- 1Department of Internal Medicine and Endocrinology, Medical University of Warsaw, Warsaw, Poland
- 2Department of Nephrology, Dialysis and Internal Medicine, Medical University of Warsaw, Warsaw, Poland
Background: Therapy with intravenous glucocorticoids (GCs) is associated with various side effects, however, the impact on bone remains elusive. Trabecular bone score (TBS) is a diagnostic tool providing information on bone microarchitecture based on images obtained from dual-energy X-ray absorptiometry. We investigated the influence of the intravenous methylprednisolone (IVMP) pulse administration on TBS in patients with moderate-to-severe Graves’ orbitopathy (GO).
Methods: Fifteen patients with GO were treated with 12 IVMP pulses (6x0.5g, 6x0.25 g on a weekly schedule). They received supplementation with 2000 IU of vitamin D and 1.0 g of calcium throughout the study period. TBS was assessed at baseline and after last IVMP pulse. To determine the difference between values at baseline and after treatment the least significant change (LSC) methodology was used. We compared pre- and posttreatment mean TBS values.
Results: We found a significant decrease of TBS in 5 out of 15 (33%) patients. Mean TBS value decreased becoming 2.4% lower than at baseline (p<0.05).
Conclusions: IVMP pulse therapy exerts negative effect on bone microarchitecture in TBS assessment. The analysis of the clinical risk factors for osteoporosis and the evaluation of bone mineral density and TBS should be considered before initiating IVMP therapy.
Introduction
Glucocorticoids (GCs) are highly effective and widely used for treatment of various autoimmune diseases. Therapy with GCs is associated with multiple side effects (1–5), including the negative impact on bone, leading to secondary osteoporosis and an increased risk of fractures (6, 7).
GCs affect bone in several ways: they decrease calcium absorption in the gut (8), reduce calcium reabsorption in the kidney (9), decrease production of sex hormones (10), cause proximal muscle weakness (11). However, the main negative mechanism involves the increase of the osteoclast lifespan, stimulation of the osteocyte and the osteoblast apoptosis, and the decrease of the osteoblastogenesis. These processes lead to enhanced bone resorption, decreased bone formation, and finally reduction of bone quality and structure (12, 13).
GCs have probably a greater impact on bone microarchitecture rather than on bone mass (14–16). Among patients treated with oral GCs individual fracture risk is increased independently of bone mineral density (BMD) as the fractures often occur with non-osteoporotic T-score values (10, 17). The effects of GCs on bone quality are not fully expressed by BMD measurement (14, 16, 18). Therefore, to identify GCs-treated patients with high risk of fracture, the analysis of other factors contributing to bone strength and resistance to fracture is needed.
The trabecular bone score (TBS) is a non-invasive technique that performs novel gray-level texture measurements on lumbar spine DXA images, and thereby enables estimating trabecular microstructure and assessment of bone quality (19). Low TBS values indicate weak bone, prone to fractures with fewer poorly connected trabeculae, whereas elevated TBS values reflect denser bone with stronger bone microarchitecture. As shown in previous studies, TBS might be a good indicator of bone health in patients treated with GCs, as it seems to be more sensitive than BMD in detecting the GCs-induced fractures (14, 20–22).
Although the negative impact of oral GCs on bone microarchitecture was demonstrated in several studies (18, 20–22), the influence of intravenous GCs remains elusive. A few studies suggest no negative impact of intravenous methylprednisolone (IVMP) on BMD (23–26). Others demonstrate loss of BMD (27, 28). There is only one pilot study reporting no change of TBS after IVMP pulse therapy (29).
GCs administered intravenously are commonly used in a variety of autoimmune diseases. IVMP therapy in weekly infusions is still the first-line treatment according to the latest European Group on Graves’ Orbitopathy (EUGOGO) recommendations in patients with moderate-to-severe and active Graves’ orbitopathy (GO) (30–32). Throughout the therapy bone protection is recommended (32). The aim of our study was to investigate early changes of TBS after IVMP therapy with cumulative dose of 4.5 g in euthyroid patients with active, moderate-to-severe GO.
Materials and Methods
Patients
Consecutive patients with active, moderate-to-severe GO were enrolled to participate in the study between 2018 to 2021. GO was diagnosed and treated according to the EUGOGO recommendations (33). The study was conducted at the Department of Internal Medicine and Endocrinology, Medical University of Warsaw. Exclusion criteria were: age < 20 years; BMI < 17 kg/m2 or > 37 kg/m2; treatment with oral or intravenous GCs within the last 6 months; any other treatment known to significantly alter bone metabolism (i.e. bisphosphonates or other drugs with anti-fracture effects, heparin, vitamin-K antagonists, proton pump inhibitors, selective serotonin reuptake inhibitors, benzodiazepines, antiepileptic, antipsychotic drugs); clinical diagnosis of osteoporosis based on the presence of low-energy fractures, or BMD measurements (DXA T score below -2.5 SD), as defined by the World Health Organization (WHO) (34). We included to the study 15 patients: 14 patients diagnosed with Graves’ disease and 1 patient with Hashimoto thyroiditis. Among patients with Graves’ disease, 11 patients were treated with antithyroid drugs (alone or according to a “block and replace” schedule) and 3 individuals who were at least 6 months after the last radical treatment (thyroidectomy and/or radioiodine therapy) received levothyroxine. One patient had Hashimoto thyroiditis treated with levothyroxine. All patients remained clinically euthyroid, with free triiodothyronine (fT3) and free thyroxine (fT4) levels within the reference range at baseline, in the last month prior to the study as well as throughout the time of the IVMP therapy. All patients were treated with IVMP pulses in a 12-week protocol (six infusions of 0.5 g, followed by six infusions of 0.25 g; cumulative dose 4.5 g) according to the current EUGOGO recommendations (33). Supplementation with 2000 IU of vitamin D and 1.0 g of calcium daily was routinely initiated in all patients at the beginning of IVMP therapy and continued throughout the study. The 25-hydroxyvitamin D [25(OH)D] concentrations below 20 ng/mL were described as deficient, concentrations of 20-30 ng/mL as suboptimal, and concentrations higher than 30 ng/mL as optimal vitamin D status, based on the guidelines for vitamin D supplementation and treatment of deficits approved in Central Europe (35). The baseline characteristics of the study group are presented in Table 1. All procedures were performed in accordance with the 1964 Helsinki declaration. Informed and written consent was obtained from all individual participants included in the study. The study was approved by the Local Bioethics Committee (KB/197/2018).
BMD and TBS Evaluation
Areal BMD (grams per cm2) of the lumbar spine (L1–L4) and the femoral neck were assessed using dual-energy X-ray absorptiometry (DXA) at baseline (within 2 weeks before IVMP therapy) and within one month after the 12th IVMP pulse. DXA scans were performed by one technician using the Hologic Discovery A Densitometer. BMD measurements were calculated, and Z-scores and T-scores were subsequently analyzed by the same physician. Osteoporosis and osteopenia were diagnosed in individuals with a T-score of the lumbar spine and/or the femoral neck ≤−2.5 standard deviation (SD) and between <−1.0 and >−2.5 SD, respectively (34).
TBS was calculated using iNsight® Software (version 3.0, Med-Imaps, Pessac, France) on the DXA lumbar spine (L1-L4) images. The TBS absolute values for the sum of vertebrae L1-L4 were reported. The absolute TBS values <1.230 were considered as the degraded microarchitecture, TBS values between ≥ 1.23 and <1.31 indicated partially disturbed bone microarchitecture, whereas TBS values ≥ 1.31 were assessed as normal (36).
The least significant change (LSC) methodology was used to evaluate the differences in BMD expressed in g/cm2 and TBS values before and after the IVMP treatment. LSC values for BMD and TBS were calculated for the DXA device in the Medical University of Warsaw’s densitometry unit and were estimated to be 3% for the lumbar spine BMD, 5.4% for the femoral neck BMD and 4.6% for TBS. An increase or decrease of BMD or TBS equal to or exceeding the LSC was considered significant.
Laboratory Evaluation
Thyroid-stimulating hormone (TSH), fT3, fT4, thyrotropin receptor antibodies (TRAb) and 25(OH)D levels were assessed at baseline and after the last IVMP pulse. TSH, fT3, fT4, TRAb and 25(OH)D were examined using an electrochemiluminescence immunoassay on Cobas 8000 Analyzer (Roche Diagnostics, Mannheim, Germany). The reference ranges for TSH, fT3, fT4 and TRAb were: 0.27–4.2 μIU/mL, 3.1–6.8 pmol/L, 12.0–22.0 pmol/L and <1.8 IU/mL, respectively.
Statistical Analysis
All analyses were performed using SPSS statistical software version 22.0 (IBM SPPS Statistics, New York, US). Continuous variables are expressed as means ± standard deviation (SD), while categorical variables are expressed as numbers (n) and percentages (%). The Shapiro-Wilk test was used to confirm or reject the normal distribution of each continuous variable. Comparisons between continuous data were performed using paired t-test (for parameters with normal distribution) or Wilcoxon rank sum test (for parameters with distribution deviations). Categorical data were analyzed using Fisher exact test. Comparisons between continuous data were performed with the Mann – Whitney U test. Pearson correlation test was performed to investigate correlations. Statistical significance was established for results with p<0.05.
Results
Baseline Data
At baseline, 3 out of 15 patients (20%) had degraded, and 2 out of 15 patients (13%) had partially disturbed microarchitecture. We found osteopenia in 4 out of 15 patients (27%): in 2 patients only in the lumbar spine BMD, in 1 patient only in the femoral neck BMD and in another patient in both measurement sites. The baseline TBS values correlated negatively with BMI (r=-0.72, p=0.003). We observed vitamin D deficiency in 2 patients.
Effect of IVMP Therapy on TBS and BMD
According to the LSC criteria, we found the following changes in TBS and BMD after 12 weeks of IVMP therapy (Figure 1):
- decrease in TBS in 5 out of 15 patients (33%)
- decrease of BMD in 2 out of 15 patients (13%; 1 in the lumbar spine BMD, 1 in the femoral neck BMD)
- increase of BMD in 7 out of 15 patients (47%; all in the lumbar spine BMD)
- no increase of the femoral neck BMD
- no increase of TBS.
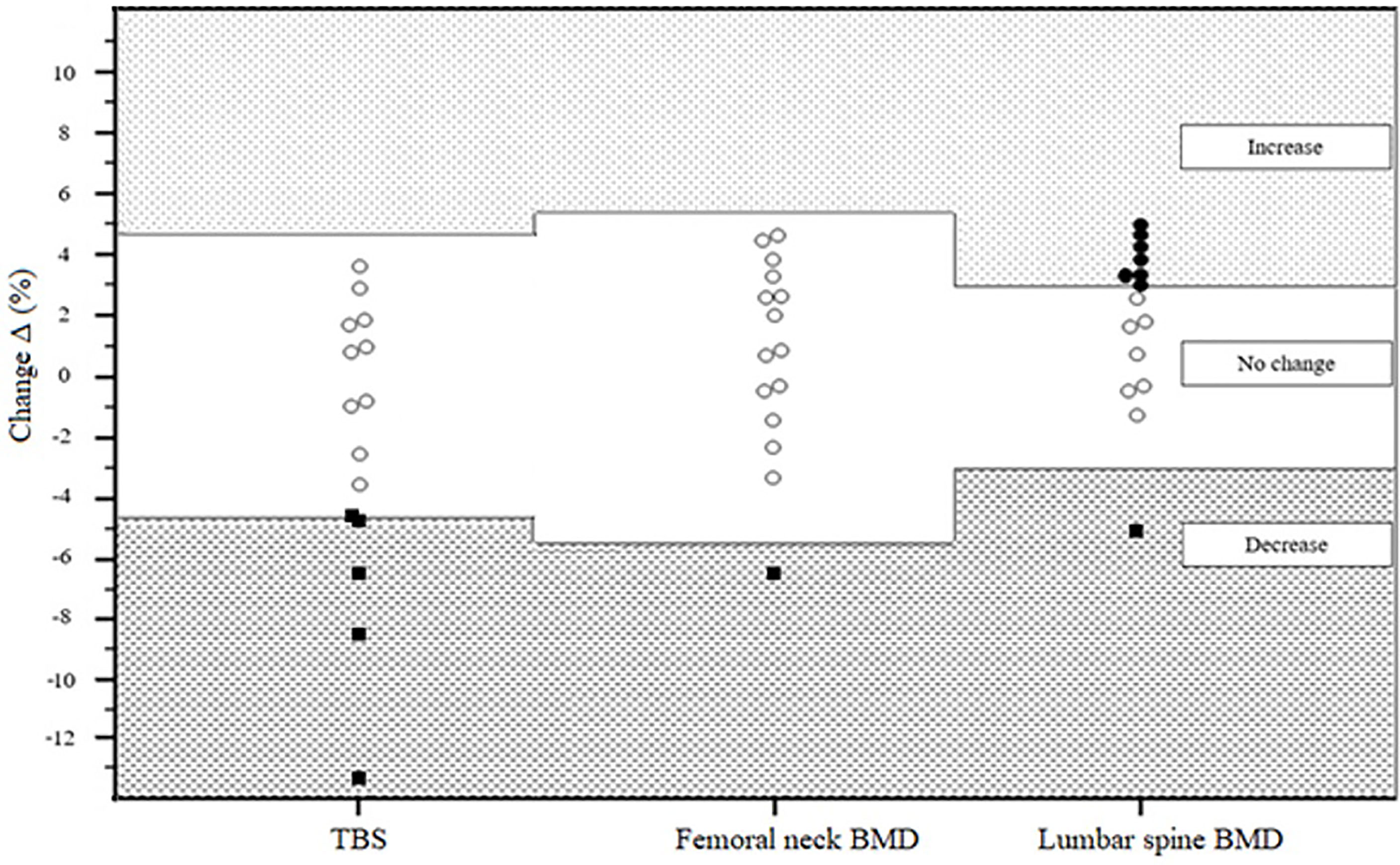
Figure 1 Percentage of TBS and BMD changes according to the LSC criteria in 15 patients after IVMP therapy. TBS trabecular bone score BMD bone mineral density LSC least significant change IVMP intravenous methylprednisolone. Bullets and squares represent individual percentage of BMD and TBS changes (black squares represent a decrease in TBS or BMD – equal to or exceeding LSC calculated to be 4.6% change for TBS, 5.4% change for femoral neck and 3% change for lumbar spine; black bullets represent an increase in BMD equal to or exceeding LSC; white bullets represent no change in TBS or BMD). BMD, bone mineral density; IVMP, intravenous methylprednisolone therapy; LSC, least significant change; TBS, trabecular bone score.
Mean TBS value decreased becoming 2.4% lower than at baseline (p=0.04). Mean lumbar spine BMD increased becoming 1.6% higher after IVMP therapy than at baseline (p=0.047). There was no significant change in mean post-treatment femoral neck BMD as compared to the baseline. (Figure 2, Table 2).
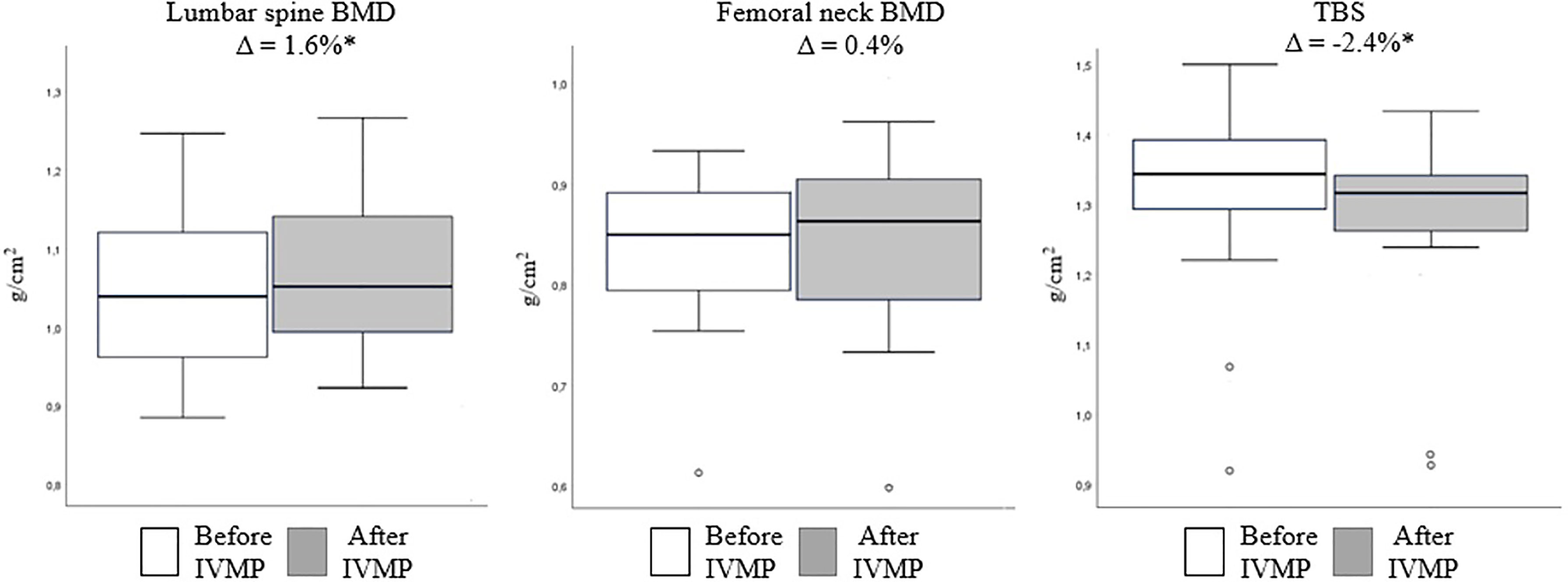
Figure 2 Mean bone mineral density and trabecular bone score values before (white) and after (gray) intravenous methylprednisolone (IVMP) therapy. Values shown above are Δ, calculated as 100 x (after IVMP value – before IVMP value)/before IVMP value. Data are expressed as mean ± SD. *p<0.05 vs. baseline. BMD, bone mineral density; TBS, trabecular bone score; IVMP, intravenous methylprednisolone therapy; SD, standard deviation.

Table 2 Comparison of bone mineral density and trabecular bone score between baseline and after intravenous methylprednisolone therapy (n = 15).
The correlations between the baseline TBS values and the changes in TBS with selected parameters are presented in Table 3.
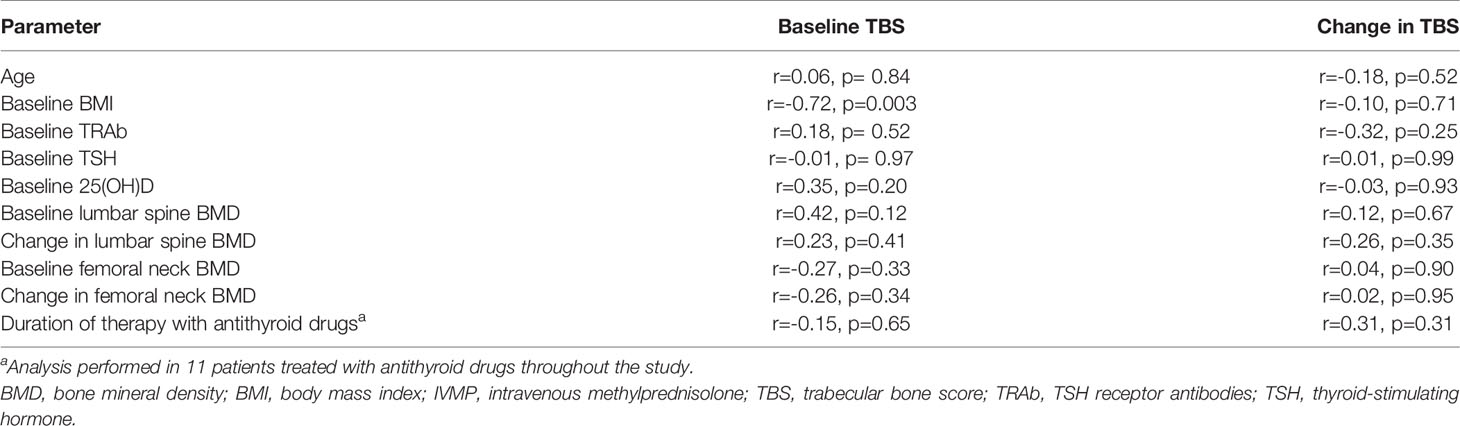
Table 3 Correlations between the baseline trabecular bone score (TBS) and change in TBS with selected parameters.
There were no significant differences between the groups with decreased TBS (drop in TBS equal to or exceeding the LSC) vs. no change in TBS after IVMP treatment as far as the selected characteristics were considered (Table 4).
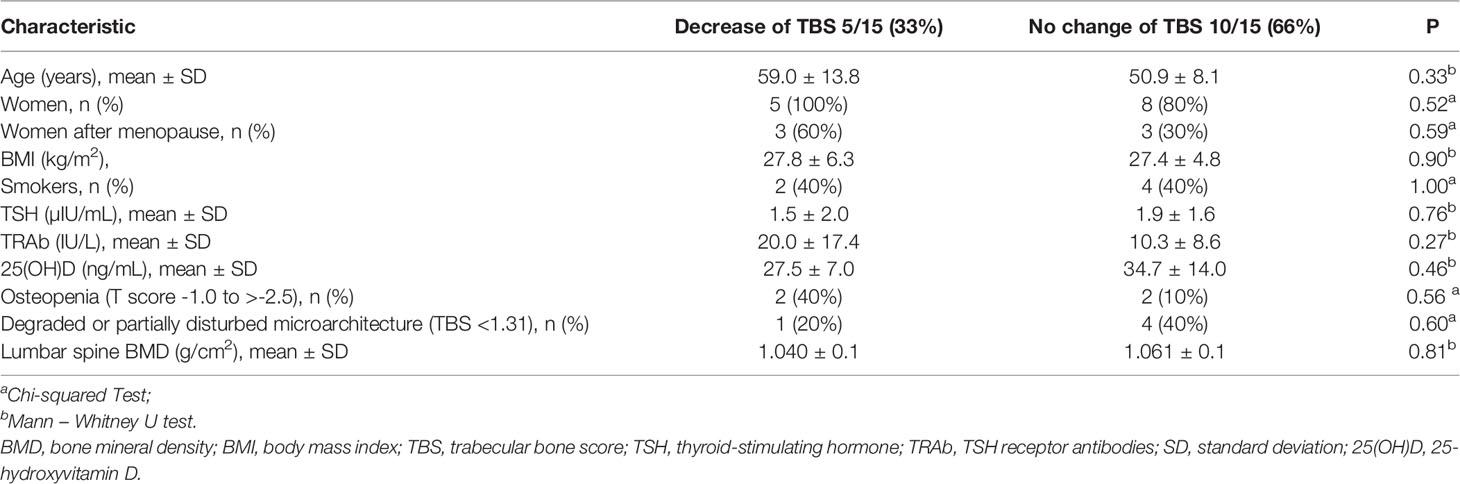
Table 4 Comparison of selected baseline characteristics of patients with and without decrease of trabecular bone score (TBS) (decrease in TBS equal to or exceeding the least significant change) after intravenous methylprednisolone therapy.
In Table 1 (of the Supplementary Material) we have included the results of BMD and TBS of our study group before and after treatment with IVMP pulses.
A decrease of TBS equal to or exceeding LSC value occurred in 2 out of 2 women and in none out of 2 men with osteopenia before IVMP therapy. In one woman with degraded TBS a decrease exceeding LSC value occurred. There was no correlation between the changes in TBS and the baseline TBS values (r =-0.03, p=0.91). However, no correlation was found between the changes of TBS and the baseline lumbar spine BMD, or the femoral neck BMD. Details are presented in Table 3.
There was no significant difference in serum 25(OH)D concentration. No correlation was found between changes in TBS and baseline vitamin D status.
Discussion
The presented study revealed a decrease of the mean TBS value in patients with GO treated with IVMP. The reduction of TBS value equal to or exceeding the LSC occurred in 33% of the patients. In contrast, we observed the overall increase of the mean BMD in the lumbar spine as well as the increase in the lumbar spine BMD exceeding the LSC value in almost half of the study group.
This is the first study showing the negative effect of the intravenous GCs on bone microarchitecture. The current results differ from research performed by Censi et al. involving GO patients treated with IVMP pulse therapy, in which no change in neither of TBS nor BMD was found (29). The possible explanations for the divergent results include different cumulative doses of IVMP (4.5 g of IVMP in the current research vs. 1.5-5.25 g of IVMP in Censi et al. study), different timing of follow-up and no analysis according to the LSC criteria in Censi et al. research. The results of our research stay in agreement with recent studies involving patients treated with oral GCs that demonstrate the decline of TBS while BMD remains unchanged (20–22). The trabecular bone microarchitecture seems to be more affected than BMD not only in patients treated with oral but with intravenous GCs as well. The findings of our study bring evidence that the adverse effects of IVMP on bone are not fully captured with BMD evaluation and that TBS adds value to the BMD assessment.
Based on previous studies, the decrease in bone quality plays a significant role in the rapid increase in fracture risk occurring in GCs-treated patients. Fractures may be better predicted when TBS is used in addition to BMD (37–40). It highlights the clinical need for methods that can identify GCs-treated individuals particularly vulnerable to steroid-induced deterioration of bone quality, as those are at increased risk of fractures. The results of current study indicate that the decrease in TBS despite the increase of BMD might be an indicator that the intravenous GCs are not so safe in terms of bone safety. Further studies with a larger study group should include vertebral and non-vertebral fracture assessment during therapy as well as in the follow-up period.
Some of the clinical risk factors need to be taken into consideration when assessing the influence of GCs on bone microarchitecture. We found a negative correlation of baseline TBS with BMI. This stays in agreement with other reports suggesting that lower TBS values are present more frequently in patients with higher BMI (41). However, we noticed no correlation between change in TBS and BMI. We found no correlations between baseline TBS, or change in TBS and baseline levels of TSH, fT4, fT3, TRAb or duration of treatment with antithyroid drugs. There were no differences in baseline TSH levels between subjects with a decrease versus those lacking any change in TBS.
Another issue that should be considered is that Graves’ disease itself has been demonstrated to have a strong correlation with decreased TBS due to the hyperthyroidism that increases bone resorption (42). Bone loss is enhanced because of the thyrotoxicosis as well as the excessive release of inflammatory cytokines (43). In the present study, all the patients had documented fT4 and fT3 within the reference range for at least one month before the first IVMP infusion as well as during the whole therapy. During the observation time the majority of patients were treated with antithyroid therapy, which had been initiated without delay in the past. Previous studies concerning the reversibility of bone loss after the initiation of antithyroid therapy have shown that bone quantity measured by BMD as well as bone quality measured using TBS improve simultaneously after thyroid function normalization (44, 45). The increase in BMD after IVMP therapy observed in current as well as in our previous study (23) were greater for sites rich in trabecular bone (lumbar spine) than with cortical bone (femoral neck), as trabecular bone is more metabolically active, and changes occur earlier than in cortical bone (46). Taking everything into consideration, we cannot exclude that the BMD changes during the therapy with intravenous GCs were secondary to the ongoing restoration of BMD after the stabilization of thyroid function as well as the reduction of the inflammatory state. However, the decrease of TBS occurring simultaneously with the increase of lumbar spine BMD indicates that in the course of the IVMP therapy the restored bone density might have been unevenly distributed within the trabecular bone. Further study and analysis are needed in order to confirm our observations as well as to identify patients particularly vulnerable to bone quality deterioration during IVMP therapy.
There are studies indicating that the supplementation with vitamin D might be effective in preventing of bone loss in GCs-treated patients (47). In our study the mean vitamin D status at baseline was optimal, the supplementation with 1.0 g of calcium and 2000 IU of vitamin D was continued through the whole study period. We did not observe any correlations between changes in TBS and baseline vitamin D status. There was also no difference between groups with and without decrease of TBS equal to or exceeding LSC as far as baseline 25(OH)D concentration was taken into account. It is noteworthy that despite the optimal vitamin D status at baseline and the sufficient vitamin D prophylaxis, the decrease in TBS could not be prevented.
The biggest limitation of our study is a small sample size including men, pre- and postmenopausal women, different age groups and the reassessment of TBS after a relatively short follow-up period. However, the strength of our study is the therapy of all subjects according to the same protocol. We consider this research as a pilot study that allows us to design larger prospective research with longer follow-up period. More studies with additional measurements of TBS and fracture assessment are needed to determine whether TBS improves with time and if the fractures occur in patients after IVMP therapy. Patients with GO sometimes require the second-line treatment including, among others, the second course of IVMP with a higher cumulative dose (7.5 g) or oral GCs, which may potentially further exacerbate bone microarchitecture. Finally, TBS is an indirect index of bone microstructure state, and the accuracy of TBS measurements might be affected by body composition (48). However, each patient served as his or her own control.
It is noteworthy that according to the latest EUGOGO recommendations (32), in the most severe forms of moderate-to-severe and active GO, a higher cumulative dose of IVMP (7.5 g) is recommended as an alternative first-line treatment. Further studies aimed to assess bone microarchitecture in patients treated with different cumulative doses of IVMP (4.5 g vs. 7.5 g) are needed. The assessment of the impact of IVMP on bone markers would have also a great value as the mechanisms through which IVMP exerts its effects on bone remain not fully recognized.
In conclusion, our study revealed that IVMP pulse therapy with cumulative dose of 4.5 g is associated with loss of bone microarchitecture in TBS assessment with no negative effect on BMD among patients with GO.
Data Availability Statement
The original contributions presented in the study are included in the article/Supplementary Material. Further inquiries can be directed to the corresponding author.
Ethics Statement
The studies involving human participants were reviewed and approved by Local Bioethical Committee of the Medical University of Warsaw. The patients/participants provided their written informed consent to participate in this study.
Author Contributions
JR and PM were responsible for the concept and design of the study. JR and KP collected patient data and prepared the data for analysis. JR, KP, JP, and PM analyzed clinical data. JR was responsible for statistical analysis, tables, and figures. All authors drafted the manuscript. PM supervised all aspects of the study, critically revised the manuscript and approved the final manuscript as submitted. All authors contributed to the article and approved the submitted version.
Conflict of Interest
The authors declare that the research was conducted in the absence of any commercial or financial relationships that could be construed as a potential conflict of interest.
Publisher’s Note
All claims expressed in this article are solely those of the authors and do not necessarily represent those of their affiliated organizations, or those of the publisher, the editors and the reviewers. Any product that may be evaluated in this article, or claim that may be made by its manufacturer, is not guaranteed or endorsed by the publisher.
Supplementary Material
The Supplementary Material for this article can be found online at: https://www.frontiersin.org/articles/10.3389/fendo.2022.893600/full#supplementary-material
References
1. Miśkiewicz P, Kryczka A, Ambroziak U, Rutkowska B, Główczyńska R, Opolski G, et al. Is High Dose Intravenous Methylprednisolone Pulse Therapy in Patients With Graves' Orbitopathy Safe? Endokrynol. Pol (2014) 65:402–13. doi: 10.5603/EP.2014.0056
2. Miśkiewicz P, Milczarek-Banach J, Bednarczuk T, Opolski G, Główczynska R. Blood Pressure Profile and N-Terminal-proBNP Dynamics in Response to Intravenous Methylprednisolone Pulse Therapy of Severe Graves' Orbitopathy. Int J Mol Sci (2018) 19:2918. doi: 10.3390/ijms19102918
3. Pelewicz K, Miśkiewicz P. Glucocorticoid Withdrawal-An Overview on When and How to Diagnose Adrenal Insufficiency in Clinical Practice. Diagnostics (Basel) (2021) 4:728. doi: 10.3390/diagnostics11040728
4. Pelewicz K, Szewczyk S, Miśkiewicz P. Treatment With Intravenous Methylprednisolone in Patients With Graves' Orbitopathy Significantly Affects Adrenal Function: Assessment of Serum, Salivary Cortisol and Serum Dehydroepiandrosterone Sulfate. J Clin Med (2020) 10:3233. doi: 10.3390/jcm9103233
5. Marcocci C, Watt T, Altea MA, Rasmussen AK, Feldt-Rasmussen U, Orgiazzi J, et al. Fatal and non-Fatal Adverse Events of Glucocorticoid Therapy for Graves' Orbitopathy: A Questionnaire Survey Among Members of the European Thyroid Association. Eur. J Endocrinol (2012) 166:247–53. doi: 10.1530/EJE-11-0779
6. Weinstein RS. Glucocorticoid-Induced Bone Disease. N Engl J Med (2011) 365:62–70. doi: 10.1056/NEJMcp1012926
7. Van Staa TP, Leufkens HG, Cooper C. The Epidemiology of Corticosteroid-Induced Osteoporosis: A Meta-Analysis. Osteoporos Int (2002) 13:777–87. doi: 10.1007/s001980200108
8. Huybers S, Naber TH, Bindels RJ, Hoenderop JG. Prednisolone-Induced Ca2+ Malabsorption Is Caused by Diminished Expression of the Epithelial Ca2+ Channel TRPV6. Am J Physiol Gastrointest Liver Physiol (2007) 1:G92–7. doi: 10.1152/ajpgi.00317.2006
9. Canalis E, Mazziotti G, Giustina A, Bilezikian JP. Glucocorticoid-Induced Osteoporosis: Pathophysiology and Therapy. Osteoporos Int (2007) 10:1319–28. doi: 10.1007/s00198-007-0394-0
10. Van Staa TP. The Pathogenesis, Epidemiology and Management of Glucocorticoid-Induced Osteoporosis. Calcif Tissue Int (2006) 79:129–37. doi: 10.1007/s00223-006-0019-1
11. Lukert BP, Raisz LG. Glucocorticoid-Induced Osteoporosis: Pathogenesis and Management. Ann Intern Med (1990) 5:352–64. doi: 10.7326/0003-4819-112-5-352
12. O'Brien CA, Jia D, Plotkin LI, Bellido T, Powers CC, Stewart SA, et al. Glucocorticoids Act Directly on Osteoblasts and Osteocytes to Induce Their Apoptosis and Reduce Bone Formation and Strength. Endocrinology (2004) 145:1835–41. doi: 10.1210/en.2003-0990
13. Weinstein RS, Jilka RL, Parfitt AM, Manolagas SC. Inhibition of Osteoblastogenesis and Promotion of Apoptosis of Osteoblasts and Osteocytes by Glucocorticoids. Potential Mech Their Deleterious Eff Bone J Clin Invest (1998) 102:274–82. doi: 10.1172/JCI2799
14. Leib ES, Winzenrieth R. Bone Status in Glucocorticoid-Treated Men and Women. Osteoporos Int (2016) 27:39–48. doi: 10.1007/s00198-015-3211-1
15. Dalle Carbonare L, Giannini S. Bone Microarchitecture as an Important Determinant of Bone Strength. J Endocrinol Invest (2004) 27:99–105. doi: 10.1007/BF03350919
16. Rice JC, Cowin SC, Bowman JA. On the Dependence of the Elasticity and Strength of Cancellous Bone on Apparent Density. J Biomech (1988) 21:155–68. doi: 10.1016/0021-9290(88)90008-5
17. Kanis JA, Johansson H, Oden A, Johnell O, de Laet C, Melton LJ III, et al. A Meta-Analysis of Prior Corticosteroid Use and Fracture Risk. J Bone Miner Res (2004) 19:893–9. doi: 10.1359/JBMR.040134
18. Nowakowska-Płaza A, Wroński J, Sudoł-Szopińska I, Głuszko P. Clinical Utility of Trabecular Bone Score (TBS) in Fracture Risk Assessment of Patients With Rheumatic Diseases Treated With Glucocorticoids. Horm Metab Res (2021) 53:499–503. doi: 10.1055/a-1528-7261
19. Harvey NC, Glüer CC, Binkley N, McCloskey EV, Brandi ML, Cooper C, et al. Trabecular Bone Score (TBS) as a New Complementary Approach for Osteoporosis Evaluation in Clinical Practice. Bone (2015) 78:216–24. doi: 10.1016/j.bone.2015.05.016
20. Florez H, Hernández-Rodríguez J, Carrasco JL, Prieto-González S, Muxi A, Filella X, et al. Vertebral Fracture Risk in Glucocorticoid-Induced Osteoporosis: The Role of Hypogonadism and Corticosteroid Boluses. RMD Open (2020) 6:e001355. doi: 10.1136/rmdopen-2020-001355
21. Chuang MH, Chuang TL, Koo M, Wang YF. Trabecular Bone Score Reflects Trabecular Microarchitecture Deterioration and Fragility Fracture in Female Adult Patients Receiving Glucocorticoid Therapy: A Pre-Post Controlled Study. BioMed Res Int (2017) 3:4210217. doi: 10.1155/2017/4210217
22. Paggiosi MA, Peel N, Eastell R. The Impact of Glucocorticoid Therapy on Trabecular Bone Score in Older Women. Osteoporos Int (2015) 26:1773–80. doi: 10.1007/s00198-015-3078-1
23. Rymuza J, Popow M, Żurecka Z, Przedlacki J, Bednarczuk T, Miśkiewicz P. Therapy of Moderate-to-Severe Graves' Orbitopathy With Intravenous Methylprednisolone Pulses Is Not Associated With Loss of Bone Mineral Density. Endocrine (2019) 64:308–15. doi: 10.1007/s12020-018-1823-x
24. Dovio A, Perazzolo L, Osella G, Ventura M, Termine A, Milano E, et al. Immediate Fall of Bone Formation and Transient Increase of Bone Resorption in the Course of High-Dose, Short-Term Glucocorticoid Therapy in Young Patients With Multiple Sclerosis. J Clin Endocrinol Metab (2004) 89:4923–8. doi: 10.1210/jc.2004-0164
25. Frediani B, Falsetti P, Bisogno S, Baldi F, Acciai C, Filippou G, et al. Effects of High Dose Methylprednisolone Pulse Therapy on Bone Mass and Biochemical Markers of Bone Metabolism in Patients With Active Rheumatoid Arthritis: A 12-Month Randomized Prospective Controlled Study. J Rheumatol (2004) 31:1083–7.
26. Schwid SR, Goodman AD, Puzas JE, McDermott MP, Mattson DH. Sporadic Corticosteroid Pulses and Osteoporosis in Multiple Sclerosis. Arch Neurol (1996) 53:753–7. doi: 10.1001/archneur.1996.00550080071014
27. Natsui K, Tanaka K, Suda M, Yasoda A, Sakuma Y, Ozasa A, et al. High-Dose Glucocorticoid Treatment Induces Rapid Loss of Trabecular Bone Mineral Density and Lean Body Mass. Osteoporos Int (2006) 17:105–8. doi: 10.1007/s00198-005-1923-3
28. Haugeberg G, Griffiths B, Sokoll KB, Emery P. Bone Loss in Patients Treated With Pulses of Methylprednisolone Is Not Negligible: A Short Term Prospective Observational Study. Ann Rheum Dis (2004) 63:940–4. doi: 10.1136/ard.2003.011734
29. Censi S, Manso J, Pandolfo G, Franceschet G, Cavedon E, Zhu YH, et al. Bone Turnover Markers, BMD and TBS After Short-Term, High-Dose Glucocorticoid Therapy in Patients With Graves' Orbitopathy: A Small Prospective Pilot Study. J Endocrinol Invest (2019) 42:859–65. doi: 10.1007/s40618-018-0992-z
30. Bartalena L, Krassas GE, Wiersinga W, Marcocci C, Salvi M, Daumerie C, et al. Efficacy and Safety of Three Different Cumulative Doses of Intravenous Methylprednisolone for Moderate to Severe and Active Graves' Orbitopathy. J Clin Endocrinol Metab (2012) 97:4454–63. doi: 10.1210/jc.2012-2389
31. Zang S, Ponto KA, Kahaly GJ. Intravenous Glucocorticoids for Graves' Orbitopathy: Efficacy and Morbidity. J Clin Endocrinol Metab (2011) 96:320–32. doi: 10.1210/jc.2010-1962
32. Bartalena L, Kahaly GJ, Baldeschi L, Dayan CM, Eckstein A, Marcocci C, et al. The 2021 European Group on Graves' Orbitopathy (EUGOGO) Clinical Practice Guidelines for the Medical Management of Graves' Orbitopathy. Eur J Endocrinol (2021) 27:G43–67. doi: 10.1530/EJE-21-0479
33. Bartalena L, Baldeschi L, Boboridis K, Eckstein A, Kahaly GJ, Marcocci C, et al. The 2016 European Thyroid Association/European Group on Graves' Orbitopathy Guidelines for the Management of Graves' Orbitopathy. Eur Thyroid J (2016) 5:9–26. doi: 10.1159/000443828
34. Kanis JA, Melton LJ 3rd, Christiansen C, Johnston CC, Khaltaev N. The Diagnosis of Osteoporosis. J Bone Miner Res (1994) 9:1137–41. doi: 10.1002/jbmr.5650090802
35. Płudowski P, Karczmarewicz E, Bayer M, Carter G, Chlebna-Sokół D, Czech-Kowalska J, et al. Practical Guidelines for the Supplementation of Vitamin D and the Treatment of Deficits in Central Europe - Recommended Vitamin D Intakes in the General Population and Groups at Risk of Vitamin D Deficiency. Endokrynol Pol (2013) 64:319–27. doi: 10.5603/EP.2013.0012
36. McCloskey EV, Odén A, Harvey NC, Leslie WD, Hans D, Johansson H, et al. A Meta-Analysis of Trabecular Bone Score in Fracture Risk Prediction and Its Relationship to FRAX. J Bone Miner Res (2016) 31:940–8. doi: 10.1002/jbmr.2734
37. van Staa TP, Leufkens HGM, Abenhaim, Zhang B, Cooper C. Oral Corticosteroids and Fracture Risk: Relationship to Daily and Cumulative Doses. Rheumatol (Oxford) (2000) 12:1383–9. doi: 10.1093/rheumatology/39.12.1383
38. Florez H, Hernández-Rodríguez J, Muxi A, Carrasco JL, Prieto-González S, Cid MC, et al. Trabecular Bone Score Improves Fracture Risk Assessment in Glucocorticoid-Induced Osteoporosis. Rheumatol (Oxford) (2020) 7:1574–80. doi: 10.1093/rheumatology/kez464
39. Hans D, Goertzen AL, Krieg MA, Leslie WD. Bone Microarchitecture Assessed by TBS Predicts Osteoporotic Fractures Independent of Bone Density: The Manitoba Study. J Bone Miner Res (2011) 11:2762–9. doi: 10.1002/jbmr.499
40. Briot K, Paternotte S, Kolta S, Eastell R, Reid DM, Felsenberg D, et al. Added Value of Trabecular Bone Score to Bone Mineral Density for Prediction of Osteoporotic Fractures in Postmenopausal Women: The OPUS Study. Bone (2013) 1:232–6. doi: 10.1016/j.bone.2013.07.040
41. Torgutalp ŞŞ, Babayeva N, Kara ÖS, Özkan Ö, Dönmez G, Korkusuz F. Trabecular Bone Score of Postmenopausal Women Is Positively Correlated With Bone Mineral Density and Negatively Correlated With Age and Body Mass Index. Menopause (2019) 26:1166–70. doi: 10.1097/GME.0000000000001375
42. Kužma M, Vaňuga P, Binkley N, Ságová I, Pávai D, Blažíček P, et al. High Serum Fractalkine Is Associated With Lower Trabecular Bone Score in Premenopausal Women With Graves’ Disease. Horm Metab Res (2018) 8:609–14. doi: 10.1055/a-0633-2814
43. Rajaii F, McCoy AN, Smith TJ. Cytokines are Both Villains and Potential Therapeutic Targets in Thyroid-Associated Ophthalmopathy: From Bench to Bedside. Expert Rev Ophthalmol (2014) 3:227–34. doi: 10.1586/17469899.2014.917960
44. Ock SY, Chung YS, Choi YJ. Changes in Bone Mineral Density and Trabecular Bone Score in Graves' Disease Patients After Anti-Thyroid Therapy. Osteoporos Sarcopenia (2016) 3:175–9. doi: 10.1016/j.afos.2016.05.004
45. Wakasugi M, Wakao R, Tawata M, Gan N, Inoue M, Koizumi K, et al. Change in Bone Mineral Density in Patients With Hyperthyroidism After Attainment of Euthyroidism by Dual Energy X-Ray Absorptiometry. Thyroid (1994) 2:179–82. doi: 10.1089/thy.1994.4.179
46. Ciarallo A, Barralet J, Tanzer M, Kremer R. An Approach to Compare the Quality of Cancellous Bone From the Femoral Necks of Healthy and Osteoporotic Patients Through Compression Testing and Microcomputed Tomography Imaging. Mcgill J Med (2006) 2:102–7.
47. Rizzoli R, Adachi JD, Cooper C, Dere W, Devogelaer JP, Diez-Perez A, et al. Management of Glucocorticoid-Induced Osteoporosis. Calcif Tissue Int (2012) 91:225–43. doi: 10.1007/s00223-012-9630-5
Keywords: graves’ orbitopathy, graves’ ophthalmopathy, trabecular bone score, EUGOGO, methylprednisolone
Citation: Rymuza J, Pelewicz K, Przedlacki J and Miśkiewicz P (2022) Therapy With Intravenous Methylprednisolone Pulses Is Associated With Loss of Bone Microarchitecture in Trabecular Bone Score -Assessment Among Patients With Moderate-to-Severe Graves’ Orbitopathy: A Pilot Study. Front. Endocrinol. 13:893600. doi: 10.3389/fendo.2022.893600
Received: 10 March 2022; Accepted: 22 June 2022;
Published: 14 July 2022.
Edited by:
Elizabeth Martha Winter, Leiden University Medical Center, NetherlandsReviewed by:
Agostino Gaudio, University of Catania, ItalyNadia Sawicka-Gutaj, Poznan University of Medical Sciences, Poland
Dariusz Kajdaniuk, Medical University of Silesia, Poland
Copyright © 2022 Rymuza, Pelewicz, Przedlacki and Miśkiewicz. This is an open-access article distributed under the terms of the Creative Commons Attribution License (CC BY). The use, distribution or reproduction in other forums is permitted, provided the original author(s) and the copyright owner(s) are credited and that the original publication in this journal is cited, in accordance with accepted academic practice. No use, distribution or reproduction is permitted which does not comply with these terms.
*Correspondence: Piotr Miśkiewicz, piotr.miskiewicz@wum.edu.pl