- Department of Endocrinology and Metabolism, The Second Affiliated Hospital of Nanchang University, Nanchang, China
Background: Evidence on the relationship between heart rate variability (HRV) and albumin-to-creatinine ratio (ACR) combined with estimated glomerular filtration rate (eGFR) in patients with type 2 diabetes mellitus (T2DM) is rare. Thus, this study aimed to investigate the relationship between heart rate variability and the risk of diabetic kidney disease (DKD) progression in diabetes patients.
Method: Overall, 747 T2DM patients who were admitted to the Second Affiliated Hospital of Nanchang University underwent 24-hour dynamic electrocardiograms for HRV analysis. Time-domain HRV measures included mean heart rate, standard deviation of the R-R interval (SDNN), SDNN index, root mean squared difference of successive RR intervals (RMSSD), and percent of adjacent RR intervals with a difference greater than 50 ms (PNN50). Frequency-domain measures included low frequency (LF), very low frequency (VLF), high frequency (HF) components and LF-to-HF ratio. The risk of DKD progression was determined by combining ACR and eGFR and stratified as low risk (Group A), moderately increased risk (Group B), high risk (Group C), and very high risk (Group D) based on the Kidney Disease: Improving Global Outcomes guidelines.
Result: There were significant differences in HRV parameters among the four risk groups (SDNN: 113 ms vs 109 ms vs 101 ms vs 81 ms, P<0.01; LF: 240.2 ms2 vs 241.1 ms2 vs 155.2 ms2 vs 141.9 ms2, P<0.01; LF-to-HF ratio: 1.70 vs 1.24 vs 1.12 vs 0.93, P<0.01; VLF: 723.7 ms2 vs 601.1 ms2 vs 446.4 ms2 vs 356.3 ms2, P<0.01). A very high risk of DKD progression was significantly associated with a lower SDNN (β=-19.5, 95% CI: -30.0 to -10.0, P<0.01), and moderately increased, high, and very high risks were associated with lower LF-to-HF ratio and VLF (P<0.05). Logistic regression analysis showed that group D had a higher risk of reduced SDNN, LF-to-HF ratio, and VLF compared with group A after adjusting for systolic blood pressure, glycated haemoglobin, haemoglobin, high-density lipoprotein cholesterol, and age (odds ratio (95% CI): 0.989 (0. 983–0.996), 0.674 (0.498–0.913), and 0.999 (0.999–1.000), respectively).
Conclusion: Cardiac autonomic dysfunction is associated with a risk of DKD progression in adults with T2DM, and reduced heart rate variability increased such risk. Thus, HRV screening may be necessary in patients with T2DM, especially those with high proteinuria.
Introduction
Cardiac autonomic neuropathy (CAN)is a common chronic complication of diabetes, occurring in 25%–75% of patients with type 2 diabetes (T2DM). CAN mainly presents as resting tachycardia, orthostatic hypotension, syncope, and cardiac dysfunction (1). However, CAN may remain in a subclinical state for several years; the patient may be asymptomatic, and a decrease in heart rate variability (HRV) may only be found on physical examination. HRV is a non-invasive indicator for detecting and quantifying CAN in diabetes patients, reflecting the dynamic balance of the sympathetic and parasympathetic nervous systems in regulating cardiovascular function. Further, the HRV test has higher sensitivity for detecting cardiac autonomic changes than conventional tests based on dynamic cardiovascular operations, thus making it helpful for the early diagnosis of CAN (2, 3).
Albuminuria is considered to be a marker of systemic endothelial dysfunction in diabetes patients, and early detection of microalbuminuria can prevent end-stage renal disease and cardiovascular complications of diabetes. Previous studies have shown that CAN is associated with higher albumin-to-creatinine ratio (ACR) and poor glycaemic control (4, 5). Further, a decrease in estimated glomerular filtration rate (eGFR) was found to be strongly associated with an increased risk of cardiovascular disease and death (6). However, evidence on whether cardiac autonomic function is associated with renal dysfunction based on changes in microalbuminuria and glomerular filtration rate is rare. The Kidney Disease: Improving Global Outcomes (KDIGO) recommends combining chronic kidney disease (CKD) staging and albuminuria category to assess the risk of progression of diabetic kidney disease (DKD) (7). Although CAN is a risk factor for progression of kidney disease in diabetes patients (8, 9), there are few studies on the relationship of HRV with ACR combined with eGFR in patients with T2DM. Therefore, this study aimed to investigate the relationship of HRV with the risk of DKD progression. Towards this goal, the risk of progression of nephropathy in T2DM patients was classified according to CKD stage and albuminuria category, and HRV was measured.
Material and methods
Study Design and Patients
This retrospective cohort study was approved by the Information Management Organization of the Second Affiliated Hospital of Nanchang University, and the need for informed consent was waived owing to the retrospective nature of the study. We included T2DM patients (mean age: 68.6 ± 11.5 years [range: 34–92 years]) who were hospitalised between January 2017 and October 2021. The exclusion criteria were type 1 diabetes mellitus, nephrotic syndrome, presence of active urinary sediment (erythrocytes, leukocytes, or cell casts), refractory hypertension, and incomplete data. After excluding 98 patients, 747 T2DM patients (337 males and 410 females) were included the analysis. The diagnosis and history of T2DM were carefully reviewed by at least two authors. Incident T2DM was defined as a diagnosis of diabetes after discharge and glycated haemoglobin (HbA1c) ≥ 6.5% or fasting blood glucose ≥ 7 mmol/L or random blood glucose ≥ 11.1 mmol/L, or have received insulin or oral hypoglycaemic drug treatment. Data were obtained from the Big Data Centre Management System/Electronic Medical Record Cohort Database of the Second Affiliated Hospital of Nanchang University.
Assessments
Height and weight were measured by standard methods, and body mass index (BMI) was calculated by dividing weight (kg) by the square of height (m). Blood pressure was measured using OMRON HEM-7130 blood pressure monitor with an appropriately sized cuff. Blood and urine samples were obtained after an overnight fast within 0 to 5 days prior to 24-hour dynamic electrocardiograms. All blood and urine samples were analysed at the Laboratory Centre of the Second Affiliated Hospital of Nanchang University. Lipid profile measurements (cholesterol, high-density lipoprotein cholesterol, low-density lipoprotein cholesterol, triglycerides) were measured using Automatic biochemical analyser (Cobas c800, Roche, Switzerland).
Whole blood haemoglobin was detected with a whole blood cell analyser (Bc6800, Mindray, Xiamen Haifei Biotechnology Co., Ltd, China), and HbA1c was measured using high-performance liquid chromatography (4500MD, AB SCIEX, USA). Urine albumin was measured using laser immunonephelometry (Siemens BN ProSpec), and urine creatinine was measured using a chromatographic stable isotope dilution electrospray mass spectrometry-mass spectrometry method. ACR (mg/mmol) was calculated as the urine albumin concentration (mg/L) divided by the urine creatinine (mmol/L). eGFR was calculated using the formula: eGFR (mL/min/1.73 m2) = 42 ×height (cm)/plasma creatinine (µmol/L).
The patients underwent a 24-hour continuous electrocardiogram recording in the supine position, using the Plus-type three-channel DCG of the American DMS company. The measurement recording started from 8–9 am and ended at 8–9 am the next day.
Variable Definitions
HRV was defined as the variations of heart rate and successive cardiac cycles under the control of the autonomic nervous system. Time-domain measures of overall HRV included the mean heart rate, standard deviation of the R-R interval (SDNN), SDNN index, root mean squared difference of successive RR intervals (RMSSD), and the percentage of adjacent RR intervals with a difference greater than 50 ms (PNN50). Frequency-domain measures included low-frequency (LF), very low-frequency (VLF), high-frequency (HF) components, and the LF-to-HF ratio, considered to be an estimate of the relative sympathetic and parasympathetic balance.
ACR combined with eGFR was used to stratify the risk of DKD progression following the KDIGO guidelines (10). The patients were classified into four risk groups: low risk (Group A), moderately increased risk (Group B), high risk (Group C), and very high risk (Group D) (Figure 1).
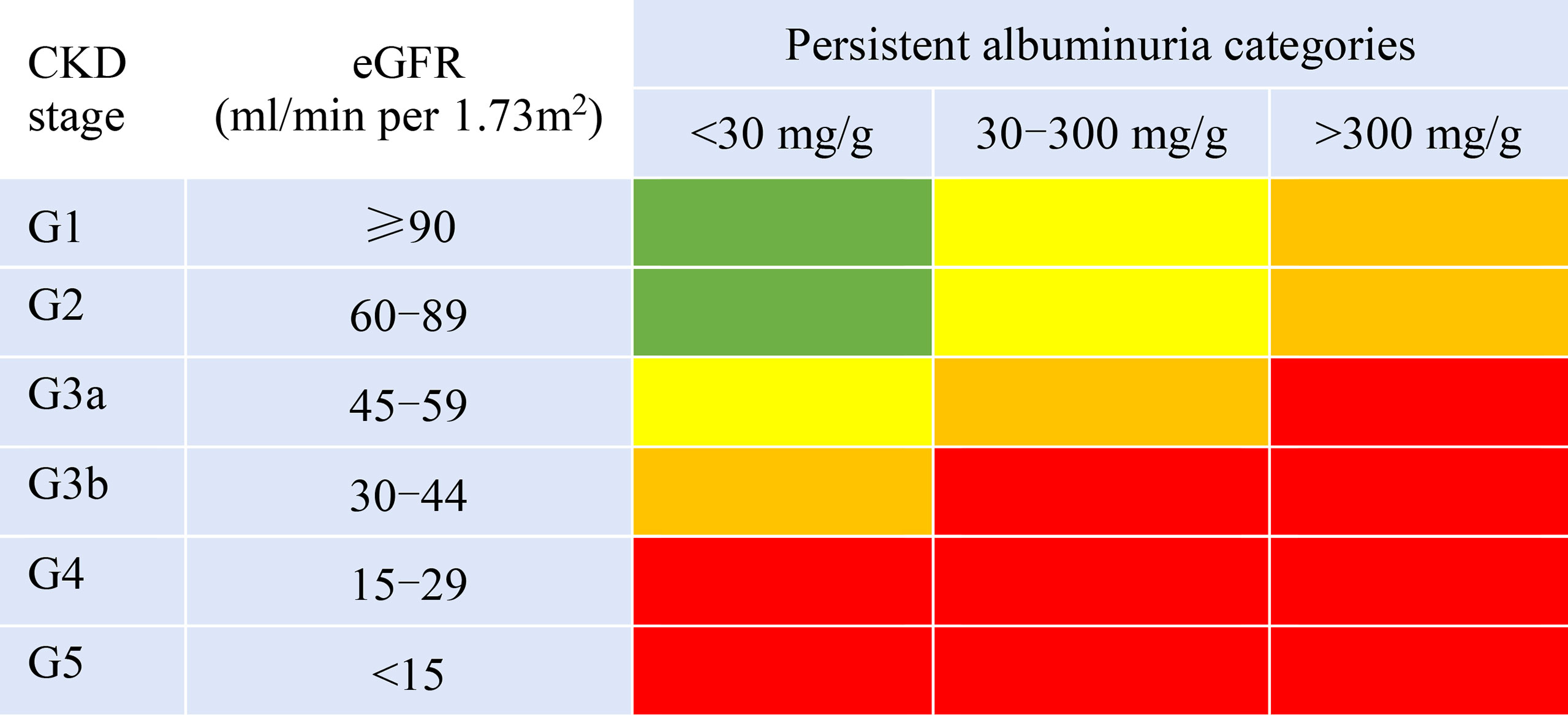
Figure 1 Prognosis of CKD by eGFR and albuminuria categories. Green areas represent low risk; yellow, moderately increased risk; orange, high risk; and red, very high risk.
Statistical Analysis
Normally distributed continuous variables were compared using one-way analysis of variance, summarised as mean ± SD for parametric data. Meanwhile, non-normal distributed variables expressed as the median with interquartile range, and categorical variables were presented as the number (percentage). Correlations between two variables were assessed using Spearman correlations. Multivariate linear regression and logistic regression analyses were used to determine the association between HRV and the risk of renal disease progression. One-way analysis of variance was used to establish whether HRV differed according to the risk of DKD progression category. If significant differences were found, individual groups were compared using the Bonferroni post hoc test. All statistical analyses were conducted using SPSS version 25 software (SPSS Inc., Version 25.0, Chicago, IL, USA). P<0.05 was considered statistically significant.
Results
The mean patient age was 68.6 ± 11.5 years. Groups A, B, C, and D included 409, 163,77, and 98 patients, respectively. There were no significant differences in sex, height, weight, BMI, and average heart rate among the four groups. Except for high-density lipoprotein cholesterol, the other lipid profiles showed no significant difference. The risk of DKD progression increased with age. Participants with higher risk of DKD progression also had significantly higher systolic blood pressure (SBP) (P<0.01) and significantly lower whole blood haemoglobin (P<0.01) (Table 1).
ACR as a continuous variable was negatively correlated with SDNN (r=-0.21, P <0.01), SDNN Index (r=-0.16, P<0.01), HF (r=-0.08, P = 0.03), LF (r=-0.15, P<0.01), LF/HF (r=-0.15, P<0.01), and VLF (r=-0.28, P<0.01). Meanwhile, eGFR was positively correlated with SDNN (r=0.16, P<0.001), LF (r=0.12, P<0.01), LF/HF (r=0.25, P<0.01), and VLF (r=0.27, P<0.01) (Table 2).
The risk of DKD progression was significantly associated with SDNN, VLF, and LF/HF in the multivariate linear regression analysis. The reduction of VLF was closely related to a moderately increased risk (β=-99.4, 95% CI: -197.5 to -1.3, P<0.04), high risk (β=-237.1, 95% CI: -369.2 to -104.9, P<0.01), and very high risk (β=-193.7, 95% CI: -320.8 to -66.6, P<0.01) of DKD progression. It was also independently related to HbA1c and haemoglobin levels. In addition, decreased LF/HF was also closely related to a moderately increased risk (β=-0.29, 95% CI: -0.49 to -0.09, P<0.01), high risk (β=-0.29, 95% CI: -0.56 to - 0.02, P=0.03), and very high risk (β=-0.38, 95% CI: -0.64 to -0.12, P<0.01) of DKD progression. It was also independently associated with SBP and haemoglobin levels. Similar results were found for SDNN, although only the difference between group A and D reached significance (Table 3 and Figure 2).
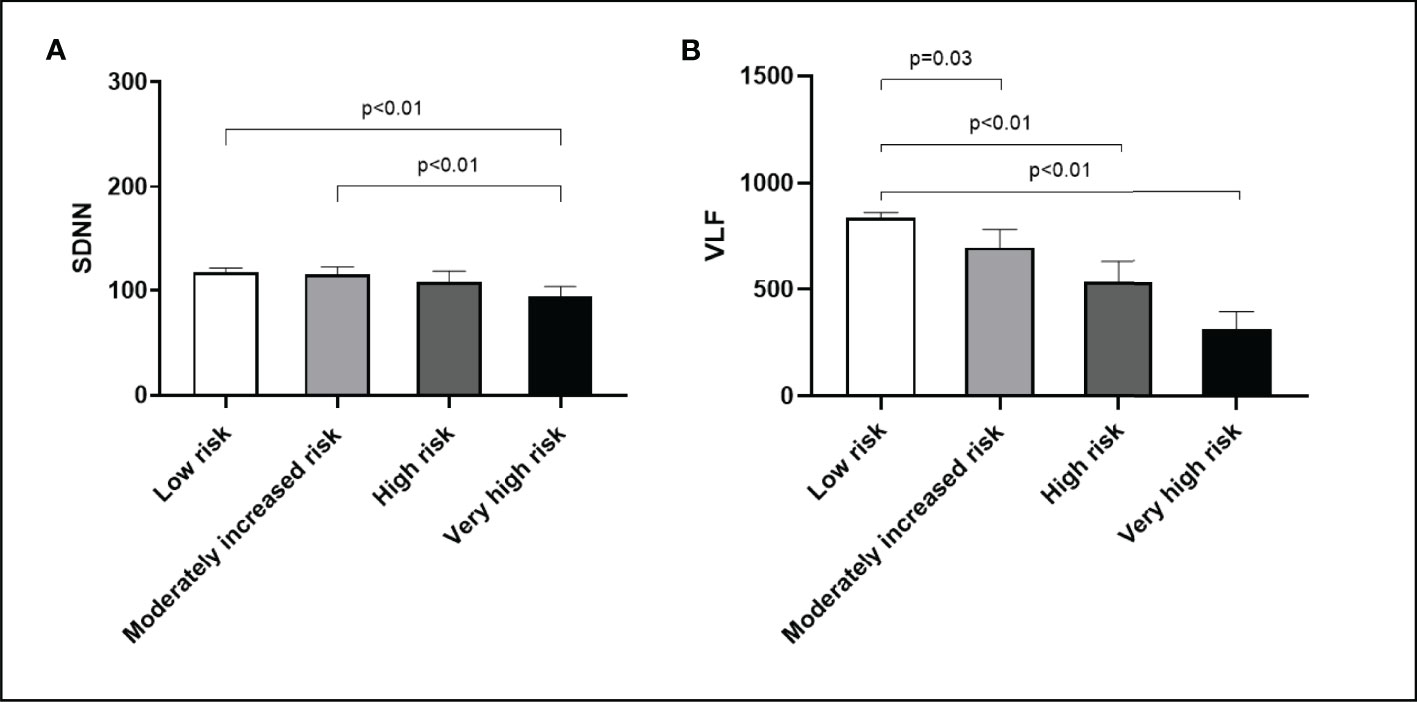
Figure 2 DKD progression risk with respect to heart rate variability. (A) SDNN. (B) VLF. Bar (one bar per column) plots are shown in (A, B) The P values are calculated using Bonferroni post hoc test after significant one-way ANOVA.
The HRV parameters SDNN, SDNN index, LF, LF/HF, and VLF decreased with increasing risk of DKD progression (P<0.01) (Table 4). Further multivariate logistic regression analysis adjusted for SBP, HbA1c, haemoglobin, HDL-cholesterol, and age showed that compared with group A, group D had a higher risk of reduced SDNN, LF/HF, and VLF (odds ratio (95% CI): 0.989 (0.983–0.996), 0.674 (0.498–0.913), and 0.999 (0.999–1.000), respectively) (Table 5).
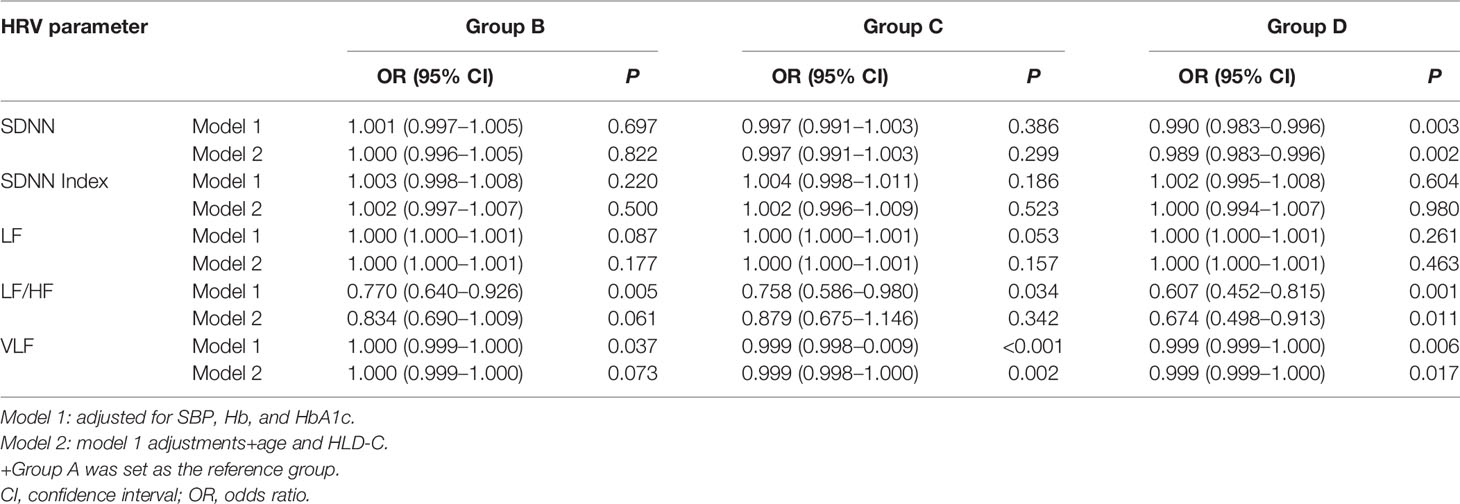
Table 5 Multivariate analysis of risk factors for moderately increased, high, and very high risks of DKD progression.
Discussion
The causal relationship between CAN and CKD is still unclear, and varying study populations and methods of CAN assessment may lead to different results. This study found that cardiac autonomic dysfunction is associated with the risk of nephropathy progression in patients with T2DM. Compared with those at low risk of DKD progression, patients with a very high risk of DKD progression had lower overall HRV (SDNN, VLF, LF/HF), and this association was independent of age, HbA1c, SBP, high-density lipoprotein cholesterol, and whole blood haemoglobin.
A study of non-diabetes patients found that although HRV reduction was not an independent risk factor for CKD, it was independently associated with eGFR reduction at baseline in patients with CKD. This suggests that CAN may be a complication rather than a cause of CKD (11). However, albuminuria, CKD, and CAN are all common microvascular complications with similar pathogenic mechanisms driven by hyperglycaemia and other risk factors in patients with diabetes. The relationship between CAN and CKD has been reported in some studies of patients with T2DM (12, 13). SDNN reflects the total tension of sympathetic and parasympathetic nerves and can be used to evaluate the overall regulation of cardiac autonomic nervous system (14). Meanwhile, HF is associated with parasympathetic activity (15), and LF is associated with both sympathetic and parasympathetic activity (16). VLF is an indicator of sympathetic activity, and the LF-to-HF ratio is considered to be an estimate of the relative sympathetic and parasympathetic balance (17).
Glomeruli and renal tubules are mainly innervated by sympathetic nerves, and changes in sympathetic nerve activity and imbalance of autonomic nerve function can affect renal homeostasis (renal vascular resistance, filtration) and accelerate the decline of renal function (13, 18). On the one hand, CAN can change the circadian rhythm of blood pressure, reduce the drop of blood pressure at night, and increase the blood flow and load of the kidney, which is an important cause of kidney damage (19). On the other hand, sympathetic nerve endings directly innervate the kidney, enhance the absorption of solutes and fluids, and affect the renal tubular function (20). Either increased sympathetic nerve activity or simultaneous changes in sympathetic and parasympathetic activity can be associated with reduced HRV and poor renal prognosis (17). The results of our study showed that the reduction of SDNN, LF/HF, and VLF was closely related to the increased risk of DKD progression. Compared with the low risk group, the very high-risk group of DKD progression had significantly lower SDNN, LF/HF, and VLF. Our findings are consistent with those of two other studies of type 1 diabetes patients that found a significantly faster progression of renal dysfunction in the HRV-poor group (4, 21).
There may be potential autonomic crosstalk between the kidneys and the cardiovascular system. Autonomic dysfunction as a broad term mainly refers to a condition in which tonic and reflex control of autonomic outflows is altered, favouring increased sympathetic nerve activity and depressed parasympathetic function (22). Autonomic dysfunction is associated with increased oxidative stress and elevated levels of proinflammatory cytokines (23, 24). Several studies suggest that oxidative stress plays a role in the development of CAN in diabetic patients (25, 26). Kidney disease is associated with a decrease in antioxidant scavenging enzymes and increase in the production of reactive oxygen species (ROS), thereby leading to elevated oxidative stress (27). Furthermore, the accumulation of reactive ROS is important determinants that promote the DKD progresses (28). Studies in DKD rats show that inhibition of ROS and nucleotide binding and oligomerization domain-like receptor family pyrin domain-containing 3 (NLRP3) inflammasome possesses kidney protection effects including attenuating urinary microalbumin excretion, and mitigating renal histopathological lesions on DN (29). Cardiac inflammation is an important pathological process involved in sympathetic overactivation induced cardiac injury (30). Sympathetic overactivation induces cardiac inflammation and dysfunction by activating the NLRP3 inflammasome in cardiomyocytes (31). However, it remains to be determined whether Oxidative stress and proinflammatory cytokines involved in sympathetic overactivation promotes DKD progression and altered cardiovascular autonomic function.
Obesity is associated with decreased parasympathetic nerve activity and increased sympathetic nerve activity (32, 33). The present study found no differences in BMI between the four risk groups for DKD progression, helping to rule out confounding effects of BMI on HRV and risk of DKD progression. However, BMI is weakly correlated with cardiac autonomic function indicators (33), and thus, more sensitive indicators of subcutaneous and visceral obesity should be incorporated in the future. Additionally, SBP increased with increased risk of kidney disease progression in the current study, whereas there was no significant difference in DBP. In the VADT study, baseline SBP was associated with decreasing eGFR and worsening ACR among veterans with T2DM, whereas DBP levels were not significantly associated with risk of renal outcomes (34). Similar conclusions were also obtained in the Australian Obesity Diabetes Study, wherein there was no direct relationship between DBP and renal outcome. However, higher pulse pressure was a significant risk factor for decreased eGFR (35). Elevated blood pressure also induces decreased parasympathetic activity and increased sympathetic activity, and elevated circadian systolic blood pressure gradients are significantly associated with autonomic dysfunction in hypertension patients (36, 37). Similarly, we found that SDNN and LF/HF reduction were closely related to SBP.
A limitation of this study was its cross-sectional design, and thus, causal and temporal relationships between cardiac autonomic function and risk of DKD progression could not be examined. Autonomic dysfunction and CKD progression may share common aetiologies such as obesity, inflammation, insulin resistance, and genetic factors (38–42), but these were not fully measured in this study.
In conclusion, reduced HRV in patients with T2DM is associated with DKD and its risk of progression. Thus, screening for HRV may be necessary in patients with T2DM, especially those with high proteinuria.
Data Availability Statement
The original contributions presented in the study are included in the article/supplementary material. Further inquiries can be directed to the corresponding author.
Ethics Statement
Written informed consent was not obtained from the individual(s) for the publication of any potentially identifiable images or data included in this article.
Author Contributions
HZ contributed to execution of the study, completed the data analysis, and wrote the article. JL contributed to critically revised the article and approved the final version. All authors contributed to the article and approved the submitted version.
Conflict of Interest
The authors declare that the research was conducted in the absence of any commercial or financial relationships that could be construed as a potential conflict of interest.
Publisher’s Note
All claims expressed in this article are solely those of the authors and do not necessarily represent those of their affiliated organizations, or those of the publisher, the editors and the reviewers. Any product that may be evaluated in this article, or claim that may be made by its manufacturer, is not guaranteed or endorsed by the publisher.
Acknowledgments
We would like to thank the members of the Information Department of the Second Affiliated Hospital of Nanchang University for providing useful data.
References
1. Vinik AI, Ziegler D. Diabetic Cardiovascular Autonomic Neuropathy. Circulation (2007) 115:387 97. doi: 10.1161/circulationaha.106.634949
2. Zhang Z, Ma Y, Fu L, Li L, Liu J, Peng H, et al. Combination of Composite Autonomic Sympto Score 31 and Heart Rate Variability for Diagnosis of Cardiovascular Autonomic Neuropathy in People With Type 2 Diabetes. J Diabetes Res (2020) 2020:5316769. doi: 10.1155/2020/5316769
3. Malpas SC, Maling TJ. Heart-Rate Variability and Cardiac Autonomic Function in Diabetes. Diabetes (1990) 39:1177–81. doi: 10.2337/diab.39.10.1177
4. Cho YH, Craig ME, Davis EA, Cotterill AM, Couper JJ, Cameron FJ, et al. Cardiac Autonomic Dysfunction is Associated With High-Risk Albumin-to-Creatinine Ratio in Young Adolescents With Type 1 Diabetes in AdDIT (Adolescent Type 1 Diabetes Cardio-Renal Interventional Trial). Diabetes Care (2015) 38:676–81. doi: 10.2337/dc14-1848
5. Shah AS, El Ghormli L, Vajravelu ME, Bacha F, Farrell RM, Gidding SS, et al. Heart Rate Variability and Cardiac Autonomic Dysfunction: Prevalence, Risk Factors, and Relationship to Arterial Stiffness in the Treatment Options for Type 2 Diabetes in Adolescents and Youth (TODAY) Study. Diabetes Care (2019) 42:2143–50. doi: 10.2337/dc19-0993
6. Weng J, Zhou Z, Guo L, Zhu D, Ji L, Luo X, et al. Incidence of Type 1 Diabetes in China, 2010-13: Population Based Study. BMJ (2018) 360:j5295. doi: 10.1136/bmj.j5295
7. Stevens PE, Levin A. Evaluation and Management of Chronic Kidney Disease: Synopsis of the Kidney Disease: Improving Global Outcomes 2012 Clinical Practice Guideline. Ann Intern Med (2013) 158:825–30. doi: 10.7326/0003-4819-158-11-201306040-00007
8. Orlov S, Cherney DZ, Pop-Busui R, Lovblom LE, Ficociello LH, Smiles AM, et al. Cardiac Autonomic Neuropathy and Early Progressive Renal Decline in Patients With Nonmacroalbuminuric Type 1 Diabetes. Clin J Am Soc Nephrol CJASN (2015) 10:1136–44. doi: 10.2215/cjn.11441114
9. Spallone V, Gambardella S, Maiello MR, Barini A, Frontoni S, Menzinger G. Relationship Between Autonomic Neuropathy, 24-H Blood Pressure Profile, and Nephropathy in Normotensive IDDM Patients. Diabetes Care (1994) 17:578–84. doi: 10.2337/diacare.17.6.578
10. Camm AJ, Malik M, Bigger JT, Breithardt G, Cohen RJ, et al. Kidney Disease: Improving Global Outcomes Diabetes Work G. KDIGO 2020 Clinical Practice Guideline for Diabetes Management in Chronic Kidney Disease. Kidney Int (2020) 98:S1–S115. doi: 10.1016/j.kint.2020.06.019
11. Thio CHL, van Roon AM, Lefrandt JD, Gansevoort RT, Snieder H. Heart Rate Variability and Its Relation to Chronic Kidney Disease: Results From the PREVEND Study. Psychosomatic Med (2018) 80:307–16. doi: 10.1097/psy.0000000000000556
12. Takebayashi K, Matsutomo R, Matsumoto S, Suetsugu M, Wakabayashi S, Aso Y, et al. Relationships Between Heart Rate Variability and Urinary Albumin Excretion in Patients With Type 2 Diabetes. Am J Med Sci (2006) 331:72–8. doi: 10.1097/00000441-200602000-00012
13. Yun JS, Ahn YB, Song KH, Yoo KD, Kim HW, Park YM, et al. The Association Between Abnormal Heart Rate Variability and New Onset of Chronic Kidney Disease in Patients With Type 2 Diabetes: A Ten-Year Follow-Up Study. Diabetes Res Clin Practice (2015) 108:31–7. doi: 10.1016/j.diabres.2015.01.031
14. Fagard RH, Pardaens K, Staessen JA, Thijs L. Power Spectral Analysis of Heart Rate Variability by Autoregressive Modelling and Fast Fourier Transform: A Comparative Study. Acta Cardiologica (1998) 53:211–8.
15. Thayer JF, Lane RD. The Role of Vagal Function in the Risk for Cardiovascular Disease and Mortality. Biol Psychol (2007) 74:224–42. doi: 10.1016/j.biopsycho.2005.11.013
16. Goldstein DS, Bentho O, Park MY, Sharabi Y. Low-Frequency Power of Heart Rate Variability is Not a Measure of Cardiac Sympathetic Tone But may be a Measure of Modulation of Cardiac Auto-Nomic Outflows by Baroreflexes. Exp Physiol (2011) 96:1255–61. doi: 10.1113/expphysiol.2010.056259
17. Heart Rate Variability. Standards of Measurement, Physiological Interpretation, and Clinical Use. Task Force of the European Society of Cardiology and the North American Society of Pacing and Electrophysiology. Eur Heart J (1996) 17:354–81.
18. Valensi P, Pariès J, Attali JR. Cardiac Autonomic Neuropathy in Diabetic Patients: Influence of Diabetes Duration, Obesity, and Microangiopathic Complications–the French Multicenter Study. Metabolism (2003) 52:815–20. doi: 10.1016/s0026-0495(03)00095-7
19. Monteagudo PT, Nóbrega JC, Cezarini PR, Ferreira SR, Kohlmann O Jr., Ribeiro AB, et al. Altered Blood Pressure Profile, Autonomic Neuropathy and Nephropathy in Insulin-Dependent Diabetic Patients. Eur J Endocrinol (1996) 135:683–8. doi: 10.1530/eje.0.1350683
20. DiBona GF. Physiology in Perspective: The Wisdom of the Body. Neural Control of the Kidney. Am J Physiol Regulatory Integr Comp Physiol (2005) 289:R633–41. doi: 10.1152/ajpregu.00258.2005
21. Weinrauch LA, Kennedy FP, Gleason RE, Keough J, D'Elia JA. Relationship Between Autonomic Function and Progression of Renal Disease in Diabetic Proteinuria: Clinical Correlations and Implications for Blood Pressure Control. Am J Hypertension (1998) 11:302–8. doi: 10.1016/s0895-7061(97)00472-x
22. Hildreth CM. Prognostic Indicators of Cardiovascular Risk in Renal Disease. Front Physiol (2011) 2:121. doi: 10.3389/fphys.2011.00121
23. Li JD, Cheng AY, Huo YL, Fan J, Zhang YP, Fang ZQ, et al. Bilateral Renal Denervation Ameliorates Isoproterenol-Induced Heart Failure Through Downregulation of the Brain Renin-Angiotensin System and Inflammation in Rat. Oxid Med Cell Longevity (2016) 2016:3562634. doi: 10.1155/2016/3562634
24. Fadaee SB, Beetham KS, Howden EJ, Stanton T, Isbel NM, Coombes JS. Oxidative Stress Associated With Decreased Heart Rate Variability in Patients With Chronic Kidney Disease. Redox Rep (2017) 22:197–204. doi: 10.1080/13510002.2016.1173326
25. Thiyagarajan R, Pal P, Pal GK, Subramanian SK, Bobby Z, Das AK, et al. Cardiovagal Modulation, Oxidative Stress, and Cardiovascular Risk Factors in Prehypertensive Subjects: Cross-Sectional Study. Am J Hypertension (2013) 26:850–7. doi: 10.1093/ajh/hpt025
26. Spallone V. Update on the Impact, Diagnosis and Management of Cardiovascular Autonomic Neuropathy in Diabetes: What Is Defined, What Is New, and What Is Unmet. Diabetes Metab J (2019) 43:3–30. doi: 10.4093/dmj.2018.0259
27. Salman IM. Cardiovascular Autonomic Dysfunction in Chronic Kidney Disease: A Comprehensive Review. Curr Hypertens Rep (2015) 17:59. doi: 10.1007/s11906-015-0571-z
28. Li S, Zheng L, Zhang J, Liu X, Wu Z. Inhibition of Ferroptosis by Up-Regulating Nrf2 Delayed the Progression of Diabetic Nephropathy. Free Radical Biol Med (2021) 162:435–49. doi: 10.1016/j.freeradbiomed.2020.10.323
29. Ding T, Wang S, Zhang X, Zai W, Fan J, Chen W, et al. Kidney Protection Effects of Dihydroquercetin on Diabetic Nephropathy Through Suppressing ROS and NLRP3 Inflammasome. Phytomedicine (2018) 41:45–53. doi: 10.1016/j.phymed.2018.01.026
30. Manolis AJ, Poulimenos LE, Kallistratos MS, Gavras I, Gavras H. Sympathetic Overactivity in Hypertension and Cardiovascular Disease. Curr Vasc Pharmacol (2014) 12:4–15. doi: 10.2174/15701611113119990140
31. Xin JZ, Wu JM, Hu GM, Gu HJ, Feng YN, Wang SX, et al. α(1)-AR Overactivation Induces Cardiac Inflammation Through NLRP3 Inflammasome Activation. Acta Pharmacol Sin (2020) 41:311–8. doi: 10.1038/s41401-019-0305-x
32. Ibacache P, Cárcamo P, Miranda C, Bottinelli A, Guzmán J, Martínez-Rosales E, et al. Improvements in Heart Rate Variability in Women With Obesity: Short-Term Effects of Sleeve Gastrectomy. Obes Surgery (2020) 30:4038–45. doi: 10.1007/s11695-020-04721-y
33. Yadav RL, Yadav PK, Yadav LK, Agrawal K, Sah SK, Islam MN. Association Between Obesity And Heart Rate Variability Indices: An Intuition Toward Cardiac Autonomic Alteration - a Risk of CVD. Diabetes Metab Syndrome Obes (2017) 10:57–64. doi: 10.2147/dmso.S123935
34. Anderson RJ, Bahn GD, Emanuele NV, Marks JB, Duckworth WC. Blood Pressure and Pulse Pressure Effects on Renal Outcomes in the Veterans Affairs Diabetes Trial (VADT). Diabetes Care (2014) 37:2782–8. doi: 10.2337/dc14-0284
35. van den Hurk K, Magliano DJ, Alssema M, Schlaich MP, Atkins RC, Reutens AT, et al. Type 2 Diabetes Strengthens the Association Between Pulse Pressure and Chronic Kidney Disease: The AusDiab Study. J Hypertension (2011) 29:953–60. doi: 10.1097/HJH.0b013e328344d9cf
36. Ohisa N, Hashimoto J, Yoshida K, Imai Y, Kaku M. [The Relationships Between Autonomic Function and Heart Rate in Essential Hypertension]. Rinsho Byori (2005) 53:195–200.
37. Zhang Y, Agnoletti D, Blacher J, Safar ME. Blood Pressure Variability in Relation to Autonomic Nervous System Dysregulation: The X-CELLENT Study. Hypertension Res (2012) 35:399–403. doi: 10.1038/hr.2011.203
38. Nowak KL, Chonchol M, Ikizler TA, Farmer-Bailey H, Salas N, Chaudhry R, et al. IL-1 Inhibition and Vascular Function in CKD. J Am Soc Nephrol JASN (2017) 28:971–80. doi: 10.1681/asn.2016040453
39. Bhati P, Alam R, Moiz JA, Hussain ME. Subclinical Inflammation and Endothelial Dysfunction are Linked to Cardiac Autonomic Neuropathy in Type 2 Diabetes. J Diabetes Metab Disord (2019) 18:419–28. doi: 10.1007/s40200-019-00435-w
40. Liu Y, Peng Y, Jin J, Chen Y, Chen C, Chen Z, et al. Insulin Resistance is Independently Associated With Cardiovascular Autonomic Neuropathy in Type 2 Diabetes. J Diabetes Investig (2021) 12:1651–62. doi: 10.1111/jdi.13507
41. Navaneethan SD, Kirwan JP, Remer EM, Schneider E, Addeman B, Arrigain S, et al. Adiposity, Physical Function, and Their Associations With Insulin Resistance, Inflammation, and Adipokines in CKD. Am J Kidney Dis (2021) 77:44–55. doi: 10.1053/j.ajkd.2020.05.028
Keywords: T2DM, HRV, DKD, ACR, eGFR
Citation: Zeng H, Liu J, Chen Z, Yu P and Liu J (2022) Cardiac Autonomic Dysfunction Is Associated With Risk of Diabetic Kidney Disease Progression in Type 2 Diabetes Mellitus. Front. Endocrinol. 13:900465. doi: 10.3389/fendo.2022.900465
Received: 20 March 2022; Accepted: 06 June 2022;
Published: 01 July 2022.
Edited by:
Baocheng Chang, Tianjin Medical University, ChinaReviewed by:
Oscar Lorenzo, Health Research Institute Foundation Jimenez Diaz (IIS-FJD), SpainLI PING, ShengJing Hospital of China Medical University, China
Copyright © 2022 Zeng, Liu, Chen, Yu and Liu. This is an open-access article distributed under the terms of the Creative Commons Attribution License (CC BY). The use, distribution or reproduction in other forums is permitted, provided the original author(s) and the copyright owner(s) are credited and that the original publication in this journal is cited, in accordance with accepted academic practice. No use, distribution or reproduction is permitted which does not comply with these terms.
*Correspondence: Jianping Liu, liujpnfm@163.com