- 1Research Group Adipocytes and Metabolism, Institute for Diabetes and Obesity, Helmholtz Diabetes Center, Helmholtz Zentrum München, German Research Center for Environmental Health GmbH, Neuherberg, Germany
- 2German Center for Diabetes Research (DZD), Neuherberg, Germany
- 3Department of Medicine, Technische Universität München, Munich, Germany
Adipose tissue is essential for energy storage and endocrine regulation of metabolism. Imbalance in energy intake and expenditure result in obesity causing adipose tissue dysfunction. This alters cellular composition of the stromal cell populations and their function. Moreover, the individual cellular composition of each adipose tissue depot, regulated by environmental factors and genetics, determines the ability of the depots to expand and maintain its endocrine and storage function. Thus, stromal cells modulate adipocyte function and vice versa. In this mini-review we discuss heterogeneity in terms of composition and fate of adipose progenitor subtypes and their interactions with and regulation by different immune cell populations. Immune cells are the most diverse cell populations in adipose tissue and play essential roles in regulating adipose tissue function via interaction with adipocytes but also with adipocyte progenitors. We specifically discuss the role of macrophages, mast cells, innate lymphoid cells and T cells in the regulation of adipocyte progenitor proliferation, differentiation and lineage commitment. Understanding the factors and cellular interactions regulating preadipocyte expansion and fate decision will allow the identification of novel mechanisms and therapeutic strategies to promote healthy adipose tissue expansion without systemic metabolic impairment.
Introduction
White adipose tissue (WAT) is an important endocrine organ playing a key role in energy homeostasis and insulin action (1–4). Therefore, its development and expansion is crucial to establish and maintain a functional metabolism. However, excessive fat accumulation results in obesity, predisposing most people to the development of insulin resistance and the metabolic syndrome. A gain in fat mass results in various changes in adipose depots including remodeling of the extracellular matrix (ECM), recruitment and activation of immune cells, upregulation of pro-inflammatory cytokines and changes in adipogenesis and adipocyte function (5). However, a number of studies described a metabolically healthy obese (MHO) phenotype (6–9). One characteristic of these subjects is that in contrast to metabolically unhealthy obesity (MUO), these individuals accumulate fat preferentially in the subcutaneous rather than visceral depots. Despite their adiposity MHO individuals are insulin sensitive, glucose tolerant and do not display ectopic lipid accumulation in organs such as the liver or skeletal muscle (7, 8). The underlying mechanisms and temporal stability of healthy adipose tissue expansion are still incompletely understood. However, it appears that there are principle mechanisms available allowing the metabolically safe storage of very large quantities of energy in adipose tissue without impairing adipose or systemic metabolic function. Various genetic polymorphisms, altering adipocyte function, could contribute to the MHO phenotype (10, 11). Differences in the preadipocyte pool, its size, composition and proliferative capacity as well as the interaction with other cells of the stromal compartment, especially the diverse immune cell populations could also play an important role in the MHO phenotype. However, these interactions in regulation of healthy adipose tissue expansion are less understood. In addition to white adipose tissue function, differences in brown fat function could also play a role in MHO. Brown adipocytes, in contrast to white adipocytes, do not act as site of energy storage, but rather dissipate energy to produce heat through UCP-1 mediated mitochondrial uncoupling (12, 13). Others and we have identified heterogeneity within murine brown adipocyte progenitors and brown adipocytes modulating overall brown fat activity and systemic metabolism (14, 15). Thus, inter-individual differences in brown adipocyte composition could also modulate the physiological response to weight gain. Moreover, the role of beige adipocytes, which refers to brown adipocyte-like cells within white adipose tissue depots, has also not been extensively studied in the context of MHO. However, in this review we will focus on factors and cell populations that regulate the fate of white adipose progenitor cells (APCs), preadipocytes and tissue homeostasis with a main focus on immune cells.
Adipose Tissue During Development and Obesity
In humans, white adipose tissue starts to develop around 14 weeks of gestation as mesenchymal lobules that differentiate into primitive fat lobules. This is initiated by mesenchymal cell condensation resulting in the development of a capillary network and vascularization, followed by the proliferation of preadipocytes and formation of definitive fat lobules, which can be observed before week 28. The number of fat lobules is determined by week 23, while the size continuous to increase until week 29 (16, 17). In mice, subcutaneous white adipose tissue (sWAT) develops prenatally, starting between E14 and E18, while visceral white adipose tissue (vWAT) develops starting at P1 (18). In humans, the total number of adipocytes increases during childhood and adolescence, reaching a constant number at around 20 years of age, irrespective of the overall fat mass stored in a person. This indicates that inter-individual differences in adipocyte numbers are defined early in life and not by weight gain in adulthood (19, 20). However, obesity can also increase the estimated number of adipocytes, albeit it remains unclear how the subjects in this study differ from those in other studies that have not observed this increase in adipocyte numbers in adulthood (21).
Regardless, turnover of adipocytes and progenitor proliferation appear much more dynamic. In mice, around 5% of preadipocytes replicate and 1-5% of adipocytes are replaced per day. In humans the annual adipocyte turnover is around 10% (19, 22). Thus, adipocyte death, de novo differentiation and proliferation of adipocyte progenitors need to be tightly regulated to maintain a constant adipocyte number throughout life. Moreover, species differences in turnover of adipocytes and adipocyte progenitor proliferation should be considered when translating murine research data to humans. This is especially true for any therapeutic strategies aiming to increase the number of thermogenic beige adipocytes in WAT through de novo differentiation or promoting hyperplasia over hypertrophy.
In addition, obese individuals generate a higher number of new adipocytes every year compared to lean individuals. Conversely, significant body fat loss can also induce proliferation of adipocyte progenitor cells, as this was observed following bariatric surgery (19, 21). Thus, both weight gain and loss can have effects on the adipocyte progenitor pool. In mice, two weeks of high fat diet (HFD) feeding increased the proliferation of preadipocytes in visceral adipose tissue either compared to mice fed a chow diet (CD) or switched to a CD from a HFD (23). This effect was lost after 7 weeks of HFD feeding. However, prolonged HFD feeding increased the number of de novo differentiated adipocytes. Thus, there are complex interactions between a hypercaloric state requiring additional storage of energy in fat, progenitor expansion, and adipose tissue growth by either increasing the number of adipocytes (hyperplasia) or increasing adipocyte size (hypertrophy) (19, 24).
Expansion of adipose tissue through an increase in adipocyte number requires regulated proliferation of the adipocyte progenitor population to prevent progenitor exhaustion enabling continued adipogenesis throughout life. Thus, a variety of cell types, such as immune, endothelial and nerve cells interact with different subpopulations of APCs and preadipocytes to regulate adipose tissue function and growth, as well as homeostasis of the preadipocyte pool.
Heterogeneity and Hierarchy in Adipose Progenitor Cells
The definition of what exactly is an adipocyte progenitor cell is often unclear. As previously discussed, various cell types, such as mesenchymal stem cell, pericytes, endothelial cells and others can give rise to adipocytes (5). Moreover, advances in genetic lineage tracing and single cell technologies have identified intermediate states and distinct adipocyte progenitor lineages, giving rise to distinct adipocyte subpopulations (25–27). Following the definitions suggested by Sakers et al., we use the term preadipocytes to refer to cells with the general ability to differentiate into adipocytes (25). In addition, we distinguish, where possible, APCs that have a higher proliferative capacity, stem like properties and are able to maintain the progenitor niche in the tissue. In the following sections, we use the more general term preadipocytes whenever it is unclear if APCs or preadipocytes were studied.
Analysis of the stromal vascular fraction (SVF) from WAT identified adipocyte progenitor populations that are Lin−:CD34+:CD29+:Sca-1+:CD24+ (CD24+) or Lin−:CD34+:CD29+:Sca-1+:CD24− (CD24-), which was confirmed using PDGFRα-cre dependent reporter mice. Around 53% of the stromal vascular cells are CD24- preadipocytes, while only 0.08% were CD24+ APCs. These studies established a principle hierarchy where CD24 expressing APCs give rise to committed CD24- preadipocytes (Figure 1) (28, 29). Moreover, transplantation of CD24+ APCs but not CD24- preadipocytes into A-zip lipodystrophic mice were able to form a complete functional WAT, demonstrating the role of APCs in regulating stromal interactions and tissue homeostasis. Moreover, Jiang et al. described differences in ‘developmental’ and ‘adult’ APCs, regulating adipose tissue development and maintenance, respectively (30). PDGFRα+ stromal cells are necessary for tissue formation and adipose tissue (AT) expansion during tissue development, whereas other APC populations contributed to adipocyte turnover and tissue homeostasis during adulthood (31). Additional studies identified further subtypes within the PDGFRα+ cell population, based on the effects of PDGFRα signaling to induce fibrosis (32). PDGFRα+ CD9high cells were proliferative and induced fibrosis, whereas PDGFRα+ CD9low cells showed an upregulation in pro-adipogenic transcription factors (Figure 1). However, PDGFRα+ CD9low cells almost completely disappeared with AT fibrosis upon HFD feeding (33). Recently, a highly proliferative dipeptidyl peptidase-4 (DPP4)+ APC population was identified that gives rise to highly adipogenic ICAM1+ preadipocytes as well as a CD142+ adipogenic cell population, which was further studied by DPP4-Cre mediated lineage tracing experiments (Figure 1) (26, 34). Moreover, various other cell surface molecules have been described to distinguish APC and preadipocyte populations and we recently showed that the amino acid transporter ASC-1 regulates the commitment of APCs to become either white or beige adipocytes (35, 36). These findings highlight the heterogeneity within adipose progenitors that gives rise to different subsets of committed preadipocytes in AT. It is important to understand the complexity and diversity that is created by these subpopulation of cells to understand the mechanisms underlying tissue homeostasis and expansion. Moreover, these cells also interact with and are regulated by other cell types, especially immune cells, resident in AT.
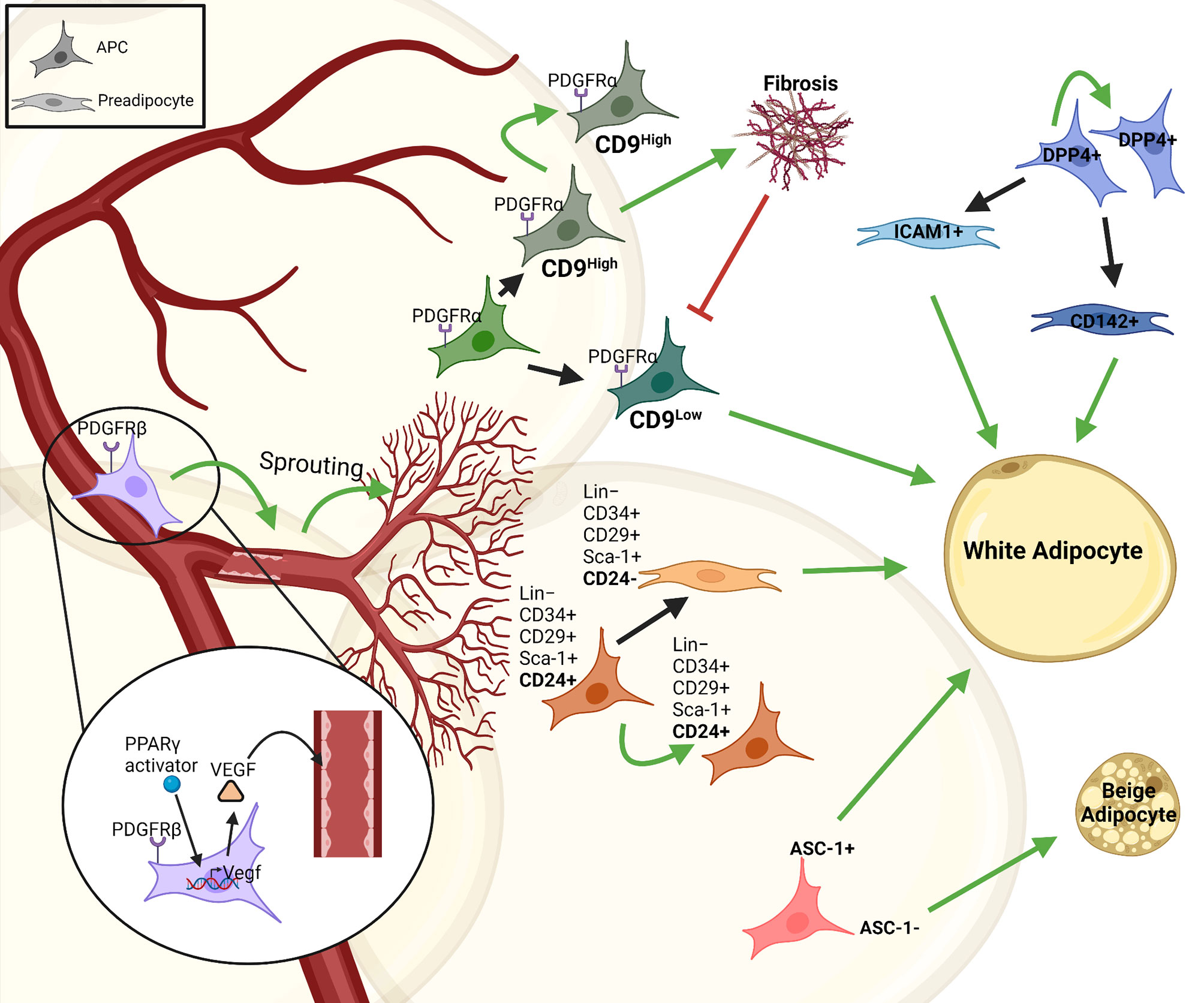
Figure 1 Heterogeneity and interactions of adipose progenitor cells. There are different subtypes of APCs with self-renewal properties in adipose tissue that give rise to various subpopulations of committed preadipocytes (e.g. CD24-, ICAM1+ or CD142+ cells). This proliferative/self-renewal capacity is represented by a green arrow that give rise to the same cell type. Some of these progenitors generate preadipocytes that differentiate into white adipocytes, such as; ASC1+, CD24+, DPP4+, while some are responsible for vascular-APC interaction including PDGFRβ+PPARγ+, others contribute to fibrosis in the tissue like PDGFRα+CD9high cells and some like ASC-1- cells give rise to beige adipocytes. The figure was generated using BioRender.com.
Interaction of Preadipocytes With Other Stromal Cells
The nervous-endocrine-immune system is an integrated regulatory network playing an essential role in maintaining homeostasis of the organism, including the adipose tissue (37, 38). The vasculature and neurons supply the adipose tissue with essential inputs such as nutrients, growth factors and signals from the autonomic nervous system. Thus, it is not surprising that in addition to adipocytes also APCs and preadipocytes continuously interact with other stromal cell types to regulate their function. APCs interact with endothelial cells through PPARγ mediated expression of VEGF, inducing vascular sprouting (Figure 1). This explains the perivascular localization of APCs (39). APCs also express PDGFRβ regulating APC-vascular niche interactions. These interactions are reciprocal as APCs are essential for vascular expansion, while vascular sprouting is essential for APC maintenance and differentiation. Most importantly, however, these interactions are necessary for adipogenesis and AT expansion (39).
In addition to the systemic inputs, immune cells modulate adipocyte function, as well as preadipocyte proliferation and differentiation either directly or indirectly (40–43). Many studies described adverse effects of chronic inflammation during obesity associated adipose tissue expansion (44). However, local inflammation is also, to some extent, necessary for healthy adipose tissue expansion and the MHO phenotype. Inhibition of pro-inflammatory pathways impairs adipogenesis, leading to ectopic lipid accumulation and systemic glucose intolerance (45, 46). The underlying mechanisms are still incompletely understood, but immune cell mediated reconfiguration of the ECM is certainly important for adipose tissue expansion. Moreover, the integration of various hormones and cytokines, in addition to nutritional cues, regulate processes of progenitor quiescence, proliferation and differentiation. To this end, we discuss some of the key regulatory inputs of different immune cell types on preadipocyte proliferation, differentiation and lineage (white versus beige adipocyte) commitment.
Macrophages
Macrophages are abundantly present and extensively studied in adipose tissue (47, 48). They play an important role in tissue homeostasis and hypertrophy induced adipose tissue inflammation and the initiation of local and systemic insulin resistance (49, 50). In general, accumulation of macrophages in adipose tissue is associated with insulin resistance and MUO (51). More recently, this was further refined as specific macrophage subtypes in visceral adipose tissue were found to be increased in diabetic obese compared to non-diabetic obese subjects (52). Previously, based on in vitro data, macrophages in adipose tissue were categorized as either M1 or M2. M1 macrophages originate from the stimulation with lipopolysaccharide (LPS), interferon (IFN)-γ or TNF-α, mediating pro-inflammatory actions by producing TNF-α, IL-6, IL-1β and nitric oxide (NO). M2 macrophages originate from the stimulation with IL-4, IL-10, IL-13, showing an anti-inflammatory phenotype by producing IL-4 and IL-10 (Figure 2) (53, 54). However, albeit widely used, the M1/M2 classification was recently challenged (55). Thus, previous in vitro data might not directly translate to the role of macrophages in adipose tissue in vivo. scRNAseq analysis of human white adipose tissues identified resident macrophages with an M1/M2-like phenotype. In contrast to the classical description of macrophages in in vitro studies, these subtypes were referred to as perivascular, inflammatory and lipid-associated macrophages. However, the interaction of these macrophage subtypes on progenitor cell fate has not been studied (47, 48, 52). Nevertheless, macrophages regulate tissue function through modulating preadipocyte expansion and commitment.
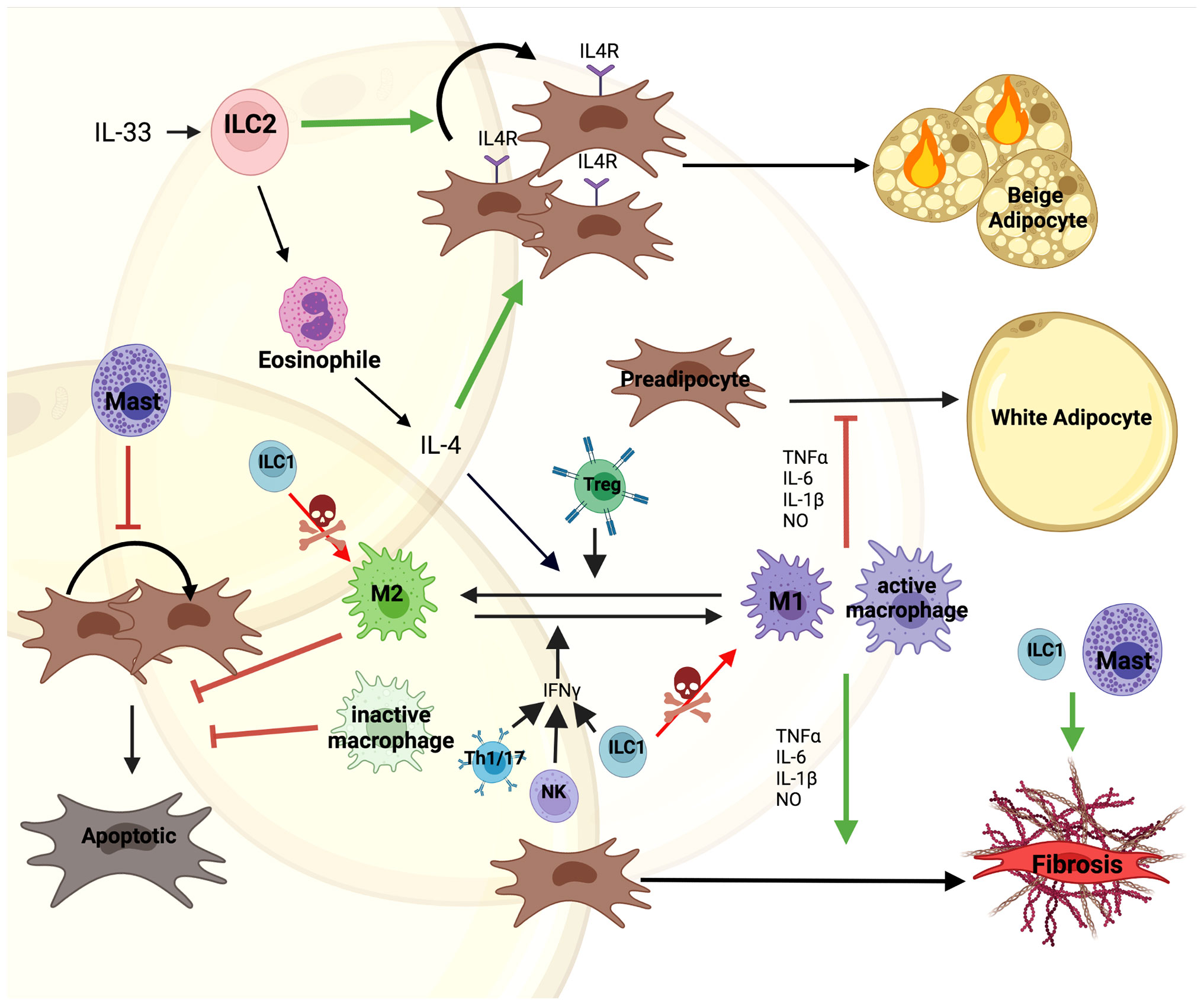
Figure 2 Direct and indirect interactions of immune cell with preadipocytes. Immune cells are one of the most diverse cell types constituting the microenvironment of AT. IL-33 mediated Type 2 Innate Lymphoid Cell (ILC2) activation induces secretion of IL-4 by eosinophils. This promotes both proliferation of IL4R expressing preadipocytes and their differentiation into beige adipocytes. Moreover, IL-4 along with Tregs promotes M1 to M2 macrophage polarization supporting M2 controlled survival of preadipocytes. On the other hand, Th1/17, Type 1 Innate Lymphoid Cells (ILC1) and Natural Killer (NK) cells secrete IFN-γ stimulating macrophage to polarize to the M1 phenotype, secreting pro-inflammatory factors, such as; TNFα, IL-6, IL-1β and nitric oxide (NO). This inhibits preadipocyte differentiation into white adipocytes and together with Mast cells induces a fibrotic phenotype. Mast cells also block proliferation of preadipocytes. Additionally, ILC1s kill macrophages and induce fibrosis in the tissue. The figure was generated using BioRender.com.
Relatively little is known on the role of macrophages and their secretome in regulating preadipocyte proliferation. Lacasa et al. used macrophage conditioned medium derived from in vitro differentiated blood monocytes of overweight patients. They showed increased proliferation but reduced differentiation of preadipocytes from non-obese donors when treated with the conditioned medium (56). Conversely, another human study found reduced preadipocyte proliferation when preadipocytes were exposed to macrophage conditioned media, which could be improved by antioxidant treatment (57). The discrepancy could be based on the different source of macrophages, as well as the physiologic and genetic background of the donors. A more detailed analysis of the impact of individual macrophage subtypes on preadipocytes is required to uncover the context specific role of macrophages on preadipocyte fate in humans.
Proliferation and differentiation are tightly linked with each other, as cell cycle exit is a prerequisite for adipocyte differentiation. Human and murine studies showed that M1 macrophages, the pro-inflammatory or classically activated macrophages, secrete factors inhibiting preadipocyte differentiation (56, 58, 59). The inhibitory effect was further confirmed by culturing CD14+ macrophages with preadipocytes of obese individuals, mediated by IKKβ/NF-κB signaling (60, 61). The block in differentiation was due to an impairment in the clonal expansion phase by inhibiting expression of cell cycle proteins such as; cyclin A, cyclin-dependent kinase 2, retinoblastoma protein, etc. (62, 63). Moreover, M1, pro-inflammatory macrophages promote preadipocytes to acquire a fibrotic phenotype, which is mediated through cytokines such as; TNF-α, IL-6 and IL-1β (Figure 2). This contributes to increased inflammation and tissue fibrosis impairing adipogenesis in both human and mice (56, 61, 64–66). However, these negative effects on preadipocyte differentiation were improved by growth hormone down-regulating IL-1β in macrophages (67).
Jang et al. compared high fat diet fed wild type to iNOS knockout mice and demonstrated that M1 macrophages produce nitric oxide impairing mitochondrial function by suppressing PGC1α expression. This inhibited adipocyte differentiation and promoted conversion of preadipocytes to a fibrotic phenotype (68). Furthermore, macrophages secrete the apoptosis inhibitor of macrophage, which also inhibited adipogenesis (69). In addition to proliferation and differentiation, maintenance and survival of existing preadipocytes is also controlled by macrophages, as macrophage conditioned medium promoted preadipocyte survival in a PDGF-dependent manner, which strongly stimulates PI3K-Akt and MEK-ERK1/2 pathways (70, 71). This effect was observed in M2 macrophages, activated by IL-4, but not activated M1 macrophages (72). Thus, the activation state of macrophages can have profound effects on preadipocyte survival, proliferation and differentiation and thereby shape the ability of the organism to store surplus energy in the future.
Mast Cells
Mast cells (MCs) are tissue resident cells of the innate immune system that accumulate in adipose tissue with obesity (73). As previously reviewed, MC recruitment and activation has pleiotropic effects on adipose tissue (74). Importantly, MC-released IFN-γ, chymase, tryptase, IL-6, and cysteinyl cathepsins promote adipogenesis as well as angiogenesis through direct actions on adipocytes and endothelial cells as well as macrophages (52, 75, 76). Moreover, MCs play an important role in the reconfiguration of the extracellular matrix. Thus, MCs are a highly dynamic and important part of the nervous-endocrine-immune system regulating adipose function. Current data on the role of mast cells on preadipocyte differentiation are restricted to murine studies and controversial. Hirai et al. showed that mast cell protease 6 (MCP-6) secreted by mature mast cells induces collagen V expression in obese adipose tissue, contributing to adipose tissue fibrosis inhibiting preadipocyte differentiation (Figure 2) (77). Mast cell deficient Kitw-sh/w-sh mice, which are fertile and non-anemic but also lack additional cell types mainly, melanocytes, and interstitial cells of Cajal, confirmed these previous findings and showed upregulated adipogenic capacity and preadipocyte proliferation (78). Similarly, mice where MCs were chemically inhibited as well as Kitw-sh/w-sh mice showed an increase in sWAT PDGFRα+ APC proliferation and induced beige (thermogenic) adipocyte differentiation (79). However, stimulation of MCs by calcium or high-glucose induced adipogenesis in 3T3-L1 preadipocytes through the production of 15-deoxy-delta-12, 14-prostaglandin J2 acting as an activator of PPARγ (80). Furthermore, Chen et al. demonstrated that mast cell derived heparin could act as the endogenous factor initiating fascial adipogenesis (81). These findings suggest an adipose depot specific effect of mast cells on adipocyte progenitors, dependent on other immune cells and the metabolic state of the organism. Moreover, available data on the role of MCs on adipocyte progenitors focus on studies in rodents, while the role in humans remains to be determined.
Innate Lymphoid Cells
Type 2 innate lymphoid cells (ILC2), which are part of the first line of the innate immune system, regulate type 2 immunity during tissue damage, parasite infection, and allergy (82–87). Apart from their role in wound healing, ILC2s also exhibit positive effects on metabolic homeostasis in visceral WAT, where they regulate eosinophils and alternatively activate macrophages (88). IL-33 mediated activation of ILC2s promote proliferation and commitment of APCs to become beige rather than white adipocytes (Figure 2) (89). In addition, an alternative pathway was described through the interaction of ILC2s with eosinophils, promoting secretion of IL-4, which activated IL-4R signaling in preadipocytes (89, 90). In the steady state, lacking IL-33, co-culture of adipose-derived ILC2s with 3T3-L1 cells induced adipocyte differentiation and lipid accumulation (91). Moreover, apart from ILC2s, ILC1s as well as NK cells play an important indirect role in modulating adipocyte-immune cell interactions. ILC1s kill adipose tissue macrophages and maintain macrophage homeostasis (Figure 2). However, this effect is abolished in obesity (92). Furthermore, adipose ILC1 secreted IFN-γ contributes to M1 macrophage polarization under HFD feeding (93, 94). Similar effects are also seen by NK cell activation (95, 96). Co-culture of ILC1 from obese subjects with lean stromal vascular cells upregulated the expression of fibrosis-related genes and ECM regulators, indicating that ILC1s promote fibrosis in obese adipose tissue (93).
T Cells
The direct effects of T cells on preadipocytes is unclear. Pan et al. showed that senescent T cells inhibit brown preadipocyte differentiation by secreting high levels of IFN-γ (97). Co-culture of active pan T cells with 3T3-L1 preadipocytes in vitro significantly suppressed differentiation (98). However, individual T cell subtypes play distinct roles in regulating inflammation in adipose tissue by modulating the switch between macrophage phenotypes. Pro-inflammatory T cells, such as; Th1 and Th17, activate pro-inflammatory macrophages by secreting IFNγ and IL-17. However, anti-inflammatory T cells, like Th2 and Foxp3+ Treg induced macrophage differentiation into the anti-inflammatory macrophages by secreting IL-4 and IL-13 (Figure 2) (99). Interestingly, the percentage of naive CD4+ and CD8+ T cells were significantly higher in insulin sensitive compared to insulin resistant obese individuals, whereas activated T cells and IL-6 levels were reduced (100). This suggests that T cells contribute to the low-grade inflammation observed in MUO, albeit the link to alterations in adipose tissue has to be made.
Here we provide an overview of selected adipose tissue resident immune cells and their role in modulating preadipocyte fate directly or indirectly (Figure 2). Due to the complex regulations between immune cells and with the various adipocyte precursor populations, the impact of these interactions on the MHO phenotype remains to be determined. Moreover, adipose tissue depot specific differences in progenitors and immune cell composition need to be considered to evaluate the role of individual immune cell populations on healthy AT expansion. Furthermore, it will be exciting to see additional in vivo data on the regulations and interdependence of progenitor cell proliferation and differentiation on each other.
Conclusion and Future Perspective
There is a rapidly increasing number of studies investigating adipocyte-preadipocyte-immune cell interaction. Thus, more and more details on the cellular subtypes and their regulation in obesity and the metabolic syndrome become available. Various computational approaches based on large–omics datasets are and will provide exciting new insights into the complex multidimensional spatial and temporal interactions of cell types, endocrine and nutritional inputs (47). This will allow a more holistic view on adipose tissues and their cellular interactions. However, these analyses are limited by the quality of the input data. Irrespective of the technical aspects of data acquisition, several points will need to be considered/improved to significantly advance our understanding of the control of adipocyte progenitor fate. One of the biggest limitations is the use of in vitro systems. Although simplification is important to identify molecular mechanism, the use of poorly defined progenitor or immune cell populations often results in data that cannot be translated to in vivo. Moreover, 2D cell culture models, most often do not allow “natural” cell-cell interactions, disregarding the effects of the ECM and biophysical properties such as oxygenation etc. Conversely, studying these mechanisms in vivo is also complex due to the lack of specific genetic models and low sample sizes. Moreover, the use of different mouse strains, in different life science disciplines, such as immunology and metabolism research also complicate the comparison of research data. To this end, novel developments such as spatial transcriptomics and single cell proteomics, could provide elegant ways to establish cell-cell interactions in vivo, most importantly in humans. Once these technical difficulties are solved, it will be fascinating to elucidate the reciprocal interaction of obesity and the metabolic syndrome with adipose stromal cell composition, function and activity. In summary, the expansion of adipose tissue, as well as the metabolic consequences of it in the context of MHO and MUO rely on a complex interplay between all cellular components, which should be studied as networks rather than linear interactions.
Author Contributions
All authors listed have made a substantial, direct, and intellectual contribution to the work, and approved it for publication.
Funding
XY received support from China Scholarship Council (No. 201908370218).
Conflict of Interest
The authors declare that the research was conducted in the absence of any commercial or financial relationships that could be construed as a potential conflict of interest.
Publisher’s Note
All claims expressed in this article are solely those of the authors and do not necessarily represent those of their affiliated organizations, or those of the publisher, the editors and the reviewers. Any product that may be evaluated in this article, or claim that may be made by its manufacturer, is not guaranteed or endorsed by the publisher.
Acknowledgments
We would like to thank Yasuhiro Onogi fruitful discussion. The figures were created with a licensed version of BioRender.com.
References
1. Friedman JM. Leptin and the Endocrine Control of Energy Balance. Nat Metab (2019) 1(8):754–64. doi: 10.1038/s42255-019-0095-y
2. Scheja L, Heeren J. The Endocrine Function of Adipose Tissues in Health and Cardiometabolic Disease. Nat Rev Endocrinol (2019) 15(9):507–24. doi: 10.1038/s41574-019-0230-6
3. Funcke JB, Scherer PE. Beyond Adiponectin and Leptin: Adipose Tissue-Derived Mediators of Inter-Organ Communication. J Lipid Res (2019) 60(10):1648–84. doi: 10.1194/jlr.R094060
4. Kershaw EE, Flier JS. Adipose Tissue as an Endocrine Organ. J Clin Endocrinol Metab (2004) 89(6):2548–56. doi: 10.1210/jc.2004-0395
5. Schoettl T, Fischer IP, Ussar S. Heterogeneity of Adipose Tissue in Development and Metabolic Function. J Exp Biol (2018) 221(Pt Suppl 1):1–17. doi: 10.1242/jeb.162958
6. Bluher M. Metabolically Healthy Obesity. Endocr Rev (2020) 41(3):1–16. doi: 10.1210/endrev/bnaa004
7. Loos RJF, Kilpelainen TO. Genes That Make You Fat, But Keep You Healthy. J Intern Med (2018) 284(5):450–63. doi: 10.1111/joim.12827
8. Ahl S, Guenther M, Zhao S, James R, Marks J, Szabo A, et al. Adiponectin Levels Differentiate Metabolically Healthy Vs Unhealthy Among Obese and Nonobese White Individuals. J Clin Endocrinol Metab (2015) 100(11):4172–80. doi: 10.1210/jc.2015-2765
9. Stefan N, Haring HU, Hu FB, Schulze MB. Metabolically Healthy Obesity: Epidemiology, Mechanisms, and Clinical Implications. Lancet Diabetes Endocrinol (2013) 1(2):152–62. doi: 10.1016/S2213-8587(13)70062-7
10. Justice AE, Karaderi T, Highland HM, Young KL, Graff M, Lu Y, et al. Protein-Coding Variants Implicate Novel Genes Related to Lipid Homeostasis Contributing to Body-Fat Distribution. Nat Genet (2019) 51(3):452–69. doi: 10.1038/s41588-018-0334-2
11. Shungin D, Winkler TW, Croteau-Chonka DC, Ferreira T, Locke AE, Magi R, et al. New Genetic Loci Link Adipose and Insulin Biology to Body Fat Distribution. Nature (2015) 518(7538):187–96. doi: 10.1038/nature14132
12. Harms M, Seale P. Brown and Beige Fat: Development, Function and Therapeutic Potential. Nat Med (2013) 19(10):1252–63. doi: 10.1038/nm.3361
13. Cannon B, Nedergaard J. Brown Adipose Tissue: Function and Physiological Significance. Physiol Rev (2004) 84(1):277–359. doi: 10.1152/physrev.00015.2003
14. Karlina R, Lutter D, Miok V, Fischer D, Altun I, Schottl T, et al. Identification and Characterization of Distinct Brown Adipocyte Subtypes in C57bl/6j Mice. Life Sci Alliance (2021) 4(1):1–19. doi: 10.26508/lsa.202000924
15. Song A, Dai W, Jang MJ, Medrano L, Li Z, Zhao H, et al. Low- and High-Thermogenic Brown Adipocyte Subpopulations Coexist in Murine Adipose Tissue. J Clin Invest (2020) 130(1):247–57. doi: 10.1172/JCI129167
16. Desoye G, Herrera E. Adipose Tissue Development and Lipid Metabolism in the Human Fetus: The 2020 Perspective Focusing on Maternal Diabetes and Obesity. Prog Lipid Res (2021) 81:101082. doi: 10.1016/j.plipres.2020.101082
17. Poissonnet CM, Burdi AR, Bookstein FL. Growth and Development of Human Adipose Tissue During Early Gestation. Early Hum Dev (1983) 8(1):1–11. doi: 10.1016/0378-3782(83)90028-2
18. Wang QA, Tao C, Gupta RK, Scherer PE. Tracking Adipogenesis During White Adipose Tissue Development, Expansion and Regeneration. Nat Med (2013) 19(10):1338–44. doi: 10.1038/nm.3324
19. Spalding KL, Arner E, Westermark PO, Bernard S, Buchholz BA, Bergmann O, et al. Dynamics of Fat Cell Turnover in Humans. Nature (2008) 453(7196):783–7. doi: 10.1038/nature06902
20. Knittle JL, Timmers K, Ginsberg-Fellner F, Brown RE, Katz DP. The Growth of Adipose Tissue in Children and Adolescents. Cross-Sectional and Longitudinal Studies of Adipose Cell Number and Size. J Clin Invest (1979) 63(2):239–46. doi: 10.1172/JCI109295
21. Petrus P, Mejhert N, Corrales P, Lecoutre S, Li Q, Maldonado E, et al. Transforming Growth Factor-Beta3 Regulates Adipocyte Number in Subcutaneous White Adipose Tissue. Cell Rep (2018) 25(3):551–60 e5. doi: 10.1016/j.celrep.2018.09.069
22. Rigamonti A, Brennand K, Lau F, Cowan CA. Rapid Cellular Turnover in Adipose Tissue. PloS One (2011) 6(3):e17637. doi: 10.1371/journal.pone.0017637
23. Kulenkampff E, Wolfrum C. Proliferation of Nutrition Sensing Preadipocytes Upon Short Term Hfd Feeding. Adipocyte (2019) 8(1):16–25. doi: 10.1080/21623945.2018.1521229
24. Hirsch J, Han PW. Cellularity of Rat Adipose Tissue: Effects of Growth, Starvation, and Obesity. J Lipid Res (1969) 10(1):77–82. doi: 10.1016/S0022-2275(20)42651-3
25. Sakers A, De Siqueira MK, Seale P, Villanueva CJ. Adipose-Tissue Plasticity in Health and Disease. Cell (2022) 185(3):419–46. doi: 10.1016/j.cell.2021.12.016
26. Merrick D, Sakers A, Irgebay Z, Okada C, Calvert C, Morley MP, et al. Identification of a Mesenchymal Progenitor Cell Hierarchy in Adipose Tissue. Science (2019) 364(6438):1–11. doi: 10.1126/science.aav2501
27. Lee KY, Luong Q, Sharma R, Dreyfuss JM, Ussar S, Kahn CR. Developmental and Functional Heterogeneity of White Adipocytes Within a Single Fat Depot. EMBO J (2019) 38(3):1–19. doi: 10.15252/embj.201899291
28. Berry R, Rodeheffer MS. Characterization of the Adipocyte Cellular Lineage in Vivo. Nat Cell Biol (2013) 15(3):302–8. doi: 10.1038/ncb2696
29. Rodeheffer MS, Birsoy K, Friedman JM. Identification of White Adipocyte Progenitor Cells In Vivo. Cell (2008) 135(2):240–9. doi: 10.1016/j.cell.2008.09.036
30. Jiang Y, Berry DC, Tang W, Graff JM. Independent Stem Cell Lineages Regulate Adipose Organogenesis and Adipose Homeostasis. Cell Rep (2014) 9(3):1007–22. doi: 10.1016/j.celrep.2014.09.049
31. Shin S, Pang Y, Park J, Liu L, Lukas BE, Kim SH, et al. Dynamic Control of Adipose Tissue Development and Adult Tissue Homeostasis by Platelet-Derived Growth Factor Receptor Alpha. Elife (2020) 9:1–21. doi: 10.7554/eLife.56189
32. Iwayama T, Steele C, Yao L, Dozmorov MG, Karamichos D, Wren JD, et al. Pdgfralpha Signaling Drives Adipose Tissue Fibrosis by Targeting Progenitor Cell Plasticity. Genes Dev (2015) 29(11):1106–19. doi: 10.1101/gad.260554.115
33. Marcelin G, Ferreira A, Liu Y, Atlan M, Aron-Wisnewsky J, Pelloux V, et al. A Pdgfralpha-Mediated Switch Toward Cd9(High) Adipocyte Progenitors Controls Obesity-Induced Adipose Tissue Fibrosis. Cell Metab (2017) 25(3):673–85. doi: 10.1016/j.cmet.2017.01.010
34. Stefkovich M, Traynor S, Cheng L, Merrick D, Seale P. Dpp4+ Interstitial Progenitor Cells Contribute to Basal and High Fat Diet-Induced Adipogenesis. Mol Metab (2021) 54:101357. doi: 10.1016/j.molmet.2021.101357
35. Onogi Y, Khalil A, Ussar S. Identification and Characterization of Adipose Surface Epitopes. Biochem J (2020) 477(13):2509–41. doi: 10.1042/BCJ20190462
36. Suwandhi L, Altun I, Karlina R, Miok V, Wiedemann T, Fischer D, et al. Asc-1 Regulates White Versus Beige Adipocyte Fate in a Subcutaneous Stromal Cell Population. Nat Commun (2021) 12(1):1588. doi: 10.1038/s41467-021-21826-9
37. Larabee CM, Neely OC, Domingos AI. Obesity: A Neuroimmunometabolic Perspective. Nat Rev Endocrinol (2020) 16(1):30–43. doi: 10.1038/s41574-019-0283-6
38. Libretti S, Puckett Y. Physiology, Homeostasis. In: Statpearls. Treasure Island, FL: StatPearls Publishing [Internet] (2022).
39. Jiang Y, Berry DC, Jo A, Tang W, Arpke RW, Kyba M, et al. A Ppargamma Transcriptional Cascade Directs Adipose Progenitor Cell-Niche Interaction and Niche Expansion. Nat Commun (2017) 8:15926. doi: 10.1038/ncomms15926
40. Cinkajzlova A, Mraz M, Haluzik M. Adipose Tissue Immune Cells in Obesity, Type 2 Diabetes Mellitus and Cardiovascular Diseases. J Endocrinol (2021) 252(1):R1–R22. doi: 10.1530/JOE-21-0159
41. Weinstock A, Moura Silva H, Moore KJ, Schmidt AM, Fisher EA. Leukocyte Heterogeneity in Adipose Tissue, Including in Obesity. Circ Res (2020) 126(11):1590–612. doi: 10.1161/CIRCRESAHA.120.316203
42. Villarroya F, Cereijo R, Gavalda-Navarro A, Villarroya J, Giralt M. Inflammation of Brown/Beige Adipose Tissues in Obesity and Metabolic Disease. J Intern Med (2018) 284(5):492–504. doi: 10.1111/joim.12803
43. Guzik TJ, Skiba DS, Touyz RM, Harrison DG. The Role of Infiltrating Immune Cells in Dysfunctional Adipose Tissue. Cardiovasc Res (2017) 113(9):1009–23. doi: 10.1093/cvr/cvx108
44. Kawai T, Autieri MV, Scalia R. Adipose Tissue Inflammation and Metabolic Dysfunction in Obesity. Am J Physiol Cell Physiol (2021) 320(3):C375–C91. doi: 10.1152/ajpcell.00379.2020
45. Wernstedt Asterholm I, Tao C, Morley TS, Wang QA, Delgado-Lopez F, Wang ZV, et al. Adipocyte Inflammation Is Essential for Healthy Adipose Tissue Expansion and Remodeling. Cell Metab (2014) 20(1):103–18. doi: 10.1016/j.cmet.2014.05.005
46. Lilja HE, Morrison WA, Han XL, Palmer J, Taylor C, Tee R, et al. An Adipoinductive Role of Inflammation in Adipose Tissue Engineering: Key Factors in the Early Development of Engineered Soft Tissues. Stem Cells Dev (2013) 22(10):1602–13. doi: 10.1089/scd.2012.0451
47. Hildreth AD, Ma F, Wong YY, Sun R, Pellegrini M, O’Sullivan TE. Single-Cell Sequencing of Human White Adipose Tissue Identifies New Cell States in Health and Obesity. Nat Immunol (2021) 22(5):639–53. doi: 10.1038/s41590-021-00922-4
48. Jaitin DA, Adlung L, Thaiss CA, Weiner A, Li B, Descamps H, et al. Lipid-Associated Macrophages Control Metabolic Homeostasis in a Trem2-Dependent Manner. Cell (2019) 178(3):686–98.e14. doi: 10.1016/j.cell.2019.05.054
49. Ruggiero AD, Key CC, Kavanagh K. Adipose Tissue Macrophage Polarization in Healthy and Unhealthy Obesity. Front Nutr (2021) 8:625331. doi: 10.3389/fnut.2021.625331
50. Ni Y, Ni L, Zhuge F, Xu L, Fu Z, Ota T. Adipose Tissue Macrophage Phenotypes and Characteristics: The Key to Insulin Resistance in Obesity and Metabolic Disorders. Obesity (Silver Spring) (2020) 28(2):225–34. doi: 10.1002/oby.22674
51. Kloting N, Fasshauer M, Dietrich A, Kovacs P, Schon MR, Kern M, et al. Insulin-Sensitive Obesity. Am J Physiol Endocrinol Metab (2010) 299(3):E506–15. doi: 10.1152/ajpendo.00586.2009
52. Muir LA, Cho KW, Geletka LM, Baker NA, Flesher CG, Ehlers AP, et al. Human Cd206+ Macrophages Associate With Diabetes and Adipose Tissue Lymphoid Clusters. JCI Insight (2022) 7(3):1–16. doi: 10.1172/jci.insight.146563
53. Russo L, Lumeng CN. Properties and Functions of Adipose Tissue Macrophages in Obesity. Immunology (2018) 155(4):407–17. doi: 10.1111/imm.13002
54. Thomas D, Apovian C. Macrophage Functions in Lean and Obese Adipose Tissue. Metabolism (2017) 72:120–43. doi: 10.1016/j.metabol.2017.04.005
55. Nahrendorf M, Swirski FK. Abandoning M1/M2 for a Network Model of Macrophage Function. Circ Res (2016) 119(3):414–7. doi: 10.1161/CIRCRESAHA.116.309194
56. Lacasa D, Taleb S, Keophiphath M, Miranville A, Clement K. Macrophage-Secreted Factors Impair Human Adipogenesis: Involvement of Proinflammatory State in Preadipocytes. Endocrinology (2007) 148(2):868–77. doi: 10.1210/en.2006-0687
57. Maumus M, Sengenes C, Decaunes P, Zakaroff-Girard A, Bourlier V, Lafontan M, et al. Evidence of in Situ Proliferation of Adult Adipose Tissue-Derived Progenitor Cells: Influence of Fat Mass Microenvironment and Growth. J Clin Endocrinol Metab (2008) 93(10):4098–106. doi: 10.1210/jc.2008-0044
58. Constant VA, Gagnon A, Yarmo M, Sorisky A. The Antiadipogenic Effect of Macrophage-Conditioned Medium Depends on Erk1/2 Activation. Metabolism (2008) 57(4):465–72. doi: 10.1016/j.metabol.2007.11.005
59. Constant VA, Gagnon A, Landry A, Sorisky A. Macrophage-Conditioned Medium Inhibits the Differentiation of 3t3-L1 and Human Abdominal Preadipocytes. Diabetologia (2006) 49(6):1402–11. doi: 10.1007/s00125-006-0253-0
60. Yarmo MN, Gagnon A, Sorisky A. The Anti-Adipogenic Effect of Macrophage-Conditioned Medium Requires the Ikkbeta/Nf-Kappab Pathway. Horm Metab Res (2010) 42(12):831–6. doi: 10.1055/s-0030-1263124
61. Liu LF, Craig CM, Tolentino LL, Choi O, Morton J, Rivas H, et al. Adipose Tissue Macrophages Impair Preadipocyte Differentiation in Humans. PloS One (2017) 12(2):e0170728. doi: 10.1371/journal.pone.0170728
62. Ide J, Gagnon A, Molgat AS, Landry A, Foster C, Sorisky A. Macrophage-Conditioned Medium Inhibits the Activation of Cyclin-Dependent Kinase 2 by Adipogenic Inducers in 3t3-L1 Preadipocytes. J Cell Physiol (2011) 226(9):2297–306. doi: 10.1002/jcp.22566
63. Yarmo MN, Landry A, Molgat AS, Gagnon A, Sorisky A. Macrophage-Conditioned Medium Inhibits Differentiation-Induced Rb Phosphorylation in 3t3-L1 Preadipocytes. Exp Cell Res (2009) 315(3):411–8. doi: 10.1016/j.yexcr.2008.10.036
64. Kang X, Liang H, Luo Y, Li Z, He F, Han X, et al. Streptococcus Thermophilus Mn-Zlw-002 Can Inhibit Pre-Adipocyte Differentiation Through Macrophage Activation. Biol Pharm Bull (2021) 44(3):316–24. doi: 10.1248/bpb.b20-00335
65. Gagnon A, Yarmo MN, Landry A, Sorisky A. Macrophages Alter the Differentiation-Dependent Decreases in Fibronectin and Collagen I/Iii Protein Levels in Human Preadipocytes. Lipids (2012) 47(9):873–80. doi: 10.1007/s11745-012-3696-8
66. Keophiphath M, Achard V, Henegar C, Rouault C, Clement K, Lacasa D. Macrophage-Secreted Factors Promote a Profibrotic Phenotype in Human Preadipocytes. Mol Endocrinol (2009) 23(1):11–24. doi: 10.1210/me.2008-0183
67. Lu C, Kumar PA, Fan Y, Sperling MA, Menon RK. A Novel Effect of Growth Hormone on Macrophage Modulates Macrophage-Dependent Adipocyte Differentiation. Endocrinology (2010) 151(5):2189–99. doi: 10.1210/en.2009-1194
68. Jang JE, Ko MS, Yun JY, Kim MO, Kim JH, Park HS, et al. Nitric Oxide Produced by Macrophages Inhibits Adipocyte Differentiation and Promotes Profibrogenic Responses in Preadipocytes to Induce Adipose Tissue Fibrosis. Diabetes (2016) 65(9):2516–28. doi: 10.2337/db15-1624
69. Kurokawa J, Arai S, Nakashima K, Nagano H, Nishijima A, Miyata K, et al. Macrophage-Derived Aim Is Endocytosed Into Adipocytes and Decreases Lipid Droplets Via Inhibition of Fatty Acid Synthase Activity. Cell Metab (2010) 11(6):479–92. doi: 10.1016/j.cmet.2010.04.013
70. Molgat AS, Gagnon A, Sorisky A. Macrophage-Induced Preadipocyte Survival Depends on Signaling Through Akt, Erk1/2, and Reactive Oxygen Species. Exp Cell Res (2011) 317(4):521–30. doi: 10.1016/j.yexcr.2010.10.024
71. Molgat AS, Gagnon A, Sorisky A. Preadipocyte Apoptosis Is Prevented by Macrophage-Conditioned Medium in a Pdgf-Dependent Manner. Am J Physiol Cell Physiol (2009) 296(4):C757–65. doi: 10.1152/ajpcell.00617.2008
72. Molgat AS, Gagnon A, Foster C, Sorisky A. The Activation State of Macrophages Alters Their Ability to Suppress Preadipocyte Apoptosis. J Endocrinol (2012) 214(1):21–9. doi: 10.1530/JOE-12-0114
73. Altintas MM, Azad A, Nayer B, Contreras G, Zaias J, Faul C, et al. Mast Cells, Macrophages, and Crown-Like Structures Distinguish Subcutaneous From Visceral Fat in Mice. J Lipid Res (2011) 52(3):480–8. doi: 10.1194/jlr.M011338
74. Elieh Ali Komi D, Shafaghat F, Christian M. Crosstalk Between Mast Cells and Adipocytes in Physiologic and Pathologic Conditions. Clin Rev Allergy Immunol (2020) 58(3):388–400. doi: 10.1007/s12016-020-08785-7
75. Liu J, Divoux A, Sun J, Zhang J, Clement K, Glickman JN, et al. Genetic Deficiency and Pharmacological Stabilization of Mast Cells Reduce Diet-Induced Obesity and Diabetes in Mice. Nat Med (2009) 15(8):940–5. doi: 10.1038/nm.1994
76. Zhou Y, Yu X, Chen H, Sjoberg S, Roux J, Zhang L, et al. Leptin Deficiency Shifts Mast Cells Toward Anti-Inflammatory Actions and Protects Mice From Obesity and Diabetes by Polarizing M2 Macrophages. Cell Metab (2015) 22(6):1045–58. doi: 10.1016/j.cmet.2015.09.013
77. Hirai S, Ohyane C, Kim YI, Lin S, Goto T, Takahashi N, et al. Involvement of Mast Cells in Adipose Tissue Fibrosis. Am J Physiol Endocrinol Metab (2014) 306(3):E247–55. doi: 10.1152/ajpendo.00056.2013
78. Ishijima Y, Ohmori S, Ohneda K. Mast Cell Deficiency Results in the Accumulation of Preadipocytes in Adipose Tissue in Both Obese and Non-Obese Mice. FEBS Open Bio (2013) 4:18–24. doi: 10.1016/j.fob.2013.11.004
79. Zhang X, Wang X, Yin H, Zhang L, Feng A, Zhang QX, et al. Functional Inactivation of Mast Cells Enhances Subcutaneous Adipose Tissue Browning in Mice. Cell Rep (2019) 28(3):792–803.e4. doi: 10.1016/j.celrep.2019.06.044
80. Tanaka A, Nomura Y, Matsuda A, Ohmori K, Matsuda H. Mast Cells Function as an Alternative Modulator of Adipogenesis Through 15-Deoxy-Delta-12, 14-Prostaglandin J2. Am J Physiol Cell Physiol (2011) 301(6):C1360–7. doi: 10.1152/ajpcell.00514.2010
81. Chen T, Zhang Y, Dong Y, Zhang D, Xia L, Sun X, et al. Mast Cell and Heparin Promote Adipogenesis in Superficial Fascia of Rats. Biochim Biophys Acta Mol Cell Biol Lipids (2021) 1866(11):159024. doi: 10.1016/j.bbalip.2021.159024
82. McKenzie ANJ, Spits H, Eberl G. Innate Lymphoid Cells in Inflammation and Immunity. Immunity (2014) 41(3):366–74. doi: 10.1016/j.immuni.2014.09.006
83. Koyasu S, Moro K. Th2-Type Innate Immune Responses Mediated by Natural Helper Cells. Ann N Y Acad Sci (2013) 1283:43–9. doi: 10.1111/nyas.12106
84. Walker JA, Barlow JL, McKenzie AN. Innate Lymphoid Cells–How Did We Miss Them? Nat Rev Immunol (2013) 13(2):75–87. doi: 10.1038/nri3349
85. Price AE, Liang HE, Sullivan BM, Reinhardt RL, Eisley CJ, Erle DJ, et al. Systemically Dispersed Innate Il-13-Expressing Cells in Type 2 Immunity. Proc Natl Acad Sci USA (2010) 107(25):11489–94. doi: 10.1073/pnas.1003988107
86. Neill DR, Wong SH, Bellosi A, Flynn RJ, Daly M, Langford TK, et al. Nuocytes Represent a New Innate Effector Leukocyte That Mediates Type-2 Immunity. Nature (2010) 464(7293):1367–70. doi: 10.1038/nature08900
87. Moro K, Yamada T, Tanabe M, Takeuchi T, Ikawa T, Kawamoto H, et al. Innate Production of T(H)2 Cytokines by Adipose Tissue-Associated C-Kit(+)Sca-1(+) Lymphoid Cells. Nature (2010) 463(7280):540–4. doi: 10.1038/nature08636
88. Molofsky AB, Nussbaum JC, Liang HE, Van Dyken SJ, Cheng LE, Mohapatra A, et al. Innate Lymphoid Type 2 Cells Sustain Visceral Adipose Tissue Eosinophils and Alternatively Activated Macrophages. J Exp Med (2013) 210(3):535–49. doi: 10.1084/jem.20121964
89. Brestoff JR, Kim BS, Saenz SA, Stine RR, Monticelli LA, Sonnenberg GF, et al. Group 2 Innate Lymphoid Cells Promote Beiging of White Adipose Tissue and Limit Obesity. Nature (2015) 519(7542):242–6. doi: 10.1038/nature14115
90. Lee MW, Odegaard JI, Mukundan L, Qiu Y, Molofsky AB, Nussbaum JC, et al. Activated Type 2 Innate Lymphoid Cells Regulate Beige Fat Biogenesis. Cell (2015) 160(1-2):74–87. doi: 10.1016/j.cell.2014.12.011
91. Miyajima Y, Ealey KN, Motomura Y, Mochizuki M, Takeno N, Yanagita M, et al. Effects of Bmp7 Produced by Group 2 Innate Lymphoid Cells on Adipogenesis. Int Immunol (2020) 32(6):407–19. doi: 10.1093/intimm/dxaa013
92. Boulenouar S, Michelet X, Duquette D, Alvarez D, Hogan AE, Dold C, et al. Adipose Type One Innate Lymphoid Cells Regulate Macrophage Homeostasis Through Targeted Cytotoxicity. Immunity (2017) 46(2):273–86. doi: 10.1016/j.immuni.2017.01.008
93. Wang H, Shen L, Sun X, Liu F, Feng W, Jiang C, et al. Adipose Group 1 Innate Lymphoid Cells Promote Adipose Tissue Fibrosis and Diabetes in Obesity. Nat Commun (2019) 10(1):3254. doi: 10.1038/s41467-019-11270-1
94. O’Sullivan TE, Rapp M, Fan X, Weizman OE, Bhardwaj P, Adams NM, et al. Adipose-Resident Group 1 Innate Lymphoid Cells Promote Obesity-Associated Insulin Resistance. Immunity (2016) 45(2):428–41. doi: 10.1016/j.immuni.2016.06.016
95. Wensveen FM, Jelencic V, Valentic S, Sestan M, Wensveen TT, Theurich S, et al. Nk Cells Link Obesity-Induced Adipose Stress to Inflammation and Insulin Resistance. Nat Immunol (2015) 16(4):376–85. doi: 10.1038/ni.3120
96. O’Rourke RW, Metcalf MD, White AE, Madala A, Winters BR, Maizlin II, et al. Depot-Specific Differences in Inflammatory Mediators and a Role for Nk Cells and Ifn-Gamma in Inflammation in Human Adipose Tissue. Int J Obes (Lond) (2009) 33(9):978–90. doi: 10.1038/ijo.2009.133
97. Pan XX, Yao KL, Yang YF, Ge Q, Zhang R, Gao PJ, et al. Senescent T Cell Induces Brown Adipose Tissue "Whitening" Via Secreting Ifn-Gamma. Front Cell Dev Biol (2021) 9:637424. doi: 10.3389/fcell.2021.637424
98. Wu H, Ghosh S, Perrard XD, Feng L, Garcia GE, Perrard JL, et al. T-Cell Accumulation and Regulated on Activation, Normal T Cell Expressed and Secreted Upregulation in Adipose Tissue in Obesity. Circulation (2007) 115(8):1029–38. doi: 10.1161/CIRCULATIONAHA.106.638379
99. Winer S, Chan Y, Paltser G, Truong D, Tsui H, Bahrami J, et al. Normalization of Obesity-Associated Insulin Resistance Through Immunotherapy. Nat Med (2009) 15(8):921–9. doi: 10.1038/nm.2001
Keywords: preadipocyte, immune cells, proliferation, differentiation, adipose tissue expansion, obesity, adipose precursor cells
Citation: Altun I, Yan X and Ussar S (2022) Immune Cell Regulation of White Adipose Progenitor Cell Fate. Front. Endocrinol. 13:859044. doi: 10.3389/fendo.2022.859044
Received: 20 January 2022; Accepted: 28 February 2022;
Published: 29 March 2022.
Edited by:
Matthias Blüher, Leipzig University, GermanyReviewed by:
Zoi Michailidou, University of Edinburgh, United KingdomAssaf Rudich, Ben-Gurion University of the Negev, Israel
Copyright © 2022 Altun, Yan and Ussar. This is an open-access article distributed under the terms of the Creative Commons Attribution License (CC BY). The use, distribution or reproduction in other forums is permitted, provided the original author(s) and the copyright owner(s) are credited and that the original publication in this journal is cited, in accordance with accepted academic practice. No use, distribution or reproduction is permitted which does not comply with these terms.
*Correspondence: Siegfried Ussar, U2llZ2ZyaWVkLnVzc2FyQGhlbG1ob2x0ei1tdW5pY2guZGU=
†These authors have contributed equally to this work and share first authorship