- Department of Obstetrics and Gynecology, Shengjing Hospital of China Medical University, Shenyang, China
Estrogen receptors (ERs) regulate multiple complex physiological processes in humans. Abnormal ER signaling may result in various disorders, including reproductive system-related disorders (endometriosis, and breast, ovarian, and prostate cancer), bone-related abnormalities, lung cancer, cardiovascular disease, gastrointestinal disease, urogenital tract disease, neurodegenerative disorders, and cutaneous melanoma. ER alpha (ERα), ER beta (ERβ), and novel G-protein-coupled estrogen receptor 1 (GPER1) have been identified as the most prominent ERs. This review provides an overview of ERα, ERβ, and GPER1, as well as their functions in health and disease. Furthermore, the potential clinical applications and challenges are discussed.
Introduction
Estrogen, a steroid compound, is primarily produced by the ovaries and placenta in females (1). The adrenal cortex of males also produce estrogen. Estrogens play important modulatory roles in physiological and pathophysiological processes (2). They perform their roles mainly by interacting with estrogen receptors (ERs). ERs are ligand-dependent transcription factors that regulate gene transcription through estrogen response elements (EREs), thereby facilitating the normal biological functions of estrogens. However, abnormal ER signaling can result in multiple disorders, including various cancers (2) and gynecological disorders, such as polycystic ovary syndrome and endometriosis (3). Three types of ERs, classical alpha (ERα) and beta (ERβ), as well as non-classical G protein-coupled estrogen receptor 1 (GPER1), are involved in several biological processes. In this review, we systematically illustrate the essential roles of these ERs in mediating various physiological and pathological processes.
Estrogens and ERs
Estrogens
Estrogen, a lipid-soluble steroid hormone, is one of the most important female sex hormones. It is predominantly produced by the ovaries, testes, and adrenal cortex, and performs various crucial physiological functions. Estrogens are divided into two major categories, endogenous and exogenous, based on their origin. Endogenous estrogens are secreted by glands or cells in the body of living organisms, including estrogen in animals and phytoestrogens (such as genistein and zearalenone) in plants (4). In contrast, exogenous estrogens are derived from synthetic estrogens, food, and drugs (5).
To date, four estrogens, estrone (E1), 17β-estradiol (E2), estriol (E3), and estetrol (E4), have been identified in humans (6). The term “estrogen” typically refers to E2 owing to its widespread distribution and active physiological functions in multiple tissues and organ systems (1). E2 plays a major role in developing secondary female sex characteristics, regulates the menstrual cycle, and growth of the endometrial lining from menarche to menopause (6). E2 also demonstrates a high affinity for ERα, ERβ, and GPER1 (7). E1 is a weaker form of estrogen owing to its lower binding affinity for ERα and ERβ and is the predominant estrogen in women undergoing menopause. E3 is the primary estrogen secreted by the placenta during pregnancy. Consequentially, E3 levels are negligible in non-pregnant women or men compared to their manifold increase during pregnancy (6). Compared to E2, E3 has a lower affinity for ERα (14%) and ERβ (21%) (6). Similar to E3, E4 is a natural fetal estrogen that is detectable only during pregnancy. E4 possesses an overall lower affinity for ERs than E2 (6). All four estrogens consist of 18 carbon atoms with similar chemical functions and structures.
The most common exogenous estrogens contain those metabolized and excreted by living organisms into the environment, selective estrogen receptor modulators (SERMs) targeting the ER for the treatment of endocrine disorders (such as diethylstilbestrol and raloxifene), and environmental pollutants generated by industrial and agricultural activities (such as polycyclic aromatic hydrocarbons and phenolic compounds) (8, 9). The affinity of these exogenous estrogens to the native receptors is relatively low (micromolar values), and their structure is different from that of natural estrogens (10).
Estrogens additionally play crucial roles in regulating the cardiovascular system, liver, pancreas, bone, brain, and immune system (2). Estrogens also participate in regulating spermatogenesis and male fertility (11). Notably, the physiological functions regulated by estrogens are mediated by ERs.
Discovery of ERs
Elwood Jensen first established the existence of ERs in 1958 by demonstrating that female reproductive tissue can take up estrogen by binding to proteins (1), suggesting that estrogen-bond receptors can stimulate gene transcription after migrating to the nucleus (12). In 1985, the first human ER, termed ERα, was cloned (13). Subsequently, ERβ (or ERβ1) was discovered by Kuiper et al. in 1996 (14). ERα and ERβ are highly homologous nuclear ERs (nERs), isolated using traditional biochemical approaches.
In 2012, a new G protein-coupled membrane receptor (mER), GPER1, was identified using molecular cloning techniques (15). The history of GPER1 stemmed from 1997, when a 7-transmembrane receptor named GPR30, was identified and cloned (16). Some years later, E2 were demonstrated to induce rapidly cell cascades through GPR30 in breast cancer cells lacking ERs, but expressing GPR30 (17, 18). Interestingly, these rapidly responses were blocked when GPR30 silenced (18). Next, experimental data indicated a direct bind to GPR30 by E2, suggesting that it may work as a membrane-bound ER (19, 20). Subsequently, GPR30 was officially renamed G protein-coupled estrogen receptor 1 (also known as GPER or GPER1) in 2007 (21, 22).
Structure of ERs
The ER protein molecule consists of A/B, C, D, and E/F domains, from amino to carboxyl terminals (Figure 1). ERα and ERβ are encoded by ESR1 on chromosome 6 (6q25.1) (23) and ESR2 on chromosome 14 (14q23.2) (24), respectively. Full-length ERα and ERβ are composed of 595 and 530 amino acids with relative molecular masses of 66 and 59 kDa, respectively. The A/B domain, which is the amino- or N-terminal domain (NTD), participates in the transcriptional activation of target genes and is associated with receptor specificity. The C domain, known as the DNA-binding domain (DBD), is highly conserved and enhances the DNA-binding ability of ERs. Domain D, a hinge region connecting the C and E domains, contains a nuclear localization signal that binds to heat shock proteins and stabilizes the DNA-binding function of the C domain. The E/F domain located in the carboxyl-terminal, also known as the ligand-binding domain (LBD), displays a complex regulatory function. Both ERα and ERβ exhibit their activation function through activation function 1 (AF1) and AF2 located in the NTD land LBD, respectively, and mediate synergistic transcriptional regulation (25). Notably, ERα and ERβ demonstrate 16%, 97%, and 59% similarity in their NTD, DBD, and LBD, respectively (26).
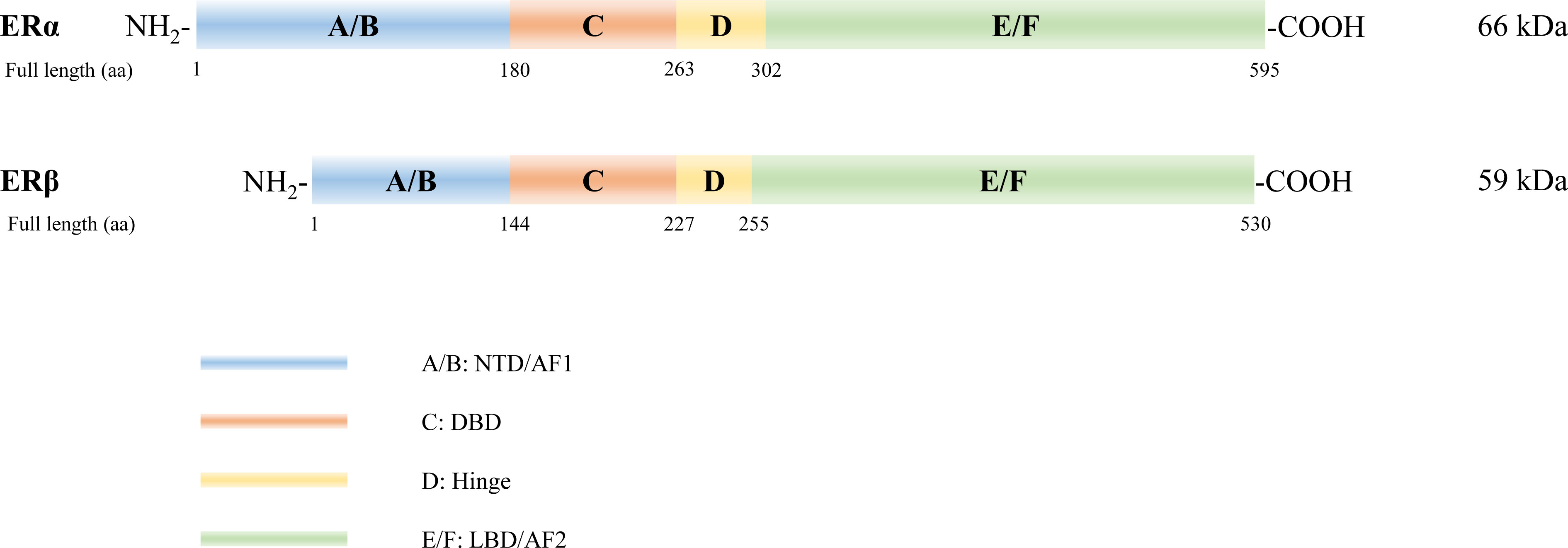
Figure 1 Structure of estrogen receptors (ERs). Six structural and functional domains are highlighted: A, B domain (amino-terminal or NH2-terminal domain [NTD], activation function 1 [AF1]); C domain (DNA binding domain [DBD]); D domain (hinge region connecting the C and E domain); E, F domain (carboxyl- or COOH-terminal, ligand-binding domain [LBD], AF2).
Several isoforms of ERα have been identified arising from alternative gene splicing, including ERα-46 (27) and ERα-36 (28) (Figure 2). ERα-46 lacks 1–173 amino acids, including AF1, and is a dominant-negative inhibitor of ERα activity in osteoblasts (29). ERα-36 lacks AF1 and AF2, and its unique 22 amino-acid sequence replaces the last 138 amino acids (30). Additionally, several ERβ splice isoforms have also been discovered (31, 32) (Figure 3). The full-length and truncated ERβ differ in their LBD. Particularly, ERβ1 (often referred to as ERβ) is a full-length construct that contains 530 amino acids. ERβ2-5 display unique LBD sequences. These differences result in truncation of the LBD and ablation of the AF2 function. Therefore, ERβ1 is the only isoform with ligand binding abilities, whereas the truncated ERβ is incapable of binding to estrogens and other investigated ligands (33).
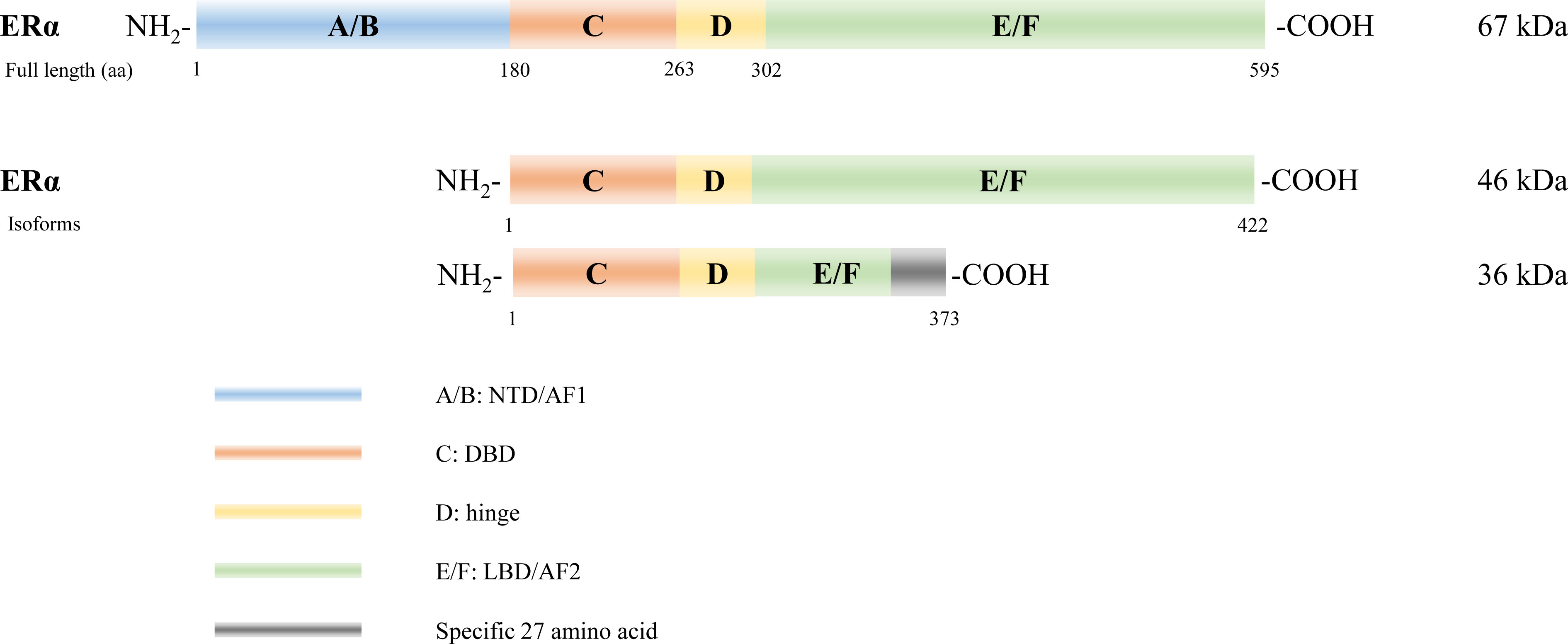
Figure 2 Estrogen receptor alpha (ERα) isoforms. Six structural and functional domains are highlighted: A, B domain (amino- or NH2-terminal domain [NTD], AF1), C domain (DNA binding domain [DBD]), D domain (hinge region connecting the C and E domain), E, F domain (carboxyl- or COOH-terminal, ligand-binding domain [LBD], AF2).
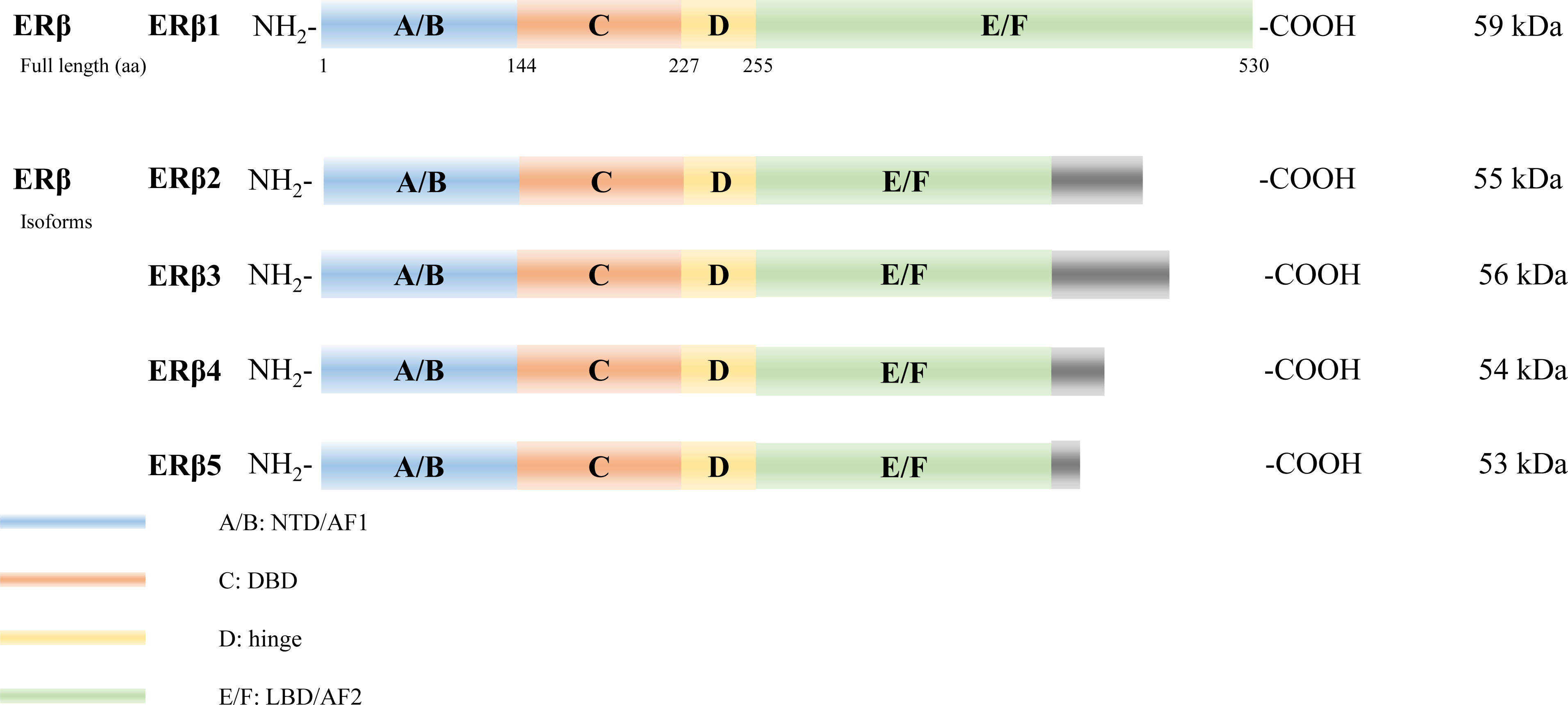
Figure 3 Estrogen receptor beta (ERβ) isoforms. Six structural and functional domains are highlighted: A, B domain (amino- or NH2-terminal domain [NTD], AF1), C domain (DNA binding domain [DBD]), D domain (hinge region connecting the C and E domain), E, F domain (carboxyl- or COOH-terminal, ligand-binding domain [LBD], AF2).
GPER1 is encoded on chromosome 7 (7p22.3) and consists of 375 amino acids, with a molecular mass of 41 kDa (15). As a typical G protein-coupled receptor, GPER1 is distinct from ERα or ERβ and has a structure comprising seven transmembrane α-helices, four extracellular segments, and four cytosolic segments (Figure 4) (34). GPER1 demonstrates a weaker binding affinity to estrogens (35).
ERs expression and signaling pathways
ERα, ERβ, and GPER1 are the three predominant ERs. ERα is primarily expressed in the reproductive tissues (e.g., uterus and ovary), bone, white adipose tissue, kidney, liver, and breast. In contrast, ERβ is expressed in male reproductive organs, central nervous system (CNS), cardiovascular system, lung, immune system, colon, and kidney (26). GPER1 is more widely distributed and expressed in the skeletal muscle, neurons, vascular endothelium, various immune cells, and target effector organs (36). Additionally, GPER1 is reportedly expressed in breast, ovarian, and lung cancer tissues (37).
Estrogens (for example, E2) bind to the traditional ERs (ERα and ERβ) and the novel receptor GPER1, exerting their genomic and non-genomic effects (Figure 5). In the genomic estrogen pathway, E2 binds to the intracellular ERα and ERβ and forms the E2-receptor dimer complex, subsequently entering the nucleus. In the nucleus, the complex binds to estrogen response elements (EREs) or activator protein-1 (Ap1) and specificity protein-1 (Sp1) on the E2-responsive gene promoters, acting as transcription factors that regulate gene transcription (1). Ultimately, estrogen-mediated gene products regulate autophagy, proliferation, apoptosis, survival, differentiation, and vasodilation under normal conditions. However, their function might be disrupted under pathological conditions. Owing to the intracellular location of the ERα and ERβ, the activation typically takes hours or more, leading to the slow “genomic effect.” E2 also mediates the non-genomic signaling pathway by binding to membrane-bound ERα, ERβ, and GPER1, which rapidly activates nuclear transcription factors by regulating the ion channel opening or the activation of related enzymes such as Ca2+ mobilization, phosphatidylinositol 3-kinase (PI3K), and mitogen-activated protein kinase (MAPK). This process does not rely on gene regulation and occurs instantaneously within a time span of seconds to minutes; thus, it is referred to as a rapid “non-genomic effect”.
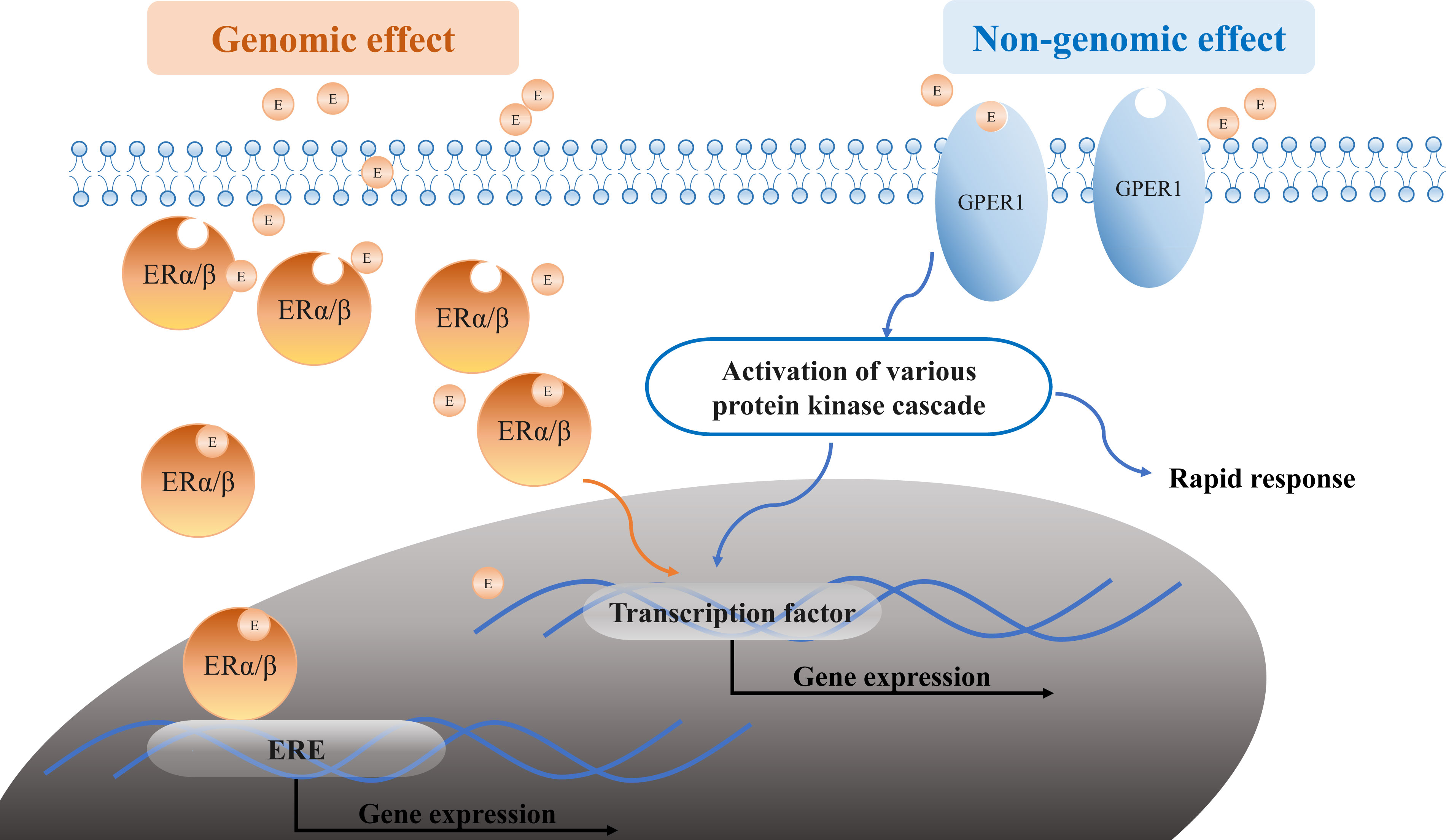
Figure 5 Estrogen signaling pathways. Estrogen or E2 (orange circle E in the graph) binds to the ERα/ERβ and GPER1, exerting its genomic and non-genomic effects. The genomic effect is shown in orange: the E2-receptor complex binds to EREs upon entry into the nucleus. The non-genomic effect is shown in blue: E2 binds to ERs in the membrane-like GPER1 and regulates the expression by modulating the ion channel opening or activation of related enzymes. E, estrogen or E2; ERα, estrogen receptor alpha; ERβ, estrogen receptor beta; GPER1, G protein-coupled estrogen receptor 1; ERE, estrogen response elements; PI3K, phosphatidylinositol 3-kinase; MAPK, mitogen-activated protein kinase.
Estrogens also regulate the immune response. ER signaling regulates hematopoietic progenitor populations during homeostasis and this likely regulates the number and type of immune cells (38). Present of E2 is necessary for hematopoietic stem cells (HSC) self-renewal. ERs are also widely expressed in many cell types involved in innate and adaptive immune responses (39). A published review suggests that ERα and ERβ mRNAs or proteins are expressed by hematopoietic progenitors and mature immune cells (40). In human tissue, the ESR1 is highest expressed in the B cell, and intermediately expressed in CD4+ T cells, CD8+ T cells, NK cells, and plasmacytoid dendritic cell. Monocytes have the lowest levels of ESR1 RNA. Interestingly, ESR1 expression level increase in monocyte-derived dendritic cells, suggesting that ESR1 is induced during dendritic cell differentiation. ESR2 have highest level in B cells and plasmacytoid dendritic cells, and lower levels in other cell types. GPER1 are also expressed in various immune cells like T cell, B cell, mononuclear, macrophage, and neutrophils (41). These evidences mean that estrogens can induce immune responses according to cellular expression of ER and the number of these cells. In addition, estrogens influence the adaptive immune response. Estrogens modulate the differentiation process and the function of neutrophils, macrophages, and natural killer cells (42).
ERs in the manifestation of diseases
ERs in breast cancer
Breast cancer is the most prevalent cancer affecting women worldwide. It is classified into three subtypes according to the expression status of ER, progesterone receptor (PR), and human epidermal growth factor receptor 2 (HER2) (1): luminal A/B (ER+PR+HER2-) (2), HER2-positive (ER-PR-HER2+), and (3) triple-negative (ER-PR-HER2-, abbreviated TNBC). Most breast cancers are luminal or ER-positive (approximately 60–80%) (43). ERs reportedly participate in numerous processes such as cell survival, proliferation, and tumor growth in breast cancer. Anti-estrogen therapy is considered the “gold standard” for treating luminal breast cancer (43). ERs affect the occurrence and development of breast cancer by binding to estrogens, thereby activating a unique signaling pathway.
ERα is overexpressed in breast cancer, which is approximately 10% in healthy tissues compared to 50–80% in breast cancer tissues (44). Additionally, ERα promotes tumor growth in breast cancer by interacting with estrogen. A study conducted in 1999 demonstrated the proliferative and differentiative roles of ERα in mammary gland development (45). ERα functions as a transcription factor for genes associated with tumor cell proliferation and growth, such as insulin-like growth factor-1 receptor (IGF1R), cyclin D1, anti-apoptotic BCL-2 protein, and vascular endothelial growth factor (VEGF). The phosphorylation of ERα serine 118 and serine 167 in breast cancer cells induces unique gene expression profiles, ultimately impacting tumor growth and morphology as well as the hormonal therapy responsiveness in patients with breast cancer (46). Anti-hormonal therapy with drugs such as tamoxifen has been widely used to treat patients with breast cancer. It exerts its effects by suppressing ERα-mediated transcriptional regulation and is used as first-line therapy. However, a significant proportion of the affected individuals eventually relapse and develop resistance to this antagonist (47). Tamoxifen can cause severe side effects in some tissues, such as those in the skeletal and cardiovascular systems, owing to its anti-estrogenic properties. Raloxifene has fewer side effects than tamoxifen. Other drugs targeting ERα, such as toremifene, fulvestrant, anastrozole, letrozole, and exemestane, have also been clinically approved for breast cancer therapy (26). Moreover, ERα splice variants are also implicated in breast cancer. ERα-46, is expressed in 70% of breast cancer tissues and occasionally shows a higher expression level than the full-length ERα-66. ERα-46 acts as a competitive inhibitor of ERα when co-expressed with ERα-66 (27). ERα-36, which lacks both AF1 and AF2, is considered a membrane-based ER that regulates membrane-initiated non-genomic signaling (28, 30). Tamoxifen acts as an agonist of ERα-36 in breast cancer and contributes to hormone therapy resistance and metastasis (48).
However, the role of ERβ in breast cancer remains controversial. The ERβ expression level decreases during breast cancer by approximately 80% in healthy tissues (44). ERβ knockdown does not affect mammary gland development. However, ERβ is occasionally associated with increased proliferation in breast cancer tissues (49). In vitro studies revealed that the re-expression of ERβ in breast cancer cells repressed cell proliferation, promoted cell apoptosis, and sensitized the tumor cells to chemotherapy (50). In addition, clinical studies have demonstrated that the absence of ERβ results in a poor prognosis (51) and resistance to hormonal therapy (52). In breast cancer, ERβ inhibited cell proliferation by suppressing the activation of MAPK and PI3K signaling pathways (53). However, a few reports support the notion that ERβ is a poor prognostic factor in breast cancer, and its expression is related to enhanced cell proliferation (54, 55). Some researchers have suggested that ERβ expression is not associated with clinical outcomes in women who have undergone menopause (56). Therefore, further experiments are required to validate our current understanding of the link between ERβ and breast cancer. Moreover, ERβ2 and ERβ5 might sensitize the breast cancer cells to therapy, thus leading to a good prognosis (57). ERβ2 is also sensitive to hormonal therapies. Although ERβ2 and ERβ5 cannot form functional homodimers, they can heterodimerize with ERα, thereby inhibiting the ERα function (26). We speculate that this might be the putative basis for their protective mechanism. Notably, ERα and ERβ form functional heterodimers when co-expressed in tissues (33). In this scenario, ERβ might inhibit the ERα-mediated gene expression.
GPER1 is thought to play a favorable role against breast cancer. In patients with breast cancer, GPER1 downregulation in the tumor tissue is associated with poor survival (58). GPER1 is detected in 60% breast cancer tissue. Among them, GPER1 expression has been confirmed in most TNBC, and the combined expression of GPER1 and ER accounts for about 40% of all cases (59). In contrast, GPER1 expression has been associated with reduced response or resistance to tamoxifen therapy in patients with breast cancer, mediated by regulating HMGB1 (high mobility group box 1) (60, 61). Recently, a study identified GPER1 as a crucial therapeutic target for triple-negative breast cancer, as it elicits an NF-κB/IL-6 signaling inhibition-mediated suppression of migration and angiogenesis (62).
In conclusion, ERα and GPER1 show promising roles in breast cancer development, whereas the role of ERβ remains controversial (Table 1; Figure 6). Specially, ERβ acts as a protective role through inhibiting the function of ERα. Moreover, ERα-46 is a competitive inhibitor of the full-length ERα. ERα-36 is associated with resistance to hormonal therapy. ERβ2 and ERβ5 play protective roles in breast cancer.
ERs in endometriosis and ovarian cancer
Endometriosis, characterized by lesions of endometrial-like tissue outside the uterus, is a chronic inflammatory disease with a phenotype similar to that of ovarian cancer. Endometriosis and ovarian cancer are hormone-dependent gynecological diseases with severe consequences on fertility and daily routine in women (166). Ovaries are the primary source of estrogen and progesterone in women. ERs affect the occurrence and progression of endometriosis (167) and ovarian cancer. In this section, we discuss the role of ERs in endometriosis and ovarian cancer.
The expression of ERα is higher than ERβ in the normal endometrium (168). Compared to normal endometrium, ectopic tissues show menstrual cycle-dependent increased ERα expression (169, 170). A study conducted on ERα-knockout mice suggested that ERα participates in the formation of endometriotic-like lesions (63). ERα likely promotes ovarian cancer development. ERα and ERβ are expressed in most patients with ovarian cancer (80%) (66). ERα is a contributing factor in developing ovarian cancer by promoting cell proliferation and migration (66). Multiple clinical studies have shown that ERα responds to hormonal therapies such as tamoxifen and aromatase inhibitors (66), which inhibit the conversion of androgen to estrogen, thereby reducing the circulating estrogen levels (66). Extensive studies have been conducted on ERα, which is reportedly mediated by long non-coding (lnc) RNAs that subsequently play a role in promoting ovarian cancer development (171). The lncRNA MIR2052HG regulates the ERα expression and confers resistance to aromatase inhibitors through lemur tyrosine kinase-3 (LMTK3) by recruiting early growth response protein 1 (EGR1) (172). The ERα expression and its role in promoting ovarian cancer progression suggest that hormonal therapy could be a viable treatment option. However, anti-estrogen therapy has a modest response rate among patients with ovarian cancer and is, therefore, not a commonly prescribed therapy (26).
ERβ expression is elevated in ectopic tissue than in the normal endometrium (169). In a mouse model of endometriosis, elevated levels of ERβ were detected in both the nucleus and cytoplasm (173). The specific mechanisms underlying the increased ERβ levels remain unclear. Hypermethylation of the ERβ promoter region could be associated with increased protein levels in the endometrial tissues (174). Methylation anomaly induces aberrant expression of GATA family members, which regulate the uterine physiology, and subsequently promotes abnormal expression of several genes, such as steroidogenic factor 1 (NR5A1) and aromatase (CYP19A1) (167, 175). Another mouse model-based study suggested that ERβ inhibits tumor necrosis factor α (TNF-α)-induced apoptosis by interacting with the cytoplasmic apoptotic machinery. The authors further demonstrated that the combined action of ERβ and the cytoplasmic inflammasome components result in the subsequent increase in interleukin (IL)-1β levels and promote cell survival, cellular proliferation, invasion, and adhesive activities of endometrial cells (64). These results indicate that ERβ overexpression facilitates the progression of endometriosis. In contrast, ERβ is generally regarded as a tumor suppressor in ovarian cancer and is associated with inhibiting cell growth and invasion (66). Decreased ERβ levels or ERβ/ERα ratio during ovarian carcinogenesis also suggests that the loss of ERβ might play a role in cancer progression. Treatment with the ERβ agonist diarylpropionitrile (DPN) or re-expression of ERβ significantly suppressed ovarian cell growth (26). Furthermore, ERβ direct affects ERα by strongly inhibiting its expression and activity, thereby exerting an anti-proliferative activity (67). In contrast to the inhibitory effect of full-length ERβ, both ERβ2 and ERβ5 isoforms are associated with pro-migratory and invasive activities in ovarian cancer (68). Cytoplasmic ERβ2 expression is associated with poor overall survival in patients with high-grade serous ovarian cancer (HGSOC) (69). High nuclear ERβ5 expression levels have also been observed in advanced ovarian cancer, especially in serous and clear cell carcinomas, and are associated with poor survival (68).
Additionally, estrogens can exert their effects through non-genomic signaling via mERs. GPER1 expression is reportedly higher in ovarian endometriosis than in the normal endometrium, and this expression is associated with estrogen levels and the extent of inflammation (176). This overexpression is also observed in endometriomas (177). Treatment with the GPER1 agonist G1 stimulated endometriotic cell proliferation as well as rapid Akt phosphorylation. In contrast, treatment with antagonist G15 reversed this proliferation and caused Akt dephosphorylation (65). Collectively, these results suggest a stimulatory role for activated GPER1 in the growth of ectopic endometrial tissues. However, GPER1 plays a complex role in ovarian cancer, demonstrating stimulatory and suppressive functions in cancer cells (66). Ignatov et al. considered GPER1 a tumor suppressor in ovarian cancer owing to its lower expression in ovarian cancer tissues than in benign tissues. The higher GPER1 expression in the early stages of ovarian cancer further emphasized its anti-tumor role (70). In contrast, Smith et al. and Zhu et al. demonstrated that GPER1 was associated with poor survival in patients with ovarian cancer (71, 72). In ERα-negative ovarian cancer cells, the G1 agonist stimulated the tumor cell proliferation and increased the number of cells in the S phase (72). Yan et al. highlighted the GPER1 ligand-independent stimulation mechanism in ovarian cancer cell proliferation, migration, and invasion (73). Albanito et al. proposed an interactive effect of ERα expression-dependent GPER1/epidermal growth factor receptor (EGFR) signaling on the growth response (74).
In summary, ERα, ERβ, and GPER1 promote endometriosis (Table 1; Figure 6). Nevertheless, ERα tends to be a promoting factor, whereas ERβ protects against ovarian cancer progression. The role of GPER1 tends to be more complex, displaying both promoting and protective effects in these disorders. Furthermore, ERβ2 and ERβ5 isoforms show a protective function in ovarian cancer. Therefore, further investigations on the role of ERs in gynecological disorders should be conducted to improve our understanding of the underlying molecular mechanisms.
ERs in prostate cancer
Prostate cancer, an epithelial malignancy of the prostate gland, is responsible for the highest number of cancer-related deaths in men. Its incidence increases with age. Several studies have demonstrated strong associations between ERs and prostate cancer, including their occurrence, development, and prognosis. However, the role of ERs in castration-resistant prostate cancer (CRPC) progression remains unclear. Serum estrogen levels are associated with the risk of prostate cancer development. This indicates that estrogen and ERs, which mediate the action of estrogens, may be risk factors (178, 179).
ERα is expressed in stromal tissues of the human prostate (180). The stromal cell receptor ERα stimulates the growth of prostatic epithelium via growth factors such as basic fibroblast growth factor (bFGF), epidermal growth factor (EGF), and IGF-1 (181). Data from animal models have revealed the indirect tumor-promoting role of ERα in the prostate epithelium. bFGF and EGF in the ventral prostate of adult rats and IGF-1 in the monkey prostate stimulated the prostatic epithelial cell proliferation (75, 76). Animal studies have shown that E2 and its association with testosterone induce prostate lesions and cancer (77). Combinatorial treatment with testosterone and E2 prevented the development of high-grade prostatic intraepithelial neoplasia (HGPIN) or prostate cancer following ERα knockdown (182). These observations indicate that ERα is crucial in the development of prostate cancer. ERα expression is upregulated in high-grade prostatic intraepithelial neoplasia (11%, 43%, 61%, and 94% in HGPIN, Gleason grade 4, grade 5, and recurrent adenocarcinoma, respectively, after therapy) (183).
In benign prostates, ERβ is primarily located in the cytoplasm and nucleus of basal epithelial cells as well as in the perinuclear region of luminal epithelial cells. In contrast to ERα, ERβ plays a tumor-suppressive role in cancer development and progression. ERβ is primarily expressed in epithelial cells (180), whereas other studies have demonstrated that ERβ is undetectable in stromal and epithelial cells (5). E2 exerts ERβ-mediated direct effects on the prostate epithelium. ERβ reportedly inhibits androgen receptor (AR) signaling, inflammation, and cell proliferation by downregulating AR signaling, inducible nitric oxide synthase (NOS), antioxidant glutathione peroxidase 3 gene, and interleukin (IL)-6. In contrast, it upregulates the tumor suppressor phosphatase and tensin homolog (PTEN) in the mouse ventral prostate (77). ERβ activation can suppress the effects of ERα and induce apoptosis in prostate cancer cells (77, 78, 184). The inhibitory role of ERβ is further supported by higher ERβ levels in primary prostate cancer, whereas ERβ is suppressed in high-grade prostate cancer (185). Markedly reduced ERβ levels can be observed in grade 4/5 carcinomas compared to that in grade 3 carcinomas (186). Five splice variants of ERα and ERβ (ERα-36, ERβ2, ERβ3, ERβ4, and ERβ5) were detected in men with and without prostate cancer (187). However, the role of ERα-36 remains unclear. In contrast to ERβ2, ERβ5 is almost entirely located in basal epithelial cells, underscoring its carcinogenic role (79).
The significance of GPER1 expression and signaling in prostate cancer biology remains unclear. GPER1 might exhibit a non-genomic estrogenic response together with ERα or ERβ (181). Contrary to its promoting function in breast cancer and endometriosis, GPER1 activation inhibits the growth of normal and malignant bladder urothelial cells (154). The suppressed GPER1 function has been demonstrated to aid the growth of prostate cancer cells. GPER1 also plays a role in sustainably activating erk1/2, c-Jun/c-fos-dependent upregulation of p21, and induction of G2 cell-cycle arrest (80). Increasing evidence suggests a protective function for GPER1 in prostate cancer. GPER1 knockout weakens these protective effects (81). A recent study also reported that treatment with G1 agonist induces massive tumor necrosis in castrated mice with CRPC (77).
In conclusion, the three ERs have distinct effects on prostate cancer, wherein ERβ and GPER1 exert tumor growth-suppressive effects (Table 1; Figure 6), and ERα, ERβ2, and ERβ5 exert tumor growth-promoting effects.
ERs in bone
Estrogens are vital for maintaining bone mineral density in humans. Osteoblasts and osteoclasts are involved in synthesizing the bone matrix and its degradation, respectively. E2 exhibits a protective role in the bone remodeling process by increasing the bone mass by inhibiting pro-osteoclastic cytokines in T cells and promoting the anti-apoptotic activity in osteoblasts (188, 189). The estrogen levels decline in women undergoing menopause, leading to a decreased bone density and increased risk of fractures (190). Hormone replacement therapy prevents this process through the mediation of ERs.
ERα and ERβ are highly expressed in osteoblasts, osteocytes, and osteoclasts (82). They are also expressed in immune cells, which are essential for bone cell regulation (40, 191, 192). The level of ERα is higher in cortical bone than trabecular bone, whereas the converse is true for ERβ (82), suggesting that ERα and ERβ may have opposing functions in these tissues. A mouse model study shows that ERα-knockout decreased cortical bone mineral density and increased that of the trabecular bone (83). In ERβ-knockout mice, cortical bone mineral density increases at 11 weeks of age, and both cortical and trabecular bone mineral density increases at 12 months of age (84, 85). The higher trabecular bone density in ERβ-knockout mice than in the ERα-knockout mice (85) suggests that ERβ plays a vital role in trabecular bone formation. The opposing effects of ERα and ERβ on the femoral bone length have also been demonstrated, with the ERβ-knockout mice developing longer femur bones than the ERα-knockout mice (193). Bone cells from ERβ-knockout mice cultured under mechanical strain showed an increase in osteoblast-like cells, whereas this increase was not observed in cells cultured from ERα-knockout mice (194). This underscores the role of ERα in augmenting the response of bone cells to mechanical strain, whereas ERβ exerts a suppressive function in this process.
Relatively few studies have been conducted on GPER1. Although GPER1 expression has been reported in osteoblasts, osteocytes, and osteoclasts (86), their role in bone growth remains controversial. Loss of GPER1 might lead to bone growth reduction in female mice (87). In male mice, GPER1 deficiency increases bone mass, mineralization, and growth plate proliferative activity (88). GPER1 activation protects the bones of ovariectomized rats from developing osteoporosis and has no adverse effects on the uterus (195). However, G1 agonist-mediated stimulation does not influence bone growth in mice (196).
In conclusion, ERs play crucial yet opposing roles in mediating bone homeostasis. ERα is important in the cortical bone, whereas ERβ is vital in the trabecular bone (Table 1; Figure 6). ERα is also involved in mechanical strain responses. GPER1 might be associated with bone growth. However, additional studies should be conducted to determine their precise role in these processes.
ERs in lung cancer
Lung cancer is the leading cause of cancer-related death worldwide. Estrogen is presumed to play an important role in lung cancer development (197). Approximately 85–90% of lung cancer cases are attributed to smoking (198). Women are at a higher risk than men to develop lung cancer regardless of their smoking status. The higher susceptibility of women to the adverse effects of tobacco could be owed to estrogen-mediated responses (197). Older women with lung adenocarcinoma have a significant survival advantage compared to their male counterparts, possibly owing to the reduced effectiveness of estrogens in promoting cancer (199). Estrogens, which are mediated by ERs, adversely affect the prognosis of patients with lung adenocarcinoma.
ERα and ERβ are both expressed in the health and normal lung tissue (200). The expression level of ERα in lung cancer remains unclear, while ERα mainly resides in the cytoplasm of lung cancer cells and is associated with a poor prognosis (90, 201). It reportedly promotes cell invasion in lung cancer, mediated by increased cross-talk with the infiltrating macrophages (90).
ERβ is highly expressed in pneumocytes and bronchial epithelial cells in healthy lung tissue and is required to maintain its extracellular matrix (202, 203). ERβ-deficient mice have fewer alveoli and a lower amount of surfactant (204). ERβ appears to be dominant in lung cancer, especially in adenocarcinoma (205). It is also present in adenocarcinoma tissues, and the absence of ERβ tends to result in poor overall survival (201). Most reports have suggested that nuclear ERβ is associated with a better prognosis, whereas its cytoplasmic form is associated with a worse prognosis (206, 207). A recent study showed that ERβ promotes lung cancer invasion by increasing C-X-C chemokine receptor type 4 (CXCR4) expression (91). Among the five identified splice variants, ERβ1 is the only full-length receptor capable of binding ligands and forming homodimers in humans. Although the other isoforms are inactive, they form heterodimers with ERβ1 to regulate their transcriptional activity (208). Overexpression of ERβ5 and EGFR is reportedly associated with poor prognosis and reduced overall survival in patients with non-small cell lung cancer (NSCLC) (209).
Increased GPER1 expression, especially in the cytoplasm, has been observed in lung cancer cells (92, 210). GPER1 is likely involved in the proliferation, migration, and invasion of cancer cells (211). GPER1 agonist G-1 play an antiproliferative and proapoptotic effects in lung cancer (212).The in vitro observations from the E2 and G1 models suggested that an increase in tumor nodules, grade, and the index could be reversed by co-administration of GPER1 inhibitor G15 (92).
In conclusion, ERα is mainly expressed in basal and smooth muscle cell, and exhibits as a promoting factor in lung cancer progression (Table 1; Figure 6). ERβ is mainly expressed in columnar epithelium and intermediate, basal and smooth muscle cells, and shows a promoting role in lung cancer. In addition, exist of ERβ in different cell areas shows different prognosis. As for GPER1, the present study shows that it exhibits an inhibiting role in tumor growth and migration. ERs in cardiovascular diseases
Cardiovascular diseases (CVD) are a collective group of disorders caused by abnormalities in the heart and blood vessels. They are the principal cause of death in both men and women. Epidemiologic studies have shown that prior to menopause, women are at a lower risk of developing CVD compared to men. Women also have decreased CVD-associated morbidity compared to age-matched men, and present with CVD 10 years later than men (213). Heart failure-related morbidity is reportedly higher in men at any age (214). Women who are pre-menopausal are at reduced risk of ischemia/reperfusion (I/R) injury compared to men (215). Women who have undergone menopause demonstrated worse survival than women who were premenopausal and age-matched men. Estrogen levels in women who have undergone menopause are negatively associated with the risk of CVD development (213). A recent study confirmed the protective effects of exogenous E2 on preexisting advanced heart failure in mice (104). Collectively, sex hormones, especially estrogens, tend to be protective factors in mediating homeostasis in patients with CVD, following the sex-related bias in CVD development. Estrogens mediate their functions by binding to ERs, which are also present in the cardiac tissues. In the following section, we explore the crucial roles of ERs in cardiovascular diseases.
Cardiac disease
Myocardial I/R injury and heart failure are two common heart-related diseases (213). I/R injury relates to the tissue damage caused by myocardial reperfusion following a period of ischemia, which is characterized by inflammation, oxidative stress, intracellular and mitochondrial calcium overload, apoptosis, or necrosis. Heart failure results from dysfunctional systolic and/or diastolic processes, manifesting as venous system blood obstruction and arterial system blood perfusion inadequacy. ERα, ERβ, and GPER1 protect against I/R injury and heart failure (213, 216).
Experiments on animal models have demonstrated the cardioprotective role of ERα in regulating I/R injury (217). ERα-knockout female rabbits exhibited significantly higher functional recovery than wild-type females, with similar effects compared to wild-type males. A study on rabbits also highlighted the cardioprotective role of ERα but not ERβ (96). Treatment with PPT (4, 4’, 4’’-[4-propyl-(1H)-pyrazole-1, 3, 5-triyl] tris-phenol, an ERα-selective agonist) in an I/R injury rabbit model decreased the infarct size. ERα-knockout male mice demonstrated reduced mitochondrial respiratory function, decreased nitrite production, and increased Ca2+ accumulation compared to control mice (97). Additionally, 16α-lactone-estradiol (16α-LE2), an ERα-selective agonist, reduced the progression of myocardial hypertrophy and systolic dysfunction during heart failure (98). Increased ERα expression also improved cardiac intercalated disk stability during heart failure in humans (99).
ERβ-knockout in mice induces severe heart failure and death, underscoring the protective role of ERβ (103). Treatment with ERβ agonist DPN improved cardiac function in mice by stimulating cardiac angiogenesis, suppressing fibrosis, and restoring hemodynamic parameters (104). ERβ is also essential for improving cardiac recovery after I/R injury by inhibiting apoptosis and preserving mitochondrial integrity (95). ERβ protects against cardiac hypertrophy, the primary risk factor for heart failure. ERβ knockout induces inflammatory pathways and modulates mitochondrial bioenergetics- and oxidative stress-related pathways (105). ERβ also suppresses cell death by inhibiting p53, thereby attenuating reactive oxygen species (ROS) in mitochondria-centered apoptotic processes (106).
GPER1 can protect the heart against I/R injury by inhibiting mitochondrial permeability transition pore (MPTP) opening (113). Another study reported enhanced protective effects of GPER1 in mitochondria, indicating that it can maintain mitochondrial structural integrity and function, thereby reducing mitophagy in I/R injury (114). Oxidative stress affects cardiac remodeling after ovarian estrogen loss. GPER1 deficiency induces cardiac remodeling through oxidative stress (115). Numerous reports have also revealed that GPER1 can decrease fibrosis and improve myocardial relaxation and diastolic function (116). The GPER1 agonist G1 reduced the risk of isoproterenol-induced heart failure in female mice (117). Treatment with G1 improved cardiac function and myocyte contractility, and reduced fibrosis. The cardioprotective ability of GPER1 is mediated by the expression of adrenergic receptors in ventricular myocytes.
In conclusion, ERα, ERβ, and GPER1 contribute to myocardial protection following I/R injury (Table 1; Figure 6). ERα exhibits functions of reducing infarct size, decreasing ROS production, and attenuating myocyte apoptosis. ERβ can attenuate apoptosis and decrease ROS production. Activation of GPER1 maintains mitochondrial function, decreases fibrosis, and improve myocardial relaxation and diastolic function.
Vascular disease
Hypertension (HTN), pulmonary hypertension (PH), and atherosclerosis are typical vascular diseases (213), wherein ERα, ERβ, and GPER1 are known to mediate protective functions. ERα activation reduces endothelial dysfunction by contributing to endothelial progenitor cell activation and VEGF upregulation (218). ERα is highly expressed in pulmonary artery smooth muscle cells (PASMCs), wherein it increases the proliferation of PASMCs via MAPK and Akt signaling and enhances vascular remodeling (93). ERα-knockout mice displayed lower recovery of cardiac function and greater difficulty in breathing (97). ERα expression reduced vasoconstriction and induced right ventricular (RV) hypertrophy in male mice (100). Loss of ERα increased the occurrence of atherosclerotic lesions through elevated serum cholesterol levels and increased high-density lipoprotein particle size (101). ERα tended to decrease vascular smooth muscle cell (VSMC) proliferation through reduced ROS-mediated extracellular signal-regulated kinase (ERK) phosphorylation (94). ERα also demonstrated anti-atherosclerotic properties such as increased antioxidation (219) and decreased lipid accumulation (220).
ERα and ERβ are both expressed in endothelial cells and VSMCs of arteries (102, 221). ERβ activation reduced endothelial dysfunction by activating endothelial progenitor cells (218), decreasing VSMC differentiation (222), and upregulating VEGF levels (218). ERα and ERβ expressions suppress apoptosis and eliminate the generation of intracellular ROS by decreasing the protein levels of ROS-generating enzymes, as well as preventing NF-κB activation in human aortic endothelial cells stimulated by H2O2 (107). In addition, estrogen rescued pre-existing severe PH by restoring the lung and RV structure in rats. The rescue ability was associated with the stimulation of neoangiogenesis, suppression of inflammation, fibrosis, and RV hypertrophy. These estrogen rescue effects are mediated by ERβ (108). ERβ-mediated increases in inducible NOS expression decrease vasoconstriction (109). ERβ decreased fibrosis, inflammation, vasoconstriction, RV remodeling, and RV hypertrophy (110, 111). ERβ has also been shown to decrease calcification (222).
GPER1 is widely distributed in the cardiovascular system and exerts protective effects on the vasculature (112). GPER1 decreases blood pressure, promotes vasodilation, and reduces the proliferation and migration of VSMCs (223). In addition, activation of GPER1 may limit fibroblast proliferation by inhibiting cyclin, cyclin B1, and CDK1 (118). E2-induced decrease in VSMC proliferation rate after injury is mediated by GPER1 rather than ERα and ERβ (224, 225). Furthermore, GPER1 activation by G1 agonist phosphorylates endothelial NOS, resulting in NO-mediated vasodilation in human endothelial cells (226). NO formation via G1 agonist is mediated by multiple pathways, such as the proto-oncogene tyrosine-protein kinase (c-Src), EGFR, PI3K, and ERK (226).
In conclusion, all three ERs plays protective roles in vascular disease (Table 1; Figure 6). ERα can reduce endothelial dysfunction, reduce RV hypertrophy, and increased antioxidation. ERβ decreases ROS production, inhibits inflammatory response, suppressed fibrosis, and decrease calcification. GPER1 reduces blood pressure, stimulates vasodilation, inhibits fibroblast proliferation, and decreases VSMC proliferation and migration.
ERs in gastrointestinal disease
Gastrointestinal diseases are a collective manifestation of esophageal, gastric, and intestinal disorders such as hepatic and pancreatic diseases. Gastrointestinal diseases are highly associated with sex differences and female estrogen status (227–231), which indirectly indicates the potential role of hormones in gastrointestinal diseases. Considering the differential effects of ERs in different gastrointestinal diseases, we explored the multifaceted roles of estrogen and ERs in this section.
Esophageal diseases
Gastroesophageal reflux disease (GERD) and esophageal cancer (EC) are the primary esophageal diseases. GERD is a recurrent disease characterized by abnormal reflux at the gastroesophageal junction. EC, one of the most common malignant tumors in the gastrointestinal tract, is divided into two histological subtypes: esophageal adenocarcinoma (EAC) and esophageal squamous cell carcinoma (ESCC). EAC can arise from chronic GERD (232). The prevalence of GERD and EC in men is reportedly higher than in women. However, the risk of disease development rapidly increases in women who have undergone menopause (227). Notably, the incidence of ECA and ESCC is 6–10 times and 2–3 times lower, respectively, in women than in men (233). Estrogens play a protective role in GERD and EC. Estrogens exhibit anti-inflammatory functions and decrease esophageal tissue damage during GERD development (232). A study conducted in female rats showed that estrogen reduces esophageal tissue damage by binding to ERs (232). Boeckxstaens et al. also reported a close relationship between an increased prevalence of reflux esophagitis and reduced estrogen levels after menopause (234). A meta-analysis further demonstrated that estrogen could decrease the risk of EC and that hormone replacement therapy was negatively correlated with the risk of EC (235). Zhang et al. demonstrated the anti-proliferative function of estrogen in ESCC, and this effect was weakened by an ER antagonist (120). Estrogen may exert an anti-proliferative effect in ESCC cells by promoting the ER-calcium signaling pathway.
ERα and ERβ are highly expressed in ESCC cell lines. However, the expression level and role of ERα in ESCC remain controversial. Nozoe et al. demonstrated that ERα is expressed in the cytoplasm of 64.4% tumor tissue in immunohistochemistry analysis (122). Dong et al. suggested that ERα is related to improved survival and depth of tumor invasion (119). In contrast, Nozoe et al. reported poorer survival in association with ERα (122). A meta-analysis of 20 studies implied that higher ERα expression levels could be associated with poorer survival (121), highlighting the facilitating role of ERα in ESCC. The role of ERα in esophageal diseases warrants further exploration.
The role of ERβ in esophageal diseases also remains controversial. Wang et al. suggested that estrogen in the areas of EC high incidence is relatively lower, and the inhibition role of estrogen against tumor is mediated by the ERβ (236). Nozoe et al. demonstrated that the ERβ is expressed in nuclei of 28.8% tumor tissue (21/73) (122). They also found that the ERβ (–) is a poor prognostic factor, indicating a protective role in the EC. A previous study has suggested that ERβ in ESCC is typically associated with adverse outcomes (124), whereas estrogen and ERβ were shown to inhibit the cell proliferation in EC (123). Relatively fewer controversial results have been documented in GERD. The unanimity of these results indicates that estrogens and their receptors are highly associated with GERD risk, and hormone replacement therapy in women who have undergone menopause increases reflux symptoms (237).
Few studies have focused on GPER1 in esophageal diseases. One study revealed that GPER1 overexpression and upregulation of the cancer suppressor gene beclin-1 in ESCC is associated with poor prognosis (125). The authors emphasized that GPER1 promotes ESCC through increased cell proliferation and metastasis. p38 MAPK inhibitor may block the effects of GPER1, indicating that its tumor-promoting role in ESCC development is mediated by p38 MAPK.
Taken together, ERα and ERβ exhibits both inhibit and promote role in esophageal diseases (Table 1; Figure 6). Whereas, GPER1 may promote cell proliferation and metastasis in through p38 MAPK pathway.
Gastric diseases
Peptic ulcers (PUs) and gastric cancer (GCs) are the most common gastric diseases. PU results in gastric and duodenal ulcers with complications such as upper gastrointestinal bleeding and gastric outlet obstruction. PU is relatively rare during pregnancy, suggesting that estrogen may exert a protective effect. The risk of developing PUs is lower in women than in men (228, 229). Women also tend to develop ulcerative lesions after their menopause (238). The decrease in serum estrogen levels weakens the gastric mucosal defense mechanisms. Additionally, the antioxidant effects of estrogens directly eliminate free oxygen radicals, activate antioxidant enzymes, inhibit the production of superoxide, and decrease the formation of PUs (239). GC is the third most lethal malignant tumor. Similar to PU, the incidence of GC in men is reportedly higher than that in women. In contrast, the possibility of developing GC appears to be similar between women who have undergone menopause and men (240). Tokunaga et al. reported the protective function of estrogens against GC (241). Untreated male rats showed increased mobility rates compared to the castrated or estrogen-treated male rats with GC (242).
ERs play pivotal roles in the occurrence and development of GC. Although ERα and ERβ are expressed during GC, ERβ, but not ERα, is abundantly expressed in GCs (243). Some studies suggest over expressed ERα (244) while some suggest low expressed ERα in GC (245). ERα overexpression increases GC cell apoptosis and inhibits cell growth and proliferation (126). Another study reported that positive ERα expression in GC cells is related to poor prognosis in patients with GC, thereby highlighting its role in modulating cell proliferation, migration, and invasion by regulating the expression of p53, p21, p27, cyclin D1, and E-cadherin (127). In addition, ERα-36 tends to be highly expressed and is associated with lymph node metastasis in GC (128).
The expression level of ERβ in tumor tissue is lower than in healthy tissue (243, 245). ERβ is likely a protective factor against GC invasiveness (129). Suppression of ERβ can promote GC cell apoptosis by inducing autophagy (130). The malignant growth of GC tumor cells is mediated by overexpression of GRP78 and GRP94 (246, 247). In addition, Guo et al. detect the expression of ERβ1, ERβ2, and ERβ5 in GC tissues, and suggest that the expression of these three ERβ variants is found in most tumor tissues, and the expression of ERβ5 is significantly higher than that of ERβ1 and ERβ2. The high expression of ERβ5 in tumor tissues is associated with high tumor stage and lymph node metastasis, suggesting poor prognosis (131).
GPER1 expression levels are reportedly downregulated in GC tissues. The lower GPER1 expression tends to be negatively correlated with the survival of patients with GC (132). It also acts as a tumor suppressor by regulating the epithelial-mesenchymal transition pathway (132). In contrast, Zhang et al. detected higher GPER1 expression levels in GC than in normal tissues and suggested that this overexpression is associated with poor survival (133).In conclusion, ERα and GPER1 both shows controversial function in the progression of GC (Table 1; Figure 6). ERβ is a protective factor against GC invasiveness. Furthermore, ERα-36 is associated with lymph node metastasis in GC and high expression of ERβ5 in tumor tissues is associated with high tumor stage and lymph node metastasis, suggesting a poor prognosis.
Intestinal diseases
Irritable bowel syndrome (IBS), inflammatory bowel disease (IBD), and colon cancer (CC) are three major intestinal disorders. IBS is characterized by disorderly bowel movements and chronic abdominal pain. Some studies have highlighted the significant roles of estrogen and ERs in the pathogenesis of IBS. IBS (230) and CC (231) are more prevalent in women than in men, suggesting that estrogen participates in IBS and CC pathophysiology. IBS symptoms are reportedly related to hormones (248). IBD can be associated with the development of colorectal cancer, with men demonstrating a higher probability of developing colorectal cancer than women (249). Men also present a higher risk of developing colitis than women, suggesting a protective function of estrogen against colitis development (250). Collectively, estrogen, mediated by ERs, plays a promising and significant role in the development of intestinal disorders.
ERα expression is higher in IBS (138), and ERα-mediated cancer cell proliferation is considered a risk factor for CC (134). ERβ expression is lower in patients with IBD (251) and protects against colitis-associated neoplasia (250). ERβ upregulation indicates a better survival outcome, whereas its downregulation results in poor overall survival (135, 135). ERβ knockout results in greater damage in the gastrointestinal system of mice (136) and enhances tumor cell proliferation in both male and female (137). These findings underscore the protective effects of ERβ on CC. Higher GPER1 levels in patients with IBS (138) have been associated with pain severity (138). The GPER1-mediated estrogenic effects regulate visceral pain and gastrointestinal motility (139). The expression and inhibition of VEGFA by E2 can be mediated by a GPER1 dependent mechanism (231). Interestingly, when ERβ is absent in patients with CC, ERα and GPER1 are activated under anoxic conditions (231).
The expression of ERα-36 and ERα-46 in CC is higher than that in normal tissue. ERα-36 is associated with tumor stage and lymph node metastasis (252), suggesting that the two splice variants might be associated with CC development. Low ERβ2 expression in CC is likely associated with the occurrence of tumors in females (253, 254).
ERα and GPER1 tend to show harmful effects, while ERβ shows a protecting role in intestinal diseases (Table 1; Figure 6). More interestingly, Erα and GPER1 and will be active when ERβ is absent. Furthermore, splice variants of ERα-36, ERα-46, and ERβ2 may be involved in the CC development.
Liver disease
The liver is a hormone-sensitive organ, and its functions are influenced by estrogens (255). Common liver diseases include hepatitis, cirrhosis, liver abscesses, and liver cancer. Estrogens and their receptors play important roles in the liver (256). The role of estrogens in the liver tends to be controversial, as they both promote and inhibit chronic liver disease and carcinogenesis (257, 258). Many studies have verified the protective role of estrogen in ovariectomized rat models, whereas elevated estrogen levels have also been associated with chronic liver disease in some clinical studies (257). The role of estrogens in the development of hepatocellular carcinoma (HCC) is also controversial roles owing to its carcinogenic and protective effects on the liver (258).
In health liver tissue, men have higher ERα expression level than women, while no significant differences in ERβ expression. In addition, ERα:ERβ is higher in health men (143). ERα and ERβ are expressed in liver tissues and have promoted liver regeneration by orchestrating liver cell proliferation and differentiation (259). ERα is lower expressed in HCC, and is demonstrated to play an inhibiting role in tumor (260). ERα is demonstrated to up-regulate the expression of Protein Tyrosine phosphatase receptor Type O (PTPRO), which plays an inhibitory role in liver cancer (140). Similarly, Dai et al. reported that ERα gene is one of the tumor suppressor genes of HCC, and its methylation is associated with no microvascular infiltration and low histological grade (141). They believed that the protective role of ERα gene in HCC is related to its methylation. MicroRNA-221 can promote the proliferation of HCC cells by inhibiting the expression of ERα (142). Li et al. (261) reported that ERα expression is also down-regulated in female hepatitis B virus-associated HCC, and increased expression of microRNA-18 can inhibit ERα translation. They also found that up-regulated expression of p53 gene or mutation of p53 gene can inhibit ERα by regulating microRNA-18, thus weakening the anticancer effect of the ERα pathway. The overall survival rate and disease-free survival rate were higher in patients ERα positive (262). In men, liver ERα levels increase during the development of HCC (263). While in hepatitis C virus-associated HCC, ERα and ERβ expression is higher (143). The author points that higher ER expression is associated with higher NF-κB and cyclin D1 expression, which related to cell proliferation and invasion. ERα variants are expressed at higher levels in tumor tissues than in peritumoral tissues, further demonstrating the promoting role of ERα in HCC (255). A study demonstrated that ERα and its two splice variants are differentially expressed in the liver: healthy liver has high expression of ERα-36; patients with cirrhosis have moderate expression levels of ERα-66, ERα-46, and ERα-36; patients with HCC do not express ERα-66, but have a moderate ERα-46 expression and a high ERα-36 expression (258). These different expression patterns suggest that genetic variability may be vital in developing liver diseases.
ERβ has been reported to be related to lipid metabolism. ERβ activation by genistein (a natural phytoestrogen) improves hepatic lipid metabolism (264). ERβ is involved in the regulation of hepatic fibrosis (265). The anti-fibrotic effect of estrogen is mediated by ERβ but not by ERα or GPER1. ERβ-selective agonists ameliorate liver cirrhosis in rats by inhibiting HSC activation and proliferation of hepatic stellate cells (266). Estrogen inhibits the proliferation of HCC cells by inhibiting tumor-associated macrophages through ERβ (144). In addition, ERα and ERβ inhibit transcription and translation of peroxisome proliferator-activated receptor γ (PPARγ) gene in a ligand-dependent manner, and PPARγ can promote the proliferation of hepatocellular carcinoma cells (145).
The role of GPER1 in the liver is complex. The expression of GPER1 in HCC tissues is lower than that in non-HCC tissues (146). The development of liver tumors accelerates in HCC rat models with GPER1 knockout, and this effect is mediated by inflammatory responses, such as increased immune cell infiltration and IL-6 (146). These results demonstrated that GPER1 activity might be an effective strategy for preventing and treating HCC. Another study found that GPER1 is a protective factor in alleviating hepatocyte necrotic apoptosis in hepatic ischemia-reperfusion injury (147).
Taken together, ERβ and GPER1 show inhibiting role in the progress of liver cancer through reducing the fibrosis and immune response, while the role of ERα remains controversial (Table 1; Figure 6). When ERα works together with ERβ, they show an inhibitory role on PPARγ, which promoting the proliferation of hepatocellular carcinoma cells.
Pancreatic disease
The pancreas is an essential digestive organ in humans. The pancreas has endocrine and exocrine functions, mainly related to glucose metabolism. The external secretion of the pancreas is pancreatic juice, which is secreted from the pancreas and produces digestive enzymes that help digest fat. Islet cells produce insulin, which lowers blood sugar, and glucagon, which increases blood sugar. Pancreatic diseases include inflammatory diseases, pancreatic cysts, and other malignancies. When pancreatitis occurs, fat in the body is difficult to digest. Estrogens are highly associated with glucose metabolism (267). Furthermore, pancreatic ductal adenocarcinoma (PDAC) is the most common pancreatic cancer, and estrogen receptors may play an important role in its progression.
ERα is significantly expressed in pancreatic cancer tissues and is related to tumor size, distant metastasis, and poor prognosis of pancreatic cancer. ERα promotes cell proliferation, tumor growth, migration, and invasion by activating epithelial-mesenchymal transformation (148). ERα knockdown induced apoptosis and G0/G1 cell cycle arrest in pancreatic cancer cells. This study further demonstrated that ERα enhances the transcription of PAI1 (plasminogen activator inhibitor 1) and activates the MEK/ERK pathway, thereby promoting pancreatic cancer progression. These findings suggest that ERα may be a novel diagnostic and therapeutic target for pancreatic cancer (148). In most studies, ERα was detected in pancreatic tumors, while ERβ was absent, indicating that the role of ERβ in pancreatic cancer is unclear (268). The ERβ expression level in some cell lines is higher, and favorable prognostic features have been found with higher ERβ expression levels (269). A recent study showed that ERβ is mainly expressed in malignant cells and is considered a negative prognostic factor and possible therapeutic target for PDAC (270). Raloxifene has been demonstrated to reduce PDAC cells by disturbing the expression of ERβ and inhibiting the IL-6/gp130/STAT3 signaling pathway, indicating ERβ may carcinogenic role in pancreatic cancer (149).
High GPER1 expression in PDAC is associated with improved survival (150). Administering GPER1 agonist G1 reduced the tumor progression and prolonged survival of the treated mice (151). Activated GPER1 causes G1-S cell cycle arrest and corresponding decreases in p-RB and c-Myc. c-Myc, which is frequently overexpressed in cancer, is a transcription factor that stimulates proliferation and growth by activating many target genes. In addition, G1 significantly improved the efficacy of PD-1 targeted immunotherapy (151).
Collectively, ERα and ERβ show promoting role in pancreatic disease and may be the therapy target (Table 1; Figure 6). Activated GPER1 can inhibit the proliferation and growth of tumor cells and improve the efficacy of immunotherapy.
ERs in urogenital tract disease
Urinary tract infections (UTIs) and urothelial carcinoma of the bladder (UCB) are the most common urogenital diseases. UTIs are bacterial infections, wherein 80% are caused by uropathogenic Escherichia coli. UCB is the most common bladder malignancy. Endogenous estrogen protects against UTIs (271–273). Postmenopausal women are more likely to develop recurrent UTIs (274). Many other studies have also emphasized the protective role of estrogens against UTIs (275, 276). In UCB, estrogen is likely related to its development. The protective roles of estrogens in UTIs and their carcinogenic role in UCB are mediated by ERs.
In UTI, ERα is related to the bacterial clearance functions. In ovariectomized mice, ERα agonist PPT treatment decreases the bacterial load in the kidney, whereas treatment with the ERα antagonist MPP (methyl-piperidino-pyrazole) impairs bacterial clearance. These results suggest that bacterial clearance in the kidney is mediated by ERα (271). Estrogen primarily stimulates urothelial cell proliferation through ERα and ERβ activation (277). High ERβ expression levels are detected in the epithelial cells of the urogenital tract, whereas the ERα expression level is much lower (278). However, significantly higher ERα expression levels are observed in bladder transitional cell carcinomas than in primary cells, but similar level of ERβ is found in that all of these urothelial cell (277, 279). Estrogen stimulates the increased ERα expression in male rats and induces urogenital carcinogenesis (152). ERβ also stimulates tumor cell growth (153) and is upregulated in UCB compared to that in the benign urothelium, suggesting an oncogenic function. Tamoxifen blocks cell proliferation (153). Teng et al. pointed that both ERα and ERβ contribute to estrogen-induced G1/S phase progression and cell proliferation in urothelial cells, and increased ERα expression may induce early cyclin D1 and cyclin E expression, thus resulting in dysregulated cell proliferation in bladder cancer cells (277).
Urothelial cells also express high GPER1 levels (154). A study suggested that E2 and the GPER1 agonist G1 would inhibit urothelial cell proliferation by mediating GPER1 activity. GPER1 overexpression also inhibited E2-induced cell proliferation, and siRNA-mediated decrease in GPER1 expression restored E2-induced cell proliferation (154). These results imply that the inhibitory effects of E2 on cell proliferation are mediated by GPER1. Mechanistically, GPER1 inhibits urothelial cell proliferation by decreasing cyclin D1 by downregulating activation of protein-1 signaling.
In conclusion, both ERα and ERβ stimulates urothelial cell proliferation and tumor cells growth, while GPER1 inhibit urothelial cell proliferation (Table 1; Figure 6). Furthermore, ERα shows function of bacterial clearance.
ERs in neurodegenerative disease
Neurodegenerative diseases are characterized by decreased mitochondrial activity, oxidative phosphorylation, and increased ROS production in the CNS. Common neurodegenerative diseases include Alzheimer’s disease (AD) and Parkinson’s disease (PD). AD is a progressive neurodegenerative pathology characterized by extracellular amyloid plaques and intracellular neurofibrillary tangles, leading to neuronal dysfunction and cell death, negatively affecting cognition and memory in patients with AD (280). Postmenopausal women reportedly show a higher prevalence of AD and faster cognitive decline than men (281, 282). Some researchers have reported inhibition of aromatase (a crucial enzyme that converts androgens into estrogens) by altering β-amyloid deposition (283). Hormone replacement therapy decreases the risk of developing AD and decelerates declining cognitive function (284). Additionally, a meta-analysis demonstrated that hormone replacement therapy could benefit postmenopausal women with neurodegenerative diseases such as AD and PD (285). Several studies have shown the neuroprotective function of estrogens, which act as antioxidants, facilitating DNA repair, inducing growth factor expression, and regulating cerebral blood flow (282). Parkinson’s disease (PD) affects motor functions, which eventually progresses to cognitive impairment. Women with lower estrogen exposure are more likely to develop PD than those with higher estrogen exposure (286). E2, rather than androgens, has a protective effect on dopamine neurons (287). E2 also appears to have a protective effect on PD progression and treatment response (288).
ERα, ERβ, and GPER1 are expressed in cortical and hippocampal neural stem/progenitor cells. ERα is mainly associated with regulating reproductive functions, including the hypothalamus and the preoptic regions (156). ERα levels in female rats are often higher than those in male rats (155). Simultaneously, ERα is abundantly present in the prefrontal cortex of nonhuman primates. Ca2+ signaling plays a vital role in maintaining normal brain function. ERα- and ERβ-selective agonists can effectively activate rapid intracellular Ca2+ influx in neurons, leading to downstream MAPK signaling and ERK phosphorylation, thus playing a neuroprotective role (289). Hyperactivation of glutamate receptors can also induce excitotoxic neuronal damage, whereas ERα can prevent this damage via its estrogen signaling mechanism. Co-activation of ERα and IGF-IR may mediate neuroprotection via ERK and Akt phosphorylation in patients with AD (290).
ERβ is widely distributed and expressed in the hippocampus, cerebral cortex, lateral septa, and medial and basolateral amygdala. ERβ expression levels are higher than those of ERα in human and rat hippocampi (155). ERβ-knockdown mouse embryos showed lower cortical neuron migration and greater apoptosis than wild-type mice. Adult mice with ERβ knockdown showed abnormal morphology during brain development, including decreased neurons in cortical regions (291). Additionally, brain-derived neurotrophic factor (BDNF) regulates synaptic genesis and maturation. Activation of ERβ expression significantly increases the BDNF protein levels in postmenopausal mice, which plays a pivotal role in promoting the survival and differentiation of brain neurons (157, 158). ERα and ERβ distinctly contribute to neuroprotection against MPTP toxicity in mice (287). The striatum is one of the basal ganglia of the brain, and it regulates muscle tone and coordinates various fine and complex movements. Damaged striatum can impair its associated functions. ERα knockout mice are more sensitive to MPTP toxicity, whereas ERβ knockout mice have lower levels of striatal dopamine transporters.
GPER1 agonists reportedly increase dendritic spine density in the hippocampus. Hippocampal GPER1 also participates in estrogenic mediation of learning and memory via rapid signaling mechanisms (159). GPER is also involved in regulating hippocampal synaptic plasticity. BDNF expression induced by selective GPER1 activation promotes synaptic plasticity (292). Activation of the PI3K/Akt pathway can be correlated with GPER1 activation-conferred protection in AD models (160) and PD (161). GPER1 also mediated the E2 signaling pathway and exerted a neuroprotective action via regulating the PI3K/Akt and MAPK pathways in PD (292).
Like in the cardiovascular system, ERα, ERβ, and GPER1 all show neuroprotective role in nervous system (Table 1; Figure 6). ERα can stimulate Ca2+ influx and glutamate receptors in neurons and thus prevent the damage of neurodegenerative diseases. ERβ expression s increases the BDNF protein levels, thus promote the survival and differentiation of brain neurons. GPER1 may increase dendritic spine density in the hippocampus and synaptic plasticity.
ERs in cutaneous melanoma
Melanoma is an aggressive tumor characterized by mutations in the oncogenes BRAF or NRAS, resulting in the overactivation of the MAPK/ERK and PI3K/Akt pathways (163). Current treatments for melanoma focus on mutated BRAF and its downstream pathways. Melanoma is traditionally considered a non-hormone-related cancer, while increasing evidence suggests a direct relationship between the sex hormones (especially estrogens) and melanoma (293). The prevalence and prognosis of melanoma are significantly associated with sex divergence. In melanoma, females possess a significant survival advantage (approximately 30%) over males (294). However, these advantages disappear in the postmenopausal period marked by reduced estrogen levels (295). Additional evidence has demonstrated that sex differences exist in tumor metastasis (296). Researchers have highlighted the vital role of estrogens in the differential manifestation of disorders between females and males.
ERα is the primary epidermal receptor in human tissues and is expressed at low levels in metastatic melanoma and pregnancy-associated melanoma (193, 293). Immunohistochemical observations from 38 patients with melanoma confirmed that ERα expression was absent malignant melanoma (297). Traditionally, ERα was thought to promote the growth of melanoma cells (298). Tian et al. demonstrated that ERα exhibited a pro-proliferative effect in melanoma by inducing the Akt and MAPK signaling pathways (162).
ERβ is predominant in all melanocytic lesions, including nevi and melanoma (163). de Giorgi et al. have found that the expression level of ERβ in melanoma is decreased when compared with health tissue (299). As the tumor progresses, the expression level of ERβ is decreasing, which is closely related to the occurrence and development of malignant melanoma (193, 300). Moreover, ERβ expression was reduced upon cellular exposure to ultraviolet (UV) light, resulting in thinner lesions with a prominent epidermis (293). In addition, the level of ERβ in women is significantly higher than that in men, which may be consistent with the better prognosis of female patients with malignant melanoma (301).These evidences imply that ERβ acts as a tumor suppressor in skin. Based on in vitro studies, ERβ ligands inhibit NRAS mutation and thus the proliferation of melanoma cells, demonstrating that ERβ activation might weaken melanoma development by preventing the PI3K/Akt pathway (163). These results suggest that ERβ agonists may be an effective treatment strategy for NRAS-mutant melanomas.
GPER1 controls melanin production and is expressed in melanoma cells of the skin (302). Specifically, GPER1 mediates melanocyte growth, differentiation, and function via intracellular cAMP-protein kinase and cAMP response element-binding protein (CREB) phosphorylation (293). GPER1 agonists inhibit melanoma cell proliferation, providing a protective effect against melanoma (302). Furthermore, GPER co-expressed with ERβ in melanoma tended to present a superior outcome, with lower Breslow thickness and mitotic rate as well as higher peritumoral lymphocyte infiltration (164). GPER1 signaling enhances melanoma cell sensitization to immunotherapy. Systemic treatment with a GPER1 agonist combined with immune checkpoint blockade dramatically increased survival in melanoma-bearing mice, with up to 50% of the mice exhibiting tumor clearance (165).
In conclusion, ERα and ERβ exhibits pro-proliferative effects in melanoma by activating the downstream pathway like Akt and MAPK signaling pathways (Table 1; Figure 6). GPER1 shows inhibiting role and can be a treatment target in the melanoma.
Conclusions
Estrogens and their roles in ER signal transduction have become pivotal in developing various diseases, thereby making them potential therapeutic targets. ERs are expressed in various organs and regulate vital functions (203). We summarized the current knowledge on the role of ERs in health and their dysfunction, which leads to various diseases (Table 1, Figure 6). The diverse roles of ERs and their expression levels in various organs have been highlighted in our review. In some diseases like breast cancer, the expression condition and the role of ERs have been fully reported. ERα is normally considered to be a negative factor in the tumor growth, and ERβ inhibited the tumor by inhibiting the effects of ERα. In addition, the ERs show protective role in the bone, cardiovascular, and nervous systems. Nevertheless, the role of ERs in many diseases like gastric diseases remains unclear or controversial, indicating more studies is need to be conducted to further investigate the role of ERs in these diseases. In addition, different isoforms of ERs also exhibit significant roles in some disease like breast cancer, indicating more exploration on these isoforms needed to be conducted. Moreover, in the view of the different roles of ERs in the different organs or diseases, more comprehensive and specific should be done to reveal the synergistic or antagonistic action or more complex roles of ERs and their isoforms in different conditions. Furthermore, more new drugs targeting specific ERs should be developing in the therapy of relevant disease. Estrogen-mediated nuclear and cytoplasmic pathways are crucial in maintaining the physiology of multiple organ systems in both males and females. Further elucidation of the molecular mechanisms by which estrogen maintains homeostasis might provide deeper insights into the associated pathogenesis and clinical therapy pathways.
Author contributions
BL and LOY data curation; PC writing original draft preparation; PC and BL writing—review and editing; LOY Supervision; PC visualization; All authors have read and agreed to the published version of the manuscript.
Conflict of interest
The authors declare that the research was conducted in the absence of any commercial or financial relationships that could be construed as a potential conflict of interest.
Publisher’s note
All claims expressed in this article are solely those of the authors and do not necessarily represent those of their affiliated organizations, or those of the publisher, the editors and the reviewers. Any product that may be evaluated in this article, or claim that may be made by its manufacturer, is not guaranteed or endorsed by the publisher.
References
1. Fuentes N, Silveyra P. Estrogen receptor signaling mechanisms. Adv Protein Chem Struct Biol (2019) 116:135–70. doi: 10.1016/bs.apcsb.2019.01.001
2. Faltas CL, LeBron KA, Holz MK. Unconventional estrogen signaling in health and disease. Endocrinology (2020) 161(4):1–7. doi: 10.1210/endocr/bqaa030
3. Tang ZR, Zhang R, Lian ZX, Deng SL, Yu K. Estrogen-receptor expression and function in female reproductive disease. Cells (2019) 8(10):1123–38. doi: 10.3390/cells8101123
4. Flasch M, Bueschl C, Del Favero G, Adam G, Schuhmacher R, Marko D, et al. Elucidation of xenoestrogen metabolism by non-targeted, stable isotope-assisted mass spectrometry in breast cancer cells. Environ Int (2022) 158:106940. doi: 10.1016/j.envint.2021.106940
5. Ohta K, Chiba Y, Kaise A, Endo Y. Structure-activity relationship study of diphenylamine-based estrogen receptor (ER) antagonists. Bioorg Med Chem (2015) 23(4):861–7. doi: 10.1016/j.bmc.2014.12.022
6. Kumar RS, Goyal N. Estrogens as regulator of hematopoietic stem cell, immune cells and bone biology. Life Sci (2021) 269:119091. doi: 10.1016/j.lfs.2021.119091
7. Russell JK, Jones CK, Newhouse PA. The role of estrogen in brain and cognitive aging. Neurotherapeutics (2019) 16(3):649–65. doi: 10.1007/s13311-019-00766-9
8. Shang Y. Molecular mechanisms of oestrogen and SERMs in endometrial carcinogenesis. Nat Rev Cancer (2006) 6(5):360–8. doi: 10.1038/nrc1879
9. Bolger R, Wiese TE, Ervin K, Nestich S, Checovich W. Rapid screening of environmental chemicals for estrogen receptor binding capacity. Environ Health Perspect (1998) 106(9):551–7. doi: 10.1289/ehp.98106551
10. Delfosse V, Maire AL, Balaguer P, Bourguet W. A structural perspective on nuclear receptors as targets of environmental compounds. Acta Pharmacol Sin (2015) 36(1):88–101. doi: 10.1038/aps.2014.133
11. O'Donnell L, Robertson KM, Jones ME, Simpson ER. Estrogen and spermatogenesis. Endocr Rev (2001) 22(3):289–318. doi: 10.1210/er.22.3.289
12. Jensen EV, Suzuki T, Numata M, Smith S, DeSombre ER. Estrogen-binding substances of target tissues. Steroids (1969) 13(4):417–27. doi: 10.1016/0039-128X(69)90053-1
13. Walter P, Green S, Greene G, Krust A, Bornert JM, Jeltsch JM, et al. Cloning of the human estrogen receptor cDNA. Proc Natl Acad Sci (1985) 82(23):7889–93. doi: 10.1073/pnas.82.23.7889
14. Kuiper G, Enmark E, Pelto-Huikko M, Nilsson S, Gustafsson JA. Cloning of a novel receptor expressed in rat prostate and ovary. Proc Natl Acad Sci (1996) 93(12):5925–30. doi: 10.1073/pnas.93.12.5925
15. Filardo EJ, Thomas P. Minireview: G protein-coupled estrogen receptor-1, GPER-1: its mechanism of action and role in female reproductive cancer, renal and vascular physiology. Endocrinology (2012) 153(7):2953–62. doi: 10.1210/en.2012-1061
16. Carmeci C, Thompson DA, Ring HZ, Francke U, Weigel RJ. Identification of a gene (GPR30) with homology to the G-protein-coupled receptor superfamily associated with estrogen receptor expression in breast cancer. Genomics (1997) 45(3):607–17. doi: 10.1006/geno.1997.4972
17. Filardo EJ, Quinn JA, Bland KI, Frackelton AR Jr. Estrogen-induced activation of erk-1 and erk-2 requires the G protein-coupled receptor homolog, GPR30, and occurs via trans-activation of the epidermal growth factor receptor through release of HB-EGF. Mol Endocrinol (2000) 14(10):1649–60. doi: 10.1210/mend.14.10.0532
18. Maggiolini M, Vivacqua A, Fasanella G, Recchia AG, Sisci D, Pezzi V, et al. The G protein-coupled receptor GPR30 mediates c-fos up-regulation by 17beta-estradiol and phytoestrogens in breast cancer cells. J Biol Chem (2004) 279(26):27008–16. doi: 10.1074/jbc.M403588200
19. Thomas P, Pang Y, Filardo EJ, Dong J. Identity of an estrogen membrane receptor coupled to a G protein in human breast cancer cells. Endocrinology (2005) 146(2):624–32. doi: 10.1210/en.2004-1064
20. Revankar CM, Cimino DF, Sklar LA, Arterburn JB, Prossnitz ER. A transmembrane intracellular estrogen receptor mediates rapid cell signaling. Science (2005) 307(5715):1625–30. doi: 10.1126/science.1106943
21. Alexander SP, Mathie A, Peters JA. Guide to receptors and channels (GRAC), 5th edition. Br J Pharmacol (2011) 164 Suppl 1(Suppl 1):S1–324. doi: 10.1111/j.1476-5381.2011.01649_1.x
22. Luo J, Liu D. Does GPER really function as a G protein-coupled estrogen receptor in vivo? Front Endocrinol (Lausanne) (2020) 11:148. doi: 10.3389/fendo.2020.00148
23. Gosden JR, Middleton PG, Rout D. Localization of the human oestrogen receptor gene to chromosome 6q24–-q27 by in situ hybridization. Cytogenet Cell Genet (1986) 43(3-4):218–20. doi: 10.1159/000132325
24. Enmark E, Pelto-Huikko M, Grandien K, Lagercrantz S, Lagercrantz J, Fried G, et al. Human estrogen receptor β-gene structure, chromosomal localization, and expression pattern. J Clin Endocrinol Metab (1997) 82(12):4258–65. doi: 10.1210/jc.82.12.4258
25. Kumar R, Zakharov MN, Khan SH, Miki R, Jang H, Toraldo G, et al. The dynamic structure of the estrogen receptor. J Amino Acids (2011) 2011:812540. doi: 10.4061/2011/812540
26. Jia M, Dahlman-Wright K, Gustafsson J. Estrogen receptor alpha and beta in health and disease. Best Pract Res Clin Endocrinol Metab (2015) 29(4):557–68. doi: 10.1016/j.beem.2015.04.008
27. Flouriot G, Brand H, Denger S, Metivier R, Kos M, Reid G, et al. Identification of a new isoform of the human estrogen receptor-alpha (hER-α) that is encoded by distinct transcripts and that is able to repress hER-α activation function 1. EMBO J (2000) 19(17):4688–700. doi: 10.1093/emboj/19.17.4688
28. Wang Z, Zhang X, Shen P, Loggie BW, Chang Y, Deuel TF. Identification, cloning, and expression of human estrogen receptor-alpha36, a novel variant of human estrogen receptor-alpha66. Biochem Biophys Res Commun (2005) 336(4):1023–7. doi: 10.1016/j.bbrc.2005.08.226
29. Denger S, Reid G, Kos M, Flouriot G, Parsch D, Brand H, et al. ERalpha gene expression in human primary osteoblasts: evidence for the expression of two receptor proteins. Mol Endocrinol (2001) 15(12):2064–77. doi: 10.1210/mend.15.12.0741
30. Shi L, Dong B, Li Z, Lu Y, Ouyang T, Li J, et al. Expression of ER-{alpha}36, a novel variant of estrogen receptor {alpha}, and resistance to tamoxifen treatment in breast cancer. J Clin Oncol (2009) 27(21):3423–9. doi: 10.1200/JCO.2008.17.2254
31. Moore JT, McKee DD, Slentz-Kesler K, Moore LB, Jones SA, Horne EL, et al. Cloning and characterization of human estrogen receptor β isoforms. Biochem Biophys Res Commun (1998) 247(1):75–8. doi: 10.1006/bbrc.1998.8738
32. Warner M, Fan X, Strom A, Wu W, Gustafsson JÅ. 25 years of ERβ: a personal journey. J Mol Endocrinol (2021) 68(1):R1–r9. doi: 10.1530/JME-21-0121
33. Swedenborg E, Power KA, Cai W, Pongratz I, Rüegg J. Regulation of estrogen receptor beta activity and implications in health and disease. Cell Mol Life Sci (2009) 66(24):3873–94. doi: 10.1007/s00018-009-0118-z
34. Barton M, Filardo EJ, Lolait SJ, Thomas P, Maggiolini M, Prossnitz ER. Twenty years of the G protein-coupled estrogen receptor GPER: Historical and personal perspectives. J Steroid Biochem Mol Biol (2018) 176:4–15. doi: 10.1016/j.jsbmb.2017.03.021
35. Prossnitz ER, Barton M. Estrogen biology: new insights into GPER function and clinical opportunities. Mol Cell Endocrinol (2014) 389(1-2):71–83. doi: 10.1016/j.mce.2014.02.002
36. Olde B, Leeb-Lundberg LM. GPR30/GPER1: searching for a role in estrogen physiology. Trends Endocrinol Metab (2009) 20(8):409–16. doi: 10.1016/j.tem.2009.04.006
37. Mizukami Y. In vivo functions of GPR30/GPER-1, a membrane receptor for estrogen: from discovery to functions in vivo. Endocr J (2010) 57(2):101–7. doi: 10.1507/endocrj.K09E-332
38. Klein SL, Flanagan KL. Sex differences in immune responses. Nat Rev Immunol (2016) 16(10):626–38. doi: 10.1038/nri.2016.90
39. Rothenberger NJ, Somasundaram A, Stabile LP. The role of the estrogen pathway in the tumor microenvironment. Int J Mol Sci (2018) 19(2):611. doi: 10.3390/ijms19020611
40. Kovats S. Estrogen receptors regulate innate immune cells and signaling pathways. Cell Immunol (2015) 294(2):63–9. doi: 10.1016/j.cellimm.2015.01.018
41. Pelekanou V, Kampa M, Kiagiadaki F, Deli A, Theodoropoulos P, Agrogiannis G, et al. Estrogen anti-inflammatory activity on human monocytes is mediated through cross-talk between estrogen receptor ERα36 and GPR30/GPER1. J Leukoc Biol (2016) 99(2):333–47. doi: 10.1189/jlb.3A0914-430RR
42. Millas I, Duarte Barros M. Estrogen receptors and their roles in the immune and respiratory systems. Anat Rec (Hoboken) (2021) 304(6):1185–93. doi: 10.1002/ar.24612
43. Szostakowska M, Trębińska-Stryjewska A, Grzybowska EA, Fabisiewicz A. Resistance to endocrine therapy in breast cancer: molecular mechanisms and future goals. Breast Cancer Res Treat (2019) 173(3):489–97. doi: 10.1007/s10549-018-5023-4
44. Huang B, Omoto Y, Iwase H, Yamashita H, Toyama T, Coombes RC, et al. Differential expression of estrogen receptor α, β1, and β2 in lobular and ductal breast cancer. Proc Natl Acad Sci U S A (2014) 111(5):1933–8. doi: 10.1073/pnas.1323719111
45. Couse JF, Korach KS. Estrogen receptor null mice: what have we learned and where will they lead us? Endocr Rev (1999) 20(3):358–417. doi: 10.1210/edrv.20.3.0370
46. Anbalagan M, Rowan BG. Estrogen receptor alpha phosphorylation and its functional impact in human breast cancer. Mol Cell Endocrinol (2015) 418 Pt 3:264–72. doi: 10.1016/j.mce.2015.01.016
47. Droog M, Beelen K, Linn S, Zwart W. Tamoxifen resistance: from bench to bedside. Eur J Pharmacol (2013) 717(1-3):47–57. doi: 10.1016/j.ejphar.2012.11.071
48. Wang Q, Jiang J, Ying G, Xie XQ, Zhang X, Xu W, et al. Tamoxifen enhances stemness and promotes metastasis of ERα36(+) breast cancer by upregulating ALDH1A1 in cancer cells. Cell Res (2018) 28(3):336–58. doi: 10.1038/cr.2018.15
49. Zhao C, Lam EW, Sunters A, Enmark E, De Bella MT, Coombes RC, et al. Expression of estrogen receptor β isoforms in normal breast epithelial cells and breast cancer: regulation by methylation. Oncogene (2003) 22(48):7600–6. doi: 10.1038/sj.onc.1207100
50. Treeck O, Juhasz-Boess I, Lattrich C, Horn F, Goerse R, Ortmann O, et al. Effects of exon-deleted estrogen receptor β transcript variants on growth, apoptosis and gene expression of human breast cancer cell lines. Breast Cancer Res Treat (2008) 110(3):507–20. doi: 10.1007/s10549-007-9749-7
51. Omoto Y, Inoue S, Ogawa S, Toyama T, Yamashita H, Muramatsu M, et al. Clinical value of the wild-type estrogen receptor β expression in breast cancer. Cancer Lett (2001) 163(2):207–12. doi: 10.1016/S0304-3835(00)00680-7
52. Hopp TA, Weiss HL, Parra IS, Cui Y, Osborne CK, Fuqua SA. Low levels of estrogen receptor β protein predict resistance to tamoxifen therapy in breast cancer. Clin Cancer Res (2004) 10(22):7490–9. doi: 10.1158/1078-0432.CCR-04-1114
53. Cotrim C, Fabris V, Doria ML, Lindberg K, Gustafsson JÅ, Amado F, et al. Estrogen receptor beta growth-inhibitory effects are repressed through activation of MAPK and PI3K signalling in mammary epithelial and breast cancer cells. Oncogene (2013) 32(19):2390–402. doi: 10.1038/onc.2012.261
54. Speirs V, Malone C, Walton DS, Kerin MJ, Atkin SL. Increased expression of estrogen receptor β mRNA in tamoxifen-resistant breast cancer patients. Cancer Res (1999) 59(21):5421–4.
55. Markey G, Cullen R, Diggin P, Hill AD, Mc Dermott EW, O'Higgins NJ, et al. Estrogen receptor-β mRNA is associated with adverse outcome in patients with breast cancer. Tumor Biol (2009) 30(4):171–5. doi: 10.1159/000236409
56. Neill O, Davies MP, Shaaban AM, Innes H, Torevell A, Sibson DR, et al. Wild-type oestrogen receptor beta (ERbetaI) mRNA and protein expression in tamoxifen-treated post-menopausal breast cancers. Br J Cancer (2004) 91(9):1694–702. doi: 10.1038/sj.bjc.6602183
57. Shaaban AM, Green AR, Karthik S, Alizadeh Y, Hughes TA, Harkins L, et al. Nuclear and cytoplasmic expression of ERbeta1, ERbeta2, and ERbeta5 identifies distinct prognostic outcome for breast cancer patients. Clin Cancer Res (2008) 14(16):5228–35. doi: 10.1158/1078-0432.CCR-07-4528
58. Ignatov T, Weißenborn C, Poehlmann A, Lemke A, Semczuk A, Roessner A, et al. GPER-1 expression decreases during breast cancer tumorigenesis. Cancer Invest (2013) 31(5):309–15. doi: 10.3109/07357907.2013.789901
59. Treeck O, Schüler-Toprak S, Ortmann O. Estrogen actions in triple-negative breast cancer. Cells (2020) 9(11):2358–73. doi: 10.3390/cells9112358
60. Ignatov T, Treeck O, Kalinski T, Ortmann O, Ignatov A. GPER-1 expression is associated with a decreased response rate to primary tamoxifen therapy of breast cancer patients. Arch Gynecol Obstet (2020) 301(2):565–71. doi: 10.1007/s00404-019-05384-6
61. Liu L, Liu S, Luo H, Chen C, Zhang X, He L, et al. GPR30-mediated HMGB1 upregulation in CAFs induces autophagy and tamoxifen resistance in ERα-positive breast cancer cells. Aging (Albany NY) (2021) 13(12):16178–97. doi: 10.18632/aging.203145
62. Liang S, Chen Z, Jiang G, Zhou Y, Liu Q, Su Q, et al. Activation of GPER suppresses migration and angiogenesis of triple negative breast cancer via inhibition of NF-κB/IL-6 signals. Cancer Lett (2017) 386:12–23. doi: 10.1016/j.canlet.2016.11.003
63. Zhao Y, Gong P, Chen Y, Nwachukwu JC, Srinivasan S, Ko C, et al. Dual suppression of estrogenic and inflammatory activities for targeting of endometriosis. Sci Transl Med (2015) 7(271):271ra9. doi: 10.1126/scitranslmed.3010626
64. Han SJ, Jung SY, Wu SP, Hawkins SM, Park MJ, Kyo S, et al. Estrogen receptor β modulates apoptosis complexes and the inflammasome to drive the pathogenesis of endometriosis. Cell (2015) 163(4):960–74. doi: 10.1016/j.cell.2015.10.034
65. Imesch P, Samartzis EP, Dedes KJ, Fink D, Fedier A. Histone deacetylase inhibitors down-regulate G-protein-coupled estrogen receptor and the GPER-antagonist G-15 inhibits proliferation in endometriotic cells. Fertil Steril (2013) 100(3):770–6. doi: 10.1016/j.fertnstert.2013.05.008
66. Langdon SP, Herrington CS, Hollis RL, Gourley C. Estrogen signaling and its potential as a target for therapy in ovarian cancer. Cancers (Basel) (2020) 12(6):1647–64. doi: 10.3390/cancers12061647
67. Bossard C, Busson M, Vindrieux D, Gaudin F, Machelon V, Brigitte M, et al. Potential role of estrogen receptor beta as a tumor suppressor of epithelial ovarian cancer. PloS One (2012) 7(9):e44787. doi: 10.1371/journal.pone.0044787
68. Chan KKL, Siu MKY, Jiang YX, Wang JJ, Wang Y, Leung THY, et al. Differential expression of estrogen receptor subtypes and variants in ovarian cancer: effects on cell invasion, proliferation and prognosis. BMC Cancer (2017) 17(1):606. doi: 10.1186/s12885-017-3601-1
69. Ciucci A, Zannoni GF, Travaglia D, Petrillo M, Scambia G, Gallo D. Prognostic significance of the estrogen receptor beta (ERβ) isoforms ERβ1, ERβ2, and ERβ5 in advanced serous ovarian cancer. Gynecol Oncol (2014) 132(2):351–9. doi: 10.1016/j.ygyno.2013.12.027
70. Ignatov T, Modl S, Thulig M, Weißenborn C, Treeck O, Ortmann O, et al. GPER-1 acts as a tumor suppressor in ovarian cancer. J Ovarian Res (2013) 6(1):51. doi: 10.1186/1757-2215-6-51
71. Smith HO, Arias-Pulido H, Kuo DY, Howard T, Qualls CR, Lee SJ, et al. GPR30 predicts poor survival for ovarian cancer. Gynecol Oncol (2009) 114(3):465–71. doi: 10.1016/j.ygyno.2009.05.015
72. Zhu CX, Xiong W, Wang ML, Yang J, Shi HJ, Chen HQ, et al. Nuclear G protein-coupled oestrogen receptor (GPR30) predicts poor survival in patients with ovarian cancer. J Int Med Res (2018) 46(2):723–31. doi: 10.1177/0300060517717625
73. Yan Y, Jiang X, Zhao Y, Wen H, Liu G. Role of GPER on proliferation, migration and invasion in ligand-independent manner in human ovarian cancer cell line SKOV3. Cell Biochem Funct (2015) 33(8):552–9. doi: 10.1002/cbf.3154
74. Albanito L, Madeo A, Lappano R, Vivacqua A, Rago V, Carpino A, et al. G Protein-coupled receptor 30 (GPR30) mediates gene expression changes and growth response to 17beta-estradiol and selective GPR30 ligand G-1 in ovarian cancer cells. Cancer Res (2007) 67(4):1859–66. doi: 10.1158/0008-5472.CAN-06-2909
75. Marengo SR, Chung LW. An orthotopic model for the study of growth factors in the ventral prostate of the rat: effects of epidermal growth factor and basic fibroblast growth factor. J Androl (1994) 15(4):277–86.
76. Tørring N, Vinter-Jensen L, Pedersen SB, Sørensen FB, Flyvbjerg A, Nexø E, et al. Systemic administration of insulin-like growth factor I (IGF-I) causes growth of the rat prostate. J Urol (1997) 158(1):222–7. doi: 10.1097/00005392-199707000-00075
77. Bonkhoff H. Estrogen receptor signaling in prostate cancer: Implications for carcinogenesis and tumor progression. Prostate (2018) 78(1):2–10. doi: 10.1002/pros.23446
78. McPherson SJ, Hussain S, Balanathan P, Hedwards SL, Niranjan B, Grant M, et al. Estrogen receptor–β activated apoptosis in benign hyperplasia and cancer of the prostate is androgen independent and TNFα mediated. Proc Natl Acad Sci (2010) 107(7):3123–8. doi: 10.1073/pnas.0905524107
79. Ramírez-de-Arellano A, Pereira-Suárez AL, Rico-Fuentes C, López-Pulido EI, Villegas-Pineda JC, Sierra-Diaz E. Distribution and effects of estrogen receptors in prostate cancer: Associated molecular mechanisms. Front Endocrinol (2022) 12. doi: 10.3389/fendo.2021.811578
80. Chan QK, Lam HM, Ng CF, Lee AY, Chan ES, Ng HK, et al. Activation of GPR30 inhibits the growth of prostate cancer cells through sustained activation of Erk1/2, c-jun/c-fos-dependent upregulation of p21, and induction of G(2) cell-cycle arrest. Cell Death Differ (2010) 17(9):1511–23. doi: 10.1038/cdd.2010.20
81. Liu S, Le May C, Wong WP, Ward RD, Clegg DJ, Marcelli M, et al. Importance of extranuclear estrogen receptor-alpha and membrane G protein-coupled estrogen receptor in pancreatic islet survival. Diabetes (2009) 58(10):2292–302. doi: 10.2337/db09-0257
82. Bord S, Horner A, Beavan S, Compston J. Estrogen receptors alpha and beta are differentially expressed in developing human bone. J Clin Endocrinol Metab (2001) 86(5):2309–14. doi: 10.1210/jcem.86.5.7513
83. Sims NA, Dupont S, Krust A, Clement-Lacroix P, Minet D, Resche-Rigon M. Deletion of estrogen receptors reveals a regulatory role for estrogen receptors-beta in bone remodeling in females but not in males. Bone (2002) 30(1):18–25. doi: 10.1016/S8756-3282(01)00643-3
84. Windahl SH, Vidal O, Andersson G, Gustafsson JA, Ohlsson C. Increased cortical bone mineral content but unchanged trabecular bone mineral density in female ERbeta(-/-) mice. J Clin Invest (1999) 104(7):895–901. doi: 10.1172/JCI6730
85. Windahl SH, Hollberg K, Vidal O, Gustafsson JA, Ohlsson C, Andersson G. Female estrogen receptor beta-/- mice are partially protected against age-related trabecular bone loss. J Bone Miner Res (2001) 16(8):1388–98. doi: 10.1359/jbmr.2001.16.8.1388
86. Heino TJ, Chagin AS, Sävendahl L. The novel estrogen receptor G-protein-coupled receptor 30 is expressed in human bone. J Endocrinol (2008) 197(2):R1–6. doi: 10.1677/JOE-07-0629
87. Mårtensson UE, Salehi SA, Windahl S, Gomez MF, Swärd K, Daszkiewicz-Nilsson J, et al. Deletion of the G protein-coupled receptor 30 impairs glucose tolerance, reduces bone growth, increases blood pressure, and eliminates estradiol-stimulated insulin release in female mice. Endocrinology (2009) 150(2):687–98. doi: 10.1210/en.2008-0623
88. Ford J, Hajibeigi A, Long M, Hahner L, Gore C, Hsieh JT. GPR30 deficiency causes increased bone mass, mineralization, and growth plate proliferative activity in male mice. J Bone Miner Res (2011) 26(2):298–307. doi: 10.1002/jbmr.209
89. Taylor AH, Al-Azzawi F. Immunolocalisation of oestrogen receptor beta in human tissues. J Mol Endocrinol (2000) 24(1):145–55. doi: 10.1677/jme.0.0240145
90. He M, Yu W, Chang C, Miyamoto H, Liu X, Jiang K, et al. Estrogen receptor α promotes lung cancer cell invasion via increase of and cross-talk with infiltrated macrophages through the CCL2/CCR2/MMP9 and CXCL12/CXCR4 signaling pathways. Mol Oncol (2020) 14(8):1779–99. doi: 10.1002/1878-0261.12701
91. Liu S, Hu C, Li M, An J, Zhou W, Guo J, et al. Estrogen receptor beta promotes lung cancer invasion via increasing CXCR4 expression. Cell Death Dis (2022) 13(1):70. doi: 10.1038/s41419-022-04514-4
92. Liu C, Liao Y, Fan S, Tang H, Jiang Z, Zhou B, et al. G Protein-coupled estrogen receptor (GPER) mediates NSCLC progression induced by 17β-estradiol (E2) and selective agonist G1. Med Oncol (2015) 32(4):104. doi: 10.1007/s12032-015-0558-2
93. Wright AF, Ewart MA, Mair K, Nilsen M, Dempsie Y, Loughlin L, et al. Oestrogen receptor alpha in pulmonary hypertension. Cardiovasc Res (2015) 106(2):206–16. doi: 10.1093/cvr/cvv106
94. Ortmann J, Veit M, Zingg S, Di Santo S, Traupe T, Yang Z, et al. Estrogen receptor-α but not -β or GPER inhibits high glucose-induced human VSMC proliferation: potential role of ROS and ERK. J Clin Endocrinol Metab (2011) 96(1):220–8. doi: 10.1210/jc.2010-0943
95. Schubert C, Raparelli V, Westphal C, Dworatzek E, Petrov G, Kararigas G, et al. Reduction of apoptosis and preservation of mitochondrial integrity under ischemia/reperfusion injury is mediated by estrogen receptor β. Biol Sex Differ (2016) 7:53. doi: 10.1186/s13293-016-0104-8
96. Booth EA, Obeid NR, Lucchesi BR. Activation of estrogen receptor-alpha protects the in vivo rabbit heart from ischemia-reperfusion injury. Am J Physiol Heart Circ Physiol (2005) 289(5):H2039–47. doi: 10.1152/ajpheart.00479.2005
97. Zhai P, Eurell TE, Cooke PS, Lubahn DB, Gross DR. Myocardial ischemia-reperfusion injury in estrogen receptor-alpha knockout and wild-type mice. Am J Physiol Heart Circ Physiol (2000) 278(5):H1640–7. doi: 10.1152/ajpheart.2000.278.5.H1640
98. Westphal C, Schubert C, Prelle K, Penkalla A, Fliegner D, Petrov G, et al. Effects of estrogen, an ERα agonist and raloxifene on pressure overload induced cardiac hypertrophy. PloS One (2012) 7(12):e50802. doi: 10.1371/journal.pone.0050802
99. Mahmoodzadeh S, Eder S, Nordmeyer J, Ehler E, Huber O, Martus P, et al. Estrogen receptor alpha up-regulation and redistribution in human heart failure. FASEB J (2006) 20(7):926–34. doi: 10.1096/fj.05-5148com
100. Frump AL, Goss KN, Vayl A, Albrecht M, Fisher A, Tursunova R, et al. Estradiol improves right ventricular function in rats with severe angioproliferative pulmonary hypertension: effects of endogenous and exogenous sex hormones. Am J Physiol Lung Cell Mol Physiol (2015) 308(9):L873–90. doi: 10.1152/ajplung.00006.2015
101. Billon-Galés A, Fontaine C, Douin-Echinard V, Delpy L, Berges H, Calippe B, et al. Endothelial estrogen receptor-alpha plays a crucial role in the atheroprotective action of 17beta-estradiol in low-density lipoprotein receptor-deficient mice. Circulation (2009) 120(25):2567–76. doi: 10.1161/CIRCULATIONAHA.109.898445
102. Christian RC, Liu PY, Harrington S, Ruan M, Miller VM, Fitzpatrick LA. Intimal estrogen receptor (ER)beta, but not ERalpha expression, is correlated with coronary calcification and atherosclerosis in pre- and postmenopausal women. J Clin Endocrinol Metab (2006) 91(7):2713–20. doi: 10.1210/jc.2005-2672
103. Pelzer T, Loza PA, Hu K, Bayer B, Dienesch C, Calvillo L, et al. Increased mortality and aggravation of heart failure in estrogen receptor-beta knockout mice after myocardial infarction. Circulation (2005) 111(12):1492–8. doi: 10.1161/01.CIR.0000159262.18512.46
104. Iorga A, Umar S, Ruffenach G, Aryan L, Li J, Sharma S, et al. Estrogen rescues heart failure through estrogen receptor beta activation. Biol Sex Differ (2018) 9(1):48. doi: 10.1186/s13293-018-0206-6
105. Kararigas G, Fliegner D, Gustafsson JÅ, Regitz-Zagrosek V. Role of the estrogen/estrogen-receptor-beta axis in the genomic response to pressure overload-induced hypertrophy. Physiol Genomics (2011) 43(8):438–46. doi: 10.1152/physiolgenomics.00199.2010
106. Liu H, Pedram A, Kim JK. Oestrogen prevents cardiomyocyte apoptosis by suppressing p38α-mediated activation of p53 and by down-regulating p53 inhibition on p38β. Cardiovasc Res (2011) 89(1):119–28. doi: 10.1093/cvr/cvq265
107. Shen D, Tian L, Shen T, Sun H, Liu P. Alpha-lipoic acid protects human aortic endothelial cells against H2O2-induced injury and inhibits atherosclerosis in ovariectomized low density lipoprotein receptor knock-out mice. Cell Physiol Biochem (2018) 47(6):2261–77. doi: 10.1159/000491537
108. Umar S, Iorga A, Matori H, Nadadur RD, Li J, Maltese F, et al. Estrogen rescues preexisting severe pulmonary hypertension in rats. Am J Respir Crit Care Med (2011) 184(6):715–23. doi: 10.1164/rccm.201101-0078OC
109. Lahm T, Crisostomo PR, Markel TA, Wang M, Wang Y, Tan J, et al. Selective estrogen receptor-alpha and estrogen receptor-beta agonists rapidly decrease pulmonary artery vasoconstriction by a nitric oxide-dependent mechanism. Am J Physiol Regul Integr Comp Physiol (2008) 295(5):R1486–93. doi: 10.1152/ajpregu.90667.2008
110. Pedram A, Razandi M, Narayanan R, Levin ER. Estrogen receptor beta signals to inhibition of cardiac fibrosis. Mol Cell Endocrinol (2016) 434:57–68. doi: 10.1016/j.mce.2016.06.018
111. Farhat MY, Chen MF, Bhatti T, Iqbal A, Cathapermal S, Ramwell PW. Protection by oestradiol against the development of cardiovascular changes associated with monocrotaline pulmonary hypertension in rats. Br J Pharmacol (1993) 110(2):719–23. doi: 10.1111/j.1476-5381.1993.tb13871.x
112. Delgado NTB, Rouver WDN, Freitas-Lima LC, Vieira-Alves I, Lemos VS, Dos Santos RL. Sex differences in the vasodilation mediated by G protein-coupled estrogen receptor (GPER) in hypertensive rats. Front Physiol (2021) 12:659291. doi: 10.3389/fphys.2021.659291
113. Bopassa JC, Eghbali M, Toro L, Stefani E. A novel estrogen receptor GPER inhibits mitochondria permeability transition pore opening and protects the heart against ischemia-reperfusion injury. Am J Physiol Heart Circ Physiol (2010) 298(1):H16–23. doi: 10.1152/ajpheart.00588.2009
114. Feng Y, Madungwe NB, da Cruz Junho CV, Bopassa JC. Activation of G protein-coupled oestrogen receptor 1 at the onset of reperfusion protects the myocardium against ischemia/reperfusion injury by reducing mitochondrial dysfunction and mitophagy. Br J Pharmacol (2017) 174(23):4329–44. doi: 10.1111/bph.14033
115. Wang H, Sun X, Lin MS, Ferrario CM, Van Remmen H, Groban L. G Protein-coupled estrogen receptor (GPER) deficiency induces cardiac remodeling through oxidative stress. Transl Res (2018) 199:39–51. doi: 10.1016/j.trsl.2018.04.005
116. Da Silva JS, Sun X, Ahmad S, Wang H, Sudo RT, Varagic J, et al. G-Protein-Coupled estrogen receptor agonist G1 improves diastolic function and attenuates cardiac renin-angiotensin system activation in estrogen-deficient hypertensive rats. J Cardiovasc Pharmacol (2019) 74(5):443–52. doi: 10.1097/FJC.0000000000000721
117. Kang S, Liu Y, Sun D, Zhou C, Liu A, Xu C, et al. Chronic activation of the G protein-coupled receptor 30 with agonist G-1 attenuates heart failure. PloS One (2012) 7(10):e48185. doi: 10.1371/journal.pone.0048185
118. Wang H, Zhao Z, Lin M, Groban L. Activation of GPR30 inhibits cardiac fibroblast proliferation. Mol Cell Biochem (2015) 405(1-2):135–48. doi: 10.1007/s11010-015-2405-3
119. Dong J, Jiang SW, Niu Y, Chen L, Liu S, Ma T, et al. Expression of estrogen receptor α and β in esophageal squamous cell carcinoma. Oncol Rep (2013) 30(6):2771–6. doi: 10.3892/or.2013.2770
120. Zhang Z, He Q, Fu S, Zheng Z. Estrogen receptors in regulating cell proliferation of esophageal squamous cell carcinoma: Involvement of intracellular Ca(2+) signaling. Pathol Oncol Res (2017) 23(2):329–34. doi: 10.1007/s12253-016-0105-2
121. Zhang D, Ku J, Yi Y, Zhang J, Liu R, Tang N. The prognostic values of estrogen receptor alpha and beta in patients with gastroesophageal cancer: A meta-analysis. Med (Baltimore) (2019) 98(46):e17954. doi: 10.1097/MD.0000000000017954
122. Nozoe T, Oyama T, Takenoyama M, Hanagiri T, Sugio K, Yasumoto K. Significance of immunohistochemical expression of estrogen receptors alpha and beta in squamous cell carcinoma of the esophagus. Clin Cancer Res (2007) 13(14):4046–50. doi: 10.1158/1078-0432.CCR-07-0449
123. Ueo H, Matsuoka H, Sugimachi K, Kuwano H, Mori M, Akiyoshi T. Inhibitory effects of estrogen on the growth of a human esophageal carcinoma cell line. Cancer Res (1990) 50(22):7212–5.
124. Zuguchi M, Miki Y, Onodera Y, Fujishima F, Takeyama D, Okamoto H, et al. Estrogen receptor α and β in esophageal squamous cell carcinoma. Cancer Sci (2012) 103(7):1348–55. doi: 10.1111/j.1349-7006.2012.02288.x
125. Yang S, Deng L, Lai Y, Liu Z. Over expression of GPR30, indicating poor prognosis and promoting proliferation, upregulates beclin-1 expression via p38MAPK signaling in esophageal squamous cell carcinoma progression. Int J Clin Exp Pathol (2018) 11(7):3426–35.
126. Zhou J, Teng R, Xu C, Wang Q, Guo J, Xu C, et al. Overexpression of ERα inhibits proliferation and invasion of MKN28 gastric cancer cells by suppressing β-catenin. Oncol Rep (2013) 30(4):1622–30. doi: 10.3892/or.2013.2610
127. Tang W, Liu R, Yan Y, Pan X, Wang M, Han X, et al. Expression of estrogen receptors and androgen receptor and their clinical significance in gastric cancer. Oncotarget (2017) 8(25):40765–77. doi: 10.18632/oncotarget.16582
128. Deng H, Huang X, Fan J, Wang L, Xia Q, Yang X, et al. A variant of estrogen receptor-alpha, ER-alpha36 is expressed in human gastric cancer and is highly correlated with lymph node metastasis. Oncol Rep (2010) 24(1):171–6.
129. Ryu WS, Kim JH, Jang YJ, Park SS, Um JW, Park SH, et al. Expression of estrogen receptors in gastric cancer and their clinical significance. J Surg Oncol (2012) 106(4):456–61. doi: 10.1002/jso.23097
130. Zhou F, Jin J, Zhou L, Wu L, Cao Y, Yan H, et al. Suppression of estrogen receptor-beta promotes gastric cancer cell apoptosis with induction of autophagy. Am J Transl Res (2020) 12(8):4397–409.
131. Guo JL, Xu CY, Jiang ZN, Dong MJ, Xie SD, Shen JG, et al. Estrogen receptor beta variants mRNA expressions in gastric cancer tissues and association with clinicopathologic parameters. Hepatogastroenterology (2010) 57(104):1584–8.
132. Tian S, Zhan N, Li R, Dong W. Downregulation of G protein-coupled estrogen receptor (GPER) is associated with reduced prognosis in patients with gastric cancer. Med Sci Monit (2019) 25:3115–26. doi: 10.12659/MSM.913634
133. Zheng S, Yang L, Dai Y, Jiang L, Wei Y, Wen H, et al. Screening and survival analysis of hub genes in gastric cancer based on bioinformatics. J Comput Biol (2019) 26(11):1316–25. doi: 10.1089/cmb.2019.0119
134. Chen J, Iverson D. Estrogen in obesity-associated colon cancer: friend or foe? protecting postmenopausal women but promoting late-stage colon cancer. Cancer Causes Control (2012) 23(11):1767–73. doi: 10.1007/s10552-012-0066-z
135. Konstantinopoulos PA, Kominea A, Vandoros G, Sykiotis GP, Andricopoulos P, Varakis I, et al. Oestrogen receptor beta (ERβ) is abundantly expressed in normal colonic mucosa, but declines in colon adenocarcinoma paralleling the tumour's dedifferentiation. Eur J Cancer (2003) 39(9):1251–8. doi: 10.1016/S0959-8049(03)00239-9
136. Saleiro D, Murillo G, Benya RV, Bissonnette M, Hart J, Mehta RG. Estrogen receptor-β protects against colitis-associated neoplasia in mice. Int J Cancer (2012) 131(11):2553–61. doi: 10.1002/ijc.27578
137. Hases L, Indukuri R, Birgersson M, Nguyen-Vu T, Lozano R, Saxena A, et al. Intestinal estrogen receptor beta suppresses colon inflammation and tumorigenesis in both sexes. Cancer Lett (2020) 492:54–62. doi: 10.1016/j.canlet.2020.06.021
138. Qin B, Dong L, Guo X, Jiang J, He Y, Wang X, et al. Expression of G protein-coupled estrogen receptor in irritable bowel syndrome and its clinical significance. Int J Clin Exp Pathol (2014) 7(5):2238–46.
139. Zielińska M, Fichna J, Bashashati M, Habibi S, Sibaev A, Timmermans JP, et al. G Protein-coupled estrogen receptor and estrogen receptor ligands regulate colonic motility and visceral pain. Neurogastroenterol Motil (2017) 29(7):1–11. doi: 10.1111/nmo.13025
140. Hou J, Xu J, Jiang R, Wang Y, Chen C, Deng L, et al. Estrogen-sensitive PTPRO expression represses hepatocellular carcinoma progression by control of STAT3. Hepatology (2013) 57(2):678–88. doi: 10.1002/hep.25980
141. Dai B, Geng L, Yu Y, Sui C, Xie F, Shen W, et al. Methylation patterns of estrogen receptor α promoter correlate with estrogen receptor α expression and clinicopathological factors in hepatocellular carcinoma. Exp Biol Med (Maywood) (2014) 239(7):883–90. doi: 10.1177/1535370214536651
142. Chen JJ, Tang YS, Huang SF, Ai JG, Wang HX, Zhang LP. HBx protein-induced upregulation of microRNA-221 promotes aberrant proliferation in HBV−related hepatocellular carcinoma by targeting estrogen receptor-α. Oncol Rep (2015) 33(2):792–8. doi: 10.3892/or.2014.3647
143. Iyer JK, Kalra M, Kaul A, Payton ME, Kaul R. Estrogen receptor expression in chronic hepatitis c and hepatocellular carcinoma pathogenesis. World J Gastroenterol (2017) 23(37):6802–16. doi: 10.3748/wjg.v23.i37.6802
144. Yang W, Lu Y, Xu Y, Xu L, Zheng W, Wu Y, et al. Estrogen represses hepatocellular carcinoma (HCC) growth via inhibiting alternative activation of tumor-associated macrophages (TAMs). J Biol Chem (2012) 287(48):40140–9. doi: 10.1074/jbc.M112.348763
145. Lin YM, Velmurugan BK, Yeh YL, Tu CC, Ho TJ, Lai TY, et al. Activation of estrogen receptors with E2 downregulates peroxisome proliferator-activated receptor γ in hepatocellular carcinoma. Oncol Rep (2013) 30(6):3027–31. doi: 10.3892/or.2013.2793
146. Wei T, Chen W, Wen L, Zhang J, Zhang Q, Yang J, et al. G Protein-coupled estrogen receptor deficiency accelerates liver tumorigenesis by enhancing inflammation and fibrosis. Cancer Lett (2016) 382(2):195–202. doi: 10.1016/j.canlet.2016.08.012
147. Li Z, Chen L, Chu H, Wang W, Yang L, et al. Estrogen alleviates hepatocyte necroptosis depending on GPER in hepatic ischemia reperfusion injury. J Physiol Biochem (2021) 78(1):125–37. doi: 10.1007/s13105-021-00846-5
148. Liu SL, Wu XS, Li FN, Yao WY, Wu ZY, Dong P, et al. ERRα promotes pancreatic cancer progression by enhancing the transcription of PAI1 and activating the MEK/ERK pathway. Am J Cancer Res (2020) 10(11):3622–43.
149. Pozios I, Seel NN, Hering NA, Hartmann L, Liu V, Camaj P, et al. Raloxifene inhibits pancreatic adenocarcinoma growth by interfering with ERβ and IL-6/gp130/STAT3 signaling. Cell Oncol (Dordr) (2021) 44(1):167–77. doi: 10.1007/s13402-020-00559-9
150. Gupta VK, Banerjee S, Saluja AK. Learning from gender disparity: Role of estrogen receptor activation in coping with pancreatic cancer. Cell Mol Gastroenterol Hepatol (2020) 10(4):862–3. doi: 10.1016/j.jcmgh.2020.07.009
151. Natale CA, Li J, Pitarresi JR, Norgard RJ, Dentchev T, Capell BC, et al. Pharmacologic activation of the G protein-coupled estrogen receptor inhibits pancreatic ductal adenocarcinoma. Cell Mol Gastroenterol Hepatol (2020) 10(4):868–80.e1. doi: 10.1016/j.jcmgh.2020.04.016
152. Prins GS, Marmer M, Woodham C, Chang W, Kuiper G, Gustafsson JA, et al. Estrogen receptor-beta messenger ribonucleic acid ontogeny in the prostate of normal and neonatally estrogenized rats. Endocrinology (1998) 139(3):874–83. doi: 10.1210/endo.139.3.5827
153. Kauffman EC, Robinson BD, Downes M, Marcinkiewicz K, Vourganti S, Scherr DS, et al. Estrogen receptor-β expression and pharmacological targeting in bladder cancer. Oncol Rep (2013) 30(1):131–8. doi: 10.3892/or.2013.2416
154. Teng J, Wang ZY, Prossnitz ER, Bjorling DE. The G protein-coupled receptor GPR30 inhibits human urothelial cell proliferation. Endocrinology (2008) 149(8):4024–34. doi: 10.1210/en.2007-1669
155. Foster TC. Role of estrogen receptor alpha and beta expression and signaling on cognitive function during aging. Hippocampus (2012) 22(4):656–69. doi: 10.1002/hipo.20935
156. Ishunina TA, Swaab DF. Hippocampal estrogen receptor-alpha splice variant TADDI in the human brain in aging and alzheimer's disease. Neuroendocrinology (2009) 89(2):187–99. doi: 10.1159/000158573
157. Lai Y-J, Yu D, Zhang JH, Chen GJ. Cooperation of genomic and rapid nongenomic actions of estrogens in synaptic plasticity. Mol Neurobiol (2017) 54(6):4113–26. doi: 10.1007/s12035-016-9979-y
158. Chhibber A, Woody SK, Karim Rumi MA, Soares MJ, Zhao L. Estrogen receptor β deficiency impairs BDNF-5-HT(2A) signaling in the hippocampus of female brain: A possible mechanism for menopausal depression. Psychoneuroendocrinology (2017) 82:107–16. doi: 10.1016/j.psyneuen.2017.05.016
159. Lymer J, Robinson A, Winters BD, Choleris E. Rapid effects of dorsal hippocampal G-protein coupled estrogen receptor on learning in female mice. Psychoneuroendocrinology (2017) 77:131–40. doi: 10.1016/j.psyneuen.2016.11.019
160. Wang Z-F, Pan Z-Y, Xu C-S, Li Z-Q. Activation of G-protein coupled estrogen receptor 1 improves early-onset cognitive impairment via PI3K/Akt pathway in rats with traumatic brain injury. Biochem Biophys Res Commun (2017) 482(4):948–53. doi: 10.1016/j.bbrc.2016.11.138
161. Bessa A, Campos FL, Videira RA, Mendes-Oliveira J, Bessa-Neto D, Baltazar G. GPER: A new tool to protect dopaminergic neurons? Biochim Biophys Acta (BBA) - Mol Basis Dis (2015) 1852(10, Part A):2035–41. doi: 10.1016/j.bbadis.2015.07.004
162. Tian W, Pang W, Ge Y, He X, Wang D, Li X, et al. Hepatocyte-generated 27-hydroxycholesterol promotes the growth of melanoma by activation of estrogen receptor alpha. J Cell Biochem (2018) 119(3):2929–38. doi: 10.1002/jcb.26498
163. Marzagalli M, Montagnani Marelli M, Casati L, Fontana F, Moretti RM, Limonta P. Estrogen receptor β in melanoma: From molecular insights to potential clinical utility. Front Endocrinol (Lausanne) (2016) 7:140. doi: 10.3389/fendo.2016.00140
164. Fábián M, Rencz F, Krenács T, Brodszky V, Hársing J, Németh K, et al. Expression of G protein-coupled oestrogen receptor in melanoma and in pregnancy-associated melanoma. J Eur Acad Dermatol Venereol (2017) 31(9):1453–61. doi: 10.1111/jdv.14304
165. Natale CA, Li J, Zhang J, Dahal A, Dentchev T, Stanger BZ, et al. Activation of G protein-coupled estrogen receptor signaling inhibits melanoma and improves response to immune checkpoint blockade. Elife (2018) 7:e31770–89. doi: 10.7554/eLife.31770
166. Mińko A, Turoń-Skrzypińska A, Rył A, Bargiel P, Hilicka Z, Michalczyk K, et al. Endometriosis-a multifaceted problem of a modern woman. Int J Environ Res Public Health (2021) 18(15):8177–30. doi: 10.3390/ijerph18158177
167. Chantalat E, Valera MC, Vaysse C, Noirrit E, Rusidze M, Weyl A, et al. Estrogen receptors and endometriosis. Int J Mol Sci (2020) 21(8):2815–32. doi: 10.3390/ijms21082815
168. Enmark E, Pelto-Huikko M, Grandien K, Lagercrantz S, Lagercrantz J, Fried G, et al. Human estrogen receptor beta-gene structure, chromosomal localization, and expression pattern. J Clin Endocrinol Metab (1997) 82(12):4258–65. doi: 10.1210/jcem.82.12.4470
169. Pellegrini C, Gori I, Achtari C, Hornung D, Chardonnens E, Wunder D, et al. The expression of estrogen receptors as well as GREB1, c-MYC, and cyclin D1, estrogen-regulated genes implicated in proliferation, is increased in peritoneal endometriosis. Fertil Steril (2012) 98(5):1200–8. doi: 10.1016/j.fertnstert.2012.06.056
170. Samartzis N, Samartzis EP, Noske A, Fedier A, Dedes KJ, Caduff R, et al. Expression of estrogen receptor alpha and beta in peritoneal and ovarian endometriosis. Fertil Steril (2001) 75(6):1198–205. doi: 10.1016/S0015-0282(01)01783-6
171. Qiu JJ, Ye LC, Ding JX, Feng WW, Jin HY, Zhang Y, et al. Expression and clinical significance of estrogen-regulated long non-coding RNAs in estrogen receptor α-positive ovarian cancer progression. Oncol Rep (2014) 31(4):1613–22. doi: 10.3892/or.2014.3000
172. Cairns J, Ingle JN, Kalari KR, Shepherd LE, Kubo M, Goetz MP, et al. The lncRNA MIR2052HG regulates ERα levels and aromatase inhibitor resistance through LMTK3 by recruiting EGR1. Breast Cancer Res (2019) 21(1):47. doi: 10.1186/s13058-019-1130-3
173. Cheng CW, Licence D, Cook E, Luo F, Arends MJ, Smith SK, et al. Activation of mutated K-ras in donor endometrial epithelium and stroma promotes lesion growth in an intact immunocompetent murine model of endometriosis. J Pathol (2011) 224(2):261–9. doi: 10.1002/path.2852
174. Xue Q, Lin Z, Cheng YH, Huang CC, Marsh E, Yin P, et al. Promoter methylation regulates estrogen receptor 2 in human endometrium and endometriosis. Biol Reprod (2007) 77(4):681–7. doi: 10.1095/biolreprod.107.061804
175. Yotova I, Hsu E, Do C, Gaba A, Sczabolcs M, Dekan S, et al. Epigenetic alterations affecting transcription factors and signaling pathways in stromal cells of endometriosis. PloS One (2017) 12(1):e0170859. doi: 10.1371/journal.pone.0170859
176. Heublein S, Vrekoussis T, Kuhn C, Friese K, Makrigiannakis A, Mayr D, et al. Inducers of G-protein coupled estrogen receptor (GPER) in endometriosis: potential implications for macrophages and follicle maturation. J Reprod Immunol (2013) 97(1):95–103. doi: 10.1016/j.jri.2012.10.013
177. Samartzis N, Samartzis EP, Noske A, Fedier A, Dedes KJ, Caduff R, et al. Expression of the G protein-coupled estrogen receptor (GPER) in endometriosis: a tissue microarray study. Reprod Biol Endocrinol (2012) 10:30. doi: 10.1186/1477-7827-10-30
178. Suzuki K, Inaba S, Takeuchi H, Takezawa Y, Fukabori Y, Suzuki T, et al. Endocrine environment of benign prostatic hyperplasia–relationships of sex steroid hormone levels with age and the size of the prostate. Nihon Hinyokika Gakkai Zasshi (1992) 83(5):664–71. doi: 10.5980/jpnjurol1989.83.664
179. Gupta L, Thakur H, Sobti RC, Seth A, Singh SK. Role of genetic polymorphism of estrogen receptor-alpha gene and risk of prostate cancer in north Indian population. Mol Cell Biochem (2010) 335(1-2):255–61. doi: 10.1007/s11010-009-0275-2
180. Di Zazzo E, Galasso G, Giovannelli P, Di Donato M, Di Santi A, Cernera G, et al. Prostate cancer stem cells: the role of androgen and estrogen receptors. Oncotarget (2016) 7(1):193–208. doi: 10.18632/oncotarget.6220
181. Lau KM, To KF. Importance of estrogenic signaling and its mediated receptors in prostate cancer. Int J Mol Sci (2016) 17(9):1434–59. doi: 10.3390/ijms17091434
182. Ricke WA, McPherson SJ, Bianco JJ, Cunha GR, Wang Y, Risbridger GP. Prostatic hormonal carcinogenesis is mediated by in situ estrogen production and estrogen receptor alpha signaling. FASEB J (2008) 22(5):1512–20. doi: 10.1096/fj.07-9526com
183. Bonkhoff H, Fixemer T, Hunsicker I, Remberger K. Estrogen receptor expression in prostate cancer and premalignant prostatic lesions. Am J Pathol (1999) 155(2):641–7. doi: 10.1016/S0002-9440(10)65160-7
184. Singh PB, Matanhelia SS, Martin FL. A potential paradox in prostate adenocarcinoma progression: oestrogen as the initiating driver. Eur J Cancer (2008) 44(7):928–36. doi: 10.1016/j.ejca.2008.02.051
185. Fixemer T, Remberger K, Bonkhoff H. Differential expression of the estrogen receptor beta (ERbeta) in human prostate tissue, premalignant changes, and in primary, metastatic, and recurrent prostatic adenocarcinoma. Prostate (2003) 54(2):79–87. doi: 10.1002/pros.10171
186. Leav I, Lau KM, Adams JY, McNeal JE, Taplin ME, Wang J, et al. Comparative studies of the estrogen receptors beta and alpha and the androgen receptor in normal human prostate glands, dysplasia, and in primary and metastatic carcinoma. Am J Pathol (2001) 159(1):79–92. doi: 10.1016/S0002-9440(10)61676-8
187. Qu LG, Wardan H, Davis ID, Iddawela M, Sluka P, Pezaro CJ. Circulating oestrogen receptor mutations and splice variants in advanced prostate cancer. BJU Int (2019) 124 Suppl 1:50–6. doi: 10.1111/bju.14797
188. Jilka RL, Hangoc G, Girasole G, Passeri G, Williams DC, Abrams JS, et al. Increased osteoclast development after estrogen loss: Mediation by interleukin-6. Science (1992) 257(5066):88–91. doi: 10.1126/science.1621100
189. Pantschenko AG, Zhang W, Nahounou M, McCarthy MB, Stover ML, Lichtler AC, et al. Effect of osteoblast-targeted expression of bcl-2 in bone: differential response in male and female mice. J Bone Miner Res (2005) 20(8):1414–29. doi: 10.1359/JBMR.050315
190. Cauley JA. Estrogen and bone health in men and women. Steroids (2015) 99:11–5. doi: 10.1016/j.steroids.2014.12.010
191. Härkönen PL, Väänänen HK. Monocyte-macrophage system as a target for estrogen and selective estrogen receptor modulators. Ann N Y Acad Sci (2006) 1089:218–27. doi: 10.1196/annals.1386.045
192. Pacifici R. Estrogen deficiency, T cells and bone loss. Cell Immunol (2008) 252(1-2):68–80. doi: 10.1016/j.cellimm.2007.06.008
193. de Giorgi V, Mavilia C, Massi D, Gozzini A, Aragona P, Tanini A, et al. Estrogen receptor expression in cutaneous melanoma: a real-time reverse transcriptase-polymerase chain reaction and immunohistochemical study. Arch Dermatol (2009) 145(1):30–6. doi: 10.1001/archdermatol.2008.537
194. Lee KC, Jessop H, Suswillo R, Zaman G, Lanyon LE. The adaptive response of bone to mechanical loading in female transgenic mice is deficient in the absence of oestrogen receptor-alpha and -beta. J Endocrinol (2004) 182(2):193–201. doi: 10.1677/joe.0.1820193
195. Kang WB, Deng YT, Wang DS, Feng D, Liu Q, Wang XS, et al. Osteoprotective effects of estrogen membrane receptor GPR30 in ovariectomized rats. J Steroid Biochem Mol Biol (2015) 154:237–44. doi: 10.1016/j.jsbmb.2015.07.002
196. Iravani M, Lagerquist MK, Karimian E, Chagin AS, Ohlsson C, Sävendahl L. Effects of the selective GPER1 agonist G1 on bone growth. Endocr Connect (2019) 8(9):1302–9. doi: 10.1530/EC-19-0274
197. Siegfried JM. Women and lung cancer: does oestrogen play a role? Lancet Oncol (2001) 2(8):506–13. doi: 10.1016/S1470-2045(01)00457-0
198. Landis SH, Murray T, Bolden S, Wingo PA. Cancer statistics, 1999. CA Cancer J Clin (1999) 49(1):8–31, 1. doi: 10.3322/canjclin.49.1.8
199. Hsu LH, Liu KJ, Tsai MF, Wu CR, Feng AC, Chu NM, et al. Estrogen adversely affects the prognosis of patients with lung adenocarcinoma. Cancer Sci (2015) 106(1):51–9. doi: 10.1111/cas.12558
200. Mollerup S, Jørgensen K, Berge G, Haugen A. Expression of estrogen receptors alpha and beta in human lung tissue and cell lines. Lung Cancer (2002) 37(2):153–9. doi: 10.1016/S0169-5002(02)00039-9
201. Kawai H, Ishii A, Washiya K, Konno T, Kon H, Yamaya C, et al. Estrogen receptor alpha and beta are prognostic factors in non-small cell lung cancer. Clin Cancer Res (2005) 11(14):5084–9. doi: 10.1158/1078-0432.CCR-05-0200
202. Carey MA, Card JW, Voltz JW, Germolec DR, Korach KS, Zeldin DC. The impact of sex and sex hormones on lung physiology and disease: lessons from animal studies. Am J Physiol Lung Cell Mol Physiol (2007) 293(2):L272–8. doi: 10.1152/ajplung.00174.2007
203. Brandenberger AW, Tee MK, Lee JY, Chao V, Jaffe RB. Tissue distribution of estrogen receptors alpha (ER-alpha) and beta (ER-beta) mRNA in the midgestational human fetus. J Clin Endocrinol Metab (1997) 82(10):3509–12. doi: 10.1210/jcem.82.10.4400
204. Morani A, Barros RP, Imamov O, Hultenby K, Arner A, Warner M, et al. Lung dysfunction causes systemic hypoxia in estrogen receptor beta knockout (ERbeta-/-) mice. Proc Natl Acad Sci U.S.A. (2006) 103(18):7165–9. doi: 10.1073/pnas.0602194103
205. Omoto Y, Kobayashi Y, Nishida K, Tsuchiya E, Eguchi H, Nakagawa K, et al. Expression, function, and clinical implications of the estrogen receptor beta in human lung cancers. Biochem Biophys Res Commun (2001) 285(2):340–7. doi: 10.1006/bbrc.2001.5158
206. Baik CS, Eaton KD. Estrogen signaling in lung cancer: an opportunity for novel therapy. Cancers (Basel) (2012) 4(4):969–88. doi: 10.3390/cancers4040969
207. Kawai H. Estrogen receptors as the novel therapeutic biomarker in non-small cell lung cancer. World J Clin Oncol (2014) 5(5):1020–7. doi: 10.5306/wjco.v5.i5.1020
208. Leung YK, Mak P, Hassan S, Ho SM. Estrogen receptor (ER)-beta isoforms: a key to understanding ER-beta signaling. Proc Natl Acad Sci U.S.A. (2006) 103(35):13162–7. doi: 10.1073/pnas.0605676103
209. Bai Y, Shen W, Zhang L, Yang Z, Xiong L, Tang H, et al. Oestrogen receptor β5 and epidermal growth factor receptor synergistically promote lung cancer progression. Autoimmunity (2018) 51(4):157–65. doi: 10.1080/08916934.2018.1486825
210. Jala VR, Radde BN, Haribabu B, Klinge CM. Enhanced expression of G-protein coupled estrogen receptor (GPER/GPR30) in lung cancer. BMC Cancer (2012) 12:624. doi: 10.1186/1471-2407-12-624
211. Słowikowski BK, Lianeri M, Jagodziński PP. Exploring estrogenic activity in lung cancer. Mol Biol Rep (2017) 44(1):35–50. doi: 10.1007/s11033-016-4086-8
212. Kurt AH, Çelik A, Kelleci BM. Oxidative/antioxidative enzyme-mediated antiproliferative and proapoptotic effects of the GPER1 agonist G-1 on lung cancer cells. Oncol Lett (2015) 10(5):3177–82. doi: 10.3892/ol.2015.3711
213. Iorga A, Cunningham CM, Moazeni S, Ruffenach G, Umar S, Eghbali M. The protective role of estrogen and estrogen receptors in cardiovascular disease and the controversial use of estrogen therapy. Biol Sex Differ (2017) 8(1):33. doi: 10.1186/s13293-017-0152-8
214. Savarese G, Lund LH. Global public health burden of heart failure. Card Fail Rev (2017) 3(1):7–11. doi: 10.15420/cfr.2016:25:2
215. Hayward CS, Kelly RP, Collins P. The roles of gender, the menopause and hormone replacement on cardiovascular function. Cardiovasc Res (2000) 46(1):28–49. doi: 10.1016/S0008-6363(00)00005-5
216. Aryan L, Younessi D, Zargari M, Banerjee S, Agopian J, Rahman S, et al. The role of estrogen receptors in cardiovascular disease. Int J Mol Sci (2020) 21(12):4314. doi: 10.3390/ijms21124314
217. Wang M, Crisostomo P, Wairiuko GM, Meldrum DR. Estrogen receptor-alpha mediates acute myocardial protection in females. Am J Physiol Heart Circ Physiol (2006) 290(6):H2204–9. doi: 10.1152/ajpheart.01219.2005
218. Hamada H, Kim MK, Iwakura A, Ii M, Thorne T, Qin G, et al. Estrogen receptors alpha and beta mediate contribution of bone marrow-derived endothelial progenitor cells to functional recovery after myocardial infarction. Circulation (2006) 114(21):2261–70. doi: 10.1161/CIRCULATIONAHA.106.631465
219. Lyle AN, Griendling KK. Modulation of vascular smooth muscle signaling by reactive oxygen species. Physiol (Bethesda) (2006) 21:269–80. doi: 10.1152/physiol.00004.2006
220. Zhu L, Shi J, Luu TN, Neuman JC, Trefts E, Yu S, et al. Hepatocyte estrogen receptor alpha mediates estrogen action to promote reverse cholesterol transport during Western-type diet feeding. Mol Metab (2018) 8:106–16. doi: 10.1016/j.molmet.2017.12.012
221. Mendelsohn ME. Protective effects of estrogen on the cardiovascular system. Am J Cardiol (2002) 89(12a):12E–17E; discussion 17E-18E. doi: 10.1016/S0002-9149(02)02405-0
222. McRobb LS, Shi J, Luu TN, Neuman JC, Trefts E, Yu S, et al. Estrogen receptor control of atherosclerotic calcification and smooth muscle cell osteogenic differentiation. Arterioscler Thromb Vasc Biol (2017) 37(6):1127–37. doi: 10.1161/ATVBAHA.117.309054
223. Owens GK, Kumar MS, Wamhoff BR. Molecular regulation of vascular smooth muscle cell differentiation in development and disease. Physiol Rev (2004) 84(3):767–801. doi: 10.1152/physrev.00041.2003
224. Smirnova NF, Fontaine C, Buscato M, Lupieri A, Vinel A, Valera MC, et al. The activation function-1 of estrogen receptor alpha prevents arterial neointima development through a direct effect on smooth muscle cells. Circ Res (2015) 117(9):770–8. doi: 10.1161/CIRCRESAHA.115.306416
225. Menazza S, Murphy E. The expanding complexity of estrogen receptor signaling in the cardiovascular system. Circ Res (2016) 118(6):994–1007. doi: 10.1161/CIRCRESAHA.115.305376
226. Fredette NC, Meyer MR, Prossnitz ER. Role of GPER in estrogen-dependent nitric oxide formation and vasodilation. J Steroid Biochem Mol Biol (2018) 176:65–72. doi: 10.1016/j.jsbmb.2017.05.006
227. Chen C, Gong X, Yang X, Shang X, Du Q, Liao Q, et al. The roles of estrogen and estrogen receptors in gastrointestinal disease. Oncol Lett (2019) 18(6):5673–80. doi: 10.3892/ol.2019.10983
228. Kurt D, Saruhan BG, Kanay Z, Yokus B, Kanay BE, Unver O, et al. Effect of ovariectomy and female sex hormones administration upon gastric ulceration induced by cold and immobility restraint stress. Saudi Med J (2007) 28(7):1021–7.
229. Sangma TK, Jain S, Mediratta PK. Effect of ovarian sex hormones on non-steroidal anti-inflammatory drug-induced gastric lesions in female rats. Indian J Pharmacol (2014) 46(1):113–6. doi: 10.4103/0253-7613.125191
230. Meleine M, Matricon J. Gender-related differences in irritable bowel syndrome: potential mechanisms of sex hormones. World J Gastroenterol (2014) 20(22):6725–43. doi: 10.3748/wjg.v20.i22.6725
231. Bustos V, Nolan ÁM, Nijhuis A, Harvey H, Parker A, Poulsom R, et al. GPER mediates differential effects of estrogen on colon cancer cell proliferation and migration under normoxic and hypoxic conditions. Oncotarget (2017) 8(48):84258–75. doi: 10.18632/oncotarget.20653
232. Iijima K, Shimosegawa T. Involvement of luminal nitric oxide in the pathogenesis of the gastroesophageal reflux disease spectrum. J Gastroenterol Hepatol (2014) 29(5):898–905. doi: 10.1111/jgh.12548
233. Vizcaino AP, Moreno V, Lambert R, Parkin DM. Time trends incidence of both major histologic types of esophageal carcinomas in selected countries, 1973-1995. Int J Cancer (2002) 99(6):860–8. doi: 10.1002/ijc.10427
234. Boeckxstaens G, El-Serag HB, Smout AJ, Kahrilas PJ. Symptomatic reflux disease: the present, the past and the future. Gut (2014) 63(7):1185–93. doi: 10.1136/gutjnl-2013-306393
235. Wang BJ, Zhang B, Yan SS, Li ZC, Jiang T, Hua CJ, et al. Hormonal and reproductive factors and risk of esophageal cancer in women: a meta-analysis. Dis Esophagus (2016) 29(5):448–54. doi: 10.1111/dote.12349
236. Wang QM, Yuan L, Qi YJ, Ma ZY, Wang LD. Estrogen analogues: promising target for prevention and treatment of esophageal squamous cell carcinoma in high risk areas. Med Sci Monit (2010) 16(7):Hy19–22.
237. Nilsson M, Johnsen R, Ye W, Hveem K, Lagergren J. Obesity and estrogen as risk factors for gastroesophageal reflux symptoms. Jama (2003) 290(1):66–72. doi: 10.1001/jama.290.1.66
238. Okada K, Inamori M, Imajyo K, Chiba H, Nonaka T, Shiba T, et al. Gender differences of low-dose aspirin-associated gastroduodenal ulcer in Japanese patients. World J Gastroenterol (2010) 16(15):1896–900. doi: 10.3748/wjg.v16.i15.1896
239. Speir E, Yu ZX, Takeda K, Ferrans VJ, Cannon RO 3rd. Antioxidant effect of estrogen on cytomegalovirus-induced gene expression in coronary artery smooth muscle cells. Circulation (2000) 102(24):2990–6. doi: 10.1161/01.CIR.102.24.2990
240. Chung HW, Noh SH, Lim JB. Analysis of demographic characteristics in 3242 young age gastric cancer patients in Korea. World J Gastroenterol (2010) 16(2):256–63. doi: 10.3748/wjg.v16.i2.256
241. Tokunaga A, Kojima N, Andoh T, Matsukura N, Yoshiyasu M, Tanaka N, et al. Hormone receptors in gastric cancer. Eur J Cancer Clin Oncol (1983) 19(5):687–9. doi: 10.1016/0277-5379(83)90186-4
242. Furukawa H, Iwanaga T, Koyama H, Taniguchi H. Effect of sex hormones on the experimental induction of cancer in rat stomach - a preliminary study. Digestion (1982) 23(3):151–5. doi: 10.1159/000198722
243. Wang M, Pan JY, Song GR, Chen HB, An LJ, Qu SX. Altered expression of estrogen receptor alpha and beta in advanced gastric adenocarcinoma: correlation with prothymosin alpha and clinicopathological parameters. Eur J Surg Oncol (2007) 33(2):195–201. doi: 10.1016/j.ejso.2006.09.009
244. Takano N, Iizuka N, Hazama S, Yoshino S, Tangoku A, Oka M. Expression of estrogen receptor-alpha and -beta mRNAs in human gastric cancer. Cancer Lett (2002) 176(2):129–35. doi: 10.1016/S0304-3835(01)00739-X
245. Qin J, Liu M, Ding Q, Ji X, Hao Y, Wu X, et al. The direct effect of estrogen on cell viability and apoptosis in human gastric cancer cells. Mol Cell Biochem (2014) 395(1-2):99–107. doi: 10.1007/s11010-014-2115-2
246. Fu Z, Wang X, Zhou H, Li Y, Chen Y, Wang Z, et al. GRP78 positively regulates estrogen−stimulated cell growth mediated by ER−α36 in gastric cancer cells. Mol Med Rep (2017) 16(6):8329–34. doi: 10.3892/mmr.2017.7615
247. Fu Z, Deng H, Wang X, Yang X, Wang Z, Liu L, et al. Involvement of ER-α36 in the malignant growth of gastric carcinoma cells is associated with GRP94 overexpression. Histopathology (2013) 63(3):325–33. doi: 10.1111/his.12171
248. Heitkemper MM, Chang L. Do fluctuations in ovarian hormones affect gastrointestinal symptoms in women with irritable bowel syndrome? Gender Med (2009) 6 Suppl 2(Suppl 2):152–67. doi: 10.1016/j.genm.2009.03.004
249. Cook LC, Hillhouse AE, Myles MH, Lubahn DB, Bryda EC, Davis JW, et al. The role of estrogen signaling in a mouse model of inflammatory bowel disease: a helicobacter hepaticus model. PloS One (2014) 9(4):e94209. doi: 10.1371/journal.pone.0094209
250. Principi M, Barone M, Pricci M, De Tullio N, Losurdo G, Ierardi E, et al. Ulcerative colitis: from inflammation to cancer. do estrogen receptors have a role? World J Gastroenterol (2014) 20(33):11496–504. doi: 10.3748/wjg.v20.i33.11496
251. Looijer-van Langen M, Hotte N, Dieleman LA, Albert E, Mulder C, Madsen KL, et al. Estrogen receptor-β signaling modulates epithelial barrier function. Am J Physiol Gastrointest Liver Physiol (2011) 300(4):G621–6. doi: 10.1152/ajpgi.00274.2010
252. Jiang H, Teng R, Wang Q, Zhang X, Wang H, Wang Z, et al. Transcriptional analysis of estrogen receptor alpha variant mRNAs in colorectal cancers and their matched normal colorectal tissues. J Steroid Biochem Mol Biol (2008) 112(1-3):20–4. doi: 10.1016/j.jsbmb.2008.07.004
253. Campbell-Thompson M, Lynch IJ, Bhardwaj B. Expression of estrogen receptor (ER) subtypes and ERbeta isoforms in colon cancer. Cancer Res (2001) 61(2):632–40.
254. Wong NA, Malcomson RD, Jodrell DI, Groome NP, Harrison DJ, Saunders PT, et al. ERbeta isoform expression in colorectal carcinoma: an in vivo and in vitro study of clinicopathological and molecular correlates. J Pathol (2005) 207(1):53–60. doi: 10.1002/path.1807
255. Wang AG, Lee KY, Kim SY, Choi JY, Lee KH, Kim WH, et al. The expression of estrogen receptors in hepatocellular carcinoma in Korean patients. Yonsei Med J (2006) 47(6):811–6. doi: 10.3349/ymj.2006.47.6.811
256. De Maria N, Manno M, Villa E. Sex hormones and liver cancer. Mol Cell Endocrinol (2002) 193(1-2):59–63. doi: 10.1016/S0303-7207(02)00096-5
257. Ezhilarasan D. Critical role of estrogen in the progression of chronic liver diseases. Hepatobil Pancreat Dis Int (2020) 19(5):429–34. doi: 10.1016/j.hbpd.2020.03.011
258. Baldissera VD, Alves AF, Almeida S, Porawski M, Giovenardi M. Hepatocellular carcinoma and estrogen receptors: Polymorphisms and isoforms relations and implications. Med Hypotheses (2016) 86:67–70. doi: 10.1016/j.mehy.2015.11.030
259. Kao TL, Kuan YP, Cheng WC, Chang WC, Jeng LB, Yeh S, et al. Estrogen receptors orchestrate cell growth and differentiation to facilitate liver regeneration. Theranostics (2018) 8(10):2672–82. doi: 10.7150/thno.23624
260. Hishida M, Nomoto S, Inokawa Y, Hayashi M, Kanda M, Okamura Y, et al. Estrogen receptor 1 gene as a tumor suppressor gene in hepatocellular carcinoma detected by triple-combination array analysis. Int J Oncol (2013) 43(1):88–94. doi: 10.3892/ijo.2013.1951
261. Li CL, Yeh KH, Liu WH, Chen CL, Chen DS, Chen PJ, et al. Elevated p53 promotes the processing of miR-18a to decrease estrogen receptor-α in female hepatocellular carcinoma. Int J Cancer (2015) 136(4):761–70. doi: 10.1002/ijc.29052
262. Han SJ, Xu GL, Jia WD, Wang YC, Li JS, Ma JL, et al. [Expression of estrogen receptor α in hepatitis b-related hepatocellular carcinoma and its clinical significance]. Zhonghua Wai Ke Za Zhi (2010) 48(24):1875–80.
263. Villa E, Colantoni A, Grottola A, Ferretti I, Buttafoco P, Bertani H, et al. Variant estrogen receptors and their role in liver disease. Mol Cell Endocrinol (2002) 193(1-2):65–9. doi: 10.1016/S0303-7207(02)00097-7
264. Qin H, Song Z, Shaukat H, Zheng W. Genistein regulates lipid metabolism via estrogen receptor β and its downstream signal Akt/mTOR in HepG2 cells. Nutrients (2021) 13(11):4015–29. doi: 10.3390/nu13114015
265. Lin L, Zhou M, Que R, Chen Y, Liu X, Zhang K, et al. Saikosaponin-d protects against liver fibrosis by regulating the estrogen receptor-β/NLRP3 inflammasome pathway. Biochem Cell Biol (2021) 99(5):666–74. doi: 10.1139/bcb-2020-0561
266. Zhang B, Zhang CG, Ji LH, Zhao G, Wu ZY. Estrogen receptor β selective agonist ameliorates liver cirrhosis in rats by inhibiting the activation and proliferation of hepatic stellate cells. J Gastroenterol Hepatol (2018) 33(3):747–55. doi: 10.1111/jgh.13976
267. Mauvais-Jarvis F, Clegg DJ, Hevener AL. The role of estrogens in control of energy balance and glucose homeostasis. Endocr Rev (2013) 34(3):309–38. doi: 10.1210/er.2012-1055
268. Satake M, Sawai H, Go VL, Satake K, Reber HA, Hines OJ, et al. Estrogen receptors in pancreatic tumors. Pancreas (2006) 33(2):119–27. doi: 10.1097/01.mpa.0000226893.09194.ec
269. Estrella JS, Ma LT, Milton DR, Yao JC, Wang H, Rashid A, et al. Expression of estrogen-induced genes and estrogen receptor β in pancreatic neuroendocrine tumors: implications for targeted therapy. Pancreas (2014) 43(7):996–1002. doi: 10.1097/MPA.0000000000000203
270. Lykoudis PM, Contis J. Estrogen receptor expression in pancreatic adenocarcinoma: Time to reconsider evidence. Pancreas (2021) 50(9):1250–3. doi: 10.1097/MPA.0000000000001921
271. Sen A, Iyer J, Boddu S, Kaul A, Kaul R. Estrogen receptor alpha differentially modulates host immunity in the bladder and kidney in response to urinary tract infection. Am J Clin Exp Urol (2019) 7(3):110–22.
272. Wang C, Symington JW, Ma E, Cao B, Mysorekar IU. Estrogenic modulation of uropathogenic escherichia coli infection pathogenesis in a murine menopause model. Infect Immun (2013) 81(3):733–9. doi: 10.1128/IAI.01234-12
273. Chen Y-Y, Su T-H, Lau H-H. Estrogen for the prevention of recurrent urinary tract infections in postmenopausal women: a meta-analysis of randomized controlled trials. Int Urogynecol J (2021) 32(1):17–25. doi: 10.1007/s00192-020-04397-z
274. Dwyer PL, O'Reilly M. Recurrent urinary tract infection in the female. Curr Opin Obstet Gynecol (2002) 14(5):537–43. doi: 10.1097/00001703-200210000-00016
275. Stamm WE, Raz R. Factors contributing to susceptibility of postmenopausal women to recurrent urinary tract infections. Clin Infect Dis (1999) 28(4):723–5. doi: 10.1086/515209
276. Hextall A. Oestrogens and lower urinary tract function. Maturitas (2000) 36(2):83–92. doi: 10.1016/S0378-5122(00)00143-2
277. Teng J, Wang ZY, Jarrard DF, Bjorling DE. Roles of estrogen receptor alpha and beta in modulating urothelial cell proliferation. Endocr Relat Cancer (2008) 15(1):351–64. doi: 10.1677/erc.1.01255
278. Mäkelä S, Strauss L, Kuiper G, Valve E, Salmi S, Santti R, et al. Differential expression of estrogen receptors alpha and beta in adult rat accessory sex glands and lower urinary tract. Mol Cell Endocrinol (2000) 164(1-2):109–16. doi: 10.1016/S0303-7207(00)00233-1
279. Kaufmann O, Baume H, Dietel M. Detection of oestrogen receptors in non-invasive and invasive transitional cell carcinomas of the urinary bladder using both conventional immunohistochemistry and the tyramide staining amplification (TSA) technique. J Pathol (1998) 186(2):165–8. doi: 10.1002/(SICI)1096-9896(1998100)186:2<165::AID-PATH155>3.0.CO;2-Y
280. Dubois B, Hampel H, Feldman HH, Scheltens P, Aisen P, Andrieu S, et al. Preclinical alzheimer's disease: definition, natural history, and diagnostic criteria. Alzheimer's Dementia (2016) 12(3):292–323. doi: 10.1016/j.jalz.2016.02.002
281. Zagni E, Simoni L, Colombo D. Sex and gender differences in central nervous system-related disorders. Neurosci J (2016) 2016:2827090–103. doi: 10.1155/2016/2827090
282. Bustamante-Barrientos FA, Méndez-Ruette M, Ortloff A, Luz-Crawford P, Rivera FJ, Figueroa CD, et al. The impact of estrogen and estrogen-like molecules in neurogenesis and neurodegeneration: Beneficial or harmful? Front Cell Neurosci (2021) 15:636176. doi: 10.3389/fncel.2021.636176
283. Overk CR, Lu PY, Wang YT, Choi J, Shaw JW, Thatcher GR, et al. Effects of aromatase inhibition versus gonadectomy on hippocampal complex amyloid pathology in triple transgenic mice. Neurobiol Dis (2012) 45(1):479–87. doi: 10.1016/j.nbd.2011.08.035
284. Yaffe K, Haan M, Byers A, Tangen C, Kuller K. Estrogen use APOE and cognitive decline: evidence of gene-environment interaction. Neurology (2000) 54(10):1949–54. doi: 10.1212/WNL.54.10.1949
285. Song YJ, Li SR, Li XW, Chen X, Wei ZX, Liu QS, et al. The effect of estrogen replacement therapy on alzheimer's disease and parkinson's disease in postmenopausal women: A meta-analysis. Front Neurosci (2020) 14:157. doi: 10.3389/fnins.2020.00157
286. Ragonese P, D'Amelio M, Salemi G, Aridon P, Gammino M, Epifanio A, et al. Risk of Parkinson disease in women: effect of reproductive characteristics. Neurology (2004) 62(11):2010–4. doi: 10.1212/WNL.62.11.2010
287. Al Sweidi S, Sánchez MG, Bourque M, Morissette M, Dluzen D, Di Paolo T. Oestrogen receptors and signalling pathways: implications for neuroprotective effects of sex steroids in parkinson's disease. J Neuroendocrinol (2012) 24(1):48–61. doi: 10.1111/j.1365-2826.2011.02193.x
288. Shulman LM. Is there a connection between estrogen and parkinson's disease? Parkinsonism Relat Disord (2002) 8(5):289–95. doi: 10.1016/S1353-8020(02)00014-7
289. Lan YL, Zhao J, Li S. Update on the neuroprotective effect of estrogen receptor alpha against alzheimer's disease. J Alzheimers Dis (2015) 43(4):1137–48. doi: 10.3233/JAD-141875
290. Yu Z, Gao W, Jiang E, Lu F, Zhang L, Shi Z, et al. Interaction between IGF-IR and ER induced by E2 and IGF-I. PloS One (2013) 8(5):e62642. doi: 10.1371/journal.pone.0062642
291. Zhao L, Woody SK, Chhibber A. Estrogen receptor β in alzheimer's disease: From mechanisms to therapeutics. Ageing Res Rev (2015) 24(Pt B):178–90. doi: 10.1016/j.arr.2015.08.001
292. Roque C, Mendes-Oliveira J, Duarte-Chendo C, Baltazar G. The role of G protein-coupled estrogen receptor 1 on neurological disorders. Front Neuroendocrinol (2019) 55:100786. doi: 10.1016/j.yfrne.2019.100786
293. Dika E, Patrizi A, Lambertini M, Manuelpillai N, Fiorentino M, Altimari A, et al. Estrogen receptors and melanoma: A review. Cells (2019) 8(11):1463–76. doi: 10.3390/cells8111463
294. Joosse A, Collette S, Suciu S, Nijsten T, Lejeune F, Kleeberg UR, et al. Superior outcome of women with stage I/II cutaneous melanoma: Pooled analysis of four European organisation for research and treatment of cancer phase III trials. J Clin Oncol (2012) 30(18):2240–7. doi: 10.1200/JCO.2011.38.0584
295. Mervic L, Leiter U, Meier F, Eigentler T, Forschner A, Metzler G, et al. Sex differences in survival of cutaneous melanoma are age dependent: an analysis of 7338 patients. Melanoma Res (2011) 21(3):244–52. doi: 10.1097/CMR.0b013e32834577c8
296. Richardson B, Price A, Wagner M, Williams V, Lorigan P, Browne S, et al. Investigation of female survival benefit in metastatic melanoma. Br J Cancer (1999) 80(12):2025–33. doi: 10.1038/sj.bjc.6690637
297. Rajabi P, Bagheri M, Hani M. Expression of estrogen receptor alpha in malignant melanoma. Adv BioMed Res (2017) 6:14. doi: 10.4103/2277-9175.200789
298. Bhari N, Schwaertz RA, Apalla Z, Salerni G, Akay BN, Patil A, et al. Effect of estrogen in malignant melanoma. J Cosmet Dermatol (2022) 21(5):1905–12. doi: 10.1111/jocd.14391
299. de Giorgi V, Gori A, Gandini S, Papi F, Grazzini M, Rossari S, et al. Oestrogen receptor beta and melanoma: a comparative study. Br J Dermatol (2013) 168(3):513–9. doi: 10.1111/bjd.12056
300. Spyropoulos C, Melachrinou M, Vasilakos P, Tzorakoleftherakis E. Expression of estrogen receptors in melanoma and sentinel lymph nodes; a "female" clinical entity or a possible treatment modality? Eur J Gynaecol Oncol (2015) 36(2):123–30.
301. Zhou JH, Kim KB, Myers JN, Fox PS, Ning J, Bassett RL, et al. Immunohistochemical expression of hormone receptors in melanoma of pregnant women, nonpregnant women, and men. Am J Dermatopathol (2014) 36(1):74–9. doi: 10.1097/DAD.0b013e3182914c64
Keywords: estrogen receptor alpha, estrogen receptor beta, signaling pathway, mediation, G-protein-coupled estrogen receptor 1
Citation: Chen P, Li B and Ou-Yang L (2022) Role of estrogen receptors in health and disease. Front. Endocrinol. 13:839005. doi: 10.3389/fendo.2022.839005
Received: 20 December 2021; Accepted: 26 July 2022;
Published: 18 August 2022.
Edited by:
Ernestina Marianna De Francesco, University of Catania, ItalyReviewed by:
Elena Ortona, National Institute of Health (ISS), ItalyNicola Amodio, Magna Græcia University, Italy
Copyright © 2022 Chen, Li and Ou-Yang. This is an open-access article distributed under the terms of the Creative Commons Attribution License (CC BY). The use, distribution or reproduction in other forums is permitted, provided the original author(s) and the copyright owner(s) are credited and that the original publication in this journal is cited, in accordance with accepted academic practice. No use, distribution or reproduction is permitted which does not comply with these terms.
*Correspondence: Bo Li, bGlibyYjeDIwMTA7JiN4MjAxMDsyMDAxQDE2My5jb20=