- 1Department of Growth and Reproduction, Rigshospitalet, Copenhagen, Denmark
- 2International Center for Research and Research Training in Endocrine Disruption of Male Reproduction and Child Health (EDMaRC), Copenhagen University Hospital - Rigshospitalet, Copenhagen, Denmark
- 3Department of Clinical Medicine, University of Copenhagen, Copenhagen, Denmark
- 4Research Centre for Integrative Physiology and Pharmacology, Institute of Biomedicine, University of Turku, Turku, Finland
- 5Centre for Population Health Research, University of Turku and Turku University Hospital, Turku, Finland
- 6Department of Paediatrics, Turku University Hospital, Turku, Finland
Introduction: Environmental exposure during fetal life may disrupt testicular development. In humans, a limited number of studies have investigated whether these adverse effects persist into adulthood. Using data from a prospective, population-based birth cohort study, The Copenhagen Mother-Child cohort, the objective was to assess if there is an association between fetal exposure to selected phenols and benzophenones and markers of testicular function in adult men.
Methods: Pregnant women were recruited in 1997–2001. Their sons were examined clinically at 18-20 years of age, with focus on adult markers of reproductive function (anogenital distance (AGD), semen quality and reproductive hormones). In total, 101 18–20-year-old men were included, whose mothers during pregnancy had a serum sample drawn and analyzed for bisphenol A (BPA) and seven other simple phenols, as well as six benzophenones. To investigate the association between chemical levels (in tertiles, T1-T3) in relation to markers of reproductive function, univariate and multiple linear regression analyses were performed.
Results: In fully adjusted analyses, increased levels of luteinizing hormone (LH) were observed with higher fetal exposure to BPA (percentage difference (95%CI)) (T2: 12% (-8%,36%) and T3: 33% (10%,62%), compared to T1) and benzophenone-3 (BP-3) (T2: 21% (-2%,49%), T3: 18% (-4%,45%)), while no clear association was seen to total testosterone (TT). Higher levels of BPA and BP-3 were associated with a lower TT/LH ratio, although only significant for BPA (p-trend=0.01). No associations were seen to AGD or markers of semen quality.
Conclusion: In conclusion, high exposure to BPA and BP-3 was associated with a compensated reduced Leydig cell function but no other changes in markers of reproductive health. As maternal levels of BPA and BP-3 were not correlated, separate effects may be at play. Larger studies on long-term reproductive consequences of prenatal exposures are warranted to validate our findings.
1 Introduction
During recent decades there has been an increase in the incidence of several male reproductive disorders and an increased focus on environmental exposures that may explain some of these trends (1). It has also been suggested that cryptorchidism, hypospadias, poor semen quality and testicular germ cell cancer may be associated through a common etiology involving abnormal fetal development of the male gonads (2–4). In modern societies humans are exposed to numerous industrial chemicals, some of which have documented endocrine disrupting abilities in wildlife and animal studies (5). Examples of such endocrine disrupting pollutants with wide-spread use are phenolic substances as well as benzophenones which are characterized as non-persistent or partly non-persistent because of their short half-lives within the body (6). Fetal life is a critical period for normal development of the male reproductive organs and thus exposure of pregnant women to such chemicals is of concern.
Among the phenols is a group of industrial chemicals which primarily are used as precursors for various types of plastics. This includes bisphenol A (BPA), the most commonly used of the phenols, which is mainly used in polycarbonates and epoxy resins used in some food and drink packaging and also in various electronic equipment, and toys (7). Other phenols, like triclosan (TCS), are known for their antibacterial properties and are commonly used in a range of personal care products (8). Humans are ubiquitously exposed to numerous phenols (9, 10). Besides being detectable in human maternal serum, BPA is also detectable in fetal serum and placenta (11). However, BPA levels in umbilical cord blood are half of those in maternal and placental samples, suggesting some protection by the placenta (12). Studies in rodents have shown that pre- or perinatal exposure to BPA is associated with impaired testis function in offspring, i.e., disrupted spermatogenesis and reduced testosterone level (13–15). However, findings in humans from longitudinal pregnancy cohorts are scarce and the majority is characterized by short follow-up. Some, but not all studies have indicated that prenatal exposures to either BPA or TCS are associated with reduced anogenital distance (AGD), a marker of prenatal androgen action in early life (16–18). To our knowledge, only one study has previously investigated the impact of BPA exposure in relation to markers of reproductive function in adulthood but did not see any clear association (19).
Because of the ultraviolet (UV) absorbing properties, benzophenones are used in a wide range of consumer products, including sunscreen formulations and skin lotions, but also as additives in e.g. plastic food packaging materials, paint, and textiles. In recent years, one of the most used UV absorbers, benzophenone-3 (BP-3) has been detected in most children and adults in US and Danish populations (20–23). Besides being present in pregnant women, several benzophenones have been shown to reach cord blood and fetal blood, although concentrations are lower than in maternal circulation (24). In vitro and in vivo studies on different species have indicated that some UV filters, e.g. BP-3 can interfere with reproductive hormones by exerting estrogenic or antiandrogenic effects (25–27). In humans, studies on benzophenones in relation to adult male reproductive outcomes are scarce and only include cross-sectional studies of young men rather than studies of in utero exposures (28, 29).
In general, human studies on prenatal exposure to environmental chemicals and male reproductive health in adulthood are scarce due to the inherent challenges in performing longitudinal studies with sufficient follow-up time while maintaining an adequate study participation rate. Thus, based on a unique mother-child cohort initiated in 1997 with repetitively, and long-term follow-up of the individuals, the aim in the present study was to investigate the association between maternal serum concentrations of phenols and benzophenones in pregnancy and reproductive function in the adult sons.
2 Material and methods
2.1 Study population
This study is part of a prospective longitudinal birth cohort study of pregnant women and their sons born between 1997 and 2001 at three hospitals in the Copenhagen area, Denmark. Pregnant women belonging to the referral areas were enrolled during the first trimester of pregnancy and a blood sample was drawn at least once during pregnancy (30), although not all women had material left for biobanking that could be used in the present study. More than 1,000 live-born boys were included in the study with repetitive examinations and when they turned 18 years, they were all invited to participate in an adult follow-up examination at the Department of Growth and Reproduction, Rigshospitalet (31). Men from this adult follow-up examination were included in the present study if a maternal serum sample from pregnancy was available for chemical analyses. In total, 101 young men were included in the study, including a pair of twins (Figure 1).
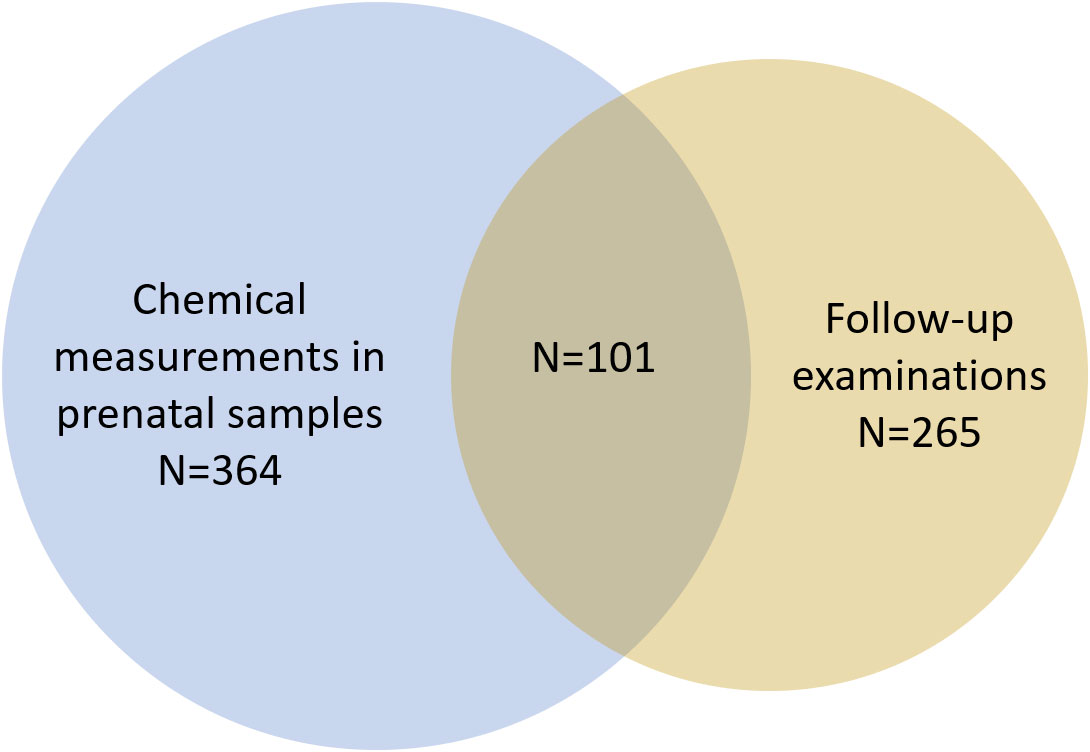
Figure 1 Overview of number of chemical measurements in prenatal samples and number of sons with a follow-up examination.
The study was performed following the Declaration of Helsinki II and approved by the Regional Committee on Health Research Ethics ((KF) 01-030/97 and H-17011468) and the Danish Data Protection Agency (1997-1200-074 and VD-2018-118/i-Suite 6358). All participants gave written informed consent prior to participation in the study.
2.2 Maternal and birth characteristics
Information on maternal age at birth, smoking habits, parity and birth weight was obtained from hospital records. Pre-pregnancy weight was obtained from self-administered questionnaires during pregnancy.
2.3 Follow-up examination of adult sons
All participating young men completed a detailed questionnaire, including information on lifestyle and general health, prior to the day of study participation, at which they delivered a semen sample, had a blood sample drawn for assessment of reproductive hormone levels, and had a physical examination (31). The examination included recording of the men’s weight and height, and BMI was calculated (kg/m2). Furthermore, anogenital distance (AGD) was determined; Anoscrotal distance (AGDAS, from the posterior base of the scrotum to the anterior wall of the anus) and anopenile distance (AGDAP, from the anterior base of the penis to the anterior wall of the anus) were measured with a calliper with the men in lithotomy position. Body fat percentage was assessed by use of a whole-body dual x-ray absorptiometry (DXA) scan (Lunar Prodigy, GE Healthcare, Madison, WI). Participants were compensated for their time (DKK 500 ≈ USD 78).
2.4 Chemical analyses of maternal serum samples
In total, serum samples from 364 pregnant women, of which 100 samples belonged to a mother whose son(s) participated in the follow-up examination, were identified and analysed for chemicals at the Department of Growth and Reproduction, Rigshospitalet (Figure 1). Samples were analysed for the total concentration (free+conjugated) of 16 chemicals using isotope dilution online Turboflow-liquid chromatography-tandem mass spectrometry (LC-MS/MS) systems equipped with heated electro spray ionization sources changing from positive to negative mode depending on the specific analytes. Prior to the chemical analysis all samples and control materials were enzymatically deconjugated as previously described (22, 24, 32). The chemicals included BPA, TCS, triclocarban (TCC), three chlorinated phenols, two phenyl phenols, and six benzophenones (for full chemical names, abbreviations, and limits of detection (LOD) see Table 1). In brief, all maternal serum samples were analyzed for the benzophenones in 13 batches and for the phenols in 14 batches, all including standards for calibration curves, two blanks, three un-spiked serum pool controls, and two times three serum pool controls spiked with a mixture of native benzophenone and phenol standards in low (QClow) and high (QChigh) concentration levels. The mean recovery was > 82% for all analytes except QClow for BP-7 (73%) and 4-PP (74%). The relative standard deviations (RSD) ranged from 7-15% in both spike levels for all analytes except QClow for BP-7 (19%), BPA (23%), TCC (26%) and 4-PP (18%) and QChigh for TCC (24%).
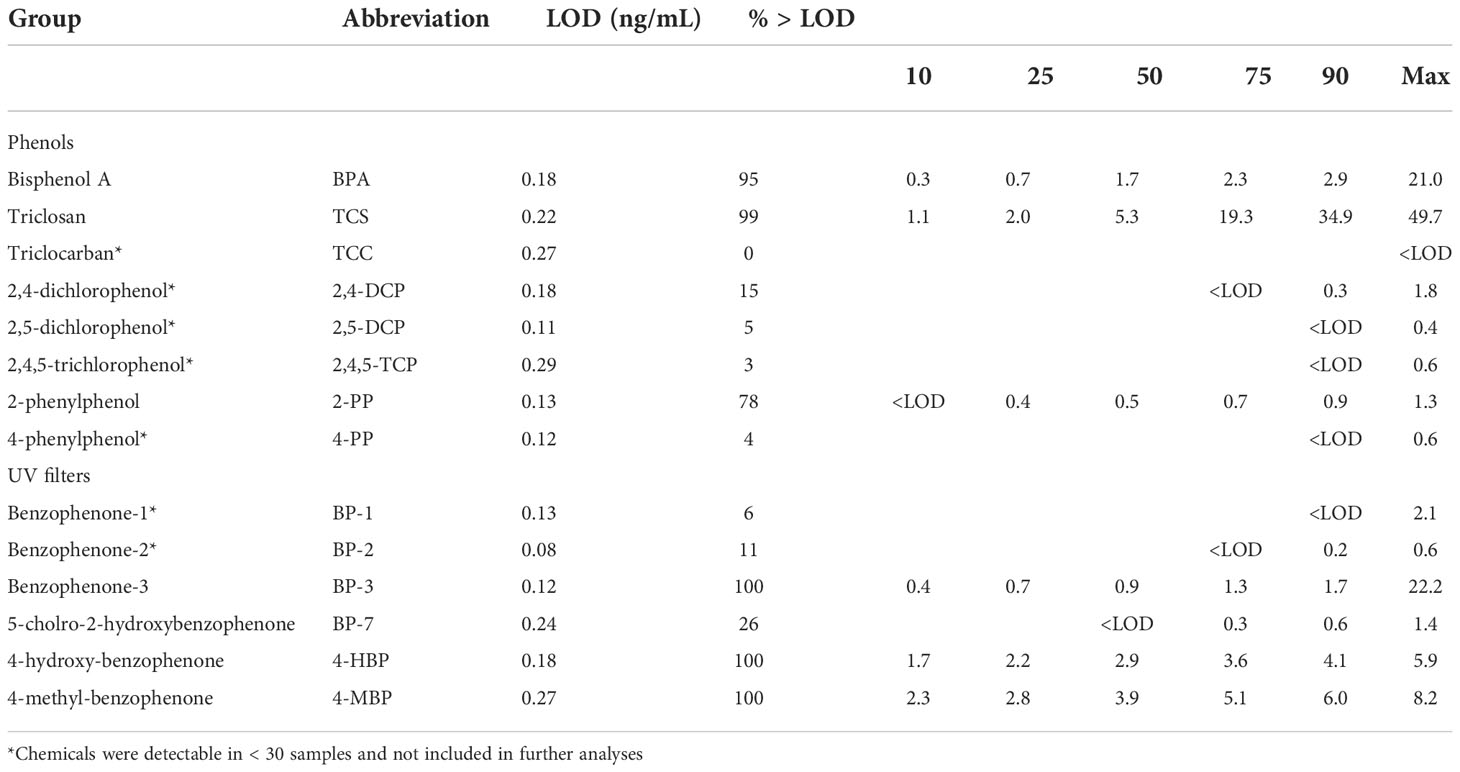
Table 1 Chemical names, abbreviations, LODs (limit of detection) and serum concentrations of phenols and benzophenones in 100 prenatal serum samples (percentiles).
2.5 Hormone analyses of serum samples from adult sons
All men had a fasting blood sample drawn in the morning. Samples were centrifuged and stored at -20°C until analysis. All serum hormone measurements were performed in the same laboratory at the Department of Growth and Reproduction in 2020. Testosterone, estradiol and sex hormone-binding globulin (SHBG) were measured by a chemiluminescent enzyme immunoassay (Access2, Beckman Coulter, Brea, CA, USA), follicle stimulating hormone (FSH) and luteinizing hormone (LH) were measured using a two-sided fluoroimmunometric assay (DELFIA, Wallac Oy, Turku, Finland), inhibin B levels were measured by an enzyme-linked immunosorbent assay (ELISA, Inhibin B gen II, Beckman Coulter). Insulin-like factor 3 (INSL3) was determined using liquid chromatography-mass spectrometry (LC-MS/MS) with a limit of detection (LOD) of 0.03 μg/L (33). Finally, insulin-like growth factor 1 (IGF-1) and insulin-like growth factor-binding protein 3 (IGFBP-3) levels were analyzed by automated chemiluminescence immunoassay (IDS-ISYS, Immunodiagnostic systems, Boldon, United Kingdom). In the full measurement range, the interassay coefficients of variation were less than 21% for INSL3, less than 8% for inhibin B, 7% for testosterone, estradiol, SHBG and IGFBP-3, and less than 5% for IGF-1, LH and FSH. Free testosterone was calculated based on the level of total testosterone and SHBG assuming a fixed albumin level of 43.0 g/L using the equation by Vermeulen et al. (34). Derived ratios between total testosterone and LH (TT/LH) and inhibin B and FSH were calculated.
2.6 Semen analyses
All men provided a semen sample by masturbation in a room close to the semen laboratory and samples were kept at 37°C until analysis. The period of ejaculation abstinence was recorded. The men had been asked to abstain from ejaculation for at least 48 h before sampling but were still included if abstinence time was shorter. Semen analyses were performed in agreement with the World Health Organization (WHO) guidelines (35). Briefly, semen volume was determined by weighing, sperm concentration was assessed using an automated sperm cell counter (NucleoCounter® NC-3000™, Chemometec A/S, Alleroed, Denmark) and the total sperm count was calculated as the product of sample volume and sperm concentration. Sperm motility was assessed by placing 10 µl semen on a glass slide and using phase-contrast microscopy. Spermatozoa were classified as either progressive motile, non- progressive motile or immotile. Fixed and Papanicalaou stained morphology slides were prepared and evaluated according to ‘strict criteria’ (36). All assessments were done in duplicates by the same observer and the average was used.
2.7 Statistical methods
Measurements for each chemical were presented as percentiles and maximum values as well as number of samples above the analytical LOD (Table 1). In further statistical analyses, all non-detectable chemical values (< LOD) were replaced by LOD/√2. To elucidate if mothers of men who participated in the follow-up examination differed from mothers of men who did not, a comparison of prenatal chemical levels for the two groups was performed using a Mann-Whitney U test and of maternal basic characteristics.
Basic characteristics and levels of reproductive hormones (n=101), semen parameters (n=99) and AGD (n=88) for the total study population are presented as medians and inter-quartile range.
For chemicals where less than 30% of the samples had concentrations above LOD, analyses of associations with outcomes were not performed. For the remaining chemicals, the marginal correlation between each of the compounds were tested using Spearman’s rank-order correlation. Associations between chemical levels in relation to reproductive hormones, semen parameters and AGD were performed using univariate and multiple linear regression analysis. To accommodate potential nonlinear associations, chemical levels were analyzed in tertiles (T1-T3) and a trend test was performed by inserting the tertiles in the model as a continuous variable.
To obtain normally distributed residuals and homoscedasticity, reproductive hormones and the two measures of AGD were transformed by natural logarithm (ln). Testicular volume, sperm concentration and total sperm count were transformed by ln whereas the variables “% morphological normal sperm” and “% progressive motile sperm” remained untransformed. Covariates were selected a priori based on literature and included potential confounders for the association as well as covariates closely related to variation in the exposure or outcome measurements (i.e., time of blood sampling and period of ejaculation abstinence). Models were adjusted stepwise; an unadjusted model of the marginal association between exposure and outcome, Model I including adjustment for maternal factors (maternal smoking (yes/no), gestational age at blood sampling (continuous variable)), and Model II including additional adjustment for covariates related to the son (smoking status of son (daily smoker/occasional smoker/non-smoker), body fat percentage (continuous variable), weekly alcohol intake (continuous variable) and physical fitness (Good or very good/moderate/poor). All adjusted analyses with reproductive hormones were further adjusted for time of blood sampling (continuous variable), adjusted analyses with AGD measurements for height of the son, adjusted analyses with semen parameters for period of abstinence (continuous variable) and adjusted analyses with sperm motility for the time between delivery of semen sample and start of motility assessment (continuous variable). Estimates based on ln-transformed outcome variables were back-transformed using the formula: 100*(exp(β)-1), as described in (37). to reflect the percentage differences in outcome variables according to tertiles of chemical levels.
In subanalyses, further adjustment for maternal age (continuous variable) and parity (1, 2, 3 or more) was performed in separate models. Also, one man with azoospermia was excluded from analyses with semen parameters as the outcome, and the findings were compared to the main findings. Finally, to account for multiple testing, all p-values from fully adjusted models were adjusted according to the Benjamini-Hochberg (38, 39) method and compared with the p-values without this adjustment.
P-values < 0.05 were considered statistically significant. Analyses were performed using SPSS v. 25 (Armonk, NY: IBM Corp, USA).
3 Results
Of the included chemicals, only BPA, TCS, 2-phenylphenol, BP-3, 4-hydroxy-benzophenone (4-HBP) and 4-methyl-benzophenone (4-MBP) could be detected in more than 30% of the samples (Table 1). BPA showed a significant weak correlation with 2- phenylphenol (r=0.20, p=0.04) whereas BP-3 was moderately correlated to 2-phenylphenol (r=0.33, p<0.01), 4-HBP (r=0.55, p<0.01) and 4-MBP (r=0.34, p<0.01). Finally, 2-phenylphenol and 4-MBP were moderately correlated (r=0.41, p<0.01) whereas no other significant correlations were observed (data not shown).
No difference in chemical concentrations was observed between mothers of men who did not participate in the follow-up examination and men who did except for TCS (Figure 2). Median TCS levels were significantly higher in mothers of men who participated in the follow-up (5.3 ng/mL vs 3.4 ng/mL, respectively, p=0.015).
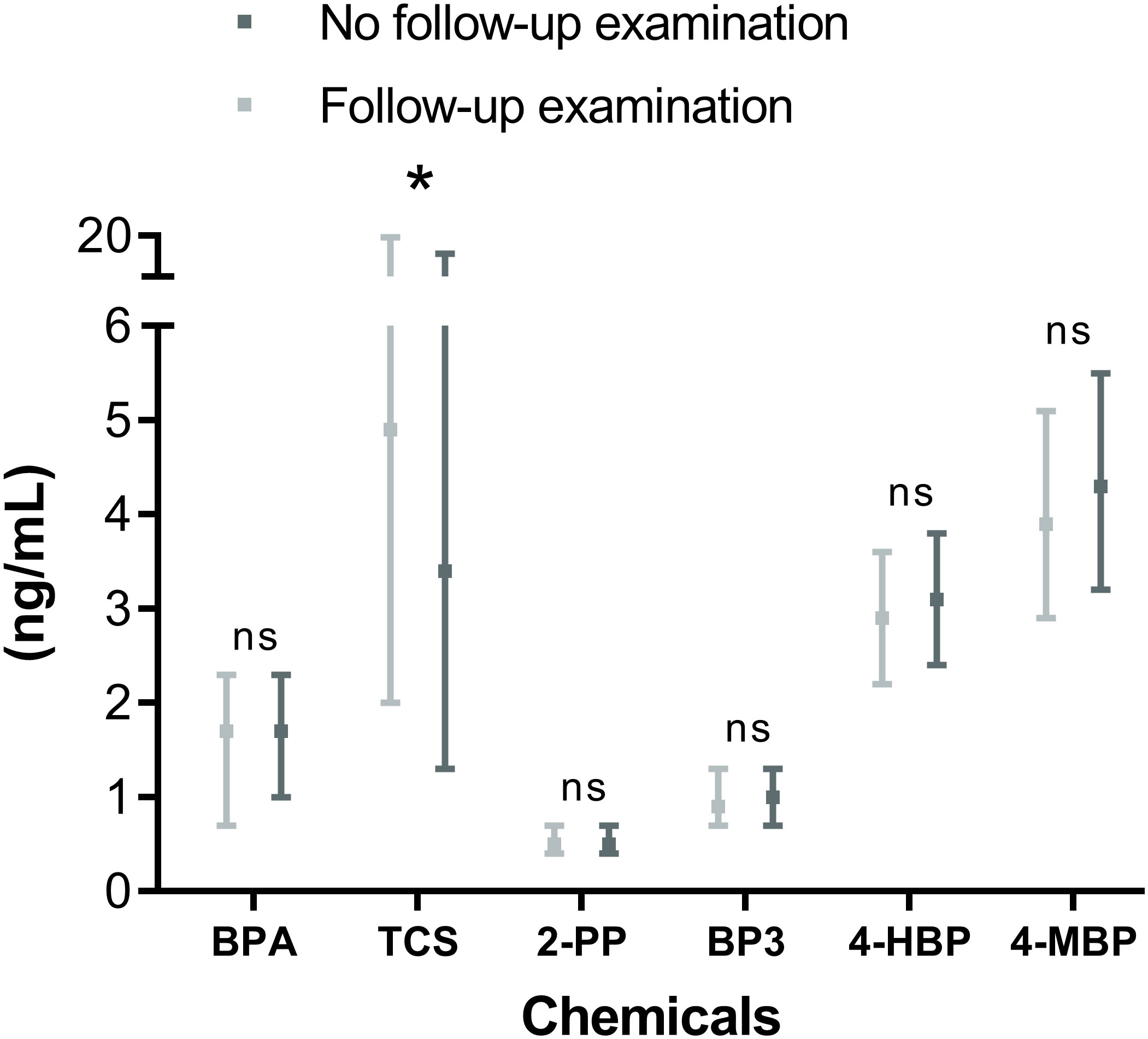
Figure 2 Comparison of chemical levels in pregnancy serum samples from mothers with sons with (n=100) or without (n=263) a follow-up examination. P-values <0.05 are indicated by an asterisk. BPA, bisphenol A; TCS, triclosan; 2-PP, 2-phenylphenol; BP-3, benzophenone-3; 4-HBP, 4-hydroxy-benzophenone; 4-MBP, 4-methyl-benzophenone.
The mothers’ median age at delivery was 31 years and their sons were 19 years old at follow-up (Table 2). Their median sperm concentration was 52 million/ml and the median total sperm count was 131 million (Table 3).
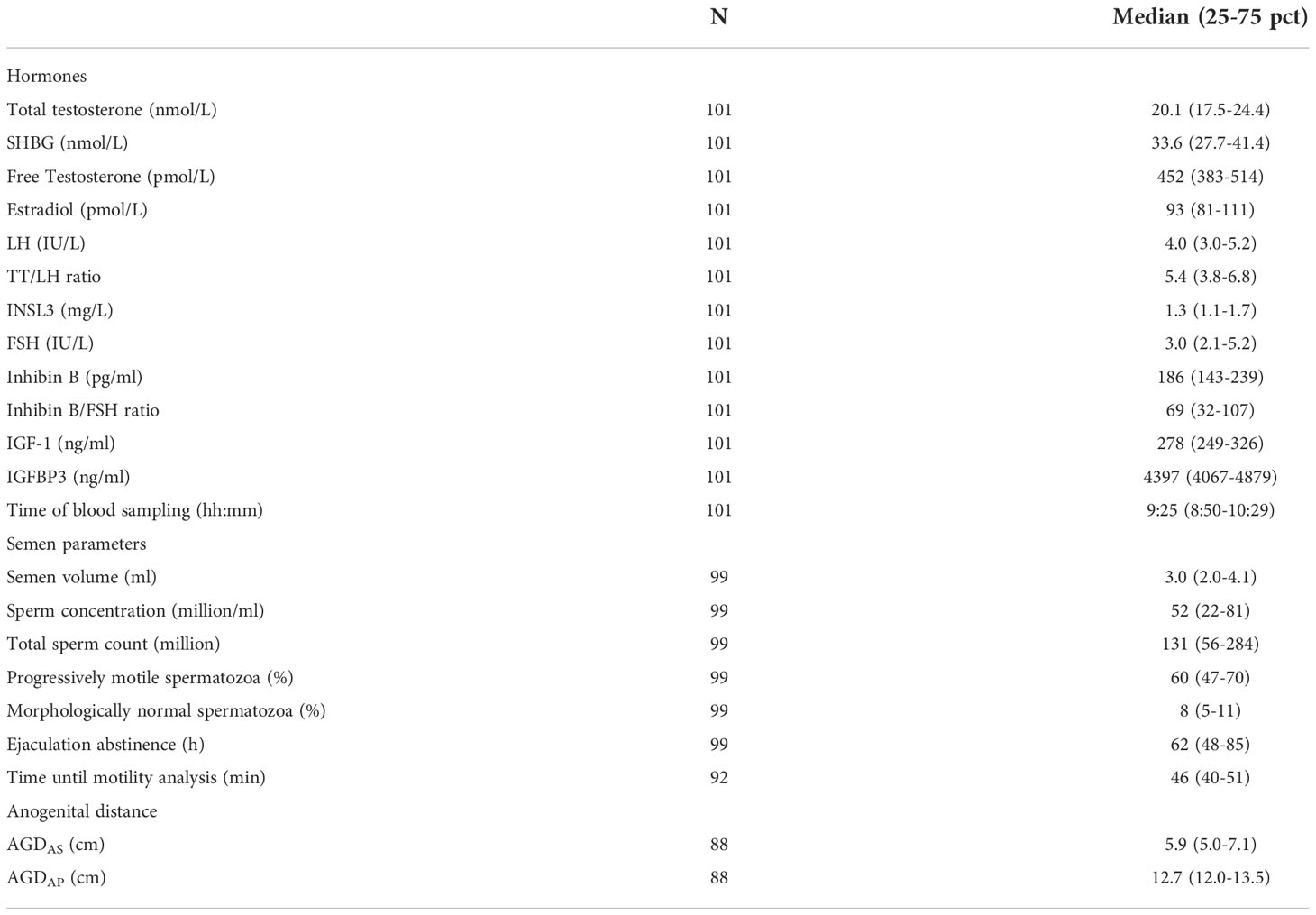
Table 3 Description of outcome variables for the study population with maternal chemical measurements and follow-up examination (n=101).
In fully adjusted analyses, higher prenatal levels of BPA (evaluated in tertiles, T1-T3) were significantly associated with higher LH levels in sons (T2: 12% (-8%,36%) and T3: 33% (10%,62%) compared to the reference category, T1, respectively) (Figure 3; Supplementary Tables 1–3) but not with total testosterone. Hence, higher BPA levels were associated to lower TT/LH ratio (T2: -20% (-35%,-1%), T3: -23% (-38%,-6%). An inverted U-shaped association was seen for BP-3 exposure and total testosterone level (T2: 16% (3%,31%), T3: -1% (-12%,12%)) and to a lesser degree for free testosterone (T2: 13% (-1%,27%) and T3: 6% (-6%,19%) compared to T1) (Figure 4; Supplementary Tables 4–6). Also, a positive association between BP-3 exposure and LH levels was seen (T2: 21% (-2%,49%), T3: 18% (-4%,45%) compared to T1). Finally, a tendency of higher INSL3 levels with higher tertile of BP-3 was seen (p-trend = 0.04). For 4-HBP a tendency was seen of higher exposure levels being associated to higher levels of INSL3 (p-trend = 0.09) as well as lower levels of IGF-1 (p-trend = 0.06). For prenatal TCS exposure, no significant associations were found. However, a tendency of higher levels of FSH with higher TCS exposure was seen. No clear associations were seen for 2-phenylphenol in relation to the observed outcomes.
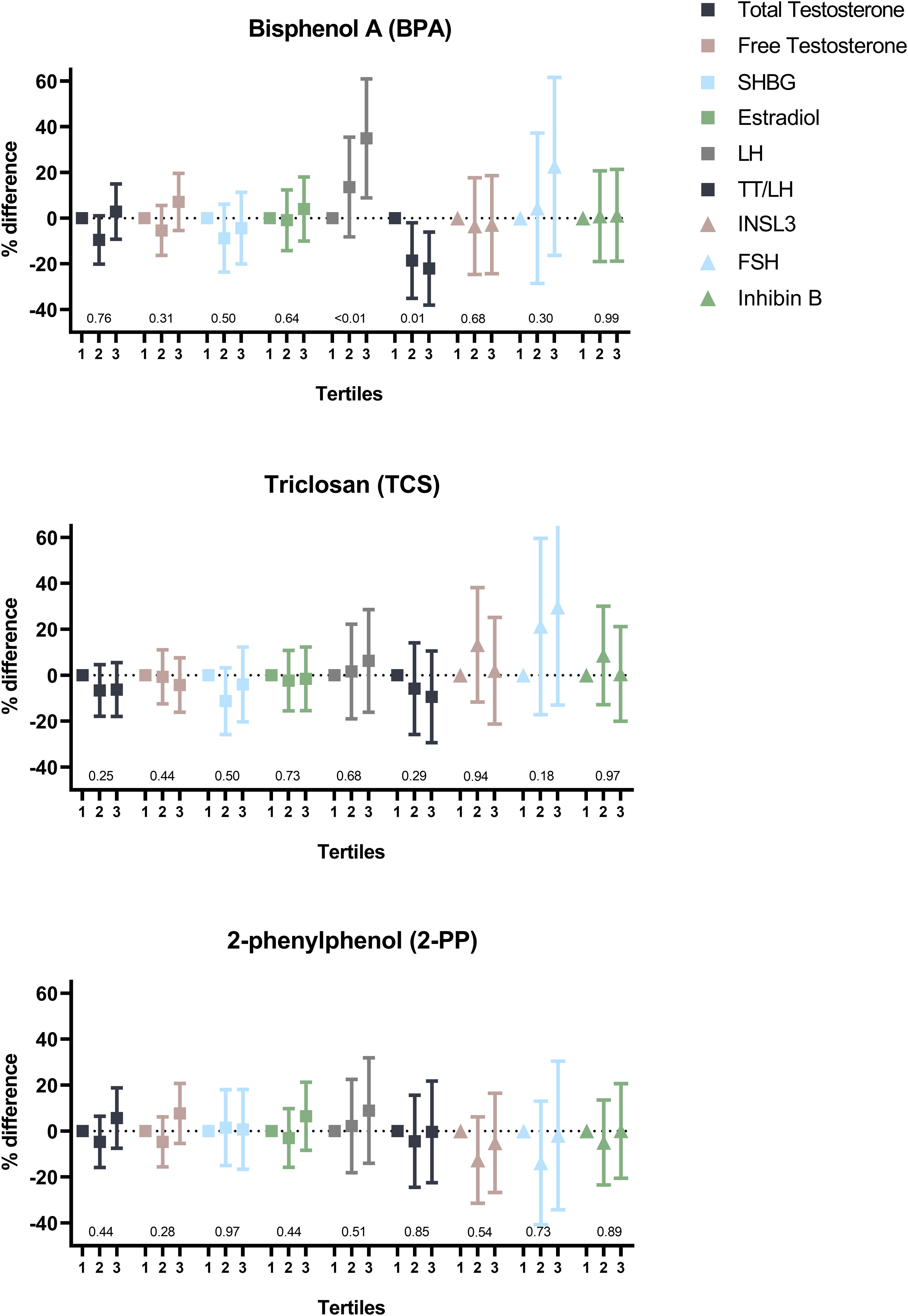
Figure 3 Percentage difference in levels of serum hormone concentrations according to tertiles of prenatal phenol exposure levels adjusted for confounders. P-values indicate trend across categories. SHBG, sex hormone-binding globulin; LH, luteinizing hormone; TT/LH, total testosterone/Luteinizing hormone ratio; INSL3, Insulin-like factor 3; FSH, follicle stimulating hormone.
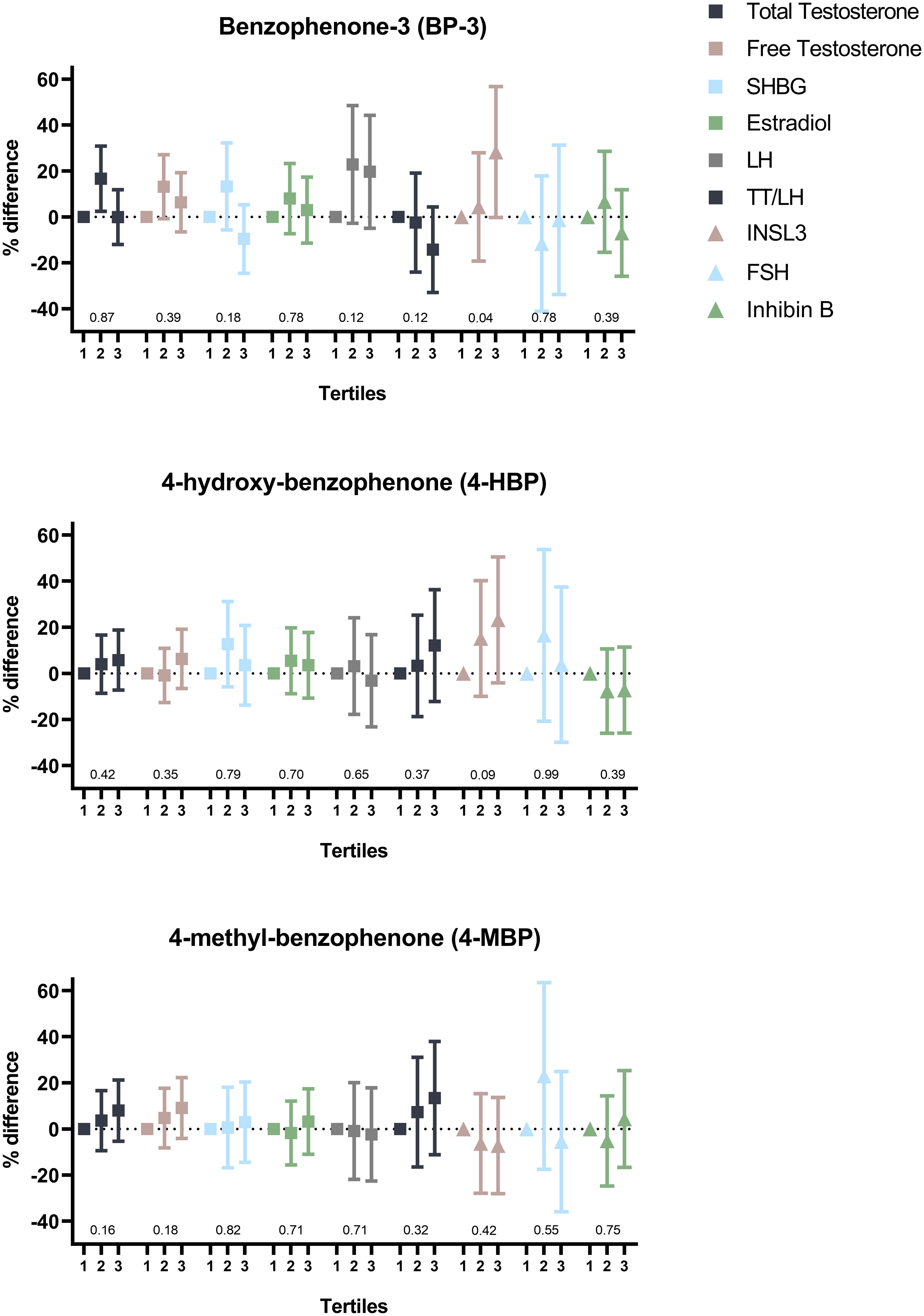
Figure 4 Percentage difference in levels of serum hormone concentrations according to tertiles of prenatal benzophenone exposure level adjusted for confounders. P-values indicate trend across categories. SHBG, sex hormone-binding globulin; LH, luteinizing hormone; TT/LH, total testosterone/Luteinizing hormone ratio; INSL3, Insulin-like factor 3; FSH, follicle stimulating hormone.
No clear associations were seen between any of the chemicals and the semen parameters and AGD with exception of 2-phenylphenol that tended to be positively associated to higher sperm concentration and total sperm count (p-trend = 0.17 and p-trend = 0.11, respectively) (Supplementary Tables 7–12).
In subanalyses, further adjustment for maternal age or parity did not alter the observed findings. In general, excluding the one azoospermic man from analyses did not change the results. After adjusting for multiplicity, no associations remained statistically significant (data not shown).
4 Discussion
In this long-term prospective study, we observed that young men prenatally exposed to the highest levels of BPA and BP-3 had higher LH levels in adulthood and a lower TT/LH ratio indicating a compensated reduced Leydig cell function. However, no associations were seen to AGD as a marker of prenatal androgen action or to markers of semen quality. Testosterone production in men is characterized by a buffer capacity so that testosterone levels to some degree can be sustained by a compensatory increase in LH stimulation. Although our observational study cannot prove causality, our findings indicate a long-lasting effect of prenatal BPA and BP-3 exposure on Leydig cell function which has been described in experimental studies. A compensated Leydig cell function has been associated with an increased all-cause mortality in a long term follow-up study (40). Thus, we speculate that the observed compensated Leydig cell function in this cohort of young men may become clinically relevant over time and reflect a potential health risk.
Several in vitro studies have shown that BPA has both estrogenic and anti-androgenic properties due to its capability to bind and activate the estrogen receptor although with much lower affinity than estradiol (41, 42) as well as the androgen receptor (43). In addition, BPA can inhibit steroidogenesis via a number of pathways in both human and rodent testis in vitro and thus, exert antiandrogenic effects (44, 45). Rodent studies of postnatal effects of in utero exposure to BPA are conflicting and differences in findings are likely explained by variations in doses and duration of exposure as well as differences between species. However, prenatal and perinatal BPA exposure has in the majority of studies been associated to reduced testis weight and testosterone level as well as a reduction in key enzymes and proteins in steroidogenesis in male offspring, indicating a disruption of Leydig cell function [reviewed in (46)].
Anogenital distance (AGD) is the distance from anus to the genitals and has in rodents been shown to be determined by androgen action in early fetal life (47). Thus, reduced AGD may be indicative of insufficient testosterone during the early stages of reproductive development. Furthermore, in adult men a shorter AGD has been associated with impaired semen quality (48). Few human studies have investigated the association between prenatal BPA exposure and AGD with conflicting results. Several studies found no association between maternal BPA levels and AGD levels in newborns (17, 18, 49) in line with our findings, whereas one study observed that higher maternal BPA levels were associated with shorter AGD among 12-month-old boys (18). Notably, in a real-life scenario, exposure to BPA occurs continuously throughout pregnancy and postnatally, which complicates human observational studies compared to controlled exposure settings in rodents and in vitro experiments.
To our knowledge, only one other longitudinal pregnancy cohort has investigated the association between prenatal BPA levels and reproductive hormones in adulthood. In contrast to our findings, results from that cohort indicated that maternal serum levels of BPA were positively associated with the sons’ sperm concentration and motility in adulthood, whereas no association was seen with levels of reproductive hormones, including LH (19). This difference may be explained by differences in BPA exposure levels which was higher in our cohort compared to the levels observed in the Raine cohort (median (max): 1.7 ng/ml (21 ng/ml) vs. 0.25 ng/ml (10.5 ng/ml), respectively). Cross-sectional studies of young adult men have observed a positive association between urinary BPA levels and LH levels in line with our findings (50, 51). However, one of the studies also observed that higher urinary levels of BPA were associated with higher levels of total testosterone and estradiol suggesting that BPA in adult men could act as a partial antagonist to both the estrogen and androgen receptor (50).
In the present study, higher levels of BP-3 were associated with higher LH levels and consequently a lower TT/LH ratio whereas an inverted U-shaped association was seen in relation to testosterone levels. Maternal levels of BPA and BP-3 were not correlated, indicating different exposure patterns and thus, separate effects may be at play. An in vivo study in rodents showed that male offspring dermally exposed to BP-3 at up to 7 weeks of age and with dermally exposed mothers during pregnancy showed a substantial reduction in plasma testosterone levels (52). This may be explained by a direct adverse effect of BP-3 on the Leydig cells or on steroidogenesis in general. Thus, a decrease in testosterone could subsequently be followed by a compensatory increase in LH in line with our findings. More studies in humans are, however, needed to confirm the associations seen for BP-3 in relation to testosterone and LH, respectively.
To our knowledge, no longitudinal studies in humans have investigated the association between prenatal BP-3 exposure and reproductive hormones in adulthood, however, few cross-sectional studies have been performed with conflicting results. In a cohort of 215 young Spanish men, higher urinary BP-3 concentrations were associated with higher FSH levels, whereas no associations were seen with LH or other reproductive hormones (28). However, in a study of 195 young Danish men, higher urinary BP-3 levels were associated with higher total testosterone and estradiol levels and lower FSH levels but only in men with a loss-of-function mutation in the filaggrin gene, important for normal skin barrier, and thereby probably generally more exposed dermally (29).The levels of BP-3 were in general higher in the Danish men compared to the Spanish men (median levels: 2.8 ng/ml vs. 1.3 ng/ml), and it is possible, that different levels of co-exposures not accounted for in the studies also could contribute to the observed differences in findings.
In contrast to cross-sectional studies, a key advantage of the present study is the use of a prospective birth cohort with information on chemical exposures during a critical period where reproductive development takes place (3) and with detailed follow-up information including a comprehensive andrological examination. Some limitations should, however, be considered when interpreting the results. Multiple factors are known or suspected to affect reproductive outcomes in the young men. This includes adult exposure to phenols and benzophenones as well as exposures from additional EDCs [reviewed in (53)] which was not taken into consideration in this study. We also did not explore simultaneous fetal exposure to other chemicals in this study, although animal studies have shown that mixed exposures to different chemical groups during fetal life may have additive effects on reproductive outcomes (54). Although we adjusted analyses of the association between exposure levels and adult reproductive function for several confounders, we could not control for variations in this over time. Furthermore, we cannot exclude, that our findings are influenced by residual confounding not accounted for in the present study.
Another limitation is the small study size, which limits the statistical power. Furthermore, no associations were significant after adjustment for multiplicity. Thus, we cannot rule out, that our findings are false positive (type I errors) and the data should be replicated in larger studies. This project applied a single prenatal exposure measurement and a single adult reproductive health assessment despite the well-known intra-individual variation in both (55), which increases the risk of exposure and outcome misclassification. However, these would be expected to be random and most likely attenuate any true association. We speculate that the endogenous negative feedback mechanism between LH and testosterone may have been the most sensitive parameter for finding subtle long-term effects of prenatal exposures. In contrast, semen quality shows a high intra-individual variation which could attenuate potential associations (56). Thus, our findings need to be replicated in larger populations. Furthermore, we cannot rule out that genetic factors could play a role for the observed associations which was however, not investigated in the present study. Another limitation is that exposure levels were analyzed in serum samples as maternal urine samples were not available. Urine is considered the preferred matrix to determine exposures of non-persistent compounds due to their short half-lives and subsequent rapid metabolization and excretion into urine (57). Also for bisphenols, urine is the preferred matrix to determine the concentrations (58), although urine alone also has been acknowledged as unsuitable to assess the full toxicokinetics of bisphenols (59). Moderate correlations have furthermore been observed between some benzophenones determined in serum compared to urine (23). Since serum concentrations are lower compared to urine concentrations, a higher proportion of the samples will be below detection level with the risk of underestimating the exposure in the lower range.
In conclusion, our findings indicate that young men prenatally exposed to BP-3 or BPA were characterized by a compensated reduced Leydig cell function in adulthood whereas no association was seen in relation to AGD or semen quality. Larger studies on long-term reproductive consequences of prenatal exposures are warranted to validate our findings.
Data availability statement
The datasets presented in this article are not readily available because the data are not publicly available due to ethical restrictions. Requests to access the datasets should be directed to Katharina M. Main, S2F0aGFyaW5hLk1haW5AcmVnaW9uaC5kaw==.
Ethics statement
The studies involving human participants were reviewed and approved by the Regional Committee on Health Research Ethics ((KF) 01-030/97 and H-17011468) and the Danish Data Protection Agency (1997-1200-074 and VD-2018-118/i-Suite 6358). The patients/participants provided their written informed consent to participate in this study.
Author contributions
Conceptualization: SH, A-MA, AJ, JT, NS, KM. Data collection: LS, HF, KM. Data analysis and interpretation: SH, LS, A-MA, LP, NJ, NS, KM. All authors had full access to the data in the study and take responsibility for the integrity and the accuracy of the data analysis. All authors critically reviewed the manuscript and approved the submitted version. All authors contributed to the article and approved the submitted version.
Funding
This study was supported by Innovation Fund Denmark (IFD 8056-00005B), Centre on Endocrine Disruptors, Danish Environmental Protection Agency (MST-611-00012), “Oda og Hans Svenningsens Fond” (F-22451-08), “Fabrikant Vilhelm Pedersen og Hustrus Legat” (NNF15OC0017642), “Fonden til Lægevidenskabens Fremme” (17-L-0297), Academy of Finland, Sigrid Juselius Foundation, Kirsten and Freddy Johansen Fund, and Turku University Hospital Special Governmental Fund.
Conflict of interest
The authors declare that the research was conducted in the absence of any commercial or financial relationships that could be construed as a potential conflict of interest.
Publisher’s note
All claims expressed in this article are solely those of the authors and do not necessarily represent those of their affiliated organizations, or those of the publisher, the editors and the reviewers. Any product that may be evaluated in this article, or claim that may be made by its manufacturer, is not guaranteed or endorsed by the publisher.
Supplementary material
The Supplementary Material for this article can be found online at: https://www.frontiersin.org/articles/10.3389/fendo.2022.1071761/full#supplementary-material
References
1. Skakkebæk NE, Andersson A, Jørgensen N, Hart RJ. Environmental factors in declining human fertility. Nat Rev Endocrinol (2022) 18(3):139–57. doi: 10.1038/s41574-021-00598-8
2. Skakkebæk NE, Rajpert-De Meyts E, Main KM. Testicular dysgenesis syndrome: An increasingly common developmental disorder with environmental aspects. Hum Reprod (2001) 16(5):972–8. doi: 10.1093/humrep/16.5.972
3. Skakkebaek NE, Rajpert-De Meyts E, Buck Louis GM, Toppari J, Andersson AM, Eisenberg ML, et al. Male Reproductive disorders and fertility trends: Influences of environment and genetic susceptibility. Physiol Rev (2015) 96(1):55–97. doi: 10.1152/physrev.00017.2015
4. Le Moal J, Goria S, Guillet A, Rigou A, Chesneau J. Time and spatial trends of operated cryptorchidism in France and environmental hypotheses: a nationwide study from 2002 to 2014. Hum Reprod (2021) 36(5):1383–94. doi: 10.1093/humrep/deaa378
5. Bergman Å, Heindel JJ, Kasten T, Kidd KA, Jobling S, Neira M, et al. The impact of endocrine disruption: A consensus statement on the state of the science. Environ Health Perspect (2013) 121(4):104–6. doi: 10.1289/ehp.1205448
6. Frederiksen H, Jensen TK, Jørgensen N, Kyhl HB, Husby S, Skakkebæk NE, et al. Human urinary excretion of non-persistent environmental chemicals: An overview of Danish data collected between 2006 and 2012. Reproduction (2014) 147(4):555–65. doi: 10.1530/REP-13-0522
7. Michałowicz J. Bisphenol a - sources, toxicity and biotransformation. Environ Toxicol Pharmacol (2014) 37(2):738–58. doi: 10.1016/j.etap.2014.02.003
8. Weatherly LM, Gosse JA. Triclosan exposure, transformation, and human health effects. J Toxicol Environ Heal Part B (2017) 20(8):447–69. doi: 10.1080/10937404.2017.1399306
9. Frederiksen H, Nielsen O, Koch HM, Skakkebaek NE, Juul A, Jørgensen N, et al. Changes in urinary excretion of phthalates, phthalate substitutes, bisphenols and other polychlorinated and phenolic substances in young Danish men; 2009–2017. Int J Hyg Environ Health (2020) 223(1):93–105. doi: 10.1016/j.ijheh.2019.10.002
10. Husøy T, Andreassen M, Hjertholm H, Carlsen MH, Norberg N, Sprong C, et al. The Norwegian biomonitoring study from the EU project EuroMix: Levels of phenols and phthalates in 24-hour urine samples and exposure sources from food and personal care products. Environ Int (2019) 132(March):105103. doi: 10.1016/j.envint.2019.105103
11. Cimmino I, Fiory F, Perruolo G, Miele C, Beguinot F, Formisano P, et al. Potential mechanisms of bisphenol a (BPA) contributing to human disease. Int J Mol Sci (2020) 21(16):1–22. doi: 10.3390/ijms21165761
12. Lukasiewicz M, Czerniecki J, Ponikwicka-Tyszko D, Sztachelska M, Hryniewicka M, Nalewajko-Sieliwoniuk E, et al. Placenta is capable of protecting the Male fetus from exposure to environmental bisphenol a. Expo Heal [Internet] (2021) 13(1):1–14. doi: 10.1007/s12403-020-00358-5
13. Rahman MS, Pang WK, Ryu DY, Park YJ, Ryu BY, Pang MG. Multigenerational impacts of gestational bisphenol a exposure on the sperm function and fertility of male mice. J Hazard Mater (2021) 416(March):125791. doi: 10.1016/j.jhazmat.2021.125791
14. Salian S, Doshi T, Vanage G. Perinatal exposure of rats to bisphenol a affects the fertility of male offspring. Life Sci (2009) 85(21–22):742–52. doi: 10.1016/j.lfs.2009.10.004
15. Hong J, Chen F, Wang X, Bai Y, Zhou R, Li Y, et al. Exposure of preimplantation embryos to low-dose bisphenol a impairs testes development and suppresses histone acetylation of StAR promoter to reduce production of testosterone in mice. Mol Cell Endocrinol (2016) 427:101–11. doi: 10.1016/j.mce.2016.03.009
16. Lassen TH, Frederiksen H, Kyhl HB, Swan SH, Main KM, Andersson AM, et al. Prenatal triclosan exposure and anthropometric measures including anogenital distance in Danish infants. Environ Health Perspect (2016) 124(8):1261–8. doi: 10.1289/ehp.1409637
17. Arbuckle TE, Agarwal A, MacPherson SH, Fraser WD, Sathyanarayana S, Ramsay T, et al. Prenatal exposure to phthalates and phenols and infant endocrine-sensitive outcomes: The MIREC study. Environ Int (2018) 120(August):572–83. doi: 10.1016/j.envint.2018.08.034
18. Sun X, Li D, Liang H, Miao M, Song X, Wang Z, et al. Maternal exposure to bisphenol a and anogenital distance throughout infancy: A longitudinal study from shanghai, China. Environ Int (2018) 121(August):269–75. doi: 10.1016/j.envint.2018.08.055
19. Hart RJ, Doherty DA, Keelan JA, Minaee NS, Thorstensen EB, Dickinson JE, et al. The impact of antenatal bisphenol a exposure on male reproductive function at 20–22 years of age. Reprod BioMed Online (2018) 36(3):340–7. doi: 10.1016/j.rbmo.2017.11.009
20. Calafat AM, Wong LY, Ye X, Reidy JA, Needham LL. Concentrations of the sunscreen agent benzophenone-3 in residents of the united states: National health and nutrition examination survey 2003-2004. Environ Health Perspect (2008) 116(7):893–7. doi: 10.1289/ehp.11269
21. Arya S, Dwivedi AK, Alvarado L, Kupesic-Plavsic S. Exposure of U.S. population to endocrine disruptive chemicals (Parabens, benzophenone-3, bisphenol-a and triclosan) and their associations with female infertility. Environ pollut (2020) 265:114763. doi: 10.1016/j.envpol.2020.114763
22. Frederiksen H, Nielsen O, Skakkebaek NE, Juul A, Andersson AM. UV Filters analyzed by isotope diluted TurboFlow-LC–MS/MS in urine from Danish children and adolescents. Int J Hyg Environ Health (2017) 220(2):244–53. doi: 10.1016/j.ijheh.2016.08.005
23. Frederiksen H, Krause M, Jørgensen N, Rehfeld A, Skakkebæk NE, Andersson AM. UV Filters in matched seminal fluid-, urine-, and serum samples from young men. J Expo Sci Environ Epidemiol (2021) 31(2):345–55. doi: 10.1038/s41370-020-0209-3
24. Krause M, Frederiksen H, Sundberg K, Jørgensen FS, Jensen LN, Nørgaard P, et al. Presence of benzophenones commonly used as UV filters and absorbers in paired maternal and fetal samples. Environ Int (2018) 110(October 2017):51–60. doi: 10.1016/j.envint.2017.10.005
25. Santamaría CG, Abud JE, Porporato MM, Meyer N, Zenclussen AC, Kass L, et al. The UV filter benzophenone 3, alters early follicular assembly in rat whole ovary cultures. Toxicol Lett (2019) 303(May 2018):48–54. doi: 10.1016/j.toxlet.2018.12.016
26. Kinnberg KL, Petersen GI, Albrektsen M, Minghlani M, Awad SM, Holbech BF, et al. Endocrine-disrupting effect of the ultraviolet filter benzophenone-3 in zebrafish, danio rerio. Environ Toxicol Chem (2015) 34(12):2833–40. doi: 10.1002/etc.3129
27. Schlumpf M, Cotton B, Conscience M, Haller V, Steinmann B, Lichtensteiger W. In vitro and in vivo estrogenicity of UV screens. Environ Health Perspect (2001) 109(3):239–44. doi: 10.1289/ehp.01109239
28. Adoamnei E, Mendiola J, Moñino-García M, Vela-Soria F, Iribarne-Durán LM, Fernández MF, et al. Urinary concentrations of benzophenone-type ultra violet light filters and reproductive parameters in young men. Int J Hyg Environ Health [Internet] (2018) 221(3):531–40. doi: 10.1016/j.ijheh.2018.02.002
29. Joensen UN, Jørgensen N, Thyssen JP, Szecsi PB, Stender S, Petersen JH, et al. Urinary excretion of phenols, parabens and benzophenones in young men: Associations to reproductive hormones and semen quality are modified by Mutations in the filaggrin gene. Environ Int (2018) 121(May):365–74. doi: 10.1016/j.envint.2018.09.020
30. Boisen KA, Chellakooty M, Schmidt IM, Kai CM, Damgaard IN, Suomi AM, et al. Hypospadias in a cohort of 1072 Danish newborn boys: Prevalence and relationship to placental weight, anthropometrical measurements at birth, and reproductive hormone levels at three months of age. J Clin Endocrinol Metab (2005) 90(7):4041–6. doi: 10.1210/jc.2005-0302
31. Scheutz Henriksen L, Holm Petersen J, Skakkebæk NE, Jørgensen N, Virtanen HE, Priskorn L, et al. Serum testosterone levels in 3-Month-Old boys predict their semen quality as young adults. J Clin Endocrinol Metab (2022) 107(7):1965–75. doi: 10.1210/clinem/dgac173
32. Frederiksen H, Aksglaede L, Sorensen K, Nielsen O, Main KM, Skakkebaek NE, et al. Bisphenol a and other phenols in urine from Danish children and adolescents analyzed by isotope diluted TurboFlow-LC-MS/MS. Int J Hyg Environ Health (2013) 216(6):710–20. doi: 10.1016/j.ijheh.2013.01.007
33. Albrethsen J, Frederiksen H, Andersson AM, Anand-Ivell R, Nordkap L, Bang AK, et al. Development and validation of a mass spectrometry-based assay for quantification of insulin-like factor 3 in human serum. Clin Chem Lab Med (2018) 56(11):1913–20. doi: 10.1515/cclm-2018-0171
34. Vermeulen A, Verdonck L, Kaufman JM. A critical evaluation of simple methods for the estimation of free testosterone in serum. J Clin Endocrinol Metab (1999) 84(10):3666–72. doi: 10.1210/jcem.84.10.6079
35. World Health Organization. WHO laboratory manual for the examination and processing of human semen. 5th edition. Geneva: World Health Organization (2010).
36. Menkveld R, Stander FS, Kotze TJ, Kruger TF, van Zyl JA. The evaluation of morphological characteristics of human spermatozoa according to stricter criteria. Hum Reprod (1990) 5(5):586–92. doi: 10.1093/oxfordjournals.humrep.a137150
37. Cornell Statistical Consulting Unit. Interpreting regression coefficients for log-transformed variables (2020). Available at: https://cscu.cornell.edu/wp-content/uploads/83_logv.pdf.
38. Benjamini Y, Hochberg Y. Controlling the false discovery rate: A practical and powerful approach to multiple testing. J R Stat Soc Ser B (1995) 57(1):289–300. doi: 10.1111/j.2517-6161.1995.tb02031.x
39. Benjamini Y, Yekutieli D. The control of the false discovery rate in multiple testing under dependency. Ann Stat (2001) 29(4):1165–88. doi: 10.1214/aos/1013699998
40. Holmboe SA, Vradi E, Jensen TK, Linneberg A, Husemoen LLN, Scheike T, et al. The association of reproductive hormone levels and all-cause, cancer, and cardiovascular disease mortality in men. J Clin Endocrinol Metab (2015) 100(12):4472–80. doi: 10.1210/jc.2015-2460
41. Wetherill YB, Akingbemi BT, Kanno J, McLachlan JA, Nadal A, Sonnenschein C, et al. In vitro molecular mechanisms of bisphenol a action. Reprod Toxicol (2007) 24(2):178–98. doi: 10.1016/j.reprotox.2007.05.010
42. Kuiper GGJM, Lemmen JG, Carlsson BO, Corton JC, Safe SH, Saag PTVANDER, et al. Interaction of estrogenic chemicals and phytoestrogens with estrogen receptor. Endocrinology (1998) 139(10):10–6. doi: 10.1210/endo.139.10.6216
43. Murata M, Kang JH, Bisphenol A. (BPA) and cell signaling pathways. Biotechnol Adv (2018) 36(1):311–27. doi: 10.1016/j.biotechadv.2017.12.002
44. Ye L, Zhao B, Hu G, Chu Y, Ge RS. Inhibition of human and rat testicular steroidogenic enzyme activities by bisphenol a. Toxicol Lett [Internet] (2011) 207(2):137–42. doi: 10.1016/j.toxlet.2011.09.001
45. Shamhari A‘Afifah, Abd Hamid Z, Budin SB, Shamsudin NJ, Taib IS. Bisphenol a and its analogues deteriorate the hormones physiological function of the Male reproductive system: A mini-review. Biomedicines (2021) 9(11):1744. doi: 10.3390/biomedicines9111744
46. Li X, Wen Z, Wang Y, Mo J, Zhong Y, Ge RS. Bisphenols and leydig cell development and function. Front Endocrinol (Lausanne) (2020) 11(July):1–14. doi: 10.3389/fendo.2020.00447
47. Welsh M, Saunders PTK, Fisken M, Scott HM, Hutchison GR, Smith LB, et al. Identification in rats of a programming window for reproductive tract masculinization, disruption of which leads to hypospadias and cryptorchidism. J Clin Invest (2008) 118(4):1479–90. doi: 10.1172/JCI34241
48. Priskorn L, Bang AK, Nordkap L, Krause M, Mendiola J, Jensen TK, et al. Anogenital distance is associated with semen quality but not reproductive hormones in 1106 young men from the general population. Hum Reprod (2019) 34(1):12–24. doi: 10.1093/humrep/dey326
49. Fisher BG, Thankamony A, Mendiola J, Petry CJ, Frederiksen H, Andersson A, et al. Maternal serum concentrations of bisphenol a and propyl paraben in early pregnancy are associated with male infant genital development. Hum Reprod. (2020) 35(4):913–28. doi: 10.1093/humrep/deaa045
50. Lassen TH, Frederiksen H, Jensen TK, Petersen JH, Joensen UN, Main KM, et al. Urinary bisphenol a levels in young men: Association with reproductive hormones and semen quality. Environ Health Perspect (2014) 122(5):478–84. doi: 10.1289/ehp.1307309
51. Adoamnei E, Mendiola J, Vela-Soria F, Fernández MF, Olea N, Jørgensen N, et al. Urinary bisphenol a concentrations are associated with reproductive parameters in young men. Environ Res (2018) 161(October 2017):122–8. doi: 10.1016/j.envres.2017.11.002
52. Krzyżanowska W, Pomierny B, Starek-Świechowicz B, Broniowska Ż, Strach B, Budziszewska B. The effects of benzophenone-3 on apoptosis and the expression of sex hormone receptors in the frontal cortex and hippocampus of rats. Toxicol Lett (2018) 296(July):63–72. doi: 10.1016/j.toxlet.2018.08.006
53. Rodprasert W, Toppari J, Virtanen HE. Endocrine disrupting chemicals and reproductive health in boys and men. Front Endocrinol (Lausanne) (2021) 12(October):1–45. doi: 10.3389/fendo.2021.706532
54. Conley JM, Lambright CS, Evans N, Cardon M, Furr J, Wilson VS, et al. Mixed “Antiandrogenic” chemicals at low individual doses produce reproductive tract malformations in the Male rat. Toxicol Sci (2018) 164(1):166–78. doi: 10.1093/toxsci/kfy069
55. Andersson AM, Carlsen E, Petersen JH, Skakkebæk NE. Comment: Variation in levels of serum inhibin b, testosterone, estradiol, luteinizing hormone, follicle-stimulating hormone, and sex hormone-binding globulin in monthly samples from healthy men during a 17-month period: Possible effects of seasons. J Clin Endocrinol Metab (2003) 88:932–7. doi: 10.1210/jc.2002-020838
56. Auger J, Eustache F, Ducot B, Blandin T, Daudin M, Diaz I, et al. Intra- and inter-individual variability in human sperm concentration, motility and vitality assessment during a workshop involving ten laboratories. Hum Reprod (2000) 15(11):2360–8. doi: 10.1093/humrep/15.11.2360
57. Barr DB, Wang RY, Needham LL. Biologic monitoring of exposure to environmental chemicals throughout the life stages: Requirements and issues for consideration for the national children’s study. Environ Health Perspect (2005) 113(8):1083–91. doi: 10.1289/ehp.7617
58. Vorkamp K, Castaño A, Antignac JP, Boada LD, Cequier E, Covaci A, et al. Biomarkers, matrices and analytical methods targeting human exposure to chemicals selected for a European human biomonitoring initiative. Environ Int (2021) 146(August 2020):106082. doi: 10.1016/j.envint.2020.106082
Keywords: male reproductive health, reproductive hormones, prenatal exposure, bisphenol A, benzophenone-3, endocrine disruption
Citation: Holmboe SA, Scheutz Henriksen L, Frederiksen H, Andersson A-M, Priskorn L, Jørgensen N, Juul A, Toppari J, Skakkebæk NE and Main KM (2022) Prenatal exposure to phenols and benzophenones in relation to markers of male reproductive function in adulthood. Front. Endocrinol. 13:1071761. doi: 10.3389/fendo.2022.1071761
Received: 16 October 2022; Accepted: 28 November 2022;
Published: 09 December 2022.
Edited by:
Yi-Xin Wang, Harvard University, United StatesReviewed by:
Chen Yingjun, Southern Medical University, ChinaSun Bin, Huazhong University of Science and Technology, China
Copyright © 2022 Holmboe, Scheutz Henriksen, Frederiksen, Andersson, Priskorn, Jørgensen, Juul, Toppari, Skakkebæk and Main. This is an open-access article distributed under the terms of the Creative Commons Attribution License (CC BY). The use, distribution or reproduction in other forums is permitted, provided the original author(s) and the copyright owner(s) are credited and that the original publication in this journal is cited, in accordance with accepted academic practice. No use, distribution or reproduction is permitted which does not comply with these terms.
*Correspondence: Stine A. Holmboe, c3RpbmUuYWdlcmdhYXJkLmhvbG1ib2VAcmVnaW9uaC5kaw==
†ORCID: Hanne Frederiksen, orcid.org/0000-0002-3180-9879