- Department of Endocrinology, Bielański Hospital, Centre of Postgraduate Medical Education, Warsaw, Poland
Background: FGF21 pharmacological treatment reverses fatty liver and lowers serum triglyceride concentration but FGF21 serum level is increased in hepatic steatosis. FGF21 secretion is induced by thyroid hormones in vitro.
Purpose: To determine the influence of thyroid hormones and metabolic changes secondary to thyroid dysfunction on FGF21 secretion in humans.
Materials and Methods: This was a case-control study. 82 hyperthyroid and 15 hypothyroid patients were recruited together with 25 healthy controls. Of those with hyperthyroidism, 56 received radioiodine treatment and 42 of them achieved hypothyroidism and then euthyroidism within one year following therapy. Radioiodine-induced hypothyroidism developed abruptly within a six week interval between clinic visits. FGF21 serum levels were determined with an ELISA method.
Results: Serum FGF21 levels did not differ in hyper- and hypothyroid patients in comparison to controls [median 103.25 (interquartile range, 60.90-189.48) and 86.10 (54.05-251.02) vs 85.20 (58.00-116.80) pg/mL P=0.200 and 0.503, respectively]. In hyperthyroid patients treated with radioiodine, serum FGF21 levels increased significantly in rapid-onset hypothyroidism in comparison to the hyperthyroid and euthyroid phase [median 160.55 (interquartile range, 92.48 - 259.35) vs 119.55 (67.78-192.32) and 104.43 (55.93-231.93) pg/mL, P=0.034 and 0.033, respectively]. The rising serum FGF21 level correlated positively with serum triglycerides (Spearman coefficient rs=0.36, P=0.017) and inversely with serum SHBG (rs=-0.41, P=0.007), but did not correlate with thyroid hormone levels.
Conclusions: There was a transient increase in FGF21 serum level during rapid-onset hypothyroidism following radioiodine treatment. There was no association between FGF21 serum level and thyroid hormones. In radioiodine-induced hypothyroidism, the rising serum FGF21 concentration correlated positively with rising serum triglycerides and negatively with falling SHBG, reflecting increased hepatic lipogenesis.
Introduction
Thyroid hormones increase basal metabolic rate, stimulate brown adipose tissue thermogenesis, and reduce cholesterol and triglyceride levels. Body mass and lipid changes are essential symptoms of thyroid dysfunction, resulting directly from altered thyroid hormone levels, but also from cross-talk with numerous metabolic factors. Fibroblast growth factor 21 (FGF21) is a novel potent metabolic regulator which actions have considerable overlap with thyroid hormones. Similar to thyroid hormones, FGF21 lowers serum lipid levels, stimulates brown adipose tissue thermogenesis, and promotes weight loss (1–3). Its secretion is induced by T3 in murine and human hepatic cell culture (4, 5). However, the stimulating effect of thyroid hormones on FGF21 release in humans is controversial, with studies demonstrating divergent results (6, 7).
FGF21 together with FGF19, and FGF23 belong to the fibroblast growth factor family with hormone-like activity. In contrast to classical fibroblast growth factors that act in an auto- and paracrine manner, hormone-like FGFs are released into the bloodstream and exert endocrine action in distant tissues (8, 9). Serum FGF21 is generated primarily in the liver (10) under nutritional stress stimuli like starvation (11, 12) or a lipotoxic diet (12–15), but also increased mitochondrial and endoplasmic reticulum stress (16, 17). Specifically in the liver, it produces essential biological action of protecting hepatocytes from metabolic stress caused by fat overload (18). FGF21 stimulates hepatic fatty acid oxidation instead of its conversion into triglycerides (19). Moreover, FGF21 reduces the flow of lipids into the liver by increased peripheral lipoprotein catabolism (20) and reduced adipocytes lipolysis (21). Exogenous FGF21 administration reduces hepatic fat content and reverses fatty liver (2, 21–24). Paradoxically, although some studies suggest direct paracrine action of FGF21 in the liver (25), others demonstrate the lack of FGF21 key receptor FGFR1c in hepatocytes, suggesting that the decrease in hepatic triglyceride deposition may be mediated indirectly (26). It has been proposed that the release of adiponectin from adipose tissue in response to liver-derived FGF21 reduces hepatic lipid content in a feedback manner (27). However, other research has demonstrated adiponectin is dispensable for FGF21 action (28), or even conversely adipokine generated in adipocytes induces FGF21 expression in the liver (29). However, this data has emerged from in vitro and animal research, or clinical studies following administration of FGF21 analogues, and so does not fully reflect physiology.
Besides plasma and hepatic lipid regulation, FGF21 exerts the critical action of controlling energy homeostasis (1, 2, 28, 30). Serum FGF21 derives predominantly, if not only from the liver (10) and exerts its action in the central nervous system (31, 32) and adipose tissue (28, 33, 34). Acting in the ventromedial hypothalamus, FGF21 diminishes sweet-taste preference that results in simple sugar intake suppression (35). In adipose tissue, FGF21 promotes glucose utilization and increases energy expenditure by enhancing insulin sensitivity, stimulating browning of white adipocytes and brown adipose tissue thermogenesis (28, 33, 34). Therefore, FGF21 induces weight loss by suppression of sucrose intake, and increase in brown adipose tissue insulin sensitivity resulting in glucose consumption for heat production instead of energy storage. Paradoxically despite its beneficial action, FGF21 is elevated in insulin resistance states i.e. fatty liver, obesity, and type 2 diabetes (36–38). It is not clear if this effect results from FGF21 resistance or compensatory increased secretion (38, 39).
The aim of the study was to investigate whether thyroid hormones directly change FGF21 secretion in hyper- and hypothyroidism, or whether metabolic challenge related to considerable changes in glucose and lipid metabolism and body composition that accompany thyroid dysfunction indirectly affect FGF21 release. Therefore, we firstly analyzed FGF21 serum levels in hyper-and hypothyroid subjects as compared to the euthyroid control group. Secondly, we analyzed circulating FGF21 in patients with Graves’ disease that were treated with radioiodine (RAI), in three different thyroid function states, namely hyperthyroidism at clinic referral, hypothyroidism induced by RAI treatment that developed abruptly within a six week interval between clinic visits, and euthyroidism after l-thyroxine treatment. These typically occur in 80% of patients within one year of undergoing treatment. Additionally, we analyzed serum adiponectin levels and its association with circulating FGF21 in the different thyroid function states. These patients were chosen because of dramatic thyroid hormone, lipid, and body composition changes that occurred in a short period of time, which triggers different metabolic counterregulatory pathways e.g. possibly FGF21 secretion. Finally, we analyzed a subgroup of thionamide-treated hyperthyroid patients pre-treatment, and three months after euthyroidism had been established.
Materials and Methods
Study Participants
We performed a case-control study enrolling 82 hyperthyroid patients with Graves’ disease and 15 hypothyroid patients with Hashimoto’s thyroiditis from the Department of Endocrinology, Centre of Postgraduate Medical Education, Bielański Hospital (Warsaw, Poland), from September 2016 to September 2020. The control group consisted of 25 healthy hospital employees. The study protocol was approved by the Bioethical Committee of Centre of Postgraduate Medical Education, Warsaw, Poland. All participants provided written informed consent.
The inclusion criteria were as follows: (1) age ≥ 18 years (2) body mass index (BMI) ≤ 35 (3) hyperthyroidism in the course of Graves’ disease (4) hypothyroidism in the course of Hashimoto’s thyroiditis. The control group was recruited from healthy volunteers that were free of thyroid disease. The exclusion criteria were as follows: (1) diabetes (2) cancer (3) pregnancy and lactation (4) abnormal hepatic function tests (elevated alanine aminotransferase (ALT) or aspartate aminotransferase (AST) >1.5 times above reference value) (5) renal dysfunction (defined glomerular filtration rate [GFR] <60 ml/min/1.73 kg\m2) (6) acute cardiorespiratory or infectious disease.
The diagnosis of hyperthyroidism in the course of Graves’ disease was established based on the typical clinical characteristics, including decreased thyroid stimulating hormone (TSH), elevated thyroid hormones (TH) and TSH receptor antibody (TRAb) serum levels. Hypothyroidism in the course of Hashimoto’s thyroiditis was diagnosed based on clinical presentation, elevated serum TSH, decreased TH levels, and the presence of thyroid peroxidase antibody (TPOAb).
Study Design
In all patients with hyper- and hypothyroidism and the control group, measurements were performed to determine: FGF21, adiponectin, TSH, TH, sex hormone-binding globulin (SHBG), lipids, glucose, insulin, and body composition at baseline.
Of the hyperthyroid patients, 56 were assigned to RAI treatment according to clinical indications and patient choice, whereas the remaining 26 were treated with thionamides. The patients treated with RAI were observed every six weeks until hypothyroidism and finally euthyroidism occurred following l-thyroxine treatment. In these patients, the above-mentioned biochemical and body composition measurements were performed at three time-points, namely initially during the hyperthyroid phase, secondly at the hypothyroid phase, and finally upon reaching euthyroidism while treated with l-thyroxine after three months of successful replacement therapy. Additionally, in the thionamide treated group, the measurements were performed pre-treatment and three months after euthyroidism had been established (Figure 1).
Anthropometric Measures and Laboratory Analyses
Body weight was measured in the morning using an electronic platform scale (Marsden BFA-220P, UK). Height was measured using a wall-mounted stadiometer. Body mass index (BMI) was calculated as body weight (kilograms) divided by height (meters2). Waist circumference was measured in the midpoint between the lowest rib and the superior margin of the iliac crest.
All patients with hyper- and hypothyroidism, and healthy controls had blood drawn from the antecubital vein after 12h of fasting, 8.00-9.00 AM. The blood was centrifuged, and serum frozen at -80°C for FGF21 and adiponectin analysis.
Serum TSH was determined using chemiluminescence immunoassay (UniCel DxI 600, Beckman Coulter, USA). Serum fT4, fT3, insulin, SHBG, TPOAb, thyroglobulin antibodies (TGAb) were determined using a chemiluminescence immunoassay (Liaison®, DiaSorin, Italy). Serum TRAb was determined with electrochemiluminescence assay (Cobas 8000, Roche Diagnostics, Switzerland).
Serum AST, ALT, creatinine, cholesterol, HDL-cholesterol (HDL-c), and triglycerides (TG) were determined on a biochemical analyzer (Cobas 6000, Roche Diagnostics, Switzerland). Plasma glucose was measured using the hexokinase method (Cobas 6000, Roche Diagnostics, Switzerland).
LDL-cholesterol (LDL-c) was calculated using the Friedewald equation. Insulin sensitivity was assessed using the homeostasis model of assessment for insulin resistance (HOMA-IR) and quantitative insulin sensitivity check index (Quicki). HOMA-IR was calculated according to the formula: fasting insulin (µIU/ml) × fasting plasma glucose (FPG, mmol\l)/22.5 and Quicki was calculated as follows: 1/(log (fasting insulin µIU/ml) + log (fasting glucose mg/dl)).
Intact serum FGF21 concentration was determined using the ELISA kit (Epitope Diagnostics, USA, Cat# KT-879, RRID: AB_2895552) according to the manufacturer’s instructions. The detectable range of the assay was 1.7-2169 pg/ml. The intra- and inter-assay coefficients of variation reported by the manufacturer were 5.7%- 6.9% respectively. This assay is highly specific for human intact FGF21 and do not cross react with FGF21 fragments.
Serum adiponectin concentration was assessed using the ELISA kit (Mediagnost, Germany, Cat# E09, RRID : AB_2813736). The detectable range of the assay was 0.27-31000 g/l and the intra- and inter-assay coefficients of variation reported by the manufacturer were 5%-7.5%, respectively. This assay is highly specific for human total adiponectin.
Body Composition Measurement
Body composition was assessed with a whole-body fan-beam dual energy X-ray absorptiometry (DXA) scan (Lunar Prodigy Advance, software v 11.40, GE Healthcare, USA). Body fat (BF), lean tissue mass (LTM), and bone mineral content (BMC) were analyzed using system software. Body regions (arms, legs, trunk, and head) were delineated and BF of particular regions and the total body were analyzed using system software.
Statistical Analysis
All statistical analyses were performed using the R statistic package, version 4.0.5. (http://cran.r-project.org). Shapiro-Wilk’s test was used to assess the normality of data distribution, and log-transformation was applied to non-normally distributed variables and normality retested. Levene’s test was used to assess the equality of variances across groups, the chi-squared test was used to assess the equality of group sizes. Mauchly’s sphericity test was used to assess the sphericity of variances. All measurements are presented as means ± SD for normally distributed data or median (interquartile range) for non-normally distributed data.
Fisher’s exact test was used to compare categorical variables between groups. Student’s unpaired t-test was used to compare normally distributed continuous variables and the Mann-Whitney U-test was used to compare non-normally distributed continuous variables. Changes in FGF21 serum levels and other measures in pair-wise comparison after RAI treatment were examined using a repeated measures ANOVA for normally distributed data and Wilcoxon-signed-rank test for non-normally distributed data even after being logarithmically transformed. For statistically significant ANOVA analyses, post-hoc Student’s paired t-tests were used to find individual points of significance. A Bonferroni correction for multiple comparisons was applied. We used a paired Student’s t-test for normally distributed data or Wilcoxon-signed-rank test for non-normally distributed data to compare variables in thionamide treated group. Relationships between continuous variables were estimated by Spearman correlation coefficient analysis, because the majority of the datasets were not normally distributed. P < 0.05 were considered significant.
Results
Baseline Characteristics of the Subjects
Baseline characteristics of the subjects are shown in Table 1. One person from the control group and one patient with hyperthyroidism were excluded because of the extremely high baseline serum FGF21 values exceeding the upper range of assay sensitivity. Studied groups and control were matched for age, gender and BMI distribution. TSH and TH levels were different between groups according to different thyroid functions. SHBG serum level was higher in hyperthyroid patients compared to the control group. Plasma glucose and serum insulin concentration, HOMA-IR, and Quicki were comparable between patients and controls. As expected, serum total cholesterol, and LDL-c levels were lower in patients with hyperthyroidism, and higher in hypothyroidism, whereas HDL-c was lower in hyperthyroidism. Unlike cholesterol, serum TG levels in hyperthyroid and hypothyroid patients did not differ compared to controls. Additionally, AST was higher in hyperthyroidism and GFR lower in hypothyroidism and higher in hyperthyroidism compared to the control group (Table 1). There was significantly lower LTM in hyperthyroid women than in healthy controls, whereas other body composition parameters in both sexes were comparable (Suppl. Tables 1, 2).
Serum FGF21 Levels in Hyper- and Hypothyroidism did not Differ in Comparison to the Control Group
Serum FGF21 levels did not differ significantly in hyperthyroid and hypothyroid patients in comparison to healthy subjects [median 103.25 (interquartile range, 60.90- 189.48) and 86.10 (54.05-251.02) vs 85.20 (58.00-116.80) pg/mL; P=0.200 and 0.503, respectively] (Table 1 and Figure 2). Similarly, serum adiponectin concentrations in hyper- and hypothyroidism did not differ as compared to controls [mean 11.67 ± 7.26 and 13.09 ± 8.34 vs 9.75 ± 5.57 µg/mL; P=0.171 and 0.195, respectively] (Table 1 and Figure 2).
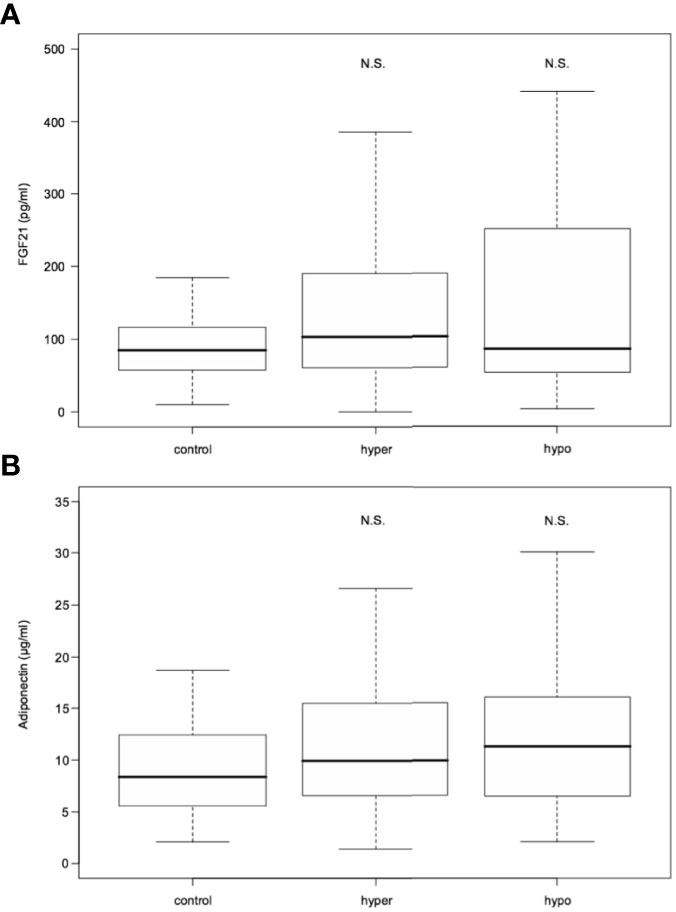
Figure 2 FGF21 (A) and adiponectin (B) serum levels in hyperthyroid and hypothyroid patients in comparison to controls. FGF21, fibroblast growth factor 21. Data presented as median (interquartile range). N.S. represents non-statistically significant difference. P<0.05 was considered significant.
Serum FGF21 Level Rose in Rapid-Onset Hypothyroidism After RAI Treatment
From among the RAI treated hyperthyroid patients, hypothyroidism occurred within one year following therapy in 42 cases. In these patients serum FGF21 levels were unchanged in hyperthyroidism as compared with euthyroidism. However, there was a significant transient increase in FGF21 serum levels in rapid-onset hypothyroidism in comparison to hyperthyroid and euthyroid phase [median 160.55 (interquartile range, 92.48-259.35) vs 119.55 (67.78-192.32) and 104.43 (55.93-231.93) pg/mL, P=0.034 and 0.033, respectively] (Table 2 and Figure 3). Furthermore, adiponectin serum concentration in rapid-onset hypothyroidism, hyperthyroidism and euthyroidism were comparable [mean 15.16 ± 6.75 vs 13.62 ± 7.16 and 16.11 ± 6.87 µg/mL, P= 0.076 and 0.913, respectively] (Table 2 and Figure 3). Moreover, no significant differences in serum levels of FGF21 or adiponectin occurred between the hyper- and euthyroid phases in the thionamide treated group (Suppl. Table 5).
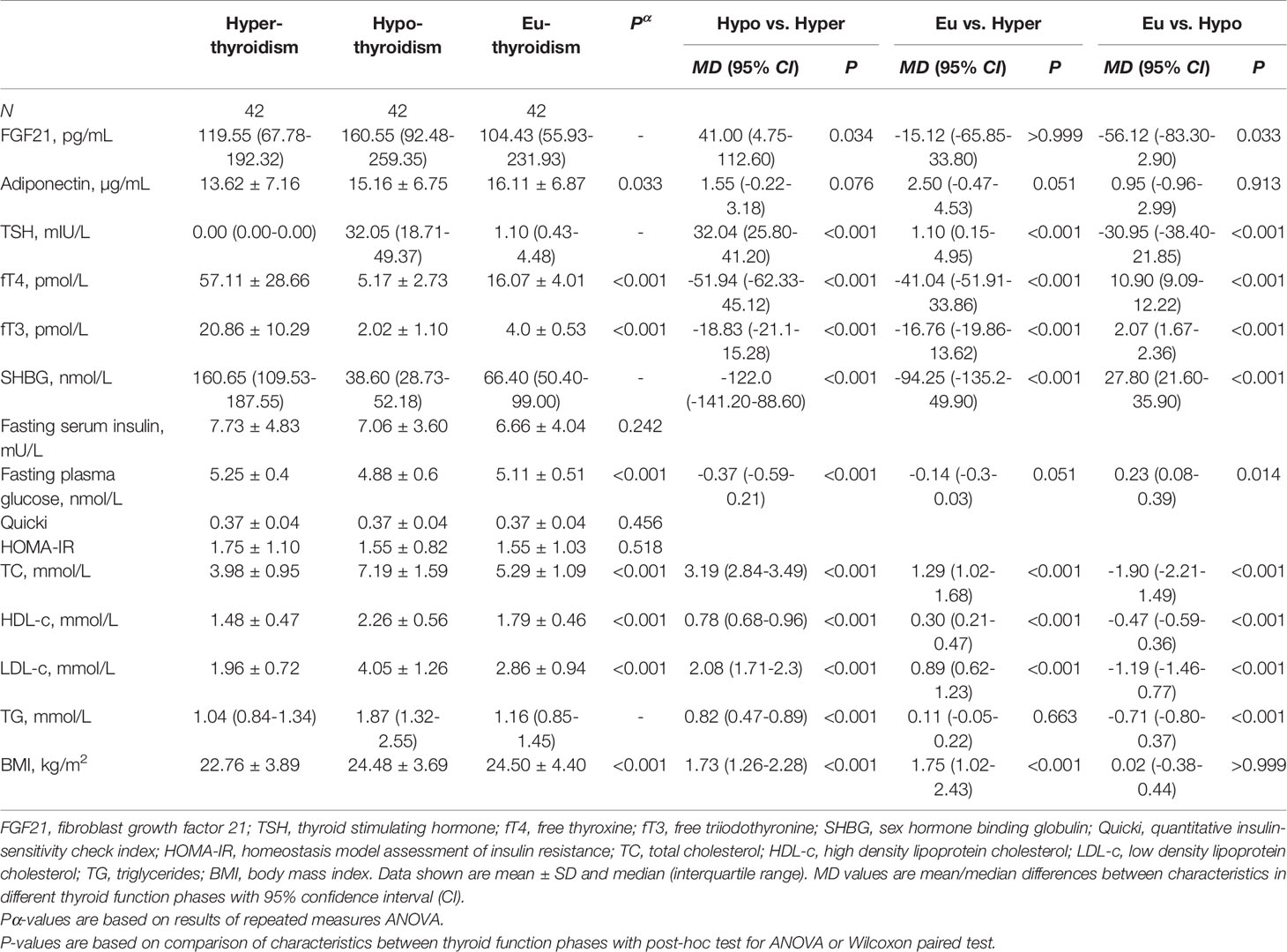
Table 2 Serum FGF21 and biochemical parameters after RAI treatment in hyper-, hypo-, and euthyroid phase.
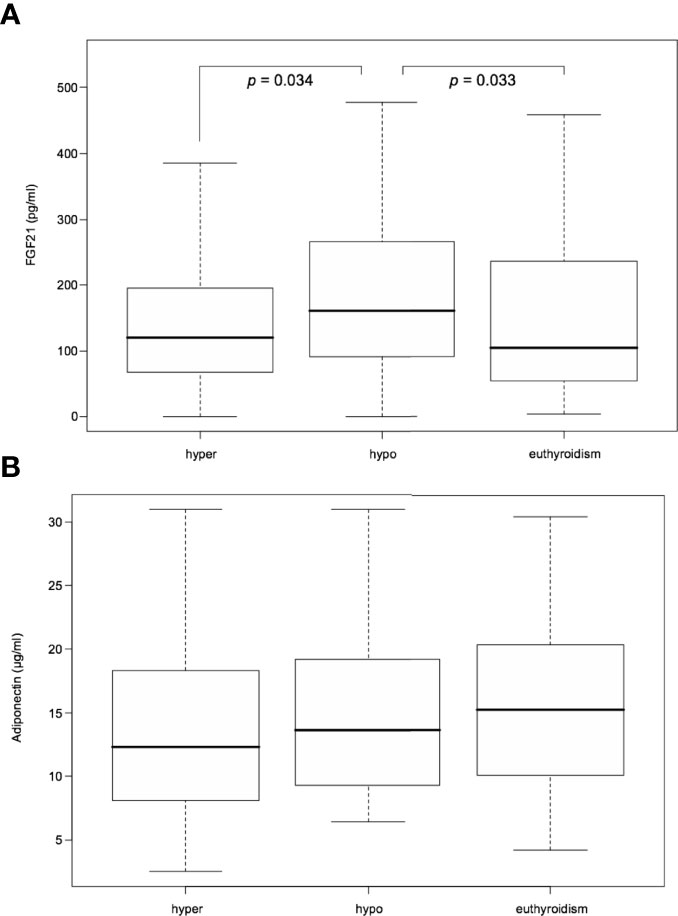
Figure 3 Serum FGF21 (A) and adiponectin (B) levels after RAI treatment in hyper-, hypo-, and euthyroid phase. FGF21, fibroblast growth factor 21. Data presented as median (interquartile range). P<0.05 was considered significant.
Elevated Serum FGF21 in Rapid-Onset Hypothyroidism After RAI Treatment Correlated Positively With Triglycerides, and Negatively With SHBG
There was no significant correlation between FGF21 serum levels and TH in any of the thyroid function states. Furthermore, there were no significant correlations found between serum FGF21 levels and lipids, as well as glucose metabolism parameters in patients with hyperthyroidism (Suppl. Table 8). On the contrary, during rapid-onset hypothyroidism following RAI treatment, rising serum FGF21 levels correlated significantly and positively with rising serum triglycerides (Spearman coefficient rs=0.36, P=0.017) and inversely with falling serum SHBG (rs=-0.41, P=0.007) (Figure 4 and Suppl. Table 9). The correlation disappeared during the subsequent euthyroid phase (Suppl. Table 10). Additionally, there were no relationships found of serum FGF21 levels and cholesterol, as well as glucose metabolism parameters during RAI-induced hypothyroidism (Suppl. Table 9). Furthermore, serum FGF21 levels did not show any significant correlation with the estimated variables in the euthyroid phase (Suppl. Table 10). Moreover, there were no significant correlations observed between serum FGF21 concentration and body composition parameters in women and men in any of the thyroid function states (Suppl. Tables 11–16).
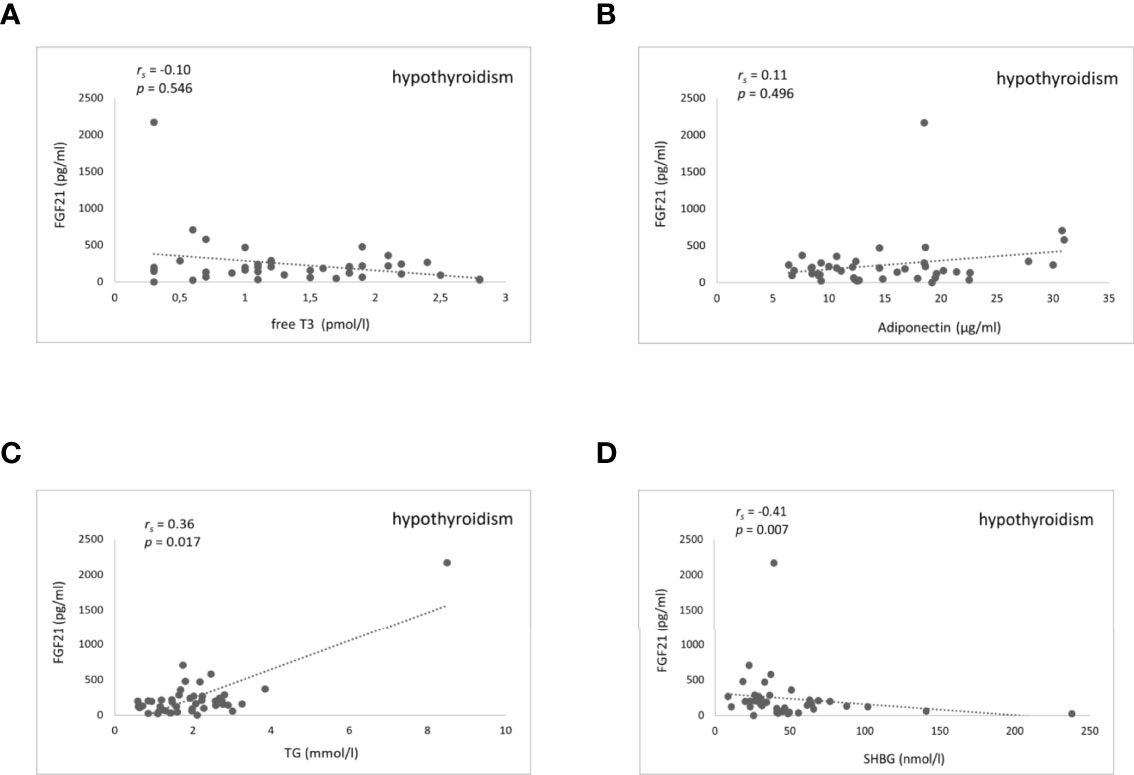
Figure 4 Scatter plots showing FGF21 and fT3, adiponectin, triglycerides, and SHBG serum levels in hypothyroidism after RAI treatment. Relationship between serum FGF21 levels and fT3 (A) adiponectin (B) triglycerides (C) and SHBG (D). FGF21, fibroblast growth factor 21; fT3, free triiodothyronine; TG, triglycerides; SHBG, sex hormone binding globulin. rs represents Spearman correlation coefficient. P<0.05 was considered significant.
There Was no Significant Correlation Between Serum FGF21 and Adiponectin
We did not observe correlations between FGF21 and adiponectin in any of the thyroid function states. Unlike FGF21, there was no correlation found of serum adiponectin, and TG, as well as SHBG concentrations in rapid-onset hypothyroidism after RAI treatment. In hyper- and hypothyroidism, adiponectin correlated positively with age (rs=0.26, P=0.020 and rs=0.43, P=0.004, respectively), although this relationship disappeared in the euthyroid phase. There was significant positive correlation with HDL-c in hyper-, hypo- and euthyroidism (rs=0.49, P<0.001, rs=0.44, P=0.003, rs=0.49, P=0.001, respectively), with total cholesterol in hyperthyroidism (rs=0.32, P=0.004) and with total cholesterol and LDL-c in hypothyroidism (rs=0.48, P=0.001 and rs=0.35, P=0.020, respectively). Moreover, adiponectin correlated inversely with BMI in the euthyroid phase (rs=-0.33, P=0.039) which was not seen in hyper- and hypothyroidism (Suppl Tables 8–10). In hyper and hypothyroid females, adiponectin correlated negatively with BMC (rs=-0.30, P=0.012 and rs=-0.49, P=0.002, respectively), whereas in the euthyroid phase the inverse correlation between adiponectin and both BMC (rs=-0.46, P=0.007) and LTM (rs=-0.37, P=0.036) occurred (Suppl Tables 11–16).
Discussion
To the best of our knowledge, this study is the first to examine TH influence on FGF21 secretion in a pairwise comparison of three different thyroid functions, namely hyper-, hypo-, and euthyroidism. In our group of RAI-treated patients, dramatic thyroid and metabolic changes occurred in a short period of time, which constitutes a favorable model of FGF21 regulatory pathways. Moreover, because of the wide interindividual variation in FGF21 serum levels in the general population, pairwise comparison represents a more reliable method of FGF21 assessment than intercohort comparison (40). However, even in these favorable settings, we did not demonstrate a stimulatory effect of TH on FGF21 release. Furthermore, we did not observe any correlation between FGF21 and TH in hyper-, hypo-, and euthyroidism.
Our results stay in agreement with studies by Bonde and colleagues, in which neither reduction in FGF21 serum level in 20 hyperthyroid patients after achieving euthyroidism was seen, nor treatment of 14 healthy subjects with liver-specific beta thyroid receptor analog eprotirome cause any change in FGF21 serum level (6). On the contrary, Xiao and colleagues demonstrated elevated FGF21 serum levels in 119 Graves’ disease hyperthyroid patients compared to healthy controls. In a subset of 41 patients from among this group, circulating FGF21 concentration declined by 59% after three months of thionamide treatment when euthyroidism had been established (7). In both studies, there was no correlation between FGF21 and T3 in hyperthyroidism, which suggests no cause-and-effect relationship and is consistent with our results.
Interestingly, both animal and cell culture studies demonstrate interrelation between TH and FGF21 secretion. It has been confirmed that T3 induces transcription of the Fgf21 gene in mouse liver and human HepG2-TRβ hepatocyte culture (4). This effect is mediated through thyroid hormone receptor-β activation (TRβ) (5). However, expression of most hepatic T3-regulated genes is induced independently of FGF21 and ensues equally in both wild-type and a mouse strain with targeted deletion of Fgf21 gene (5). This leads to the conclusion that FGF21 is dispensable for the majority of TH action in the liver.
Although we did not find a relationship between hyperthyroidism and FGF21 secretion, we have observed a transient FGF21 rise during rapid-onset hypothyroidism after RAI treatment. In this setting, FGF21 serum level positively correlated with rising serum triglycerides, and negatively with SHBG, which was typically reduced in hypothyroidism. Additionally, no correlation was found between serum FGF21 levels and TH. Because FGF21 strongly induces hepatic FFA oxidation (19), we hypothesized that sudden β-oxidation breakdown due to TH decline triggers FGF21 release as a compensatory reaction.
TH are a key player in FFA metabolism. The mainstay of this action is the induction of carnitine palmitoyltransferase- Iα (CPT-Iα), which is the rate-limiting enzyme in the hepatic β-oxidation pathway (41, 42). CPT-Iα converts long-chain fatty acyl-CoAs to acylcarnitines for transport across the mitochondrial membrane for β-oxidation (43). We hypothesized, that in rapid-onset hypothyroidism, CPT-Iα activity suddenly declines with a subsequent inhibition of FFA oxidation and increased hepatic lipogenesis. In this setting, the rise in serum triglycerides is a marker of intrahepatic mitochondrial β-oxidation breakdown and induction of lipogenesis. Moreover, sudden SHBG reduction in the hypothyroid state may favor hepatic lipid accretion. It has been demonstrated that enhanced de novo lipogenesis in the liver, a process consisting of conversion of acetyl-coenzyme A to free fatty acids, is crucial for NAFLD development and can down regulate SHBG synthesis (44, 45). Additionally, in human liver biopsy samples, enhanced TG accumulation and acetyl-coenzyme A carboxylase (ACC) mRNA expression, being the rate limiting enzyme controlling de novo lipogenesis, correlates negatively with SHBG mRNA and protein levels. Furthermore, SHBG overexpression decreases ACC expression induced by high-glucose culture conditions in HepG2 cells (46).. This implicates a protective function of SHBG in a fatty liver formation and is consistent with our results, where falling SHBG serum concentration associated with FGF21 release.
It had been shown previously that exogenous administration of FGF21 significantly lowers circulating and intrahepatic triglycerides, reverses fatty liver, and reduces signs of NASH and hepatic fibrosis (23). Administration of the FGF21 analogue pegbelfermin in humans with NASH causes at least a 30% relative reduction in hepatic fat content assessed in magnetic resonance imaging-proton density fat fraction in over half of the patients and a significant reduction of serum triglyceride levels (23). FGF21 increases fatty acid oxidation in the liver but also reduces lipid flux into the liver by promoting triglyceride-rich lipoproteins catabolism in adipose tissue and suppression of white adipose tissue postprandial lipolysis (19–21). However, these data emerge from animal experiments or human studies after exogenous administration of FGF21 analogues, which do not fully reflect physiology. In our study, we confirmed the physiological endogenous rise of FGF21 secretion accompanying sudden lipid overload, demonstrating its protective function against mitochondrial stress. Indeed, hepatic transcription of fgf21 gene in mice is triggered by endoplasmic reticulum (17) and oxidative stress (16) resulting from obesity and NAFLD as a compensatory reaction. On the other hand, FGF21 resistance due to attenuated receptor signaling and consequently impaired induction of target genes in diet-induced obesity (DIO) mice has also been described (38). It leads to the conclusion that both mechanisms can be involved and increased FGF21 serum levels in obesity related comorbidities may be a concert of compensatory increased secretion and receptor resistance.
In our study, we did not observe the link between FGF21 and adiponectin. In rapid-onset hypothyroidism after RAI treatment, rising FGF21 serum concentration was not accompanied by augmented adiponectin secretion. Moreover, adiponectin did not correlate with rising FGF21 serum levels. Therefore, we have not confirmed the hypothesis that the hepatic action of FGF21 is mediated indirectly via increased adiponectin secretion (27), a result that is consistent with other studies demonstrating adiponectin is dispensable for FGF21 action (28).
One limitation of our study was the relatively small sample size and inequality of sex distribution, reducing the strength of the sex-specific body composition assessment.
In conclusion, this study did not exhibit interdependency between TH and FGF21 secretion. Serum FGF21 concentrations in hyper- and hypothyroidism did not differ in comparison to the control group. Furthermore no correlation between FGF21 and T3 has been demonstrated.
Instead, we observed a significant rise of FGF21 serum level in conditions of metabolic challenge of rapid-onset hypothyroidism after RAI treatment. This rise demonstrated a significant positive correlation with rising serum triglyceride and negative correlation with falling SHBG concentration. We conclude that FGF21 induction in physiological conditions of increased hepatic lipogenesis suggests a compensatory reaction protecting the liver against excessive triglyceride accumulation.
Data Availability Statement
The datasets presented in this study can be found in online repositories. The names of the repository/repositories and accession number(s) can be found in the article/Supplementary Material.
Ethics Statement
The studies involving human participants were reviewed and approved by Bioethical Committee of Centre of Postgraduate Medical Education, Warsaw, Poland. The patients/participants provided their written informed consent to participate in this study.
Author Contributions
ES, WZ and MG-C designed the project. The first draft of the manuscript was written by ES. ES, WZ, JS-S, HJ, and MG-C wrote, reviewed, and edited the manuscript. Data collection was performed by ES. PG performed laboratory measurements and reviewed the manuscript. ES performed the statistical analysis. All authors contributed to the final version of the manuscript and approved it for publication.
Funding
This study was supported by grant of the Centre of Postgraduate Medical Education (No. 506-1-08-02).
Conflict of Interest
The authors declare that the research was conducted in the absence of any commercial or financial relationships that could be construed as a potential conflict of interest.
Publisher’s Note
All claims expressed in this article are solely those of the authors and do not necessarily represent those of their affiliated organizations, or those of the publisher, the editors and the reviewers. Any product that may be evaluated in this article, or claim that may be made by its manufacturer, is not guaranteed or endorsed by the publisher.
Acknowledgments
We thank Prof. Stefan Zgliczyński for his invaluable advice in formulating the research questions and methodology.
Supplementary Material
The Supplementary Material for this article can be found online at: https://www.frontiersin.org/articles/10.3389/fendo.2022.900034/full#supplementary-material
References
1. Coskun T, Bina HA, Schneider MA, Dunbar JD, Hu CC, Chen Y, et al. Fibroblast Growth Factor 21 Corrects Obesity in Mice. Endocrinology (2008) 149:6018–27. doi: 10.1210/en.2008-0816
2. Xu J, Lloyd DJ, Hale C, Stanislaus S, Chen M, Sivits G, et al. Fibroblast Growth Factor 21 Reverses Hepatic Steatosis, Increases Energy Expenditure, and Improves Insulin Sensitivity in Diet- Induced Obese Mice. Diabetes (2009) 58:250–9. doi: 10.2337/db08-0392
3. Talukdar S, Zhou Y, Li D, Rossulek M, Dong J, Somayaji V, et al. A Long-Acting FGF21 Molecule, PF-05231023, Decreases Body Weight and Improves Lipid Profile in non-Human Primates and Type 2 Diabetic Subjects. Cell Metab (2016) 23:427–40. doi: 10.1016/j.cmet.2016.02.001
4. Adams AC, Astapova I, Fisher FM, Badman MK, Kurgansky KE, Flier JS, et al. Thyroid Hormone Regulates Hepatic Expression of Fibroblast Growth Factor 21 in a Pparα-Dependent Manner. J Biol Chem (2010) 285:14078–82. doi: 10.1074/jbc.C110.107375
5. Zhang A, Sieglaff DH, York JP, Suh JH, Ayers SD, Winnier GE, et al. Thyroid Hormone Receptor Regulates Most Genes Independently of Fibroblast Growth Factor 21 in Liver. J Endocrinol (2015) 224:289–301. doi: 10.1530/JOE-14-0440
6. Bonde Y, Breuer O, Lutjohann D, Angelin B, Rudling M. Thyroid Hormone Reduces PCSK9 and Stimulates Bile Acid Synthesis in Humans. J Lipid Res (2014) 55:2408–15. doi: 10.1194/jlr.M051664
7. Xiao F, Lin M, Huang P, Zeng J, Zeng X, Zhang H, et al. Elevated Serum Fibroblast Growth Factor 21 Levels in Patients With Hyperthyroidism. J Clin Endocrinol Metab (2015) 100:3800–5. doi: 10.1210/jc.2015-1797
8. Beenken A, Mohammadi M. The Structural Biology of the FGF19 Subfamily. In: Kuro-o M, editor. Endocrine FGFs and Klothos. Advances in Experimental Medicine and Biology, vol. 728 . New York, NY: Springer (2012). p. 1–24. doi: 10.1007/978-1-4614-0887-1_1
9. BonDurant LD, Pothoff MJ. Fibroblast Growth Factor 21: A Versatile Regulator of Metabolic Homeostasis. Annu Rev Nutr (2018) 38:173–96. doi: 10.1146/annurev-nutr-071816-064800
10. Markan KR, Naber MC, Ameka MK, Anderegg MD, Mangelsdorf DJ, Kliewer SA, et al. Circulating FGF21 is Liver Derived and Enhances Glucose Uptake During Refeeding and Overfeeding. Diabetes (2014) 63:4057–63. doi: 10.2337/db14-0595
11. Inagaki T, Dutchak P, Zhao G, Ding X, Gautron L, Parameswara V, et al. Endocrine Regulation of the Fasting Response by Pparα-Mediated Induction of Fibroblast Growth Factor 21. Cell Metab (2007) 5:415–25. doi: 10.1016/j.cmet.2007.05.003
12. Badman MK, Pissios P, Kennedy A, Koukos G, Flier JS, Maratos-Flier E. Hepatic Fibroblast Growth Factor 21 is Regulated by Pparα and is a Key Mediator of Hepatic Lipid Metabolism in Ketotic States. Cell Metab (2007) 5:426–37. doi: 10.1016/j.cmet.2007.05.002
13. Iizuka K, Takeda J, Horikawa Y. Glucose Induces FGF21 mRNA Expression Through ChREBP Activation in Rat Hepatocytes. FEBS Lett (2009) 583:2882–6. doi: 10.1016/j.febslet.2009.07.053
14. Dushay JR, Toschi E, Mitten EK, Fisher FM, Herman MA, Maratos-Flier E. Fructose Ingestion Acutely Stimulates FGF21 Levels in Humans. Mol Metab (2015) 4:51–7. doi: 10.1016/j.molmet.2014.09.008
15. Laeger T, Henagan TM, Albarado DC, Redman LM, Bray GA, Noland RC, et al. FGF21 is an Endocrine Signal of Protein Restriction. J Clin Invest (2014) 124:3913–22. doi: 10.1172/JCI74915
16. Ye D, Wang Y, Li H, Jia W, Man K, Lo CM, et al. Fibroblast Growth Factor 21 Protects Against Acetaminophen-Induced Hepatotoxicity by Potentiating Peroxisome-Proliferator-Activated Receptor Coactivator Protein-1α-Mediated Antioxidant Capacity in Mice. Hepatology (2014) 60:977–89. doi: 10.1002/hep.27060
17. Kim SH, Kim KH, Kim H-K, Kim M-J, Back SH, Konishi M, et al. Fibroblast Growth Factor 21 Participates in Adaptation to Endoplasmic Reticulum Stress and Attenuates Obesity-Induced Hepatic Metabolic Stress. Diabetologia (2015) 58:809–18. doi: 10.1007/s00125-014-3475-6
18. Jensen-Cody SO, Pothoff MJ. Hepatokines and Metabolism: Deciphering Communication From the Liver. Mol Metab (2021) 44:101138. doi: 10.1016/j.molmet.2020.101138
19. Pothoff MJ, Inagaki T, Satapati S, Ding X, He T, Goetz R, et al. FGF21 Induces PGC-1α and Regulates Carbohydrate and Fatty Acid Metabolism During the Adaptive Starvation Response. Proc Natl Acad Sci USA (2009) 106:10853–8. doi: 10.1073/pnas.0904187106
20. Schlein C, Talukdar S, Heine M, Fisher AW, Krott LM, Nilsson SK, et al. FGF21 Lowers Plasma Triglycerides by Accelerating Lipoprotein Catabolism in White and Brown Adipose Tissues. Cell Metab (2016) 23:441–53. doi: 10.1016/j.cmet.2016.01.006
21. Kaufman A, Abuqayyas L, Denney WS, Tillman EJ, Rolph T. AKR-001, an Fc-FGF21 Analog, Showed Sustained Pharmacodynamic Effects on Insulin Sensitivity and Lipid Metabolism in Type 2 Diabetes Patients. Cell Rep Med (2020) 1:100057. doi: 10.1016/j.xcrm.2020.100057
22. Harrison SA, Rinella ME, Abdelmalek MF, Trotter JF, Paredes AH, Arnold HL, et al. NGM282 for Treatment of non-Alcoholic Steatohepatitis: A Multicentre, Randomised, Double-Blind, Placebo-Controlled, Phase 2 Trial. Lancet (2018) 391:1174–85. doi: 10.1016/S0140-6736(18)30474-4
23. Sanyal A, Charles ED, Neuschwander-Tetri B, Loomba R, Harrison SA, Abdelmalek MF, et al. Pegbelfermin (BMS-986036), a PEGylated Fibroblast Growth Factor 21 Analogue, in Patients With non-Alcoholic Steatohepatitis: A Randomised, Double-Blind, Placebo-Controlled, Phase 2a Trial. Lancet (2018) 392:2705–17. doi: 10.1016/S0140-6736(18)31785-9
24. Charles ED, Neuschwander-Tetri B, Frias JP, Kundu S, Luo Y, Tirucherai GS, et al. Pegbelfermin (BMS-986036), PEGylated FGF21, in Patients With Obesity and Type 2 Diabetes: Results From a Randomized Phase 2 Study. Obesity (2019) 27:41–9. doi: 10.1002/oby.22344
25. Fisher FM, Estall JL, Adams AC, Antonellis PJ, Bina HA, Flier JS, et al. Integrated Regulation of Hepatic Metabolism by Fibroblast Growth Factor 21 (FGF21) In Vivo. Endocrinology (2011) 152:2996–3004. doi: 10.1210/en.2011-0281
26. Kurosu H, Choi M, Ogawa Y, Dickson AS, Goetz R, Eliseenkova AV, et al. Tissue-Specific Expression of βklotho and Fibroblast Growth Factor (FGF) Receptor Isoforms Determines Metabolic Activity of FGF19 and FGF21. J Biol Chem (2007) 282:26687–95. doi: 10.1074/jbc.M704165200
27. Holland WL, Adams AC, Brozinick JT, Bui HH, Miyauchi Y, Kusminski CM, et al. An FGF21-Adiponectin-Ceramide Axis Controls Energy Expenditure and Insulin Action in Mice. Cell Metab (2013) 17:790–7. doi: 10.1016/j.cmet.2013.03.019
28. Bon Durant LD, Ameka M, Naber MC, Markan KR, Idiga SO, Acevedo MR, et al. FGF21 Regulates Metabolism Through Adipose-Dependent and -Independent Mechanisms. Cell Metab (2017) 25:935–44. doi: 10.1016/j.cmet.2017.03.005
29. Han MS, Perry RJ, Camporez JP, Scherer PE, Shulman GI, Gao G, et al. A Feed-Forward Regulatory Loop in Adipose Tissue Promotes Signalling by the Hepatokine FGF21. Genes Dev (2021) 35:133–46. doi: 10.1101/gad.344556.120
30. Kharitonenkov A, Shiyanova TL, Koester A, Ford AM, Micanovic R, Galbreath EJ, et al. FGF-21 as a Novel Metabolic Regulator. J Clin Invest (2005) 115:1627–35. doi: 10.1172/JCI23606
31. Bookout AL, de Groot MHM, Owen BM, Lee S, Gautron L, Lawrence HL, et al. FGF21 Regulates Metabolism and Circadian Behavior by Acting on the Nervous System. Nat Med (2013) 19:1147–52. doi: 10.1038/nm.3249
32. von Holstein-Rathlou S, Bon Durant LD, Peltekian L, Naber MC, Yin TC, Claflin KE, et al. FGF21 Mediates Endocrine Control of Simple Sugar Intake and Sweet Taste Preference by the Liver. Cell Metab (2016) 23:335–43. doi: 10.1016/j.cmet.2015.12.003
33. Lewis JE, Monnier C, Marshall H, Fowler M, Green R, Cooper S, et al. Whole-Body and Adipose Tissue- Specific Mechanisms Underlying the Metabolic Effects of Fibroblast Growth Factor 21 in the Siberian Hamster. Mol Metab (2020) 31:45–54. doi: 10.1016/j.molmet.2019.10.009
34. Huang Z, Zhong L, Lee JTH, Zhang J, Wu D, Geng L, et al. The FGF21–CCL11 Axis Mediates Beiging of White Adipose Tissues by Coupling Sympathetic Nervous System to Type 2 Immunity. Cell Metab (2017) 26:493–508. doi: 10.1016/j.cmet.2017.08.003
35. Jensen-Cody SO, Flippo KH, Claflin KE, Yavuz Y, Sapouckey SA, Walters GC, et al. FGF21 Signals to Glutamatergic Neurons in the Ventromedial Hypothalamus to Suppress Carbohydrate Intake. Cell Metab (2020) 32:273–86. doi: 10.1016/j.cmet.2020.06.008
36. Zhang X, Yeung DCY, Karpisek M, Stejskal D, Zhou ZG, Liu F, et al. Serum FGF21 Levels are Increased in Obesity and are Independently Associated With the Metabolic Syndrome in Humans. Diabetes (2008) 57:1246–53. doi: 10.2337/db07-1476
37. Li H, Dong K, Fang Q, Hou X, Zhou M, Bao Y, et al. High Serum Level of Fibroblast Growth Factor 21 is an Independent Predictor of non-Alcoholic Fatty Liver Disease: A 3-Year Prospective Study in China. J Hepatol (2013) 58:557–63. doi: 10.1016/j.jhep.2012.10.029
38. Fisher FM, Chui PC, Antonellis PJ, Bina HA, Kharitonenkov A, Flier JS, et al. Obesity is a Fibroblast Growth Factor 21 (FGF21)-Resistant State. Diabetes (2010) 59:2781–9. doi: 10.2337/db10-0193
39. Hale C, Chen MM, Stanislaus S, Chinookoswong N, Hager T, Wang M, et al. Lack of Overt FGF21 Resistance in Two Mouse Models of Obesity and Insulin Resistance. Endocrinology (2012) 153:69–80. doi: 10.1210/en.2010-1262
40. Gälman C, Lundåsen T, Kharitonenkov A, Bina HA, Eriksson M, Hafström I, et al. The Circulating Metabolic Regulator FGF21 is Induced by Prolonged Fasting and Pparα Activation in Man. Cell Metab (2008) 8:169–74. doi: 10.1016/j.cmet.2008.06.014
41. Jackson-Hayes L, Song S, Lavrentyev EN, Wood PA, Cook GA, Park EA. A Thyroid Hormone Response Unit Formed Between the Promoter and First Intron of the Carnitine Palmitoyltransferase-Ialpha Gene Mediates the Liver- Specific Induction by Thyroid Hormone. J Biol Chem (2003) 278:7964 –72. doi: 10.1074/jbc.M211062200
42. Zhang Y, Ma K, Song S, Elam MB, Cook GA, Park EA. Peroxisomal Proliferator- Activated Receptor-Gamma Coactivator-1 Alpha (PGC-1 Alpha) Enhances the Thyroid Hormone Induction of Carnitine Palmitoyltransferase I (CPT-I Alpha). J Biol Chem (2004) 279:53963–71. doi: 10.1074/jbc.M406028200
43. Bonnefont JP, Djouadi F, Prip-Buus C, Gobin S, Munnich A, Bastin J. Carnitine Palmitoyltransferases 1 and 2: Biochemical, Molecular and Medical Aspects. Mol Aspects Med (2004) 25:495–520. doi: 10.1016/j.mam.2004.06.004
44. Kawano Y, Cohen DE. Mechanisms of Hepatic Triglyceride Accumulation in non-Alcoholic Fatty Liver Disease. J Gastroenterol (2013) 48:434–41. doi: 10.1007/s00535-013-0758-5
45. Selva DM, Hogeveen KN, Innis SM, Hammond GL. Monosaccharide- Induced Lipogenesis Regulates the Human Hepatic Sex Hormone-Binding Globulin Gene. J Clin Invest (2007) 117:3979–87. doi: 10.1172/JCI32249
Keywords: FGF21, hyperthyroidism, hypothyroidism, SHBG, triglycerides, lipogenesis, NAFLD
Citation: Szczepańska E, Glinicki P, Zgliczyński W, Słowińska-Srzednicka J, Jastrzębska H and Gietka-Czernel M (2022) FGF21 Is Released During Increased Lipogenesis State Following Rapid-Onset Radioiodine-Induced Hypothyroidism. Front. Endocrinol. 13:900034. doi: 10.3389/fendo.2022.900034
Received: 19 March 2022; Accepted: 20 June 2022;
Published: 14 July 2022.
Edited by:
Marian Elizabeth Ludgate, Cardiff University, United KingdomReviewed by:
Nadia Sawicka-Gutaj, Poznan University of Medical Sciences, PolandEwelina Szczepanek-Parulska, Poznan University of Medical Sciences, Poland
Copyright © 2022 Szczepańska, Glinicki, Zgliczyński, Słowińska-Srzednicka, Jastrzębska and Gietka-Czernel. This is an open-access article distributed under the terms of the Creative Commons Attribution License (CC BY). The use, distribution or reproduction in other forums is permitted, provided the original author(s) and the copyright owner(s) are credited and that the original publication in this journal is cited, in accordance with accepted academic practice. No use, distribution or reproduction is permitted which does not comply with these terms.
*Correspondence: Ewa Szczepańska, ZXN6Y3plcGFuc2thQGNta3AuZWR1LnBs; Piotr Glinicki, cGdsaW5pY2tpQGNta3AuZWR1LnBs