- 1Department of Obstetrics and Gynecology, Shengjing Hospital of China Medical University, Shenyang, China
- 2Neonatal Division of the Department of Pediatrics, The Fourth Affiliated Hospital of Harbin Medical University, Harbin, China
- 3Department of Radiology, The Fourth Affiliated Hospital of Harbin Medical University, Harbin, China
- 4Department of Colorectal Cancer Surgery, Shengjing Hospital of China Medical University, Shenyang, China
- 5Department of General Surgery, Shengjing Hospital of China Medical University, Shenyang, China
Oxytocin (OT) is a nonapeptide mainly produced in the supraoptic and paraventricular nuclei. OT in the brain and blood has extensive functions in both mental and physical activities. These functions are mediated by OT receptors (OTRs) that are distributed in a broad spectrum of tissues with dramatic sexual dimorphism. In both sexes, OT generally facilitates social cognition and behaviors, facilitates parental behavior and sexual activity and inhibits feeding and pain perception. However, there are significant differences in OT levels and distribution of OTRs in men from women. Thus, many OT functions in men are different from women, particularly in the reproduction. In men, the reproductive functions are relatively simple. In women, the reproductive functions involve menstrual cycle, pregnancy, parturition, lactation, and menopause. These functions make OT regulation of women’s health and disease a unique topic of physiological and pathological studies. In menstruation, pre-ovulatory increase in OT secretion in the hypothalamus and the ovary can promote the secretion of gonadotropin-releasing hormone and facilitate ovulation. During pregnancy, increased OT synthesis and preterm release endow OT system the ability to promote maternal behavior and lactation. In parturition, cervix expansion-elicited pulse OT secretion and uterine OT release accelerate the expelling of fetus and reduce postpartum hemorrhage. During lactation, intermittent pulsatile OT secretion is necessary for the milk-ejection reflex and maternal behavior. Disorders in OT secretion can account for maternal depression and hypogalactia. In menopause, the reduction of OT secretion accounts for many menopausal symptoms and diseases. These issues are reviewed in this work.
1 Introduction
Why should we concern women’s health and diseases? Women exhibit menstrual cycle, pregnancy, parturition, lactation, menopause and other unique physiological activities, such as maternal behaviors. Correspondingly, women have some unique reproduction-associated diseases, such as postpartum depression and menopausal syndromes. Women’s biological activities are regulated not only by the hypothalamic-pituitary-gonad (HPG) axis, but also by oxytocin (OT). While OT commonly influences sexual behaviors, production of sex steroids and the maturation of gemmates of both sexes (1), it differently influences women’s health and disease at different reproductive stages. In this work, we review the roles of OT in women’s health and disease.
2 General View of the OT System
OT, a classical neuropeptide, is mainly produced in hypothalamic OT neurons. Changes in OT neuronal activity can modulate cognitive, endocrine and physical activities as well as autonomic and visceral neural activities. In addition, scattered OT cells are also present in extrahypothalamic brain regions and peripheral sites, exerting autocrine and paracrine functions at local levels.
2.1 Histological Features of the OT System
OT neurons in the brain are largely aggregated in several neuroendocrine nuclei, typically the supraoptic nucleus (SON) and paraventricular nucleus (PVN). In the SON and PVN, most OT neurons send axons to the posterior pituitary and are magnocellular neuroendocrine cells. These OT neurons can release OT into the blood from OTergic terminals in the posterior pituitary and into the forebrain from OTergic axon collaterals (2). Another type of OT neurons in the CNS is parvocellular OT neurons. They are mainly present in the parvocellular division of the PVN and project to the brainstem and spinal cord but not the posterior pituitary (3). Alongside the hypothalamic OT neurons, some OT cells are also present in extrahypothalamic regions in the CNS and peripheral sites, such as amygdala, the median preoptic nucleus, uterus, placenta, amnion, corpus luteum, testis, heart and colon (4–6). Notably, OT gene expression in chorio-decidual tissues can increase three- to fourfold around the time of labor onset (7–10), supporting OT functional role in parturition. These histological features allow OT to modulate body functions at multiple levels and patterns including neuromodulation, neurosecretion, endocrine, autocrine and paracrine effects.
2.2 Features of OT Neuronal Secretion and Its Regulation
OT release from OT neurons is microdomain-specific (11). In response to changes in the neurochemical environment around OT neurons, changes in cytosolic Ca2+ level and/or firing activity occur. Increased firing rate causes OT release at the axonal terminals via excitation-secretion coupling. Increased cytosolic Ca2+ level triggers OT release from somata and dendrites. The somatodendritic OT release can be in synchrony with the firing activity under physiological conditions, such as suckling stimulation (12). It can be independent of the firing activity under some pathological conditions, such as maternal depression following offspring deprivation (13) and cesarean delivery (14).
The electrical activity, cytosolic Ca2+ level and the resultant OT secretion are under the regulation of extracellular and intracellular factors (Figure 1). These factors include changes in neurochemical environment (15), tonic and clustered synaptic inputs (16, 17), intercellular junctional coupling such as connexin 36 (18), and astrocytic plasticity (19, 20). Moreover, autoregulation of OT neuronal activity is a key regulator of OT neuronal activity (21). By activation of OT receptors (OTRs), OT causes activation of a series of intracellular signaling events, such as increased cyclooxygenase-2 (21), extracellular signal-regulated protein kinases 1 and 2 (22), and protein kinase A (13). These signals can activate hyperpolarization-activated cyclic nucleotide-gated channel 3 (13, 23), which can promote OT secretion (24, 25). Under the regulation of these extracellular and intracellular factors, OT neuronal activity and OT secretion can meet the demands of body activity in response to environmental changes.
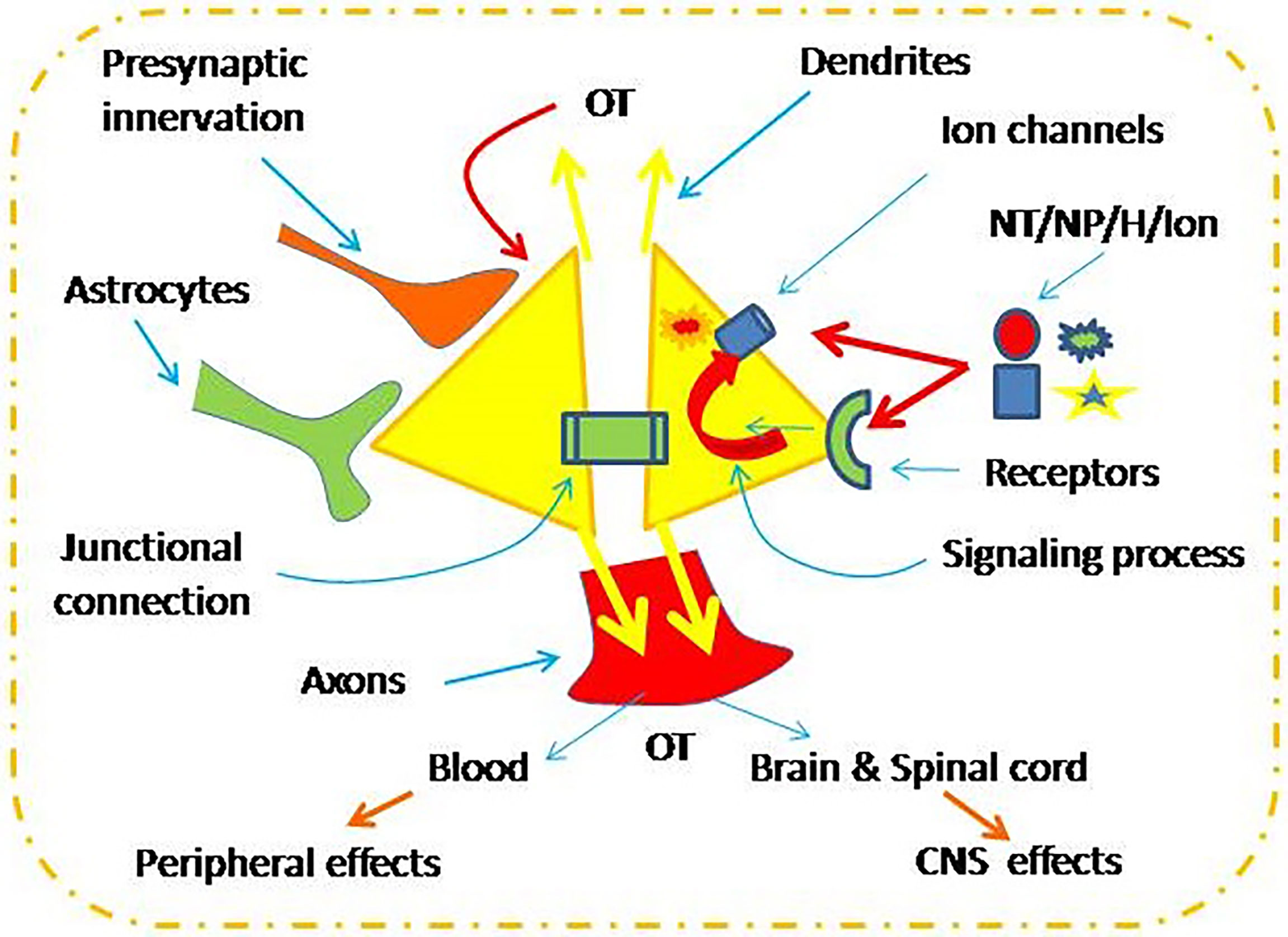
Figure 1 Neurochemical regulation of oxytocin (OT) neuronal activity. CNS, the central nervous system; NT/NP/H/Ion, neurotransmitters, neuropeptide, hormone and ions.
2.3 Expression of OTRs and Its Regulation
OTRs are the protein product encoded by OTR gene that is localized at 3p25-3p26.2 in the human chromosome (4). OTR belongs to the G-protein-coupled receptor superfamily and its activation is regulated by cholesterol as an allosteric modulator (26). The expression profile of OTRs is a tissue- and stage-specific, such as upregulation of the nuclear fractions in term myometrium and down-regulation in non-pregnant myometrium. The OTR gene appears to be highly methylated. Methylation around intron 1 and in intron 3 might contribute to tissue-specific suppression of the gene. OTRs are also regulated by the mechanisms of desensitization, which causes the loss of ligand-binding activity of the protein as well as suppression of OTR mRNA transcription. OTR mRNAs are present in different sizes, for instance 3.6 kb in human breast and 4.4 kb in ovary, endometrium, and myometrium. In posttranslational modifications, OTRs are further palmitoylated and glycosylated (27, 28). In addition, species differences are present and may be due to the existence of different clones of OTR genes of the myometrium and the hypothalamus at different reproductive stages (29, 30). Thus, the phenotype of OTRs from different species and different tissues of the same species could appear in different sizes.
In mammals, OTRs have been identified in a broad spectrum of tissues, including the kidney, heart, thymus, pancreas, adipocytes and other sites in addition to the CNS (4). Expressions of OTRs in the hypothalamus, uterus, and mammary glands are stimulated by estrogen (31). In females, OTRs are specifically localized in the myoepithelial cells of the mammary glands, and in the myometrium and endometrium of the uterus. Peripheral actions of OT are commonly associated with smooth muscle contraction, particularly within the female and male reproductive tracts (32).
OT expression during pregnancy and parturition in females has some unique features unseen in males. Changes in brain OT binding sites during pregnancy may influence the sudden onset of maternal behavior in female rats at parturition. Transcriptional regulation of OTR gene expression mediates changes in receptor density in the brain in a region-specific manner during pregnancy, such as the uterus (33), the PVN, SON, the bed nucleus of stria terminalis (BNST) and the medial preoptic area (mPOA) (34). Moreover, peak OTR mRNA expression was observed at parturition in the SON, brainstem regions, mPOA, BNST, and olfactory bulbs. Postpartum OTR expression in all brain regions returned to levels observed in virgin rats (35). These features of OTR expression are in agreement with the demands of establishing maternal behaviors and parturition.
Correspondingly, the uterus transitions from a quiescent non-contractile state to an active contractile state at the end of pregnancy. This is in association with increased OT/OTR signals (10, 36). Uterine quiescence requires prevention of excessive Ca2+ influx through voltage-dependent Ca2+channels at the human myometrial smooth muscle cells. Through most time of pregnancy, the K+ leak current is dominant and maintains the cell at a sufficiently negative membrane potential to prevent premature uterine contraction. However, increased myometrial OT/OTR expression near the term (10, 36) significantly increases OTR-associated Gαq-protein activation of protein kinase C (33), which then inhibits the activity of sodium-activated, high-conductance, K+ leak channel. This results in depolarization of the uterine smooth muscle cells and calcium entry that causes uterine contraction (37). This effect is associated with the induction of cyclooxygenase 2, production of prostaglandin F2α and connexin 43 of the uterus (38, 39).
3 OT Functions and Sexual Dimorphism
3.1 General Functions
The functions of OT depend on the distribution of OTRs and relative change in OT levels. In the brain and spinal cord, activation of OTRs is associated with a variety mental activities, such as social memory, pair-bonding, maternal behavior, and aggression and instinctive behaviors such as sexual activity, anxiety, feeding and pain perception (40–42). In the circulation, OT can facilitate parturition and the milk-ejection reflex and regulate activities of other organ systems (43, 44). Locally-produced OT can promote the differentiation of thymic cells, inhibit inflammation (45), protect the heart from ischemic injury (46), and suppress metastasis of colorectal cancer (5) among many other functions (1, 4).
In the CNS sites, OT can regulate social activity, instinctive behaviors, and visceral functions. For example, by activation of inhibitory neurotransmission in the medial frontal cortex, the amygdala and hippocampus, OT can promote social recognition and pro-social behaviors while reducing stress and fear (47–49). Released from parvocellular OT neurons in the PVN, OT can act on the ventral tegmental area (VTA) to activate rewarding process. OT can suppress nociception ad pain by acting on the periaqueductal gray and spinal cord. By acting on the dorsal vagal complex, OT can regulate visceral activities via vagus. By innervating median eminence and median preoptic area, OT can increase gonadotropin-releasing hormone (GnRH) release and the activity of HPG axis (1, 50). In addition, intrahypothalamic OT can inhibit corticotrophin-releasing hormone neurons and social stress via acting on the parvocellular division of the PVN (51).
By contrast, circulating and locally-produced OT can influence body functions at cellular, tissue, organ and system levels. For instance, OT can promote insulin secretion (52) and immunological homeostasis (53), protects cardiovascular system (46), suppresses colorectal cancer migration (5), and potentially antagonizes COVID-19 pathogenesis (54). In addition, OT is a natriuretic hormone that acts directly on the kidneys (55). On the other hand, its synthesis and secretion are regulated by changes in plasma osmolality and blood volume (56, 57). This process proceeds in an estrogen-dependent manner in females (58, 59). Thus, OT extensively modulates body functions.
3.2 Sexual Dimorphism of OT Functions
In studies on OT functions, significant difference between males and females emerges, which is particularly significant in psychological and reproductive functions.
3.2.1 OT/OTR Signaling
Sexual dimorphism of OT functions is based on expression levels of OT and OTRs. For instance, serum OT levels are significantly higher among women than men (60), which makes women more sensitive to OT level reduction and likely accounts for menstrual pain (61) and higher incidence of depression in women (62). By contrast, OT binding sites in the VMH and dorsal horns are significantly high in males relative to females (63), which may contribute to the central regulatory actions of OT on feeding, reproduction via VMH (64) and nociception via the spinal cord (65). Males also exhibit higher OTR levels in the medial amygdala irrelevant to the reproductive status (66), which likely makes men less fearful facing stressful challenges because OT acing on the medial amygdala inhibits fear. Higher OTR levels in the nucleus accumbens are present at breeding males but not breeding females (66), which makes paternal behaviors conditional (67) and more rewarding (68). The sex dimorphism in the distribution OT and OTRs sets a histological basis for gender-specific functions and behaviors.
3.2.2 Psychological Functions
The most relevant OT-associated sexual dimorphism is present in empathy, social skills and other higher brain functions. OT facilitates familiar-partner preference with females being more significant and it increases trust in others and reduces anxious emotion, especially for males (69). Autism spectrum disorder is relatively low in the female gender. This is related to the higher levels of OT and better pragmatic language in girls than boys (70). In nulliparous women, OT enhances attention to the baby schema and morphological characteristics of an infant’s face (71). In animal studies, the sexual dimorphisms of OT psychological functions are also identified. For example, in prairie vole, compared to females, males with less OTR expression perform better than females in a spatial memory and spatial learning test (72). Intranasal administration of OT (IAO) before the acquisition or recall sessions enhances conditioned safety memory in female rats while OT has no effects in male rats (73). In male prairie voles exposure to IAO during the peri-adolescent period impairs adult pair bonding in a dose-dependent fashion while IAO appears to facilitate pair bonding in females. This is associated with that IAO in females but not in males increases OTR binding in the nucleus accumbens shell (74). Thus, differences in the OT/OTR signaling determine sex dimorphisms between males and females.
3.2.3 Peripheral OT Effects
In association with hypothalamic OT neuronal activity and OT secretion, OT can extensively modulate body activities at peripheral sites. In the rat with left ventricle infarction, OTR is down-regulated in females while up-regulated in males. Thus, OTR signaling and OT protection are suppressed stronger in ischemic myocardium in females than males. It also accounts for why females have higher risk of heart failure and death following myocardial infarction relative to males (75). Moreover, OT is involved in bone formation in both sexes during development; however, OT treatment has no effect on male osteoporosis because estrogen amplifies OT local autocrine and paracrine secretion (76). Decreased OT and increased OTR occur in male but not female alcohol- dependent rats and patients (77). Thus, differences in OT/OTR signaling also exist in the sex dimorphism of circulation and peripheral OT functions.
3.2.4 Male Reproductive Functions
Among all OT functions, the most dramatic sexual dimorphism is reproductive functions. Relative to the periodic changes in females’ reproductive physiology, males do not have dramatic monthly periodic alteration in reproductive endocrine activity and reproductive functions. It is known that plasma OT levels increase during sexual arousal and are significantly higher during orgasm/ejaculation in both women and men (78). In the CNS levels, OT from the PVN in the VTA of rats induces penile erection by activating OT neurons-spinal cord pathway (79). This pathway involves activation of dopamine, glutamate and other neurons in the VTA that project to nucleus accumbens, prefrontal cortex, amygdala, and other forebrain regions (80). Moreover, this OT-PVN-VTA-forebrain pathways play a role in the motivational and rewarding aspects of sexual behavior (81). At peripheral sites, OT directly targets the erectile tissues including corpus spongiosum and corpus cavernosum, and promotes ejaculation by increasing sperm number and contracting ejaculatory tissues (82). Thus, OT is a pivotal regulator of male reproductive functions.
3.3 Maternal Behavior
Maternal behavior begins before breastfeeding near the term and is clearly associated with the actions of OT. For example, in OT knockout mice, maternal behavior is disrupted (83) and maternal behavior is reduced significantly when OTR signaling is blocked (84). By contrast, IAO rapidly increases maternal care in mice (85). It is believed that OT neurons in the PVN are sensitive for the smell of offspring in lactating and multiple-parturient rats (86). OT neurons in the PVN and SON project to the hippocampus, amygdala, ventral striatum, hypothalamus, nucleus accumbens and brainstem nuclei; they can extensively modulate maternal behaviors (2, 3). By modulation of the maternal behaviors, OT convincingly promotes human development as recently reviewed (87, 88).
Notably, women with peripartum exposure to synthetic OT have a higher relative risk of depressive or anxiety disorder diagnosis or antidepressant/anxiolytic prescription within the first year postpartum than women without synthetic OT exposure (89). This is likely due to the suppression of excessive OT on OT neuronal activity via a mechanism of post-excitation inhibition of OT neuronal activity (14, 23, 90).
3.4 OT and Diseases
Under pathological conditions, reduced OT release or disorders in OTR signaling can cause many diseases, such as social stress (51), schizophrenia and depression (91), obesity (92), lactation failure, postpartum depression (13, 13) and even mammary tumor (93). For instance, schizophrenia is a form of mental disorders and involves dysregulation of the OT system. From animal models to human studies, observations have revealed that OT can improve the psychopathology of patients with schizophrenia by regulating social cognition and behavior (94). Postpartum depression is associated with disorders on maternal OT system. As reported in rats, lack of suckling stimulation and bolus OT release during lactation can decrease maternal blood OT levels and cause milk insufficiency and maternal depression in lactating mothers deprived of offspring (13, 95) or following cesarean delivery (14). However, disrupted maternal behavior and lactation performance can be largely improved by IAO during offspring deprivation (13) or following cesarean delivery (14). Obviously, disorder in OT system activity contributes to disorders in mental activity while IAO has the potential to correct abnormal social behaviors such as those in schizophrenia and postpartum depression.
Notably, in mediating OT actions, the efficiency of OTRs depends on their gene polymorphisms, expression levels and posttranslational modification (96–98). For example, the GG homozygotes of OTR rs2254298 are associated with childhood adversity (99); DNA methylation in the 1st intron of the OTR gene causes common learning and behavioral impairments (100). Thus, OT-associated diseases are not only associated with disorders in OT secretion but also with abnormality of OT/OTR signaling.
4 OT Functions at Different Reproductive Stages of Women
4.1 Menstrual Cycle
It is well established that a menstrual cycle is regulated by HPG axis. However, studies also highlight a pivotal role of OT in menstrual cycle. For instance, increased brain OT level can increase GnRH secretion, specifically at pre-ovulation stage (101, 102). Consistently, plasma OT is significantly higher during ovulatory phases than the luteal phase in ovulating women (103). In rats, c-Fos expression in the SON is significantly higher and OT neurons-associated astrocytic processes retract significantly during the proestrus (104, 105). These findings support functional activation of OT neurons and increased OT release before ovulation. Thus, the stimulatory effect of OT on GnRH secretion can promote luteinizing hormone (LH) secretion, facilitate ovulation and prepare uterus environment for pregnancy.
By contrast, exogenous OT can shorten postpartum estrous interval but triggering a delay in ovulation while a higher dose of OT could stimulate the growth of small, medium, and total follicles in postpartum buffaloes (106). Estradiol level increase is correlated with OT release from the pituitary and causes more luteal OT secretion (107). Thus, OT secretion and estrogen release before luteal phase can form a positive feedback loop and they together facilitate ovulation (Figure 2A).
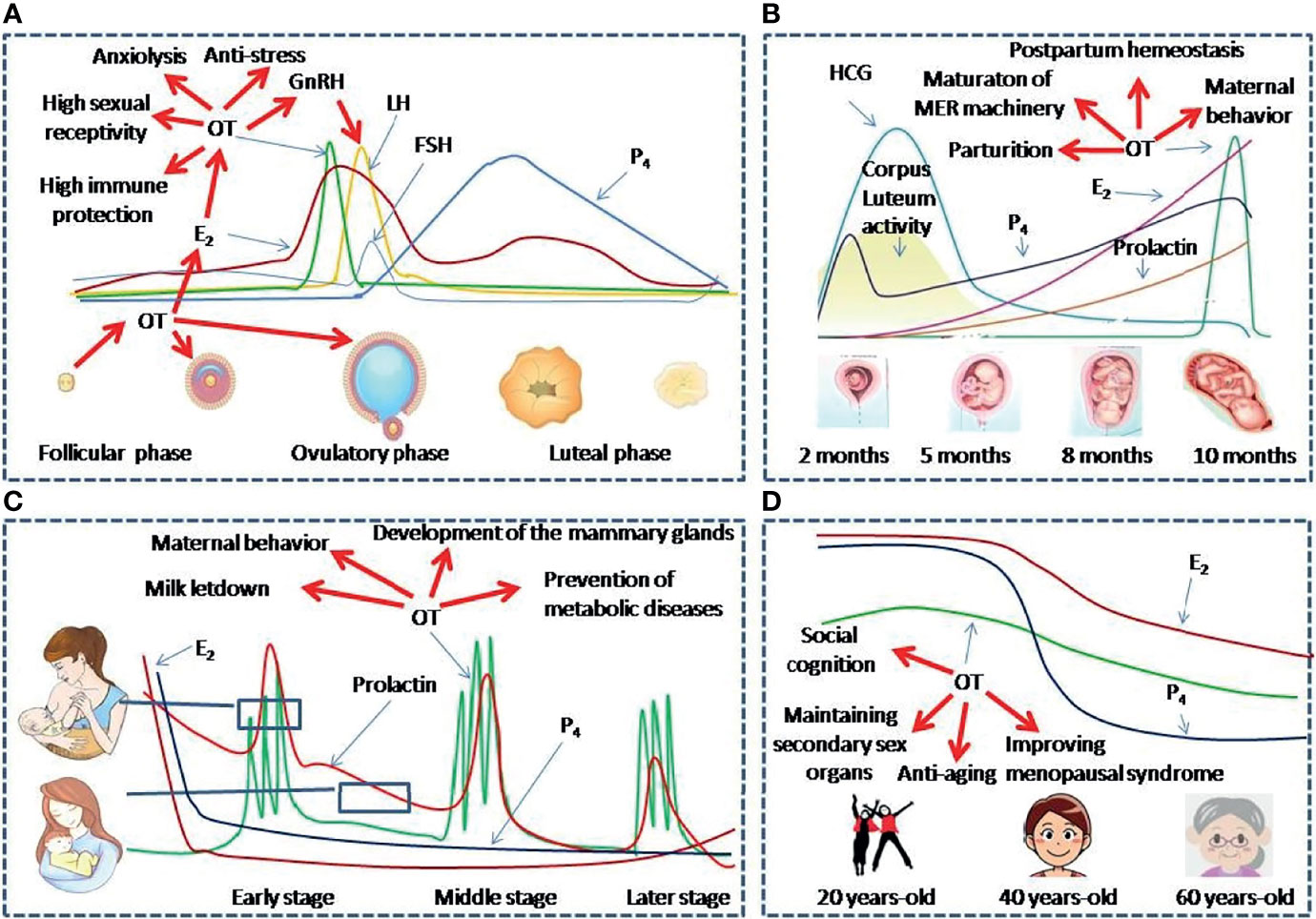
Figure 2 OT functions at different reproductive stages of women. (A–D) Panels show levels and functions of OT and associated reproductive hormones during Menstruation (A), Pregnancy and parturition (B), Lactation (C) and Peri-menopausal stage (D), respectively. E2, estradiol; FSH, follicle-stimulating hormone; GnRH, gonadotropin-releasing hormone; HCG, Human Chorionic Gonadotropin; LH, Luteinizing hormone; MER, the milk-ejection reflex; P4, progesterone.
During pregnancy, persistent release of uterine progesterone and estrogen interrupts the periodical activity of the HPG axis. Moreover, increased progesterone also inhibits OT release (108). They together cause the cessation of menstrual cycles. Following parturition, breastfeeding delays the resumption of normal ovarian cycles by disrupting the pattern of pulsatile release of GnRH from the hypothalamus. Intermittent bolus release of OT during suckling, its disruption of normal interaction of OT with the HPG axis and inhibition of energy intake also play a key role in lactation-associated amenorrhea (92). When suckling stimulus declines near weanling, preovulatory LH surge restores and ovulation takes place with the formation of a corpus luteum of variable normality.
By contrast, women with a history of primary or secondary dysmenorrhea have lower blood OT concentrations during menses, which is associated with worse menstrual pain and pain-related behavior (61). In women with early life sexual abuse, OT can positively modulate menstruation-related mood disorders (109). In addition, OT can enhance conditioned safety memory (73), elicit maternal behavior towards alien pups in virgin females (110), alleviate chronic pelvic pain (111) and rehabilitate anorexia nervosa (112). Thus, OT extensively modulates mental and physical activities and menstruation-associated diseases.
4.2 Pregnancy and Parturition
4.2.1 OT and Pregnancy
During pregnancy as marked by the production of human chorionic gonadotropin, OT production increases gradually and prepares for the demands of parturition and breastfeeding (Figure 2B). As reported, gestation gradually increases OT synthesis and OTR expressions in magnocellular OT neurons in the SON and PVN and in forebrain neurons, such as the mPOA (34). However, OT release from OT neurons does not increase during pregnancy until the time shortly before parturition. This is clearly beneficial for avoiding abortion during pregnancy. By contrast, blockade of OTR during mid-late gestation delays OT release and causes hypogalactia during lactation (113). Thus, the development of OT/OTR signaling is an adaptive response for maintaining the safety of pregnancy. However, increased OT synthesis and preterm OT release in the hypothalamus are necessary for the maturation of hypothalamic machinery that allows OT to be released in bolus intermittently during parturition and lactation (113). Thus, OT actions during pregnancy highly match peri-partum physiological demands.
4.2.2 OT and Parturition
Shortly before the parturition, progesterone inhibition of OT neuronal excitability via endogenous opioids and GABA is weakened (108), which allows OT release in bolus in response to extension of cervix. The mechanical stretch of the cervix can activate magnocellular OT neurons in burst-like firing activity, which determines a bolus release of OT from the posterior pituitary (114). OT further initiates a self-sustaining cycle of uterine contractions until fetus is expelled. In this process, uterine OT release is also increased, which intensifies circulating OT-evoked uterine contraction by increasing prostaglandin F production. As a result, parturition is accelerated (115) and postpartum hemorrhage is reduced (116). Thus, OT is an essential factor for natural delivery.
Notably, nocturnal and pulsatile OT release often occurs at the end of parturition. For example, plasma OT and nocturnal uterine activity in the dams increase progressively during late pregnancy and delivery in rhesus monkeys (117). This is associated with the effect of light/darkness on the pulsatile OT release (118), which determines the high incidence of parturition during night.
4.3 Lactation
In all mammals, OT is a hormone necessary for mothers to nurse their offspring through the milk-ejection reflex. Clearly, in OT-knockout mice, the pups cannot obtain milk from the mother because the dams fail to eject milk for the offspring to obtain through suckling the nipples (119). Consistently, conditioned OTR knockout dams experience high rate of pup mortality (120).
Successful breastfeeding depends on coordinated activities of numerous humoral factors. For instance, the increased hypothalamic OT release during suckling promotes prolactin secretion from the anterior pituitary. They together make milk production and ejection available for the baby during breastfeeding (121). This effect is different from the increased prolactin during pregnancy that acts to maintain the corpus luteum of the ovary and helps to sustain pregnancy but has no direct association with OT release (122). Under physiological condition and normal nutrition, breastfeeding in normally developed women relies on the milk-ejection reflex. In this reflex, suckling stimulation at the nipples activates OT neurons, causes OT release in a bolus into the blood, and results in the ejection of milk from the teat (Figure 2C). The activation of OT neurons appears as intermittently recurrent and simultaneous increase in the firing rate in a large pool of OT neurons over several seconds, which causes the bolus release of OT at OTergic neural terminals in the posterior pituitary (44). Without this synchronized burst firing of OT neurons, OT release from one or a few of OT neurons or only one side of the hypothalamus cannot trigger full milk ejections (123, 124). In the synchronized activation of OT neurons, simultaneous retraction of astrocytic processes from OT neurons, shared synapses, increased gap junctional coupling and cellular apposition are logically important contributors (44). However, for coordinated inter-nuclear burst synchrony among OT neurons, a synchronized signal from the mammillary body complex in the ventroposterior hypothalamus is the most important event. This is because disruption of it but not other brain regions disrupts inter-nuclear burst synchrony (125, 126).
Under the drive of exogenous factors including synaptic input, astrocytic plasticity and interneuronal interactions (Figure 1), a series of OTR-associated signaling events are activated during suckling. These events include mobilization of βɣ subunits (16), induction of cyclooxygenase 2 and prostaglandin production (21), phosphorylation of extracellular signal-regulated protein kinase 1 and 2 (22), activation of protein kinase A (13, 127), and filamentous actin reorganization (21, 22) among many others (44). These factors express in a different spatiotemporal order to modulate ion channel activity on the membrane (128), such as hyperpolarization-activated cyclic nucleotide-gated channel 3 (13, 23), and thus trigger burst firing in OT neurons and OT release. Nevertheless, detailed neural circuit between OT neurons and their regulatory network and the cellular mechanisms underlying burst firing remain to be explored.
About lactation-associated health issue, it has been extensively accepted that normal breastfeeding can reduce the incidence of postpartum depression, maternal obesity, diabetes, and even breast cancer. OT is necessary for these benefits of breastfeeding. As revealed in animal study, maternal behavior in OT knockout mice is incomplete (83). Lack of suckling stimulation and bolus OT release during lactation can decrease maternal blood OT levels and cause hypogalactia and maternal depression (13, 95). By contrast, IAO can largely restore maternal behavior and lactation performance as stated above. Importantly, OT can also suppress breast cancer. This is because OT can reduce the oxidative stress of the mammary glands and the occurrence of pre-cancer lesions in the mammary glands (129). Moreover, OT can down-regulate the NF-κB and up-regulation of miR-195. These molecules can promote cell apoptosis, inhibit cell proliferation and consequently, decrease the mammary tumor volume and weight (130). Thus, OT is not only essential for normal breastfeeding but also for the long-term health benefits of women.
4.4 Menopause
Menopause is the end of women’s menstrual cycles following decrease of reproductive hormones. From the middle age, blood OT levels decrease gradually (131). Decreased levels of OT can largely account for diminished sexual ability and vagal activity and reduced estrogen levels (Figure 2D).
4.4.1 Sex Organs and Bone Metabolism
Menopausal atrophy of accessory reproductive organs is a common sign of reduced ovary functions. However, topical OT application can reverse vaginal atrophy (132, 133). Similarly, a high proportion of women develop osteoporosis after menopause, which increases the incidence of bone fractures. In animals, ovariectomy elicits bone loss and increased bone marrow adiposity (134). Administration of OT can normalize ovariectomy-induced osteopenia in mice by restoring osteoblast/osteoclast cross talk via the receptor activator of nuclear factor-κB ligand/osteoprotegerin axis (135). Thus, OT is a potential treatment of menopausal osteoporosis.
4.4.2 Body Mass
Weight gain in menopausal women has been frequently reported (136). Obesity and menopause are independent negative predictors of plasma OT levels (131). Daily administration of OT can normalize body weight and intraabdominal fat depots in ovariectomized mice. This effect is mediated by inhibition of adipocyte precursor’s differentiation with a tendency to lower adipocyte size by shifting fuel utilization favoring lipid oxidation (135). In peri- and postmenopausal female rats, intraperitoneally administering OT also reduces serum triglyceride and low-density lipoprotein-cholesterol levels in naturally premenopausal or menopausal rats (137). Thus, OT can be a preventive factor of postmenopausal obesity, diabetes and the associated cardiovascular diseases (46).
4.4.3 Cardiovascular Effects
Cardiovascular diseases increase dramatically in postmenopausal women. This is also associated with reduction of ovary functions and its influence on OT secretion. When estrogen production decreases, its activation of estrogen receptors on preautonomic PVN OT neurons is also weakened. Resultantly, OT regulation of HPG axis and baroreflex is weakened. This has been shown in ovariectomized rats (138). By contrast, OT can protect the cardiovascular system by maintaining cardiovascular integrity, suppressing atherosclerotic alterations and coronary artery disease, inhibiting metabolic disorders, inflammation and apoptosis and promoting regeneration and repair injuries (46).
As a whole, reduced OT secretion during aging can causes cardiovascular disease, osteoporosis, urinary incontinence, sexual malfunction, obesity, low metabolism after menopause. Thus, improvement of OT secretion can be an important strategy of anti-aging in women.
5 Conclusion
OT system can extensively modulate women’s physiology, particularly the cognitive and reproductive functions. While OT has common effects on the mental and physical activities of both men and women, different OT levels and OTR expressions at different reproductive stages regulate women’s reproductive activities differently. The influence of OT on women’s health mainly manifests as its modulation of menstrual cycle, pregnancy, production, lactation and menopause. Under pathological conditions, abnormality in OT secretion and/or OTR expressions can cause a series of female-specific diseases. Thus, further study on OT involvement in women’s health and disease is warranted. These studies should focus on women-specific mental and physical effects of OT and the underlying mechanisms, particularly during different stages of reproduction.
Author Contributions
NL and MM: Conceived the study, wrote, revised and edited the text. HY and LH: Discussed the key contents, revised text and drew the figures. All authors contributed to the article and approved the submitted version.
Conflict of Interest
The authors declare that the research was conducted in the absence of any commercial or financial relationships that could be construed as a potential conflict of interest.
Publisher’s Note
All claims expressed in this article are solely those of the authors and do not necessarily represent those of their affiliated organizations, or those of the publisher, the editors and the reviewers. Any product that may be evaluated in this article, or claim that may be made by its manufacturer, is not guaranteed or endorsed by the publisher.
References
1. Yang HP, Wang L, Han L, Wang SC. Nonsocial Functions of Hypothalamic Oxytocin. ISRN Neurosci (2013) 2013:179272. doi: 10.1155/2013/179272
2. Zhang B, Qiu L, Xiao W, Ni H, Chen L, Wang F, et al. Reconstruction of the Hypothalamo-Neurohypophysial System and Functional Dissection of Magnocellular Oxytocin Neurons in the Brain. Neuron (2021) 109:331–46.e337. doi: 10.1016/j.neuron.2020.10.032
3. Eliava M, Melchior M, Knobloch-Bollmann HS, Wahis J, Da Silva Gouveia M, Tang Y, et al. A New Population of Parvocellular Oxytocin Neurons Controlling Magnocellular Neuron Activity and Inflammatory Pain Processing. Neuron (2016) 89:1291–304. doi: 10.1016/j.neuron.2016.01.041
4. Gimpl G, Fahrenholz F. The Oxytocin Receptor System: Structure, Function, and Regulation. Physiol Rev (2001) 81:629–83. doi: 10.1152/physrev.2001.81.2.629
5. Ma M, Li L, Chen H, Feng Y. Oxytocin Inhibition of Metastatic Colorectal Cancer by Suppressing the Expression of Fibroblast Activation Protein-Alpha. Front Neurosci (2019) 13:1317. doi: 10.3389/fnins.2019.01317
6. Kelly AM, Seifert AW. Distribution of Vasopressin and Oxytocin Neurons in the Basal Forebrain and Midbrain of Spiny Mice (Acomys Cahirinus). Neuroscience (2021) 468:16–28. doi: 10.1016/j.neuroscience.2021.05.034
7. Mitchell BF, Chibbar R. Synthesis and Metabolism of Oxytocin in Late Gestation in Human Decidua. Adv Exp Med Biol (1995) 395:365–80.
8. Chibbar R, Wong S, Miller FD, Mitchell BF. Estrogen Stimulates Oxytocin Gene Expression in Human Chorio-Decidua. J Clin Endocrinol Metab (1995) 80:567–72. doi: 10.1210/jcem.80.2.7852522
9. Miller FD, Chibbar R, Mitchell BF. Synthesis of Oxytocin in Amnion, Chorion and Decidua: A Potential Paracrine Role for Oxytocin in the Onset of Human Parturition. Regul Pept (1993) 45:247–51. doi: 10.1016/0167-0115(93)90214-S
10. Chibbar R, Miller FD, Mitchell BF. Synthesis of Oxytocin in Amnion, Chorion, and Decidua may Influence the Timing of Human Parturition. J Clin Invest (1993) 91:185–92. doi: 10.1172/JCI116169
11. Ludwig M, Sabatier N, Bull PM, Landgraf R, Dayanithi G, Leng G. Intracellular Calcium Stores Regulate Activity-Dependent Neuropeptide Release From Dendrites. Nature (2002) 418:85–9. doi: 10.1038/nature00822
12. Augustine RA, Seymour AJ, Campbell RE, Grattan DR, Brown CH. Integrative Neuro-Humoral Regulation of Oxytocin Neuron Activity in Pregnancy and Lactation. J Neuroendocrinol (2018) (8):e12569. doi: 10.1111/jne.12569
13. Li D, Liu X, Liu H, Li T, Jia S, Wang X, et al. Key Roles of Cyclooxygenase 2-Protein Kinase A-Hyperpolarization-Activated Cyclic Nucleotide-Gated Channel 3 Pathway in the Regulation of Oxytocin Neuronal Activity in Lactating Rats With Intermittent Pup-Deprivation. Neuroscience (2021) 452:13–25. doi: 10.1016/j.neuroscience.2020.10.016
14. Li T, Jia SW, Hou D, Wang X, Li D, Liu Y, et al. Oxytocin Modulation of Maternal Behavior and Its Association With Immunological Activity in Rats With Cesarean Delivery. ASN Neuro (2021) 13:17590914211014731. doi: 10.1177/17590914211014731
15. Munesue SI, Liang M, Harashima A, Zhong J, Furuhara K, Boitsova EB, et al. Transport of Oxytocin to the Brain After Peripheral Administration by Membrane-Bound or Soluble Forms of Receptors for Advanced Glycation End-Products. J Neuroendocrinol (2021) 33:e12963. doi: 10.1111/jne.12963
16. Wang YF, Hatton GI. Dominant Role of Betagamma Subunits of G-Proteins in Oxytocin-Evoked Burst Firing. J Neurosci (2007) 27:1902–12. doi: 10.1523/JNEUROSCI.5346-06.2007
17. Popescu IR, Buraei Z, Haam J, Weng FJ, Tasker JG. Lactation Induces Increased IPSC Bursting in Oxytocinergic Neurons. Physiol Rep (2019) 7:e14047. doi: 10.14814/phy2.14047
18. Wang P, Wang SC, Li D, Li T, Yang HP, Wang L, et al. Role of Connexin 36 in Autoregulation of Oxytocin Neuronal Activity in Rat Supraoptic Nucleus. ASN Neuro (2019) 11:1759091419843762. doi: 10.1177/1759091419843762
19. Wang YF, Hatton GI. Astrocytic Plasticity and Patterned Oxytocin Neuronal Activity: Dynamic Interactions. J Neurosci (2009) 29:1743–54. doi: 10.1523/JNEUROSCI.4669-08.2009
20. Wang P, Qin D, Wang YF. Oxytocin Rapidly Changes Astrocytic GFAP Plasticity by Differentially Modulating the Expressions of pERK 1/2 and Protein Kinase A. Front Mol Neurosci (2017) 10:262. doi: 10.3389/fnmol.2017.00262
21. Wang YF, Hatton GI. Mechanisms Underlying Oxytocin-Induced Excitation of Supraoptic Neurons: Prostaglandin Mediation of Actin Polymerization. J Neurophysiol (2006) 95:3933–47. doi: 10.1152/jn.01267.2005
22. Wang YF, Hatton GI. Interaction of Extracellular Signal-Regulated Protein Kinase 1/2 With Actin Cytoskeleton in Supraoptic Oxytocin Neurons and Astrocytes: Role in Burst Firing. J Neurosci (2007) 27:13822–34. doi: 10.1523/JNEUROSCI.4119-07.2007
23. Li D, Liu H, Liu X, Wang H, Li T, Wang X, et al. Involvement of Hyperpolarization-Activated Cyclic Nucleotide-Gated Channel 3 in Oxytocin Neuronal Activity in Lactating Rats With Pup Deprivation. ASN Neuro (2020) 12:1759091420944658. doi: 10.1177/1759091420944658
24. Qiu DL, Chu CP, Tsukino H, Shirasaka T, Nakao H, Kato K, et al. Neuromedin U Receptor-2 mRNA and HCN Channels mRNA Expression in NMU-Sensitive Neurons in Rat Hypothalamic Paraventricular Nucleus. Neurosci Lett (2005) 374:69–72. doi: 10.1016/j.neulet.2004.10.033
25. Pires Da Silva M, De Almeida Moraes DJ, Mecawi AS, Rodrigues JA, Varanda WA. Nitric Oxide Modulates HCN Channels in Magnocellular Neurons of the Supraoptic Nucleus of Rats by an S-Nitrosylation-Dependent Mechanism. J Neurosci (2016) 36:11320–30. doi: 10.1523/JNEUROSCI.1588-16.2016
26. Lemel L, Niescierowicz K, Garcia-Fernandez MD, Darre L, Durroux T, Busnelli M, et al. The Ligand-Bound State of a G Protein-Coupled Receptor Stabilizes the Interaction of Functional Cholesterol Molecules. J Lipid Res (2021) 62:100059. doi: 10.1016/j.jlr.2021.100059
27. Kimura T, Saji F, Nishimori K, Ogita K, Nakamura H, Koyama M, et al. Molecular Regulation of the Oxytocin Receptor in Peripheral Organs. J Mol Endocrinol (2003) 30:109–15. doi: 10.1677/jme.0.0300109
28. Kiss A, Mikkelsen JD. Oxytocin–anatomy and Functional Assignments: A Minireview. Endocr Regul (2005) 39:97–105.
29. Kawamata M, Mitsui-Saito M, Kimura T, Takayanagi Y, Yanagisawa T, Nishimori K. Vasopressin-Induced Contraction of Uterus is Mediated Solely by the Oxytocin Receptor in Mice, But Not in Humans. Eur J Pharmacol (2003) 472:229–34. doi: 10.1016/S0014-2999(03)01914-9
30. Yoshida M, Takayanagi Y, Inoue K, Kimura T, Young LJ, Onaka T, et al. Evidence That Oxytocin Exerts Anxiolytic Effects via Oxytocin Receptor Expressed in Serotonergic Neurons in Mice. J Neurosci (2009) 29:2259–71. doi: 10.1523/JNEUROSCI.5593-08.2009
31. Richter ON, Kubler K, Schmolling J, Kupka M, Reinsberg J, Ulrich U, et al. Oxytocin Receptor Gene Expression of Estrogen-Stimulated Human Myometrium in Extracorporeally Perfused Non-Pregnant Uteri. Mol Hum Reprod (2004) 10:339–46. doi: 10.1093/molehr/gah039
32. Camerino C. The New Frontier in Oxytocin Physiology: The Oxytonic Contraction. Int J Mol Sci (2020) 21:5144. doi: 10.3390/ijms21145144
33. Yulia A, Johnson MR. Myometrial Oxytocin Receptor Expression and Intracellular Pathways. Minerva Ginecol (2014) 66:267–80.
34. Bealer SL, Lipschitz DL, Ramoz G, Crowley WR. Oxytocin Receptor Binding in the Hypothalamus During Gestation in Rats. Am J Physiol Regul Integr Comp Physiol (2006) 291:R53–8. doi: 10.1152/ajpregu.00766.2005
35. Meddle SL, Bishop VR, Gkoumassi E, Van Leeuwen FW, Douglas AJ. Dynamic Changes in Oxytocin Receptor Expression and Activation at Parturition in the Rat Brain. Endocrinology (2007) 148:5095–104. doi: 10.1210/en.2007-0615
36. Alotaibi MF. The Response of Rat and Human Uterus to Oxytocin From Different Gestational Stages In Vitro. Gen Physiol Biophys (2017) 36:75–82. doi: 10.4149/gpb_2016022
37. Ferreira JJ, Butler A, Stewart R, Gonzalez-Cota AL, Lybaert P, Amazu C, et al. Oxytocin can Regulate Myometrial Smooth Muscle Excitability by Inhibiting the Na(+) -Activated K(+) Channel, Slo2.1. J Physiol (2019) 597:137–49. doi: 10.1113/JP276806
38. Yoshida M, Takayanagi Y, Ichino-Yamashita A, Sato K, Sugimoto Y, Kimura T, et al. Functional Hierarchy of Uterotonics Required for Successful Parturition in Mice. Endocrinology (2019) 160:2800–10. doi: 10.1210/en.2019-00499
39. Devost D, Zingg HH, Hebert TE. The MAP Kinase ERK5/MAPK7 is a Downstream Effector of Oxytocin Signaling in Myometrial Cells. Cell Signal (2021) 90:110211. doi: 10.1016/j.cellsig.2021.110211
40. Lee HJ, Macbeth AH, Pagani JH, Young WS. Oxytocin: The Great Facilitator of Life. Prog Neurobiol (2009) 88:127–51. doi: 10.1016/j.pneurobio.2009.04.001
41. Insel TR. The Challenge of Translation in Social Neuroscience: A Review of Oxytocin, Vasopressin, and Affiliative Behavior. Neuron (2010) 65:768–79. doi: 10.1016/j.neuron.2010.03.005
42. Veening JG, De Jong T, Barendregt HP. Oxytocin-Messages via the Cerebrospinal Fluid: Behavioral Effects; a Review. Physiol Behav (2010) 101:193–210. doi: 10.1016/j.physbeh.2010.05.004
43. Viero C, Shibuya I, Kitamura N, Verkhratsky A, Fujihara H, Katoh A, et al. REVIEW: Oxytocin: Crossing the Bridge Between Basic Science and Pharmacotherapy. CNS Neurosci Ther (2010) 16:e138–156. doi: 10.1111/j.1755-5949.2010.00185.x
44. Hou D, Jin F, Li J, Lian J, Liu M, Liu X, et al. Model Roles of the Hypothalamo-Neurohypophysial System in Neuroscience Study. Biochem Pharmacol (Los Angel) (2016) 5:211. doi: 10.4172/2167-0501.1000211
45. Li T, Wang P, Wang SC, Wang YF. Approaches Mediating Oxytocin Regulation of the Immune System. Front Immunol (2016) 7:693. doi: 10.3389/fimmu.2016.00693
46. Wang P, Wang SC, Yang H, Lv C, Jia S, Liu X, et al. Therapeutic Potential of Oxytocin in Atherosclerotic Cardiovascular Disease: Mechanisms and Signaling Pathways. Front Neurosci (2019) 13:454. doi: 10.3389/fnins.2019.00454
47. Cherepanov SM, Gerasimenko M, Yuhi T, Furuhara K, Tsuji C, Yokoyama S, et al. Oxytocin Ameliorates Impaired Social Behavior in a Chd8 Haploinsufficiency Mouse Model of Autism. BMC Neurosci (2021) 22:32. doi: 10.1186/s12868-021-00631-6
48. Runyan A, Lengel D, Huh JW, Barson JR, Raghupathi R. Intranasal Administration of Oxytocin Attenuates Social Recognition Deficits and Increases Prefrontal Cortex Inhibitory Postsynaptic Currents Following Traumatic Brain Injury. eNeuro (2021) 8:ENEURO.0061-21.2021. doi: 10.1523/ENEURO.0061-21.2021
49. Wei F, Li W, Ma B, Deng X, Zhang L, Zhao L, et al. Experiences Affect Social Behaviors via Altering Neuronal Morphology and Oxytocin System. Psychoneuroendocrinology (2021) 129:105247. doi: 10.1016/j.psyneuen.2021.105247
50. Smith CJW, Dibenedictis BT, Veenema AH. Comparing Vasopressin and Oxytocin Fiber and Receptor Density Patterns in the Social Behavior Neural Network: Implications for Cross-System Signaling. Front Neuroendocrinol (2019) 53:100737. doi: 10.1016/j.yfrne.2019.02.001
51. Russell AL, Tasker JG, Lucion AB, Fiedler J, Munhoz CD, Wu TJ, et al. Factors Promoting Vulnerability to Dysregulated Stress Reactivity and Stress-Related Disease. J Neuroendocrinol (2018) 30:e12641. doi: 10.1111/jne.12641
52. Gu P, Lin Y, Wan Q, Su D, Shu Q. Oxytocin Signal Contributes to the Adaptative Growth of Islets During Gestation. Endocr Connect (2021) 10:694–706. doi: 10.1530/EC-21-0043
53. Wang P, Yang HP, Tian S, Wang L, Wang SC, Zhang F, et al. Oxytocin-Secreting System: A Major Part of the Neuroendocrine Center Regulating Immunologic Activity. J Neuroimmunol (2015) 289:152–61. doi: 10.1016/j.jneuroim.2015.11.001
54. Wang SC, Wang YF. Cardiovascular Protective Properties of Oxytocin Against COVID-19. Life Sci (2021) 270:119130. doi: 10.1016/j.lfs.2021.119130
55. Soares TJ, Coimbra TM, Martins AR, Pereira AG, Carnio EC, Branco LG, et al. Atrial Natriuretic Peptide and Oxytocin Induce Natriuresis by Release of cGMP. Proc Natl Acad Sci USA (1999) 96:278–83. doi: 10.1073/pnas.96.1.278
56. Greenwood MP, Mecawi AS, Hoe SZ, Mustafa MR, Johnson KR, Al-Mahmoud GA, et al. A Comparison of Physiological and Transcriptome Responses to Water Deprivation and Salt Loading in the Rat Supraoptic Nucleus. Am J Physiol Regul Integr Comp Physiol (2015) 308:R559–568. doi: 10.1152/ajpregu.00444.2014
57. Dutra SGV, Paterson A, Monteiro LRN, Greenwood MP, Greenwood MP, Amaral LS, et al. Physiological and Transcriptomic Changes in the Hypothalamic-Neurohypophysial System After 24 H of Furosemide-Induced Sodium Depletion. Neuroendocrinology (2021) 111:70–86. doi: 10.1159/000505997
58. Mecawi AS, Vilhena-Franco T, Araujo IG, Reis LC, Elias LL, Antunes-Rodrigues J. Estradiol Potentiates Hypothalamic Vasopressin and Oxytocin Neuron Activation and Hormonal Secretion Induced by Hypovolemic Shock. Am J Physiol Regul Integr Comp Physiol (2011) 301:R905–15. doi: 10.1152/ajpregu.00800.2010
59. Vilhena-Franco T, Mecawi AS, Elias LL, Antunes-Rodrigues J. Oestradiol Potentiates Hormone Secretion and Neuronal Activation in Response to Hypertonic Extracellular Volume Expansion in Ovariectomised Rats. J Neuroendocrinol (2011) 23:481–9. doi: 10.1111/j.1365-2826.2011.02133.x
60. Kunitake Y, Imamura Y, Mizoguchi Y, Matsushima J, Tateishi H, Murakawa-Hirachi T, et al. Serum Oxytocin Levels and Logical Memory in Older People in Rural Japan. J Geriatr Psychiatry Neurol (2021) 34:156–61. doi: 10.1177/0891988720915526
61. Oladosu FA, Tu FF, Garfield LB, Garrison EF, Steiner ND, Roth GE, et al. Low Serum Oxytocin Concentrations Are Associated With Painful Menstruation. Reprod Sci (2020) 27:668–74. doi: 10.1007/s43032-019-00071-y
62. Albert PR. Why is Depression More Prevalent in Women? J Psychiatry Neurosci (2015) 40:219–21. doi: 10.1503/jpn.150205
63. Uhl-Bronner S, Waltisperger E, Martinez-Lorenzana G, Condes Lara M, Freund-Mercier MJ. Sexually Dimorphic Expression of Oxytocin Binding Sites in Forebrain and Spinal Cord of the Rat. Neuroscience (2005) 135:147–54. doi: 10.1016/j.neuroscience.2005.05.025
64. Oliveira LLB, Del Bianco-Borges B, Franci CR. Estradiol and the Feeding State Modulate the Interaction Between Leptin and the Nitrergic System in Female Rats. Neuropeptides (2020) 84:102096. doi: 10.1016/j.npep.2020.102096
65. Breton JD, Veinante P, Uhl-Bronner S, Vergnano AM, Freund-Mercier MJ, Schlichter R, et al. Oxytocin-Induced Antinociception in the Spinal Cord is Mediated by a Subpopulation of Glutamatergic Neurons in Lamina I-II Which Amplify GABAergic Inhibition. Mol Pain (2008) 4:19. doi: 10.1186/1744-8069-4-19
66. Mooney SJ, Coen CW, Holmes MM, Beery AK. Region-Specific Associations Between Sex, Social Status, and Oxytocin Receptor Density in the Brains of Eusocial Rodents. Neuroscience (2015) 303:261–9. doi: 10.1016/j.neuroscience.2015.06.043
67. Horrell ND, Hickmott PW, Saltzman W. Neural Regulation of Paternal Behavior in Mammals: Sensory, Neuroendocrine, and Experiential Influences on the Paternal Brain. Curr Top Behav Neurosci (2019) 43:111–60. doi: 10.1007/7854_2018_55
68. Olazabal DE. Role of Oxytocin in Parental Behaviour. J Neuroendocrinol (2018) 30:e12594. doi: 10.1111/jne.12594
69. Lu Q, Lai J, Du Y, Huang T, Prukpitikul P, Xu Y, et al. Sexual Dimorphism of Oxytocin and Vasopressin in Social Cognition and Behavior. Psychol Res Behav Manag (2019) 12:337–49. doi: 10.2147/PRBM.S192951
70. Miller M, Bales KL, Taylor SL, Yoon J, Hostetler CM, Carter CS, et al. Oxytocin and Vasopressin in Children and Adolescents With Autism Spectrum Disorders: Sex Differences and Associations With Symptoms. Autism Res (2013) 6:91–102. doi: 10.1002/aur.1270
71. Holtfrerich SKC, Pfister R, El Gammal AT, Bellon E, Diekhof EK. Endogenous Testosterone and Exogenous Oxytocin Influence the Response to Baby Schema in the Female Brain. Sci Rep (2018) 8:7672. doi: 10.1038/s41598-018-26020-4
72. Rice MA, Hobbs LE, Wallace KJ, Ophir AG. Cryptic Sexual Dimorphism in Spatial Memory and Hippocampal Oxytocin Receptors in Prairie Voles (Microtus Ochrogaster). Horm Behav (2017) 95:94–102. doi: 10.1016/j.yhbeh.2017.08.003
73. Kreutzmann JC, Fendt M. Intranasal Oxytocin Compensates for Estrus Cycle-Specific Reduction of Conditioned Safety Memory in Rats: Implications for Psychiatric Disorders. Neurobiol Stress (2021) 14:100313. doi: 10.1016/j.ynstr.2021.100313
74. Guoynes CD, Simmons TC, Downing GM, Jacob S, Solomon M, Bales KL. Chronic Intranasal Oxytocin has Dose-Dependent Effects on Central Oxytocin and Vasopressin Systems in Prairie Voles (Microtus Ochrogaster). Neuroscience (2018) 369:292–302. doi: 10.1016/j.neuroscience.2017.11.037
75. Stone G, Choi A, Meritxell O, Gorham J, Heydarpour M, Seidman CE, et al. Sex Differences in Gene Expression in Response to Ischemia in the Human Left Ventricular Myocardium. Hum Mol Genet (2019) 28:1682–93. doi: 10.1093/hmg/ddz014
76. Breuil V, Trojani MC, Ez-Zoubir A. Oxytocin and Bone: Review and Perspectives. Int J Mol Sci (2021) 22:8551. doi: 10.3390/ijms22168551
77. Hansson AC, Spanagel R. No Changes in the Oxytocin System in Alcohol-Dependent Female Rodents and Humans: Towards a Sex-Specific Psychopharmacology in Alcoholism. Addict Biol (2021) 26:e12945. doi: 10.1111/adb.12945
78. Dekker A, Wenzlaff F, Biedermann SV, Briken P, Fuss J. VR Porn as "Empathy Machine"? Perception of Self and Others in Virtual Reality Pornography. J Sex Res (2021) 58:273–8. doi: 10.1080/00224499.2020.1856316
79. Melis MR, Melis T, Cocco C, Succu S, Sanna F, Pillolla G, et al. Oxytocin Injected Into the Ventral Tegmental Area Induces Penile Erection and Increases Extracellular Dopamine in the Nucleus Accumbens and Paraventricular Nucleus of the Hypothalamus of Male Rats. Eur J Neurosci (2007) 26:1026–35. doi: 10.1111/j.1460-9568.2007.05721.x
80. Peris J, Macfadyen K, Smith JA, De Kloet AD, Wang L, Krause EG. Oxytocin Receptors are Expressed on Dopamine and Glutamate Neurons in the Mouse Ventral Tegmental Area That Project to Nucleus Accumbens and Other Mesolimbic Targets. J Comp Neurol (2017) 525:1094–108. doi: 10.1002/cne.24116
81. Magon N, Kalra S. The Orgasmic History of Oxytocin: Love, Lust, and Labor. Indian J Endocrinol Metab (2011) 15 (Suppl 3):S156–61. doi: 10.4103/2230-8210.84851
82. Alwaal A, Breyer BN, Lue TF. Normal Male Sexual Function: Emphasis on Orgasm and Ejaculation. Fertil Steril (2015) 104:1051–60. doi: 10.1016/j.fertnstert.2015.08.033
83. Caldwell HK, Aulino EA, Freeman AR, Miller TV, Witchey SK. Oxytocin and Behavior: Lessons From Knockout Mice. Dev Neurobiol (2017) 77:190–201. doi: 10.1002/dneu.22431
84. Lind CM, Birky NK, Porth AM, Farrell TM. Vasotocin Receptor Blockade Disrupts Maternal Care of Offspring in a Viviparous Snake, Sistrurus Miliarius. Biol Open (2017) 6:283–9. doi: 10.1242/bio.022616
85. Guoynes CD, Marler CA. An Acute Dose of Intranasal Oxytocin Rapidly Increases Maternal Communication and Maintains Maternal Care in Primiparous Postpartum California Mice. PLoS One (2021) 16:e0244033. doi: 10.1371/journal.pone.0244033
86. Munetomo A, Ishii H, Miyamoto T, Sakuma Y, Kondo Y. Puerperal and Parental Experiences Alter Rat Preferences for Pup Odors via Changes in the Oxytocin System. J Reprod Dev (2016) 62:17–27. doi: 10.1262/jrd.2015-046
87. Scatliffe N, Casavant S, Vittner D, Cong X. Oxytocin and Early Parent-Infant Interactions: A Systematic Review. Int J Nurs Sci (2019) 6:445–53. doi: 10.1016/j.ijnss.2019.09.009
88. Sue Carter C. Oxytocin and Human Evolution. Curr Top Behav Neurosci (2018) 35:291–319. doi: 10.1007/7854_2017_18
89. Kroll-Desrosiers AR, Nephew BC, Babb JA, Guilarte-Walker Y, Moore Simas TA, Deligiannidis KM. Association of Peripartum Synthetic Oxytocin Administration and Depressive and Anxiety Disorders Within the First Postpartum Year. Depress Anxiety (2017) 34:137–46. doi: 10.1002/da.22599
90. Wang YF, Ponzio TA, Hatton GI. Autofeedback Effects of Progressively Rising Oxytocin Concentrations on Supraoptic Oxytocin Neuronal Activity in Slices From Lactating Rats. Am J Physiol Regul Integr Comp Physiol (2006) 290:R1191–1198. doi: 10.1152/ajpregu.00725.2005
91. Davies C, Paloyelis Y, Rutigliano G, Cappucciati M, De Micheli A, Ramella-Cravaro V, et al. Oxytocin Modulates Hippocampal Perfusion in People at Clinical High Risk for Psychosis. Neuropsychopharmacology (2019) 44:1300–9. doi: 10.1038/s41386-018-0311-6
92. Kerem L, Lawson EA. Oxytocin, Eating Behavior, and Metabolism in Humans. Handb Clin Neurol (2021) 180:89–103. doi: 10.1016/B978-0-12-820107-7.00006-9
93. Li D, San M, Zhang J, Yang A, Xie W, Chen Y, et al. Oxytocin Receptor Induces Mammary Tumorigenesis Through Prolactin/P-STAT5 Pathway. Cell Death Dis (2021) 12:588. doi: 10.1038/s41419-021-03849-8
94. Goh KK, Chen CH, Lane HY. Oxytocin in Schizophrenia: Pathophysiology and Implications for Future Treatment. Int J Mol Sci (2021) 22:2146. doi: 10.3390/ijms22042146
95. Li D, Li T, Yu J, Liu X, Jia S, Wang X, et al. Astrocytic Modulation of Supraoptic Oxytocin Neuronal Activity in Rat Dams With Pup-Deprivation at Different Stages of Lactation. Neurochem Res (2021) 46:2601–11. doi: 10.1007/s11064-020-03129-5
96. Chander RJ, Mather KA, Cleary R, Grainger SA, Thalamuthu A, Numbers K, et al. The Influence of Rs53576 Polymorphism in the Oxytocin Receptor (OXTR) Gene on Empathy in Healthy Adults by Subtype and Ethnicity: A Systematic Review and Meta-Analysis. Rev Neurosci (2021) 33:43–57. doi: 10.1515/revneuro-2021-0038
97. Busnelli M, Chini B. Molecular Basis of Oxytocin Receptor Signalling in the Brain: What We Know and What We Need to Know. Curr Top Behav Neurosci (2018) 35:3–29. doi: 10.1007/7854_2017_6
98. Thaler L, Brassard S, Booij L, Kahan E, Mcgregor K, Labbe A, et al. Methylation of the OXTR Gene in Women With Anorexia Nervosa: Relationship to Social Behavior. Eur Eat Disord Rev (2020) 28:79–86. doi: 10.1002/erv.2703
99. Zhang J, Yang C, Leng J, Liu J, Gong P, Esposito G. OXTR Moderates Adverse Childhood Experiences on Depressive Symptoms Among Incarcerated Males. J Psychiatr Res (2021) 140:221–7. doi: 10.1016/j.jpsychires.2021.05.043
100. Siu MT, Goodman SJ, Yellan I, Butcher DT, Jangjoo M, Grafodatskaya D, et al. DNA Methylation of the Oxytocin Receptor Across Neurodevelopmental Disorders. J Autism Dev Disord (2021) 51:3610–23. doi: 10.1007/s10803-020-04792-x
101. Selvage DJ, Johnston CA. Interaction Between Norepinephrine, Oxytocin, and Nitric Oxide in the Stimulation of Gonadotropin-Releasing Hormone Release From Proestrous Rat Basal Hypothalamus Explants. J Neuroendocrinol (2004) 16:819–24. doi: 10.1111/j.1365-2826.2004.01235.x
102. Vanakara P, Dafopoulos K, Papastergiopoulou L, Kallitsaris A, Papageorgiou I, Messinis IE. Oxytocin is Not Important for the Control of Gonadotrophin Secretion in the Late Follicular Phase of the Cycle. Clin Endocrinol (Oxf) (2007) 66:816–21. doi: 10.1111/j.1365-2265.2007.02818.x
103. Evans JJ, Reid RA, Wakeman SA, Croft LB, Benny PS. Evidence That Oxytocin is a Physiological Component of LH Regulation in Non-Pregnant Women. Hum Reprod (2003) 18:1428–31. doi: 10.1093/humrep/deg291
104. Liu XY, Hou D, Wang J, Lv C, Jia S, Zhang Y, et al. Expression of Glial Fibrillary Acidic Protein in Astrocytes of Rat Supraoptic Nucleus Throughout Estrous Cycle. Neuro Endocrinol Lett (2016) 37:41–5.
105. Wang SC, Parpura V, Wang YF. Astroglial Regulation of Magnocellular Neuroendocrine Cell Activities in the Supraoptic Nucleus. Neurochem Res (2021) 46:2586–600. doi: 10.1007/s11064-020-03172-2
106. Murtaza S, Sattar A, Ahmad N, Jamil Ahmad M, Akhtar S, Ahmad E, et al. Effect of Exogenous Administration of Oxytocin on Postpartum Follicular Dynamics, Oestrous Rate and Ovulation in Nili-Ravi Buffaloes. Reprod Domest Anim (2021) 56:1369–76. doi: 10.1111/rda.14001
107. Mccracken JA, Custer EE, Eldering JA, Robinson AG. The Central Oxytocin Pulse Generator: A Pacemaker for the Ovarian Cycle. Acta Neurobiol Exp (Wars) (1996) 56:819–32.
108. Brunton PJ, Bales J, Russell JA. Allopregnanolone and Induction of Endogenous Opioid Inhibition of Oxytocin Responses to Immune Stress in Pregnant Rats. J Neuroendocrinol (2012) 24:690–700. doi: 10.1111/j.1365-2826.2012.02295.x
109. Crowley SK, Pedersen CA, Leserman J, Girdler SS. The Influence of Early Life Sexual Abuse on Oxytocin Concentrations and Premenstrual Symptomatology in Women With a Menstrually Related Mood Disorder. Biol Psychol (2015) 109:1–9. doi: 10.1016/j.biopsycho.2015.04.003
110. Scott N, Prigge M, Yizhar O, Kimchi T. A Sexually Dimorphic Hypothalamic Circuit Controls Maternal Care and Oxytocin Secretion. Nature (2015) 525:519–22. doi: 10.1038/nature15378
111. Flynn MJ, Campbell TS, Robert M, Nasr-Esfahani M, Rash JA. Intranasal Oxytocin as a Treatment for Chronic Pelvic Pain: A Randomized Controlled Feasibility Study. Int J Gynaecol Obstet (2021) 152:425–32. doi: 10.1002/ijgo.13441
112. Russell J, Maguire S, Hunt GE, Kesby A, Suraev A, Stuart J, et al. Intranasal Oxytocin in the Treatment of Anorexia Nervosa: Randomized Controlled Trial During Re-Feeding. Psychoneuroendocrinology (2018) 87:83–92. doi: 10.1016/j.psyneuen.2017.10.014
113. Lipschitz DL, Crowley WR, Bealer SL. Central Blockade of Oxytocin Receptors During Late Gestation Disrupts Systemic Release of Oxytocin During Suckling in Rats. J Neuroendocrinol (2003) 15:743–8. doi: 10.1046/j.1365-2826.2003.01052.x
114. Higuchi T, Okere CO. Role of the Supraoptic Nucleus in Regulation of Parturition and Milk Ejection Revisited. Microsc Res Tech (2002) 56:113–21. doi: 10.1002/jemt.10016
115. Selin L, Berg M, Wennerholm UB, Dencker A. Dosage of Oxytocin for Augmentation of Labor and Women's Childbirth Experiences: A Randomized Controlled Trial. Acta Obstet Gynecol Scand (2021) 100:971–8. doi: 10.1111/aogs.14042
116. Ibrahim ZM, Sayed Ahmed WA, Abd El-Hamid EM, Taha OT, Elbahie AM. Carbetocin Versus Oxytocin for Prevention of Postpartum Hemorrhage in Hypertensive Women Undergoing Elective Cesarean Section. Hypertens Pregnancy (2020) 39:319–25. doi: 10.1080/10641955.2020.1768268
117. Hirst JJ, Haluska GJ, Cook MJ, Hess DL, Novy MJ. Comparison of Plasma Oxytocin and Catecholamine Concentrations With Uterine Activity in Pregnant Rhesus Monkeys. J Clin Endocrinol Metab (1991) 73:804–10. doi: 10.1210/jcem-73-4-804
118. Hirst JJ, Haluska GJ, Cook MJ, Novy MJ. Plasma Oxytocin and Nocturnal Uterine Activity: Maternal But Not Fetal Concentrations Increase Progressively During Late Pregnancy and Delivery in Rhesus Monkeys. Am J Obstet Gynecol (1993) 169:415–22. doi: 10.1016/0002-9378(93)90099-5
119. Winslow JT, Insel TR. The Social Deficits of the Oxytocin Knockout Mouse. Neuropeptides (2002) 36:221–9. doi: 10.1054/npep.2002.0909
120. Macbeth AH, Stepp JE, Lee HJ, Young WS. 3rd, and Caldwell, H.KNormal Maternal Behavior, But Increased Pup Mortality, in Conditional Oxytocin Receptor Knockout Females. Behav Neurosci (2010) 124:677–85. doi: 10.1037/a0020799
121. Grattan DR, Ladyman SR. Neurophysiological and Cognitive Changes in Pregnancy. Handb Clin Neurol (2020) 171:25–55. doi: 10.1016/B978-0-444-64239-4.00002-3
122. Uvnas Moberg K, Ekstrom-Bergstrom A, Buckley S, Massarotti C, Pajalic Z, Luegmair K, et al. Maternal Plasma Levels of Oxytocin During Breastfeeding-A Systematic Review. PloS One (2020) 15:e0235806. doi: 10.1371/journal.pone.0235806
123. Wang YF, Negoro H, Honda K. Effects of Hemitransection of the Midbrain on Milk-Ejection Burst of Oxytocin Neurones in Lactating Rat. J Endocrinol (1995) 144:463–70. doi: 10.1677/joe.0.1440463
124. Wang YF, Negoro H, Honda K. Milk Ejection Bursts of Supraoptic Oxytocin Neurones During Bilateral and Unilateral Suckling in the Rat. J Neuroendocrinol (1996) 8:427–31. doi: 10.1046/j.1365-2826.1996.04703.x
125. Wang YF, Negoro H, Honda K. Effect of Interhemispheric Sections of the Hypothalamus on Milk-Ejection Bursts of Supraoptic Oxytocin Neurones During Bilateral and Unilateral Suckling in the Rat. Neurosci Lett (1997) 227:17–20. doi: 10.1016/S0304-3940(97)00300-5
126. Wang YF, Negoro H, Higuchi T. Lesions of Hypothalamic Mammillary Body Desynchronise Milk-Ejection Bursts of Rat Bilateral Supraoptic Oxytocin Neurones. J Neuroendocrinol (2013) 25:67–75. doi: 10.1111/j.1365-2826.2012.02368.x
127. Li D, Liu X, Li T, Wang X, Jia S, Wang P, et al. Involvement of Protein Kinase A in Oxytocin Neuronal Activity in Rat Dams With Pup Deprivation. Neurochem Res (2021) 46:980–91. doi: 10.1007/s11064-020-03218-5
128. Tasker JG, Prager-Khoutorsky M, Teruyama R, Lemos JR, Amstrong WE. Advances in the Neurophysiology of Magnocellular Neuroendocrine Cells. J Neuroendocrinol (2020) 32:e12826. doi: 10.1111/jne.12826
129. Liu X, Jia S, Zhang Y, Wang Y-F. Pulsatile But Not Tonic Secretion of Oxytocin Plays the Role of Anti-Precancerous Lesions of the Mammary Glands in Rat Dams Separated From the Pups During Lactation. Mathews J Neurol (2016) 1:002.
130. Khori V, Alizadeh AM, Khalighfard S, Heidarian Y, Khodayari H. Oxytocin Effects on the Inhibition of the NF-Kappab/Mir195 Pathway in Mice Breast Cancer. Peptides (2018) 107:54–60. doi: 10.1016/j.peptides.2018.07.007
131. Maestrini S, Mele C, Mai S, Vietti R, Di Blasio A, Castello L, et al. Plasma Oxytocin Concentration in Pre- and Postmenopausal Women: Its Relationship With Obesity, Body Composition and Metabolic Variables. Obes Facts (2018) 11:429–39. doi: 10.1159/000492001
132. Jonasson AF, Edwall L, Uvnas-Moberg K. Topical Oxytocin Reverses Vaginal Atrophy in Postmenopausal Women: A Double-Blind Randomized Pilot Study. Menopause Int (2011) 17:120–5. doi: 10.1258/mi.2011.011030
133. Zohrabi I, Abedi P, Ansari S, Maraghi E, Shakiba Maram N, Houshmand G. The Effect of Oxytocin Vaginal Gel on Vaginal Atrophy in Postmenopausal Women: A Randomized Controlled Trial. BMC Womens Health (2020) 20:108. doi: 10.1186/s12905-020-00935-5
134. Elabd C, Basillais A, Beaupied H, Breuil V, Wagner N, Scheideler M, et al. Oxytocin Controls Differentiation of Human Mesenchymal Stem Cells and Reverses Osteoporosis. Stem Cells (2008) 26:2399–407. doi: 10.1634/stemcells.2008-0127
135. Beranger GE, Pisani DF, Castel J, Djedaini M, Battaglia S, Amiaud J, et al. Oxytocin Reverses Ovariectomy-Induced Osteopenia and Body Fat Gain. Endocrinology (2014) 155:1340–52. doi: 10.1210/en.2013-1688
136. Kujath AS, Quinn L, Elliott ME, Varady KA, Lecaire TJ, Carter CS, et al. Oxytocin Levels are Lower in Premenopausal Women With Type 1 Diabetes Mellitus Compared With Matched Controls. Diabetes Metab Res Rev (2015) 31:102–12. doi: 10.1002/dmrr.2577
137. Erdenebayar O, Kato T, Kawakita T, Kasai K, Kadota Y, Yoshida K, et al. Effects of Peripheral Oxytocin Administration on Body Weight, Food Intake, Adipocytes, and Biochemical Parameters in Peri- and Postmenopausal Female Rats. Endocr J (2021) 68:7–16. doi: 10.1507/endocrj.EJ19-0586
138. De Melo VU, Saldanha RR, Dos Santos CR, De Campos Cruz J, Lira VA, Santana-Filho VJ, et al. Ovarian Hormone Deprivation Reduces Oxytocin Expression in Paraventricular Nucleus Preautonomic Neurons and Correlates With Baroreflex Impairment in Rats. Front Physiol (2016) 7:461. doi: 10.3389/fphys.2016.00461
Keywords: lactation, menstruation, parturition, pregnancy, menopause
Citation: Liu N, Yang H, Han L and Ma M (2022) Oxytocin in Women’s Health and Disease. Front. Endocrinol. 13:786271. doi: 10.3389/fendo.2022.786271
Received: 01 October 2021; Accepted: 19 January 2022;
Published: 15 February 2022.
Edited by:
Ben Nephew, Worcester Polytechnic Institute, United StatesReviewed by:
Andre Souza Mecawi, Federal University of São Paulo, BrazilDavid Olson, University of Alberta, Canada
Copyright © 2022 Liu, Yang, Han and Ma. This is an open-access article distributed under the terms of the Creative Commons Attribution License (CC BY). The use, distribution or reproduction in other forums is permitted, provided the original author(s) and the copyright owner(s) are credited and that the original publication in this journal is cited, in accordance with accepted academic practice. No use, distribution or reproduction is permitted which does not comply with these terms.
*Correspondence: Mingxing Ma, bXhtYUBjbXUuZWR1LmNu