- Department of Human Molecular Genetics and Biochemistry, Sackler School of Medicine, Tel Aviv University, Tel Aviv, Israel
The breast and ovarian cancer susceptibility gene (BRCA1) is a tumor suppressor whose mutation has been associated with the development of breast, ovarian and, probably, other malignancies at young ages. The BRCA1 gene product participates in multiple biological pathways including the DNA damage response, transcriptional control, cell growth and apoptosis. Inactivating germline mutations of the BRCA1 gene can be detected in a substantial portion of families with inherited breast and/or ovarian cancer. While the genomic and cancer-related actions of BRCA1 have been extensively investigated, not much information exists regarding the cellular and circulating factors involved in regulation of BRCA1 expression and action. The present review article dissects the emerging role of BRCA1 as an important regulator of various endocrine and metabolic axes. Experimental and clinical evidence links BRCA1 with a number of peptide and steroid hormones. Furthermore, comprehensive analyses identified complex interactions between the insulin/insulin-like growth factor-1 (IGF1) signaling axis and BRCA1. The correlation between metabolic disorders, including diabetes and the metabolic syndrome, and BRCA1 mutations, are discussed in this article.
Discovery and Early Characterization of BRCA1
The race for the identification of the gene responsible for inherited breast and ovarian cancer ended in 1994 with the cloning of the BRCA1 gene by Miki and colleagues (1, 2). Positional cloning methodology allowed identification of a 17q-linked gene whose mutation affected susceptibility to breast and ovarian cancer. The BRCA1 gene encodes a predicted protein of 1863 amino acids, containing a distinct ring finger element in its N-terminal domain. The high penetrance of the BRCA1 gene was recognized early on in the course of BRCA1 characterization by analyses showing that mutation carriers have an increased lifetime risk of developing breast (40–85%) and/or ovarian (16–64%) cancers (3–8). In classical terms, BRCA1 fits the criteria of a candidate tumor suppressor gene and some of its archetypal biological activities are described in the next section.
While inactivating BRCA1 germline mutations are linked to a small portion of the total number of breast tumor cases worldwide, the cloning and subsequent characterization of the BRCA1 gene had an unprecedented impact on our understanding of breast cancer etiology (9). In fact, lessons learned from molecular and genetic analyses of BRCA1 transcended the area of familial breast and ovarian cancer and are regarded as universal biological paradigms in cancer (10). In addition to its genomic and cancer-related activities, more recent evidence revealed that BRCA1 displays a number of metabolic and hormone-like types of action. The present review article focuses on the involvement of tumor suppressor BRCA1 in endocrine system control. Our assay attempts to shed new light on the rapidly expanding spectrum of actions of BRCA1. Their potential clinical ramifications are discussed in detail.
BRCA1 Develops its Identity
Early studies identified BRCA1 as a critical player in the maintenance of genomic stability (11–13). Consistent with this role, cells with a defective BRCA1 gene exhibit a series of typical anomalies, including impaired DNA damage response, defects in homologous recombination with ensuing low efficiency DNA repair, and faulty cell cycle checkpoints (14–16). BRCA1 was shown to interact with a wide range of molecules, including BARD1 (via its N-terminal ring finger domain), DNA repair enzymes (mainly via its central domain), and transcriptional activators (primarily via two tandem BRCA1 C-terminal, or BRCT, motifs) (17–19) (Figure 1). A number of excellent review articles focusing on the physical and functional interactions of BRCA1 have been published (18, 20).
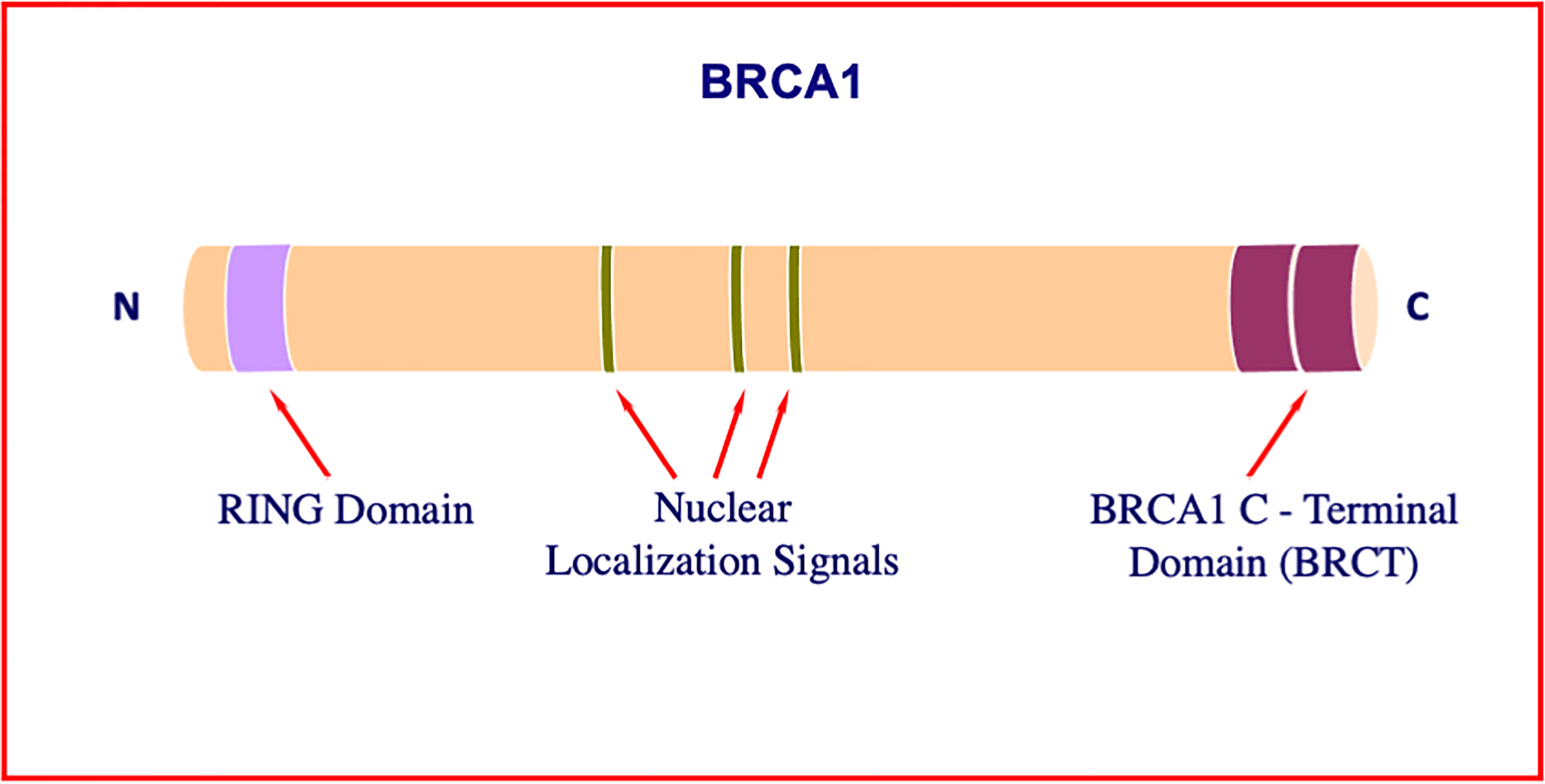
Figure 1 Structure of BRCA1. The BRCA1 gene encodes a 1863-amino acid protein with tumor suppressor activity. BRCA1 plays a critical role in DNA damage sensing and it forms a complex that repairs double-strand breaks. The N-terminal portion of the molecule includes a particular type of zinc finger element, termed RING motif. Among other roles, this domain interacts with proteins involved in BRCA1 ubiquitination. The central portion of the molecule includes a number of nuclear localization signals. The tandem C-terminal BRCT domain has important roles in DNA repair, transcription regulation and tumor suppressive functions.
The involvement of BRCA1 in transcriptional regulation is supported by solid experimental evidence (21, 22). Specifically: (i) BRCA1 is predominantly found within the nucleus (23, 24), albeit DNA damage as well as viral infection can alter its subcellular distribution (25); (ii) BRCA1 has been identified as a component of the RNA polymerase II holoenzyme by a number of biochemical criteria (19); (iii) the C-terminal domain of BRCA1 is highly acidic and exhibits a potent transcriptional transactivation activity (21, 26); and (iv) the N-terminal ring finger element resembles a similar motif described in several DNA-binding proteins, including the Wilms’ tumor suppressor, WT1 (1). Finally, a novel transcriptional mechanism responsible for autoregulation of BRCA1 gene transcription has been described (27). This regulatory loop involves the formation of a multimeric complex that contains, in addition to BRCA1, nuclear proteins E2F1 and RB. This complex displays a constitutive repressive activity pattern that leads to inhibition of BRCA1 transcription. Disruption of the complex by various genotoxic stresses results in displacement of the BRCA1 protein from the BRCA1 promoter region with subsequent upregulation of BRCA1 transcription.
BRCA1 Inhibits IGF1 Receptor Gene Expression and Action
The insulin-like growth factors (IGF1, IGF2) have an important role in the development and maturation of the mammary gland. In addition, IGFs are key players in breast cancer initiation and progression (28–31). Epidemiological analyses conducted over the past twenty-five years identified IGF1 as a risk factor for breast cancer (32–34). These population studies are in agreement with IGF1 function as a progression factor during the cell cycle (35, 36). Furthermore, studies reflect the well-established pro-survival role of IGF1 as well as its involvement in metabolic and nutritional control. The cell-surface IGF1 receptor (IGF1R), which mediates the biological actions of both IGF1 and IGF2, is regarded as a central player in breast cancer (31, 37–39). Constitutive activation of the IGF1R tyrosine kinase domain is a common event in cancer cells, although the prognostic significance of IGF1R levels and activation status in clinical settings remain unsettled (40).
Molecular, genetic and biochemical analyses identified complex physical and functional interactions between BRCA1 and the IGF1 signaling pathway (41, 42). Consistent with its tumor suppressor role, wild-type BRCA1 was shown to repress IGF1R gene transcription and promoter activity as well as endogenous IGF1R levels in breast cancer cells (43, 44). In contrast, a truncated form of BRCA1 (185delAG, a mutation with a high incidence among Ashkenazi Jews) was unable to inhibit IGF1R promoter activity (45, 46). The mechanism of action of BRCA1 involves interaction with Sp1, a zinc-finger transactivator of the IGF1R gene (Figure 2). Specifically, binding of BRCA1 to Sp1 prevents Sp1 from binding the IGF1R promoter region, leading to reduction in IGF1R levels and ensuing decrease in IGF1-mediated proliferation. In agreement with the inability of mutant BRCA1 to suppress IGF1R transcription, primary breast tumors derived from BRCA1 mutation carrier patients expressed significantly higher levels of IGF1R than sporadic breast tumors (47).
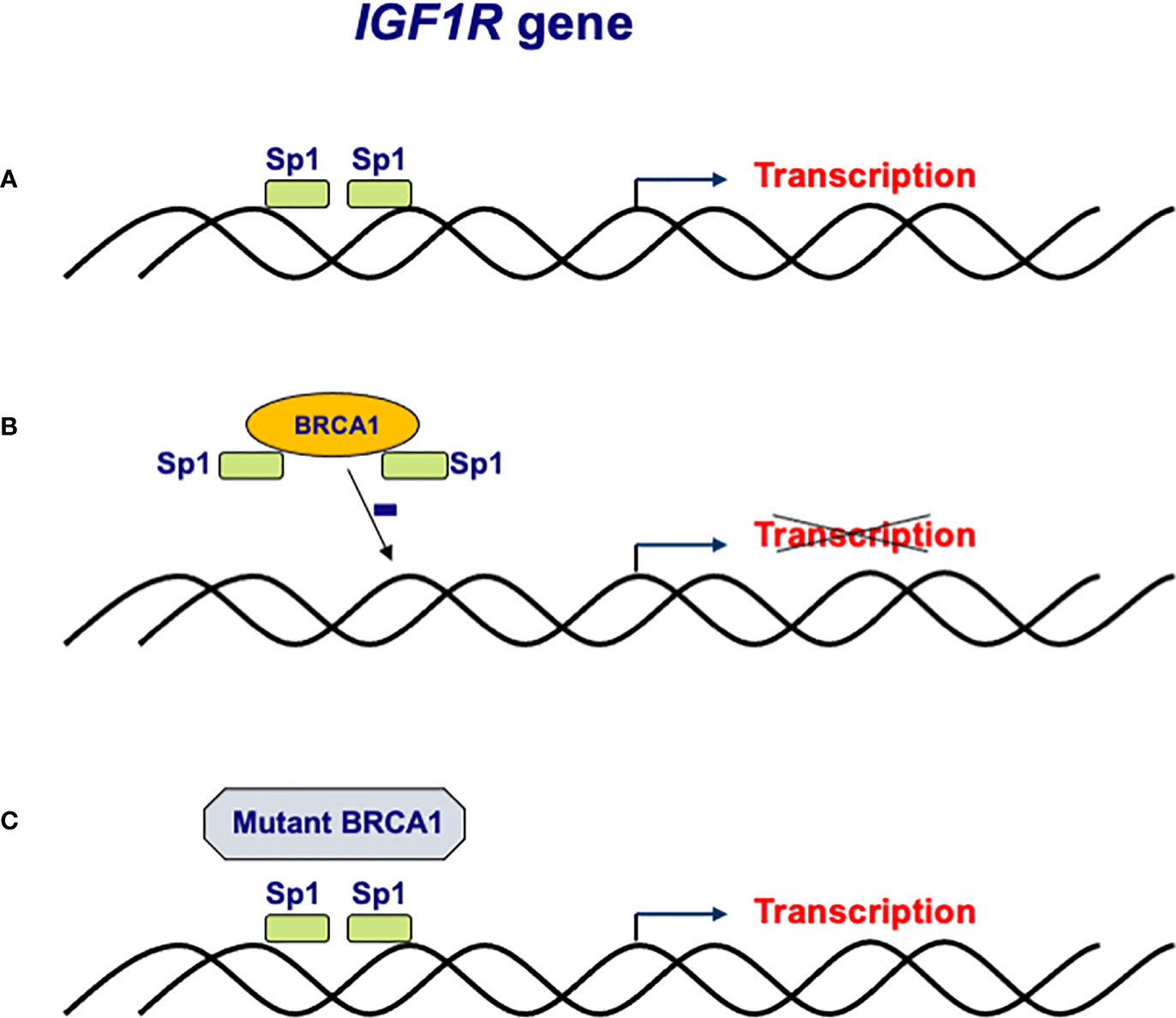
Figure 2 Regulation of IGF1R gene expression by BRCA1. (A) The IGF1R gene promoter includes a number of high affinity binding sites for transcription factor Sp1 in its proximal region. (B) The mechanism of action of BRCA1 involves binding to, and sequestration of, Sp1, thus preventing its binding to cis-elements in the promoter region. Lack of Sp1 binding leads to a reduction in IGF1R promoter activity and IGF1R levels. (C) Loss-of-function mutation of BRCA1 in familial and, potentially, sporadic breast cancer may lead to inactivation of BRCA1. Mutant BRCA1 is unable to bind Sp1 and suppress IGF1R gene transcription. Enhanced IGF1R levels are usually associated with augmented cell proliferation.
Of importance, the transcriptional activity of BRCA1 is strongly dependent on the cellular status of tumor suppressor p53. Thus, BRCA1 was capable of repressing IGF1R transcription in both p53-expressing and p53-null cellular backgrounds, but not in mutant p53-containing cells (48). As a corollary, loss-of-function mutation of the p53 gene in human cancer may affect the capacity of BRCA1 to repress IGF1R gene expression, with major clinical implications (49). In addition to breast cancer, BRCA1 was identified as a transcriptional repressor of the IGF1R gene in prostate and endometrial cancer cells (50–53).
Besides the IGF1R gene, BRCA1 was shown to target other components of the insulin-IGF1 axis. Intratumoral IGF1 concentrations were elevated in tumors from BRCA1 or BRCA2 mutation carriers compared with matched sporadic tumors (54). In addition, BRCA1 was shown to interact with the insulin receptor substrate-1 (IRS-1) promoter and to inhibit its activity (55). This effect at the promoter level was associated with epigenetic modifications of histone H3 and H4, leading to a transcriptional repressive chromatin configuration. Consistent with this inhibitory role, BRCA1-deficient mammary tumor cells exhibited high levels of IRS-1. Furthermore, suppression of IRS-1 using RNA interference markedly inhibited cell growth. Table 1 summarizes key concepts on the interaction between BRCA1 and the IGF1 axis.
Developmental and Hormonal Regulation of BRCA1 Expression
Ontogenetic analysis of BRCA1 gene expression in the normal mouse showed that BRCA1 is highly expressed in rapidly proliferating cells (56). In addition, BRCA1 expression is induced by positive growth signals at the cell cycle point where cells become committed to replicate their DNA and undergo cell division (57, 58). Maximal BRCA1 expression was detected during the pre-replicative (G1) phase of the cell cycle (59), and it was proved that BRCA1 is involved in the control of the G1-S and G2-M transition checkpoints (12, 16, 60). Finally, structure-function analyses have demonstrated that the tandem BRCT domain is essential in cell cycle checkpoint control by interacting in a phosphorylation-dependent fashion with specific DNA damage-induced proteins (61).
While BRCA1 has an important role in regulating IGF1R gene expression, as described in the previous section, experimental evidence indicates that both IGF1 and IGF2 stimulate BRCA1 expression in a dose-dependent fashion (62). The effect of the growth factors was mediated at the transcriptional level, as revealed by transfection experiments using BRCA1 promoter-luciferase reporter constructs. Given the fact that IGFs regulate cell division by controlling events that occur mainly during G1, it is reasonable to assume that at least part of the bioactivities of the IGFs are mediated by BRCA1. This concept is supported by experiments showing that BRCA1 silencing was associated with a two-fold increase in the IGF1-induced portion of cells that arrested at SubG0, and with a ~33% reduction in the portion of cells at the M-phase. In view of the fact that IGF1 is mainly produced by stromal cells whereas IGF2 biosynthesis occurs directly in breast tumor cells, data indicate that BRCA1 gene expression is potentially regulated by both autocrine (IGF2) and paracrine/endocrine (IGF1) stimuli (54).
Finally, AKT, a downstream IGF1 target, was shown to regulate BRCA1 stability independent of new protein synthesis (63). Hence, IGF1 signaling is capable of modulating BRCA1 abundance at various levels of regulation. Taken together, studies suggest that a feedback loop controls expression and action of the IGF1 and BRCA1 signaling pathways in a synchronized manner. Deregulated expression of BRCA1 as a result of aberrant IGF signaling might bear consequences in breast cancer development (41).
Metabolic Roles of BRCA1
The impact of obesity and diabetes on cancer risk in BRCA1 mutation carriers has been the topic of major clinical concern (64–66). While obesity and hyperinsulinemia are well established risk factors for breast cancer, and given the fact that BRCA1 exhibits a number of metabolic types of action, it is of medical relevance to explore the effects of a defective BRCA1 pathway on the linkage between diabetes and breast pathologies. The BRCA1-induced metabolic reprogramming of breast cancer cells was examined using global metabolomics and transcriptomics platforms (67). Wild-type BRCA1 induced numerous metabolic modifications, including a marked inhibition of glycolysis. Thus, all glycolysis indicators were largely (~50%) decreased in BRCA1wild-type, in comparison to BRCA1 mutant, cells. Five major enzymes of this pathway, including HK2 and PFKFB3, and both pyruvate and lactate were down-regulated by BRCA1 transfection. On the other hand, the tricarboxylic acid (TCA) cycle and oxidative phosphorylation were activated in BRCA1-expressing cells. In addition, BRCA1 induced a decrease of ketone bodies and free fatty acids, which were probably employed to supply Acetyl-CoA for the TCA cycle. Furthermore, BRCA1-transfected cells displayed enhanced activity of antioxidative pathways, most likely as a result of ROS production by oxidative phosphorylation. The overall implication of these analyses is that BRCA1 is capable or reversing the Warburg effect (see Table 2). The impact of this novel mechanism on tumor suppression has yet to be assessed.
A recent study examined the impact of reduced BRCA1 expression on metabolic reprogramming of ovarian cancer cells (68). Authors showed that BRCA1 depletion led to diminished mitochondrial respiration and reduced ATP concentrations. Of interest, these metabolic alterations sensitized the cells to agents that inhibit mitochondrial activity and glucose import. Hence, inhibition of energy metabolism might constitute a useful strategy to target BRCA1-deficient high grade serous ovarian cancer, a type of tumor characterized by frequent BRCA1 loss (69).
Bordeleau et al. examined the medical histories of 6,052 women with BRCA1 or BRCA2 mutations, half of whom had been diagnosed with breast cancer (70). Authors reported that there was no excess of diabetes among patients with breast cancer in the period before diagnosis, compared with control individuals without cancer. However, there was a doubling in the risk of diabetes among BRCA1 or BRCA2 mutation carriers in the 15-year period after diagnosis of breast cancer. Importantly, the risk was even higher for women with a BMI higher than 25. Authors suggested that the enhanced risk might be linked to weight gain after tumor therapy. Finally, Oliverio et al. examined the risk of metabolic exposures with respect to a number of BRCA1/2 variants in a cohort of 438 women carriers of BRCA1/2 mutations (71). Authors reported that loss-of-function variant carriers had significantly higher levels of plasma glucose and serum insulin than nonsynonymous variant carriers. Given that BRCA mutations confer a lower ability to repair DNA damage, authors suggested that mutation carriers may be more sensitive to the proliferative effects of insulin.
Involvement of BRCA1 in Lipogenesis
Given the connection between obesity and cancer risk, as described above, studies investigated the potential role of BRCA1 in regulation of lipogenesis and energy metabolism. Mutant BRCA1 has been associated with increased lipogenesis due to relaxation of the repressive action of wild-type BRCA1 on acetyl-CoA carboxylase, a key enzyme in fatty acid synthesis (72). Furthermore, BRCA1 mutation carriers seem to have decreased blood IGF-binding proteins concentrations and, sometimes, lack an allele containing cytosine–adenine repeats in the IGF1 gene promoter, which has been linked to decreased insulin sensitivity (7).
A recent study by Koobotse et al. reported that loss of BRCA1 in breast cancer cells led to downregulation of a phosphorylated and inactive form of acetyl CoA carboxylase-α (ACCA) (73). This effect was linked to a concomitant increase in levels of fatty acid synthase (FASN). In addition, IGF1 stimulated de-phosphorylation of ACCA by inhibiting the interaction between BRCA1 and phospho-ACCA. In consequence, deficit of BRCA1 increased the non-genomic effects of IGF1 as well as the mitogenic response of cells to IGF1. Furthermore, the effect of high glucose, as compared to physiological concentrations, on the tumor suppressive role of BRCA1 was investigated (74). Normal glucose levels blocked ACCA dephosphorylation by enhancing the association between BRCA1 and phospho-ACCA. The mitogenic response of breast cancer cells to IGF1 was decreased under physiological glucose values whereas no differences were seen in normal mammary epithelial cells. Hence, it is reasonable to assume that normal glucose concentrations facilitate the role of BRCA1 as a metabolic restraint of IGF1 actions. As a corollary, maintaining physiological levels of glucose may improve BRCA1 function and delay breast cancer progression.
In conclusion, the association between metabolic disorders, including diabetes and the metabolic syndrome, and BRCA1 and BRCA2 mutations is of major clinical relevance and warrants further investigation.
Interactions Between BRCA1 and Steroid Hormones
Early studies have identified functional interactions between BRCA1 and a number of steroid hormones, including the estrogen receptor-α (ERα) and androgen receptor (AR). BRCA1 inhibited the estradiol-inducible transcriptional activity of ERα in breast and prostate cancer cells whereas cancer-associated BRCA1-mutant cells did not exhibit depressed ERα activity (75, 76). On the other hand, estrogens are capable of enhancing BRCA1 expression, probably as a result of the mitogenic activity of estrogens. In addition, a direct effect of estrogens was suggested by studies showing that estradiol directly stimulates BRCA1 promoter activity.
Rosen et al. suggested that BRCA1 regulation of ERα signaling might be important in sporadic carcinogenesis given that this type of breast cancer, unlike BRCA1-associated tumors, are usually ERα-positive and often exhibit loss of BRCA1 expression. Loss of BRCA1 could result in unopposed estrogen stimulation of mammary epithelial cell proliferation (77). The impact of BRCA1/2 mutations on steroid hormone activity was assessed by examining endometrial thickness for each menstrual cycle day as an index of hormone regulation in a cohort of 228 women in the UK Familial Ovarian Cancer Screening Study (78). In addition, estradiol and progesterone titers for the same days were measured. Authors reported that BRCA1/2 mutation carriers were exposed to enhanced levels of both steroid hormones. Higher values of estradiol in mutation carriers are consistent with a potential carcinogenic role of this hormone in the ovary.
Evidence for functional interactions between BRCA1 and androgens was suggested by experiments showing differential regulation of the IGF1R gene by BRCA1 in androgen receptor (AR) positive, as compared to AR negative, prostate cancer cells (50). BRCA1 was expressed at relatively high levels in prostate cancer compared with a low BRCA1 immunostaining in normal prostate epithelium. In addition, there was a negative correlation between IGF1R and BRCA1 expression levels in AR-negative prostate cancer cells whereas in cells with an active AR there was a positive correlation. Finally, cotransfection experiments revealed that BRCA1 expression enhanced AR transcriptional activity. Taken together, analyses identified a new mechanism for IGF1R and AR stimulation of prostate cancer, and further support the relevance of targeting AR and IGF1R in this type of tumors with BRCA1 serving as a marker for defining the target activity.
BRCA1 Mutations and Reproduction
The potential impact of BRCA1 mutations on reproduction and fertility has been the focus of major interest (79). However, despite many efforts involved data remain controversial. A study by Kwiatkowski et al. suggested that BRCA1 mutations increase fertility in families at hereditary breast/ovarian cancer risk (80). Authors evaluated the following hypothesis: if mutations that favor cancer development have survived selection pressure through generations, it is reasonable to assume that these mutations must provide clear advantages that compensate for the reduction in life expectancy. Analyses were conducted on 2,150 families with hereditary cancer, including approximately 96,000 individuals. Authors reported that fertility advantages were seen in a subgroup of 746 BRCA1 mutation carriers and 483 non-carriers from BRCA1 mutated families. Female carriers were less often nulliparous (9.1% of carriers in comparison to 16.0% of non-carriers) and had more children (1.8 ± 1.4 vs 1.5 ± 1.3). Likewise, male carriers had more children (1.7 ± 1.3 vs 1.4 ± 1.3). While moderate, this increase in fertility in both male and female carriers is suggestive of a mechanism that compensates for shortening of the reproductive phase of life.
An additional report based on two longitudinal studies provides evidence that female BRCA1/2 mutation carriers had more children, shorter birth intervals and reproduced later in life when compared to matched controls (81). Authors suggested that the positive correlation between BRCA1/2 mutations and fertility can probably be explained by reported associations between BRCA mutations and telomere length and between telomere length and fertility.
In contrast, a negative impact of BRCA1/2 mutations on fertility was suggested by studies showing that these mutations negatively affect ovarian reserve through accumulated DNA damage (82). Ovarian stimulation was performed in 126 women with breast cancer by using letrozole and gonadotropins for the purpose of fertility preservation by embryo or oocyte cryopreservation. Compared to controls, BRCA1, but not BRCA2, mutation positive women produced lower numbers of eggs (7.4 vs 12.4) and had very high chances of low response to ovarian stimulation. Therefore, authors suggested that BRCA1 mutations are associated with occult primary ovarian insufficiency. Finally, a study by Shapira et al. shows no evidence of an association between BRCA1/2 mutations with a lower ovarian response in IVF treatment (83).
Interactions Between BRCA1 and Stress Hormones
The stress hormone hydrocortisone (cortisol) was shown to repress BRCA1 gene expression in a mouse mammary cell line (84). Furthermore, hydrocortisone was also demonstrated to inhibit the stimulatory effect of estrogen on BRCA1 expression, hence interfering with estrogen-related signaling in mammary epithelial cells. Hence, down-regulation of BRCA1 by cortisol may constitute a distinct pathological mechanism for involvement of stress hormones in breast carcinogenesis.
In addition, studies have identified a direct role for the unliganded glucocorticoid receptor (GR) in BRCA1 upregulation in the absence of hydrocortisone (85). GR was shown to physically interact with the BRCA1 gene promoter in the absence of hydrocortisone, whereas the positive effect of GR was lost upon addition of the ligand. Given the fact that low levels of BRCA1 have been correlated with the initiation and progression of sporadic breast cancer, this molecular mechanism may explain the finding that prolonged stress signaling increases breast cancer risk.
Conclusions
Studies summarized in the present review article emphasize the emerging role of BRCA1 as an important player in metabolic and endocrine regulation. While BRCA1 was discovered by virtue of its roles in cancer biology and its genomic activities, accumulating evidence indicates that BRCA1 displays a spectrum of actions that do not fall within the classical cancer-related types of action.
Among other physiological activities, BRCA1 was shown to induce the metabolic reprogramming of breast cancer cells with ensuing reversal of the Warburg effect. BRCA1 governs important steps of the lipogenetic pathway and has a key role in energy metabolism. BRCA1 interacts with several hormones, including IGF1, estrogens and androgens, cortisol, etc. In addition, BRCA1 seems to be involved in the process of reproduction.
In conclusion, a better understanding of the complex physical and functional interactions between BRCA1 and hormonal and metabolic pathways will have major basic and translational relevance.
Author Contributions
The author confirms being the sole contributor of this work and has approved it for publication.
Conflict of Interest
The author declares that the research was conducted in the absence of any commercial or financial relationships that could be construed as a potential conflict of interest.
Publisher’s Note
All claims expressed in this article are solely those of the authors and do not necessarily represent those of their affiliated organizations, or those of the publisher, the editors and the reviewers. Any product that may be evaluated in this article, or claim that may be made by its manufacturer, is not guaranteed or endorsed by the publisher.
Acknowledgments
HW is the incumbent of the Lady Davis Chair in Biochemistry.
References
1. Miki Y, Swensen J, Shattuck-Eidens D, Futreal PA, Harshman K, Tavtigian S, et al. A Strong Candidate for the Breast and Ovarian Cancer Susceptibility Gene BRCA1. Science (1994) 266:66–71. doi: 10.1126/science.7545954
2. Hall JM, Lee MK, Newman B, Morrow JE, Anderson LA, Huey B, et al. Linkage of Early-Onset Familial Breast Cancer to Chromosome 17q21. Science (1990) 250:1684–9. doi: 10.1126/science.2270482
3. Castilla LH, Couch FJ, Erdos MR, Hoskins KF, Calzone K, Garber JE, et al. Mutations in the BRCA1 Gene in Families With Early-Onset Breast and Ovarian Cancer. Nat Genet (1994) 8:387–91. doi: 10.1038/ng1294-387
4. Futreal PA, Liu Q, Shattuck-Eidens D, Cochran C, Harshman K, Tavtigian S, et al. BRCA1 Mutations in Primary Breast and Ovarian Carcinomas. Science (1994) 266:120–2. doi: 10.1126/science.7939630
6. King MC, Marks JH, Mandell JB. Breast and Ovarian Cancer Risks Due to Inherited Mutations in BRCA1 and BRCA2. Science (2003) 302:643–6. doi: 10.1126/science.1088759
7. Berstein LM. Endocrinology of the Wild and Mutant BRCA1 Gene and Types of Hormonal Carcinogenesis. Future Oncol (2008) 4:23–39. doi: 10.2217/14796694.4.1.23
8. Barcellos-Hoff MH, Kleinberg DL. Breast Cancer Risk in BRCA1 Mutation Carriers: Insights From Mouse Models. Ann Oncol (2013) 24:Viii8–viii12. doi: 10.1093/annonc/mdt305
9. Narod SA, Foulkes WD. BRCA1 and BRCA2: 1994 and Beyond. Nat Rev Cancer (2004) 4:665–76. doi: 10.1038/nrc1431
10. Turner NC, Reis-Filho JS, Russell AM, Springall RJ, Ryder K, Steele D, et al. BRCA1 Dysfunction in Sporadic Basal-Like Breast Cancer. Oncogene (2007) 26:2126–32. doi: 10.1038/sj.onc.1210014
11. Roy R, Chun J, Powell SN. BRCA1 and BRCA2: Different Roles in a Common Pathway of Genome Protection. Nat Rev Cancer (2012) 12:68–78. doi: 10.1038/nrc3181
12. Xu B, Kim S, Kastan MB. Involvement of Brca1 in s-Phase and G2-Phase Checkpoints After Ionizing Radiation. Mol Cell Bio (2001) 21:3445–50. doi: 10.1128/MCB.21.10.3445-3450.2001
13. Deng CX. BRCA1: Cell Cycle Checkpoint, Genetic Instability, DNA Damage Response and Cancer Evolution. Nucleic Acids Res (2006) 34:1416–26. doi: 10.1093/nar/gkl010
14. Gudas JM, Li T, Nguyen H, Jensen D, Rauscher FJ III, Cowan KH. Cell Cycle Regulation of BRCA1 Messenger RNA in Human Breast Epithelial Cells. Cell Growth Diff (1996) 7:717–23.
15. Kerr P, Ashworth A. New Complexities for BRCA1 and BRCA2. Curr Biol (2001) 11:R668–676. doi: 10.1016/S0960-9822(01)00389-X
16. Yarden RI, Pardo-Reoyo S, Sgagias M, Cowan KH, Brody LC. BRCA1 Regulates the G2/M Checkpoint by Activating Chk1 Kinase Upon DNA Damage. Nat Genet (2002) 30:285–9. doi: 10.1038/ng837
17. Rosen EM, Fan S, Ma Y. BRCA1 Regulation of Transcription. Cancer Lett (2006) 236:175–85. doi: 10.1016/j.canlet.2005.04.037
18. Mullan PB, Quinn JE, Harkin DP. The Role of BRCA1 in Transcriptional Regulation and Cell Cycle Control. Oncogene (2006) 25:5854–63. doi: 10.1038/sj.onc.1209872
19. Scully R, Anderson SF, Chao DM, Wei W, Ye L, Young RA, et al. BRCA1 is a Component of the RNA Polymerase II Holoenzyme. Proc Natl Acad Sci USA (1997) 94:5605–10. doi: 10.1073/pnas.94.11.5605
20. Silver DP, Livingston DM. Mechanisms of BRCA1 Tumor Suppression. Cancer Discovery (2012) 2:679–84. doi: 10.1158/2159-8290.CD-12-0221
21. Chapman MS, Verma IM. Transcriptional Activation by BRCA1. Nature (1996) 382:678–9. doi: 10.1038/382678a0
22. Welcsh PL, Lee MK, Gonzalez-Hernandez RM, Black DJ, Mahadevappa M, Swisher EM, et al. BRCA1 Transcriptionally Regulates Genes Involved in Breast Tumorigenesis. Proc Natl Acad Sci USA (2002) 99:7560–5. doi: 10.1073/pnas.062181799
23. Jin Y, Xu XL, Yang MC, Wei F, Ayi TC, Bowcock AM, et al. Cell Cycle-Dependent Colocalization of BARD1 and BRCA1 Proteins in Discrete Nyclear Domains. Proc Natl Acad Sci USA (1997) 94:12075–80. doi: 10.1073/pnas.94.22.12075
24. Scully R, Chen J, Ochs RL, Keegan K, Hoekstra M, Feunteun J, et al. Dynamic Changes of BRCA1 Subnuclear Location and Phosphorylation State are Initiated by DNA Damage. Cell (1997) 90:425–35. doi: 10.1016/S0092-8674(00)80503-6
25. Maul GG, Jensen DE, Ishov AM, Herlyn M, Rauscher FJ. III. Nuclear Redistribution of BRCA1 During Viral Infection. Cell Growth Diff (1998) 9:743–55.
26. Monteiro ANA, August A, Hanafusa H. Evidence for a Transcriptional Activation Function of BRCA1 C-Terminal Region. Proc Natl Acad Sci USA (1996) 93:13595–9. doi: 10.1073/pnas.93.24.13595
27. De Siervi A, De Luca P, Byun JS, Di LJ, Fufa T, Haggerty CM, et al. Transcriptional Autoregulation by BRCA1. Cancer Res (2010) 70:532–42. doi: 10.1158/0008-5472.CAN-09-1477
28. Lann D, LeRoith D. The Role of Endocrine Insulin-Like Growth Factor-I and Insulin in Breast Cancer. J Mamm Gland Biol Neoplasia (2008) 13:371–9. doi: 10.1007/s10911-008-9100-x
29. Farabaugh S, Boone D, Lee A. Role of IGF1R in Breast Cancer Subtypes, Stemness, and Lineage Differentiation. Front Endocrinol (Lausanne) (2015) 6:59. doi: 10.3389/fendo.2015.00059
30. Rota L, Wood T. Crosstalk of the Insulin-Like Growth Factor Receptor With the Wnt Signaling Pathway in Breast Cancer. Front Endocrinol (Lausanne) (2015) 6:92. doi: 10.3389/fendo.2015.00092
31. Yee D, Lee AV. Crosstalk Between the Insulin-Like Growth Factors and Estrogens in Breast Cancer. J Mamm Gland Biol Neoplasia (2000) 5:107–15. doi: 10.1023/a:1009575518338
32. Hankinson SE, Willett WC, Colditz GA, Hunter DJ, Michaud DS, Deroo B, et al. Circulating Concentrations of Insulin-Like Growth Factor-I and Risk of Breast Cancer. Lancet (1998) 351:1393–6. doi: 10.1016/S0140-6736(97)10384-1
33. Pollak M. The Insulin and Insulin-Like Growth Factor Receptor Family in Neoplasia: An Update. Nat Rev Cancer (2012) 12:159–69. doi: 10.1038/nrc3215
34. Renehan AG, Zwahlen M CM, O’Dwyer ST, Shalet SM, Egger M. Insulin-Like Growth Factor-I, IGF Binding Protein-3, and Cancer Risk: Systematic Review and Meta-Regression Analysis. Lancet (2004) 363:1346–53. doi: 10.1016/S0140-6736(04)16044-3
35. Baserga R, Peruzzi F, Reiss K. The IGF-1 Receptor in Cancer Biology. Int J Cancer (2003) 107:873–7. doi: 10.1002/ijc.11487
36. Dupont J, Pierre A, Froment P, Moreau C. The Insulin-Like Growth Factor Axis in Cell Cycle Pregression. Horm Metab Res (2003) 35:740–50. doi: 10.1055/s-2004-814162
37. LeRoith D, Roberts CT Jr. The Insulin-Like Growth Factor System and Cancer. Cancer Lett (2003) 195:127–37. doi: 10.1016/S0304-3835(03)00159-9
38. Werner H. The Pathophysiological Significance of IGF-I Receptor Overexpression: New Insights. Ped Endocrinol Rev (2009) 7:2–5.
39. Werner H, Laron Z. Role of the GH-IGF1 System in Progression of Cancer. Mol Cell Endocrinol (2020) 518:111003. doi: 10.1016/j.mce.2020.111003
40. Yerushalmi R, Gelmon KA, Leung S, Gao D, Cheang M, Pollak M, et al. Insulin-Like Growth Factor Receptor (IGF-IR) in Breast Subtypes. Breast Cancer Res Treat (2012) 132:131–42. doi: 10.1007/s10549-011-1529-8
41. Werner H, Bruchim I. IGF-1 and BRCA1 Signalling Pathways in Familial Cancer. Lancet Oncol (2012) 13:e537–44. doi: 10.1016/S1470-2045(12)70362-5
42. Werner H. Tumor Suppressors Govern Insulin-Like Growth Factor Signaling Pathways: Implications in Metabolism and Cancer. Oncogene (2012) 31:2703–14. doi: 10.1038/onc.2011.447
43. Maor SB, Abramovitch S, Erdos MR, Brody LC, Werner H. BRCA1 Suppresses Insulin-Like Growth Factor-I Receptor Promoter Activity: Potential Interaction Between BRCA1 and Sp1. Mol Gen Metab (2000) 69:130–6. doi: 10.1006/mgme.1999.2958
44. Abramovitch S, Glaser T, Ouchi T, Werner H. BRCA1-Sp1 Interactions in Transcriptional Regulation of the IGF-IR Gene. FEBS Lett (2003) 541:149–54. doi: 10.1016/s0014-5793(03)00315-6
45. Abeliovich D, Kaduri L, Lerer I, Weinberg N, Amir G, Sagi M, et al. The Founder Mutations 185delag and 5382insc in BRCA1 and 6174delt in BRCA2 Appear in 60% of Ovarian Cancer and 30% of Early-Onset Breast Cancer Patients Among Ashkenazi Women. Am J Hum Genet (1997) 60:505–14.
46. Shiri-Sverdlov SR, Oefner P, Green L, Baruch RG, Wagner T, Kruglikova A, et al. Mutational Analysis of BRCA1 and BRCA2 in Ashkenazi and Non-Ashkenazi Jewish Women With Familial Breast and Ovarian Cancer. Hum Mutat (2000) 16:491–501. doi: 10.1002/1098-1004(200012)16:6<491::AID-HUMU6>3.0.CO;2-J
47. Maor S, Yosepovich A, Papa MZ, Yarden RI, Mayer D, Friedman E, et al. Elevated Insulin-Like Growth Factor-I Receptor (IGF-IR) Levels in Primary Breast Tumors Associated With BRCA1 Mutations. Cancer Lett (2007) 257:236–43. doi: 10.1016/j.canlet.2007.07.019
48. Abramovitch S, Werner H. Functional and Physical Interactions Between BRCA1 and P53 in Transcriptional Regulation of the IGF-IR Gene. Horm Metab Res (2003) 35:758–62. doi: 10.1055/s-2004-814154
49. Werner H, Sarfstein R, LeRoith D, Bruchim I. Insulin-Like Growth Factor 1 Signaling Axis Meets P53 Genome Protection Pathways. Front Oncol (2016) 6:159. doi: 10.3389/fonc.2016.00159
50. Schayek H, Haugk K, Sun S, True LD, Plymate SR, Werner H. Tumor Suppressor BRCA1 is Expressed in Prostate Cancer and Control IGF1-R Gene Transcription in an Androgen Receptor-Dependent Manner. Clin Cancer Res (2009) 15:1558–65. doi: 10.1158/1078-0432.CCR-08-1440
51. Amichay K, Kidron D, Attias-Geva Z, Schayek H, Sarfstein R, Fishman A, et al. BRCA1 is Expressed in Uterine Serous Carcinoma and Controls Insulin-Like Growth Factor I Receptor Gene Expression in USC Cell Lines. Int J Gynecol Cancer (2012) 22:748–54. doi: 10.1097/IGC.0b013e318254011f
52. Bruchim I, Amichay K, Kidron D, Attias Z, Biron-Shental T, Drucker L, et al. BRCA1/2 Germline Mutations in Jewish Patients With Uterine Serous Carcinoma. Int J Gynecol Cancer (2010) 20:1148–53. doi: 10.1111/IGC.0b013e3181ef622d
53. Pennington KP, Walsh T, Lee M, Pennil C, Novetsky AP, Agnew KJ, et al. BRCA1, TP53, and CHEK2 Germline Mutations in Uterine Serous Carcinoma. Cancer (2013) 119:332–8. doi: 10.1002/cncr.27720
54. Hudelist G, Wagner T, Rosner M, Fink-Retter A, Gschwantler-Kaulich D, Czerwenka K, et al. Intratumoral IGF-I Protein Expression is Selectively Upregulated in Breast Cancer Patients With BRCA1/2 Mutations. Endocr Related Cancer (2007) 14:1053–62. doi: 10.1677/ERC-06-0075
55. Shukla V, Coumoul X, Cao L, Wang RH, Xiao C, Xu X, et al. Absence of the Full-Length Breast Cancer-Associated Gene-1 Leads to Increased Expression of Insulin-Like Growth Factor Signaling Axis Members. Cancer Res (2006) 66:7151–7. doi: 10.1158/0008-5472.CAN-05-4570
56. Marquis ST, Rajan JV, Wynshaw-Boris A, Xu J, Yin G-Y, Abel KJ, et al. The Developmental Pattern of BRCA1 Expression Implies a Role in Differentiation of the Breast and Other Tissues. Nat Genet (1995) 11:17–26. doi: 10.1038/ng0995-17
57. Satterwhite DJ, Matsunami N, White RL. TGF-Beta1 Inhibits BRCA1 Expression Through a Pathway That Requires Prb. Biochem Biophys Res Comm (2000) 276:686–92. doi: 10.1006/bbrc.2000.3510
58. Thomas JE, Smith M, Tonkinson JL, Rubinfeld B, Polakis P. Induction of Phosphorylation on BRCA1 During the Cell Cycle and After DNA Damage. Cell Growth Diff (1997) 8:801–9.
59. Vaughn JP, Davis PL, Jarboe MD, Huper G, Evans AC, Wiseman RW, et al. BRCA1 Expression is Induced Before DNA Synthesis in Both Normal and Tumor-Derived Breast Cells. Cell Growth Diff (1996) 7:711–5.
60. Somasundaram K, Zhang H, Zeng YX, Houvras Y, Peng Y, Zhang H, et al. Arrest of the Cell Cycle by the Tumour-Suppressor BRCA1 Requires the CDK-Inhibitor P21waf1/Cip1. Nature (1997) 389:187–90. doi: 10.1038/38291
61. Varma AK, Brown RS, Birrane G, Ladias JAA. Structural Basis for Cell Cycle Checkpoint Control by the BRCA1-Ctip Complex. Biochemistry (2005) 44:10941–6. doi: 10.1021/bi0509651
62. Maor S, Papa MZ, Yarden RI, Friedman E, Lerenthal Y, Lee SW, et al. Insulin-Like Growth Factor-I Controls BRCA1 Gene Expression Through Activation of Transcription Factor Sp1. Horm Metab Res (2007) 39:179–85. doi: 10.1055/s-2007-970415
63. Nelson AC, Lyons TR, Young CD, Hansen KC, Anderson CM, Holt JT. Akt Regulates BRCA1 Stability in Response to Hormone Signaling. Mol Cell Endocrinol (2010) 319:129–42. doi: 10.1016/j.mce.2010.01.019
64. Clayton P, Banerjee I, Murray PG, Renehan AG. Growth Hormone, the Insulin-Like Growth Factor Axis, Insulin and Cancer Risk. Nat Rev Endocrinol (2011) 7:11–24. doi: 10.1038/nrendo.2010.171
65. Antoniou A, Pharoah PD, Narod S, Risch HA, Eyfjord JE, Hopper JL, et al. Average Risks of Breast and Ovarian Cancer Associated With BRCA1 or BRCA2 Mutations Detected in Case Series Unselected for Family History: A Combined Analysis of 22 Studies. Am J Hum Genet (2003) 72:1117–30. doi: 10.1086/375033
66. Narod SA. BRCA Mutations in the Management of Breast Cancer: The State of the Art. Nat Rev Clin Oncol (2010) 7:702–7. doi: 10.1038/nrclinonc.2010.166
67. Privat M, Radosevic-Robin N, Aubel C, Cayre A, Penault-Llorca F, Marceau G, et al. BRCA1 Induces Major Energetic Metabolism Reprogramming in Breast Cancer Cells. PloS One (2014) 9:e102438. doi: 10.1371/journal.pone.0102438
68. Kanakkanthara A, Kurmi K, Ekstrom TL, Hou X, Purfeerst ER, Heinzen EP, et al. BRCA1 Deficiency Upregulates NNMT, Which Reprograms Metabolism and Sensitizes Ovarian Cancer Cells to Mitochondrial Metabolic Targeting Agents. Cancer Res (2019) 79:5920–9. doi: 10.1158/0008-5472.CAN-19-1405
69. Bruchim I, Fishman A, Friedman E, Goldberg I, Chetrit A, Barshack I, et al. Analyses of P53 Expression Pattern and BRCA Mutations in Patients With Double Primary Breast and Ovarian Cancer. Int J Gynecol Cancer (2004) 14:251–8. doi: 10.1111/j.1048-891X.2004.014208.x
70. Bordeleau L, Lipscombe L, Lubinski J, Ghadirian P, Foulkes WDN, Neuhausen S, et al. Diabetes and Breast Cancer Among Women With BRCA1 and BRCA2 Mutations. Cancer (2011) 117:1812–8. doi: 10.1002/cncr.25595
71. Oliverio A, Bruno E, Colombo M, Paradiso A, Tommasi S, Daniele A, et al. BRCA1/2 Variants and Metabolic Factors: Results From a Cohort of Italian Female Carriers. Cancers (Basel) (2020) 12:3584. doi: 10.3390/cancers12123584
72. Moreau K, Dizin E, Ray H, Luquain C, Lefai E, Foufelle F, et al. BRCA1 Affects Lipid Synthesis Through its Interaction With Acetyl-Coa Carboxylase. J Biol Chem (2006) 281:3172–81. doi: 10.1074/jbc.M504652200
73. Koobotse M, Holly J, Perks C. Elucidating the Novel BRCA1 Function as a Non-Genomic Metabolic Restraint in ER-Positive Breast Cancer Cell Lines. Oncotarget (2018) 9:33562–76. doi: 10.18632/oncotarget.26093
74. Koobotse MO, Schmidt D, Holly JMP, Perks CM. Glucose Concentration in Cell Culture Medium Influences the BRCA1-Mediated Regulation of the Lipogenic Action of IGF-I in Breast Cancer Cells. Int J Mol Sci (2020) 21:8674. doi: 10.3390/ijms21228674
75. Fan S, Ma YX, Wang C, Yuan R, Meng Q, Wang J-A, et al. Role of Direct Interaction in BRCA1 Inhibition of Estrogen Receptor Activity. Oncogene (2001) 20:77–87. doi: 10.1038/sj.onc.1204073
76. Xu J, Fan S, Rosen EM. Regulation of the Estrogen-Inducible Gene Expression Profile by the Breast Cancer Susceptibility Gene BRCA1. Endocrinology (2005) 146:2031–47. doi: 10.1210/en.2004-0409
77. Rosen EM, Fan S, Pestell RG, Goldberg ID. BRCA1 in Hormone-Responsive Cancers. Trends Endocrinol Metab (2003) 14:378–85. doi: 10.1016/j.tem.2003.08.001
78. Widschwendter M, Rosenthal AN, Philpott S, Rizzuto I, Fraser L, Hayward J, et al. The Sex Hormone System in Carriers of BRCA1/2 Mutations: A Case-Control Study. Lancet Oncol (2013) 14:1226–32. doi: 10.1016/S1470-2045(13)70448-0
79. Daum H, Peretz T, Laufer N. BRCA Mutations and Reproduction. Fertil Steril (2018) 109:33–8. doi: 10.1016/j.fertnstert.2017.12.004
80. Kwiatkowski F, Arbre M, Bidet Y, Laquet C, Uhrhammer N, Bignon YJ. BRCA Mutations Increase Fertility in Families at Hereditary Breast/Ovarian Cancer Risk. PloS One (2015) 10:e0127363. doi: 10.1371/journal.pone.0127363
81. Smith KR, Hanson HA, Mineau GP, Buys SS. Effects of BRCA1 and BRCA2 Mutations on Female Fertility. Proc Biol Sci (2012) 279:1389–95. doi: 10.1098/rspb.2011.1697
82. Oktay K, Kim JY, Barad D, Babayev SN. Association of BRCA1 Mutations With Occult Primary Ovarian Insufficiency: A Possible Explanation for the Link Between Infertility and Breast/Ovarian Cancer Risks. J Clin Oncol (2010) 28:240–4. doi: 10.1200/JCO.2009.24.2057
83. Shapira M, Raanani H, Feldman B, Srebnik N, Dereck-Haim S, Manela D, et al. BRCA Mutation Carriers Show Normal Ovarian Response in In Vitro Fertilization Cycles. Fertil Steril (2015) 104:1162–7. doi: 10.1016/j.fertnstert.2015.07.1162
84. Antonova L, Mueller CR. Hydrocortisone Down-Regulates the Tumor Suppressor Gene BRCA1 in Mammary Cells: A Possible Molecular Link Between Stress and Breast Cancer. Genes Chrom Cancer (2008) 47:341–52. doi: 10.1002/gcc.20538
Keywords: BRCA1, tumor suppressors, p53, insulin-like growth factor-1 (IGF1), estrogen receptor, transcription
Citation: Werner H (2022) BRCA1: An Endocrine and Metabolic Regulator. Front. Endocrinol. 13:844575. doi: 10.3389/fendo.2022.844575
Received: 28 December 2021; Accepted: 07 March 2022;
Published: 31 March 2022.
Edited by:
Claire Perks, University of Bristol, United KingdomReviewed by:
Patricia Alejandra Pennisi, Centro de Investigaciones Endocrinológicas “Dr. César Bergadá” (CEDIE)(CONICET), ArgentinaKatia Scotlandi, Rizzoli Orthopedic Institute (IRCCS), Italy
Copyright © 2022 Werner. This is an open-access article distributed under the terms of the Creative Commons Attribution License (CC BY). The use, distribution or reproduction in other forums is permitted, provided the original author(s) and the copyright owner(s) are credited and that the original publication in this journal is cited, in accordance with accepted academic practice. No use, distribution or reproduction is permitted which does not comply with these terms.
*Correspondence: Haim Werner, hwerner@post.tau.ac.il