass. Surg Obes Relat Dis (2014) 4:576–82. doi: 10.1016/j.soard.2014.01.025
118. Morales E, Porrini E, Martin-Taboada M, Luis-Lima S, Vila-Bedmar R, González de Pablos I, et al. Renoprotective Role of Bariatric Surgery in Patients With Established Chronic Kidney Disease. Clin Kidney J (2020) 9:2037–46. doi: 10.1093/ckj/sfaa266
119. Nehus E, Mitsnefes M. Childhood Obesity and the Metabolic Syndrome. Pediatr Clin North Am (2019) 1:31–43. doi: 10.1016/j.pcl.2018.08.004
120. Chang AR, Chen Y, Still C, Craig Wood G, Lester Kirchner H, Lewis M, et al. Bariatric Surgery is Associated With Improvement in Kidney Outcomes. Kidney Int (2016) 1:164–71. doi: 10.1016/j.kint.2016.02.039
121. Cohen JB, Cohen DL. Cardiovascular and Renal Effects of Weight Reduction in Obesity and the Metabolic Syndrome. Curr Hypertens Rep (2015) 5:34. doi: 10.1007/s11906-015-0544-2
122. Masajtis-Zagajewska A, Majer J, Nowicki M. Losartan and Eprosartan Induce a Similar Effect on the Acute Rise in Serum Uric Acid Concentration After an Oral Fructose Load in Patients With Metabolic Syndrome. J Renin Angiotensin Aldosterone Syst (2021) 25:2214978. doi: 10.1155/2021/2214978
123. Stuard S, Belcaro G, Cesarone MR, Ricci A, Dugall M, Cornelli U, et al. Kidney Function in Metabolic Syndrome May Be Improved With Pycnogenol. Panminerva Med (2010) Suppl 1:27–32.
124. Beloborodova AV, Morozova TE, Shilov EM. An Experience of the Use of Angiotensin II Receptor Blocker Losartan in Patients With Metabolic Syndrome and Chronic Kidney Disease. Kardiologiia (2010) 8:32–6.
125. Li H, Li M, Liu P, Wang Y, Zhang H, Li H, et al. Telmisartan Ameliorates Nephropathy in Metabolic Syndrome by Reducing Leptin Release From Perirenal Adipose Tissue. Hypertension (2016) 2:478–90. doi: 10.1161/HYPERTENSIONAHA.116.07008
126. Uzu T, Nishimura M, Fujii T, Sakaguchi M, Kanasaki M, Isshiki K, et al. Benidipine Attenuates Glomerular Hypertension and Reduces Albuminuria in Patients With Metabolic Syndrome. Hypertens Res (2007) 2:161–5. doi: 10.1291/hypres.30.161
127. Agrawal V, Shah A, Rice C, Barry A, Peter A. Impact of Treating the Metabolic Syndrome on Chronic Kidney Disease. Nat Rev Nephrol (2009) 9:520–8. doi: 10.1038/nrneph.2009.114
128. Malur P, Menezes A, DiNicolantonio JJ, O’Keefe JH, Lavie CJ. The Microvascular and Macrovascular Benefits of Fibrates in Diabetes and the Metabolic Syndrome: A Review. Mo Med (2017) 6:464–71.
129. Velarde GP, Choudhary N, Bravo-Jaimes K, Smotherman C, Sherazi S, Kraemer DF. Effect of Atorvastatin on Lipogenic, Inflammatory and Thrombogenic Markers in Women With the Metabolic Syndrome. Nutr Metab Cardiovasc Dis (2021) 2:634–40. doi: 10.1016/j.numecd.2020.10.002
130. Athyros VG, Mikhailidis DP, Liberopoulos EN, Kakafika AI, Karagiannis A, Papageorgiou AA, et al. Effect of Statin Treatment on Renal Function and Serum Uric Acid Levels and Their Relation to Vascular Events in Patients With Coronary Heart Disease and Metabolic Syndrome: A Subgroup Analysis of the GREek Atorvastatin and Coronary Heart Disease Evaluation (GREACE) Study. Nephrol Dial Transplant (2007) 1:118–27. doi: 10.1093/ndt/gfl538
131. Monhart V. Treatment of Dyslipidemia in Patients With Metabolic Syndrome and Chronic Kidney Disease. Vnitr Lek (2009) 7–8:671–8.
132. Anagnostis P, Katsiki N, Adamidou F, Athyros VG, Karagiannis A, Kita M, et al. 11beta-Hydroxysteroid Dehydrogenase Type 1 Inhibitors: Novel Agents for the Treatment of Metabolic Syndrome and Obesity-Related Disorders? Metabolism (2013) 1:21–33. doi: 10.1016/j.metabol.2012.05.002
133. Oh H, Jeong K-H, Young Han H, Joo Son H, Sung Kim S, Jung Lee H, et al. A Potent and Selective 11β-Hydroxysteroid Dehydrogenase Type 1 Inhibitor, SKI2852, Ameliorates Metabolic Syndrome in Diabetic Mice Models. Eur J Pharmacol (2015) 5:139–48. doi: 10.1016/j.ejphar.2015.10.042
134. Schnackenberg CG, Costell MH, Krosky DJ, Cui J, Wu CW, Hong VS, et al. Chronic Inhibition of 11 Beta -Hydroxysteroid Dehydrogenase Type 1 Activity Decreases Hypertension, Insulin Resistance, and Hypertriglyceridemia in Metabolic Syndrome. BioMed Res Int (2013) 427640:1-10. doi: 10.1155/2013/427640
135. Nagasawa K, Matsuura N, Takeshita Y, Ito S, Sano Y, Yamada Y, et al. Attenuation of Cold Stress-Induced Exacerbation of Cardiac and Adipose Tissue Pathology and Metabolic Disorders in a Rat Model of Metabolic Syndrome by the Glucocorticoid Receptor Antagonist RU486. Nutr Diabetes (2016) 4:e207. doi: 10.1038/nutd.2016.14
136. Tagi VM, Samvelyan S, Chiarelli F. Metabolic Syndrome in Children. Minerva Pediatr (2020) 4:312–25. doi: 10.23736/S0026-4946.20.05834-X
137. Prakash S, Rai U, Kosuru R, Tiwari V, Singh S. Amelioration of Diet-Induced Metabolic Syndrome and Fatty Liver With Sitagliptin via Regulation of Adipose Tissue Inflammation and Hepatic Adiponectin/AMPK Levels in Mice. Biochimie (2020) 168:198–209. doi: 10.101/j.biochi.2019.11.005
138. Ishii M, Shibata R, Kondo K, Kambara T, Shimizu Y, Tanigawa T, et al. Vildagliptin Stimulates Endothelial Cell Network Formation and Ischemia-Induced Revascularization via an Endothelial Nitric-Oxide. Synthase-Dependent Mechanism. J Biol Chem (2014) 289:27235–45. doi: 10.1074/jbc.M114.557835
139. Cicek FA, Tokcaer-Keskin Z, Ozcinar E, Bozkus Y, Can Akcali K, Turan B. Di-Peptidyl Peptidase-4 Inhibitor Sitagliptin Protects Vascular Function in Metabolic Syndrome: Possible Role of Epigenetic Regulation. Mol Biol Rep (2014) 41:4853–63. doi: 10.1007/s11033-014-3392-2
140. Rizzo M, Nikolic D, Patti AM, Mannina C, Montalto G, McAdams BS, et al. GLP-1 Receptor Agonistsand Reduction of Cardiometabolic Risk: Potential Underlying Mechanisms. Biochim Biophys Acta Mol Basis Dis (2018) 1864:2814–21. doi: 10.1016/j.bbadis.2018.05.012
141. Nauck MA, Quast DR, Wefers J, Meier JJ. GLP-1 Receptor Agonists in the Treatment of Type 2 Diabetes—State-Of-the-Art. Mol Metab (2021) 46:101102. doi: 10.1016/j.molmet.2020.101102
142. Cheng L, Fu Q, Zhou L, Fan Y, Liu F, Fan Y, et al. Dapagliflozin, Metformin, Monotherapy or Both in Patients With Metabolic Syndrome. Sci Rep (2021) 1:24263. doi: 10.1038/s41598-021-03773-z
143. Ng H-Y, Leung F-F, Kuo W-H, Lee W-C, Lee C-T. Dapagliflozin and Xanthine Oxidase Inhibitors Improve Insulin Resistance and Modulate Renal Glucose and Urate Transport in Metabolic Syndrome. Clin Exp Pharmacol Physiol (2021) 12:1603–12. doi: 10.1111/1440-1681.13574
144. Herat LY, Matthews J, Azzam O, Schlaich MP, Matthews VB. Targeting Features of the Metabolic Syndrome Through Sympatholytic Effects of SGLT2 Inhibition. Curr Hypertens Rep (2022) 2:67–74. doi: 10.1007/s11906-022-01170-z
145. Och A, Och M, Nowak R, Dominika P, Rafał P. Berberine, a Herbal Metabolite in the Metabolic Syndrome: The Risk Factors, Course, and Consequences of the Disease. Molecules (2022) 4:1351. doi: 10.3390/molecules27041351
146. Li J, Li J, Ni J, Zhang C, Jia J, Wu G, et al. Berberine Relieves Metabolic Syndrome in Mice by Inhibiting Liver Inflammation Caused by a High-Fat Diet and Potential Association With Gut Microbiota. Front Microbiol (2022) 12:752512. doi: 10.3389/fmicb.2021.752512
147. Cao C, Su M. Effects of Berberine on Glucose-Lipid Metabolism, Inflammatory Factors and Insulin Resistance in Patients With Metabolic Syndrome. Exp Ther Med (2019) 4:3009–14. doi: 10.3892/etm.2019.7295
148. Jamialahmadi T, Guest PC, Tasbandi A, Al-Rasadi K, Sahebkar A. Measuring the Effects of Berberine on Serum Prooxidant-Antioxidant Balance in Metabolic Syndrome. Methods Mol Biol (2022) 2343:309–18. doi: 10.1007/978-1-0716-1558-4_22
149. García-Arroyo FE, Gonzaga-Sánchez G, Tapia E, Muñoz-Jiménez I, Manterola-Romero L, Osorio-Alonso H, et al. Osthol Ameliorates Kidney Damage and Metabolic Syndrome Induced by a High-Fat/High-Sugar Diet. Int J Mol Sci (2021) 5:2431. doi: 10.3390/ijms22052431
150. Leao Rodríguez-Fierros F, Guarner-Lans V, Soto ME, Manzano-Pech L, Díaz-Díaz E, Soria-Castro E, et al. Modulation of Renal Function in a Metabolic Syndrome Rat Model by Antioxidants in Hibiscus Sabdariffa L. Molecules (2021) 7:2074. doi: 10.3390/molecules26072074
151. Canali R, Comitato R, Schonlau F, Virgili F. The Anti-Inflammatory Pharmacology of Pycnogenol in Humans Involves COX-2 and 5-LOX mRNA Expression in Leukocytes. Int Immunopharmacol (2009) 9:1145–9. doi: 10.1016/j.intimp.2009.06.001
152. Zibadi S, Rohdewald PJ, Park D, Watson RR. Reduction of Cardiovascular Risk Factors in Subjects With Type 2 Diabetes by Pycnogenol Supplementation. Nutr Res (2008) 20:315–20. doi: 10.1016/j.nutres.2008.03.003
153. Turnbaugh PJ, Hamady M, Yatsunenko T, Cantarel BL, Duncan A, Ley RE, et al. A Core Gut Microbiome in Obese and Lean Twins. Nature (2009) 7228:480–4. doi: 10.1038/nature07540
154. Croci S, D’Apolito LI, Gasperi V, Catani MV, Savini I. Dietary Strategies for Management of Metabolic Syndrome: Role of Gut Microbiota Metabolites. Nutrients (2021) 5:1389. doi: 10.3390/nu13051389
155. Fahed G, Aoun L, Bou Zerdan M, Allam S, Bou Zerdan M, Bouferraa Y, et al. Metabolic Syndrome: Updates on Pathophysiology and Management in 2021. Int J Mol Sci (2022) 2:786. doi: 10.3390/ijms23020786
156. Bernini LJ, Simão AN, Alfieri DF, Lozovoy MA, Mari NL, de Souza CH, et al. Beneficial Effects of Bifidobacterium Lactis on Lipid Profile and Cytokines in Patients With Metabolic Syndrome: A Randomized Trial. Effects of Probiotics on Metabolic Syndrome. Nutrition (2016) 6:716–9. doi: 10.1016/j.nut.2015.11.001
157. Imenshahidi M, Hossenzadeh H. Effects of Glycine on Metabolic Syndrome Components: A Review. J Endocrinol Invest (2022) 5:927–39. doi: 10.1007/s40618-021-01720-3
158. Díaz-Flores M, Cruz M, Duran-Reyes G, Munguia-Miranda C, Loza-Rodríguez H, Pulido-Casas E. Oral Supplementation With Glycine Reduces Oxidative Stress in Patients With Metabolic Syndrome, Improving Their Systolic Blood Pressure. Can J Physiol Pharmacol (2013) 10:855–60. doi: 10.1139/cjpp-2012-0341
159. Maldonado M, Huang T, Yang L, Xu L, Ma L. Human Umbilical Cord Wharton Jelly Cells Promote Extra-Pancreatic Insulin Formation and Repair of Renal Damage in STZ-Induced Diabetic Mice. Cell Commun Signal (2017) 15:43. doi: 10.1186/s12964-017-0199-5
160. Hwa Lee R, Jeong Seo M, Reger RL, Spees JL, Pulin AA, Olson SD, et al. Multipotent Stromal Cells From Human Marrow Home to and Promote Repair of Pancreatic Islets and Renal Glomeruli in Diabetic NOD/scid Mice. Proc Natl Acad Sci USA (2006) 103:17438–43. doi: 10.1073/pnas.0608249103
Keywords: metabolic syndrome, chronic kidney disease, diagnosis, pathological characteristics, therapeutics
Citation: Lin L, Tan W, Pan X, Tian E, Wu Z and Yang J (2022) Metabolic Syndrome-Related Kidney Injury: A Review and Update. Front. Endocrinol. 13:904001. doi: 10.3389/fendo.2022.904001
Received: 25 March 2022; Accepted: 09 May 2022;
Published: 23 June 2022.
Edited by:
Congjuan Luo, The Affiliated Hospital of Qingdao University, ChinaReviewed by:
Kun Ling Ma, Zhejiang University, ChinaGuangyan Cai, Chinese PLA General Hospital, China
Copyright © 2022 Lin, Tan, Pan, Tian, Wu and Yang. This is an open-access article distributed under the terms of the Creative Commons Attribution License (CC BY). The use, distribution or reproduction in other forums is permitted, provided the original author(s) and the copyright owner(s) are credited and that the original publication in this journal is cited, in accordance with accepted academic practice. No use, distribution or reproduction is permitted which does not comply with these terms.
*Correspondence: Jurong Yang, NjUwMjMwQGhvc3BpdGFsLmNxbXUuZWR1LmNu