- Shanghai Clinical Research Center of Bone Disease, Department of Osteoporosis and Bone Diseases, Shanghai Sixth People’s Hospital Affiliated to Shanghai Jiao Tong University School of Medicine, Shanghai, China
Aim: To investigate the relationship between gene polymorphisms involved in the vitamin D metabolic pathway and serum uric acid (UA) levels in Chinese women.
Methods: Fifteen key genes within the vitamin D metabolic pathway were screened using 96 single nucleotide polymorphisms in a group of 1,206 (37.96 ± 13.08 years) unrelated healthy Chinese women (aged 20–85 years). Blood and urine tests were performed at the same time. The Wilcoxon Mann–Whitney test was used to compare groups aged ≤50 years and >50 years. The mean serum UA values were computed within each group of homozygous referent, heterozygous, and homozygous variant genotypes for each single nucleotide polymorphism.
Results: The exclusion process left 1,169 participants (38.16 ± 13.13 years) for analysis. One single nucleotide polymorphism in the CUBN gene (rs3740165) was identified as being significantly associated with serum UA levels in the group aged over 50 years. The wild type (C/C) population had higher serum UA levels in this group (P<0.001). In women aged over 50 years, allele C was associated with a higher risk of hyperuricemia than allele T (odds ratio 2.752, 95% confidence interval 1.458–5.192; P = 0.002). There was also a higher risk of hyperuricemia in genotype TC + CC compared with genotype TT (odds ratio 3.326, 95% confidence interval 1.513–7.313; P = 0.003) in women over 50 years of age.
Conclusion: The results suggest that the CUBN gene contributes to variability in serum UA levels in healthy Chinese Han women over 50 years of age.
1. Introduction
Historically, serum uric acid (UA) has been known as a waste by-product that in excess may cause kidney stones and gouty arthritis (1, 2). It also seems to play an important role in multiple metabolic, hemodynamic, and homeostatic abnormalities, such as type 2 diabetes, cardiovascular disorders, hypertension, and dyslipidemia (3–6). Many studies indicate that UA is a key factor in the development of the metabolic syndrome (7–9).
Genetic research has demonstrated that UA levels are highly heritable (10). Three UA membrane transporters, SLC22A12, SLC2A9, and ABCG2, play key roles in the regulation of serum UA (11–16). About 30 gene variants have been identified, explaining nearly 7% of the variation in serum UA concentration by genome-wide association analyses (17). Thus, a large number of variations have not been explained.
Vitamin D deficiency is a major health problem worldwide. Over 1 billion individuals worldwide have been diagnosed with vitamin D deficiency or insufficiency (18), which causes both skeletal (19, 20) and extra-skeletal diseases (21, 22). Animal and human studies have suggested that the metabolic pathways of UA and vitamin D are related. In rats, induction of increased circulating UA suppresses 1α-hydroxylase, which leads to increased parathyroid hormone (PTH) levels and decreased 1α,25-dihydroxyvitamin D levels (22). Furthermore, in humans, administration of allopurinol reduces serum UA levels, which leads to a reduction in PTH levels and an increase in 1α,25-dihydroxyvitamin D levels (23, 24). Vitamin D insufficiency is significantly associated with elevated UA levels among postmenopausal Chinese Han women (24).
The GC (GC vitamin D binding protein) gene is an important gene in the vitamin D metabolic pathway (25, 26). Thakkinstian et al. suggested a potential causal association between the GC gene and UA through the 25-hydroxyvitamin D3 (25(OH)D) mediator (27). We, therefore, hypothesized that serum UA is associated with gene polymorphisms within the vitamin D metabolic pathway.
There are several genes within the vitamin D metabolic pathway, of which CUBN is one. CUBN encodes the protein cubilin, which is located in the epithelium of the intestine and kidneys. It acts as a co-transporter and helps in the uptake of lipoprotein, iron, and vitamins. In the proximal tubule cells, cubilin takes part in reabsorption of vitamin D binding protein from glomerular filtrates and assists in the synthesis of 1α,25-dihydroxyvitamin D3 (28, 29). CUBN consists of an amino terminal region involved in protein trimerization and membrane anchoring, followed by eight epidermal-growth-factor-type B repeats and 27 contiguous CUB (complement C1r/C1s, Uegf, BMP1) domains. CUBN has an amino terminal furin cleavage site, which is probably involved in proteolytic processing in the trans-Golgi and possibly in shedding the plasma-membrane-bound protein in the gut (30).
We screened 96 common variants of genes involved in the vitamin D metabolic pathway, which we reported previously (25), to explore the association between these gene variations and serum UA levels in 1,206 Chinese women.
2. Materials and methods
2.1. Study participants
A total of 1,518 Chinese woman living in Shanghai were recruited from several community centers. All participants were of Han ethnicity and in generally good health. The average menopausal age among 134,010 Chinese women was reported as 48.6 years old (31). Women’s serum UA levels increase after menopause; therefore, we chose the age of 50 years to divide participants into two groups.
We conducted a survey that provided the participants’ sex, age, and medical histories. After exclusion criteria were met, we recruited a total of 1,206 women (aged 20–85 years) with complete information. We excluded individuals with an estimated glomerular filtration rate <30 mL/min/1.73 m2 (32), hepatic disease, cancer, gout, hyperuricemia, myocardial infarction, heart failure, stroke, hypertensive encephalopathy, retinal hemorrhage, being pregnant, or any of the following medications that may alter serum urate (diuretic, allopurinol, corticosteroids, beta blockers, antituberculotics, quinolones, sulfonylureas, metformin, immunosuppressants, nicotinic acid, pancreatin, tumor chemotherapeutics, aspirin and levodopa). The study was approved by the Ethics Committee of the Shanghai Jiao Tong University Affiliated Sixth People’s Hospital. All participants signed informed consent forms before entering the study.
2.2. Laboratory measurements
We obtained fasting blood samples from the participants between 8:00 h and 10:00 h in the morning. Serum levels of calcium, phosphorus, and alkaline phosphatase were tested using a Hitachi 7600–020 automatic biochemistry analyzer. The intra-assay and inter-assay coefficients of variations (CVs) were 1.5% and 2.0% for calcium, 2.1% and 2.3% for phosphorus, and 2.5% and 4.5% for alkaline phosphatase. Serum creatinine was detected with the sarcosine oxidase-PAP method using the creatinine kit from the Shanghai KEHUA Bio-engineering Corporation (China). Serum UA was measured with an enzymatic method using the Pureauto SUA kit (Japan). The CV of detecting UA was lower than 5%. The linear range of UA is 11.896–4758.4 μmol/L. Total cholesterol, triglycerides, and glucose were measured using kits from Roche Diagnostics. The following measurements of biochemical markers were also made using electrochemiluminescence immunoassays from Roche Diagnostics: total P1NP kit for serum procollagen type 1 N-terminal propeptide (P1NP), β-crosslaps kit for β-cross-linked C-telopeptide of type 1 collagen (β-CTX), 25-hydroxyvitamin D3 kit for 25(OH)D, and intact PTH kit for PTH. The intra-assay and inter-assay CVs were 2.3% and 2.8% for P1NP, 2.5% and 3.5% for β-CTX, and 1.4% and 2.9% for PTH, respectively (26). The lower limit of detection of 25(OH)D was 4 ng/mL (10 mmol/L). The intra-assay CVs for 25(OH)D were 5.7% at the level of 25.2 ng/mL, 5.7% at the level of 39.9 ng/mL, and 5.4% at the level of 65.6 ng/mL. The inter-assay CVs for 25(OH)D were 9.9% at the level of 25.2 ng/mL, 7.3% at the level of 39.9 ng/mL, and 6.9% at the level of 65.6 ng/mL (25).
2.3. Candidate genes and tag SNP selection
Fifteen candidate genes were selected according to the following criteria (1): biological importance in vitamin D transportation, metabolism, or degradation; and (2) evidence of a significant association in a previous genome-wide association analysis. The selected genes were CUBN, GC, CYP24A1, ACADSB, CYP2J2, NADSYN1, CYP2R1, CYP11A1, DHCR7, CYP2C9, PTH, CYP1A1, CYP3A4, CYP27A1, and CYP27B1. The basic characteristics of these genes are shown in Table 1. The detailed functions of the candidate genes have been described previously (25). For the studied genes, tagging single nucleotide polymorphisms (SNPs) were selected from the International HapMap Project (http://www.hapmap.org/cgi-perl/gbrowse/hapmap3_B36), based on the criteria described in our former research (25).
2.4. SNP genotyping
Genomic DNA was extracted from peripheral blood samples and purified using routine methods. Genotyping was performed using the high-throughput Sequenom genotyping platform (MassARRAY matrix-assisted laser desorption/ionization-time of flight mass spectroscopy system; Sequenom, San Diego, CA, USA). Genotype frequencies were tested against the Hardy-Weinberg equilibrium using the chi-squared test.
2.5. Statistical analysis
Routine statistical analysis was performed using R software (https://www.r-project.org/). Baseline characteristics of participants in each age group were compared. The Kolmogorov–Smirnov test was used to evaluate data distribution for normality. All the data were skewed distributed; therefore, the Wilcoxon Mann–Whitney test was performed and all values were expressed as median and interquartile range. The mean serum UA values were computed within each group of homozygous referent, heterozygous, and homozygous variant genotypes for each SNP. A linear regression model was used to analyze the association between SNPs and serum UA. We used the LOWESS method to show the changes in serum UA in three different genotype carriers with age growth. The Bonferroni correction was used to adjust for multiple testing. The results were regarded as statistically significant at a value of P<0.05.
3. Results
A total of 1,206 participants were recruited. Thirty-seven participants were excluded because less than 95% of the markers were successfully genotyped across all the SNPs. This exclusion process left 1,169 participants (38.16 ± 13.13 years) for analysis (aged 20–85 years). The inclusion process of the participants is shown in Figure 1. Among the women included in the current analysis, 940 (80.4%) were aged ≤50 years and 229 (19.6%) participants were aged over 50 years. The clinical characteristics and mean laboratory values of the 1,169 women are shown in Table 2. Ninety-five SNPs passed quality control criteria with genotyping call rates of greater than 90%. The basic characteristics of the SNPs are listed in the supplementary information (Table S1).
Generally, women aged over 50 years had a higher body mass index and higher serum levels of UA, total cholesterol, triglycerides, P1NP, and β-CTX as well as a lower estimated glomerular filtration rate than women aged ≤50 years (P<0.001, Table 2). No association between biochemical markers including calcium, phosphorus, alkaline phosphatase, glucose, total cholesterol, triglycerides, PTH, 25(OH)D, P1NP, and β-CTX level with the studied polymorphisms was found.
One SNP in the CUBN gene (rs3740165) was identified as being significantly associated with serum UA in the group aged over 50 years. The wild-type (C/C) population (n=6) had higher serum UA levels in this group. The average serum UA level was 345.14 μmol/L in the wild type (C/C) population, 298.49 μmol/L in the heterozygous-type (T/C) population (n=55), and 271.20 μmol/L in the mutant (T/T) population (n=168) (P=0.00023). The findings suggested that the presence of C alleles could increase the serum UA level of Chinese Han women aged over 50 years. The mean and standard error of serum UA levels in various genotypes after age stratification is shown in Table 3. There were no significant associations between the rs3740165 polymorphism and serum UA levels in all participants or in the group of women aged ≤50 years.

Table 3 The mean and standard error of serum uric acid for different genotypes after age stratification.
The number of participants with the rs3740165 genotypes TT, TC, and CC was 878 (75.1%), 266 (22.8%), and 25 (2.1%), respectively. The number of participants with the genotype CC was small; therefore, we grouped the study participants into two groups, the TT group and the TC + CC group, and we used logistic regression to perform further analysis. In the group over 50 years of age, the C allele was associated with a higher risk of hyperuricemia than allele T (odds ratio 2.752, 95% confidence interval 1.458–5.192; P = 0.002). There was also a higher risk of hyperuricemia in genotype TC + CC compared with genotype TT (odds ratio 3.326, 95% confidence interval 1.513–7.313; P = 0.003) in the over 50 years group (Table 4). The changes in serum UA in the three different genotype carriers with age growth is presented in Figure 2.
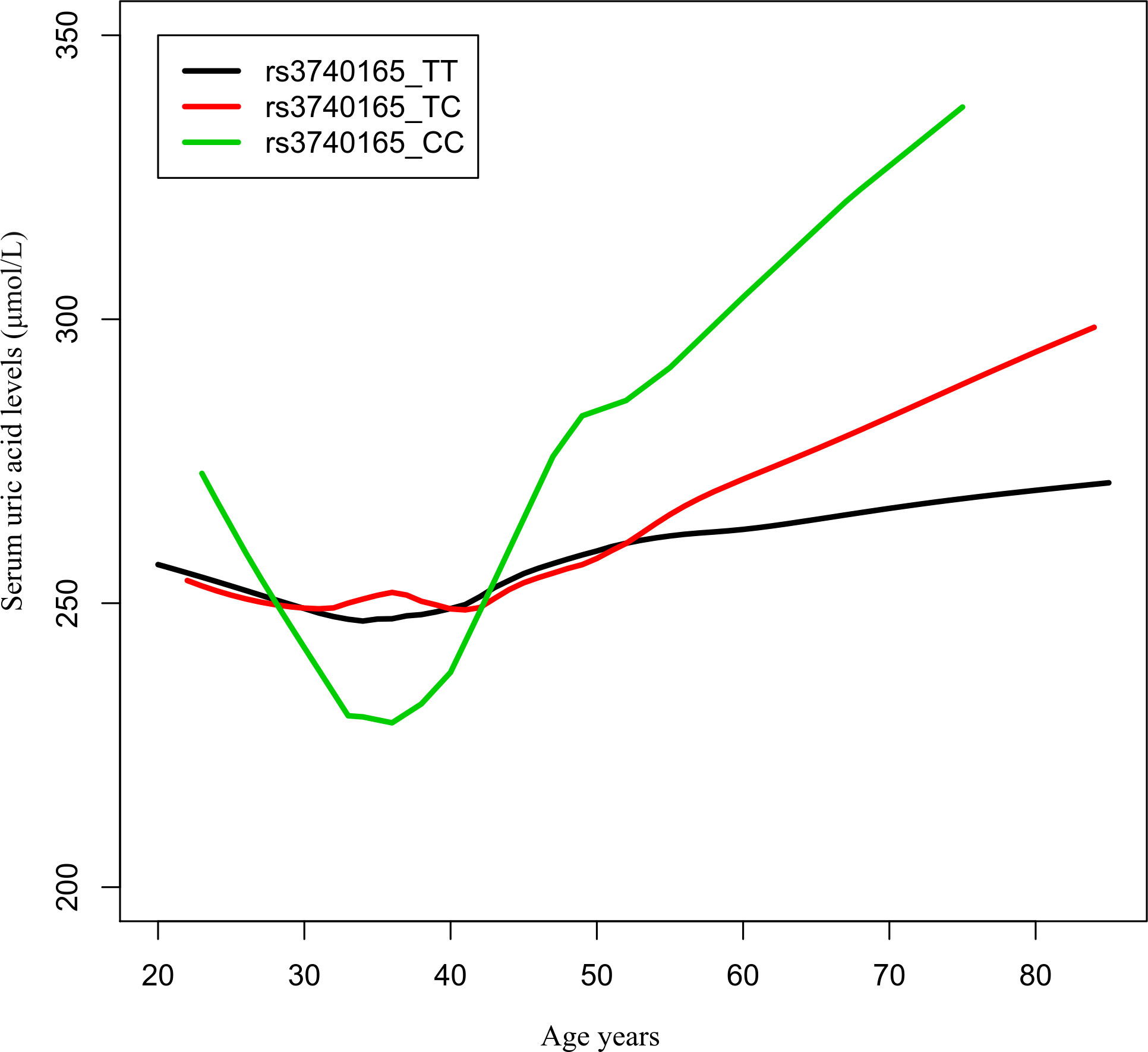
Figure 2 Age−urea acid curves of rs3740165 genotypes. The changes in serum uric acid in three different genotype carriers with age growth.
4. Discussion
The present study investigated the association of 15 candidate genes in the vitamin D metabolic pathway with serum UA levels. The serum UA level was significantly higher in women over 50 years versus women aged ≤50 years. Postmenopausal women have a higher body mass index, higher blood lipid levels, and higher levels of bone turnover markers (33). We observed that genetic variation in the CUBN gene is associated with serum UA levels in Chinese Han women over 50 years. Individuals with the C allele of rs3740165 had significantly higher serum UA levels than did T allele carriers. To the best of our knowledge, this is the first study to investigate the common allelic variant in the CUBN gene and its association with serum UA levels.
The CUBN gene is associated with several diseases. CUBN gene mutation may cause Imerslund–Grasbeck syndrome (34, 35) (OMIM 261100), which is a rare autosomal recessive disease characterized by vitamin B12 malabsorption resulting in megaloblastic anemia and asymptomatic proteinuria. In addition, links have been found between CUBN and the development and progression of cancers, such as gastric cancer (36), breast cancer (37), renal cell carcinoma (38), and colorectal cancer (39).
The CUBN gene is also associated with proteinuria and renal function. For example, human C-terminal CUBN variants are associated with chronic proteinuria and normal renal function (40). Ahluwalia et al. identified a rare missense (A1690V) variant in CUBN associated with albuminuria in a European meta-analysis (41). Serum UA level is one of the most sensitive indicators of human renal function. No other reports exist concerning the relationship between the CUBN genotype and serum UA level.
The SNP in the CUBN gene (rs3740165) was identified as being significantly associated with serum UA in women over 50 years, but not in the group aged ≤50 years. This difference may exist for the following reasons. First, quantitative trait loci that regulate serum UA levels in humans may be sex-specific, site-specific, and age-specific. Second, serum UA level is a complex trait, and these are generally controlled by common polymorphisms, resulting in more subtle changes in gene function or expression.
There are genetic factors in urinary serum UA excretion, and several genes are involved in excretion of UA in the urine as well as reabsorption within the kidney; these include SLC2A9, SLC22A12, SLC17A1, SLC17A3, and ABCG2 (13, 16, 42–44). The GC gene also has a potential causal association with UA (27). Our findings increase the gene spectrum of serum-UA-related genes and open new avenues for a better understanding of the heritable basis of hyperuricemia.
Our study had the following strengths (1): dense markers and those previously reported in genome-wide association analyses within 15 candidate genes involved in vitamin D metabolism were selected (2); by conducting this study in a healthy population, the analysis of the genetic impact on serum UA levels was not confounded by the potential impact of disease; and (3) in order to avoid the influence of age on serum UA levels, we analyzed the data in groups of women ≤50 years and >50 years.
Nevertheless, the results of our study should be interpreted with the following limitations in mind. First, the main weakness of the study was the small sample group and the difference in the numbers of women aged >50 years and ≤50 years. We should enlarge our sample size in future studies to improve statistical power and to avoid bias. Second, owing to the difficulty in recruiting male participants, the participants in this study were all women. We plan to add male data in order to analyze data from both sexes. Third, UA levels vary across the menstrual cycle (45), but this aspect was not considered in the study. We will unify the menstrual cycle phase when testing the UA level of premenopausal women in future research.
In conclusion, our study provides evidence that genetic variation in the CUBN gene is associated with serum UA levels in Chinese Han women over 50 years of age. We suggest that further investigations are conducted including larger sample sizes, male participants, and other ethnic groups.
Data availability statement
The raw data supporting the conclusions of this article will be made available by the authors, without undue reservation. Requests to access these datasets should be directed toZ3VqaWVtZWk4MUAxNjMuY29t.
Ethics statement
The studies involving human participants were reviewed and approved by the Ethics Committee of the Shanghai Jiao Tong University Affiliated Sixth People’s Hospital. The participants provided their written informed consent to participate in this study.
Author contributions
ZZ designed the study and revised the manuscript. JG collected the blood samples, analyzed the genetic results, did the statistical analysis, and draft the manuscript. HY, CW, HZ, and WH collected clinical information of the participants. All authors read and approved the final manuscript.
Acknowledgments
We are grateful to our participants in this study.
Funding
The study was supported by the National Key Research and Development Program of China (2018YFA0800801), the National Natural Science Foundation of China (NSFC) (82270933, 81974126, 82070903, 81870618, 82170895).
Conflict of interest
The authors declare that the research was conducted in the absence of any commercial or financial relationships that could be construed as a potential conflict of interest.
Publisher’s note
All claims expressed in this article are solely those of the authors and do not necessarily represent those of their affiliated organizations, or those of the publisher, the editors and the reviewers. Any product that may be evaluated in this article, or claim that may be made by its manufacturer, is not guaranteed or endorsed by the publisher.
Supplementary material
The Supplementary Material for this article can be found online at: https://www.frontiersin.org/articles/10.3389/fendo.2022.1059964/full#supplementary-material
References
1. Edwards NL. The role of hyperuricemia and gout in kidney and cardiovascular disease. Cleve Clin J Med (2008) 75(Suppl 5):S13–6. doi: 10.3949/ccjm.75.suppl_5.s13
2. Mandell BF. Clinical manifestations of hyperuricemia and gout. Cleve Clin J Med (2008) 75(Suppl 5):S5–8. doi: 10.3949/ccjm.75.Suppl_5.S5
3. Johnson RJ, Kang DH, Feig D, Kivlighn S, Kanellis J, Watanabe S, et al. Is there a pathogenetic role for uric acid in hypertension and cardiovascular and renal disease? Hypertension (2003) 41:1183–90. doi: 10.1161/01.hyp.0000069700.62727.c5
4. Lippi G, Targher G, Montagnana M, Salvagno GL, Guidi GC. High serum uric acid as a novel risk factor for type 2 diabetes: Response to dehghan et al. Diabetes Care (2008) 31:e68. doi: 10.2337/dc08-0468
5. Holme I, Aastveit AH, Hammar N, Jungner I, Walldius G. Uric acid and risk of myocardial infarction, stroke and congestive heart failure in 417,734 men and women in the apolipoprotein mortality risk study (Amoris). J Intern Med (2009) 266:558–70. doi: 10.1111/j.1365-2796.2009.02133.x
6. Zhang S, Du T, Li M, Lu H, Lin X, Yu X. Combined effect of obesity and uric acid on nonalcoholic fatty liver disease and hypertriglyceridemia. Medicine (2017) 96:e6381. doi: 10.1097/md.0000000000006381
7. Oda E, Kawai R, Sukumaran V, Watanabe K. Uric acid is positively associated with metabolic syndrome but negatively associated with diabetes in Japanese men. Intern Med (2009) 48:1785–91. doi: 10.2169/internalmedicine.48.2426
8. Puig JG, Martinez MA. Hyperuricemia, gout and the metabolic syndrome. Curr Opin Rheumatol (2008) 20:187–91. doi: 10.1097/BOR.0b013e3282f4b1ed
9. Nakagawa T, Cirillo P, Sato W, Gersch M, Sautin Y, Roncal C, et al. The conundrum of hyperuricemia, metabolic syndrome, and renal disease. Intern Emerg Med (2008) 3:313–8. doi: 10.1007/s11739-008-0141-3
10. Yang Q, Guo CY, Cupples LA, Levy D, Wilson PW, Fox CS. Genome-wide search for genes affecting serum uric acid levels: The framingham heart study. Metabolism (2005) 54:1435–41. doi: 10.1016/j.metabol.2005.05.007
11. Iwai N, Mino Y, Hosoyamada M, Tago N, Kokubo Y, Endou H. A high prevalence of renal hypouricemia caused by inactive Slc22a12 in Japanese. Kidney Int (2004) 66:935–44. doi: 10.1111/j.1523-1755.2004.00839.x
12. Jang WC, Nam YH, Park SM, Ahn YC, Park SH, Choe JY, et al. T6092c polymorphism of Slc22a12 gene is associated with serum uric acid concentrations in Korean Male subjects. Clin Chim Acta (2008) 398:140–4. doi: 10.1016/j.cca.2008.09.008
13. Ware EB, Riehle E, Smith JA, Zhao W, Turner ST, Kardia SL, et al. Slc2a9 genotype is associated with Slc2a9 gene expression and urinary uric acid concentration. PloS One (2015) 10:e0128593. doi: 10.1371/journal.pone.0128593
14. Stark K, Reinhard W, Neureuther K, Wiedmann S, Sedlacek K, Baessler A, et al. Association of common polymorphisms in Glut9 gene with gout but not with coronary artery disease in a Large case-control study. PloS One (2008) 3:e1948. doi: 10.1371/journal.pone.0001948
15. Dehghan A, Kottgen A, Yang Q, Hwang SJ, Kao WL, Rivadeneira F, et al. Association of three genetic loci with uric acid concentration and risk of gout: A genome-wide association study. Lancet (2008) 372:1953–61. doi: 10.1016/s0140-6736(08)61343-4
16. Yamagishi K, Tanigawa T, Kitamura A, Kottgen A, Folsom AR, Iso H. The Rs2231142 variant of the Abcg2 gene is associated with uric acid levels and gout among Japanese people. Rheumatol (Oxford) (2010) 49:1461–5. doi: 10.1093/rheumatology/keq096
17. Kottgen A, Albrecht E, Teumer A, Vitart V, Krumsiek J, Hundertmark C, et al. Genome-wide association analyses identify 18 new loci associated with serum urate concentrations. Nat Genet (2013) 45:145–54. doi: 10.1038/ng.2500
19. Rovner AJ, Miller RS. Vitamin d deficiency and insufficiency in children with osteopenia or osteoporosis. Pediatrics (2008) 122:907–8. doi: 10.1542/peds.2008-1743
21. Artaza JN, Mehrotra R, Norris KC. Vitamin d and the cardiovascular system. Clin J Am Soc Nephrol (2009) 4:1515–22. doi: 10.2215/cjn.02260409
22. Mathieu C, Gysemans C, Giulietti A, Bouillon R. Vitamin d and diabetes. Diabetologia (2005) 48:1247–57. doi: 10.1007/s00125-005-1802-7
23. Yilmaz H, Kaya M, Sahin M, Delibasi T. Is vitamin d status a predictor glycaemic regulation and cardiac complication in type 2 diabetes mellitus patients? Diabetes Metab Syndr (2012) 6:28–31. doi: 10.1016/j.dsx.2012.05.007
24. Peng H, Li H, Li C, Chao X, Zhang Q, Zhang Y. Association between vitamin d insufficiency and elevated serum uric acid among middle-aged and elderly Chinese han women. PloS One (2013) 8:e61159. doi: 10.1371/journal.pone.0061159
25. Zhang Z, He JW, Fu WZ, Zhang CQ, Zhang ZL. An analysis of the association between the vitamin d pathway and serum 25-hydroxyvitamin d levels in a healthy Chinese population. J Bone Miner Res (2013) 28:1784–92. doi: 10.1002/jbmr.1926
26. Hu WW, Zhang Z, He JW, Fu WZ, Wang C, Zhang H, et al. Establishing reference intervals for bone turnover markers in the healthy shanghai population and the relationship with bone mineral density in postmenopausal women. Int J Endocrinol (2013) 2013:513925. doi: 10.1155/2013/513925
27. Thakkinstian A, Anothaisintawee T, Chailurkit L, Ratanachaiwong W, Yamwong S, Sritara P, et al. Potential causal associations between vitamin d and uric acid: Bidirectional mediation analysis. Sci Rep (2015) 5:14528. doi: 10.1038/srep14528
28. Kaseda R, Hosojima M, Sato H, Saito A. Role of megalin and cubilin in the metabolism of vitamin D(3). Ther Apher Dial (2011) 15(Suppl 1):14–7. doi: 10.1111/j.1744-9987.2011.00920.x
29. Nykjaer A, Fyfe JC, Kozyraki R, Leheste JR, Jacobsen C, Nielsen MS, et al. Cubilin dysfunction causes abnormal metabolism of the steroid hormone 25(Oh) vitamin D(3). Proc Natl Acad Sci USA (2001) 98:13895–900. doi: 10.1073/pnas.241516998
30. Mahmood A, Shao JS, Alpers DH. Rat enterocytes secrete slps containing alkaline phosphatase and cubilin in response to corn oil feeding. Am J Physiol Gastrointest Liver Physiol (2003) 285:G433–41. doi: 10.1152/ajpgi.00466.2002
31. Yang L, Lin L, Kartsonaki C, Guo Y, Chen Y, Bian Z, et al. Menopause characteristics, total reproductive years, and risk of cardiovascular disease among Chinese women. Circ Cardiovasc Qual Outcomes (2017) 10:e004235. doi: 10.1161/circoutcomes.117.004235
32. Levey AS, Stevens LA, Schmid CH, Zhang YL, Castro AF 3rd, Feldman HI, et al. A new equation to estimate glomerular filtration rate. Ann Intern Med (2009) 150:604–12. doi: 10.7326/0003-4819-150-9-200905050-00006
33. Wingrove CS, Walton C, Stevenson JC. The effect of menopause on serum uric acid levels in non-obese healthy women. Metabolism (1998) 47:435–8. doi: 10.1016/s0026-0495(98)90056-7
34. Ciancio JIR, Furman M, Banka S, Grunewald S. Profound vitamin d deficiency in four siblings with imerslund-grasbeck syndrome with homozygous cubn mutation. JIMD Rep (2019) 49:43–7. doi: 10.1002/jmd2.12072
35. Hauck FH, Tanner SM, Henker J, Laass MW. Imerslund-gräsbeck syndrome in a 15-Year-Old German girl caused by compound heterozygous mutations in cubn. Eur J Pediatr (2008) 167:671–5. doi: 10.1007/s00431-007-0571-3
36. Zhao L, Wei Y, Song A, Li Y. Association study between genome-wide significant variants of vitamin B12 metabolism and gastric cancer in a han Chinese population. IUBMB Life (2016) 68:303–10. doi: 10.1002/iub.1485
37. Wang H, Zhao L, Liu H, Luo S, Akinyemiju T, Hwang S, et al. Variants in Snai1, Amdhd1 and cubn in vitamin d pathway genes are associated with breast cancer risk: A Large-scale analysis of 14 gwass in the drive study. Am J Cancer Res (2020) 10:2160–73.
38. Gremel G, Djureinovic D, Niinivirta M, Laird A, Ljungqvist O, Johannesson H, et al. A systematic search strategy identifies cubilin as independent prognostic marker for renal cell carcinoma. BMC Cancer (2017) 17:9. doi: 10.1186/s12885-016-3030-6
39. Al-Tassan NA, Whiffin N, Hosking FJ, Palles C, Farrington SM, Dobbins SE, et al. A new gwas and meta-analysis with 1000genomes imputation identifies novel risk variants for colorectal cancer. Sci Rep (2015) 5:10442. doi: 10.1038/srep10442
40. Bedin M, Boyer O, Servais A, Li Y, Villoing-Gaudé L, Tête MJ, et al. Human c-terminal cubn variants associate with chronic proteinuria and normal renal function. J Clin Invest (2020) 130:335–44. doi: 10.1172/jci129937
41. Ahluwalia TS, Schulz CA, Waage J, Skaaby T, Sandholm N, van Zuydam N, et al. A novel rare cubn variant and three additional genes identified in europeans with and without diabetes: Results from an exome-wide association study of albuminuria. Diabetologia (2019) 62:292–305. doi: 10.1007/s00125-018-4783-z
42. Enomoto A, Kimura H, Chairoungdua A, Shigeta Y, Jutabha P, Cha SH, et al. Molecular identification of a renal urate anion exchanger that regulates blood urate levels. Nature (2002) 417:447–52. doi: 10.1038/nature742
43. Reginato AM, Mount DB, Yang I, Choi HK. The genetics of hyperuricaemia and gout. Nat Rev Rheumatol (2012) 8:610–21. doi: 10.1038/nrrheum.2012.144
44. Hollis-Moffatt JE, Phipps-Green AJ, Chapman B, Jones GT, van Rij A, Gow PJ, et al. The renal urate transporter Slc17a1 locus: Confirmation of association with gout. Arthritis Res Ther (2012) 14:R92. doi: 10.1186/ar3816
Keywords: uric acid, vitamin D, pathway, polymorphism, gene
Citation: Gu J, Yue H, Wang C, Zhang H, Hu W and Zhang Z (2022) Vitamin D pathway gene variation rs3740165 is associated with serological uric acid levels in healthy Chinese women. Front. Endocrinol. 13:1059964. doi: 10.3389/fendo.2022.1059964
Received: 02 October 2022; Accepted: 25 November 2022;
Published: 13 December 2022.
Edited by:
Giacomina Brunetti, University of Bari Aldo Moro, ItalyReviewed by:
Nan Jiang, Southern Medical University, ChinaMarta Karaźniewicz-Łada, Poznan University of Medical Sciences, Poland
Copyright © 2022 Gu, Yue, Wang, Zhang, Hu and Zhang. This is an open-access article distributed under the terms of the Creative Commons Attribution License (CC BY). The use, distribution or reproduction in other forums is permitted, provided the original author(s) and the copyright owner(s) are credited and that the original publication in this journal is cited, in accordance with accepted academic practice. No use, distribution or reproduction is permitted which does not comply with these terms.
*Correspondence: Zhenlin Zhang, emhhbmd6bEBzanR1LmVkdS5jbg==