- 1Department of Radiology, Beijing Jishuitan Hospital, Beijing, China
- 2International Medical Center, West China Hospital, Sichuan University, Chengdu, China
- 3Department of Epidemiology and Biostatistics, Beijing Research Institute of Traumatology and Orthopedics, Beijing Jishuitan Hospital, Beijing, China
- 4Department of Sport and Exercise Sciences, Durham University, Durham, United Kingdom
Purpose: The etiology of age-related bone loss is less clear in men. This study is aimed to observe the variations of endogenous sex hormone concentrations with increasing of age in men, and investigate their relations to bone mass, marrow adiposity, and muscle adiposity.
Methods: A total of 199 community-dwelling Chinese men (aged 41 to 82 years) were included and measured of serum total estradiol, total testosterone, and follicle-stimulating hormone (FSH) concentrations by enzyme-linked immunosorbent assay (ELISA). Vertebral trabecular volumetric bone mineral density (vBMD) was measured by quantitative computed tomography for all participants, and vertebral marrow fat content and erector muscle fat content were quantified by Chemistry-shift-encoding magnetic resonance imaging in 62 participants.
Results: In this population, FSH concentration increased (p < 0.001) gradually with aging. Lower vBMD was independently associated with higher FSH concentration (β = -0.216, p < 0.001), but not with total estradiol or total testosterone. For each standard deviation increase in FSH there was a 50% higher risk of an individual having osteopenia or osteoporosis (vBMD < 120 mg/cm3). Marrow fat content and erector muscle fat content were greater in osteopenic and osteoporotic men, but there were no associations with sex hormones concentrations.
Conclusion: In summary, FSH but not total estradiol or total testosterone is related to vertebral trabecular vBMD in middle-aged and older Chinese men. Neither marrow adiposity nor muscle adiposity is associated with sex hormones.
Introduction
Although men do not experience a phase of accelerated bone loss similar to the menopause in women, their bone health status declines gradually with age (1). According to the World Health Organization (WHO) diagnostic standard using bone mineral density (BMD) measured by dual-energy X-ray absorptiometry (DXA), approximately 3-6% of men have osteoporosis and 28-47% have osteopenia, and the prevalence of osteoporosis rises to 19% in men who are aged 50 and older (2, 3). While it is established that the primary cause of menopausal-bone loss in women is driven by ovarian failure, in men, the etiology of age-related bone loss is less clear.
In men, sex steroids have an important role in the maintenance of bone strength, as demonstrated by the rapid reductions in bone density following androgen deprivation therapy (4). There is evidence of a small and progressive age-related decline in free testosterone, estradiol and to a lesser extent, progesterone (5, 6) and increases in luteinizing hormone, follicle-stimulating hormone (FSH) and sex hormone-binding globulin (SHBG) (7). However, research into the role of age-related sex hormone changes in the development of male osteoporosis has primarily focused on testosterone and estradiol. Endogenous testosterone levels have been related to bone turnover markers, BMD, and hip structural geometry in normal aging men (8–11). The actions of testosterone on the male skeleton are partly mediated by the aromatization of testosterone to estradiol, with estrogen deficiency also contributing to age-related bone loss in men, and there is cross-sectional and longitudinal evidence to suggest that bioavailable estradiol is more strongly associated with male BMD and fracture (10, 12, 13). Testosterone has been suggested to also play an indirect role in male skeletal health with aging by allowing for relative maintenance of balance and muscle strength in men compared to women (14). SHBG levels in men increase with age and influence the amount of hormone that is available to enter cells, and are predictive of vertebral fracture (15, 16) and/or lower bone density (17).
Relatively less attention has been directed toward the role of other sex steroids in male bone health, particularly of FSH. In women, a sharp rise in serum FSH during late perimenopause coincides with the most rapid rate of bone loss (18) and epidemiological data suggest that bone turnover markers and BMD in perimenopausal and early postmenopausal women are independent of serum estrogen, but negatively associated with FSH (19–21). However, the relationship between FSH and bone mass in men has not yet been fully explored.
Musculoskeletal fragility associated with sarcopenia (loss of muscle mass) and osteopenia (loss of bone mass) can result in fall and fracture. People are diagnosed with osteoporosis often combining with muscles weakness, and increased spine kyphosis leading vertebral fractures (22). Partial androgen deficiency may contribute to the age-related decrease in muscle mass and strength and to the higher risk of fall and fractures in elderly men (23, 24).
In the present cross-sectional study, we observed the variations of endogenous sex hormone concentrations with increasing of age, and investigated their relations to bone mass. Considering the potential roles of marrow adiposity and paravertebral muscle fragility in bone loss and vertebral fractures in old men which may be correlated with sex hormones, we furthermore explored the relationships between endogenous sex hormones concentrations and vertebral marrow fat content as well as paravertebral muscle fat content.
Methodology
Study Subjects
A total of 199 men aged from 41 and 82 years were recruited from a population study to investigate the degeneration of spine and knee (China Action on Spine and Hip study). All subjects were healthy adults who have lived in Beijing for more than 5 years, and the subjects with the following conditions were excluded: (1) spine or knee disorders due to congenital, tumor or tuberculosis; (2) a history of spine or knee injury or surgery; (3) suffering from other major diseases (such as infection, tumor, rheumatic immune disease, renal failure, coronary heart disease, stroke, and mental diseases) and taking bone metabolism regulating drugs; (4) heart pacemaker, coronary stent, orthopedic implants, and implant teeth; and (5) familial hereditary disease (25). The regional ethics committee approved the study and all participants provided signed, informed consent. The study was carried out in accordance with The Code of Ethics of the World Medical Association (Declaration of Helsinki).
All participants received blood serum tests for the measurement of total estradiol, total testosterone and FSH, and a quantitative computed tomography (QCT) scan for the measurement of vertebral trabecular volumetric BMD (vBMD). Sixty-two participants received Chemistry-shift-encoding magnetic resonance imaging (CSE-MRI) (mDixon-MRI) scans for vertebral marrow fat content and erector muscle fat content.
QCT Scan and Measurements
We performed quantitative CT scan using a Toshiba CT scanner (Aquilion Prime ESX-302A; Toshiba Medical Systems Corporation, Otawara, Japan) with a five-rod calibration phantom (Model 3 phantom; Mindways Inc., Austin, TX, USA) placed beneath the subject and scanned simultaneously. The scanning parameters were as follows: 120kV, 187mAs, table height 120cm, 1 mm slice thickness, field of view (FOV) 500 mm. The scan range included 2 cm above L1 vertebra to 2 cm below L5 vertebra. Reconstruction parameters were standard algorithm, 1 mm section thickness and interval, and 400 mm display FOV.
The CT data were transferred to QCT workstation and analyzed using Mindways QCT PRO three-dimensional (3D) spine module software version 4.2. On the 3D reconstructed images, an elliptical cylinder region of interest (ROI) was individually placed in the central part of L2, L3, and L4 vertebral bodies. The ROIs contained the largest areas of the trabecular bone, but not included the cortical bone or basivertebral plexus. The values of vBMD of L2, L3, and L4 were automatically output. vBMD of lumbar spine was calculated as the mean value of vBMD of L2 to L4.
CSE-MRI Scan and Measurements
On the same day as the QCT examination, the participants underwent a multiecho 3D spoiled gradient-echo sequence, referred to as an mDixon-Quant study, by using a 3.0-T MRI system with a 32-channel torso body coil (Ingenia, Philips Healthcare, Best, the Netherlands). The mDixon-Quant sequence is a 3D-FFE sequence, and uses multiple acquired echoes to generate water, fat, T2*, R2*, and in-phase and oppose-phase images synthesized from the water-fat images. The scan parameters of the single breath-hold mDixon-Quant were as follows: repetition time, 9.1 ms; echo time 1, 1.33 ms; six echoes with echo time shift, 1.3 ms; FOV, 360 × 330 × 120 mm3; flip angle, 3°; voxel size, 2.5 × 2.5 × 3.0 mm3; sensitivity encoding, 2; number of signal average, 2; and scan time, 12.5 s.
The CSE-MRI dataset were processed with ISP version 7 software (Philips Healthcare, Best, the Netherlands). ROIs for marrow fat content measurement were drawn manually encompassing the largest region of the cancellous bone of vertebral bodies on central L2, L3, and L4 axial image eliminating the vertebral cortex, schmorl’s nodules or hemangiomas. Marrow fat content was calculated as the mean value of L2, L3, and L4 marrow fat contents. The fat content of erector muscle was measured on the same central L3 image. Clear cavities of fat at the periphery of the erector muscle visually identify the edge of the muscle.
Sex Hormone Analyses
For the assessment of endogenous hormone concentrations, a fasted, morning blood sample was taken from each participant on the same day as the imaging examinations. Blood was centrifuged, separated and refrigerated at -80°C until assay. Serum was sent to the laboratory at the Dopbio Biotechnology Co., Ltd for determination of testosterone, estradiol, and FSH concentrations.
Hormone assays were conducted by enzyme-linked immunosorbent assay (ELISA) using the MULTISKAN MK3 automated analyzer (Thermo Scientific, USA). Serum total estradiol concentrations were measured with a solid phase ELISA (DRG Estradiol ELISA, EIA-2693) based to the principle of competitive binding. Inter- and intra-assay coefficients of variation (CV) averaged 9.0% and 10.9%, respectively over the assay range. Serum FSH concentrations were measured with a two-step capture enzyme immunoassay test using constant amounts of two monoclonal antibodies (ALPCO FSH ELISA, 11-FSHHU-E01). One monoclonal antibody specific for FSH is immobilized onto the microplate and another monoclonal antibody specific for a different region of FSH is conjugated to horseradish peroxidase. Inter- and intra-assay CV averaged 4.2% and 5.2%, respectively. Total testosterone concentrations were determined with a competitive enzyme immunoassay (R&D Testosterone Parameter Assay Kit, KGE010). A monoclonal antibody specific for testosterone bounded to the goat anti-mouse antibody coated onto the microplate and then testosterone in a serum sample competed with a fixed amount of horseradish peroxidase-labeled testosterone for sites on the monoclonal antibody. Inter- and intra-assay CV averaged 3.3% and 6.2%, respectively.
Statistical Analysis
Data management and analyses were completed using SPSS version 22.0 (SPSS Inc., Chicago, IL, USA). Distributions of the variables were examined for departure from normality by the Shapiro-Wilk test. Data with normal distribution are presented as the mean ± standard deviation (SD), while data with skewed distribution are presented as the median (interquartile, IQR). For the inter-group comparisons, t test was used for normally distributed variables and Mann-Whitney U test was used for skewed variables. Multivariate linear regression analysis was performed to estimate the contributions of each sex hormone to vBMD, marrow fat content, and erector muscle fat content, with and without adjustments for age, BMI, and other sex hormones. According to the criteria suggested by the International Society for Clinical Densitometry in 2007 (26) and by the American College of Radiology in 2008 (27) for QCT, the thresholds of vBMD were < 120 mg/cm3 for osteopenia and < 80 mg/cm3 for osteoporosis. The odds ratios (ORs) and 95% confidence intervals (95% CI) of osteopenia/osteoporosis (defined as vBMD < 120 mg/cm3), higher marrow fat content (marrow fat content ≥ 45%), and higher muscle fat content (erector muscle fat content ≥ 10%) were calculated by logistic regression models with and without adjustments for age, BMI, and other sex hormones, in which sex hormone levels were fitted as continuous variables with results expressed in terms of per SD increase in hormone concentration.
Results
The general characteristics of the study participants are shown in Table 1. Participants were stratified into four sub-groups according to age, and the characteristics of each group and inter-group comparisons are also presented in Table 1. FSH concentrations were higher with increasing age (p < 0.001), but there were no age related differences in total estradiol concentration (p = 0.90). With aging, lumbar spine vertebral vBMD decreased (p < 0.001) and erector muscle fat content increased (p = 0.034) gradually. There were no significant age-related differences in marrow fat content (p = 0.574).
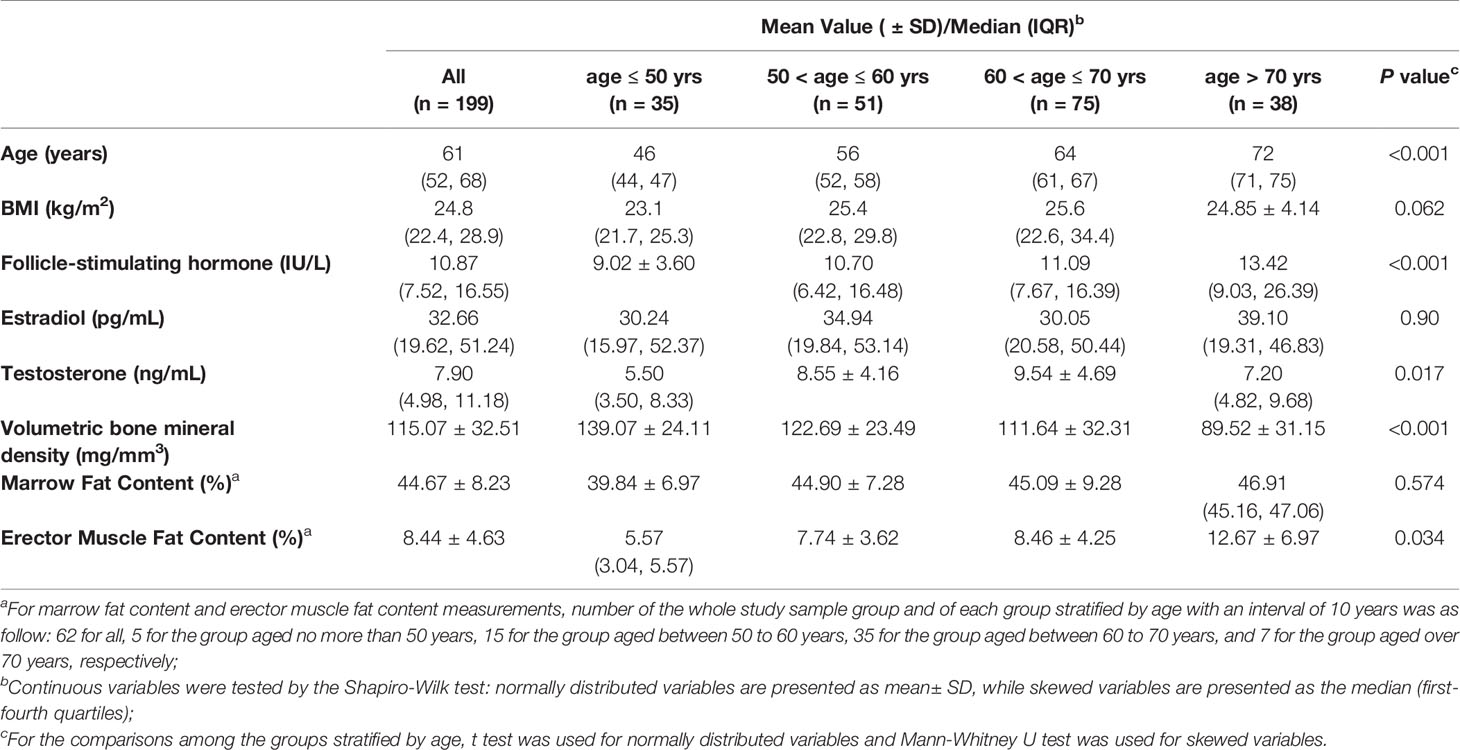
Table 1 Demographic and biologic characteristics of 199 men overall, and of the men at different levels of age.
Further sub-group analysis was completed according to presence of osteopenia and osteoporosis (normal bone mass: vBMD ≥ 120 mg/mm3 and osteopenia/osteoporosis: vBMD < 120 mg/mm3) and of obesity (non-obesity: BMI < 25 kg/m2 and obesity: BMI ≥ 25 kg/m2), respectively, and inter-group comparisons are shown in Table 2. Participants who were osteopenic or osteoporotic had significantly higher FSH concentrations (p = 0.005), marrow fat content (p = 0.002), and erector muscle fat content (p = 0.003). Obese participants had significantly higher total testosterone concentrations (p = 0.004), but there were no differences in vBMD or fat content measurements.
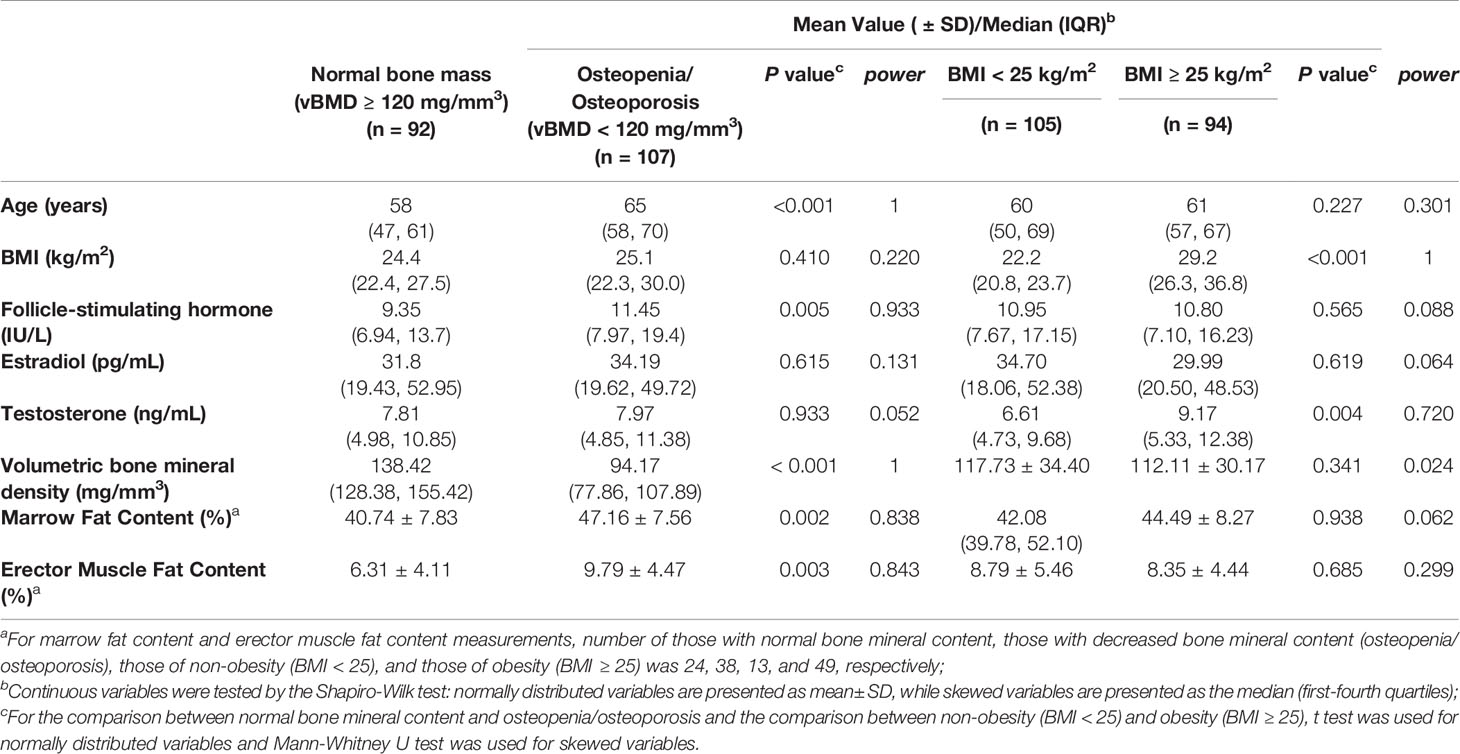
Table 2 Comparisons of demographic and biologic characteristics between the participants with normal bone mineral content and with decreased bone mineral content (osteopenia or osteoporosis), and between non-obese and obese participants.
Associations between sex hormone concentrations, vBMD, and fat content measurements are shown in Table 3. Lower vBMD was associated with higher FSH concentration (β = -0.216, p < 0.001), but not with total estradiol or total testosterone concentration (Table 3), after adjustment for age, BMI, and other sex hormones. Neither bone marrow fat content nor erector muscle fat content was associated with sex hormone concentrations after adjustment for age, BMI, and other sex hormones.

Table 3 Multivariate linear regression analysis showing the factors determining vBMD and fat measurements.
Adjusted and non-adjusted logistic regression analysis found that for each SD increase in FSH there was a 50% higher risk of an individual having osteopenia or osteoporosis (Table 4). All the three sex hormones showed no significant contributions to the risk of higher marrow fat content or higher erector fat content after multivariate adjustment.
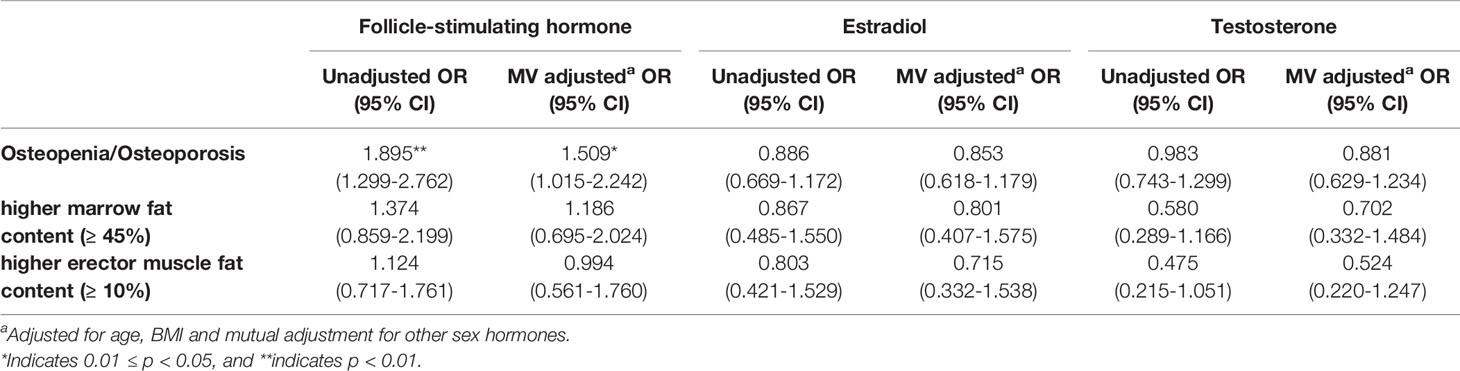
Table 4 OR of osteopenia/osteoporosis, higher marrow fat, and higher muscle fat (erector muscle fat content ≥ 10%) per SD increase in FSH, estradiol, and testosterone concentrations in men.
Discussion
This study is the first to explore associations between sex hormones, bone density, marrow adiposity and muscle adiposity in community-dwelling middle and older aged men. In doing so, we found that high concentration of FSH, but not total testosterone or estradiol, was a risk factor for lower vBMD, osteopenia and osteoporosis. While marrow fat content and erector muscle fat content were greater in osteopenic and osteoporotic men, there were no associations with sex hormones concentrations.
Our primary finding was of significant associations between high FSH and low vertebral trabecular vBMD in men. There is accumulating evidence that FSH acts on bone through both direct and indirect mechanisms. First, FSH acts directly through the FSHR on osteoclast precursors to increase osteoclastogenesis by sensitizing MAP kinase, NF-κB, and Akt pathways, and blocking FSHRs or the β-subunit of FSH, can prevent bone loss in mice independent of estrogen (28). Second, FSH increases expression of the receptor activator of NF-κB (29) and indirectly stimulates osteoclastogenesis by releasing cytokines, namely IL-1β, TNF-α, and IL-6 in proportion to the surface expression of FSHR (21, 30). Elsewhere, in-vivo evidence regarding the relationship between FSH and bone mass in men is limited. Hsu B, et al. reported that lower levels of serum FSH were protective against bone loss and were negatively associated with incident fractures in a cohort of elderly men (31). In addition, Jing, et al. have demonstrated associations between FSH, lumbar spine BMD and osteoporosis in men with type 2 diabetes mellitus (32).
In this study, we found no associations between total estradiol, total testosterone and vertebral trabecular vBMD. Elsewhere, studies have supported estradiol as the crucial sex steroid for bone health in men, and others, provide evidence for testosterone and SHBG (8–11, 32–37). In elderly men, bioavailable estradiol has been found to be positively associated with BMD at multiple sites (8, 10, 11, 33–35), and higher bioavailable estradiol is suggested to be a protective factor for osteopenia (9). Total estradiol has also been reported to be a significant determinant of bone mass in elderly men (10, 24, 34, 36). In a study involving a diverse sample of men (Black, Hispanic, and White, aged 30 to 79 years), total and free estradiol showed larger positive correlations with BMD outcomes and correlations between estradiol levels and hip BMD were robust after multivariate-adjustment, whereas no correlation between total or free estradiol and lumbar spine BMD after multivariate-adjustment (8). In aging Chinese men, both bioavailable and total estradiol have been demonstrated with a positive association with both total hip and lumbar spine BMD after multivariate- or age-adjustment (32, 37). The bioavailable sex steroids comprise the fractions that are free or associated with albumin in the circulation (38), and it is these fractions that have rapid access to target tissues (39). The free fraction constitutes only 1-3% of the total circulating sex steroids (38). In contrast, the fraction bound to SHBG does not have free access to target tissue. As SHBG levels increase with age in men, measurement of total testosterone or estradiol levels does not accurately reflect the actual levels of these steroids available to tissue.
The evidence around the role of testosterone for bone health in elderly men is more conflicting, than the evidence for estradiol (9–11, 24, 34, 36). A study on American men aged 20–90 years found that men with free testosterone level at the lowest quartile were more likely to be osteopenic (9). A study by Van Den Beld et al. also found a positive and significant association between testosterone levels (total, free, and bioavailable fraction and androgen index) and proximal femoral BMD in older men (10). The Swedish Osteoporotic Fractures in Men (MrOS) Study discovered that free testosterone was an independent positive predictor of BMD in total body, total hip, femur trochanter, and arm but not in the lumbar spine (11). The Hong Kong MrOS study also found a positive and significant relationship between femoral and vertebral BMD and free testosterone in Chinese men aged 65 years and above (37). However, discrepancies exist among different studies, and some have reported no associations between bone health status in men and total testosterone (8, 9, 34), and studies have found that the relationship between testosterone and BMD is not consistent at different testing sites (35). The effects of testosterone can be exerted either directly through the androgen receptor or indirectly through aromatization to estrogens and further through estrogen receptor-α and/or –β (40). These sex steroid receptors are expressed in bone, and experimental animal studies, using sex steroid receptor-inactivated transgenic mouse model, have indicated that each of these three receptors mediate the site-specific skeletal effects of sex steroids (41, 42).
To date, most studies have been conducted using DXA measured BMD, which cannot separate trabecular from cortical bone. Trabecular bone is known to have a more rapid rate of age-related loss than cortical bone. This may diminish the sensitivity of DXA for assessing osteoporosis. QCT is a truly three dimensional technique for quantifying volumetric trabecular bone density that is not affected by spine degeneration and abdominal aortic calcification (43). Khosla et al, measured vBMD using QCT and showed that vertebral trabecular vBMD was significantly associated with bioavailable estradiol and bioavailable testosterone in the entire group of men (22 to 91 years) (33). After adjusting for age, bioavailable testosterone was more strongly associated with vertebral trabecular vBMD than bioavailable estradiol in the middle-aged men (40-59 years), while bioavailable estradiol was the best predictor of vBMD at most sites in the elderly men (≥ 60 years) (33).
In the current study, there were no associations between sex hormone concentrations and marrow fat content, despite evidence that marrow fat increases with age (44, 45). Mistry et al, have reported that marrow adiposity is negatively associated with both total estradiol and total testosterone in aging men (74 to 95 years of age) after adjusting for age and total percent fat (46). The possible reasons for the discrepancies with Mistry’s results may be firstly, the number size of this study (n = 62) was small and secondly, the participants in this study are much younger than those in Mistry’s study (61 years vs. 82.4 years). FSH receptor cDNA and protein have been identified on osteoclasts and mesenchymal stem cells (28). An animal study showed that both sham-operated and ovariectomized mice presented a decrease in marrow adipose volume after FSH antibody treatments (47). Our study did not find a significant association between marrow fat content and FSH concentration in this middle-aged to older male population, and furthermore, the increase of FSH was not a risk factor for higher marrow fat content.
In the context of muscle aging, it is important to remember that it is not just a decline in muscle mass which contributes to the deterioration of muscle function. Factors underpinning muscle quality come into play, including muscle composition, aerobic capacity and metabolism, fatty infiltration, insulin resistance, fibrosis and neural activation (48). Observational studies have shown that lean mass and strength are reduced in men with low testosterone levels (10, 23), and testosterone replacement increases lean mass in men with hypogonadism (49). In the present cross-sectional study, total testosterone concentration was detected with a weakly negative correlation with erector muscle fat content by unadjusted linear regression analysis, however, the correlation was insignificant after adjusting for age, BMI, total estradiol concentration, and FSH concentration. The increase of total testosterone for 1 SD was not a significant protective factor for higher erector muscle fat content (≥ 10%). Considering lean mass, muscle area, and muscle strength were preserved until gonadal steroid deficiency was more mark according to Finkelstein et al. (a testosterone level ≤ 200 ng per deciliter) (50), our results are not unexpected.
The main limitation of this study was the relative small sample size (n = 199), especially only 62 participants had MR measurements of marrow fat content and muscle fat content, and as a result, the relationships between sex hormones and MR-quantified marrow fat and muscle fat content may not be fully revealed. In addition, luteinizing hormone and SHBG were not measured, and therefore, free estradiol and free testosterone could not be calculated and investigated in this study. The smoking and drinking history were not obtained in this study, and therefore, neither multivariate linear regression analyses for the contributions of sex hormones to vBMD nor ORs of osteopenia/osteoporosis were adjusted for smoking and drinking.
Conclusion
FSH but not total estradiol or total testosterone is related to vertebral trabecular vBMD in middle-aged and older Chinese men. Neither marrow adiposity nor muscle adiposity is associated with total estradiol, total testosterone, or FSH.
Data Availability Statement
The original contributions presented in the study are included in the article/supplementary files. Further inquiries can be directed to the corresponding author.
Ethics Statement
The studies involving human participants were reviewed and approved by Beijing Jishuitan Hospital Ethics Committee. The patients/participants provided their written informed consent to participate in this study.
Author Contributions
The first authorship of LX and QZ is of an equal rank. LX and QZ designed the study and prepared the first draft of the paper. KL, YZ, and YL contributed the experimental work and data collection. KH and LW edited the draft. CW was responsible of the statistical analysis of the data. XC supervised the study and paper organization. All authors revised the paper critically for intellectual content and approved the final version. All authors agree to be accountable for the work and to ensure that any questions relating to the accuracy and integrity of the paper are investigated and properly resolved.
Funding
This work was funded by the grants from the National Key Research and Development Program of China [No. 2020YFC2004902], the Beijing Hospitals Authority Clinical Medicine Development of Special Funding Support [No. ZYLX202107], the National Natural Science Foundation of China [No. 81771831, 2018], and the High Level Talents “Discipline backbone” Project of Beijing Jishuitan Hospital [No. XKGG2021123].
Conflict of Interest
The authors declare that the research was conducted in the absence of any commercial or financial relationships that could be construed as a potential conflict of interest.
Publisher’s Note
All claims expressed in this article are solely those of the authors and do not necessarily represent those of their affiliated organizations, or those of the publisher, the editors and the reviewers. Any product that may be evaluated in this article, or claim that may be made by its manufacturer, is not guaranteed or endorsed by the publisher.
Acknowledgments
We thank our study participants for contributing their time and efforts. We wish to thank Dopbio Biotechnology for their technical supports in blood testing. We also thank National Natural Science Foundation of China for the fund support to this study.
References
1. Melton LJ 3rd, Khosla S, Achenbach SJ, O'Connor MK, O'Fallon WM, Riggs BL. Effects of Body Size and Skeletal Site on the Estimated Prevalence of Osteoporosis in Women and Men. Osteoporos Int (2000) 11(11):977–83. doi: 10.1007/s001980070037
2. Looker AC, Orwoll ES, Johnston CC Jr, Lindsay RL, Wahner HW, Dunn WL, et al. Prevalence of Low Femoral Bone Density in Older U.S. Adults From NHANES III. J Bone Miner Res (1997) 12(11):1761–8. doi: 10.1359/jbmr.1997.12.11.1761
3. Melton LJ 3rd, Atkinson EJ, O’Connor MK, O'Fallon WM, Riggs BL. Bone Density and Fracture Risk in Men. J Bone Miner Res (1998) 13(12):1915–23. doi: 10.1359/jbmr.1998.13.12.1915
4. Daniell HW, Dunn SR, Ferguson DW, Lomas G, Niazi Z, Stratte PT. Progressive Osteoporosis During Androgen Deprivation Therapy for Prostate Cancer. J Urol (2000) 163(1):181–6. doi: 10.1097/00005392-200001000-00043
5. Wu IC, Lin XZ, Liu PF, Tsai WL, Shiesh SC. Low Serum Testosterone and Frailty in Older Men and Women. Maturitas (2010) 67(4):348–52. doi: 10.1016/j.maturitas.2010.07.010
6. Walther A, Philipp M, Lozza N, Ehlert U. The Rate of Change in Declining Steroid Hormones: A New Parameter of Healthy Aging in Men? Oncotarget (2016) 7(38):60844–57. doi: 10.18632/oncotarget.11752
7. Feldman HA, Longcope C, Derby CA, Johannes CB, Araujo AB, Coviello AD, et al. Age Trends in the Level of Serum Testosterone and Other Hormones in Middle-Aged Men: Longitudinal Results From the Massachusetts Male Aging Study. J Clin Endocrinol Metab (2002) 87(2):589–98. doi: 10.1210/jcem.87.2.8201
8. Araujo AB, Travison TG, Leder BZ, McKinlay JB. Correlations Between Serum Testosterone, Estradiol, and Sex Hormone-Binding Globulin and Bone Mineral Density in a Diverse Sample of Men. J Clin Endocrinol Metab (2008) 93(6):2135–41. doi: 10.1210/jc.2007-1469
9. Paller CJ, Shiels MS, Rohrmann S, Basaria S, Rifai N, Nelson W, et al. Relationship of Sex Steroid Hormones With Bone Mineral Density (BMD) in a Nationally Representative Sample of Men. Clin Endocrinol (Oxf) (2009) 70(1):26–34. doi: 10.1111/j.1365-2265.2008.03300.x
10. van den Beld AW, de Jong FH, Grobbee DE, Pols HA, Lamberts SW. Measures of Bioavailable Serum Testosterone and Estradiol and Their Relationships With Muscle Strength, Bone Density, and Body Composition in Elderly Men. J Clin Endocrinol Metab (2000) 85(9):3276–82. doi: 10.1210/jcem.85.9.6825
11. Mellström D, Johnell O, Ljunggren O, Eriksson AL, Lorentzon M, Mallmin H, et al. Free Testosterone Is an Independent Predictor of BMD and Prevalent Fractures in Elderly Men: MrOS Sweden. J Bone Miner Res (2006) 21(4):529–35. doi: 10.1359/jbmr.060110
12. Khosla S, Melton LJ 3rd, Atkinson EJ, O'Fallon WM. Relationship of Serum Sex Steroid Levels to Longitudinal Changes in Bone Density in Young Versus Elderly Men. J Clin Endocrinol Metab (2001) 86(8):3555–61. doi: 10.1210/jcem.86.8.7736
13. Mellström D, Vandenput L, Mallmin H, Holmberg AH, Lorentzon M, Odén A, et al. Older Men With Low Serum Estradiol and High Serum SHBG Have an Increased Risk of Fractures. J Bone Miner Res (2008) 23(10):1552–60. doi: 10.1359/jbmr.080518
14. Khosla S, Melton LJ 3rd, Riggs BL. The Unitary Model for Estrogen Deficiency and the Pathogenesis of Osteoporosis: Is a Revision Needed? J Bone Miner Res (2011) 26(3):441–51. doi: 10.1002/jbmr.262
15. Cawthon PM, Schousboe JT, Harrison SL, Ensrud KE, Black D, Cauley JA, et al. Sex Hormones, Sex Hormone Binding Globulin, and Vertebral Fractures in Older Men. Bone (2016) 84:271–8. doi: 10.1016/j.bone.2016.01.009
16. Vandenput L, Mellström D, Kindmark A, Johansson H, Lorentzon M, Leung J, et al. High Serum SHBG Predicts Incident Vertebral Fractures in Elderly Men. J Bone Miner Res (2016) 31(3):683–9. doi: 10.1002/jbmr.2718
17. Hsu B, Seibel MJ, Cumming RG, Blyth FM, Naganathan V, Bleicher K, et al. Progressive Temporal Change in Serum SHBG, But Not in Serum Testosterone or Estradiol, Is Associated With Bone Loss and Incident Fractures in Older Men: The Concord Health and Ageing in Men Project. J Bone Miner Res (2016) 31(12):2115–22. doi: 10.1002/jbmr.2904
18. Zaidi M, Lizneva D, Kim SM, Sun L, Iqbal J, New MI, et al. FSH, Bone Mass, Body Fat, and Biological Aging. Endocrinology (2018) 159(10):3503–14. doi: 10.1210/en.2018-00601
19. Sowers MR, Greendale GA, Bondarenko I, Finkelstein JS, Cauley JA, Neer RM, et al. Endogenous Hormones and Bone Turnover Markers in Pre- and Perimenopausal Women: SWAN. Osteoporos Int (2003) 14(3):191–7. doi: 10.1007/s00198-002-1329-4
20. Gallagher CM, Moonga BS, Kovach JS. Cadmium, Follicle-Stimulating Hormone, and Effects on Bone in Women Age 42–60 Years, NHANES III. Environ Res (2010) 110(1):105–11. doi: 10.1016/j.envres.2009.09.012
21. Cannon JG, Cortez-Cooper M, Meaders E, Stallings J, Haddow S, Kraj B, et al. Follicle-Stimulating Hormone, Interleukin-1, and Bone Density in Adult Women. Am J Physiol Regul Integr Comp Physiol (2010) 298(3):R790–8. doi: 10.1152/ajpregu.00728.2009
22. Hsu WL, Chen CY, Tsauo JY, Yang RS. Balance Control in Elderly People With Osteoporosis. J Formos Med Assoc (2014) 113(6):334–9. doi: 10.1016/j.jfma.2014.02.006
23. Baumgartner RN, Waters DL, Gallagher D, Morley JE, Garry PJ. Predictors of Skeletal Muscle Mass in Elderly Men and Women. Mech Aging Dev (1999) 107(2):123–36. doi: 10.1016/s0047-6374(98)00130-4
24. Szulc P, Claustrat B, Marchand F, Delmas PD. Increased Risk of Falls and Increased Bone Resorption in Elderly Men With Partial Androgen Deficiency: The MINOS Study. J Clin Endocrinol Metab (2003) 88(11):5240–7. doi: 10.1210/jc.2003-030200
25. Zhang Y, Zhou Z, Wu C, Zhao D, Wang C, Cheng X, et al. Population-Stratified Analysis of Bone Mineral Density Distribution in Cervical and Lumbar Vertebrae of Chinese From Quantitative Computed Tomography. Korean J Radiol (2016) 17(5):581–9. doi: 10.3348/kjr.2016.17.5.581
26. Engelke K, Adams JE, Armbrecht G, Augat P, Bogado CE, Bouxsein ML, et al. Clinical Use of Quantitative Computed Tomography and Peripheral Quantitative Computed Tomography in the Management of Osteoporosis in Adults: The 2007 ISCD Official Positions. J Clin Densitom (2008) 11(1):123–62. doi: 10.1016/j.jocd.2007.12.010
27. American College of Radiology. Reston, “ACR Practice Guide Line for the Performance of Quantitative Computed Tomogramphy (QCT) Bone Densitometry (Resolution33) (2008). Available at: http://www.acr.org/w/media/ACR/Documents/PGTS/guidelines/QCT.pdf.
28. Sun L, Peng Y, Sharrow AC, Iqbal J, Zhang Z, Papachristou DJ, et al. FSH Directly Regulates Bone Mass. Cell (2006) 125(2):247–60. doi: 10.1016/j.cell.2006.01.051
29. Cannon JG, Kraj B, Sloan G. Follicle-Stimulating Hormone Promotes RANK Expression on Human Monocytes. Cytokine (2011) 53(2):141–4. doi: 10.1016/j.cyto.2010.11.011
30. Iqbal J, Sun L, Kumar TR, Blair HC, Zaidi M. Follicle-Stimulating Hormone Stimulates TNF Production From Immune Cells to Enhance Osteoblast and Osteoclast Formation. Proc Natl Acad Sci USA (2006) 103(40):14925–30. doi: 10.1073/pnas.0606805103
31. Hsu B, Cumming RG, Seibel MJ, Naganathan V, Blyth FM, Bleicher K, et al. Reproductive Hormones and Longitudinal Change in Bone Mineral Density and Incident Fracture Risk in Older Men: The Concord Health and Aging in Men Project. J Bone Miner Res (2015) 30(9):1701–8. doi: 10.1002/jbmr.2493
32. Jing Y, Wang X, Yu J, Wang X, Zhou Y, Tao B, et al. Follicle-Stimulating Hormone and Estradiol are Associated With Bone Mineral Density and Risk of Fracture in Men With Type 2 Diabetes Mellitus. J Diabetes (2020) 12(6):426–37. doi: 10.1111/1753-0407.13011
33. Khosla S, Melton LJ 3rd, Robb RA, Camp JJ, Atkinson EJ, Oberg AL, et al. Relationship of Volumetric BMD and Structural Parameters at Different Skeletal Sites to Sex Steroid Levels in Men. J Bone Miner Res (2005) 20(5):730–40. doi: 10.1359/JBMR.041228
34. Khosla S, Melton LJ 3rd, Atkinson EJ, O’Fallon WM, Klee GG, Riggs BL. Relationship of Serum Sex Steroid Levels and Bone Turnover Markers With Bone Mineral Density in Men and Women: A Key Role for Bioavailable Estrogen. J Clin Endocrinol Metab (1998) 83(7):2266–74. doi: 10.1210/jcem.83.7.4924
35. Venkat K, Desai M, Arora MM, Singh P, Khatkhatay MI. Age-Related Changes in Sex Steroid Levels Influence Bone Mineral Density in Healthy Indian Men. Osteoporos Int (2009) 20(6):955–62. doi: 10.1007/s00198-008-0765-1
36. Amin S, Zhang Y, Sawin CT, Evans SR, Hannan MT, Kiel DP, et al. Association of Hypogonadism and Estradiol Levels With Bone Mineral Density in Elderly Men From the Framingham Study. Ann Intern Med (2000) 133(12):951–63. doi: 10.7326/0003-4819-133-12-200012190-00010
37. Woo J, Kwok T, Leung JC, Ohlsson C, Vandenput L, Leung PC. Sex Steroids and Bone Health in Older Chinese Men. Osteoporos Int (2012) 23(5):1553–62. doi: 10.1007/s00198-011-1552-y
38. Södergård R, Bäckström T, Shanbhag V, Carstensen H. Calculation of Free and Bound Fractions of Testosterone and Estradiol-17 Beta to Human Plasma Proteins at Body Temperature. J Steroid Biochem (1982) 16(6):801–10. doi: 10.1016/0022-4731(82)90038-3
39. Manni A, Pardridge WM, Cefalu W, Nisula BC, Bardin CW, Santner SJ, et al. Bioavailability of Albumin-Bound Testosterone. J Clin Endocrinol Metab (1985) 61(4):705–10. doi: 10.1210/jcem-61-4-705
40. Riggs BL, Khosla S, Melton LJ 3rd. Sex Steroids and the Construction and Conservation of the Adult Skeleton. Endocr Rev (2002) 23(3):279–302. doi: 10.1210/edrv.23.3.0465
41. Sims NA, Clément-Lacroix P, Minet D, Fraslon-Vanhulle C, Gaillard-Kelly M, Resche-Rigon M, et al. A Functional Androgen Receptor Is Not Sufficient to Allow Estradiol to Protect Bone After Gonadectomy in Estradiol Receptor-Deficient Mice. J Clin Invest (2003) 111(9):1319–27. doi: 10.1172/JCI17246
42. Vidal O, Lindberg MK, Hollberg K, Baylink DJ, Andersson G, Lubahn DB, et al. Estrogen Receptor Specificity in the Regulation of Skeletal Growth and Maturation in Male Mice. Proc Natl Acad Sci U S A (2000) 97(10):5474–9. doi: 10.1073/pnas.97.10.5474
43. Xu XM, Li N, Li K, Li XY, Zhang P, Xuan YJ, et al. Discordance in Diagnosis of Osteoporosis by Quantitative Computed Tomography and Dual-Energy X-Ray Absorptiometry in Chinese Elderly Men. J Orthop Translat (2018) 18:59–64. doi: 10.1016/j.jot.2018.11.003
44. Kugel H, Jung C, Schulte O, Heindel W. Age- and Sex-Specific Differences in the 1H-Spectrum of Vertebral Bone Marrow. J Magn Reson Imaging (2001) 13(2):263–8. doi: 10.1002/1522-2586(200102)13:2<263::aid-jmri1038>3.0.co;2-m
45. Griffith JF, Yeung DK, Ma HT, Leung JC, Kwok TC, Leung PC. Bone Marrow Fat Content in the Elderly: A Reversal of Sex Difference Seen in Younger Subjects. J Magn Reson Imaging (2012) 36(1):225–30. doi: 10.1002/jmri.23619
46. Mistry SD, Woods GN, Sigurdsson S, Ewing SK, Hue TF, Eiriksdottir G, et al. Sex Hormones are Negatively Associated With Vertebral Bone Marrow Fat. Bone (2018) 108:20–4. doi: 10.1016/j.bone.2017.12.009
47. Liu P, Ji Y, Yuen T, Rendina-Ruedy E, DeMambro VE, Dhawan S, et al. Blocking FSH Induces Thermogenic Adipose Tissue and Reduces Body Fat. Nature (2017) 546(7656):107–12. doi: 10.1038/nature22342
48. Curtis E, Litwic A, Cooper C, Denison E. Determinants of Muscle and Bone Aging. J Cell Physiol (2015) 230(11):2618–25. doi: 10.1002/jcp.25001
49. Page ST, Amory JK, Bowman FD, Anawalt BD, Matsumoto AM, Bremner WJ, et al. Exogenous Testosterone (T) Alone or With Finasteride Increases Physical Performance, Grip Strength, and Lean Body Mass in Older Men With Low Serum T. J Clin Endocrinol Metab (2005) 90(3):1502–10. doi: 10.1210/jc.2004-1933
Keywords: bone mineral density, marrow adiposity, muscle adiposity, follicle-stimulating hormone, men
Citation: Xu L, Zhao Q, Li K, Zhang Y, Wang C, Hind K, Wang L, Liu Y and Cheng X (2022) The Role of Sex Hormones on Bone Mineral Density, Marrow Adiposity, and Muscle Adiposity in Middle-Aged and Older Men. Front. Endocrinol. 13:817418. doi: 10.3389/fendo.2022.817418
Received: 18 November 2021; Accepted: 31 January 2022;
Published: 21 February 2022.
Edited by:
Abdul Malik Tyagi, Emory University, United StatesReviewed by:
Bing Han, Shanghai Jiao Tong University, ChinaVikram Khedgikar, Brigham and Women’s Hospital and Harvard Medical School, United States
Copyright © 2022 Xu, Zhao, Li, Zhang, Wang, Hind, Wang, Liu and Cheng. This is an open-access article distributed under the terms of the Creative Commons Attribution License (CC BY). The use, distribution or reproduction in other forums is permitted, provided the original author(s) and the copyright owner(s) are credited and that the original publication in this journal is cited, in accordance with accepted academic practice. No use, distribution or reproduction is permitted which does not comply with these terms.
*Correspondence: Xiaoguang Cheng, eGlhbzY1QDI2My5uZXQ=
†These authors have contributed equally to this work and share first authorship