- 1Department of Reproductive Medicine, The Second Hospital of Hebei Medical University, Shijiazhuang, China
- 2Shenzhen Key Laboratory of Reproductive Immunology for Peri-Implantation, Shenzhen Zhongshan Institute for Reproduction and Genetics, Shenzhen Zhongshan Urology Hospital, Shenzhen, Guangdong, China
- 3Second Department of Respiratory and Critical Care Medicine, The Second Hospital of Hebei Medical University, Shijiazhuang, Hebei, China
The local Renin-Angiotensin System (RAS) has been demonstrated to exist in a wide range of tissues and organs, In the female reproductive system, it is mainly found in the ovary, uterus and placenta. The RAS system is made up of a series of active substances and enzymes, in addition to the circulating endocrine renin-angiotensin system. The active peptides Angiotensin II (Ang II) and Angiotensin (1-7) (Ang-(1-7)), in particular, appear to have distinct activities in the local RAS system, which also controls blood pressure and electrolytes. Therefore, in addition to these features, angiotensin and its receptors in the reproductive system seemingly get involved in reproductive processes, such as follicle growth and development, as well as physiological functions of the placenta and uterus. In addition, changes in local RAS components may induce reproductive diseases as well as pathological states such as cancer. In most tissues, Ang II and Ang- (1-7) seem to maintain antagonistic effects, but this conclusion is not always true in the reproductive system, where they play similar functions in some physiological and pathological roles. This review investigated how Ang II, Ang- (1-7) and their receptors were expressed, localized, and active in the female reproductive system. This review also summarized their effects on follicle development, uterine and placental physiological functions. The changes of local RAS components in a series of reproductive system diseases including infertility related diseases and cancer and their influence on the occurrence and development of diseases were elucidated. This article reviews the physiological and pathological roles of Ang II and Ang- (1-7) in female reproductive system,a very intricate system of tissue factors that operate as agonists and antagonists was found. Besides, the development of novel therapeutic strategies targeting components of this system may be a research direction in future.
Introduction
It is commonly known that the ovary, uterus, and placenta all contain local renin-angiotensin systems (1). However, its physiological and pathological significance in female reproduction is still unknown. Ang II and Ang- (1-7) are two of the RAS system’s prominent active peptides. Ang II and Ang- (1-7) are bioactive substances involved in blood pressure regulation. These compounds are mostly linked to the incidence and progression of cardiovascular disorders (2). The biological effects of Ang-(1-7) are often in opposition to those of Ang II (3). With the depth of its research, recent studies have shown that it has a close bearing on physiological functions and pathological changes of the reproductive system (4–6).
The AT1 and AT2 receptors are the two main subtypes of Ang II in classical RAS, which is generated by Ang I in response to ACE (7). Ang II acts through AT1 using various signaling mechanisms, similar in some ways to those brought on by the EGF family and hormones mobilized by Ca 2+. On the contrary, phosphatases are primarily used to convey signals caused by activation of the AT 2 receptor. As a result, AT 2 receptor activation is thought to inhibit AT 1 receptor-mediated signaling (8).
Ang-(1-7) is generated through four metabolic pathways. The first is the hydrolysis of Ang I by enzymes such as enkephalin (NEP). Secondly, the angiotensin converting enzyme 2 (ACE2) cleaves Ang II to produce Ang-(1-7). Thirdly, ACE and NEP hydrolyze Ang-(1-9) to form Ang-(1-7). Additionally, Ang- (1-7) can be produced directly from Ang- (1-12) (9, 10). Among them, the cleavage of Ang II by ACE2 is the primary mechanism of production of Ang- (1-7) (11, 12). Although previous literature has demonstrated that Ang- (1-7) can act through AT1, AT2 and MAS receptors, it has radically differing affinities for AT1 and AT2 receptors (13, 14). MAS1 proto-oncogene G protein-coupled receptors are another route via which Ang- (1-7) can exert its effects (15), this is the main way that Ang- (1-7) plays its function. Ang II can contractile blood vessels, increase blood pressure, promote aldosterone secretion and promote cell proliferation through AT1 receptor. Ang II can inhibit cell proliferation, promote vascular dilatation, promote cell differentiation and induce cell apoptosis through AT2 receptor. Ang- (1-7) can diastolic blood vessels, reduce blood pressure, inhibit myocardial remodeling, improve endothelial function and inhibit cell proliferation through Mas receptor (Figure 1).
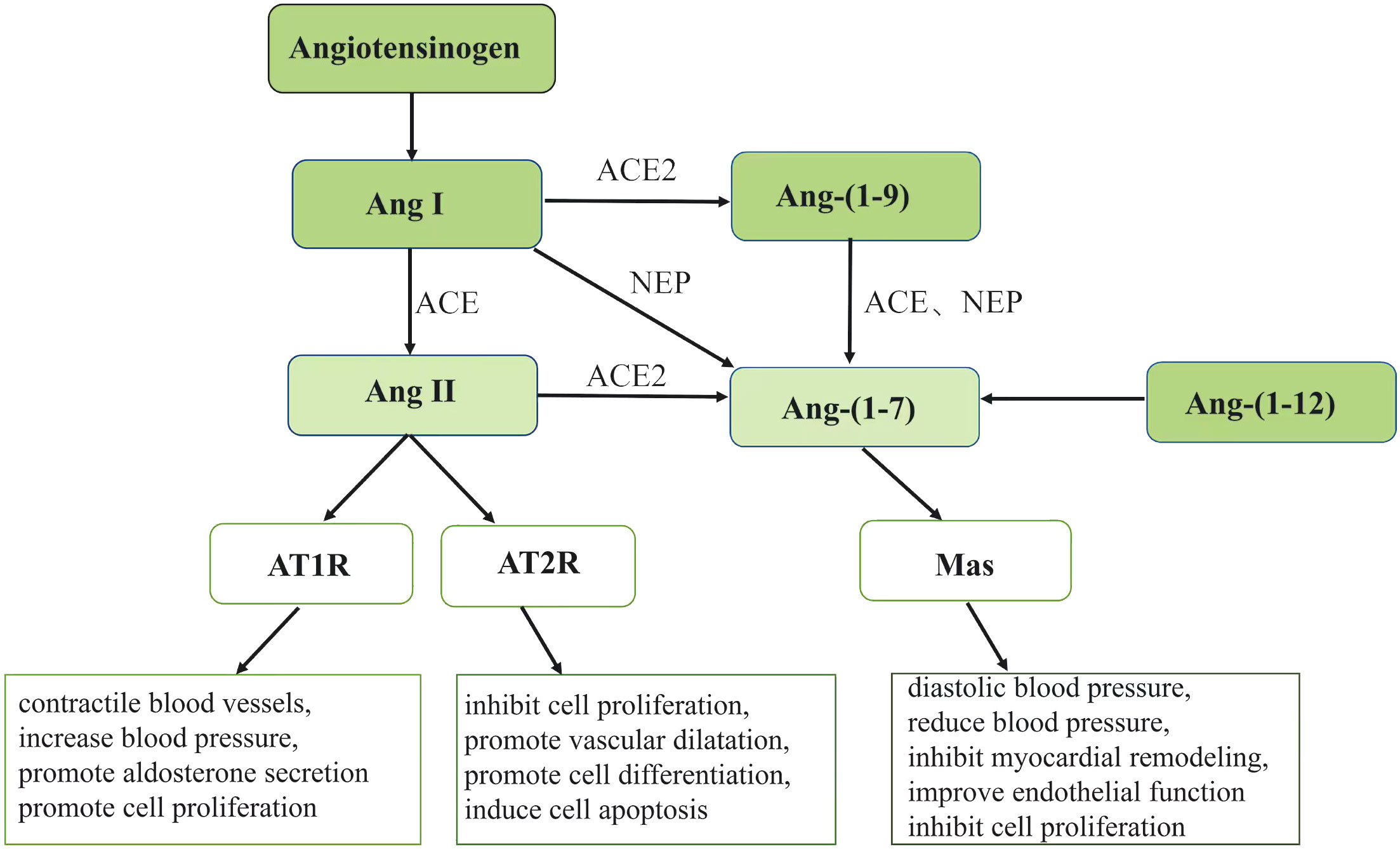
Figure 1 Ang, angiotensin; ACE, angiotensin converting enzyme; ACE2,angiotensin converting enzyme 2; AT1R, angiotensin receptor 1; AT2R, angiotensin receptor 2; NEP, enkephalin enzymes. The figure shows the pathway of Ang II and Ang- (1-7) generation. Angiotensinogen can generate Ang I and then hydrolyze Ang I to Ang II under the action of ACE. The generated Ang II can play different physiological roles through different receptors AT1R and AT2R.Ang- (1-7) can be generated through four pathways under the action of different enzymes and exert its physiological effects through Mas receptors.
Changes in local Ang II and Ang- (1-7) activity can cause illnesses of the reproductive system, which in turn can lead to fertility issues. The content of this review is to describe the positive and negative effects of Ang II, Ang-(1-7) and their receptors on the biology of the ovary, the uterus, and the placenta. It provided an overview of Ang II and Ang- (1-7) expression, location, metabolism, and action in many reproductive organs, and elucidated their roles in physiological functions of the reproductive system. Finally, the variations in Ang II and Ang- (1-7) expression and activity in pertinent disorders were investigated. Additionally, their effects on the onset and progression of illnesses as well as their potential for the therapy of connected disorders were explored.
Ang II, Ang- (1-7) and physiology of female reproductive system ovary
The primary human organ from which plasma reninogen is derived is the ovary (12).
Renin, which catalyzes the transformation of angiotensinogen into Ang I, is a precursor of prorenin. Ang I can either be immediately hydrolyzed to Ang- (1–7) or transformed into Ang II. Following that, Ang II was hydrolyzed by angiotensin converting enzyme 2 (ACE2) (16).
The follicular fluid contains Ang II and Ang- (1-7). While Ang- (1-7) is substantially less concentrated in the follicular fluid than it is in the plasma, Ang II is more abundant in the follicular fluid than it is in the plasma (2). This difference may be owing to the extra-ovarian origin of Ang II or the shorter half-life of Ang- (1-7) in follicular fluid. There was evidence that AT1R and AT2R were present in granulosa cells of primordial, primary, and secondary follicles (17). In the corpus luteum, microvascular endothelial cells express both AT1R and AT2R, although their expression varies during the course of the cycle: While AT1R expression does not change, AT2R expression decreases in the middle of the luteal phase and increases in the late luteal phase (14–16). All developmental stages of follicles are immune-responsive to Ang-(1-7) and Mas, except for the corpus luteum, ovarian stroma, and blood vessels (2, 18). The expression of Ang- (1-7) and Mas also varies in different developmental stages of follicles. In the granulosa layer of follicles, immunostaining can be observed in primary follicles, and strong immune response can be observed in secondary follicles. The Ang- (1-7) labeling is weak in preovulatory follicles, and Mas is moderately strong labeling. In secondary follicles, there was a significant immunoreactivity for Ang- (1-7) and Mas, and both were negative in preovulatory follicles. In the cytoplasm of large and small luteal cells, Ang- (1-7) labeling was weak, but Mas labeling was strong. Postmenopausal women’s ovarian stromal cells stained moderately with Ang- (1-7) and Mas (2, 18).
Relevant studies have demonstrated that Ang II and its receptors have regulatory effects on oocyte maturation (19–22), In-vitro culture of bovine cumulus oocyte complex with Ang II can induce oocyte nucleus maturation (21, 23). Aside from that, Ang II also contributes to ovulation. Ang II can affect ovulation in cattle and rats through AT2R. Moreover, this effect can be prevented by inhibiting AT2R (24). Furthermore, the results showed that AT2R was also involved in follicular atresia through apoptosis (25, 26). Also, Ang- (1-7) participates in oocyte maturation and ovulation. Ang- (1-7) can promote the recovery of oocyte meiosis and induce ovulation in the absence of gonadotrophins, and an antagonist specific to Ang-(1-7), A779, inhibits these functions (18).
The plasma Ang-(1-7) levels in gonadotropin-stimulated women were shown to be elevated in a study of patients undergoing ovarian stimulation (COS) with human urine gonadotropin (HMG) and/or recombinant FSH (rFSH) (18) (2). Additionally, a linear relationship between Ang-(1-7) concentrations in human follicular fluid and the percentage of developed oocytes was discovered. However, in women with poor ovarian reserve function, AT1 receptor expression in granulosa cells was inversely linked with the amount of mature oocytes (27) (Table 1)
The expression and distribution of Ang II, Ang- (1-7) and their receptors are not the same among different species in ovary, which indicates that Ang II, Ang- (1-7) and their receptors may have different physiological effects. As the main bioactive substances in the renin angiotensin system, Ang II and Ang- (1-7) generally perform different functions and maintain a relative balance. Interestingly, in the ovary both seem to act in the same direction and play a crucial part in the physiological function of the ovary.
Endometrial
The endometrium undergoes periodic proliferation, differentiation and shedding during the menstrual cycle. Changes in the endometrium, driven by ovarian steroid hormones, play a crucial role in embryo implantation and pregnancy establishment (28). As reported by previous studies, Ang II and Ang- (1-7) and their related receptors can be detected in the endometrium throughout the menstrual cycle and change with the menstrual cycle (29). Ang II is most expressed in the proliferative phase, when it is predominantly located in glandular epithelium and stroma (30). Its expression is reduced during the secretory phase and is restricted to vascular stromal cells surrounding spiral arterioles in the endometrium (30, 31). The uterus has expression of both the Ang II receptor type 1 (AT1) and the Ang II receptor type 2 (AT2) proteins. The majority of AT1 is found in the endometrium, which has a high level of expression during the late proliferative phase but a lower level of expression during the secretory phase. AT2 was principally expressed in myometrium and uterine artery (32). Some studies have suggested that Ang II receptor activation can bring about apoptotic changes in the vascular wall (33). therefore, Ang II may be involved in endometrial vasoconstriction as well as vascular remodeling during menstruation (34). The expression of Ang- (1-7) was weak in endometrial glands at the early and middle stages of proliferation. Nonetheless, at the late stage of secretion, the expression of Ang- (1-7) increased and peaked. Ang- (1-7) was detected in the early stage of endometrial stromal hyperplasia, but not in the endothelium of endometrial vessels in the late secretory stage (30). The Ang- (1-7) receptor Mas, in contrast to Ang II receptor, only minimally enhanced in the glandular epithelium of the middle and late secretory stage, and did not change significantly with the fluctuation of ovarian steroid hormones. An experiment in which ovariectomized rats were given with estrogen or estrogen in combination with progesterone provided additional evidence that the expression of Mas receptors in the endometrium is not regulated by female sexual hormones (35, 36) (Figure 2)
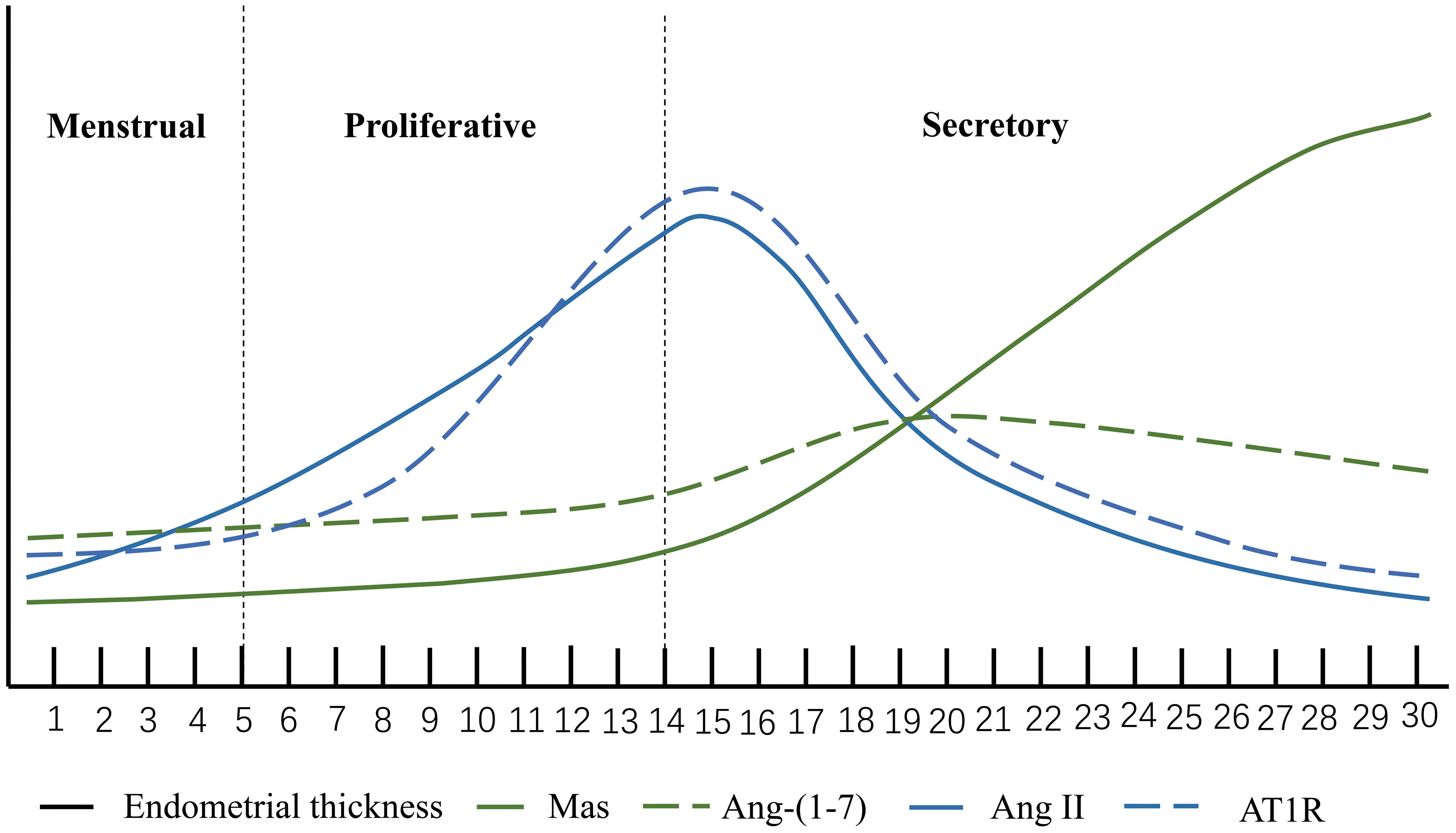
Figure 2 Ang II, Ang-(1-7), AT1R and Mas. The horizontal axis represents the menstrual cycle. The height of the vertical coordinate. The expression level of the molecule at each stage of the menstrual cycle.
However, there are still few studies bound up with the physiological function of local renin-angiotensin system in the uterus. Two studies on human endometrial cells cultured in vitro found that Ang II could induce cell proliferation and increase cell activity, but these effects could be offset by adding Ang- (1-7) (37, 38). Ang II can promote the transdifferentiation of endometrial epithelial cells into myofibroblasts. Except for this, Ang II has the ability to induce the activation of collagen type I as well as fibronectin. A recent study significantly prevented the biochemical and histopathologic changes resulted from endometrial hyperplasia by treating rats with angiotensin II receptor blockers. This means that the imbalance of local uterine RAS system and Ang II activation may trigger endometrial fibrosis, but this effect can also be inhibited by Ang- (1-7) (39, 40).
Placenta
As a specialized connection for maternal exchange, the placenta supports hormone secretion, gas and nutrient exchange, as well as the establishment of an immune barrier (41). In the placental villi, high quantities of Ang II were found, and the expression of renin and ACE was also observed. AT1R and AT2R were detected in trophoblast cells (42). Different from the weak expression of AT2R, the expression of AT1R was quite high at the transcriptional level as well as the translational level throughout the whole pregnancy (42). All these circumstances suggest that placenta also has local RAS. As early as the second week of embryonic development, local RAS can promote blastocyst implantation, and Ang II can also heighten endometrial cell permeability by promoting decidual cell differentiation, thereby allowing trophoblast cell invasion into the maternal endometrium (43). Numerous studies have shown the pivotal function of uteroplacental RAS in spiral arterial remodeling and angiogenesis (44, 45). It has been shown that Ang II can influence the production of vascular endothelial growth factor (VEGF) and placental growth factor (PlGF) in vascular endothelium and smooth muscle cells via activating AT1R in these cell types (46). In normal pregnancy, both VEGF and PlGF may be found in the trophoblast as well as the maternal decidua. In addition to this, VEGF and PlGF play a significant part in the development of the placental vasculature by controlling the growth of blood vessels in the chorionic villi (47). AT1R signaling in trophoblast cells also helps to enhance the synthesis of antiangiogenic molecules such as soluble Flt1 (sFlt1) and soluble endocrine hormone (sEng) (48), which helps to maintain a healthy equilibrium in placental angiogenesis. In addition to upregulation that occurs in trophoblast as a result of AT1R activation, sFlt1 expression also activates AT2R and Mas receptors via the binding of Ang II and Ang (1-7), respectively. This is not the case with sEng, indicating that the control of sEng is primarily dependent upon AT1R signaling and does not call for the involvement of AT2R or Mas receptors (49–51) (Figure 3)
It has been shown that angiotensin II is involved in the control of placental blood flow, hormone production, and immunological function. According to one research, Ang II may cause vasoconstriction in separate chorionic plate arteries (52). Pregnant mice lacking AT2R experience a gradual rise in blood pressure, indicating that AT2R signaling in uteroplacental arteries is crucial for maintaining a healthy placental perfusion balance (53, 54). Ang II may modify the impact of nutrition transport by affecting blood vessels. The enhanced secretion of hPL and SP1 and the synthesis of estrogen might both be boosted by Ang II, according to in-vitro tests on placental explants (48). The use of certain pharmacological blockers lends credence to the hypothesis that this function is mediated by AT1R and is associated with calcium signaling. In addition, it has been shown that Ang II, when co-cultured with trophoblast cells, affects monocyte adhesion in vitro and modulates immunity in the placenta (55–57).
There are few studies on the expression, localization and function of Ang- (1-7) in the placenta, and more evidence is needed to reveal its interaction with the placenta.
Ang II, Ang- (1-7) and the pathophysiology of female reproductive system ovarian hyperstimulation syndrome
The most dangerous side effect of ovulation induction is a condition called ovarian hyperstimulation syndrome (OHSS), which has a high fatality rate as well as a high morbidity rate. Ovarian enlargement, increased vascular permeability, extracellular fluid accumulation, and electrolyte imbalance are the hallmarks of severe OHSS (58).
High renin activity was found in both follicular fluid and plasma in patients with OHSS and was found to be directly associated with the severity of ovarian hyperstimulation syndrome (59–61). Several results showed that ascites from patients with severe OHSS had considerably greater amounts of reninogen and angiotensin II than pleural effusion and plasma did (62, 63). This result suggests that besides the peripheral circulation, the RAS system is also activated locally in the ovary. Activated ovarian RAS may cause ovarian enlargement and extracellular fluid accumulation in OHSS patients by inducing neovascularization and increasing capillary permeability.
Several researches have evaluated the effectiveness of medications that inhibit angiotensin converting enzyme and drugs that block angiotensin II receptors (64). Enalapril oral treatment was thought to have a 40% reduction in the occurrence of OHSS in a rabbit model, according to Morris et al. Nonetheless, Sahin et al. observed that neither hindering ACE nor blocking AngII receptor improved the ascites of OHSS in rabbits (65). Two clinical trials investigated the efficacy of dual renin-angiotensin blockade (a combination of angiotensin receptor blocker and angiotensin converting enzyme inhibitor) in preventing OHSS in IVF patients. It was found that this treatment may prevent some of the symptoms of OHSS and maintain a higher pregnancy rate after transfer of frozen-thawed embryos. However, OHSS cannot be completely eliminated by this treatment (66, 67).
Polycystic ovary syndrome
Polycystic ovary syndrome (PCOS) is a complex illness that affects both the endocrine and metabolic systems. Its typical clinical phenotype includes Hyperandrogenism, insulin resistance, anovulation/anovulation and polycystic changes of the ovary are the most common causes of anovulation infertility (68).
There have been some studies on the expression changes of RAS components in patients with PCOS (69). According to Alphan et al., PCOS patients had greater blood total renin concentrations than non-PCOS patients (70). According to a survey, total renin concentrations were greater in obese PCOS women, and they were associated with fasting insulin and free testosterone levels. Although there was no discernible difference between PCOS women and controls in terms of plasma renin activity, ACE activity and Ang II levels were both markedly elevated in PCOS patients (71). Based on these findings, PCOS patients have an active renin-angiotensin system. A recent research, however, found that the ACE2-Ang- (1-7) -Mas axis in the ovary was hindered in polycystic ovarian rats. But in this study, a rat model characterized only by polycystic ovaries was used. Under the circumstances, RAS system expression levels are affected by multiple hormonal changes. As a consequence, the results of this experiment cannot preferably express the changes of RAS components in PCOS (72). In the endometrium of PCOS patients, a study of 15 endometrium of PCOS patients showed that the mRNA expression levels of ACE2, AT1, AT2 and Mas receptors in the endometrium of PCOS patients were higher than those in the control groups (73), These results make it clear that the elevated expression level of RAS components may affect the development of endometrium and play a role in its pathological process.
Insulin resistance and hyperandrogenemia are important clinical expressions of PCOS, and they are not independent. For example, insulin resistance can induce the increase of androgen level. As a multitude of studies have shown that Ang- (1-7) receptors can alleviate a myriad of metabolic diseases through Mas receptors and also counteract a range of adverse effects of Ang II, hypertensive drugs that block the action of Ang II can improve insulin resistance (74). Insulin resistance and hyperandrogenemia are the most common symptoms in PCOS patients. Studies have shown that Ang- (1-7) can not only improve the action of insulin, but also increase the delivery of insulin to target organs through vasodilation (75). A range of evidence confirms that angiotensin receptor blockers and angiotensin converting enzyme inhibitors can improve insulin resistance (76). One study showed that lisinopril increased insulin sensitivity and reduced total and free testosterone in PCOS patients with hypertension (77). Angiotensin receptor antagonists and angiotensin converting enzyme inhibitors have positive effects on symptom improvement in PCOS patients. As mentioned above, both hyperandrogenism and insulin resistance are improved by hindering the RAS system, which may provide a new strategy for treating PCOS (77).
Additionally, some research have shown that the D allele of the ACE gene may be linked to acanthosis and a greater risk of insulin resistance in PCOS patients (78, 79). Other investigations have verified that, despite the ACE gene polymorphism not being linked to the pathophysiology of PCOS, it may be strongly linked to a number of metabolic diseases such insulin resistance, hyperlipidemia, and hyperandrogenemia (80, 81). And because it is bound up with steroid production in the ovary, it may also be connected with the exacerbation of clinical expressions of PCOS (82).
Ovarian cancer
Ovarian cancer (OC) is the most deadly kind of cancer that may develop in a woman’s reproductive system. OC seriously affects life and health, but its pathogenesis remains unclear at present. The formation of tumors may be linked to the enhanced local expression of Ang II and its receptors in the ovary (83), Ang- (1-7), on the other hand, can reduce the growth, migration potential and invasiveness of tumors by several mechanisms against Ang II.
Takayasu et al. (84) Studies examining AT1R expression in human ovarian cancer and attempting to determine whether AT1R blockers could inhibit tumor progression showed AT1R expression in 85% invasive ovarian adenocarcinomas, 66% borderline malignancies, and 14% benign cystadenomas. In AT1R positive patients, there was a considerable increase in the amount of VEGF expression and tumor microvessel density. In-vitro studies proved that AT1R blockers could lessen the invasion potential and VEGF secretion of ovarian cancer cells. Previous research has shown that invasive and borderline diseased epithelial ovarian tumors had greater AT1R levels than healthy ovaries. Furthermore, earlier research has shown that individuals with ovarian cancer who have high AT1R levels had a poorer prognosis (83). Serum ACE levels are elevated in OC patients. As for epithelial OC patients, serum ACE levels do not vary according to patient stage and pathological subtype. This situation suggests that serum angiotensin I converting enzyme may be a marker of disseminated germinoma (85).
Ang II and ovarian cancer cells can hasten the metastasis of epithelial ovarian cancer (EOC) through interaction (86). Higher blood AT1-AA titers were shown to be related with the late advancement of EOC in patients, according to the findings of another investigation. What’s more, higher serum AT1-AA titers may significantly contribute to the progression of EOC by facilitating cancer cell migration and angiogenesis (87). In ovarian cancer, high levels of AT1R expression are linked to increased rates of tumor angiogenesis and a poor prognosis, and AT1R blockers have been shown to inhibit peritoneal spread of ovarian cancer in a mouse model (88). In vitro and in vivo experiments on OC cell lines have shown that Candesartan, an AT1 receptor antagonist, can not only inhibit the invasion ability of tumor cells in vitro, but also inhibit the growth and progression of tumor and the formation of blood vessels in mice (84, 88). Therefore, a series of studies have confirmed that Ang II and Ang- (1-7) can participate in the pathological process of ovarian cancer by affecting the proliferation, migration and angiogenesis of tumor cells (6, 55, 89, 90).
Endometriosis
Endometriosis (Ems) is an estrogen-dependent condition marked by the development of adenocarcinoma and endometrial stromal cells outside of the uterine covered mucosa and myometrium. Although endometriosis is a benign disease, it can result in abdominal pain, menstrual abnormalities, and infertility, which disadvantageously affects about 10 percent of women in childbearing age (91).
The pathogenesis of endometriosis remains unclear. Worse still, the etiology and pathology of infertility are more complex. In the endometrium of people with endometriosis, there is enhanced expression of the Ang-1-7 receptor Mas, and the high expression of Mas may further the occurrence of endometriosis (92). Angiotensin type 1 receptor is involved in angiogenesis and affects endometrial growth, invasion and regression (93). In another trial using the AT1R antagonist telmisartan to treat mice endometriosis, it inhibited angiogenesis, immune cell content, and lesion growth in endometriosis (94). Tanshinone IIA not only significantly inhibits the growth of ectopic endometrium, but also alleviates EMs-induced pain syndrome by regulating RAS in neurons (95). However, on account of the physiological differences between human and mouse endometrium, a more complete theoretical and experimental basis is essential for applying this result to patients with endometriosis needs.
Studies on endometriosis and ACE gene polymorphism suggest that ACE gene polymorphism has some connection with the susceptibility and development of endometriosis. Furthermore, the ACE gene polymorphism can inhibit EMS-related pain by blocking the expression of Ang II and AT2R (95–98). On the other hand, some studies have borne out that ACE gene polymorphisms and haplotypes are not associated with endometriosis, and no association has been found between ACE gene polymorphisms and the prevalence, progression, or number of lesions of endometriosis (99–101).
Endometrial cancer
Endometrial cancer (EC) is a primary epithelial malignant tumor of the endometrium, accounting for 20%-30% of female reproductive tract malignant tumors. It is generally believed that abnormal hormone secretion, genetic factors and environmental factors are connected to the onset and progression of the illness (102).
As we all know, the endometrium is responsible for the expression of all RAS components. The mRNA levels of AT1R, ACE1, and ACE2 were shown to be greater in tumor tissues of patients with endometrial cancer (103), compared to the levels found in neighboring non-cancerous tissues. The high expression of these factors supports the possibility that RAS contributes significantly to the emergence of endometrial cancer. The impact of Ang II on endometrial cancer cells was investigated in a study using endometrial cell lines. The results indicated that Ang II could augment the potential of cancer cell proliferation, metastasis and adhesion, and was associated with the highly differentiated state of cancer cells (104). However, to some degree, the capacity of tumor cells to migrate and invade may be impeded by silencing of the Ang II AT1R receptor, but this change is not absolute (105). Hence, Ang II may have a more complex network of effects in endometrial cancer, which needs further exploration. In endometrial cancer, Ang II increases the production of VEGF in a dose-dependent way and stimulate angiogenesis. Overexpression of Ang II-degrading enzymes and small interfering RNA were able to suppress both the immunoreactivity of VEGF as well as the number of blood vessels that were present in the tumor (106). One study observed that the AT1R blocker telmisartan significantly inhibited the growth of human endometrial tumors without toxic effects. This may provide a new strategy for future treatment of EC (105).
ACE gene polymorphism may be associated with the pathogenesis of endometrial cancer, and may be related to the development of the disease as well as the age of onset (31, 107, 108). Ang II may affect the progression of endometrial cancer by promoting the proliferation, differentiation and invasion of tumor cells. At present, few data can throw light upon the expression of Ang- (1-7) and its receptor in endometrial cancer and its effect on endometrial cancer, which may be the direction of future research, so as to further improve the research in endometrial cancer.
Preeclampsia
Preeclampsia (PE), which is a distinct pregnancy illness and a leading cause of maternal, fetal, and neonatal mortality with no proven cure other than delivery, is defined as the start of new-onset hypertension and proteinuria after 20 weeks of gestation (109). According to the performance of different pregnancy stages, PE is divided into early onset and late onset. Although the symptoms are similar, the causes of the two types are not the same (110). Early-onset PE is more commonly thought to be due to defects in placental development, so we are going to put our attention on early-onset PE.
Although the specific pathogenesis of PE is not adequately understood, numerous studies have shown that placental ischemia caused by reduced uteroplacental blood flow is the primary foundation of this pregnancy condition (111, 112). In PE pregnant women, the levels of Ang II and Ang- (1-7) were found to be much lower than those seen in normal pregnant women. Interestingly, the pressor response of Ang II and the amount of autoantibodies to the AT1 receptor are elevated (113). At1-aa can give rise to vasoconstriction through AT1R on vascular smooth muscle, and also promote the upregulation of various active substances (114–116), among which NADP upregulation enhances ROS formation, PAI-1(plasminogen activator inhibitor -1) reduces trophoblast invasion (117, 118). The increase of circulating sFlt1 level can result in the decrease of VEGF and PLGF bioavailability (50). Clinically, hypertensive individuals’ risk of developing PE may be determined by assessing the ratio of circulating sFlt1/PIGF, which has the highest sensitivity and specificity in predicting and diagnosing PE. The decrease of free VEGF level can induce the expression of endothelin-1, touch off the decrease of renin secretion, further strengthen the negative regulation of blood volume, and thus cause the poor perfusion of placenta, which are associated with the development of the illness in its various stages (119). Aside from this, the increase of placental renin gene expression and protein level in transcription factor replacement and (Stox1) knockout mice gave rise to gestational hypertension through this change, which was alleviated by Ang II receptor blocker treatment, indicating that stox1 contributes to the development of PE through Ang II (120, 121).
There are different opinions on the expression of Ang- (1-7) in PE. One research revealed that early in pregnancy, PE patients’ levels of Ang-(1-7) were greater than those of normal pregnant women, and this difference only existed in women with female fetuses (122). We believe that the pathogenesis of PE has a bearing on the imbalance of RAS. In future studies, we need to learn more about how Ang II and Ang- (1-7) are expressed and look into the reasons why PE patients have atypical Ang II and Ang- (1-7) expressions. Patients with PE have RAS disorder, especially the activation of AT1R can participate in the pathogenesis of PE by promoting the validation reaction-mediated oxidative stress and other pathways (123). Angiotensin antagonists and angiotensin-converting enzyme inhibitors can lower blood pressure, but cannot be recommended as oral drugs due to their teratogenic effects.
Coronavirus disease 2019
Coronavirus disease 2019 (COVID-19) is an infectious disease caused by severe acute respiratory syndrome coronavirus 2 (SARS-CoV-2). It is associated with respiratory tract involvement and typical symptoms include dry cough, dyspnoea, fever, etc (124). In addition, the understanding of whether women’s reproductive systems can be affected by the coronavirus continues to improve (125). ACE2 is a key enzyme in the RAS of this disease, not only regulating the expression of Ang II and Ang-(1-7), but also acting as a receptor to mediate infection of target cells by SARS-CoV-2 (126). ACE2 is present at all stages of follicular development. Ang II is mainly expressed in granulosa cells and Ang-(1-7) is expressed primarily in intermembrane cells. Both Ang II and Ang-(1-7) are involved in the development and maturation of oocytes (6). Two studies in women of childbearing age showed no significant effect of SARS-CoV-2 on ovarian reserve function (127, 128). Another analysis of 32 in vitro fertilization (IVF) patients revealed there were no obvious differences in follicular function, including but not limited to hormone synthesis, trigger response and embryo quality (129). However, it was also suggested that SARS-CoV-2 could cause a decrease in ACE2 expression, leading to an increase in Ang II and an imbalance in the expression of Ang II and Ang-(1-7) in the ovary. Consequently, it induced enhanced local inflammation, fibrosis, apoptosis and other pathological responses in the ovary, further affecting the reproductive function of the ovary. The most attention in research was paid to the effect of SARS-CoV-2 on menstruations. A series of studies indicated that SARS-CoV-2 has a significant impact on menstrual patterns, menstrual volume and dysmenorrhea (4, 130, 131). This might be because ACE2, Ang II and Ang-(1-7) can maintain cyclic changes in the endometrium by participating in vasoconstriction as well as cell renewal and proliferation. Since ACE2, which functioned as the SARS-CoV-2 receptor, was low in abundance in the endometrium, it did not show susceptibility to the coronavirus (132). Intriguingly, studies suggested that female age can be a risk factor for COVID-19. This was achieved because the expression of ACE2 in the endometrium increased with age (4). ACE2 was widely expressed in the placenta, including trophoblast cells, perivascular and vascular smooth muscle cells of decidual vessels as well as decidual stromal cells (133). This inferred that the placenta was more prone to the effects of SARS-CoV-2. Furthermore, the expression of ACE2 was not constant in the placenta. The level of ACE2 expression decreased with increasing gestational age, suggesting a greater likelihood of transplacental transmission of SARS-CoV-2 in early gestation (134). Although it was theoretically possible for SARS-CoV-2 to be transmitted to infants through the placenta. However, studies demonstrated that most newborns were not found to be infected after birth. It was hypothesized that this was possibly due to the barrier effect of the placenta, but the exact mechanisms of action remained unclear (135). Other than the possible effects mentioned above, SARS-CoV-2 could also affect endocrine organs such as the thyroid gland, thus impacting the endocrine system of patients (136, 137). This might further cause alterations in female fertility. Due to limited clinical data, the understanding of the impact of SARS-CoV-2 on the female reproductive system is not comprehensive and profound. Therefore, predicting the impacts of SARS-CoV-2 on the female reproductive system and the specific mechanisms of action will be an essential direction for future research.
Conclusion
In conclusion, the female reproductive system does in fact include a local renin-angiotensin system. Numerous studies have also demonstrated that angiotensin, including Ang II and Ang- (1-7), can be produced locally in the reproductive system. RAS affects oocyte maturation and excretion, endometrial periodic changes and hormone production. RAS is considered to be an important way to regulate physiological functions of the reproductive system. However, the functions that Ang II and Ang- (1-7) play in the physiology and pathology of the female reproductive system are still not fully understood. Furthermore, most of the previous studies remain at the level of nonfunctional description. The intricate dependency network in the peptide-hormone system in issue, together with species variations, are the main causes of the interpretation challenges. Undoubtedly, further study in this field is required to fully comprehend how RAS affects female fertility. Likewise, the intensive study in the future is indisputably essential to determine the contribution of RAS to a wide range of related diseases.
Author contributions
YL and ZZ contributed to conceived and designed the review. YL wrote the paper. RJ and HH did the document retrieval. LZ and MC polished the paper. ZZ and WP checked the paper. All authors listed have made a substantial and intellectual contribution to the review and approved it for publication.
Funding
This study was supported by S&T Program of Hebei (22377742D).
Conflict of interest
The authors declare that the research was conducted in the absence of any commercial or financial relationships that could be construed as a potential conflict of interest.
Publisher’s note
All claims expressed in this article are solely those of the authors and do not necessarily represent those of their affiliated organizations, or those of the publisher, the editors and the reviewers. Any product that may be evaluated in this article, or claim that may be made by its manufacturer, is not guaranteed or endorsed by the publisher.
References
1. Herr D, Bekes I, Wulff C. Local renin-angiotensin system in the reproductive system. Front Endocrinol (2013) 4(150):00150. doi: 10.3389/fendo.2013.00150
2. Reis FM, Bouissou DR, Pereira VM, Camargos AF, dos Reis AM, Santos RA. Angiotensin-(1-7), its receptor mas, and the angiotensin-converting enzyme type 2 are expressed in the human ovary. Fertil Steril (2011) 95(1):176–81. doi: 10.1016/j.fertnstert.2010.06.060
3. Mahon JM, Carr RD, Nicol AK, Henderson IW. Angiotensin(1-7) is an antagonist at the type 1 angiotensin II receptor. J Hypertens (1994) 12(12):1377–81. doi: 10.1097/00004872-199412000-00010
4. Henarejos-Castillo I, Sebastian-Leon P, Devesa-Peiro A, Pellicer A, Diaz-Gimeno P. SARS-CoV-2 infection risk assessment in the endometrium: viral infection-related gene expression across the menstrual cycle. Fertil Steril (2020) 114(2):223–32. doi: 10.1016/j.fertnstert.2020.06.026
5. Shabanian S, Khazaie M, Ferns GA, Arjmand MH. Local renin-angiotensin system molecular mechanisms in intrauterine adhesions formation following gynecological operations, new strategy for novel treatment. J Obstet Gynaecol (2022) 42(6):1613–8. doi: 10.1080/01443615.2022.2036972
6. Domińska K. Involvement of ACE2/Ang-(1-7)/MAS1 axis in the regulation of ovarian function in mammals. Int J Mol Sci (2020) 21(13):4572. doi: 10.3390/ijms21134572
7. Peach MJ. Renin-angiotensin system: biochemistry and mechanisms of action. Physiol Rev (1977) 57(2):313–70. doi: 10.1152/physrev.1977.57.2.313
8. Hersh EV, Wolff M, Moore PA, Theken KN, Daniell H. A pair of "ACEs". J Dent Res (2022) 101(1):5–10. doi: 10.1177/00220345211047510
9. Rice GI, Thomas DA, Grant PJ, Turner AJ, Hooper NM. Evaluation of angiotensin-converting enzyme (ACE), its homologue ACE2 and neprilysin in angiotensin peptide metabolism. Biochem J (2004) 383(Pt 1):45–51. doi: 10.1042/BJ20040634
10. Santos RAS, Sampaio WO, Alzamora AC, Motta-Santos D, Alenina N, Bader M, et al, et al. The ACE2/Angiotensin-(1-7)/MAS axis of the renin-angiotensin system: Focus on angiotensin-(1-7). Physiol Rev (2018) 98(1):505–53. doi: 10.1152/physrev.00023.2016
11. Vickers C, Hales P, Kaushik V, Dick L, Gavin J, Tang J, et al. Hydrolysis of biological peptides by human angiotensin-converting enzyme-related carboxypeptidase. J Biol Chem (2002) 277(17):14838–43. doi: 10.1074/jbc.M200581200
12. Brosnihan KB, Neves LA, Anton L, Joyner J, Valdes G, Merrill DC. Enhanced expression of ang-(1-7) during pregnancy. Braz J Med Biol Res (2004) 37(8):1255–62. doi: 10.1590/S0100-879X2004000800017
13. Teixeira LB, Parreiras-E-Silva LT, Bruder-Nascimento T, Duarte DA, Simões SC, Costa RM, et al. Ang-(1-7) is an endogenous β-arrestin-biased agonist of the AT(1) receptor with protective action in cardiac hypertrophy. Sci Rep (2017) 7(1):017–12074. doi: 10.1038/s41598-017-12074-3
14. Bosnyak S, Jones ES, Christopoulos A, Aguilar MI, Thomas WG, Widdop RE. Relative affinity of angiotensin peptides and novel ligands at AT1 and AT2 receptors. Clin Sci (2011) 121(7):297–303. doi: 10.1042/CS20110036
15. Gironacci MM, Adamo HP, Corradi G, Santos RA, Ortiz P, Carretero OA. Angiotensin (1-7) induces MAS receptor internalization. Hypertension (2011) 58(2):176–81. doi: 10.1161/HYPERTENSIONAHA.111.173344
16. Ferrario CM, Groban L, Wang H, Sun X, VonCannon JL, Wright KN, et al. The renin-angiotensin system biomolecular cascade: a 2022 update of newer insights and concepts. Kidney Int Suppl (2011) 12(1):36–47. doi: 10.1016/j.kisu.2021.11.002
17. Pountain SJ, Pipkin FB, Hunter MG. The ontogeny of components of the renin-angiotensin system in the porcine fetal ovary. Anim Reprod Sci (2010) 117(1-2):119–26. doi: 10.1016/j.anireprosci.2009.03.006
18. Cavallo IK, Dela Cruz C, Oliveira ML, Del Puerto HL, Dias JA, Lobach VN, et al. Angiotensin-(1-7) in human follicular fluid correlates with oocyte maturation. Hum Reprod (2017) 32(6):1318–24. doi: 10.1093/humrep/dex072
19. Yoshimura T, Ito M, Matsui K, Okamura H. Effects of highly purified eicosapentaenoic acid on vascular reactivity to angiotensin II and norepinephrine in pregnant rabbits. Artery (1997) 22(5):242–50.
20. Ferreira R, Gasperin B, Santos J, Rovani M, Santos RA, Gutierrez K, et al. Angiotensin II profile and mRNA encoding RAS proteins during bovine follicular wave. J Renin Angiotensin Aldosterone Syst (2011) 12(4):475–82. doi: 10.1177/1470320311403786
21. Giometti IC, Bertagnolli AC, Ornes RC, da Costa LF, Carambula SF, Reis AM, et al. Angiotensin II reverses the inhibitory action produced by theca cells on bovine oocyte nuclear maturation. Theriogenology (2005) 63(4):1014–25. doi: 10.1016/j.theriogenology.2004.05.022
22. Erman A, Chen-Gal B, van Dijk DJ, Sulkes J, Kaplan B, Boner G, et al. Ovarian angiotensin-converting enzyme activity in humans: relationship to estradiol, age, and uterine pathology. J Clin Endocrinol Metab (1996) 81(3):1104–7. doi: 10.1210/jcem.81.3.8772583
23. Li YH, Jiao LH, Liu RH, Chen XL, Wang H, Wang WH.. Localization of angiotensin II in pig ovary and its effects on oocyte maturation in vitro. Theriogenology (2004) 61(2-3):447–59. doi: 10.1016/S0093-691X(03)00246-2
24. Obermüller N, Gentili M, Gauer S, Gretz N, Weigel M, Geiger H, et al. Immunohistochemical and mRNA localization of the angiotensin II receptor subtype 2 (AT2) in follicular granulosa cells of the rat ovary. J Histochem Cytochem (2004) 52(4):545–8. doi: 10.1177/002215540405200413
25. Tanaka M, Ohnishi J, Ozawa Y, Sugimoto M, Usuki S, Naruse M, et al. Characterization of angiotensin II receptor type 2 during differentiation and apoptosis of rat ovarian cultured granulosa cells. Biochem Biophys Res Commun (1995) 207(2):593–8. doi: 10.1006/bbrc.1995.1229
26. Kotani E, Sugimoto M, Kamata H, Fujii N, Saitoh M, Usuki S, et al. Biological roles of angiotensin II via its type 2 receptor during rat follicle atresia. Am J Physiol (1999) 276(1):E25–33. doi: 10.1152/ajpendo.1999.276.1.E25
27. Peña O, Palumbo A, González-Fernández R, Hernández J, Naftolin F, Avila J. Expression of angiotensin II type 1 (AT1) and angiotensin II type 2 (AT2) receptors in human granulosa-lutein (GL) cells: correlation with infertility diagnoses. Fertil Steril (2010) 93(5):1601–8. doi: 10.1016/j.fertnstert.2009.03.092
28. Kalenga MK, de Gasparo M, de Hertogh R, Whitebread S, Vankrieken L, Thomas K, et al. [Angiotensin II receptors in the human placenta are type AT1]. Reprod Nutr Dev (1991) 31(3):257–67.
29. Lumbers ER, Wang Y, Delforce SJ, Corbisier de Meaultsart C, Logan PC, Mitchell MD, et al. Decidualisation of human endometrial stromal cells is associated with increased expression and secretion of prorenin. Reprod Biol Endocrinol (2015) 13(129):015–0127. doi: 10.1186/s12958-015-0127-8
30. Ahmed A, Li XF, Shams M, Gregory J, Rollason T, Barnes NM, et al. Localization of the angiotensin II and its receptor subtype expression in human endometrium and identification of a novel high-affinity angiotensin II binding site. J Clin Invest (1995) 96(2):848–57. doi: 10.1172/JCI118131
31. Pringle KG, Delforce SJ, Wang Y, Ashton KA, Proietto A, Otton G, et al. Renin-angiotensin system gene polymorphisms and endometrial cancer. Endocr Connect (2016) 5(3):128–35. doi: 10.1530/EC-15-0112
32. Rosenfeld CR, DeSpain K, Word RA, Liu XT. Differential sensitivity to angiotensin II and norepinephrine in human uterine arteries. J Clin Endocrinol Metab (2012) 97(1):138–47. doi: 10.1210/jc.2011-1818
33. Gan L, Liu D, Liu J, Chen E, Chen C, Liu L, et al. CD38 deficiency alleviates ang II-induced vascular remodeling by inhibiting small extracellular vesicle-mediated vascular smooth muscle cell senescence in mice. Signal Transduct Target Ther (2021) 6(1):021–00625. doi: 10.1038/s41392-021-00625-0
34. Henriet P, Gaide Chevronnay HP, Marbaix E. The endocrine and paracrine control of menstruation. Mol Cell Endocrinol (2012) 358(2):197–207. doi: 10.1016/j.mce.2011.07.042
35. Neves LA, Stovall K, Joyner J, Valdés G, Gallagher PE, Ferrario CM, et al. ACE2 and ANG-(1-7) in the rat uterus during early and late gestation. Am J Physiol Regul Integr Comp Physiol (2008) 294(1):31.
36. Vaz-Silva J, Tavares RL, Ferreira MC, Honorato-Sampaio K, Cavallo IK, Santos RA, et al. Tissue specific localization of angiotensin-(1-7) and its receptor mas in the uterus of ovariectomized rats. J Mol Histol (2012) 43(5):597–602. doi: 10.1007/s10735-012-9427-x
37. Shan T, Zhang L, Zhao C, Chen W, Zhang YA. Angiotensin-(1-7) and angiotensin II induce the transdifferentiation of human endometrial epithelial cells in vitro. Mol Med Rep (2014) 9(6):2180–6. doi: 10.3892/mmr.2014.2128
38. Shan T, Shang W, Zhang L, Zhao C, Chen W, Zhang Y, et al. Effect of angiotensin-(1-7) and angiotensin II on the proliferation and activation of human endometrial stromal cells in vitro. Int J Clin Exp Pathol (2015) 8(8):8948–57.
39. Zimmerman D, Burns KD. Angiotensin-(1-7) in kidney disease: a review of the controversies. Clin Sci (2012) 123(6):333–46. doi: 10.1042/CS20120111
40. Lauer D, Slavic S, Sommerfeld M, Thöne-Reineke C, Sharkovska Y, Hallberg A, et al. Angiotensin type 2 receptor stimulation ameliorates left ventricular fibrosis and dysfunction via regulation of tissue inhibitor of matrix metalloproteinase 1/matrix metalloproteinase 9 axis and transforming growth factor β1 in the rat heart. Hypertension (2014) 63(3):30. doi: 10.1161/HYPERTENSIONAHA.113.02522
41. Maltepe E, Fisher SJ. Placenta: the forgotten organ. Annu Rev Cell Dev Biol (2015) 31:523–52. doi: 10.1146/annurev-cellbio-100814-125620
42. Thapa L, He CM, Chen HP. Study on the expression of angiotensin II (ANG II) receptor subtype 1 (AT1R) in the placenta of pregnancy-induced hypertension. Placenta (2004) 25(7):637–41. doi: 10.1016/j.placenta.2004.01.026
43. Lumbers ER, Pringle KG. Roles of the circulating renin-angiotensin-aldosterone system in human pregnancy. Am J Physiol Regul Integr Comp Physiol (2014) 306(2):2. doi: 10.1152/ajpregu.00034.2013
44. Hagemann A, Nielsen AH, Poulsen K. The uteroplacental renin-angiotensin system: a review. Exp Clin Endocrinol (1994) 102(3):252–61. doi: 10.1055/s-0029-1211289
45. Leal CRV, Costa LB, Ferreira GC, Ferreira AM, Reis FM, Simões E, et al. Renin-angiotensin system in normal pregnancy and in preeclampsia: A comprehensive review. Pregnancy Hypertens (2022) 28:15–20. doi: 10.1016/j.preghy.2022.01.011
46. Pan P, Fu H, Zhang L, Huang H, Luo F, Wu W, et al. Angiotensin II upregulates the expression of placental growth factor in human vascular endothelial cells and smooth muscle cells. BMC Cell Biol (2010) 11(36):1471–2121. doi: 10.1186/1471-2121-11-36
47. Delforce SJ, Lumbers ER, Morosin SK, Wang Y, Pringle KG. The angiotensin II type 1 receptor mediates the effects of low oxygen on early placental angiogenesis. Placenta (2019) 75:54–61. doi: 10.1016/j.placenta.2018.12.001
48. Anton L, Merrill DC, Neves LA, Gruver C, Moorefield C, Brosnihan KB. Angiotensin II and angiotensin-(1-7) decrease sFlt1 release in normal but not preeclamptic chorionic villi: an in vitro study. Reprod Biol Endocrinol (2010) 8(135):1477–7827. doi: 10.1186/1477-7827-8-135
49. Espino YSS, Martinez-Portilla RJ, Torres-Torres J, Solis-Paredes JM, Estrada-Gutierrez G, Hernandez-Pacheco JA, et al. Novel ratio soluble fms-like tyrosine kinase-1/Angiotensin-II (sFlt-1/ANG-II) in pregnant women is associated with critical illness in COVID-19. Viruses (2021) 13(10):1906. doi: 10.3390/v13101906
50. Oogaki Y, Ozawa R, Seshima K, Shinoda R, Torii Y, Takahashi H, et al. Uncaria tomentosa extract (AC-11) improves pregnancy hypertension together with suppression of sFlt-1 and sEng. Pregnancy Hypertens (2021) 26:127–32. doi: 10.1016/j.preghy.2021.10.013
51. Zhou CC, Ahmad S, Mi T, Xia L, Abbasi S, Hewett PW, et al. Angiotensin II induces soluble fms-like tyrosine kinase-1 release via calcineurin signaling pathway in pregnancy. Circ Res (2007) 100(1):88–95. doi: 10.1161/01.RES.0000254703.11154.18
52. Espinoza J, Betancourt A, Belfort MA, Shamshirsaz AA, Fox KA, Yallampalli C, et al. Placental growth factor blunts uterine artery responses to angiotensin II. Bjog (2019) 126(8):1058–64. doi: 10.1111/1471-0528.15656
53. Hering L, Herse F, Geusens N, Verlohren S, Wenzel K, Staff AC, et al. Effects of circulating and local uteroplacental angiotensin II in rat pregnancy. Hypertension (2010) 56(2):311–8. doi: 10.1161/HYPERTENSIONAHA.110.150961
54. Tamanna S, Lumbers ER, Morosin SK, Delforce SJ, Pringle KG. ACE2: a key modulator of the renin-angiotensin system and pregnancy. Am J Physiol Regul Integr Comp Physiol (2021) 321(6):R833–43. doi: 10.1152/ajpregu.00211.2021
55. Tamanna S, Morosin SK, Delforce SJ, van Helden DF, Lumbers ER, Pringle KG, et al. Renin-angiotensin system (RAS) enzymes and placental trophoblast syncytialisation. Mol Cell Endocrinol (2022) 547(111609):22. doi: 10.1016/j.mce.2022.111609
56. Schmid M, Sollwedel A, Thuere C, Wafula PO, Zenclussen ML, Müller DN, et al. Murine pre-eclampsia induced by unspecific activation of the immune system correlates with alterations in the eNOS and AT1 receptor expression in the kidneys and placenta. Placenta (2007) 28(7):688–700. doi: 10.1016/j.placenta.2006.10.008
57. Cornelius DC, Amaral LM, Harmon A, Wallace K, Thomas AJ, Campbell N, et al. An increased population of regulatory T cells improves the pathophysiology of placental ischemia in a rat model of preeclampsia. Am J Physiol Regul Integr Comp Physiol (2015) 309(8):19. doi: 10.1152/ajpregu.00154.2015
58. Blumenfeld Z. The ovarian hyperstimulation syndrome. Vitam Horm (2018) 107:423–51. doi: 10.1016/bs.vh.2018.01.018
59. Manno M, Tomei F. Renin-angiotensin system activation during severe OHSS: cause or effect? Fertil Steril (2008) 89(2):488. doi: 10.1016/j.fertnstert.2007.12.003
60. Navot D, Margalioth EJ, Laufer N, Birkenfeld A, Relou A, Rosler A, et al. Direct correlation between plasma renin activity and severity of the ovarian hyperstimulation syndrome. Fertil Steril (1987) 48(1):57–61. doi: 10.1016/S0015-0282(16)59290-5
61. Delbaere A, Bergmann PJ, Gervy-Decoster C, Camus M, de Maertelaer V, Englert Y. Prorenin and active renin concentrations in plasma and ascites during severe ovarian hyperstimulation syndrome. Hum Reprod (1997) 12(2):236–40. doi: 10.1093/humrep/12.2.236
62. Ong AC, Eisen V, Rennie DP, Homburg R, Lachelin GC, Jacobs HS, et al. The pathogenesis of the ovarian hyperstimulation syndrome (OHS): a possible role for ovarian renin. Clin Endocrinol (1991) 34(1):43–9. doi: 10.1111/j.1365-2265.1991.tb01734.x
63. Delbaere A, Bergmann PJ, Englert Y. Features of the renin-angiotensin system in ascites and pleural effusion during severe ovarian hyperstimulation syndrome. J Assist Reprod Genet (1997) 14(5):241–4. doi: 10.1007/BF02765823
64. Morris RS, et al. Inhibition of ovarian-derived prorenin to angiotensin cascade in the treatment of ovarian hyperstimulation syndrome. Hum Reprod (1995) 10(6):1355–8. doi: 10.1093/HUMREP/10.6.1355
65. Sahin Y, et al. Effects of angiotensin converting enzyme inhibitor cilazapril and angiotensin II antagonist saralasin in ovarian hyperstimulation syndrome in the rabbit. Gynecol Endocrinol (1997) 11(4):231–6. doi: 10.3109/09513599709152539
66. Ando H, et al. Dual renin-angiotensin blockade therapy in patients at high risk of early ovarian hyperstimulation syndrome receiving IVF and elective embryo cryopreservation: a case series. Hum Reprod (2003) 18(6):1219–22. doi: 10.1093/humrep/deg268
67. Ata B, et al. Dual renin-angiotensin blockage and total embryo cryopreservation is not a risk-free strategy in patients at high risk for ovarian hyperstimulation syndrome. Fertil Steril (2008) 90(3):531–6. doi: 10.1016/j.fertnstert.2007.07.1309
68. Ndefo UA, Eaton A, Green MR. Polycystic ovary syndrome: a review of treatment options with a focus on pharmacological approaches. P T (2013) 38(6):336–55.
69. Jaatinen TA, Matinlauri I, Anttila L, Koskinen P, Erkkola R, Irjala K. Serum total renin is elevated in women with polycystic ovarian syndrome. Fertil Steril (1995) 63(5):1000–4. doi: 10.1016/S0015-0282(16)57537-2
70. Alphan Z, Berberoglu Z, Gorar S, Candan Z, Aktas A, Aral Y, et al. Increased total renin levels but not angiotensin-converting enzyme activity in obese patients with polycystic ovary syndrome. Med Princ Pract (2013) 22(5):475–9. doi: 10.1159/000351572
71. Arefi S, Mottaghi S, Sharifi AM. Studying the correlation of renin-angiotensin-system (RAS) components and insulin resistance in polycystic ovary syndrome (PCOs). Gynecol Endocrinol (2013) 29(5):470–3. doi: 10.3109/09513590.2013.769513
72. Pereira VM, Reis FM, Casalechi M, Reis AM. Angiotensin-(1-7), angiotensin-converting enzyme 2 and mas receptor in rat polycystic ovaries. Protein Pept Lett (2021) 28(3):249–54. doi: 10.2174/0929866527666200826104410
73. Qin S, Zhou YJ, Liu Y, Shen HM, Li XD, Yan X. [Expression and significance of ACE2-Ang-(1-7)-Mas axis in the endometrium of patients with polycystic ovary syndrome]. Zhonghua Yi Xue Za Zhi (2013) 93(25):1989–92.
74. Underwood PC, Adler GK. The renin angiotensin aldosterone system and insulin resistance in humans. Curr Hypertens Rep (2013) 15(1):59–70. doi: 10.1007/s11906-012-0323-2
75. Zhao S, Sun W, Jiang P. Role of the ACE2/Ang-(1-7)/Mas axis in glucose metabolism. Rev Cardiovasc Med (2021) 22(3):769–77. doi: 10.31083/j.rcm2203083
76. Souza-Mello V. Hepatic structural enhancement and insulin resistance amelioration due to AT1 receptor blockade. World J Hepatol (2017) 9(2):74–9. doi: 10.4254/wjh.v9.i2.74
77. Hacihanefioglu B, Somunkiran A, Mahmutoglu I, Sercelik A, Toptani S, Kervancioglu E. Effect of hypertension therapy with the angiotensin-converting enzyme inhibitor lisinopril on hyperandrogenism in women with polycystic ovary syndrome. Fertil Steril (2002) 77(3):526–8. doi: 10.1016/S0015-0282(01)03210-1
78. Deepika ML, Reddy KR, Rani VU, Balakrishna N, Latha KP, Jahan P, et al. Do ACE I/D gene polymorphism serve as a predictive marker for age at onset in PCOS? J Assist Reprod Genet (2013) 30(1):125–30. doi: 10.1007/s10815-012-9906-8
79. Karabulut A, Turgut S, Turgut G. Angiotensin converting enzyme gene insertion/deletion polymorphism in patients with polycystic ovary syndrome. Gynecol Endocrinol (2010) 26(6):393–8. doi: 10.3109/09513591003632167
80. Ożegowska K, Bogacz A, Bartkowiak-Wieczorek J, Seremak-Mrozikiewicz A, Pawelczyk L. Association between the angiotensin converting enzyme gene insertion/deletion polymorphism and metabolic disturbances in women with polycystic ovary syndrome. Mol Med Rep (2016) 14(6):5401–7. doi: 10.3892/mmr.2016.5910
81. Ma WD, Fan P, Liu HW, Liu QQ, Hu KF, Bai H, et al. [Clinical study of the impact of ACE I/D gene variation on the clinical parameters of patients with polycystic ovary syndrome]. Sichuan Da Xue Xue Bao Yi Xue Ban (2021) 52(5):877–82. doi: 10.12182/20210960207
82. Sun J, Fan H, Che Y, Cao Y, Wu X, Sun HX, et al. Association between ACE gene I/D polymorphisms and hyperandrogenism in women with polycystic ovary syndrome (PCOS) and controls. BMC Med Genet (2009) 10(64):1471–2350. doi: 10.1186/1471-2350-10-64
83. Beyazit F, Ayhan S, Celik HT, Gungor T. Assessment of serum angiotensin-converting enzyme in patients with epithelial ovarian cancer. Arch Gynecol Obstet (2015) 292(2):415–20. doi: 10.1007/s00404-015-3661-x
84. Suganuma T, Ino K, Shibata K, Kajiyama H, Nagasaka T, Mizutani S, et al. Functional expression of the angiotensin II type 1 receptor in human ovarian carcinoma cells and its blockade therapy resulting in suppression of tumor invasion, angiogenesis, and peritoneal dissemination. Clin Cancer Res (2005) 11(7):2686–94. doi: 10.1158/1078-0432.CCR-04-1946
85. Cotter TP, Kealy NP, Duggan PF, Curtain A, Bredin CP. Elevated serum angiotensin converting enzyme levels in metastatic ovarian dysgerminoma. Respir Med (1997) 91(4):237–9. doi: 10.1016/S0954-6111(97)90045-7
86. Zhang Q, Yu S, Lam MMT, Poon TCW, Sun L, Jiao Y, et al. Angiotensin II promotes ovarian cancer spheroid formation and metastasis by upregulation of lipid desaturation and suppression of endoplasmic reticulum stress. J Exp Clin Cancer Res (2019) 38(1):019–1127. doi: 10.1186/s13046-019-1127-x
87. Song L, Zhang SL, Bai KH, Yang J, Xiong HY, Li X, et al. Serum agonistic autoantibodies against type-1 angiotensin II receptor titer in patients with epithelial ovarian cancer: a potential role in tumor cell migration and angiogenesis. J Ovarian Res (2013) 6(1):1757–2215. doi: 10.1186/1757-2215-6-22
88. Ino K, Shibata K, Kajiyama H, Yamamoto E, Nagasaka T, Nawa A, et al. Angiotensin II type 1 receptor expression in ovarian cancer and its correlation with tumour angiogenesis and patient survival. Br J Cancer (2006) 94(4):552–60. doi: 10.1038/sj.bjc.6602961
89. Domińska K, Urbanek KA, Kowalska K, Habrowska-Górczyńska DE, Kozieł MJ, Ochędalski T, et al. The influence of angiotensin peptides on survival and motility of human high-grade serous ovarian cancer cells in serum starvation conditions. Int J Mol Sci (2021) 23(1):52. doi: 10.3390/ijms23010052
90. Park YA, Choi CH, Do IG, Song SY, Lee JK, Cho YJ, et al. Dual targeting of angiotensin receptors (AGTR1 and AGTR2) in epithelial ovarian carcinoma. Gynecol Oncol (2014) 135(1):108–17. doi: 10.1016/j.ygyno.2014.06.031
91. Saunders PTK, Horne AW. Endometriosis: Etiology, pathobiology, and therapeutic prospects. Cell (2021) 184(11):2807–24. doi: 10.1016/j.cell.2021.04.041
92. Nakajima T, Chishima F, Nakao T, Hayashi C, Kasuga A, Shinya K, et al. The expression of MAS1, an angiotensin (1-7) receptor, in the eutopic proliferative endometria of endometriosis patients. Gynecol Obstet Invest (2018) 83(6):600–7. doi: 10.1159/000490561
93. Hsieh YY, Chang CC, Chen SY, Chen CP, Lin WH, Tsai FJ, et al. XRCC1 399 arg-related genotype and allele, but not XRCC1 His107Arg, XRCC1 Trp194Arg, KCNQ2, AT1R, and hOGG1 polymorphisms, are associated with higher susceptibility of endometriosis. Gynecol Endocrinol (2012) 28(4):305–9. doi: 10.3109/09513590.2011.631624
94. Nenicu A, Körbel C, Gu Y, Menger MD, Laschke MW. Combined blockade of angiotensin II type 1 receptor and activation of peroxisome proliferator-activated receptor-γ by telmisartan effectively inhibits vascularization and growth of murine endometriosis-like lesions. Hum Reprod (2014) 29(5):1011–24. doi: 10.1093/humrep/deu035
95. Chen ZZ, Gong X. Tanshinone IIA contributes to the pathogenesis of endometriosis via renin angiotensin system by regulating the dorsal root ganglion axon sprouting. Life Sci (2020) 240(117085):20. doi: 10.1016/j.lfs.2019.117085
96. Kowalczyńska LJ, Ferenc T, Wojciechowski M, Mordalska A, Pogoda K, Malinowski A. Endometriosis and RAS system gene polymorphisms: the association of ACE A2350G polymorphism with endometriosis in polish individuals. DNA Cell Biol (2014) 33(5):328–35. doi: 10.1089/dna.2013.2255
97. Hsieh YY, Chang CC, Tsai FJ, Hsu CM, Lin CC, Tsai CH. Angiotensin I-converting enzyme ACE 2350*G and ACE-240*T-related genotypes and alleles are associated with higher susceptibility to endometriosis. Mol Hum Reprod (2005) 11(1):11–4. doi: 10.1093/molehr/gah131
98. Hsieh YY, Lee CC, Chang CC, Wang YK, Yeh LS, Lin CS. Angiotensin I-converting enzyme insertion-related genotypes and allele are associated with higher susceptibility of endometriosis and leiomyoma. Mol Reprod Dev (2007) 74(7):808–14. doi: 10.1002/mrd.20474
99. Trovó de Marqui AB. Genetic polymorphisms and endometriosis: contribution of genes that regulate vascular function and tissue remodeling. Rev Assoc Med Bras (1992) 58(5):620–32. doi: 10.1590/s0104-42302012000500022
100. Kowalczyńska LJ, Tomasz F, Wojciechowski M, Mordalska A, Pogoda K, Malinowski A. ACE I/D polymorphism in polish patients with endometriosis. Ginekol Pol (2011) 82(2):102–7.
101. Lamp M, Saare M, Laisk T, Karro H, Kadastik U, Metspalu A, et al. Genetic variations in vascular endothelial growth factor but not in angiotensin I-converting enzyme genes are associated with endometriosis in Estonian women. Eur J Obstet Gynecol Reprod Biol (2010) 153(1):85–9. doi: 10.1016/j.ejogrb.2010.07.021
102. Amant F, Moerman P, Neven P, Timmerman D, Van Limbergen E, Vergote I. Endometrial cancer. Lancet (2005) 366(9484):491–505. doi: 10.1016/S0140-6736(05)67063-8
103. Delforce SJ, Lumbers ER, Corbisier de Meaultsart C, Wang Y, Proietto A, Otton G, et al. Expression of renin-angiotensin system (RAS) components in endometrial cancer. Endocr Connect (2017) 6(1):9–19. doi: 10.1530/EC-16-0082
104. Nowakowska M, Matysiak-Burzyńska Z, Kowalska K, Płuciennik E, Domińska K, Piastowska-Ciesielska AW, et al. Angiotensin II promotes endometrial cancer cell survival. Oncol Rep (2016) 36(2):1101–10. doi: 10.3892/or.2016.4887
105. Matysiak-Burzyńska ZE, Nowakowska M, Domińska K, Kowalska K, Płuciennik E, Piastowska-Ciesielska AW. Silencing of angiotensin receptor 1 interferes with angiotensin II oncogenic activity in endometrial cancer. J Cell Biochem (2018) 119(11):9110–21. doi: 10.1002/jcb.27174
106. Watanabe Y, Shibata K, Kikkawa F, Kajiyama H, Ino K, Hattori A, et al. Adipocyte-derived leucine aminopeptidase suppresses angiogenesis in human endometrial carcinoma via renin-angiotensin system. Clin Cancer Res (2003) 9(17):6497–503.
107. Correa-Noronha SA, Noronha SM, Alecrim C, Mesquita Ade C, Brito GS, Junqueira MG, et al. Association of angiotensin-converting enzyme I gene I/D polymorphism with endometrial but not with ovarian cancer. Gynecol Endocrinol (2012) 28(11):889–91. doi: 10.3109/09513590.2012.683060
108. Freitas-Silva M, Pereira D, Coelho C, Bicho M, Lopes C, Medeiros R. Angiotensin I-converting enzyme gene insertion/deletion polymorphism and endometrial human cancer in normotensive and hypertensive women. Cancer Genet Cytogenet (2004) 155(1):42–6. doi: 10.1016/j.cancergencyto.2004.03.020
109. Jung E, Romero R, Yeo L, Gomez-Lopez N, Chaemsaithong P, Jaovisidha A, et al. The etiology of preeclampsia. Am J Obstet Gynecol (2022) 226(2S):S844–66. doi: 10.1016/j.ajog.2021.11.1356
110. Roberts JM, Rich-Edwards JW, McElrath TF, Garmire L, Myatt L, Global Pregnancy Collaboration. Subtypes of preeclampsia: Recognition and determining clinical usefulness. Hypertension (2021) 77(5):1430–41. doi: 10.1161/HYPERTENSIONAHA.120.14781
111. Overton E, Tobes D, Lee A. Preeclampsia diagnosis and management. Best Pract Res Clin Anaesthesiol (2022) 36(1):107–21. doi: 10.1016/j.bpa.2022.02.003
112. Chappell LC, Cluver CA, Kingdom J, Tong S. Pre-eclampsia. Lancet (2021) 398(10297):341–54. doi: 10.1016/S0140-6736(20)32335-7
113. van der Graaf AM, Toering TJ, van der Wiel MWK, Frenay AS, Wallukat G, Dechend R, et al. Angiotensin II responsiveness after preeclampsia: translational data from an experimental rat model and early-onset human preeclampsia. J Hypertens (2017) 35(12):2468–78. doi: 10.1097/HJH.0000000000001474
114. LaMarca B, Wallace K, Granger J. Role of angiotensin II type I receptor agonistic autoantibodies (AT1-AA) in preeclampsia. Curr Opin Pharmacol (2011) 11(2):175–9. doi: 10.1016/j.coph.2011.01.003
115. Sahay AS, Patil VV, Sundrani DP, Joshi AA, Wagh GN, Gupte SA, et al. A longitudinal study of circulating angiogenic and antiangiogenic factors and AT1-AA levels in preeclampsia. Hypertens Res (2014) 37(8):753–8. doi: 10.1038/hr.2014.71
116. Cunningham MW Jr., Castillo J, Ibrahim T, Cornelius DC, Campbell N, Amaral L, et al. AT1-AA (Angiotensin II type 1 receptor agonistic autoantibody) blockade prevents preeclamptic symptoms in placental ischemic rats. Hypertension (2018) 71(5):886–93. doi: 10.1161/HYPERTENSIONAHA.117.10681
117. Menkhorst E, Zhou W, Santos L, Zhang JG, St-Pierre Y, Young MJ, et al. Galectin-7 dysregulates renin-angiotensin-aldosterone and NADPH oxide synthase pathways in preeclampsia. Pregnancy Hypertens (2022) 30:130–6. doi: 10.1016/j.preghy.2022.09.008
118. Bodova KB, Biringer K, Dokus K, Ivankova J, Stasko J, Danko J. Fibronectin, plasminogen activator inhibitor type 1 (PAI-1) and uterine artery Doppler velocimetry as markers of preeclampsia. Dis Markers (2011) 30(4):191–6. doi: 10.1155/2011/219350
119. Zhang Y, Liang B, Meng F, Li H. Effects of nrf-2 expression in trophoblast cells and vascular endothelial cells in preeclampsia. Am J Transl Res (2021) 13(3):1006–21.
120. Parchem JG, Kanasaki K, Lee SB, Kanasaki M, Yang JL, Xu Y, et al. STOX1 deficiency is associated with renin-mediated gestational hypertension and placental defects. JCI Insight (2021) 6(2):141588. doi: 10.1172/jci.insight.141588
121. van Dijk M, Oudejans CB. STOX1: Key player in trophoblast dysfunction underlying early onset preeclampsia with growth retardation. J Pregnancy (2011) 521826(10):15. doi: 10.1155/2011/521826
122. Merrill DC, Karoly M, Chen K, Ferrario CM, Brosnihan KB. Angiotensin-(1-7) in normal and preeclamptic pregnancy. Endocrine (2002) 18(3):239–45. doi: 10.1385/ENDO:18:3:239
123. Wang C, Zhou X, Liu H, Huang S. Three polymorphisms of renin-angiotensin system and preeclampsia risk. J Assist Reprod Genet (2020) 37(12):3121–42. doi: 10.1007/s10815-020-01971-8
124. Yüce M, Filiztekin E, Özkaya KG. COVID-19 diagnosis -a review of current methods. Biosens Bioelectron (2021) 172:112752. doi: 10.1016/j.bios.2020.112752
125. Chen F, Zhu S, Dai Z, Hao L, Luan C, Guo Q, et al. Effects of COVID-19 and mRNA vaccines on human fertility. Hum Reprod (2021) 37(1):5–13. doi: 10.1093/humrep/deab238
126. Jackson CB, Farzan M, Chen B, Choe H. Mechanisms of SARS-CoV-2 entry into cells. Nat Rev Mol Cell Biol (2022) 23(1):3–20. doi: 10.1038/s41580-021-00418-x
127. Madendag IC, Madendag Y, Ozdemir AT. COVID-19 disease does not cause ovarian injury in women of reproductive age: an observational before-and-after COVID-19 study. Reprod BioMed Online (2022) 45(1):153–8. doi: 10.1016/j.rbmo.2022.03.002
128. Kolanska K, Hours A, Jonquière L, Mathieu d'Argent E, Dabi Y, Dupont C, et al. Mild COVID-19 infection does not alter the ovarian reserve in women treated with ART. Reprod BioMed Online (2021) 43(6):1117–21. doi: 10.1016/j.rbmo.2021.09.001
129. Bentov Y, Beharier O, Moav-Zafrir A, Kabessa M, Godin M, Greenfield CS, et al. Ovarian follicular function is not altered by SARS-CoV-2 infection or BNT162b2 mRNA COVID-19 vaccination. Hum Reprod (2021) 36(9):2506–13. doi: 10.1093/humrep/deab182
130. Chadchan SB, Popli P, Maurya VK, Kommagani R. The SARS-CoV-2 receptor, angiotensin-converting enzyme 2, is required for human endometrial stromal cell decidualization†. Biol Reprod (2021) 104(2):336–43. doi: 10.1093/biolre/ioaa211
131. Kala S, Dunk C, Acosta S, Serghides L. Periconceptional exposure to lopinavir, but not darunavir, impairs decidualization: a potential mechanism leading to poor birth outcomes in HIV-positive pregnancies. Hum Reprod (2020) 35(8):1781–96. doi: 10.1093/humrep/deaa151
132. Abhari S, Kawwass JF. Endometrial susceptibility to SARS CoV-2: explained by gene expression across the menstrual cycle? Fertil Steril (2020) 114(2):255–6. doi: 10.1016/j.fertnstert.2020.06.046
133. Aghaamoo S, Ghods K, Rahmanian M. Pregnant women with COVID-19: the placental involvement and consequences. J Mol Histol (2021) 52(3):427–35. doi: 10.1007/s10735-021-09970-4
134. Arthurs AL, Jankovic-Karasoulos T, Roberts CT. COVID-19 in pregnancy: What we know from the first year of the pandemic. Biochim Biophys Acta Mol Basis Dis (2021) 1867(12):166248. doi: 10.1016/j.bbadis.2021.166248
135. Komine-Aizawa S, Takada K, Hayakawa S. Placental barrier against COVID-19. Placenta (2020) 99:45–9. doi: 10.1016/j.placenta.2020.07.022
136. Geslot A, Chanson P, Caron P. Covid-19, the thyroid and the pituitary - the real state of play. Ann Endocrinol (Paris) (2022) 83(2):103–8. doi: 10.1016/j.ando.2021.12.004
Keywords: Angiotensin II, Angiotensin-(1-7), Renin-Angiotensin System, ovary, endometrial, placenta
Citation: Liu Y, Hao H, Lan T, Jia R, Cao M, Zhou L, Zhao Z and Pan W (2022) Physiological and pathological roles of Ang II and Ang- (1-7) in the female reproductive system. Front. Endocrinol. 13:1080285. doi: 10.3389/fendo.2022.1080285
Received: 26 October 2022; Accepted: 05 December 2022;
Published: 23 December 2022.
Edited by:
Hsun-Ming Chang, China Medical University Hospital, TaiwanReviewed by:
Leandro Ceotto Freitas Lima, Academy of Sciences of the Czech Republic (ASCR), CzechiaKedra Wallace, University of Mississippi Medical Center, United States
Copyright © 2022 Liu, Hao, Lan, Jia, Cao, Zhou, Zhao and Pan. This is an open-access article distributed under the terms of the Creative Commons Attribution License (CC BY). The use, distribution or reproduction in other forums is permitted, provided the original author(s) and the copyright owner(s) are credited and that the original publication in this journal is cited, in accordance with accepted academic practice. No use, distribution or reproduction is permitted which does not comply with these terms.
*Correspondence: Wensen Pan, ZGVlcHJlc3BpcmF0aW9uQDE2My5jb20=; Zhiming Zhao, ZG9jdG9yX3poYW96aGFvQHNpbmEuY29t