- 1Postgraduate Program in Environment, Universidade Ceuma, São Luís, Brazil
- 2Postgraduate Program in Physical Therapy, Universidade Federal de São Carlos, São Carlos, Brazil
- 3Postgraduate Program in Adult Health, Universidade Federal do Maranhão, São Luís, Brazil
- 4Department of Physical Therapy, Universidade Ceuma, São Luís, Brazil
- 5Department of Medicine, Universidade Federal do Maranhão, Pinheiro, Brazil
- 6Department of Physical Therapy, Universidade Federal de São Carlos, São Carlos, Brazil
Diabetes mellitus (DM) is a chronic metabolic disease characterized by high blood glucose levels, causing serious damage to the cardiovascular, respiratory, renal and other systems. The prevalence of type 2 diabetes mellitus (T2DM) was 6.28% in 2017, considering all age groups worldwide (prevalence rate of 6,059 cases per 100,000), and its global prevalence is projected to increase to 7,079 cases per 100,000 by 2030. Furthermore, these individuals are often affected by diabetic myopathy, which is the failure to preserve muscle mass and function in the course of DM. This happens in type 1 diabetes mellitus (T1DM) and T2DM. As skeletal muscle plays a key role in locomotion and glucose homeostasis, diabetic myopathy may contribute to additional complications of the disease. In addition, chronic hyperglycemia is associated with lung functional changes seen in patients with DM, such as reduced lung volumes and compliance, inspiratory muscle strength, and lung elastic recoil. Thus, the weakness of the inspiratory muscles, a consequence of diabetic myopathy, can influence exercise tolerance. Thus, moderate strength training in T2DM can contribute to the gain of peripheral muscle strength. Although the literature is robust on the loss of mass and consequent muscle weakness in diabetic myopathy, triggering pathophysiological factors, the impact on functional capacity, as well as the prescription of physical exercise for this condition deserves to be further explored. This review aims to explore the consequences of diabetic myopathy and its implication in rehabilitation from prescription to safety in the practice of physical exercises for these individuals.
Introduction
Muscle as a Key Structure in Diabetes Mellitus: Diabetic Myopathy
Diabetic myopathy is a disease that affects individuals with type 1 (T1DM) or type 2 diabetes mellitus (T2DM). Diabetes mellitus (DM) is associated with reduced mitochondrial function, including decreased mitochondrial number (1), impaired lipid oxidation (2, 3), and excessive production of reactive oxygen species (ROS) (4–6). This scenario of mitochondrial imbalance induces atrophy, transition from oxidative to glycolytic fiber type (7, 8), resulting in skeletal muscle changes, such as muscle weakness, consequent exercise intolerance and decreased quality of life (9, 10).
Glucose uptake in skeletal muscle can occur through several signaling pathways that lead to translocation of the glucose transporter 4 (GLUT4). Thus, there are separate pathways for exercise- and insulin-stimulated glucose uptake. However, the individuals with DM have glucose uptake disorders in peripheral tissues (11).
There are numerous signaling pathways that lead to the intracellular translocation of GLUT4 to the surface of the muscle cell, being glucose-dependent pathways such as insulin receptor substrate-1 (IRS1) and phosphatidylinositol 3-kinase (PI 3-kinase), which then require intracellular insulin signaling to initiate its binding to a specific membrane receptor mentioned above (12). On the other hand, there are insulin-independent signaling pathways, which are activated through muscle contractions, such as adenosine monophosphate-activated protein kinase (AMPK), nitric oxide (NO), bradykinin, AKT, ROS and calcium (13).
In view of the above, it is known that individuals with T2DM have reduced insulin-stimulated glucose uptake in skeletal muscle, since they have insulin resistance. However, exercise-stimulated glucose uptake is maintained during resistance to insulin, as it does not depend on the AKT signaling pathway of the GLUT4 translocation (11).
Impact of DM on Exercise Capacity
Many are the mechanisms that can lead to a decrease in exercise capacity in this population, with different degrees of functional impairment in the most diverse systems that cooperate in the delivery and use of oxygen were observed, because the supply, demand and consumption of this energy substrate are the result of an interaction of multiple physiological functions, such as pulmonary, cardiovascular and musculoskeletal functions (aerobic capacity, force generation and perception of fatigue) (14).
A recent published review brings with it an integrated approach to physiology in light of the limitations observed in this population that culminate in reduced exercise capacity. Based on cardiopulmonary exercise test (CPET) results, the authors elucidate four pathophysiological determinants that may explain exercise limitation, namely: a) cardiogenic (inadequate adjustment of cardiac output); b) skeletal myogenic (reduced ability to generate force and early fatigue); c) vasogenic (insufficient perfusion and oxygenation); d) neurogenic (impaired cardiopulmonary neural control during exercise) (14).
Therapy for most chronic diseases involves physical training to slow or reverse disease progression (15). During physical exercise, hormones such as insulin and glucagon are responsible for controlling glucose absorption, and the balance between insulin and regulatory hormones may vary based on the type, intensity and duration of exercise (16).
In individuals with T1DM, in the face of aerobic exercise, there is a failure in the circulating levels of insulin, limiting the production of glucose by the liver and facilitating an increase in the elimination of glucose in the skeletal muscle. Contrastingly, during anaerobic exercise, an increase in catecholamines and a failure in circulating levels of insulin at the end of vigorous exercise in individuals with T1DM increases glucose production by the liver while limiting glucose elimination from skeletal muscle, as well, due to this imbalance in glucose production and utilization, circulating glucose levels increase and hyperglycemia may occur (16, 17).
The aerobic exercise promotes improvement in glycemic control observed by fasting glucose and glycated hemoglobin (HbA1c) when compared to control groups (18, 19). On the other hand, a systematic review and meta-analysis showed that aerobic training does not alter HbA1c levels in T1DM (20), although there is an improvement in insulin sensitivity and reduced exogenous insulin requirements in this population (21).
Cuff et al. (22), studying women with T2DM, report that the increase in muscle mass achieved by resistance training contributes to glucose uptake without altering the intrinsic ability of the muscle to respond to insulin, while aerobic exercise improves its absorption capacity through greater insulin action, independently of changes in muscle mass. That is, the type of exercise seems to act differently on muscle glucose metabolism. This fact can be explained by studies that showed that resistance exercise can significantly increase the strength of the skeletal muscles and consequently the cross-sectional area of the muscles, resulting in an increase in the number of insulin receptors and an improvement in insulin sensitivity (23, 24).
Furthermore, previous studies have shown that concurrent exercise, that is, aerobic and resistance exercise in the same section, in patients with DM, lead to an improvement in HbA1c as well as an increase in muscle strength (25, 26).
Exercise Improves Mitochondrial Function in Diabetic Myopathy Muscles
Moderate levels of ROS are required for normal muscle strength production; however, excess ROS can lead to muscle fatigue and contractile dysfunction (27). Major endogenous sources of ROS in skeletal muscle include mitochondria, nicotinamide adenine dinucleotide phosphate hydrogen oxidase (NOX) and xanthine oxidase (XO) (28). Under physiological conditions, ROS are released as by-products of cellular respiration by mitochondria. Thus, mitochondria-derived superoxide anion radical can be observed in both resting and exercising muscle (29).
However, the physical training has been used as an important component in improving insulin sensitivity and muscle glucose uptake, capable of favoring the translocation of the GLUT4, in addition to contributing to the increase in muscle mass and, consequently, increase carbohydrate metabolism and prevent the deleterious effects of myopathy (30). Additionally, the regulation and maintenance of the mitochondrial network constitute mechanisms important underlying factors to improve metabolic capacity, which is one of the most important skeletal muscle adaptations, tissue with high expression of mitochondria and strongly dependent on oxidative phosphorylation for energy formation (31, 32).
Although it is known that the mitochondria of individuals with DM, especially T2DM, are morphologically smaller, quantitatively less numerous and with a lower oxidative capacity, physical exercise can contribute to the restoration of the content and functionality of these organelles (33, 34). The mechanism that justifies these benefits is associated with the fact that physical exercise contributes, in a comprehensive way, to the activation of the peroxisome proliferator-activated gamma coactivator (PGC-1α), a transcriptional coactivator responsible for regulating genes involved in energy metabolism and mitochondrial biogenesis, responsive to both acute and chronic physical training as the musculature adapts to new metabolic demands (31, 34, 35).
Nevertheless, oxidative stress biomarkers play a relevant role in the pathogenesis of DM and the complications associated with the disease, in addition to being a major contributor to mitochondrial dysfunction (36, 37). In this context, changes in the oxidant and antioxidant balance are associated with high blood glucose concentrations, leading to the production of ROS and lower antioxidant activity (37). The diabetic condition of hyperglycemia leads to the induction of ROS productivity and this productivity is associated with several cellular sources that produce free radicals, especially those involving normal or abnormal metabolism of cellular substrate, such as: non-enzymatic glycation of proteins, electron transport in mitochondria, glucose oxidation and glycation of antioxidant enzymes. Furthermore, it is known that the ketogenic condition, characterized by high levels of ketone bodies, can contribute to ROS formation via lipid peroxidation. The subsequent increase in these ROS associated with the hyperglycemic diabetic state leads to decreased insulin gene expression and secretion, deteriorates cellular function, increases insulin resistance, and can ultimately lead to apoptosis (27, 38–41).
Different training modalities have been investigated in this population. However, there is a difficulty in recommending a training intervention for patients with DM that is much superior in terms of effectiveness when it comes to improvement in mitochondrial function, oxidative stress and myopathy, although, still, the literature supports the prescription of combined exercises (aerobic of moderate intensity with resistance training) in view of the benefits achieved in glycemic control and insulin sensitivity, which end up presenting positive results in these deficits (42–44).
Aerobic training has been well documented in terms of qualitative and quantitative improvements in mitochondria, contributing to increased sensitivity to insulin and skeletal muscle mitochondrial protein (42–44). However, it is not surprising that the increase in aerobic capacity resulting from this type of training improves mitochondrial quantity and functionality since this type of training is highly dependent on oxidative phosphorylation and this metabolic pathway occurs essentially in the mitochondria.
Other types of physical training, including strength training and high-intensity interval training (HIIT), have also been reported to be able to increase mitochondrial content and functionality in the skeletal muscle of patients with DM. Particularly, HIIT leads to similar metabolic adaptations compared to traditional resistance training when it comes to improving maximal oxygen uptake and increasing mitochondrial content in healthy individuals. In the diabetic population, this exercise modality still lacks investigations, although, a previous study demonstrated that HIIT can be effective not only in improving glucose metabolism but also resulting in an increase in mitochondrial content in patients with T2DM (45).
Exercise Attenuates Oxidative Stress and Increases the Antioxidant Capacity in Muscle Against Diabetic Myopathy
Several interrelated mechanisms may explain the increase in ROS and decrease in antioxidant protection in this population. However, the production of ROS cannot always be understood as a villain, since in adequate concentrations they are involved in important physiological processes. Although not fully understood, glycolysis, inactivation of antioxidant enzymes, disturbance in nitric oxide metabolism and intracellular formation of advanced glycation end products are responsible for increased production of free radicals and consequently imbalance between oxidation-reduction (36, 46).
The regular physical exercise continues to be recommended based on strong evidence, however, such benefits offered by the different exercise modalities depend on the training dose. Several studies have proved that although regular physical training leads to antioxidant protection, strenuous exercise can induce an increase in oxidative stress with the activation of metabolism of lipids, proteins and even genetic material (36, 47, 48).
Although skeletal muscle contraction and physical exercise contribute to the development of a stressful oxidative condition in the intracellular and extracellular environment, capable of contributing to the condition of insulin resistance and glucose intolerance; however, exercise-induced ROS are considered important mediators of glucose metabolism, leading to consider this paradox between harms, advantages and their dual role in glucose homeostasis as challenging (49).
Paradoxically, among the mechanisms capable of increasing insulin sensitivity and glucose uptake during and after exercise, the reactions that characterize oxidation-reduction have gained prominence in recent years due to the ability of redox signaling to be essential for optimal physiological functioning. and improved insulin-stimulated glucose uptake (50, 51). In this context, the benefits achieved by exercise are directly linked to the transient increase in the generation of ROS that promotes, over time, with the practice of regular exercise, adaptation and adequate regulation of antioxidant defenses and reduction of systemic oxidative stress markers that, consequently, will culminate in improvements in insulin regulation and glucose metabolism (49).
In fact, in DM, physical exercise reduces the production of ROS and leads to adaptations in the antioxidant capacity, preventing cell damage (52). But how can exercise mitigate oxidative stress and increase the antioxidant capacity of skeletal muscle? The answer to this question is multifactorial and even depends on the type of exercise performed, considering that the formation of ROS depends on the type, intensity, duration and frequency of exercise, as well as the individual antioxidant potential. In skeletal muscle, the amount of ROS produced during exercise, related to the factors previously mentioned in the different types of training, lead to substantial increases in enzymatic and non-enzymatic antioxidant efficiency, especially superoxide dismutase 1 and 2 (SOD1 and SOD2), which can increase its efficiency from 20 to 110%, and glutathione peroxidase (GPX1), which can increase its effectiveness by 180%, in addition to favoring greater mitochondrial functionality in capturing free radicals (52, 53).
Evaluation and Safety for Exercise Prescription in Diabetic Myopathy
Among the exercise capacity assessment methods, the CPET remains the gold standard for this purpose. The main care for performing CPET in these individuals is the management of hypoglycemia, hyperglycemia and ketosis, and knowledge of the medications used and planning prior to the test are strongly recommended. CPET should not be performed in the presence of ketosis, regardless of glycemic level (54–56).
The aerobic training improves glycemic control in patients with T1DM (57), despite the association between continuous aerobic exercise and hypoglycemia (58). On the other hand, the literature is robust in demonstrating that exercise sessions composed of strength training exercises or HITT reduce the risk of hypoglycemia during and after physical exertion, when compared to aerobic exercises performed in a continuous in insulin-dependent patients (17, 59). In this scenario, a recent consensus on T1DM strongly recommends the practice of anaerobic exercises for these patients, since this type of physical demand is associated with smaller declines in blood glucose or even increases in glucose levels (60). Thus, anaerobic exercises seem to be safe, especially in those who use insulin.
Therefore, recently published literature aiming to provide general considerations and new directions in the management of T1DM suggests that a training program composed of a combination of HIIT and strength exercise can minimize the rapid drop in blood glucose associated with exercise in T1DM. They further speculate that this is due to increased hepatic glucose production stimulated by catecholamine release and an increased transient inhibition of insulin-mediated glucose uptake due to lactate production (61).
Although CPET is the gold standard for assessing exercise capacity and prescribing safe exercise, unfortunately, a minority of centers have such a resource, as it is an expensive test and requires a large number of trained professionals. Given this, some safe and viable assessment tools can be used, mainly in T2DM, such as the six-minute walk test and the step test. As important as aerobic exercise is strength training, which can be based on 1-repetition maximum assessment, as demonstrated by studies with individuals with T1DM and T2DM (62–64).
Not least, the autonomic nervous system, which is significantly impaired in individuals with DM, deserves attention when evaluating these patients. Heart rate variability (HRV) is one of the most used tools to assess autonomic control in both T1DM and T2DM individuals. It can be performed using linear measurements in the time and frequency domains in addition to non-linear measurements. Other autonomic assessment methods include: heart rate response to the Valsalva maneuver, diastolic blood pressure response to isometric exercise, systolic blood pressure response to standing, heart rate response to standing and holter.
Finally, a more targeted assessment for the diagnosis of sensorimotor neuropathy can be achieved through nerve conduction velocity studies (65–67).
Novel Approaches of How to Prevent the Progression of the Disease and Improve Physical Fitness
The benefits of secondary prevention strategies for chronic disease, including T2DM, targeting lifestyle modification and risk factor management have been well established worldwide (68, 69). However, unfortunately, the adherence of these patients considering the lifestyle changes from a more sedentary to an active pattern is still a big challenge (70, 71). Despite the numerous benefits of formal rehabilitation (clinical centers or home-rehabilitation) in improving physical health and quality of life, combined with benefits in glycemic control, it is important to consider that the sessions can be relatively monotonous and unattractive over time. Therefore, other ways of delivering these services must be emphatically promoted by the public and private sector. Therefore, innovative and cost-effective interventions centered on the patients’ preference to enhance adherence in physical training programs are urgently needed.
In this context, programs that include multimodal interventions, consisting of physical exercise, nutritional changes, behavioral therapy and cognitive approaches have shown interesting results in the medium (72) and long term (73, 74). Considering the behavioral changes related to a healthier lifestyle, recent technologies have been incorporated as a way to expand the distribution of new interventional approaches to patients with T2DM. Mobile text messaging interventions can improve glycemic control (75, 76) and improve active behavior (77) of these patients. Technology-assisted can provide self-monitoring, and it was concluded that the technology-assisted self-monitoring approach was beneficial, safe, and feasible to use for positive lifestyle change.
Apps and text messages can help patients in monitoring, managing and expanding their education in disease control. Furthermore, such technologies have proved to be interesting participant engagement strategies, adjusting to the routine of many patients (78, 79). In addition, personalized coaching, goal setting, peer support groups, use of technology as physical activity monitors were proven to increase the level of physical activity for large patient populations (80). Figure 1 summarizes the components of rehabilitation program on the population with T2DM considering the patient preference.
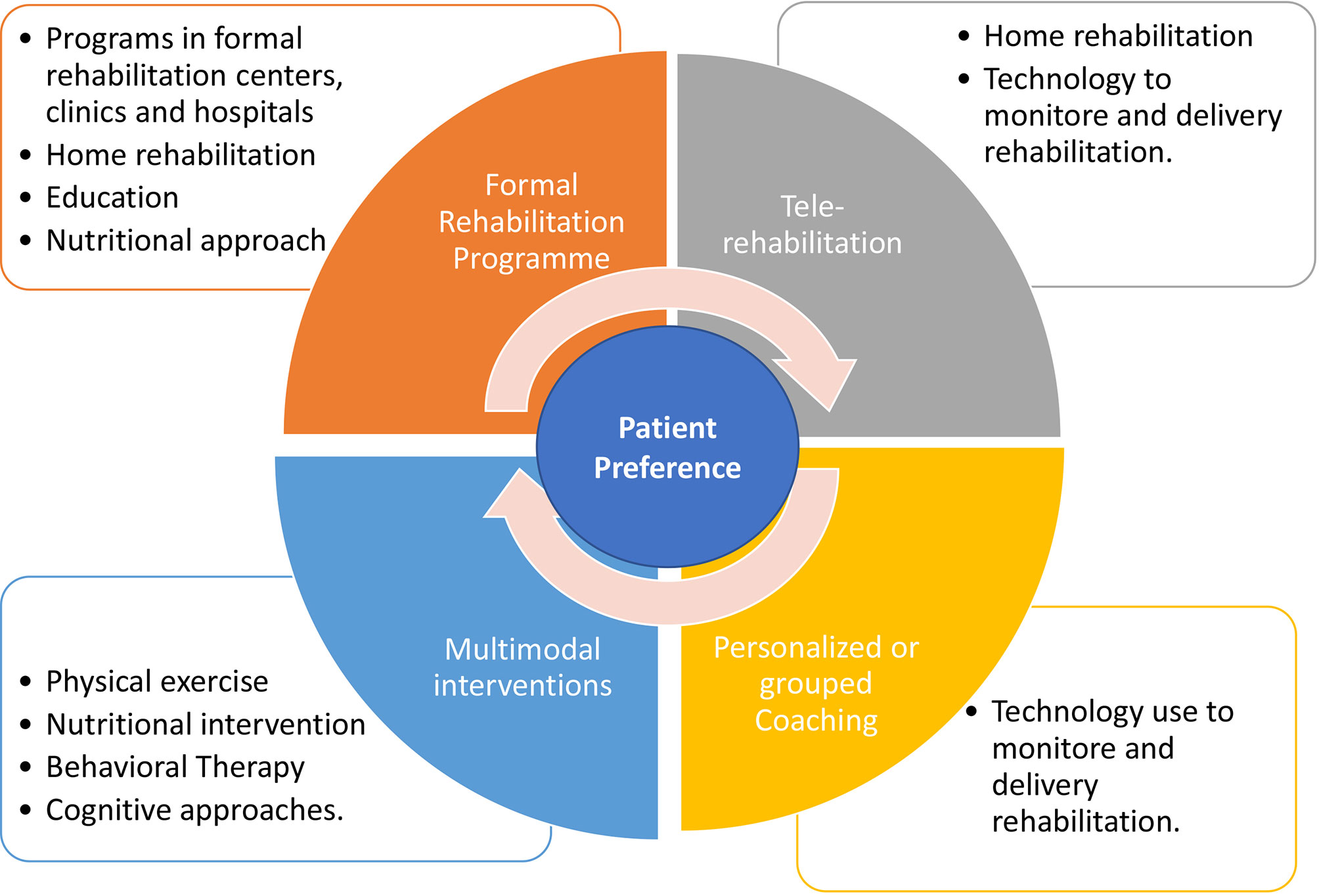
Figure 1 Summary of components of rehabilitation program on the population with T2DM considering the patient preference.
Discussion
Patients with diabetic myopathy are unable to exercise and, consequently, perform their activities of daily living in an even more impaired way. However, the literature remains very heterogeneous and these mechanisms need further clarification in view of the divergences found in the scientific literature that address the theme (14).
In this context, cardiopulmonary rehabilitation involving a combined exercise program can improve antioxidant capacity and attenuate oxidative stress and functional improvements (52), and, attenuating the pathophysiological mechanisms of diabetic myopathy proving to be safe and effective in improving exercise capacity and consequently functionality (25), being a cornerstone of non-pharmacological therapeutic strategies in DM. Finally, rehabilitation programs can improve the prognosis of mortality for all the causes that, in this population, is notably high (14, 67, 81).
There are many studies addressing diabetic neuropathies but few focusing on diabetic myopathy. Based on the limited number of studies to date, it is evident that further studies on the effects of physical exercise in the treatment and progression of diabetic myopathy be carried out with an adequate sample of patients. Besides that, controlled, randomized and double-blind studies, are essential to clarify the main protective effects that physical exercise can develop in the body of individuals who are affected by the disease.
In addition, studies assessing interventions to improve the muscle mass and strength in diabetic patients, before diabetic myopathy development, should be encouraged, as well as studies addressing to investigate the PGC-1 α function in the diabetic myopathy during the rehabilitation’s programs utilizing the exercise.
Author Contributions
DBD, ADSA, ADVF, LFSA, CLG, GCPL, PRB, ASG-A, and ABS designed, wrote and revised this manuscript. All authors contributed to the article and approved the submitted version.
Funding
This work as partially supported by Coordenação de Aperfeiçoamento de Pessoal de Nível Superior (CAPES, finance code 001).
Conflict of Interest
The authors declare that the research was conducted in the absence of any commercial or financial relationships that could be construed as a potential conflict of interest.
Publisher’s Note
All claims expressed in this article are solely those of the authors and do not necessarily represent those of their affiliated organizations, or those of the publisher, the editors and the reviewers. Any product that may be evaluated in this article, or claim that may be made by its manufacturer, is not guaranteed or endorsed by the publisher.
References
1. Pinti MV, Fink GK, Hathaway QA, Durr AJ, Kunovac A, Hollander JM. Mitochondrial Dysfunction in Type 2 Diabetes Mellitus: An Organ-Based Analysis. Am J Physiol Endocrinol Metab (2019) 316:E268–85. doi: 10.1152/AJPENDO.00314.2018
2. Boudina S, Sena S, Theobald H, Sheng X, Wright JJ, Xia XH, et al. Mitochondrial Energetics in the Heart in Obesity-Related Diabetes: Direct Evidence for Increased Uncoupled Respiration and Activation of Uncoupling Proteins. Diabetes (2007) 56:2457–66. doi: 10.2337/DB07-0481
3. Boushel R, Gnaiger E, Schjerling P, Skovbro M, Kraunsøe R, Dela F. Patients With Type 2 Diabetes Have Normal Mitochondrial Function in Skeletal Muscle. Diabetologia (2007) 50:790–6. doi: 10.1007/S00125-007-0594-3
4. Anello M, Lupi R, Spampinato D, Piro S, Masini M, Boggi U, et al. Functional and Morphological Alterations of Mitochondria in Pancreatic Beta Cells From Type 2 Diabetic Patients. Diabetologia (2005) 48:282–9. doi: 10.1007/S00125-004-1627-9
5. Angermueller C, Clark SJ, Lee HJ, Macaulay IC, Teng MJ, Hu TX, et al. Parallel Single-Cell Sequencing Links Transcriptional and Epigenetic Heterogeneity. Nat Methods (2016) 13:229–32. doi: 10.1038/nmeth.3728
6. Antonetti DA, Reynet C, Kahn CR. Increased Expression of Mitochondrial-Encoded Genes in Skeletal Muscle of Humans With Diabetes Mellitus. J Clin Invest (1995) 95:1383–8. doi: 10.1172/JCI117790
7. Arruda AP, Pers BM, Parlakgül G, Güney E, Inouye K, Hotamisligil GS. Chronic Enrichment of Hepatic Endoplasmic Reticulum–Mitochondria Contact Leads to Mitochondrial Dysfunction in Obesity. Nat Med (2014) 20:1427–35. doi: 10.1038/nm.3735
8. Asmann YW, Stump CS, Short KR, Coenen-Schimke JM, Guo ZK, Bigelow ML, et al. Skeletal Muscle Mitochondrial Functions, Mitochondrial DNA Copy Numbers, and Gene Transcript Profiles in Type 2 Diabetic and Nondiabetic Subjects at Equal Levels of Low or High Insulin and Euglycemia. Diabetes (2006) 55:3309–19. doi: 10.2337/DB05-1230
9. Romanello V, Sandri M. Mitochondrial Quality Control and Muscle Mass; Maintenance. Front Physiol (2016) 6:422. doi: 10.3389/FPHYS.2015.00422
10. Larsson L, Degens H, Li M, Salviati L, Lee Y, Thompson W, et al. Sarcopenia: Aging-Related Loss of Muscle Mass and Function. Physiol Rev (2019) 99:427–511. doi: 10.1152/PHYSREV.00061.2017
11. Merz KE, Thurmond DC. Role of Skeletal Muscle in Insulin Resistance and Glucose Uptake. Compr Physiol (2020) 10:785–809. doi: 10.1002/CPHY.C190029
12. Boucher J, Kleinridders A, Ronald Kahn C. Insulin Receptor Signaling in Normal and Insulin-Resistant States. Cold Spring Harb Perspect Biol (2014) 6:1–23. doi: 10.1101/CSHPERSPECT.A009191
13. Alvim RO, Cheuhen MR, Machado SR, Sousa AGP, Santos PCJL. General Aspects of Muscle Glucose Uptake. Acad Bras Cienc (2015) 87:351–68. doi: 10.1590/0001-3765201520140225
14. Nesti L, Pugliese NR, Sciuto P, Natali A. Type 2 Diabetes and Reduced Exercise Tolerance: A Review of the Literature Through an Integrated Physiology Approach. Cardiovasc Diabetol (2020) 19:1–17. doi: 10.1186/S12933-020-01109-1
15. Weston KS, Wisløff U, Coombes JS. High-Intensity Interval Training in Patients With Lifestyle-Induced Cardiometabolic Disease: A Systematic Review and Meta-Analysis. Br J Sports Med (2014) 48:1227–34. doi: 10.1136/BJSPORTS-2013-092576
16. Yardley J, Mollard R, MacIntosh A, MacMillan F, Wicklow B, Berard L, et al. Vigorous Intensity Exercise for Glycemic Control in Patients With Type 1 Diabetes. Can J Diabetes (2013) 37:427–32. doi: 10.1016/J.JCJD.2013.08.269
17. Yardley JE, Sigal RJ, Perkins BA, Riddell MC, Kenny GP. Resistance Exercise in Type 1 Diabetes. Can J Diabetes (2013) 37:420–6. doi: 10.1016/J.JCJD.2013.07.020
18. Kirwan JP, Sacks J, Nieuwoudt S. The Essential Role of Exercise in the Management of Type 2 Diabetes. Cleve Clin J Med (2017) 84:S15. doi: 10.3949/CCJM.84.S1.03
19. Colberg SR, Sigal RJ, Yardley JE, Riddell MC, Dunstan DW, Dempsey PC, et al. Physical Activity/Exercise and Diabetes: A Position Statement of the American Diabetes Association. Diabetes Care (2016) 39:2065–79. doi: 10.2337/DC16-1728
20. Kennedy A, Nirantharakumar K, Chimen M, Pang TT, Hemming K, Andrews RC, et al. Does Exercise Improve Glycaemic Control in Type 1 Diabetes? A Systematic Review and Meta-Analysis. PloS One (2013) 8:1–10. doi: 10.1371/JOURNAL.PONE.0058861
21. Yardley JE, Kenny GP, Perkins BA, Riddell MC, Goldfield GS, Donovan L, et al. Resistance Exercise in Already-Active Diabetic Individuals (READI): Study Rationale, Design and Methods for a Randomized Controlled Trial of Resistance and Aerobic Exercise in Type 1 Diabetes. Contemp Clin Trials (2015) 41:129–38. doi: 10.1016/J.CCT.2014.12.017
22. Cuff DJ, Meneilly GS, Martin A, Ignaszewski A, Tildesley HD, Frohlich JJ. Effective Exercise Modality to Reduce Insulin Resistance in Women With Type 2 Diabetes. Diabetes Care (2003) 26:2977–82. doi: 10.2337/DIACARE.26.11.2977
23. Fonseca RM, Roschel H, Tricoli V, De Souza EO, Wilson JM, Laurentino GC, et al. Changes in Exercises are More Effective Than in Loading Schemes to Improve Muscle Strength. J strength Cond Res (2014) 28:3085–92. doi: 10.1519/JSC.0000000000000539
24. Kristiansen MS, Uhrbrand A, Hansen M, Shiguetomi-Medina JM, Vissing K, Stødkilde-Jørgensen H, et al. Concomitant Changes in Cross-Sectional Area and Water Content in Skeletal Muscle After Resistance Exercise. Scand J Med Sci Sports (2014) 24:e260–8. doi: 10.1111/SMS.12160
25. Bassi D, Mendes RG, Arakelian VM, Caruso FCR, Cabiddu R, Júnior JCB, et al. Potential Effects on Cardiorespiratory and Metabolic Status After a Concurrent Strength and Endurance Training Program in Diabetes Patients - a Randomized Controlled Trial. Sport Med - Open (2015) 2:31. doi: 10.1186/s40798-016-0052-1
26. Zhao X, He Q, Zeng Y, Cheng L. Effectiveness of Combined Exercise in People With Type 2 Diabetes and Concurrent Overweight/Obesity: A Systematic Review and Meta-Analysis. BMJ Open (2021) 11:e046252. doi: 10.1136/BMJOPEN-2020-046252
27. Kaneto H, Katakami N, Matsuhisa M, Matsuoka TA. Role of Reactive Oxygen Species in the Progression of Type 2 Diabetes and Atherosclerosis. Mediators Inflamm (2010) 2010:1–11. doi: 10.1155/2010/453892
28. Steinbacher P, Eckl P. Impact of Oxidative Stress on Exercising Skeletal Muscle. Biomolecules (2015) 5:356–77. doi: 10.3390/BIOM5020356
29. Zuo L, Zhou T, Pannell BK, Ziegler AC, Best TM. Biological and Physiological Role of Reactive Oxygen Species–the Good, the Bad and the Ugly. Acta Physiol (Oxf) (2015) 214:329–48. doi: 10.1111/APHA.12515
30. Stanford KI, Goodyear LJ. Exercise and Type 2 Diabetes: Molecular Mechanisms Regulating Glucose Uptake in Skeletal Muscle. Adv Physiol Educ (2014) 38:308–14. doi: 10.1152/ADVAN.00080.2014
31. Yan Z, Lira VA, Greene NP. Exercise Training-Induced Regulation of Mitochondrial Quality. Exerc Sport Sci Rev (2012) 40:159. doi: 10.1097/JES.0B013E3182575599
32. Kelley DE, He J, Menshikova EV, Ritov VB. Dysfunction of Mitochondria in Human Skeletal Muscle in Type 2 Diabetes. Diabetes (2002) 51:2944–50. doi: 10.2337/DIABETES.51.10.2944
33. Menshikova EV, Ritov VB, Fairfull L, Ferrell RE, Kelley DE, Goodpaster BH. Effects of Exercise on Mitochondrial Content and Function in Aging Human Skeletal Muscle. J Gerontol A Biol Sci Med Sci (2006) 61:534. doi: 10.1093/GERONA/61.6.534
34. Baar K, Wende AR, Jones TE, Marison M, Nolte LA, Chen M, et al. Adaptations of Skeletal Muscle to Exercise: Rapid Increase in the Transcriptional Coactivator PGC-1. FASEB J (2002) 16:1879–86. doi: 10.1096/FJ.02-0367COM
35. Smiles WJ, Camera DM. More Than Mitochondrial Biogenesis: Alternative Roles of PGC-1α in Exercise Adaptation. J Physiol (2015) 593:2115. doi: 10.1113/JP270177
36. Giacco F, Brownlee M. Oxidative Stress and Diabetic Complications. Circ Res (2010) 107:1058. doi: 10.1161/CIRCRESAHA.110.223545
37. Henriksen EJ, Diamond-Stanic MK, Marchionne EM. Oxidative Stress and the Etiology of Insulin Resistance and Type 2 Diabetes. Free Radic Biol Med (2011) 51:993–9. doi: 10.1016/J.FREERADBIOMED.2010.12.005
38. D’Souza DM, Al-Sajee D, Hawke TJ. Diabetic Myopathy: Impact of Diabetes Mellitus on Skeletal Muscle Progenitor Cells. Front Physiol (2013) 4:379. doi: 10.3389/fphys.2013.00379
39. Matough FA, Budin SB, Hamid ZA, Alwahaibi N, Mohamed J. The Role of Oxidative Stress and Antioxidants in Diabetic Complications. Sultan Qaboos Univ Med J (2012) 12:5. doi: 10.12816/0003082
40. Babizhayev MA, Strokov IA, Nosikov VV, Savel’yeva EL, Sitnikov VF, Yegor EY, et al. The Role of Oxidative Stress in Diabetic Neuropathy: Generation of Free Radical Species in the Glycation Reaction and Gene Polymorphisms Encoding Antioxidant Enzymes to Genetic Susceptibility to Diabetic Neuropathy in Population of Type I Diabetic Patients. Cell Biochem Biophys (2015) 71:1425–43. doi: 10.1007/S12013-014-0365-Y
41. Asmat U, Abad K, Ismail K. Diabetes Mellitus and Oxidative Stress-A Concise Review. Saudi Pharm J SPJ Off Publ Saudi Pharm Soc (2016) 24:547–53. doi: 10.1016/J.JSPS.2015.03.013
42. Phielix E, Meex R, Moonen-Kornips E, Hesselink MKC, Schrauwen P. Exercise Training Increases Mitochondrial Content and Ex Vivo Mitochondrial Function Similarly in Patients With Type 2 Diabetes and in Control Individuals. Diabetologia (2010) 53:1714–21. doi: 10.1007/S00125-010-1764-2
43. Hey-Mogensen M, Højlund K, Vind BF, Wang L, Dela F, Beck-Nielsen H, et al. Effect of Physical Training on Mitochondrial Respiration and Reactive Oxygen Species Release in Skeletal Muscle in Patients With Obesity and Type 2 Diabetes. Diabetologia (2010) 53:1976–85. doi: 10.1007/S00125-010-1813-X
44. Sparks LM, Johannsen NM, Church TS, Earnest CP, Moonen-Kornips E, Moro C, et al. Nine Months of Combined Training Improves Ex Vivo Skeletal Muscle Metabolism in Individuals With Type 2 Diabetes. J Clin Endocrinol Metab (2013) 98:1694–702. doi: 10.1210/JC.2012-3874
45. Little JP, Gillen JB, Percival ME, Safdar A, Tarnopolsky MA, Punthakee Z, et al. Low-Volume High-Intensity Interval Training Reduces Hyperglycemia and Increases Muscle Mitochondrial Capacity in Patients With Type 2 Diabetes. J Appl Physiol (2011) 111:1554–60. doi: 10.1152/JAPPLPHYSIOL.00921.2011
46. Atalay M, Laaksonen DE. Diabetes, Oxidative Stress and Physical Exercise. J Sports Sci Med (2002) 1:1.
47. Rains JL, Jain SK. Oxidative Stress, Insulin Signaling, and Diabetes. Free Radic Biol Med (2011) 50:567–75. doi: 10.1016/J.FREERADBIOMED.2010.12.006
48. Kawamura T, Muraoka I. Exercise-Induced Oxidative Stress and the Effects of Antioxidant Intake From a Physiological Viewpoint. Antioxid (Basel Switzerland) (2018) 7:1–19. doi: 10.3390/ANTIOX7090119
49. McKeegan K, Mason SA, Trewin AJ, Keske MA, Wadley GD, Della Gatta PA, et al. Reactive Oxygen Species in Exercise and Insulin Resistance: Working Towards Personalized Antioxidant Treatment. Redox Biol (2021) 44:1–17. doi: 10.1016/J.REDOX.2021.102005
50. Parker L, Stepto NK, Shaw CS, Serpiello FR, Anderson M, Hare DL, et al. Acute High-Intensity Interval Exercise-Induced Redox Signaling Is Associated With Enhanced Insulin Sensitivity in Obese Middle-Aged Men. Front Physiol (2016) 7:411. doi: 10.3389/FPHYS.2016.00411
51. Radak Z, Zhao Z, Koltai E, Ohno H, Atalay M. Oxygen Consumption and Usage During Physical Exercise: The Balance Between Oxidative Stress and ROS-Dependent Adaptive Signaling. Antioxid Redox Signal (2013) 18:1208–46. doi: 10.1089/ARS.2011.4498
52. de Sousa CV, Sales MM, Rosa TS, Lewis JE, de Andrade RV, Simões HG. The Antioxidant Effect of Exercise: A Systematic Review and Meta-Analysis. Sports Med (2017) 47:277–93. doi: 10.1007/S40279-016-0566-1
53. Powers SK, Deminice R, Ozdemir M, Yoshihara T, Bomkamp MP, Hyatt H. Exercise-Induced Oxidative Stress: Friend or Foe? J Sport Heal Sci (2020) 9:415–25. doi: 10.1016/J.JSHS.2020.04.001
54. Monaco CMF, Perry CGR, Hawke TJ. Diabetic Myopathy: Current Molecular Understanding of This Novel Neuromuscular Disorder. Curr Opin Neurol (2017) 30:545–52. doi: 10.1097/WCO.0000000000000479
55. Shah S, Sonawane P, Nahar P, Buge K, Vaidya S. Are We Ignoring Diabetic Disability: A Cross Sectional Study of Diabetic Myopathy. Indian J Med Sci (2011) 65:186–92. doi: 10.4103/0019-5359.106609
56. Wild S, Roglic G, Green A, Sicree R, King H. Global Prevalence of Diabetes: Estimates for the Year 2000 and Projections for 2030. Diabetes Care (2004) 27:1047–53. doi: 10.2337/DIACARE.27.5.1047
57. Tonoli C, Heyman E, Roelands B, Buyse L, Cheung SS, Berthoin S, et al. Effects of Different Types of Acute and Chronic (Training) Exercise on Glycaemic Control in Type 1 Diabetes Mellitus: A Meta-Analysis. Sports Med (2012) 42:1059–80. doi: 10.1007/BF03262312
58. Galassetti P, Riddell MC. Exercise and Type 1 Diabetes (T1DM). Compr Physiol (2013) 3:1309–36. doi: 10.1002/CPHY.C110040
59. García-García F, Kumareswaran K, Hovorka R, Hernando ME. Quantifying the Acute Changes in Glucose With Exercise in Type 1 Diabetes: A Systematic Review and Meta-Analysis. Sports Med (2015) 45:587–99. doi: 10.1007/S40279-015-0302-2
60. Riddell MC, Gallen IW, Smart CE, Taplin CE, Adolfsson P, Lumb AN, et al. Exercise Management in Type 1 Diabetes: A Consensus Statement. Lancet Diabetes Endocrinol (2017) 5:377–90. doi: 10.1016/S2213-8587(17)30014-1
61. Farinha JB, Krause M, Rodrigues-Krause J, Reischak-Oliveira A. Exercise for Type 1 Diabetes Mellitus Management: General Considerations and New Directions. Med Hypotheses (2017) 104:147–53. doi: 10.1016/J.MEHY.2017.05.033
62. Hernández-Ochoa EO, Llanos P, Lanner JT. The Underlying Mechanisms of Diabetic Myopathy. J Diabetes Res (2017) 2017:7485738. doi: 10.1155/2017/7485738
63. Corrêa APS, Ribeiro JP, Balzan FMH, Mundstock L, Ferlin EL, Moraes RS. Inspiratory Muscle Training in Type 2 Diabetes With Inspiratory Muscle Weakness. Med Sci Sports Exerc (2011) 43:1135–41. doi: 10.1249/MSS.0B013E31820A7C12
64. Albarrati A, Taher M, Nazer R. Effect of Inspiratory Muscle Training on Respiratory Muscle Strength and Functional Capacity in Patients With Type 2 Diabetes Mellitus: A Randomized Clinical Trial. J Diabetes (2021) 13:292–8. doi: 10.1111/1753-0407.13106
65. Jurisic-Erzen D, Starcevic-Klasan G, Ivanac D, Peharec S, Girotto D, Jerkovic R. The Effects of Alpha-Lipoic Acid on Diabetic Myopathy. J Endocrinol Investig 2017 412 (2017) 41:203–9. doi: 10.1007/S40618-017-0720-0
66. Khan MAB, Hashim MJ, King JK, Govender RD, Mustafa H, Kaabi J. Epidemiology of Type 2 Diabetes - Global Burden of Disease and Forecasted Trends. J Epidemiol Glob Health (2020) 10:107–11. doi: 10.2991/JEGH.K.191028.001
67. Hernández-Ochoa EO, Vanegas C. Diabetic Myopathy and Mechanisms of Disease. Biochem Pharmacol Open Access (2015) 4:1–5. doi: 10.4172/2167-0501.1000E179
68. Figueira FR, Umpierre D, Cureau FV, Zucatti ATN, Dalzochio MB, Leitão CB, et al. Association Between Physical Activity Advice Only or Structured Exercise Training With Blood Pressure Levels in Patients With Type 2 Diabetes: A Systematic Review and Meta-Analysis. Sports Med (2014) 44:1557–72. doi: 10.1007/S40279-014-0226-2
69. Acosta-Manzano P, Rodriguez-Ayllon M, Acosta FM, Niederseer D, Niebauer J. Beyond General Resistance Training. Hypertrophy Versus Muscular Endurance Training as Therapeutic Interventions in Adults With Type 2 Diabetes Mellitus: A Systematic Review and Meta-Analysis. Obes Rev (2020) 21:1–35. doi: 10.1111/OBR.13007
70. da Rocha RB, Silva CS, Cardoso VS. Self-Care in Adults With Type 2 Diabetes Mellitus: A Systematic Review. Curr Diabetes Rev (2020) 16:598–607. doi: 10.2174/1573399815666190702161849
71. Bekele H, Asefa A, Getachew B, Belete AM. Barriers and Strategies to Lifestyle and Dietary Pattern Interventions for Prevention and Management of TYPE-2 Diabetes in Africa, Systematic Review. J Diabetes Res (2020) 2020:1–14. doi: 10.1155/2020/7948712
72. Callisaya ML, Daly RM, Sharman JE, Bruce D, Davis TME, Greenaway T, et al. Feasibility of a Multi-Modal Exercise Program on Cognition in Older Adults With Type 2 Diabetes – A Pilot Randomised Controlled Trial. BMC Geriatr (2017) 17:1–10. doi: 10.1186/S12877-017-0635-9
73. Rodriguez-Mañas L, Laosa O, Vellas B, Paolisso G, Topinkova E, Oliva-Moreno J, et al. Effectiveness of a Multimodal Intervention in Functionally Impaired Older People With Type 2 Diabetes Mellitus. J Cachexia Sarcopenia Muscle (2019) 10:721–33. doi: 10.1002/JCSM.12432
74. Izquierdo M, Laosa O, Cadore EL, Abizanda P, Garcia-Garcia FJ, Hornillos M, et al. Two-Year Follow-Up of a Multimodal Intervention on Functional Capacity and Muscle Power in Frail Patients With Type 2 Diabetes. J Am Med Dir Assoc (2021) 22:1906–11. doi: 10.1016/J.JAMDA.2021.06.022
75. Sahin C, Courtney KL, Naylor P, Rhodes RE. Tailored Mobile Text Messaging Interventions Targeting Type 2diabetes Self-Management: A Systematic Review and Ameta-Analysis. Digit Heal (2019) 5:205520761984527. doi: 10.1177/2055207619845279
76. Huo X, Krumholz HM, Bai X, Spatz ES, Ding Q, Horak P, et al. Effects of Mobile Text Messaging on Glycemic Control in Patients With Coronary Heart Disease and Diabetes Mellitus: A Randomized Clinical Trial. Circ Cardiovasc Qual Outcomes (2019) 12:1–10. doi: 10.1161/CIRCOUTCOMES.119.005805
77. Connelly J, Kirk A, Masthoff J, Macrury S. The Use of Technology to Promote Physical Activity in Type 2 Diabetes Management: A Systematic Review. Diabetes Med (2013) 30:1420–32. doi: 10.1111/DME.12289
78. Du Y, Dennis B, Rhodes SL, Sia M, Ko J, Jiwani R, et al. Technology-Assisted Self-Monitoring of Lifestyle Behaviors and Health Indicators in Diabetes: Qualitative Study. JMIR Diabetes (2020) 5:1–12. doi: 10.2196/21183
79. Joseph RP, Ainsworth BE, Vega-López S, Adams MA, Hollingshead K, Hooker SP, et al. Rationale and Design of Smart Walk: A Randomized Controlled Pilot Trial of a Smartphone-Delivered Physical Activity and Cardiometabolic Risk Reduction Intervention for African American Women. Contemp Clin Trials (2019) 77:46–60. doi: 10.1016/J.CCT.2018.12.011
80. Sazlina SG, Browning C, Yasin S. Interventions to Promote Physical Activity in Older People With Type 2 Diabetes Mellitus: A Systematic Review. Front Public Heal (2013) 1:71. doi: 10.3389/FPUBH.2013.00071
Keywords: diabetes mellitus, diabetic myopathy, cardiometabolic rehabilitation, physical exercise, muscular strength
Citation: Bassi-Dibai D, Santos-de-Araújo AD, Dibai-Filho AV, de Azevedo LFS, Goulart CdL, Luz GCP, Burke PR, Garcia-Araújo AS and Borghi-Silva A (2022) Rehabilitation of Individuals With Diabetes Mellitus: Focus on Diabetic Myopathy. Front. Endocrinol. 13:869921. doi: 10.3389/fendo.2022.869921
Received: 05 February 2022; Accepted: 21 March 2022;
Published: 14 April 2022.
Edited by:
Ahmed Lawan, University of Alabama in Huntsville, United StatesReviewed by:
Kisuk Min, The University of Texas at El Paso, United StatesCopyright © 2022 Bassi-Dibai, Santos-de-Araújo, Dibai-Filho, de Azevedo, Goulart, Luz, Burke, Garcia-Araújo and Borghi-Silva. This is an open-access article distributed under the terms of the Creative Commons Attribution License (CC BY). The use, distribution or reproduction in other forums is permitted, provided the original author(s) and the copyright owner(s) are credited and that the original publication in this journal is cited, in accordance with accepted academic practice. No use, distribution or reproduction is permitted which does not comply with these terms.
*Correspondence: Daniela Bassi-Dibai, danielabassifisio@gmail.com