- 1Department of Endocrinology, Beijing Hospital, National Center of Gerontology, Institute of Geriatric Medicine, Chinese Academy of Medical Science, Beijing, China
- 2Department of Endocrinology, Beijing Friendship Hospital, Capital Medical University, Beijing, China
Our study aimed to evaluate the exposure–response relationship between incretin-based medications and the risk of major adverse cardiovascular events (MACE) using cardiovascular outcome trials (CVOTs). Eleven CVOTs with incretin-based medications were included. The median follow-up time, percentage of time exposure, and hazard ratio (HR) of MACE were obtained from each CVOT. The pharmacokinetic parameters of glucagon-like peptide-1 receptor agonists (GLP-1 RAs) and dipeptidyl peptidase-4 inhibitor (DPP-4) were obtained from published studies. Regression analysis was performed to assess the relationship between drug exposure and MACE HR. Cutoff values were determined from the ROC curves. The linear regression results indicated that log Cmax, log AUC0–24h, and log AUCCVOT are negatively correlated with MACE HR (R2 = 0.8494, R2 = 0.8728, and R2 = 0.8372, respectively; all p < 0.0001). The relationship between drug exposure (log Cmax, log AUC0–24h, and log AUCCVOT) and MACE HR strongly corresponded with the log (inhibitor) vs. response curve (R2 = 0.8383, R2 = 0.8430, and R2 = 0.8229, respectively). The cutoff values in the ROC curves for log Cmax, log AUC0–24h, and log AUCCVOT, were 2.556, 3.868, and 6.947, respectively (all p = 0.007). A Fisher’s exact test revealed that these cutoff values were significantly related to cardiovascular benefits (all p < 0.05). Our study revealed a linear exposure–response relationship between drug exposure and MACE HR. We conclude that the cardiovascular benefits of incretin-based therapies may occur with higher doses of GLP-1 RAs and with increased exposure.
Introduction
Type 2 diabetes (T2DM) is frequently accompanied by various cardiovascular complications. Further, cardiovascular complications are the leading cause of disability and death in patients with T2DM (1). Numerous in vitro studies and animal studies have investigated the effects of dipeptidyl peptidase-4 (DPP-4) inhibitors and GLP-1 receptor agonists (GLP-1 RAs) on the cardiovascular system (2, 3). Considering the results of cardiovascular outcome trials (CVOTs) with incretin-based medications, significant heterogeneity in cardiovascular effects could be found between DPP-4 inhibitors and GLP-1 RAs. While the results obtained with DPP-4 inhibitors (alogliptin, saxagliptin, sitagliptin, and linagliptin) demonstrated cardiovascular safety (4–7), the majority of GLP-1 RAs (including liraglutide, albiglutide, dulaglutide, and subcutaneous semaglutide) significantly decreased the risk of major adverse cardiovascular events (MACE) (8–11). Furthermore, the meta-analysis results suggested that the GLP-1RA class could reduce the risk of MACE, cardiovascular mortality, and all-cause mortality (12). However, the results of the ELIXA study with lixisenatide (cardiovascular safety) (13), the PIONEER 6 study with oral semaglutide (cardiovascular safety) (14), and the EXSCEL study with extended exenatide (a trend toward reduction in MACE) (15), cast doubt on the existence of a class effect for GLP-1 RAs.
Although our overall understanding of the cardiovascular benefits of GLP-1RAs has evolved over time, head-to-head comparisons between different incretin-based drugs in the completed CVOTs have been lacking. Hence, differences in baseline characteristics/trial execution may account for the divergent MACE results. However, evidence from several studies suggest associations between cardiovascular risk reduction and HbA1c reduction (16), non-glycemic effects (17), or time of exposure to GLP-1 RAs (18). In the present study, we evaluated the exposure–response relationship of incretin-based medications by determining the effects of drug exposure on MACE risk in cardiovascular outcome trials.
Research Design and Methods
In total, 11 CVOTs comparing add-on therapy using a DPP-4 inhibitor or GLP-1RA with a placebo were included. HbA1c reduction, weight loss, median follow-up time, percentage of time exposure to the trial drug, and hazard ratio (HR) of 3-point MACE in each individual CVOT were obtained from the records. Although the plasma concentration of GLP-1 RAs or GLP-1 was not measured in the CVOTs, the clinical pharmacokinetic and pharmacodynamic properties of these trial drugs have previously been established and published. The maximum observed plasma concentration of GLP-1 RA or active GLP-1 (Cmax), the mean area under the curve (AUC0–24h) with multiple doses, and the total AUC in CVOT (AUCCVOT) were used to assess drug exposure (Table 1). AUCCVOT was calculated by substituting the median follow-up time and percentage of time exposure to the trial drug using Eq. 1, as follows:
Measurements of normalized cyclic adenosine monophosphate (cAMP) production, induced by GLP-1 RAs (19), was used to calibrate Cmax, AUC0–24h, and AUCCVOT. Using the calibrated Cmax, calibrated AUC0–24h, and calibrated AUCCVOT, a sensitivity analysis was conducted to assess the exposure–response relationship.
Statistical Analyses
The values of Cmax, AUC0–24h, and AUCCVOT were obtained as continuous variables and analyzed after natural logarithmic transformation. Linear regression analysis was used to assess the relationship between drug exposure (log Cmax, log AUC0–24h, or log AUCCVOT) and MACE HR, HbA1c reduction, and weight loss. Nonlinear regression analysis was used to examine the exposure–response relationship by fitting a log (inhibitor) vs. response curve. Continuous variables were evaluated using receiver operating characteristic (ROC) curves. Cutoff values were determined following an assessment of the ROC curves. Fisher’s exact tests were used to compare categorical variables. A p value < 0.05 was used to determine statistical significance. All analyses were performed using GraphPad Prism 6 (GraphPad Software, San Diego, CA, USA).
Results
Drug Exposure
Cmax, AUC0–24h, and AUCCVOT of GLP-1 RAs and native GLP-1 are reported in Table 2. According to the pharmacodynamic studies of alogliptin, saxagliptin, sitagliptin, and linagliptin, the DPP4 inhibitors significantly increased native GLP-1 levels in patients with T2DM (compared with placebo), yielding an average level of ≤ 19.0 pmol/L (Table 2 and Figure S1) (20–23).
Pharmacokinetic studies of GLP-1 RAs revealed that the plasma concentrations of GLP-1 RAs in T2DM patients varied significantly. The peak plasma concentration of lixisenatide was 187.2 pg/mL (38.5 pmol/L) after multiple daily injections (24), while the steady-state plasma concentration of once-weekly exenatide reached 300 pg/mL (71.4 pmol/L) after multiple dosing (25). In contrast, several different pharmacokinetic studies have revealed that the steady-state concentrations of other GLP-1 RAs (including dulaglutide, liraglutide, albiglutide, subcutaneous semaglutide, and oral semaglutide) reach substantially higher (nanomolar) levels (Table 2 and Figure S1) (26–30).
MACE Risk in CVOTs
According to the results of our statistical analysis of the primary outcomes in the CVOTs, several of the add-on therapies demonstrated cardiovascular noninferiority when compared with placebo. These included alogliptin [EXAMINE trial, HR, 0.96; p < 0.001 for noninferiority and p = 0.32 for superiority] (4), saxagliptin (SAVOR-TIMI 53 trial, HR, 1.00; p < 0.001 for noninferiority and p = 0.99 for superiority) (5), sitagliptin (TECOS trial, HR, 0.98; p < 0.001 for noninferiority and p = 0.65 for superiority) (6), linagliptin (CARMELINA trial, HR, 1.02; p < 0.001 for noninferiority and p = 0.74 for superiority) (7), lixisenatide (ELIXA trial, HR, 1.02; p < 0.001 for noninferiority and p = 0.81 for superiority) (13), once-weekly exenatide (EXSCEL trial, HR, 0.91; p < 0.001 for noninferiority and p = 0.06 for superiority) (15), and oral semaglutide (PIONEER 6 trial, HR, 0.79; p < 0.001 for noninferiority and p = 0.17 for superiority) (14).
Conversely, liraglutide (LEADER trial, HR, 0.87; p < 0.001 for noninferiority and p = 0.01 for superiority) (8), albiglutide (HARMONY trial, HR, 0.78; p < 0.0001 for noninferiority and p = 0.0006 for superiority) (9), dulaglutide (REWIND trial, HR, 0.88; p = 0.026 for superiority) (10), and subcutaneous semaglutide (SUSTAIN-6 trial, HR, 0.74; p < 0.0001 for noninferiority and p = 0.02 for superiority) (11) all demonstrated cardiovascular superiority over placebo (Table 2).
Exposure–Response Relationship
The linear regression results demonstrate that log Cmax, log AUC0–24h, and log AUCCVOT negatively correlate with MACE HR (R2 = 0.8494, R2 = 0.8728, and R2 = 0.8372, respectively; p < 0.0001; Figures 1A–C). The relationship between drug exposure (log Cmax, log AUC0–24h, or log AUCCVOT) and MACE HR showed a good correspondence with the fitted curve (R2 = 0.8383; R2 = 0.8430; R2 = 0.8229, respectively; Figures 1D–F). The ROC curve was used to evaluate drug exposure and define cutoff values (Figure 2). For the log Cmax, log AUC0–24h, and Log AUCCVOT ROC curves, the cutoff values were 2.556, 3.868, and 6.947, respectively (all p = 0.007). Detailed results reporting areas under the curve, sensitivity, and specificity are shown in Table 3. These cutoff values were all significantly related to cardiovascular superiority (all p < 0.05, Fisher’s exact test).
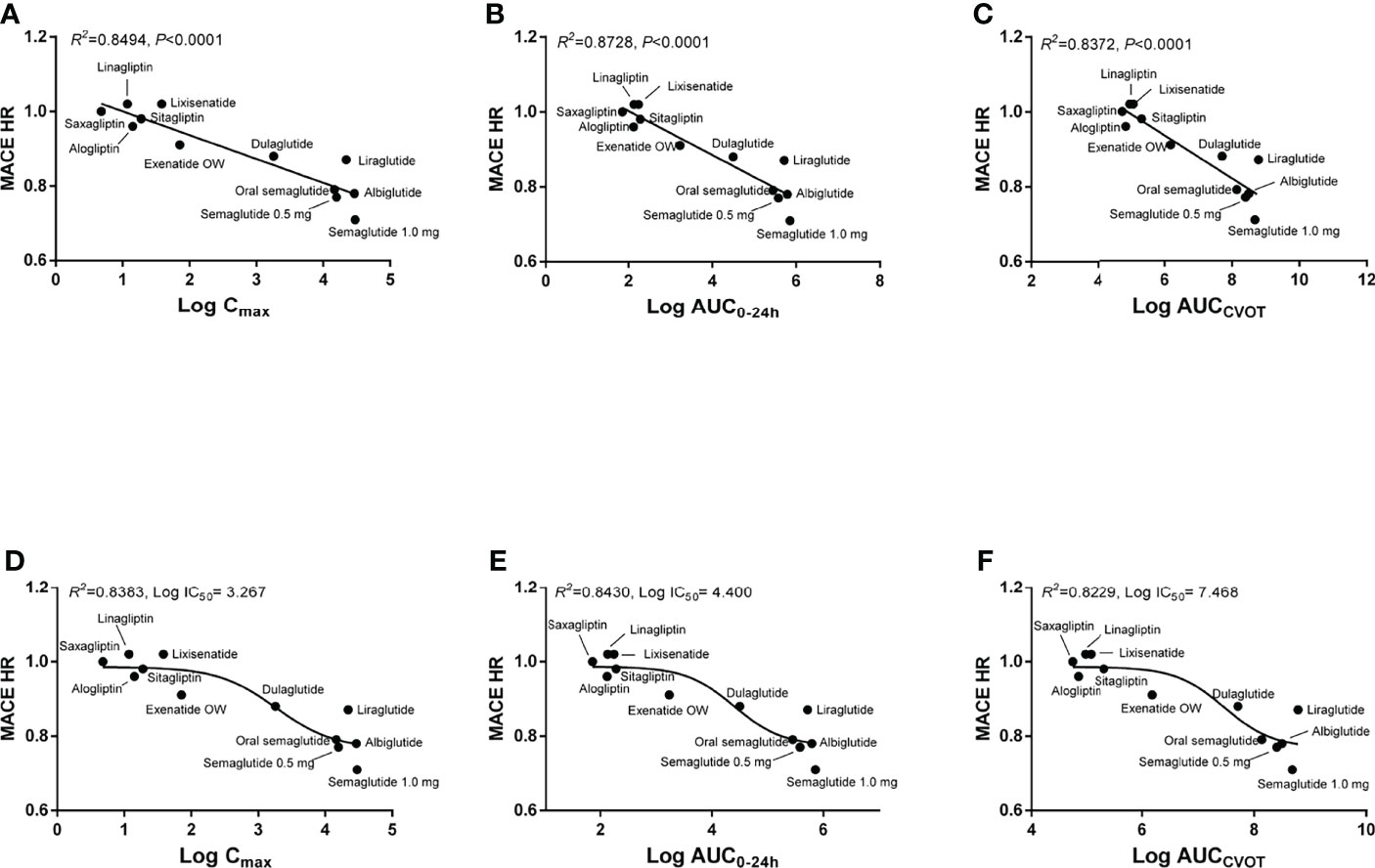
Figure 1 Correlation between drug exposure and MACE HR. (A) Linear regression analysis between log Cmax and MACE HR; (B) Linear regression analysis between log AUC0–24h and MACE HR; (C) Linear regression analysis between log AUCCVOT and MACE HR; (D) Nonlinear regression analysis between log Cmax and MACE HR; (E) Nonlinear regression analysis between log AUC0–24h and MACE HR; (F) Nonlinear regression analysis between log AUCCVOT and MACE HR.
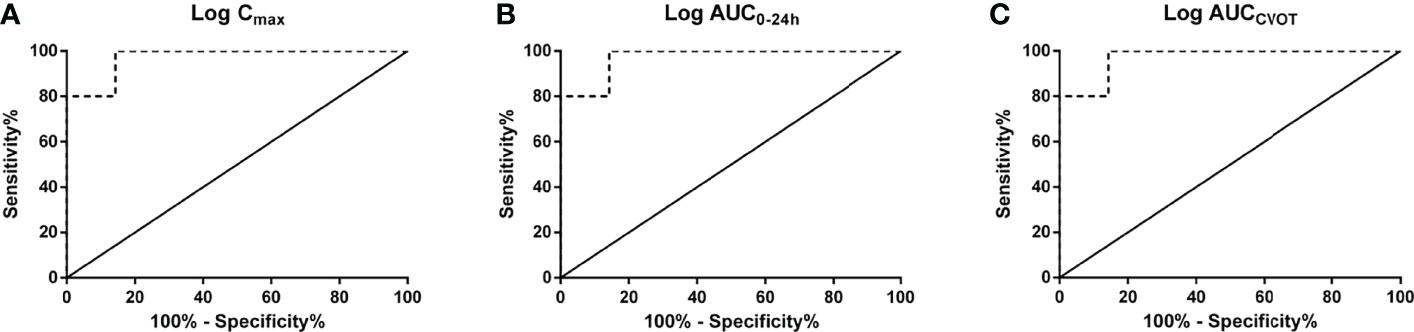
Figure 2 Receiver operating characteristic (ROC) curves. (A) log Cmax; (B) log AUC0–24h; (C) log AUCCVOT.
A similar correlation existed in the secondary prevention cohorts with a history of CVD (p < 0.01; Figure S2), but not in the primary prevention cohorts without a history of CVD (Supplementary Figure S3). The relationships between drug exposure and HbA1c reduction (compared with placebo) and between drug exposure and weight loss (compared with placebo) were also examined (Supplementary Figures S4, S5). In both instances, statistically significant relationships were observed (p < 0.01). A sensitivity analysis was also performed on calibrated Cmax, calibrated AUC0–24h, and calibrated AUCCVOT. The results were generally consistent with the results of the aforementioned analysis (Supplementary Figures S6–S10 and Table S1).
Discussion
In healthy subjects, basal plasma levels of native GLP-1 are generally below 10 pmol/L, while postprandial levels of GLP-1 rise to 10–30 pmol/L (31–33). In comparison, prediabetes or T2DM subjects generally demonstrate lower basal GLP-1 levels, and/or a reduction in GLP-1 response to oral glucose load (33). DPP4 inhibitors reportedly raise the postprandial levels of native GLP-1 approximately 2–4 fold (20, 34). However, the concentrations of most GLP-1 RAs (except lixisenatide and once-weekly exenatide) are substantially higher than the reported physiological concentrations of GLP-1.
Our results indicate that the exposure concentrations of DPP4 inhibitor (lixisenatide) and once-weekly exenatide associated with cardiovascular safety are low, as the concentrations of these drugs were close to physiological levels (picomolar levels). In contrast, the exposure concentrations of other GLP-1 RAs (except oral semaglutide), which are associated with cardiovascular benefits, are higher (nanomolar levels). A strong relationship between the exposure concentration and MACE HR was demonstrated through regression analysis (p < 0.0001). Moreover, a good fit was obtained between the observed relationship and the theoretically constructed model. The exposure–response relationship of GLP-1 was also demonstrated in a cross-sectional study, and linear regression analysis showed that higher glucose-stimulated GLP-1 levels were associated with clinically relevant lower blood pressure (associated with beneficial effects on the cardiovascular system) (35). In addition, a strong relationship was demonstrated between the mean and total drug exposure and MACE HR.
Drug exposure is dependent on both concentration and time. When only concentration was considered, our ROC results suggest that log Cmax > 2.556 (i.e., Cmax > 359.7 pmol/L) could be a predictor for cardiovascular benefits. However, when both concentration and time were considered, our results indicate that log AUC0–24h > 3.868 and log AUCCVOT > 6.947 could be predictors for cardiovascular superiority. These results suggest that the drug should be continuously used at a steady-state Cmax of 307.5 pmol/L per day for 3.29 years consecutively (the value was obtained by dividing AUCCVOT by AUC0–24h). Native GLP-1 levels when using DPP4 inhibitors and GLP-1 RA levels (lixisenatide and once-weekly exenatide) were both less than the predicted Cmax values. Hence, these regimens show cardiovascular safety only. For PIONEER 6, the median exposure time was only 15.9 months. We predict that better results may be obtained by extending the exposure time with oral semaglutide.
The extent to which exendin-4-based agonists differ in cardiovascular effects from GLP-1-based agonists has been extensively debated. Although CVOTs do not provide evidence of any cardiovascular benefits of using exendin-4-based agonists, there is no evidence to suggest that exendin-4-based agonists attenuate activation of GLP-1 receptor signaling (2). The present study demonstrates significant associations between GLP-1 RA drug exposure and HbA1c reduction and between GLP-1 RA drug exposure and weight loss. HbA1c reduction and weight loss may both play important roles in mediating MACE benefits (16, 36). Compared with other GLP-1 RAs, the therapeutic benefits (HbA1c reduction, weight loss, and cardiovascular benefits) of using recommended and approved doses of lixisenatide or once-weekly exenatide, which reflect a tradeoff between the adverse effects and the therapeutic benefits (HbA1c reduction and weight loss) observed in phase 2–3 studies, are significantly reduced (37). Suboptimal GLP-1 RA drug exposure with exendin-4-based agonists may be a critical cause of their lack of cardiovascular benefits (similar time exposure, but lower dose exposure).
The importance of supraphysiological doses of GLP-1 was originally proposed by J.J. Holst (38). Holst considered moderately elevated GLP-1 concentrations to have a significant effect on pancreatic islets, higher concentrations to slow gastric emptying and reduce food intake, and much higher concentrations to lead to side effects (nausea, diarrhea, and vomiting) (38). Our study provides evidence to support the notion that higher GLP-1 RA drug exposure is associated with additional cardiovascular benefit.
This study has several limitations. The parameters for drug exposure were obtained from different studies and may be biased. Although the parameters for drug exposure were calibrated using normalized cAMP, a degree of bias may be inevitable. In addition, our study is based on trial level analyses using the published literature, and not on patient level analyses. Thus, some inferences based on these results may ultimately prove to be misleading.
In conclusion, our study demonstrates a good exposure–response relationship between drug exposure and MACE HR. Our results suggest that the cardiovascular benefits of incretin-based therapies may occur with higher exposure to GLP-1 RAs.
Data Availability Statement
The original contributions presented in the study are included in the article/Supplementary Material. Further inquiries can be directed to the corresponding author.
Author Contributions
QP, MY, contributed to data collection and analysis. LG contributed to the study design and interpretation. All authors approved the manuscript. All authors contributed to the article and approved the submitted version.
Funding
This work was supported by the China International Medical Foundation (grant Z-2017-26-1902).
Conflict of Interest
The authors declare that the research was conducted in the absence of any commercial or financial relationships that could be construed as a potential conflict of interest.
Publisher’s Note
All claims expressed in this article are solely those of the authors and do not necessarily represent those of their affiliated organizations, or those of the publisher, the editors and the reviewers. Any product that may be evaluated in this article, or claim that may be made by its manufacturer, is not guaranteed or endorsed by the publisher.
Acknowledgments
The authors acknowledge Dr. Yale Duan for his help in statistical analysis and medical writing.
Supplementary Material
The Supplementary Material for this article can be found online at: https://www.frontiersin.org/articles/10.3389/fendo.2022.893971/full#supplementary-material
References
1. An Y, Zhang P, Wang J, Gong Q, Gregg EW, Yang W, et al. Cardiovascular and All-Cause Mortality Over a 23-Year Period Among Chinese With Newly Diagnosed Diabetes in the Da Qing IGT and Diabetes Study. Diabetes Care (2015) 38(7):1365–71. doi: 10.2337/dc14-2498
2. Drucker DJ. The Ascending GLP-1 Road From Clinical Safety to Reduction of Cardiovascular Complications. Diabetes (2018) 67(9):1710–9. doi: 10.2337/dbi18-0008
3. Bistola V, Lambadiari V, Dimitriadis G, Ioannidis I, Makrilakis K, Tentolouris N, et al. Possible Mechanisms of Direct Cardiovascular Impact of GLP-1 Agonists and DPP4 Inhibitors. Heart Fail Rev (2018) 23(3):377–88. doi: 10.1007/s10741-018-9674-3
4. White WB, Cannon CP, Heller SR, Nissen SE, Bergenstal RM, Bakris GL, et al. Alogliptin After Acute Coronary Syndrome in Patients With Type 2 Diabetes. N Engl J Med (2013) 369(14):1327–35. doi: 10.1056/NEJMoa1305889
5. Scirica BM, Bhatt DL, Braunwald E, Steg PG, Davidson J, Hirshberg B, et al. Saxagliptin and Cardiovascular Outcomes in Patients With Type 2 Diabetes Mellitus. N Engl J Med (2013) 369(14):1317–26. doi: 10.1056/NEJMoa1307684
6. Green JB, Bethel MA, Armstrong PW, Buse JB, Engel SS, Garg J, et al. Effect of Sitagliptin on Cardiovascular Outcomes in Type 2 Diabetes. N Engl J Med (2015) 373(3):232–42. doi: 10.1056/NEJMoa1501352
7. Rosenstock J, Perkovic V, Johansen OE, Cooper ME, Kahn SE, et al. Effect of Linagliptin vs Placebo on Major Cardiovascular Events in Adults With Type 2 Diabetes and High Cardiovascular and Renal Risk: The Carmelina Randomized Clinical Trial. JAMA (2019) 321(1):69–79. doi: 10.1001/jama.2018.18269
8. Marso SP, Daniels GH, Brown-Frandsen K, Kristensen P, Mann JF, Nauck MA, et al. Liraglutide and Cardiovascular Outcomes in Type 2 Diabetes. N Engl J Med (2016) 375(4):311–22. doi: 10.1056/NEJMoa1603827
9. Hernandez AF, Green JB, Janmohamed S, D'Agostino RB Sr, Granger CB, Jones NP, et al. Albiglutide and Cardiovascular Outcomes in Patients With Type 2 Diabetes and Cardiovascular Disease (Harmony Outcomes): A Double-Blind, Randomised Placebo-Controlled Trial. Lancet (2018) 392(10157):1519–29. doi: 10.1016/S0140-6736(18)32261-X
10. Gerstein HC, Colhoun HM, Dagenais GR, Diaz R, Lakshmanan M, Pais P, et al. Dulaglutide and Cardiovascular Outcomes in Type 2 Diabetes (REWIND): A Double-Blind, Randomised Placebo-Controlled Trial. Lancet (2019) 394(10193):121–30. doi: 10.1016/S0140-6736(19)31149-3
11. Marso SP, Bain SC, Consoli A, Eliaschewitz FG, Jódar E, Leiter LA, et al. Semaglutide and Cardiovascular Outcomes in Patients With Type 2 Diabetes. N Engl J Med (2016) 375(19):1834–44. doi: 10.1056/NEJMoa1607141
12. Bethel MA, Patel RA, Merrill P, Lokhnygina Y, Buse JB, Mentz RJ, et al. Cardiovascular Outcomes With Glucagon-Like Peptide-1 Receptor Agonists in Patients With Type 2 Diabetes: A Meta-Analysis. Lancet Diabetes Endocrinol (2018) 6(2):105–13. doi: 10.1016/S2213-8587(17)30412-6
13. Pfeffer MA, Claggett B, Diaz R, Dickstein K, Gerstein HC, Køber LV, et al. Lixisenatide in Patients With Type 2 Diabetes and Acute Coronary Syndrome. N Engl J Med (2015) 373(23):2247–57. doi: 10.1056/NEJMoa1509225
14. Husain M, Birkenfeld AL, Donsmark M, Dungan K, Eliaschewitz FG, Franco DR, et al. Oral Semaglutide and Cardiovascular Outcomes in Patients With Type 2 Diabetes. N Engl J Med (2019) 381(9):841–51. doi: 10.1056/NEJMoa1901118
15. Holman RR, Bethel MA, Mentz RJ, Thompson VP, Lokhnygina Y, Buse JB, et al. Effects of Once-Weekly Exenatide on Cardiovascular Outcomes in Type 2 Diabetes. N Engl J Med (2017) 377(13):1228–39. doi: 10.1056/NEJMoa1612917
16. Giugliano D, Maiorino MI, Bellastella G, Chiodini P, Esposito K. Glycemic Control, Preexisting Cardiovascular Disease, and Risk of Major Cardiovascular Events in Patients With Type 2 Diabetes Mellitus: Systematic Review With Meta-Analysis of Cardiovascular Outcome Trials and Intensive Glucose Control Trials. J Am Heart Assoc (2019) 8(12):e012356. doi: 10.1161/JAHA.119.012356
17. Nauck MA, Meier JJ, Cavender MA, Abd El Aziz M, Drucker DJ. Cardiovascular Actions and Clinical Outcomes With Glucagon-Like Peptide-1 Receptor Agonists and Dipeptidyl Peptidase-4 Inhibitors. Circulation (2017) 136(9):849–70. doi: 10.1161/CIRCULATIONAHA.117.028136
18. Caruso I, Cignarelli A, Giorgino F. Heterogeneity and Similarities in GLP-1 Receptor Agonist Cardiovascular Outcomes Trials. Trends Endocrinol Metab (2019) 30(9):578–89. doi: 10.1016/j.tem.2019.07.004
19. Jones B, Buenaventura T, Kanda N, Chabosseau P, Owen BM, Scott R, et al. Targeting GLP-1 Receptor Trafficking to Improve Agonist Efficacy. Nat Commun (2018) 9(1):1602. doi: 10.1038/s41467-018-03941-2
20. Christopher R, Covington P, Davenport M, Fleck P, Mekki QA, Wann ER, et al. Pharmacokinetics, Pharmacodynamics, and Tolerability of Single Increasing Doses of the Dipeptidyl Peptidase-4 Inhibitor Alogliptin in Healthy Male Subjects. Clin Ther (2008) 30(3):513–27. doi: 10.1016/j.clinthera.2008.03.005
21. Henry RR, Smith SR, Schwartz SL, Mudaliar SR, Deacon CF, Holst JJ, et al. Effects of Saxagliptin on Beta-Cell Stimulation and Insulin Secretion in Patients With Type 2 Diabetes. Diabetes Obes Metab (2011) 13(9):850–8. doi: 10.1111/j.1463-1326.2011.01417.x
22. Murai K, Katsuno T, Miyagawa J, Matsuo T, Ochi F, Tokuda M, et al. Very Short-Term Effects of the Dipeptidyl Peptidase-4 Inhibitor Sitagliptin on the Secretion of Insulin, Glucagon, and Incretin Hormones in Japanese Patients With Type 2 Diabetes Mellitus: Analysis of Meal Tolerance Test Data. Drugs R D (2014) 14(4):301–8. doi: 10.1007/s40268-014-0072-6
23. Nakamura Y, Tsuji M, Hasegawa H, Kimura K, Fujita K, Inoue M, et al. Anti-Inflammatory Effects of Linagliptin in Hemodialysis Patients With Diabetes. Hemodial Int Int Symp Home Hemodial (2014) 18(2):433–42. doi: 10.1111/hdi.12127
24. Barnett AH. Lixisenatide: Evidence for Its Potential Use in the Treatment of Type 2 Diabetes. Core Evid (2011) 6:67–79. doi: 10.2147/CE.S15525
25. Murphy CE. Review of the Safety and Efficacy of Exenatide Once Weekly for the Treatment of Type 2 Diabetes Mellitus. Ann Pharmacot (2012) 46(6):812–21. doi: 10.1345/aph.1Q722
26. Ingwersen SH, Petri KC, Tandon N, Yoon KH, Chen L, Vora J, et al. Liraglutide Pharmacokinetics and Dose-Exposure Response in Asian Subjects With Type 2 Diabetes From China, India and South Korea. Diabetes Res Clin Pract (2015) 108(1):113–9. doi: 10.1016/j.diabres.2015.01.001
27. Seino Y, Nakajima H, Miyahara H, Kurita T, Bush MA, Yang F, et al. Safety, Tolerability, Pharmacokinetics and Pharmacodynamics of Albiglutide, a Long-Acting GLP-1-Receptor Agonist, in Japanese Subjects With Type 2 Diabetes Mellitus. Curr Med Res Opin (2009) 25(12):3049–57. doi: 10.1185/03007990903372999
28. Carlsson Petri KC, Ingwersen SH, Flint A, Zacho J, Overgaard RV. Semaglutide s.c. Once-Weekly in Type 2 Diabetes: A Population Pharmacokinetic Analysis. Diabetes Ther (2018) 9(4):1533–47. doi: 10.1007/s13300-018-0458-5
29. Geiser JS, Heathman MA, Cui X, Martin J, Loghin C, Chien JY, et al. Clinical Pharmacokinetics of Dulaglutide in Patients With Type 2 Diabetes: Analyses of Data From Clinical Trials. Clin Pharmacokinet (2016) 55(5):625–34. doi: 10.1007/s40262-015-0338-3
30. Food and Drugs Administration. Drug RYBELSUS: Drug Details: Label Information (2019). Available at: https://www.accessdata.fda.gov/drugsatfda_docs/label/2019/213051s000lbl.pdf (Accessed 1 Aug 2020).
31. Alsalim W, Omar B, Pacini G, Bizzotto R, Mari A, Ahrén B. Incretin and Islet Hormone Responses to Meals of Increasing Size in Healthy Subjects. J Clin Endocrinol Metab (2015) 100(2):561–8. doi: 10.1210/jc.2014-2865
32. Herrmann C, Göke R, Richter G, Fehmann HC, Arnold R, Göke B, et al. Glucagon-Like Peptide-1 and Glucose-Dependent Insulin-Releasing Polypeptide Plasma Levels in Response to Nutrients. Digestion (1995) 56(2):117–26. doi: 10.1159/000201231
33. Færch K, Torekov SS, Vistisen D, Johansen NB, Witte DR, Jonsson A, et al. GLP-1 Response to Oral Glucose Is Reduced in Prediabetes, Screen-Detected Type 2 Diabetes, and Obesity and Influenced by Sex: The ADDITION-PRO Study. Diabetes (2015) 64(7):2513–25. doi: 10.2337/db14-1751
34. Drucker DJ, Nauck MA. The Incretin System: Glucagon-Like Peptide-1 Receptor Agonists and Dipeptidyl Peptidase-4 Inhibitors in Type 2 Diabetes. Lancet (2006) 368(9548):1696–705. doi: 10.1016/S0140-6736(06)69705-5
35. Lundgren JR, Færch K, Witte DR, Jonsson AE, Pedersen O, Hansen T, et al. Greater Glucagon-Like Peptide-1 Responses to Oral Glucose are Associated With Lower Central and Peripheral Blood Pressures. Cardiovasc Diabetol (2019) 18(1):130. doi: 10.1186/s12933-019-0937-7
36. Yanagawa T. Strong Association Between Weight Reduction and Suppression of Cardiovascular Events in Recent Clinical Trials of DPP4 Inhibitors, GLP-1 Receptor Agonists, and SGLT2 Inhibitors. J Clin Med Res (2018) 10(10):796–7. doi: 10.14740/jocmr3587w
37. Nauck MA, Meier JJ. MANAGEMENT OF ENDOCRINE DISEASE: Are All GLP-1 Agonists Equal in the Treatment of Type 2 Diabetes? Eur J Endocrinol (2019) 181(6):R211–34. doi: 10.1530/EJE-19-0566
Keywords: type 2 diabetes mellitus, cardiovascular outcome trials, glucagon-like peptide-1 receptor agonist, dipeptidyl peptidase-4 inhibitor, incretin
Citation: Pan Q, Yuan M and Guo L (2022) Exposure–Response Analysis of Cardiovascular Outcome Trials With Incretin-Based Therapies. Front. Endocrinol. 13:893971. doi: 10.3389/fendo.2022.893971
Received: 11 March 2022; Accepted: 22 April 2022;
Published: 26 May 2022.
Edited by:
Si Jin, Huazhong University of Science and Technology, ChinaReviewed by:
Zhelong Liu, Huazhong University of Science and Technology, ChinaGuangda Xiang, General Hospital of Central Theater Command, China
Copyright © 2022 Pan, Yuan and Guo. This is an open-access article distributed under the terms of the Creative Commons Attribution License (CC BY). The use, distribution or reproduction in other forums is permitted, provided the original author(s) and the copyright owner(s) are credited and that the original publication in this journal is cited, in accordance with accepted academic practice. No use, distribution or reproduction is permitted which does not comply with these terms.
*Correspondence: Lixin Guo, Z2x4MTIxOEAxNjMuY29t
†These authors have contributed equally to this work