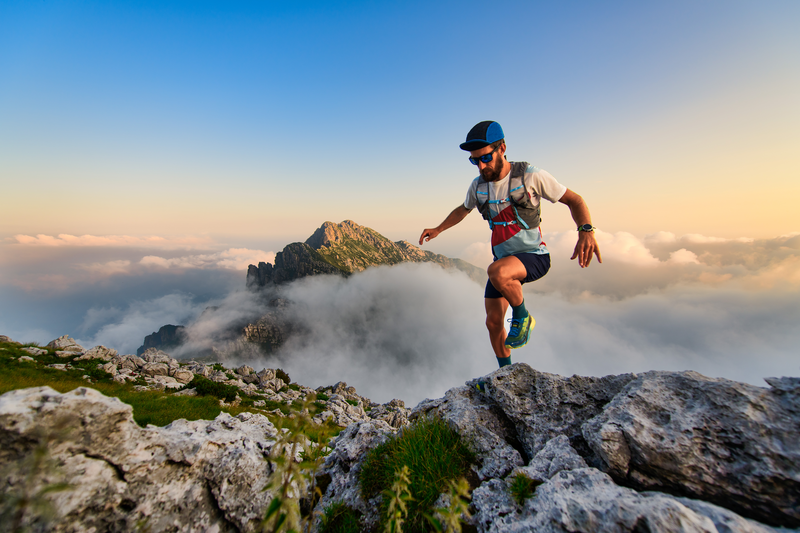
95% of researchers rate our articles as excellent or good
Learn more about the work of our research integrity team to safeguard the quality of each article we publish.
Find out more
ORIGINAL RESEARCH article
Front. Endocrinol. , 15 December 2022
Sec. Bone Research
Volume 13 - 2022 | https://doi.org/10.3389/fendo.2022.1087110
This article is part of the Research Topic Imaging of Adipose Tissue in Bone and Muscle: Implications for Osteoporosis, Sarcopenia and Frailty View all 11 articles
Background and purpose: Weight loss and muscle mass loss are common in patients with chronic obstructive pulmonary disease (COPD). Muscle density and fat infiltration based on CT images may be more sensitive than muscle mass by DXA in the assessment of sarcopenia for COPD patients. However, the age-related changes of cross-sectional trunk muscle compositions based on lung CT scans are still unknown. Thus, we aimed to investigate over time the change in muscle density, size, and fat deposition of L1-level trunk muscles in patients with COPD.
Materials and methods: 129 male COPD patients with a second chest CT scan (from 2013-2019 to 2014-2020) were enrolled. The CT images at first and second CT scans are analyzed by OsiriX software. Trunk muscles at the level of the 1st lumbar vertebrae were selected for analysis. Attenuation of lumbar vertebrae 1 was also measured from chest CT images. The pulmonary function values were calculated based on forced expiratory volume in 1 second (FEV1) and forced vital capacity (FVC).
Results: The mean age of the 129 patients with COPD was 69.7 years. The durations of COPD of this cohort were from 8-17 years. The mean area and density of L1 trunk muscles were 85.5 cm2 and 36.4 HU. At baseline, muscle area and density and vertebral density were negatively associated with age (p<0.0001), while the intermuscular fat area and the fat infiltration ratio were not significantly associated with age (p>0.05). The per-year loss of trunk muscle area was 2.83 cm2 (p<0.0001) which accounts for 3.3% decrease per year, and the per-year decrease of trunk muscle density was 2.41 HU (p<0.0001) which accounts for 6.6% decrease per year. The per-year increase of intermuscular fat in trunk muscles was 0.57 cm2 (p=0.006) which accounts for 11.1% increase per year. The bone density loss was 5.63 HU/per year (p<0.0001).
Conclusion: Men with COPD had accelerated muscle loss as well as increased fat infiltration. Compared to muscle quantity loss, the decline in muscle quality is much larger, indicating the importance of relevant interventions focusing on improving muscle quality.
Chronic obstructive pulmonary disease (COPD) is the fourth leading cause of death worldwide and is often characterized by chronic inflammation and extrapulmonary changes that impair quality of life and physical activity (1). Thus, weight loss and muscle mass loss are common in patients with COPD (2), and the risk of developing sarcopenia in COPD patients is increasing, with a prevalence ranging from 15% to 55% (3). Furthermore, sarcopenia appears to negatively affect clinical outcomes related to function and health in patients with COPD (4). Therefore, early detection of sarcopenia might be critical to better designing therapeutic interventions like pulmonary rehabilitation.
Skeletal muscle mass is usually measured by dual energy X-ray absorptiometry (DXA) and bioelectrical impedance analysis (BIA). Unlike DXA/BIA, which only measures muscle quantity, CT measures both muscle quantity (e.g., muscle cross-sectional area [CSA] or muscle index) and muscle quality (e.g, muscle attenuation or fat infiltration) (5). CT-based muscle metrics show promise in predicting the risk of osteoporotic fracture (6–8) and hip refracture (9), as well as the mortality (10–12). Muscle density and fat infiltration may be more sensitive than muscle mass by DXA in the assessment of sarcopenia for COPD patients.
In clinical practice, routine lung CT is often performed in COPD patients to help characterize COPD phenotypes and screen for lung cancer (13). Therefore, compared with DXA, the use of lung CT might offer the opportunity to routinely assess the sarcopenia in COPD patients. However, the age-related changes of cross-sectional trunk muscle compositions based on lung CT scans are still unknown, which impedes the understanding of the relationships between muscle metrics and COPD.
In this retrospective follow-up cohort study, by using state-of-the-art imaging, we aimed to investigate over time the change in muscle density, size, and fat deposition of L1-level trunk muscles in patients with COPD. We also aimed to explore the relations among muscle measurements, duration of COPD, and pulmonary function.
The retrospective study was approved by the Institutional Review Board of Xiangya Hospital, and the informed consent from the patients was waived. We searched both the medical record and the PACS for all patients who met the following inclusion criteria: a male aged 50 years or older with a diagnosis of COPD according to the Global Initiative for Chronic Obstructive Lung Disease (GOLD) criteria (14) between Jan 1, 2013, and Dec 31, 2019; two diagnostic chest CT scans with an interval time of at least 3 months. This yielded 129 male patients with a second chest CT scan (from 2013-2019 to 2014-2020).
Patients with other conditions that cause sarcopenia including chronic liver disease, end stage renal disease, and active cancer were excluded. Bronchial asthma, interstitial pneumonia, or bronchiectasis, thoracic or upper lumbar vertebrae degenerative disease or history of operation on the vertebrae, without a second chest CT scan were also excluded.
CT imaging was performed with two CT scanners (Aquilion one 320, Toshiba, Tokyo, Japan; SOMATOM Definition, Siemens, Erlangen, Germany). All patients were scanned in the supine position during deep inspiration without intravenous contrast. The two scans of most COPD patients were performed on a same scanner to avoid the inter-scanner differences. Scan parameters were 120kVp, auto current setting based on BMI, 1mm slice thickness, 50 cm field of view, and 512×512 matrix in spiral and standard reconstructions.
The DICOM images of COPD patients at first and second CT scans are analyzed by OsiriX software (Lite version 10.0.2, Pixmeo, Geneva, Switzerland) which was downloaded from http://www.osirix-viewer.com/, and was previously assessed as a user-friendly image analysis software package for the Apple Mac OS. All muscle measurements were acquired by one of the investigators who, in preparation for the measurements, received training in chest CT imaging assessments focusing on trunk muscle morphology. For practice purposes, a sample of about 20 images was analyzed with the OsiriX software application prior to the beginning of the measurement study. Trunk muscles at the level of the 1st lumbar vertebrae were selected for analysis. Using the ‘pencil’ tool to outline the muscles as ROIs. Then open the ‘growing’ window, -29 to 150 HU as the threshold to segment muscle tissue from fat (Figures 1A, B). The area and mean CT values were displayed in the Segmentation preview box. The pre-segmentation ROI was defined as “Muscle Fat”, and the area of pre-segmentation ROI minus the area of segmented muscle was the fat area (intermuscular fat).
Figure 1 Muscle measurements at two screening chest CTs in 66-year-old man with COPD. (A) refers to L1 level CT image of 1st CT scan with the measurement of trunk muscle density (31.9 HU) and size (140.7 cm2). (B) refers to L1 level CT image of 2nd CT scan performed 3 years after the initial one with the measurement of trunk muscle density (26.1 HU) and size (114.8 cm2).
Attenuation of lumbar vertebrae 1 (L1) was also measured by the same investigator with the OsiriX software application from chest CT images. A circular region of interest (ROI) was drawn at the mid-vertebral body, avoiding the cortical bone and the posterior internal vertebral venous plexus.
The pulmonary function values were calculated based on forced expiratory volume in 1 second (FEV1) and forced vital capacity (FVC) by using standardized equipment (MasterScreen, JAEGER/Carefusion, Hoechberg, Germany).
Demographic and anthropometric covariates included age, height, weight, duration of COPD. Health-related covariates included hemoglobin, mean hemoglobin concentration, mean corpuscular hemoglobin, white blood cell count, red blood cell count, platelet distribution width, mean platelet volume.
Baseline characteristics were described with mean ± standard deviation for continuous variables and frequency and percent for categorical variables. Generalized linear models were used to study the association of muscle indexes with age, duration of disease, and pulmonary function. Moreover, we assessed the impact of COPD duration, age, and pulmonary function on muscle indexes by categorizing participants with COPD using 10 years, age using 70 years, and FEV1% using 40 as the cut-off value. Comparisons were made by two-sample t-test or Wilcoxon Signed Rank tests for continuous variables.
A two-sided P value less than 0.05 was considered statistically significant. All analyses were performed using SAS 9.4.
The demographic data and baseline measurements were shown in Table 1. The mean age of the 129 patients with COPD was 69.7 years. The durations of COPD of this cohort were from 8-17 years. The mean area and density of L1 trunk muscles were 85.5 cm2 and 36.4 HU, and the mean bone attenuation of L1 was 113.8 HU. This COPD cohort had a mean FEV1 of 40.3%. The blood routine examinations of this cohort were normal.
At baseline, Figure 2A shows that muscle area and density and vertebral density were negatively associated with age (p<0.0001), while the intermuscular fat area and the fat infiltration ratio were not significantly associated with age (p>0.05). The duration of COPD was only negatively associated with muscle area (p=0.0011), but was not associated with muscle and vertebral density (Figure 2B). Figure 2C shows that FEV1, % was not significantly associated with any measurement.
Figure 2 Correlation between baseline muscle and bone indexes with age (A), duration of disease (B), and pulmonary function (C).
The per-year changes in muscle and bone indexes were not significantly associated with age, duration of COPD and FEV1, % (Figures 3A–C).
Figure 3 Correlation between per-year change in muscle and bone indexes with age (A), duration of disease (B), and pulmonary function (C).
Table 2 shows the absolute changes and per year changes in muscle and bone indexes between the two CT scans for the male COPD patients. The per-year loss of trunk muscle area was 2.83 cm2 (p<0.0001) which accounts for 3.3% decrease per year, and the per-year decrease of trunk muscle density was 2.41 HU (p<0.0001) which accounts for 6.6% decrease per year. The per-year increase of intermuscular fat area in trunk muscles was 0.57 cm2 (p=0.006) which accounts for 11.1% increase per year. For male COPD patients, the bone density loss was 5.63 HU/per year (p<0.0001). For the subgroup analysis, the group aged under 70 years had a higher intermuscular fat area (0.98 cm2) increase per year compared to that of the group aged over 70 years (0.09 cm2). However, the difference of muscle fat infiltration per year between two age groups was border significant (p=0.04). The COPD duration and the pulmonary function had no effect on the per-year change of all muscle indexes (Table 3).
To the best of our knowledge, our findings first demonstrated the rate of decline in muscle size and density in older COPD patients, and we discovered that older male COPD patients had accelerated loss of muscle size and density, as well as increased intermuscular fat deposits. Our results also indicate that compared to muscle quantity decreased, the rate of muscle quality loss is doubled, implying that the interventions for sarcopenia of COPD patients should be focused on muscle quality.
In the last decade, there has been a rapid increase in the use of CT measured muscle CSA and density in the evaluation of sarcopenia, especially for opportunistic applications. A recent review showed that the total abdominal wall muscles are mostly favored for muscle CSA and density measurements, and measuring trunk muscle in an axial slice is the best standard for CT-based calculation of total body muscle mass (15). Further, a recent opportunistic use of CT study demonstrated that the L1 trunk muscle measurement allows sarcopenia assessment using both chest and abdominal CT scans (11), which indicates our outcomes could generate the potential yield of opportunistic CT screening of sarcopenia for COPD patients.
The decline rate of muscle mass in older adults was reported as approximately 0.51%, which is much lower than that of muscle strength, which was 2.5 to 4% in a year (16). The cross-sectional results showed that muscle mass decreased less than 0.5% per year in both genders when compared the young adults (18 to 45 years old) and the elderly (65 years old or over) (16). However, our results indicated that the muscle size loss in older male patients with COPD was 3.3% in a year, which is much higher than older adults without COPD.
Inflammation is the main feature of chronic obstructive pulmonary disease. Usually, the inflammatory response of COPD patients is not limited to the lungs but is also accompanied by systemic chronic inflammation (1). Systemic chronic inflammatory responses can lead to sarcopenia in COPD patients (1). On the other hand, sarcopenia was found to be associated with increased levels of systemic inflammation in COPD patients (17). In addition to the inflammation effect, physical inactivity caused by COPD is also a critical trigger for muscle loss as inactivity results in disuse-atrophy (3). Thus, in our study, the muscle loss of COPD patients was much larger than the numbers in the reports with normal elderly men without COPD.
The observations of larger declines of muscles, especially the muscle density, are meaningful because previous reports have demonstrated reduced attenuation in the range of 3–6 HU among people with strength deconditioning and low back pain (18, 19). Furthermore, the 2.41 HU decrease in COPD men per year is parallel to a 15% decrease over four decades in a previous report (20). Notably, the mean value (36.9HU) of muscle density in our study was adjacent to the myosteatosis diagnostic cut points (from ≤22.0 HU to ≤44.4 HU) for muscle attenuation in cancer patients (12). L1 trunk muscle density may be a potential risk factor for mortality in patients with COPD as other reports (14, 21).
Insight of the muscle size and density decreases and increased fat infiltration observed among male COPD patients in this study is also important to recognize the potential for intervention strategies that could be implemented to mitigate these detriments to muscle health. A study ever showed that muscle quality is more impaired in COPD patients with sarcopenia (22). Our results might indicate the importance of a rapid increase in muscle quality favoring a combination of resistance and aerobic exercises as commonly applied in pulmonary rehabilitation. Exercise training programs are of high importance, and it is a limitation of our study that we did not assess routine physical activity and exercises in this cohort.
Our study has several limitations. First, not all participants were stable COPD patients. Some of them came to our hospital for the second CT scan because of the aggravating COPD for in-hospital treatments. Second, we did not perform a detailed assessment of physical function, which may have added to a better understanding of the causes of accelerated loss of muscle in COPD patients. Third, we did not collect information about smoking history, which is known to affect muscle loss (23). Forth, the inflammation related information is not available in this study. One cause of the COPD related muscle loss is the inflammation status of the COPD patients.
In conclusion, COPD men had accelerated muscle loss and increased muscle fat infiltration. Compared to muscle size loss, the decline in muscle density was much larger, indicating the importance of relevant interventions focusing on improving muscle quality.
The raw data supporting the conclusions of this article will be made available by the authors, without undue reservation.
The studies involving human participants were reviewed and approved by Medical Ethics Committee of Xiangya Hospital of Central South University. Written informed consent for participation was not required for this study in accordance with the national legislation and the institutional requirements.
All authors contributed to this study. YW: Designing the study and preparing the initial draft. ZZ: Data collect and analysis. SL: Data analysis and interpretation. JF and JC: Data acquisition, data analysis. YP: Critically revising the final manuscript. XP: Designing the study, conceptualization and critical revision for intellectual content. All authors contributed to the article and approved the submitted version.
The authors declare that the research was conducted in the absence of any commercial or financial relationships that could be construed as a potential conflict of interest.
All claims expressed in this article are solely those of the authors and do not necessarily represent those of their affiliated organizations, or those of the publisher, the editors and the reviewers. Any product that may be evaluated in this article, or claim that may be made by its manufacturer, is not guaranteed or endorsed by the publisher.
1. Sepulveda-Loyola W, Osadnik C, Phu S, Morita AA, Duque G, Probst VS. Diagnosis, prevalence, and clinical impact of sarcopenia in COPD: a systematic review and meta-analysis. J cachexia sarcopenia muscle (2020) 11(5):1164–76. doi: 10.1002/jcsm.12600
2. Kwan HY, Maddocks M, Nolan CM, Jones SE, Patel S, Barker RE, et al. The prognostic significance of weight loss in chronic obstructive pulmonary disease-related cachexia: a prospective cohort study. J cachexia sarcopenia muscle (2019) 10(6):1330–8. doi: 10.1002/jcsm.12463
3. van Bakel SIJ, Gosker HR, Langen RC, Schols A. Towards personalized management of sarcopenia in COPD. Int J Chron Obstruct Pulmon Dis (2021) 16:25–40. doi: 10.2147/COPD.S280540
4. Benz E, Trajanoska K, Lahousse L, Schoufour JD, Terzikhan N, De Roos Eetal/> Sarcopenia in COPD: a systematic review and meta-analysis. Eur Respir Rev (2019) 28(154):190049. doi: 10.1183/16000617.0049-201910.1183/16000617.0049-2019
5. Wang L, Yin L, Zhao Y, Su Y, Sun W, Chen S, et al. Muscle density, but not size, correlates well with muscle strength and physical performance. J Am Med Directors Assoc (2021) 22(4):751–9 e2. doi: 10.1016/j.jamda.2020.06.052
6. Wang L, Yin L, Zhao Y, Su Y, Sun W, Liu Y, et al. Muscle density discriminates hip fracture better than computed tomography X-ray absorptiometry hip areal bone mineral density. J cachexia sarcopenia Muscle (2020) 11(6):1799–812. doi: 10.1002/jcsm.12616
7. Wang L, Li S, Liu Y, Li K, Yin L, Su Y, et al. Greater bone marrow fat and myosteatosis are associated with lower vBMD but not asymptomatic vertebral fracture. Eur Radiol (2022). doi: 10.1007/s00330-022-08979-x
8. Lang T, Cauley JA, Tylavsky F, Bauer D, Cummings S, Harris TB. Computed tomographic measurements of thigh muscle cross-sectional area and attenuation coefficient predict hip fracture: The health, aging, and body composition study. J Bone Mineral Res (2010) 25(3):513–9. doi: 10.1359/jbmr.090807
9. Wang L, Yin L, Yang M, Ge Y, Liu Y, Su Y, et al. Muscle density is an independent risk factor of second hip fracture: a prospective cohort study. J cachexia sarcopenia muscle (2022) 13(3):1927–37. doi: 10.1002/jcsm.12996
10. Wang L, Yin L, Yang M, Cheng X. Muscle composition and the imminent mortality risk after hip fracture. J cachexia sarcopenia Muscle (2022). doi: 10.1002/jcsm.13090
11. Lenchik L, Lenoir KM, Tan J, Boutin RD, Callahan KE, Kritchevsky SB, et al. Opportunistic measurement of skeletal muscle size and muscle attenuation on computed tomography predicts 1-year mortality in Medicare patients. journals gerontology Ser A Biol Sci Med Sci (2019) 74(7):1063–9. doi: 10.1093/gerona/gly183
12. McGovern J, Dolan RD, Horgan PG, Laird BJ, McMillan DC. Computed tomography-defined low skeletal muscle index and density in cancer patients: observations from a systematic review. J cachexia sarcopenia muscle (2021) 12(6):1408–17. doi: 10.1002/jcsm.12831
13. Mazzone P, Powell CA, Arenberg D, Bach P, Detterbeck F, Gould MK, et al. Components necessary for high-quality lung cancer screening: American college of chest physicians and American thoracic society policy statement. Chest (2015) 147(2):295–303. doi: 10.1378/chest.14-2500
14. Tanimura K, Sato S, Fuseya Y, Hasegawa K, Uemasu K, Sato A, et al. Quantitative assessment of erector spinae muscles in patients with chronic obstructive pulmonary disease. novel chest computed tomography-derived index for prognosis. Ann Am Thorac Soc (2016) 13(3):334–41. doi: 10.1513/AnnalsATS.201507-446OC
15. Amini B, Boyle SP, Boutin RD, Lenchik L. Approaches to assessment of muscle mass and myosteatosis on computed tomography: A systematic review. journals gerontology Ser A Biol Sci Med Sci (2019) 74(10):1671–8. doi: 10.1093/gerona/glz034
16. Mitchell WK, Williams J, Atherton P, Larvin M, Lund J, Narici M. Sarcopenia, dynapenia, and the impact of advancing age on human skeletal muscle size and strength; a quantitative review. Front Physiol (2012) 3:260. doi: 10.3389/fphys.2012.00260
17. Byun MK, Cho EN, Chang J, Ahn CM, Kim HJ. Sarcopenia correlates with systemic inflammation in COPD. Int J Chron Obstruct Pulmon Dis (2017) 12:669–75. doi: 10.2147/COPD.S130790
18. Hicks GE, Simonsick EM, Harris TB, Newman AB, Weiner DK, Nevitt MA, et al. Trunk muscle composition as a predictor of reduced functional capacity in the health, aging and body composition study: the moderating role of back pain. journals gerontology Ser A Biol Sci Med Sci (2005) 60(11):1420–4. doi: 10.1093/gerona/60.11.1420
19. Aubrey J, Esfandiari N, Baracos VE, Buteau FA, Frenette J, Putman CT, et al. Measurement of skeletal muscle radiation attenuation and basis of its biological variation. Acta Physiol (Oxf) (2014) 210(3):489–97. doi: 10.1111/apha.12224
20. Anderson DE, D'Agostino JM, Bruno AG, Demissie S, Kiel DP, Bouxsein ML. Variations of CT-based trunk muscle attenuation by age, sex, and specific muscle. journals gerontology Ser A Biol Sci Med Sci (2013) 68(3):317–23. doi: 10.1093/gerona/gls168
21. Tanimura K, Sato S, Sato A, Tanabe N, Hasegawa K, Uemasu K, et al. Accelerated loss of antigravity muscles is associated with mortality in patients with COPD. Respiration (2020) 99(4):298–306. doi: 10.1159/000506520
22. van de Bool C, Gosker HR, van den Borst B, Op den Kamp CM, Slot IG, Schols AM. Muscle quality is more impaired in sarcopenic patients with chronic obstructive pulmonary disease. J Am Med Directors Assoc (2016) 17(5):415–20. doi: 10.1016/j.jamda.2015.12.094
23. Attaway AH, Welch N, Yadav R, Bellar A, Hatipoglu U, Meli Y, et al. Quantitative computed tomography assessment of pectoralis and erector spinae muscle area and disease severity in chronic obstructive pulmonary disease referred for lung volume reduction. COPD (2021) 18(2):191–200. doi: 10.1080/15412555.2021.1897560
Keywords: chronic obstructive pulmonary disease, L1-trunk muscle, muscle size, muscle density, change
Citation: Wang Y, Li S, Zhang Z, Sun S, Feng J, Chen J, Pei Y and Peng X (2022) Accelerated loss of trunk muscle density and size at L1 vertebral level in male patients with COPD. Front. Endocrinol. 13:1087110. doi: 10.3389/fendo.2022.1087110
Received: 02 November 2022; Accepted: 28 November 2022;
Published: 15 December 2022.
Edited by:
Ling Wang, Beijing Jishuitan Hospital, ChinaReviewed by:
Yimin Ma, Beijing Jishuitan Hospital, ChinaCopyright © 2022 Wang, Li, Zhang, Sun, Feng, Chen, Pei and Peng. This is an open-access article distributed under the terms of the Creative Commons Attribution License (CC BY). The use, distribution or reproduction in other forums is permitted, provided the original author(s) and the copyright owner(s) are credited and that the original publication in this journal is cited, in accordance with accepted academic practice. No use, distribution or reproduction is permitted which does not comply with these terms.
*Correspondence: Xianjing Peng, cGVuZ3hpYW5qaW5nQGNzdS5lZHUuY24=
†These authors have contributed equally to this work
Disclaimer: All claims expressed in this article are solely those of the authors and do not necessarily represent those of their affiliated organizations, or those of the publisher, the editors and the reviewers. Any product that may be evaluated in this article or claim that may be made by its manufacturer is not guaranteed or endorsed by the publisher.
Research integrity at Frontiers
Learn more about the work of our research integrity team to safeguard the quality of each article we publish.