- 1Department of Obstetrics, Shanghai Key Laboratory of Maternal Fetal Medicine, Shanghai First Maternity and Infant Hospital, School of Medicine, Tongji University, Shanghai, China
- 2Department of Reproductive Immunology, Shanghai Key Laboratory of Maternal Fetal Medicine, Shanghai First Maternity and Infant Hospital, School of Medicine, Tongji University, Shanghai, China
- 3Department of Nursing, Shanghai Key Laboratory of Maternal Fetal Medicine, Shanghai First Maternity and Infant Hospital, School of Medicine, Tongji University, Shanghai, China
Objective: We aimed to determine the association between maternal characteristics and isolated maternal hypothyroxinemia (IMH).
Methods: Pregnancies registered at Shanghai First Maternity and Infant Hospital between January 2014 and September 2020 were included in this cross-sectional study. IMH was defined as free thyroxine (FT4) levels below the 10th percentile with TSH within the normal reference range. Multivariate logistic regression models were used to identify potential risk factors for IMH, including demographic information, anthropometric measurements and nutritional status.
Results: A total of 54586 singleton pregnancies were included, involving 6084 women with IMH and 48502 euthyroid women. Multivariate logistic regression analyses showed that the variables for women with ages ≥35 (adjusted OR = 1.30, 95% CI:1.20–1.40), non-local residence (adjusted OR = 1.16, 95% CI:1.09–1.23), multiparas (adjusted OR = 1.11, 95% CI:1.03–1.21), pre-pregnancy overweight (adjusted OR = 1.37, 95% CI:1.27–1.49) or obesity (adjusted OR = 1.35, 95% CI:1.18–1.54), and iron deficiency (adjusted OR = 1.27, 95% CI:1.20–1.35) were independent risk factors for IMH in the overall study population, which were identical to those in the first trimester subgroup.
Conclusions: Maternal characteristics were associated with the onset of IMH. Maternal age, residence of origin, parity, pre-pregnancy body mass index (BMI) and iron status should be comprehensively considered to evaluate the risk of IMH, according to which obstetricians could determine an optimal assessment time for thyroid function.
Introduction
Thyroid hormone (TH) is crucial for normal fetal development and increases by approximately 50% during pregnancy (1), guaranteeing the proliferation and migration, as well as other developmental processes of fetal brain cells (2). It has been proven in animal studies that the lack of TH at an early stage of pregnancy leads to severe brain defects (3, 4). Isolated maternal hypothyroxinemia (IMH) is a kind of mild thyroid insufficiency characterized by low free thyroxine (FT4) but normal thyroid-stimulating hormone (TSH) levels in pregnant women. Some epidemiological studies have shown that IMH diagnosed in the first trimester is not only associated with impaired mental and motor neurodevelopment, such as lower IQ levels (5) and slower response speeds (6), but also higher risks for psychiatric disorders of the offspring (7–9). In addition to the effect on fetal neurodevelopment, IMH may also increase the risk of obstetric complications, such as preterm delivery, hypertension disorders, and gestational diabetes (10).
The etiology of IMH is not fully understood. Iodine deficiency is theoretically recognized as a major factor because the insufficient supply of iodine would probably lead to a prior production of T3 to T4 (11). However, some studies from iodine-deficient areas have shown conflicting results that there was no difference in urinary iodine concentration (UIC) between women with IMH and euthyroid pregnant women (12, 13). Furthermore, iron deficiency (ID) has recently been raised as another potential risk factor for IMH because women with ID had a significantly higher prevalence of hypothyroxinemia than those without in two independent cohorts (14, 15). Other factors, including obesity, environmental pollution, and angiogenic factors, were also found to be associated with lower FT4 levels, but their role in IMH remains uncertain (16–18).
It is believed that the detrimental effect of IMH on fetal neurodevelopment can be prevented or rescued by timely levothyroxine administration (3), and the ideal treatment should be ahead of the sixth week of gestation, at which time early neurogenesis starts. Currently, the first obstetric visit in China is commonly set at the late stage of the first trimester, which is too late for optimal treatment to ensure normal fetal brain development. Therefore, an early identification of IMH risk factors based on maternal characteristics seems to be preferable and reliable. However, there were limited studies comprehensively estimated the potential risks from this perspective.
The main aim of this study was to investigate the association between maternal characteristics and the risk of isolated hypothyroxinemia in a large obstetric care center in Shanghai, where iodine status was defined as adequate (19, 20).
Material and Methods
Study Population
This is a cross-sectional study based on electronic medical registers. A total of 91812 pregnant women who underwent antenatal health care and planned to deliver at Shanghai First Maternity and Infant Hospital between January 2014 and September 2020 were included, from which 2516 were excluded due to a fault or lack of key variables, and 9670 for not receiving thyroid hormone tests. The exclusion criteria for this study were as follows: 1) women with a twin pregnancy; 2) women with a history of thyroid disease, including overt or sub-clinical hyperthyroidism, hypothyroidism, and thyroid cancer; 3) women who used thyroid interfering medication, including levothyroxine, glucocorticoids, and rifampicin; 4) women who got pregnant using assisted reproductive technology (ART). After the exclusion of women diagnosed with overt hypothyroidism (n=875), subclinical hypothyroidism (n=2349), overt hyperthyroidism (n=860), subclinical hyperthyroidism (n=884), hyperthyroxinemia (n=972) and isolated TPOAb positivity (n=5760) during pregnancy, we chose women with isolated maternal hypothyroxinemia (n=6084) and unaffected pregnancies (48502) as the final study population (Figure 1). The study was approved by the Ethics Committee of the Shanghai First Maternity and Infant Hospital.
Maternal Characteristics and Medical History
Demographic information, including ethnicity, age, parity, BMI before pregnancy, medical history, and medication usage, was obtained through questionnaires. Information on obstetric outcomes, date of birth, and fetal sex was obtained from discharge records. Anthropometric measurements, including height and weight, were performed when participants were recruited and were used to calculate the pre-pregnancy BMI.
Laboratory Measurements
Maternal venous serum samples were obtained for the measurements of FT4, TSH, TPOAb, serum ferritin (SF), and 25-hydroxyvitamin D. FT4, TSH and TPOAb were measured using a chemiluminescence immunoassay (ADVIA Centaur, Siemens Healthcare Diagnostics). The minimum detectable concentrations of FT4, TSH and TPOAb were 1.3 pmol/L, 0.005 mIU/L, and 28 U/mL, respectively. The intra-assay coefficients of variation were 3.3%, 2.2%, and 2.5% at FT4 concentrations of 9.3, 19.0, and 38.8 pmol/L, respectively; 2.3%, 2.9%, and 4.5% at TSH concentrations of 0.60, 30.3, and 109 mIU/L, respectively; and 6.8% and 1.3% at TPOAb concentrations of 70.8 and 458.5 U/mL, respectively. SF measurements were carried out using a UniCel DxI 800 immunology analyzer and kits (Beckman Coulter, California, America). The intra-assay coefficients of variation were 2.6%, 3.6%, and 3.9% at ferritin concentrations of 37.2, 118.9, and 311.8 ng/mL, respectively. 25-hydroxyvitamin D levels were analyzed using a chemiluminescence particle immunoassay (Architect Alinity, Longford, Ireland). The intra-assay coefficients of variation were 2.2%, 2.1%, and 1.9% at 25-hydroxyvitamin D concentrations of 20, 39.7, and 75.6 ng/mL respectively.
Definition
We built a population-based, trimester-specific reference range for FT4 and TSH levels according to the guideline (21) (Table S1). Isolated maternal hypothyroxinemia was defined as FT4 levels below the 10th, the 5th and the 2.5th percentile respectively based on previous studies and the ATA guideline (22), with TSH levels within the reference range in the present study. Based on the manufacturer-defined cutoff, iron deficiency was defined as serum ferritin lower than 12 µg/L, and TPOAb > 60 IU/ml was considered positive. Women over 35 years old was defined as advanced age. The non-local residence was defined as participants who were not born or settled permanently in Shanghai. Women who did not belong to the Han population were defined as ethnic minorities. BMI was classified into four groups according to the reference range for Asian populations: <18.5 kg/m2 (underweight), 18.5 to <23 kg/m2 (normal), 23 to <27.5 kg/m2 (overweight), and ≥ 27.5 kg/m2 (23). All the covariates were further described in Table S2.
Statistical Analyses
Histogram plotting was used to evaluate the data distribution. Continuous variables with a normal distribution are presented as the mean ± SD (Standard Deviation) and were compared between two groups using Student t-test. Skewed distributed variables are presented as medians (interquartile ranges) and were analyzed with the Wilcoxon test. Categorical variables are presented as cases (percentages), and the chi-square test was used for comparisons between two groups. The primary analysis was performed using the 10th percentile definition to include more subjects and find their similar characteristics. Multivariate logistic regression models were used to identify potential risk factors for IMH, and Ln-transformed TSH and average 25-OH-D concentration were included as continuous variables in the model. The 2.5th and 5th percentile cut-offs were used respectively in this analysis since these cut-offs were also widely used. All statistical analyses were conducted in SAS 9.4 (SAS Institute Inc., Cary, NC, USA). A P value < 0.05 was considered statistically significant.
Results
The study population had a mean (SD) age of 30.21 (3.75) years and BMI of 21.08 (2.74) kg/m2. The prevalence of IMH was 10.21%, 10.58%, and 9.9% for the first, second and third trimesters, respectively.
Compared with euthyroid women, women with IMH were more likely to have advanced age (17.49% vs. 12.61%, P < 0.001), prepregnant overweight or obesity (18.56% vs. 14.20%, P < 0.001), be from a non-local population (72.47 vs. 68.39%, P < 0.001), be multiparas (31.10 vs. 24.79%, P <0.001), have higher TSH levels (1.50 vs. 1.27, P<0.001), and have decreased serum ferritin levels (23.25 vs. 26.08, P<0.001) (Table 2). There was no difference in ethnicity, fetal sex or 25-hydroxyvitamin D levels between the two groups (Table 1).
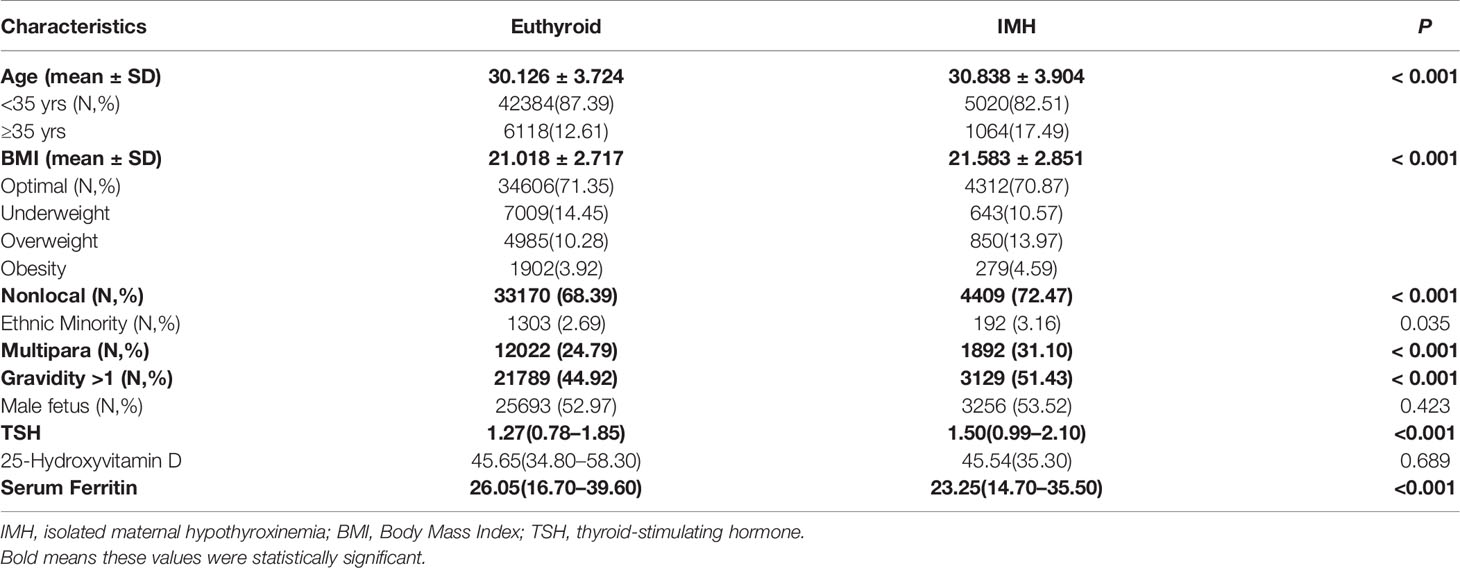
Table 1 Univariate analysis of maternal characteristics and metabolic parameters between euthyroid women and women with isolated hypothyroxinemia.
Multivariate logistic regression analysis showed that variables for advanced age (OR = 1.30, 95% CI:1.20–1.40), being a non-local resident (OR = 1.16, 95% CI:1.09–1.23), being multiparas (OR = 1.11, 95% CI:1.03–1.21), having prepregnant overweight (OR = 1.37, 95% CI:1.27–1.49) or obesity (OR = 1.35, 95% CI:1.18–1.54), and having iron deficiency (OR = 1.27, 95% CI:1.20–1.35) were independent risk factors for IMH when the cut-off was defined as 10th percentile. Meanwhile advanced age (OR = 1.34, 95% CI:1.16–1.44), being a non-local resident (OR = 1.22, 95% CI:1.12–1.33), being multiparas (OR = 1.15, 95% CI:1.03–1.29), having prepregnant overweight (OR = 1.47, 95% CI:1.32–1.63), and having iron deficiency (OR = 1.29, 95% CI:1.20–1.40) were independent risk factors for IMH when defined as FT4 lower than 10th percentile. And for IMH set as the lowest 2.5th percentile FT4 levels, advanced age (OR = 1.35, 95% CI:1.21–1.50), being ethnic minority(OR = 1.43 95% CI:1.09–1.87), being a non-local resident (OR = 1.21, 95% CI:1.07–1.36), being multiparas (OR = 1.26, 95% CI:1.08–1.48), having prepregnant overweight (OR = 1.55, 95% CI:1.34–1.79), and having iron deficiency (OR = 1.29, 95% CI:1.16–1.44) were independent risk factors. Ethnicity, fetal sex, and the level of 25-Hydroxyvitamin D were not associated with hypothyroxinemia (Table 2).
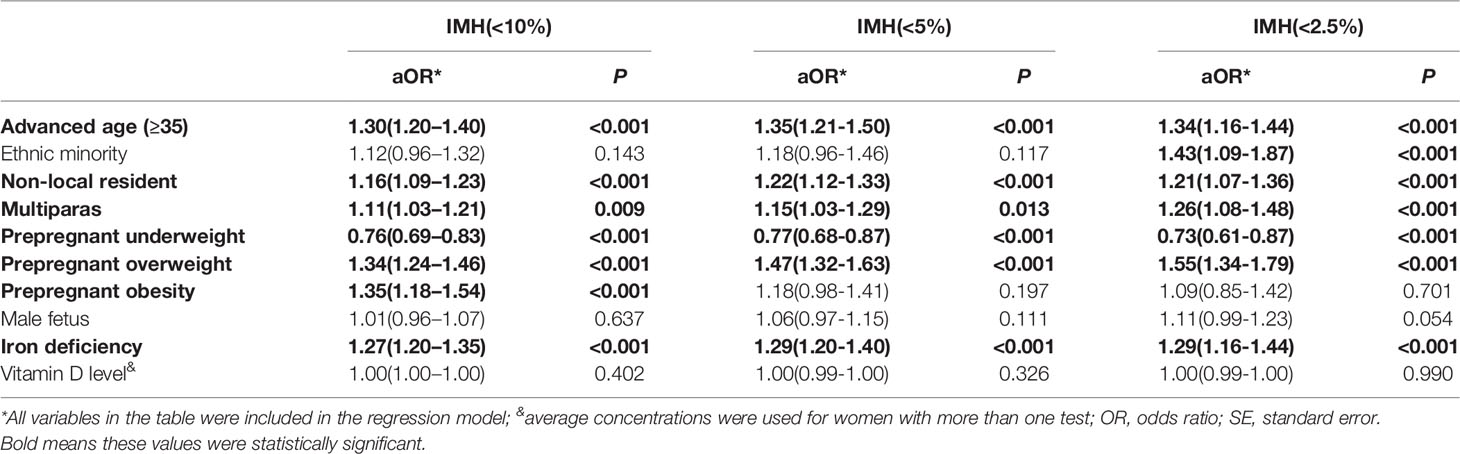
Table 2 Multivariable logistic regression analysis to demonstrate the association between maternal characteristics and hypothyroxinemia.
We performed stratified analysis by the assessment time of thyroid function to further determine risk factors for IMH in different trimesters (Table 3). For the first trimester, the variables for increased age (adjusted OR = 1.15, 95% CI:1.04–1.27), nonlocal residence (adjusted OR = 1.14, 95% CI:1.06–1.24), multiparas (adjusted OR = 1.26, 95% CI:1.13–1.40), prepregnant overweight (adjusted OR = 1.28, 95% CI:1.15–1.42), prepregnant obesity (adjusted OR = 1.21, 95% CI:1.02–1.45), and iron deficiency (adjusted OR = 1.30, 95% CI:1.21–1.40) were associated with a higher risk for IMH, which was similar to the overall analysis. In the second trimester, except for minority participants (adjusted OR= 1.26, 95% CI: 1.01–1.57), the estimates were in line with those in the first trimester; however, most of the estimates were insignificant in the third trimester.
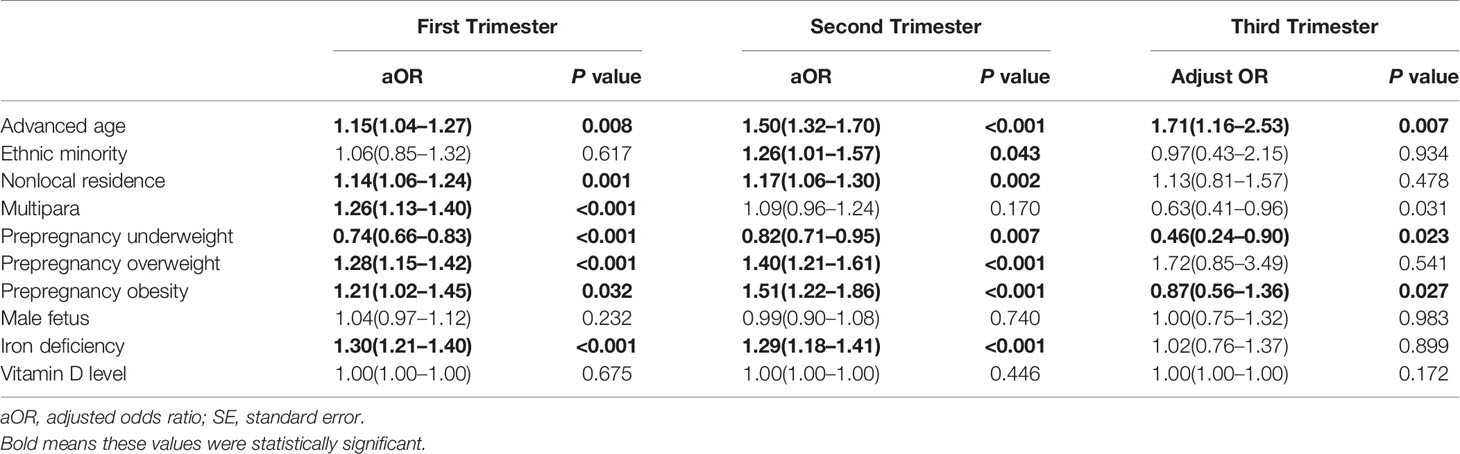
Table 3 Association between maternal risk factors and IMH in different trimesters using multivariable logistic regression analysis.
Discussion
In the present study, we used the information on maternal characteristics, which were not restricted to the course of pregnancy, to explore the risk factors for IMH. We found that the variables for advanced maternal age, non-local residence, multiparas, pre-pregnancy overweight or obesity, and ID were independent risk factors for IMH.
Fetal thyroid does not start to exert secretion function until 16–20 weeks of gestation, before which time all the hormones needed rely on maternal transmission. The insufficient of thyroid hormone during this crucial period may lead to long-lasting and irreversible damage to fetal brain morphology and neurocognitive development in offspring (5–10). Prompt treatment with thyroxine has been proven effective to rescue the damage from maternal hypothyroxinemia in the early days of pregnancy in animal studies (3), but levothyroxine treatment initiated at 8-20 weeks of gestation did not reduce the intellectual disability in offspring of mild maternal TH insufficiency in clinical studies (24), which means the identification of IMH as early as possible is necessary and urgent.
In previous studies, Etemadi et al. (12) observed a 1.6-fold increased risk of isolated hypothyroxinemia in pregnant women older than 30 years, 1.72-fold increased risk for multiple prior pregnancies, 1.57-fold increased risk in women in rural areas, and 3.34-fold increased risk in capital provinces in Iran. Yu and colleagues found that increased BMI was associated with a higher prevalence of mild and severe hypothyroxinemia in pregnant women, and advanced age was associated with a greater risk of mild but not severe hypothyroxinemia in nonpregnant women of reproductive age (25). In Tengs’ study (15), maternal age and increased BMI were risk factors for hypothyroxinemia in the first and second trimesters, but the inclusive predictors were no longer significant in the third trimester. However, maternal factors included in these studies were not comprehensive mainly because the screening value for IMH was not their core research aim.
Increased age and parity were significantly related to lower thyroxine levels in our study, which was consistent with Etemadis’ findings (12). The underlying mechanism has not yet been completely elucidated, but an age-dependent decrease in serum T4 concentration has been reported in a large-population study (26). This decrease may result from changes in thyroid gland function or peripheral TH metabolism (27), but there are also conjectures that decreased iodine storage is the major cause of hypothyroxinemia in older women and multipara women (12, 28). BMI has been found to be inversely related to FT4 levels in pregnant women in previous studies (29, 30), and an obvious increase in IMH prevalence was observed when the BMI crossed 24 kg/m2 during early pregnancy in Hans’ research (16). As an increase in T3 could be seen in association with low T4 levels, some researchers speculated that more T4 was stimulated to convert to T3 by fat-derived leptin (31). It is worth noting that the effect of overweight and obese was also observed in non-pregnant groups, including males and females. Pregnant women from other regions of China had a slightly higher chance of being diagnosed with IMH in our research, which may be a result of long-term iodized salt promotion because Shanghai was classified as an iodine-sufficient/excessive region in the latest two national surveys (19, 20).
We also determined the association between nutritional status, including serum ferritin levels and vitamin D levels, and the risk of IMH, finding that ID was 1.27 times more likely to be diagnosed as IMH in our study. ID has been reported to be associated with lower T4 levels or a higher prevalence of IMH in pregnant women in other studies (14, 15, 32). Iron deficiency is known to have multiple effects on the thyroid axis and importantly affects thyroid hormone synthesis by reducing the activity of heme-dependent thyroid peroxidase (33). There was no difference in serum vitamin D levels between the IMH and euthyroid groups in our study. It has been reported that vitamin D levels have inverse relationships with thyroglobulin antibodies, and therefore may be an indicator of Hashimoto’s thyroiditis. Vitamin D is thought to affect thyroid function via the immune system and its role in the infection process, and there is still a lack of evidence supporting that vitamin D has a direct effect on thyroid hormone metabolism (34).
There are several limitations in our study. First, we did not elucidate the potential impact of iodine deficiency on our results due to a lack of urinary iodine concentration data. However, as previous studies showed, the causal effects of UIC on isolated hypothyroxinemia are still controversial, amid which several studies observed no direct association between UIC levels and the prevalence of IMH, neither other researcher found alterations in maternal thyroxine levels after iodine supplementation in areas with mild to moderate iodine deficiency. Furthermore, given that iodized salt use has been mandatory in China for decades, other potent forms of iodine supplementation would be hard to implement even though a slight influence was found. Second, we measured FT4, rather the FT4 index, to assess maternal T4 when defining IMH, which might lead to marginal biases in reflecting the real prevalence of IMH in the population. However, we used method-specific and trimester-specific reference ranges, as recommended by the American Thyroid Association (21), to control the biases as much as possible. Third, we didn’t describe the relation between the maternal characteristics and pregnancy outcomes via IMH, which would be more convictive for their screening potentials, but limited to the nature of our research method it was hard to determine the causality among the three variables.
Conclusion
Our study suggested that maternal characteristics were associated with the onset of IMH, including age, area of residence, parity, pre-pregnancy BMI and iron status. Obstetricians should comprehensively evaluate the risk of IMH and then determine an optimal assessment time of thyroid function.
Data Availability Statement
The original contributions presented in the study are included in the article/Supplementary Material. Further inquiries can be directed to the corresponding author.
Author Contributions
YL researched data and wrote the manuscript. SH, XL, NG, and GL researched data. QD oversaw data collection and analysis, contributed to writing of the manuscript, and reviewed and edited the manuscript. All authors were involved in writing of the paper and had final approval of the submitted and published versions.
Funding
This work was supported by the research grants from the Health and Family Planning Committee of Pudong New Area (approval number: PW2019D-9) and the Shanghai Science and Technology Commission (approval number: 20Y11907900).
Conflict of Interest
The authors declare that the research was conducted in the absence of any commercial or financial relationships that could be construed as a potential conflict of interest.
Publisher’s Note
All claims expressed in this article are solely those of the authors and do not necessarily represent those of their affiliated organizations, or those of the publisher, the editors and the reviewers. Any product that may be evaluated in this article, or claim that may be made by its manufacturer, is not guaranteed or endorsed by the publisher.
Supplementary Material
The Supplementary Material for this article can be found online at: https://www.frontiersin.org/articles/10.3389/fendo.2022.843324/full#supplementary-material
References
1. Glinoer D. The Regulation of Thyroid Function in Pregnancy: Pathways of Endocrine Adaptation From Physiology to Pathology. Endocr Rev (1997) 18(3):404–33. doi: 10.1210/edrv.18.3.0300
2. Prezioso G, Giannini C, Chiarelli F. Effect of Thyroid Hormones on Neurons and Neurodevelopment. Horm Res Paediatr (2018) 90(2):73–81. doi: 10.1159/000492129
3. Ausó E, Lavado-Autric R, Cuevas E, Del Rey FE, Morreale De Escobar G, Berbel P. A Moderate and Transient Deficiency of Maternal Thyroid Function at the Beginning of Fetal Neocorticogenesis Alters Neuronal Migration. Endocrinology (2004) 145(9):4037–47. doi: 10.1210/en.2004-0274
4. Calvo R, Obregón MJ, Ruiz de Oña C, Escobar del Rey F, Morreale de Escobar G. Congenital Hypothyroidism, as Studied in Rats. Crucial Role of Maternal Thyroxine But Not of 3,5,3’-Triiodothyronine in the Protection of the Fetal Brain. J Clin Invest (1990) 86(3):889–99. doi: 10.1172/JCI114790
5. Ghassabian A, El Marroun H, Peeters RP, Jaddoe VW, Hofman A, Verhulst FC, et al. Downstream Effects of Maternal Hypothyroxinemia in Early Pregnancy: Nonverbal IQ and Brain Morphology in School-Age Children. J Clin Endocrinol Metab (2014) 99(7):2383–90. doi: 10.1210/jc.2013-4281
6. Finken MJ, van Eijsden M, Loomans EM, Vrijkotte TG, Rotteveel J. Maternal Hypothyroxinemia in Early Pregnancy Predicts Reduced Performance in Reaction Time Tests in 5- to 6-Year-Old Offspring. J Clin Endocrinol Metab (2013) 98(4):1417–26. doi: 10.1210/jc.2012-3389
7. Modesto T, Tiemeier H, Peeters RP, Jaddoe VW, Hofman A, Verhulst FC, et al. Maternal Mild Thyroid Hormone Insufficiency in Early Pregnancy and Attention-Deficit/Hyperactivity Disorder Symptoms in Children. JAMA Pediatr (2015) 169(9):838–45. doi: 10.1001/jamapediatrics.2015.0498
8. Román GC, Ghassabian A, Bongers-Schokking JJ, Jaddoe VW, Hofman A, de Rijke YB, et al. Association of Gestational Maternal Hypothyroxinemia and Increased Autism Risk. Ann Neurol (2013) 74(5):733–42. doi: 10.1002/ana.23976
9. Gyllenberg D, Sourander A, Surcel HM, Hinkka-Yli-Salomäki S, McKeague IW, Brown AS. Hypothyroxinemia During Gestation and Offspring Schizophrenia in a National Birth Cohort. Biol Psychiatry (2016) 79(12):962–70. doi: 10.1016/j.biopsych.2015.06.014
10. Cleary-Goldman J, Malone FD, Lambert-Messerlian G, Sullivan L, Canick J, Porter TF, et al. Maternal Thyroid Hypofunction and Pregnancy Outcome. Obstet Gynecol (2008) 112(1):85–92. doi: 10.1097/AOG.0b013e3181788dd7
11. Vermiglio F, Lo Presti VP, Scaffidi Argentina G, Finocchiaro MD, Gullo D, Squatrito S, et al. Maternal Hypothyroxinaemia During the First Half of Gestation in an Iodine Deficient Area With Endemic Cretinism and Related Disorders. Clin Endocrinol (Oxf) (1995) 42(4):409–15. doi: 10.1111/j.1365-2265.1995.tb02650.x
12. Etemadi A, Amouzegar A, Mehran L, Tohidi M, Azizi F, Moradi K, et al. Isolated Hypothyroxinemia in Iranian Pregnant Women, the Role of Iodine Deficiency: A Population-Based Cross-Sectional Study. Thyroid (2020) 30(2):262–9. doi: 10.1089/thy.2019.0047
13. Amouzegar A, Khazan M, Hedayati M, Azizi F. An Assessment of the Iodine Status and the Correlation Between Iodine Nutrition and Thyroid Function During Pregnancy in an Iodine Sufficient Area. Eur J Clin Nutr (2014) 68(3):397–400. doi: 10.1038/ejcn.2013.273
14. Maldonado-Araque C, Valdés S, Lago-Sampedro A, Lillo-Muñoz JA, Garcia-Fuentes E, Perez-Valero V, et al. Iron Deficiency Is Associated With Hypothyroxinemia and Hypotriiodothyroninemia in the Spanish General Adult Population: Di@bet.es Study. Sci Rep (2018) 8(1):6571. doi: 10.1038/s41598-018-24352-9
15. Teng X, Shan Z, Li C, Yu X, Mao J, Wang W, et al. Iron Deficiency May Predict Greater Risk for Hypothyroxinemia: A Retrospective Cohort Study of Pregnant Women in China. Thyroid (2018) 28(8):968–75. doi: 10.1089/thy.2017.0491
16. Han C, Li C, Mao J, Wang W, Xie X, Zhou W, et al. High Body Mass Index Is an Indicator of Maternal Hypothyroidism, Hypothyroxinemia, and Thyroid-Peroxidase Antibody Positivity During Early Pregnancy. BioMed Res Int (2015) 2015:351831. doi: 10.1155/2015/351831
17. Zhao Y, Cao Z, Li H, Su X, Yang Y, Liu C, et al. Air Pollution Exposure in Association With Maternal Thyroid Function During Early Pregnancy. J Hazard Mater (2019) 367:188–93. doi: 10.1016/j.jhazmat.2018.12.078
18. Korevaar TI, Steegers EA, de Rijke YB, Visser WE, Jaddoe VW, Visser TJ, et al. Placental Angiogenic Factors Are Associated With Maternal Thyroid Function and Modify hCG-Mediated FT4 Stimulation. J Clin Endocrinol Metab (2015) 100(10):E1328–34. doi: 10.1210/jc.2015-2553
19. Li Y, Teng D, Ba J, Chen B, Du J, He L, et al. Efficacy and Safety of Long-Term Universal Salt Iodization on Thyroid Disorders: Epidemiological Evidence From 31 Provinces of Mainland China. Thyroid (2020) 30(4):568–79. doi: 10.1089/thy.2019.0067
20. Shan Z, Chen L, Lian X, Liu C, Shi B, Shi L, et al. Iodine Status and Prevalence of Thyroid Disorders After Introduction of Mandatory Universal Salt Iodization for 16 Years in China: A Cross-Sectional Study in 10 Cities. Thyroid (2016) 26(8):1125–30. doi: 10.1089/thy.2015.0613
21. Thyroid Disease in Pregnancy: ACOG Practice Bulletin, Number 223. Obstet Gynecol (2020) 135(6):e261–74. doi: 10.1097/AOG.0000000000003893
22. Alexander EK, Pearce EN, Brent GA, Brown RS, Chen H, Dosiou C, et al. Guidelines of the American Thyroid Association for the Diagnosis and Management of Thyroid Disease During Pregnancy and the Postpartum. Thyroid (2017) 27(3):315–89. doi: 10.1089/thy.2016.0457
23. Appropriate Body-Mass Index for Asian Populations and Its Implications for Policy and Intervention Strategies. Lancet (2004) 363(9403):157–63. doi: 10.1016/S0140-6736(03)15268-3
24. Thompson W, Russell G, Baragwanath G, Matthews J, Vaidya B, Thompson-Coon J. Maternal Thyroid Hormone Insufficiency During Pregnancy and Risk of Neurodevelopmental Disorders in Offspring: A Systematic Review and Meta-Analysis. Clin Endocrinol (Oxf) (2018) 88(4):575–84. doi: 10.1111/cen.13550
25. Yu X, Shan Z, Li C, Mao J, Wang W, Xie X, et al. Iron Deficiency, an Independent Risk Factor for Isolated Hypothyroxinemia in Pregnant and Nonpregnant Women of Childbearing Age in China. J Clin Endocrinol Metab (2015) 100(4):1594–601. doi: 10.1210/jc.2014-3887
26. Hollowell JG, Staehling NW, Flanders WD, Hannon WH, Gunter EW, Spencer CA, et al. Serum TSH, T(4), and Thyroid Antibodies in the United States Population (1988 to 1994): National Health and Nutrition Examination Survey (NHANES III). J Clin Endocrinol Metab (2002) 87(2):489–99. doi: 10.1210/jcem.87.2.8182
27. Franceschi C, Ostan R, Mariotti S, Monti D, Vitale G. The Aging Thyroid: A Reappraisal Within the Geroscience Integrated Perspective. Endocr Rev (2019) 40(5):1250–70. doi: 10.1210/er.2018-00170
28. Furnica RM, Lazarus JH, Gruson D, Daumerie C. Update on a New Controversy in Endocrinology: Isolated Maternal Hypothyroxinemia. J Endocrinol Invest (2015) 38(2):117–23. doi: 10.1007/s40618-014-0203-5
29. Knight BA, Shields BM, Hattersley AT, Vaidya B. Maternal Hypothyroxinaemia in Pregnancy Is Associated With Obesity and Adverse Maternal Metabolic Parameters. Eur J Endocrinol (2016) 174(1):51–7. doi: 10.1530/EJE-15-0866
30. Gowachirapant S, Melse-Boonstra A, Winichagoon P, Zimmermann MB. Overweight Increases Risk of First Trimester Hypothyroxinaemia in Iodine-Deficient Pregnant Women. Matern Child Nutr (2014) 10(1):61–71. doi: 10.1111/mcn.12040
31. Zimmermann-Belsing T, Brabant G, Holst JJ, Feldt-Rasmussen U. Circulating Leptin and Thyroid Dysfunction. Eur J Endocrinol (2003) 149(4):257–71. doi: 10.1530/eje.0.1490257
32. Zimmermann MB, Burgi H, Hurrell RF. Iron Deficiency Predicts Poor Maternal Thyroid Status During Pregnancy. J Clin Endocrinol Metab (2007) 92(9):3436–40. doi: 10.1210/jc.2007-1082
33. Zimmermann MB. The Influence of Iron Status on Iodine Utilization and Thyroid Function. Annu Rev Nutr (2006) 26:367–89. doi: 10.1146/annurev.nutr.26.061505.111236
Keywords: isolated maternal hypothyroxinemia, maternal characteristics, risk factor, fetal neurodevelopment, iron deficiency
Citation: Liu Y, Li G, Guo N, Liu X, Huang S and Du Q (2022) Association Between Maternal Characteristics and the Risk of Isolated Maternal Hypothyroxinemia. Front. Endocrinol. 13:843324. doi: 10.3389/fendo.2022.843324
Received: 25 December 2021; Accepted: 08 March 2022;
Published: 12 April 2022.
Edited by:
Marian Elizabeth Ludgate, Cardiff University, United KingdomReviewed by:
Giorgio Radetti, Ospedale di Bolzano, ItalyOnyebuchi Okosieme, Cwm Taf University Health Board, United Kingdom
Copyright © 2022 Liu, Li, Guo, Liu, Huang and Du. This is an open-access article distributed under the terms of the Creative Commons Attribution License (CC BY). The use, distribution or reproduction in other forums is permitted, provided the original author(s) and the copyright owner(s) are credited and that the original publication in this journal is cited, in accordance with accepted academic practice. No use, distribution or reproduction is permitted which does not comply with these terms.
*Correspondence: Qiaoling Du, qldu2004@126.com