- 1Department of Fundamental and Community Nursing, School of Nursing, Nanjing Medical University, Nanjing, China
- 2Department of Neurosurgery, The Affiliated Brain Hospital of Nanjing Medical University, Nanjing, China
- 3Department of General Practice, Ninghai Road Community Health Service Center, Nanjing, China
- 4Department of Epidemiology, Shanghai Cancer Institute, Shanghai, China
Background: Non-alcoholic fatty liver disease (NAFLD) is recognized to be closely associated with endoplasmic reticulum stress and mitochondrial dysfunction, while previous studies have emphasized the important role of calcium homeostasis from the mitochondria-associated endoplasmic reticulum membrane (MAM) in the endoplasmic reticulum and mitochondria. This article will assess the association between genetic polymorphisms of Ca2+ transport proteins and molecular chaperones in MAM and NAFLD risk.
Methods: A case-control study was conducted in a community of Nanjing, China during April to December 2020. 2701 subjects were enrolled and genotyped for 6 genetic variants in HSPA5 and ITPR2 genes. Logistic regression analysis was used to assess impact of these variants on NAFLD risk.
Results: After adjusting for age, gender, total cholesterol and glucose, we identified that HSPA5 rs12009 variant genotypes (recessive model: OR= 0.801, 95% CI= 0.652-0.986, P= 0.036), rs430397 variant genotypes (recessive model: OR= 0.546, 95% CI= 0.314-0.950, P= 0.032), and ITPR2 rs11048570 variant genotypes (recessive model: OR= 0.673, 95% CI= 0.453-0.999, P= 0.049) were associated with a reduced risk of NAFLD. Multivariate stepwise regression analysis indicated that gender, glucose, body mass index, triglycerides and favorable alleles were independent influencers of NAFLD (all P< 0.05). The area under the receiver operating characteristic curve was 0.764 (95% CI= 0.745-0.783, P< 0.001).
Conclusion: The variant genotypes of Ca2+ transport-associated genes HSPA5 (rs12009 and rs430397) and ITPR2 (rs11048570) might contribute to the reduction of the NAFLD risk in Chinese Han population, which can provide new insight into NAFLD pathogenesis.
1. Introduction
Non-alcoholic fatty liver disease (NAFLD) has become the leading chronic liver disease, burdening around 24% of the global population (1). Based on recent studies, the prevalence of NAFLD in China is approximately 29.1%-29.8%, similar to the overall level in Asia (29.6%) and gradually approaching that in many Western countries (1–4). NAFLD is an umbrella term that often manifests as liver steatosis in the initial stage, which can spread to non-alcoholic steatohepatitis (NASH), the latter may progress to advanced liver fibrosis, cirrhosis, or hepatocellular carcinoma (HCC) (5). Over the last decades, the growing evidences have shown that NAFLD is a multisystem disease, affecting extra-hepatic organs and regulatory pathways (6), such as type 2 diabetes mellitus (T2DM), cardiovascular disease (CVD) and chronic kidney disease (CKD). Overall, NAFLD contributes to an increasing clinical burden worldwide and may pose a serious threat to people’s health.
Although steady progress has been made in clarifying NAFLD pathogenesis, targeting therapeutics, and facilitating drug development, hard challenges remain. Because of the complex physiological mechanisms and apparent heterogeneity of disease phenotypes, early prevention and healthy lifestyle remain crucial to many patients with NAFLD (7). Besides, there is no standard NAFLD-specific therapy, although some drugs are in advanced stages of development (8). Consequently, it is essential to further investigate the pathogenesis of NAFLD for its early prevention and drug development. the currently well-established “multiple-hit” theory suggests that multiple injuries interacting with genetically susceptible subjects could induce NAFLD, including insulin resistance (IR), endoplasmic reticulum stress (ERS), mitochondrial dysfunction, gut microbiota, nutritional factors, hormones secreted by adipose tissue, as well as genetic and epigenetic factors (9). Meanwhile, the “multiple-hit” theory considers that persistent ERS and mitochondrial dysfunction are important triggers for the development of NAFLD, while IR is central to the pathogenesis of NAFLD (9). In particular, the endoplasmic reticulum and mitochondria are closely related to calcium homeostasis, and the exchange of substances between them and calcium transport has been demonstrated to be associated with NAFLD (10).
Calcium (Ca2+), as the most plentiful mineral in the body, is involved in all important cellular functions at the cellular level, such as cell growth, differentiation, metabolism, motility and gene expression (11). In the context of metabolic regulation, Ca2+ is required for the insulin and glucagon secretion by the pancreas. Conversely, these hormones also control metabolic reactions in target tissues, such as glycogen breakdown, lipid biosynthesis, gluconeogenesis and ATP production, in a Ca2+-dependent manner. Accordingly, the abnormal Ca2+ signaling pathway is thought to be one of the contributing factors to the occurrence of hepatic steatosis (12). Particularly, abnormal Ca2+ transport in the mitochondria-associated endoplasmic reticulum membranes (MAM) region is closely associated with IR, ERS, and mitochondrial dysfunction (13). Since the MAM region is widely involved in cellular activities such as lipid synthesis and transportation, regulation of mitochondrial dynamics, inflammatory vesicle formation, ERS, autophagy and apoptosis (14), which in turn may have important effects on the occurrence and progression of NAFLD. Moreover, recent studies have implicated that Ca2+ signaling in the MAM region can influence hepatic gluconeogenesis, adipogenesis, inflammation, and other biological processes affecting overall metabolism (15), and has been widely explored in the pathogenesis of metabolic diseases such as obesity and T2DM (16). Therefore, we hypothesize that Ca2+ signaling in the MAM region may play an important role in NAFLD pathogenesis.
Growing evidence indicates that numerous proteins and genes involved in Ca2+ signal transduction are present in the MAM region (17), where Ca2+ transport proteins and molecular chaperones can interact to form a Ca2+ transport protein cluster (18). The more common of those are the inositol triphosphate receptor-2 (IP3Rs) on the endoplasmic/sarcoplasmic reticulum and the voltage-dependent anion channel (VDAC) on the outer mitochondrial membrane, containing the encoded protein IP3R2 (gene ITPR2) and the Ca2+ transport protein VDAC1 (gene VDAC1) (17). It has been found that IP3R2 in the MAM region interacts with VDAC1 and enhances the formation of the IP3R2-VDAC1-MICU1 complex, which in turn triggers mitochondrial Ca2+ overload and early hepatic IR (19). Moreover, some other studies have revealed the role of contacts and facilitated exchanges between the ER and the mitochondria through the inositol 1,4,5-trisphosphate receptor, type 2 (ITPR2) in regulating senescence and aging (20). Meanwhile, the MAM region is enriched with molecular chaperone proteins present on the endoplasmic reticulum or mitochondria involved in the regulation of Ca2+ transport proteins, such as glucose-regulated protein 75 (GRP75, gene HSPA9) and 78-kDa glucose-regulated protein (GRP78, gene HSPA5) (21). The chaperone glucose-regulated protein, 78/immunoglobulin binding protein (GRP78/Bip), protects cells from cytotoxicity induced by DNA damage or ERS (22). Indeed, studies indicated that GRP78 may maintain mitochondrial permeability thereby protecting cells from ERS-induced apoptosis (23). Moreover, GRP78 acts as a receptor for a variety of ligands including VDAC, and attenuate ERS (24). In view of the above, polymorphisms of the genes involved in Ca2+ transport may affect the onset of NAFLD by regulating Ca2+ homeostasis.
This study aimed to investigate the correlation between the genetic polymorphisms of Ca2+ transporter gene (ITPR2, VDAC1) and its related chaperones (HSPA5, HSPA9, SIGMAR1, CANX, PPID) and NAFLD in Chinese population, to provide new ideas for further exploring the genetic susceptibility mechanism of NAFLD. And this study screened new biomarkers for NAFLD risk prediction and further provided theoretical basis for screening and early intervention of NAFLD high-risk populations.
2. Methods
2.1. Study participants and design
The sample included NAFLD patients and healthy controls recruited from a certain community in Nanjing during April to December 2020, and the research team recorded and linked with the medical information management center and the chronic disease management department of the district, and screened out the eligible target population according to the diagnosis and admission criteria. All participants gave informed consent.
The inclusion criteria were as follows: (1) Han Chinese; (2) age ≥18 years old with informed consent; (3) those who are capable of giving fasting blood samples; (4) those who are able to communicate and complete questionnaires. Exclusion criteria were: (1) those with infection, acute and chronic autoimmune diseases, malignant tumors; (2) those with a history of viral hepatitis, primary liver cancer, alcohol-related liver disease and other liver diseases; excessive drinking (male ≥30g/d, female ≥20g/d); (3) those who were expected to undergo liver transplantation within one year, or those who had complications such as variceal bleeding or ascites in the advanced stage of liver disease; (4) those with type 2 diabetes, obesity and metabolic syndrome; (5) those who had used drugs or present exacerbated steatohepatitis (e.g., glucocorticoids); (6) patients with a history of psychiatric disorders.
NAFLD cases were diagnosed according to the Guideline of prevention and treatment for non-alcoholic fatty liver disease: a 2018 update (25). The following criteria were specifically required: (1) no history of alcohol consumption or average alcohol consumption < 30g per day for men and < 20g per day for women; (2) exclusion of specific diseases that can result in fatty liver, including viral hepatitis, total parenteral nutrition, hepatomegaly and drug-related liver disease; (3) histological changes on liver biopsy were consistent with the pathological diagnostic criteria for fatty liver disease. Given the difficulty in acquiring a hepatic histological diagnosis, in addition to the first two points, NAFLD could also be diagnosed if any one or two of the following features were present: (i) the hepatic imaging manifestations meet the imaging diagnostic criteria for diffuse fatty liver and there is no other explanation; (ii) patients with metabolic syndrome-related components, such as visceral obesity, hyperglycemia, blood lipid disorder, and hypertension, presenting with unexplained serum alanine aminotransferase (ALT) and/or serum aspartate aminotransferase (AST) and γ-glutamyl transpeptidase (GGT) for more than six months. The controls were collected from the same community during the study period and randomly assigned to the control group by the frequency matching method. Namely, the control group was proportionally matched to the case group based on the same distribution characteristics of age ( ± 3 years) and sex to ensure that the matching factors were distributed in the same proportion in both groups.
Based on the review of literature, we estimated the frequency of gene variant in the general population was 10%-20%, odds ratio (OR) was 1.5, two-tailed test a was 0.05, and test power (1-b) was 80%. Finally, it was estimated by the NCSS-PASS 11 software (Dawson version; Kaysville, Ut) that the minimum sample size was 534. This study had a sample size large enough to guarantee the production of reliable results.
The study was conducted in accordance with the World Medical Association Declaration of Helsinki on Ethical Principles for Medical Research Involving Human Subjects and was approved by the Institutional Ethics Review Committee of Nanjing Medical University (Nanjing, China). Written informed consent was obtained prior to blood testing and genetic analysis.
2.2. Clinical information and blood sample collection
The demographic characteristics and clinical information of all participants were obtained from self-designed scales and electronic medical records. All participants underwent abdominal ultrasound and blood biochemical tests. 5-ml of EDTA-anticoagulated venous blood was collected from the study subjects under fasting conditions in the morning. Centrifuged within 2 hours according to standard methods (4,000 g/min, 10 min), and subsequently serum, leukocytes and erythrocytes were separated into 1.5-ml centrifuge tubes (two copies of each were kept) and frozen at -80°C. The serum was used for further blood biochemical and liver function index tests, while the whole blood was used to extract genomic DNA by magnetic bead method and stored at -20°C for later genotyping tests.
2.3. SNP selection and genotyping
Target single nucleotide polymorphisms (SNPs) screening was performed in the following steps (1): Aiming to investigate expression patterns of Ca2+ transporter protein genes from MAM that may be involved in human liver tissues, database queries and interrogations were performed on the Human Protein Atlas database (https://www.proteinatlas.org/), which reports both protein production and gene expression in different tissues and organs; we also searched the Genotype-Tissue Expression Project (GTEx) (https://gtexportal.org/home/) and the Function Annotation of The Mammalian Genome 5 (FANTOM5) (http://fantom.gsc.riken.jp/5/), which record the results of gene expression and transcription levels. Based on previous literature data about the MAM region, we searched for proteins involved in Ca2+ transport. Some of the search terms are as follows: IP3R1, 2, 3; VDAC1, 2; GRP75; GRP79; CYPD; SIG1R; ERP44; Calnexin; NLRP3, etc. 7 genes (ITPR2, VDAC1, HSPA5, HSPA9, SIGMAR1, CANX, PPID) were finally screened according to their gene expression and transcription levels in liver tissues (Supplementary Table 1). Keeping track of the literature reports to improve the selection of relevant genes is needed (2). Genotype information of Chinese Han population (CHB) for the above 7 genes was downloaded from the Thousand Genome Project database (http://www.1000genomes.org/) (3). Importing information into Haploview 4.2 (Broad Institute, Cambridge, MA, USA), setting Hardy-Weinberg (H-W) p-value cutoff= 0.05, the correlation coefficient r2≥ 0.8, the minor allele frequency (MAF)> 0.05 to obtain the corresponding tagSNPs (4). Searching the NCBI dbSNP database (https://www.ncbi.nlm.nih.gov/snp), the RegulomeDB database (http://www.regulomedb.org/), and the SNP Function Prediction database (https://snpinfo.niehs.nih.gov/snpinfo/snpfunc.html) and other websites in order to screen out taqSNPs with potential functional significance. Finally, 6 tagSNPs (HSPA5-rs1140763, rs12009, rs430397 and ITPR2-rs10771283, rs11048570, rs2230372) were selected for genotyping (Supplementary Table 2). The genotyping assay was performed by TaqMan-based real-time quantitative polymerase chain reaction technique (Applied Biosystems, USA) and on the Light Cycler 480 II Real-Time PCR System (Roche, Switzerland).
The following measures were applied to control the data quality; (1) genotyping was blinded so that all technicians were ignorant of the participants’ clinical data; (2) replicate experiments were performed in a 10% random sample with a 100% replication rate. All SNPs were genotyped with a success rate above 95%. All trials followed the manufacturer’s instructions.
2.4. Statistical analysis
Statistical analyses were performed with SPSS 26.0 (SPSS Inc., Chicago, IL, USA), Stata 16.0 (StataCorp LP, College Station, TX, USA) and genetics software (Haploview 4.2, HAPSTATA 3.0, etc.). Distributions of demographic and clinical characteristics among case and control groups were compared by the Chi-square (χ2) test for categorical data, Student’s t-test for measurement data, or one-way analysis of variance (ANOVA) when appropriate. Logistic regression analysis was used to calculate odds ratio (OR) and 95% confidence interval (95% CI) to assess the associations between genotypes and risk of NAFLD, adjusting for gender, age, total cholesterol (TC), and glucose (GLU). Each SNP was analyzed using codominant model (mutant homozygous type vs. wild homozygous type and heterozygous type vs. wild homozygous type, respectively), dominant model (mutant homozygous type+ heterozygous type vs. wild homozygous type), recessive model (mutant homozygous type vs. heterozygous type+ wild homozygous type), and additive models (mutant homozygous type vs. heterozygous type vs. wild homozygous type). False discovery rate (FDR) corrections were used for multiple comparisons, considering the PFDR≤ 0.25 as modest confidence that the correlation represented a positive result (26). The Cochran-Armitage trend test was used to analyze the combined effect of independent positive SNPs. Stratified analysis of positive SNPs was conducted, and heterogeneity between subgroups was calculated by Q-test. Multivariate stepwise logistic regression analysis was performed to determine independent predictors of NAFLD. A receiver-operating characteristic curve (ROC) represented the risk prediction model for NAFLD, with its predictive power expressed as area under the receiver operating characteristic curve (AUROC). The Hardy Weinberg equilibrium (HWE) was tested using a goodness of-fit χ2-test among case and control subjects. Haploview software was used to estimate Linkage disequilibrium (LD) parameters (i.e., D and r2), and PHASE 2.1 software to analyses haplotype frequencies. All statistical analyses were two-sided with a significance level of P< 0.05.
3. Results
3.1. Basic characteristics of study subjects
A total of 2701 subjects were enrolled in this study. The distribution of demographic characteristics and clinical biochemical indices of the subjects are shown in Table 1. Although we chose to match the characteristics by gender and age (tolerance error of 3 years) and to select the control group in a ratio of approximately 2:1 with cases, the difference in gender was too large and a substantial sample would be lost if matched completely. We therefore ultimately chose to maximize gender matching on the age-matched results and included gender as confounder in the subsequent data analysis to minimize the effect of confounders on the results. There were no statistically significant differences between the two groups regarding mean age, dietary patterns and dietary preferences (all P> 0.05). However, there were statistically significant differences in gender, TC, GLU, triglyceride (TG) and body mass index (BMI) (all P< 0.05).
3.2. Associations of HSPA5 and ITPR2 SNPs with NAFLD risk
Three genetic models (dominant, recessive, and additive models) were applied to elucidate the association between the selected SNPs and susceptibility to NAFLD. The genotype distribution and logistic regression analysis results of the 6 SNPs between the two study groups were shown in Table 2. Logistic regression results adjusted for age, sex, TC, and GLU showed that three SNPs (HSPA5-rs12009, HSPA5-rs430397, and ITPR2-rs11048570) were associated with susceptibility to NAFLD (all P< 0.05). Compared with subjects carrying the rs12009-GG+GA genotype, those carrying the rs12009-AA genotype had a significantly decreased risk of developing NAFLD (recessive model: adjusted OR= 0.801, 95% CI= 0.652-0.986, P= 0.036). Individuals carrying the wild pure genotype rs430397-TT had a significantly reduced risk of NAFLD than those carrying the rs430397-CC genotype (adjusted OR= 0.541, 95% CI= 0.310-0.945, P= 0.031). Meanwhile, individuals carrying the rs430397-CC+CT genotype had a significantly lower risk of NAFLD compared to those carrying the rs430397-TT genotype (recessive model: adjusted OR= 0.546, 95% CI= 0.314-0.950, P= 0.032). We also found that participants with the rs11048570-AA genotype had a reduced susceptibility to NAFLD compared with the rs11048570-GG genotype (adjusted OR= 0.664, 95% CI= 0.444-0.993, P= 0.046). In addition, individuals carrying the rs11048570-AA genotype appear to be less vulnerable to NAFLD compared with the rs11048570-GG+GA genotype (recessive model: adjusted OR= 0.673, 95% CI= 0.453-0.999, P= 0.049). FDR correcting for multiple comparisons outcomes were statistically significant (all PFDR≤ 0.25, Supplementary Table 3). However, no significant association was discovered between HSPA5-rs1140763, ITPR2-rs10771283 and ITPR2-rs2230372 variants (all P> 0.05).
3.3. Combined effect and stratified analysis
As shown in Table 3, the combined effect of rs12009-A, rs430397-T, and rs11048570-A on NAFLD was assessed by counting the allele numbers of these SNPs. The results showed that the 3 alleles were favorable alleles, and participants with “4-6” favorable alleles had a significantly lower risk of NAFLD compared to study participants with “0” protective alleles, adjusted OR= 0.575 (95% CI: 0.390-0.848, P= 0.005).
Subsequently, stratified analyses were conducted to eliminate potential biases regarding sex, age, TC, and GLU as a way to further explore the combined effects of rs12009-A, rs430397-T, and rs11048570-A. Considering the reasonableness of results, age stratification was based on the mean and median age of the sample population; the remaining factors were stratified according to data in the “Recommendations for the prevention and treatment of dyslipidemia” “Practice Guidelines (2020) for Integrated Management of Cardiovascular Disease in Primary Hospitals” and “Guideline of prevention and treatment for nonalcoholic fatty liver disease: a 2018 update” (25, 27, 28). After analysis, a significant association between the favorable alleles and the risk of NAFLD could be found in the male subgroup (adjusted OR= 0.786, 95% CI= 0.649-0.952, P= 0.014) and in the age> 42 years subgroup (adjusted OR= 0.765, 95% CI= 0.597-0.980, P= 0.034), which were showed in Table 4. Besides, heterogeneity test showed no statistical significance in all subgroups (all P> 0.05).
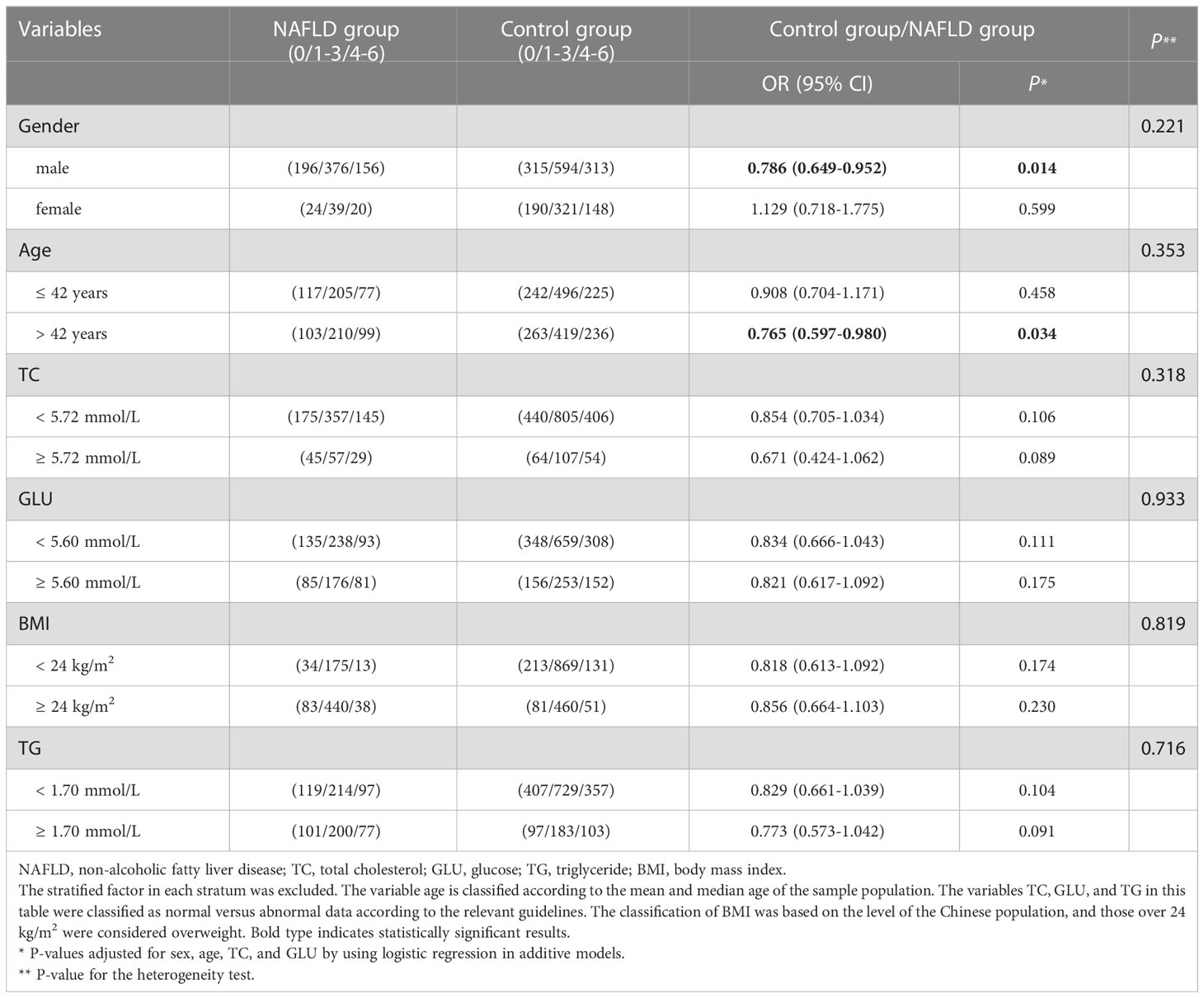
Table 4 Stratified analysis of the association of the combined effects of HSPA5-rs12009, HSPA5-rs430397, and ITPR2-rs11048570 with NAFLD risk.
3.4. Haplotype analysis of the combined effect of HSPA5 SNPs on susceptibility of NAFLD
Since the above analyses confirmed that HSPA5 rs12009 and rs430397 were related to risk of NAFLD, and the genetic loci were all consistent with Hardy Weinberg equilibrium, we further performed haplotype analysis of these SNPs to predict susceptibility to NAFLD. The Linkage disequilibrium (LD) information of these SNPs was shown in Figure 1 (D’= 0.94, r2 = 0.223). Haplotype analysis demonstrated that (Supplementary Table 4), four haplotype frequencies were calculated: GC> AC> AT> GT, with the highest frequency of GC in both NAFLD and control populations, but no statistically significant difference in haplotype frequency (P> 0.05).
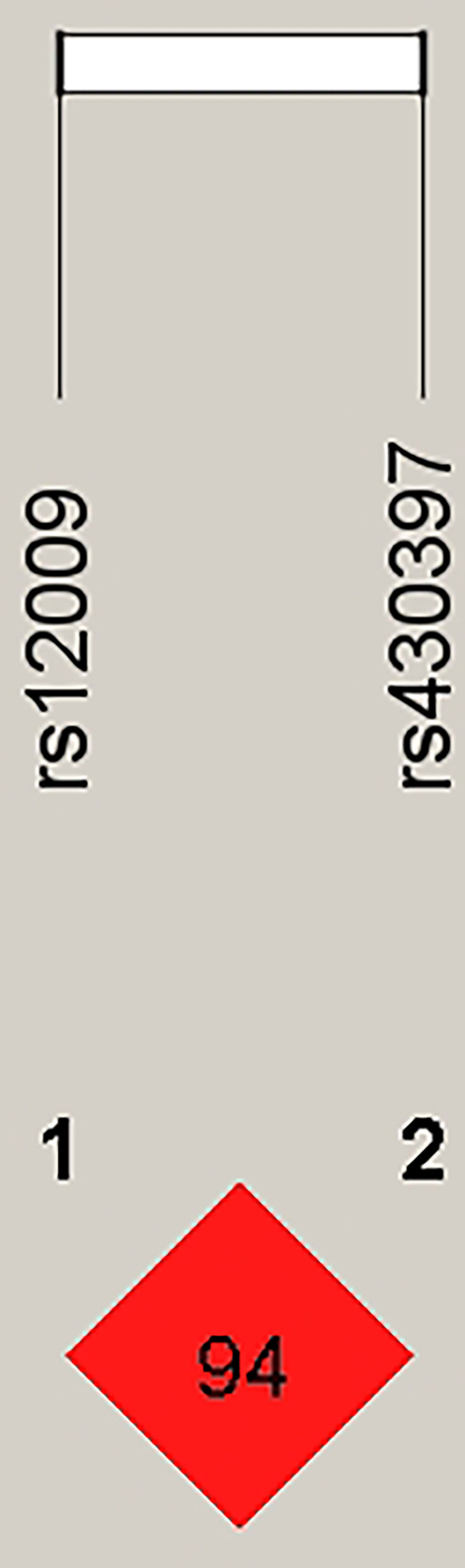
Figure 1 Linkage disequilibrium (LD) plot of HSPA5-rs12009 and HSPA5-rs430397. The number 98 in the red box represents a strong linkage disequilibrium between HSPA5-rs12009 and HSPA5-rs430397.
3.5. Influence factors of NAFLD
A stepwise regression model was performed with gender, age, GLU, BMI, TG, TC, and combined favorable alleles (HSPA5 rs12009-A, HSPA5 rs430397-T, and ITPR2 rs11048570-A). The results showed that gender (OR= 0.364, 95% CI= 0.278-0.475, P< 0.001) and favorable alleles (OR= 0.926, 95% CI= 0.858-0.999, P= 0.047) were independent protective factors of NAFLD. Conversely, higher GLU (OR= 1.368, 95% CI= 1.125- 1.664, P= 0.002) and BMI (OR= 3.558, 95% CI= 2.929-4.322, P< 0.001), TG (OR= 2.284, 95% CI= 1.879-2.777, P< 0.001) were independent risk factors of NAFLD (Table 5). Subsequently, based on the 5 variables mentioned above, we constructed a combined factor model to assess the risk of NAFLD. The AUROC of this combined model was 0.764 (95% CI= 0.745-0.783, P< 0.001) showed in Figure 2. At a cutoff value of 0.427, the sensitivity and specificity of this novel model were 77.8% and 64.9%, respectively. The positive likelihood ratio and negative likelihood ratio were 2.22 and 0.34, respectively. Finally, the results were found to be statistically significant after the Hosmer-Lemeshow test (P= 0.091> 0.05), proving that this model showed good agreement between predicted risk and observed outcomes.
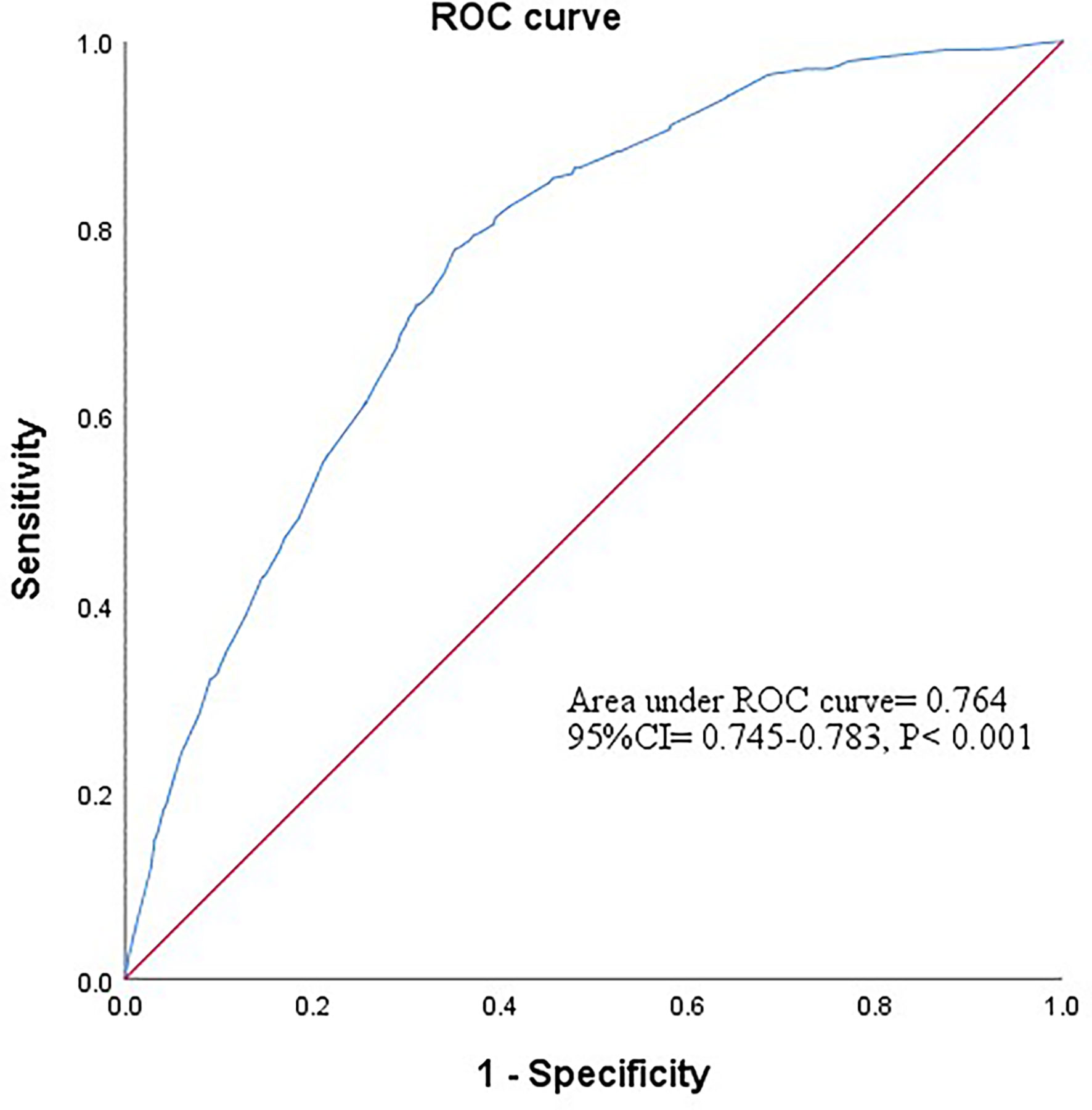
Figure 2 The ROC curve of combined factor model based on the data of gender, glucose, body mass index, triglycerides and favorable alleles. Based on the results of stepwise regression analysis, a combined factor model was constructed for the five variables of gender, glucose, body mass index, triglycerides and favorable alleles to assess the risk of NAFLD. The AUROC for this combined model was 0.764 (95% CI = 0.745-0.783, P<0.001).
4 Discussion
To the best of our knowledge, this was the first study to investigate the association between Ca2+ transport protein genetic polymorphisms and the risk of NAFLD. In this case-control study, we found that three SNPs, HSPA5-rs12009, HSPA5-rs430397 and ITPR2-rs11048570, were independent influencing factors for NAFLD. Additionally, rs12009-A, rs430397-T and rs11048570-A were associated with decreased risk of NAFLD as favorable alleles. The discovery and exploration of favorable alleles may provide a new direction for the development of drugs that complement the future treatment of NAFLD.
NAFLD is emerging rapidly as a major global health problem without an approved treatment. There is therefore an urgent demand to identify potential predictors and pharmacological targets. It has been demonstrated that ERS, mitochondrial dysfunction and abnormal lipid metabolism were involved in the pathogenesis of NAFLD (29). Studies have shown that Ca2+ homeostasis is related to ERS and mitochondrial dysfunction (30). Moreover, some studies have suggested that dysregulation of Ca2+ homeostasis in the MAM region played an important role in hepatic metabolism (31). GRP78 and IP3R2, the proteins encoded by HSPA5 and ITPR2 involved in this study, exert important physiological and pathological functions in the MAM region. Regarding GRP78, a master ER chaperone, is also present at the MAM, which is responsible for maintaining the ER permeability barrier during protein translocation, guiding protein folding and assembly, and targeting misfolded proteins for degradation, releasing and activating the unfolded protein response (UPR) sensors to maintain ER homeostasis (32, 33). Several studies have demonstrated that loss of GRP78 in metabolic organs, including the liver, may lead to primary or compensatory outcomes (34–36), such as compensatory ERS, and imbalances in glucose metabolism. Some experiments in GRP78-deficient mice suggested that deficiency of GRP78 may result in susceptibility to various acute or chronic liver diseases, and that GRP78 may be a regulator of hepatic steatosis and hepatocellular carcinoma. In MAM, IP3R2 resides near the ion channel VDAC, located in the outer mitochondrial membrane. This structure facilitates Ca2+ uptake into the mitochondrial matrix via the low-affinity mitochondrial Ca2+ uniporter (MCU) (37). Furthermore, IP3R2 is the major Ca2+ release channel of MAM in hepatocytes (38), may play an important role in lipid homeostasis, as it has been found that ITPR mutant Drosophila store excess triglycerides and become obese even on a normal diet (39). Furthermore, one study showed that ITPR2 expression was significantly reduced or absent in patients with steatosis and NASH (40). However, several works indicated that IP3R2 was a driver of senescence in human cells (20, 41–44), and ITPR2 knockout mice had suppressed Ca2+ fluxes in the MAM region and displayed less immunosenescence as well as less hepatic steatosis and fibrosis (20). Besides, some studies of human HCCs discovered that hepatitis B virus (HBV) DNA targets integrated hepatocyte DNA, while ITPR2 showed a high prevalence of HBV integration, which may indicate that ITPR2 plays an important role in hepatocarcinogenesis (45, 46).
Although there is no evidence directly suggesting that genetic polymorphisms in the Ca2+ transport protein of this study are associated with NAFLD outcomes, these genetic variants continue to play an essential role in ERS and mitochondrial dysfunction, and have been extensively explored in other diseases (47–49). It was found that HSPA5-rs12009 was significantly associated with knee osteoarthritis (KOA) severe progression in an exploratory cohort (50). Further presented studies suggested that HSPA5-rs430397 was associated with ERS (51). Meanwhile, a case-control study showed that HSPA5-rs430397 effectively predicted the primary hepatocellular carcinoma (52) and liver fibrosis (53), which also complemented our study hypothesis and results. Moreover, there are experimental results showing that the locus ITPR2-rs11048570 is significantly associated with Kashin-Beck Disease in the Han Chinese. It seems to be no direct connection between ITPR2-rs11048570 and NAFLD in previous studies, but they have confirmed the importance of ITPR2 in regulating apoptosis. It is observed that apoptotic stimuli could trigger IP3R mediated Ca2+ signaling, leading to mitochondrial release of cytochrome C and initiation of the complete apoptosis program (54). It is also demonstrated that phosphorylation of IP3Rs diminished Ca2+ flux from ER to mitochondria and significantly reduced cellular sensitivity to apoptotic stimuli (55). Although the potential molecular mechanism of ITPR2 involved in the development of NAFLD remains unclear, it is reasonable to speculate that ITPR2 contributes to abnormal hepatocyte apoptosis in the liver of patients with NAFLD. This article complements the association studies of HSPA5-rs12009, HSPA5-rs430397 and ITPR2-rs11048570 with NAFLD to some extent.
In addition, we found that combined effects of rs12009-A, rs430397-T, and rs11048570-A alleles were associated with the decreased risk of NAFLD. And as a result of stratified analysis, our report showed that the combined effects of these gene variants were statistically significant in the male and the age> 42 years subgroup, and there was no significant heterogeneity within each subgroup variable, suggesting that these variables did not alter this association. In fact, some studies have pointed to a higher incidence of NAFLD in men, and the gender differences may be related to differences in hormone, serum TG, and blood glucose levels (56). Besides, the prevalence of NAFLD increases with age and studies have speculated that the increased risk of NAFLD may contribute to the high prevalence of metabolic diseases in the elderly (57). These above studies are all consistent with our findings.
This study also demonstrated that favorable alleles were independent protective factors of NAFLD; male, higher GLU, BMI and TG levels were independent risk factors of NAFLD, which is accordance with the results of previous studies (56, 58–60). We combined these independent factors to assess NAFLD risk and the AUROC for this combined model was 0.764 (P< 0.001). The results showed that combined factors described above were efficient in assessing NAFLD risk, which may provide new approaches for early screening of high-risk populations.
Although this study was the first to explore the association of HSPA5 and ITPR2 gene polymorphisms with susceptibility to NAFLD and obtained some meaningful results, there were still several limitations. First, the representation of our sample may be insufficient. The subjects enrolled in this study were from a single community with a relatively small female population. To address this issue, the frequency-matching of gender and age was used in the design stage, and multivariate analysis and stratified analysis were utilized to minimize the effects of confounding factors. Second, we selected only a few key genes in the numerous pathways of Ca2+ transport, which may not adequately analyze the relationship between genetic factors and the risk of NAFLD. Thus, we attempted to use haplotype analysis, which laid the foundation for completing genetic information of related SNPs and searching for haplotypes associated with NAFLD, which was important for the study of Ca2+ transporter gene polymorphisms and their combinatorial distribution and the role of haplotype genotype distribution in disease mechanisms. Third, in view of the complexity of the pathogenesis of NAFLD, it is necessary to further explore the impact of gene polymorphisms and their combination with environmental factors on disease risk in a multicenter population of different races. Finally, the biological mechanism by which SNPs are related to the outcome of NAFLD is poorly understood. Therefore, replication studies with functional characterization of these SNPs in various lager multicenter population of different races are required to confirm our findings.
5 Conclusion
In conclusion, our findings suggested that gene polymorphisms of Ca2+ transport proteins and molecular chaperones play an important role in the development of NAFLD, particularly when associated with older age or males. Overall, HSPA5 gene (rs12009, rs430397) and ITPR2 gene (rs11048570) were associated with susceptibility to NAFLD within Chinese population.
Data availability statement
The original contributions presented in the study are included in the article/Supplementary Material. Further inquiries can be directed to the corresponding author.
Ethics statement
The studies involving human participants were reviewed and approved by Institutional Ethics Review Committee of Nanjing Medical University. The patients/participants provided their written informed consent to participate in this study.
Author contributions
ZT and YD: Data curation, Formal analysis, Investigation, Writing – original draft, Writing – review & editing. RZ, MZ, QG and LZ: Data curation, Investigation, Software. HW, YC, RJ and WZ: Investigation, Methodology, Resources. JW: Conceptualization, Funding acquisition, Project administration, Supervision, Writing – review and editing. All authors contributed to the article and approved the submitted version.
Funding
This work was supported by the Six Talent Peaks Project in Jiangsu Province, China (2019, WSN-049), “333 Project’ of Jiangsu Province, Priority Academic Program Development of Jiangsu Higher Education Institutions (Nursing Science, 2018, No.87), Research and Innovation Team Project of School of Nursing, Nanjing Medical University, and Graduate Research and Innovation Projects in Jiangsu Province, China (KYCX21_1553), Advantages of general practice in Pudong New Area (PWYq2020-03).
Acknowledgments
We thank all the students, doctors, and nurses who participated in this work.
Conflict of interest
The authors declare that the research was conducted in the absence of any commercial or financial relationships that could be construed as a potential conflict of interest.
Publisher’s note
All claims expressed in this article are solely those of the authors and do not necessarily represent those of their affiliated organizations, or those of the publisher, the editors and the reviewers. Any product that may be evaluated in this article, or claim that may be made by its manufacturer, is not guaranteed or endorsed by the publisher.
Supplementary material
The Supplementary Material for this article can be found online at: https://www.frontiersin.org/articles/10.3389/fendo.2022.1056283/full#supplementary-material
References
1. Younossi Z, Anstee QM, Marietti M, Hardy T, Henry L, Eslam M, et al. Global burden of NAFLD and NASH: Trends, predictions, risk factors and prevention. Nat Rev Gastroenterol Hepatol (2018) 15(1):11–20. doi: 10.1038/nrgastro.2017.109
2. Zhou J, Zhou F, Wang W, Zhang XJ, Ji YX, Zhang P, et al. Epidemiological features of NAFLD from 1999 to 2018 in China. Hepatol (Baltimore Md) (2020) 71(5):1851–64. doi: 10.1002/hep.31150
3. Li J, Zou B, Yeo YH, Feng Y, Xie X, Lee DH, et al. Prevalence, incidence, and outcome of non-alcoholic fatty liver disease in Asia, 1999-2019: a systematic review and meta-analysis. Lancet Gastroenterol Hepatol (2019) 4(5):389–98. doi: 10.1016/S2468-1253(19)30039-1
4. Díaz LA, Fuentes-López E, Ayares G, Idalsoaga F, Arnold J, Márquez-Lomas A, et al. The establishment of public health policies and the burden of non-alcoholic fatty liver disease in the americas. Lancet Gastroenterol Hepatol (2022) 7(6):552–9. doi: 10.1016/S2468-1253(22)00008-5
5. Friedman SL, Neuschwander-Tetri BA, Rinella M, Sanyal AJ. Mechanisms of NAFLD development and therapeutic strategies. Nat Med (2018) 24(7):908–22. doi: 10.1038/s41591-018-0104-9
6. Byrne CD, Targher G. NAFLD: a multisystem disease. J Hepatol (2015) 62(1 Suppl):S47–64. doi: 10.1016/j.jhep.2014.12.012
7. Mundi MS, Velapati S, Patel J, Kellogg TA, Abu Dayyeh BK, Hurt RT. Evolution of NAFLD and its management. Nutr Clin Pract Off Publ Am Soc Parenteral Enteral Nutr (2020) 35(1):72–84. doi: 10.1002/ncp.10449
8. Powell EE, Wong VW, Rinella M. Non-alcoholic fatty liver disease. Lancet (London England) (2021) 397(10290):2212–24. doi: 10.1016/S0140-6736(20)32511-3
9. Buzzetti E, Pinzani M, Tsochatzis EA. The multiple-hit pathogenesis of non-alcoholic fatty liver disease (NAFLD). Metabolism: Clin Experimental (2016) 65(8):1038–48. doi: 10.1016/j.metabol.2015.12.012
10. Jin C, Kumar P, Gracia-Sancho J, Dufour JF. Calcium transfer between endoplasmic reticulum and mitochondria in liver diseases. FEBS Letters (2021) 595(10):1411–21. doi: 10.1002/1873-3468.14078
11. Chen CC, Hsu LW, Chen KD, Chiu KW, Chen CL, Huang KT. Emerging roles of calcium signaling in the development of non-alcoholic fatty liver disease. Int J Mol Sci (2021) 23(1):256. doi: 10.3390/ijms23010256
12. Amaya MJ, Nathanson MH. Calcium signaling in the liver. Compr Physiol (2013) 3(1):515–39. doi: 10.1002/cphy.c120013
13. Filadi R, Theurey P, Pizzo P. The endoplasmic reticulum-mitochondria coupling in health and disease: Molecules, functions and significance. Cell Calcium (2017) 62:1–15. doi: 10.1016/j.ceca.2017.01.003
14. Hayashi T, Rizzuto R, Hajnoczky G, Su TP. MAM: more than just a housekeeper. Trends Cell Biol (2009) 19(2):81–8. doi: 10.1016/j.tcb.2008.12.002
15. Arruda AP, Hotamisligil GS. Calcium homeostasis and organelle function in the pathogenesis of obesity and diabetes. Cell Metab (2015) 22(3):381–97. doi: 10.1016/j.cmet.2015.06.010
16. Romero-Gómez M, Zelber-Sagi S, Trenell M. Treatment of NAFLD with diet, physical activity and exercise. J Hepatol (2017) 67(4):829–46. doi: 10.1016/j.jhep.2017.05.016
17. Giorgi C, De Stefani D, Bononi A, Rizzuto R, Pinton P. Structural and functional link between the mitochondrial network and the endoplasmic reticulum. Int J Biochem Cell Biol (2009) 41(10):1817–27. doi: 10.1016/j.biocel.2009.04.010
18. Szabadkai G, Bianchi K, Várnai P, De Stefani D, Wieckowski MR, Cavagna D, et al. Chaperone-mediated coupling of endoplasmic reticulum and mitochondrial Ca2+ channels. J Cell Biol (2006) 175(6):901–11. doi: 10.1083/jcb.200608073
19. Dong Z, Wang J, Qiu T, Wu J, An Y, Shi X, et al. Perfluorooctane sulfonate induces mitochondrial calcium overload and early hepatic insulin resistance via autophagy/detyrosinated alpha-tubulin-regulated IP3R2-VDAC1-MICU1 interaction. Sci total Environment (2022) 825:153933. doi: 10.1016/j.scitotenv.2022.153933
20. Ziegler DV, Vindrieux D, Goehrig D, Jaber S, Collin G, Griveau A, et al. Calcium channel ITPR2 and mitochondria-ER contacts promote cellular senescence and aging. Nat Commun (2021) 12(1):720. doi: 10.1038/s41467-021-20993-z
21. Gutiérrez T, Simmen T. Endoplasmic reticulum chaperones tweak the mitochondrial calcium rheostat to control metabolism and cell death. Cell Calcium (2018) 70:64–75. doi: 10.1016/j.ceca.2017.05.015
22. Reddy RK, Mao C, Baumeister P, Austin RC, Kaufman RJ, Lee AS. Endoplasmic reticulum chaperone protein GRP78 protects cells from apoptosis induced by topoisomerase inhibitors: Role of ATP binding site in suppression of caspase-7 activation. J Biol Chem (2003) 278(23):20915–24. doi: 10.1074/jbc.M212328200
23. Shu CW, Sun FC, Cho JH, Lin CC, Liu PF, Chen PY, et al. GRP78 and raf-1 cooperatively confer resistance to endoplasmic reticulum stress-induced apoptosis. J Cell Physiol (2008) 215(3):627–35. doi: 10.1002/jcp.21340
24. Gonzalez-Gronow M, Gopal U, Austin RC, Pizzo SV. Glucose-regulated protein (GRP78) is an important cell surface receptor for viral invasion, cancers, and neurological disorders. IUBMB Life (2021) 73(6):843–54. doi: 10.1002/iub.2502
25. National Workshop on Fatty Liver and Alcoholic Liver Disease CSoH, Chinese Medical Association, Fatty Liver Expert Committee CMDA. Guideline of prevention and treatment for nonalcoholic fatty liver disease. J Prac Hepatol (2018) 21(02):177–86. doi: 10.3969/j.issn.1672-5069.2018.02.007
26. Bismeijer T, van der Velden BHM, Canisius S, Lips EH, Loo CE, Viergever MA, et al. Radiogenomic analysis of breast cancer by linking MRI phenotypes with tumor gene expression. Radiology (2020) 296(2):277–87. doi: 10.1148/radiol.2020191453
27. Education BHABDPaTABRfCDCaH. Practice Guidelines. For integrated management of cardiovascular disease in primary hospitals. Chin J Front Med Science(Electronic Version) (2020) 12(08):1–73. doi: 10.12037/YXQY.2020.08-01
28. Fang Q, Wang ZL, Ning TH, Shao G, Chen ZJ, Lu ZL, et al. Recommendations for the prevention and treatment of dyslipidemia. Chin J Cardiol (1997) 03):10+2+4+1+3.
29. Wang J, He W, Tsai PJ, Chen PH, Ye M, Guo J, et al. Mutual interaction between endoplasmic reticulum and mitochondria in nonalcoholic fatty liver disease. Lipids Health Disease (2020) 19(1):72. doi: 10.1186/s12944-020-01210-0
30. Mei Y, Thompson MD, Cohen RA, Tong X. Endoplasmic reticulum stress and related pathological processes. J Pharmacol Biomed Analysis (2013) 1(2):1000107.
31. Rieusset J. Endoplasmic reticulum-mitochondria calcium signaling in hepatic metabolic diseases. Biochim Biophys Acta Mol Cell Res (2017) 1864(6):865–76. doi: 10.1016/j.bbamcr.2017.01.001
32. Ma Y, Hendershot LM. The role of the unfolded protein response in tumour development: Friend or foe? Nat Rev Cancer (2004) 4(12):966–77. doi: 10.1038/nrc1505
33. Ron D, Walter P. Signal integration in the endoplasmic reticulum unfolded protein response. Nat Rev Mol Cell Biol (2007) 8(7):519–29. doi: 10.1038/nrm2199
34. Zhu G, Ye R, Jung DY, Barron E, Friedline RH, Benoit VM, et al. GRP78 plays an essential role in adipogenesis and postnatal growth in mice. FASEB J (2013) 27(3):955–64. doi: 10.1096/fj.12-213330
35. Ye R, Jung DY, Jun JY, Li J, Luo S, Ko HJ, et al. Grp78 heterozygosity promotes adaptive unfolded protein response and attenuates diet-induced obesity and insulin resistance. Diabetes (2010) 59(1):6–16. doi: 10.2337/db09-0755
36. Ye R, Mareninova OA, Barron E, Wang M, Hinton DR, Pandol SJ, et al. Grp78 heterozygosity regulates chaperone balance in exocrine pancreas with differential response to cerulein-induced acute pancreatitis. Am J Pathology (2010) 177(6):2827–36. doi: 10.2353/ajpath.2010.100368
37. Rizzuto R, Marchi S, Bonora M, Aguiari P, Bononi A, De Stefani D, et al. Ca(2+) transfer from the ER to mitochondria: When, how and why. Biochim Biophys Acta (2009) 1787(11):1342–51. doi: 10.1016/j.bbabio.2009.03.015
38. Hirata K, Pusl T, O'Neill AF, Dranoff JA, Nathanson MH. The type II inositol 1,4,5-trisphosphate receptor can trigger Ca2+ waves in rat hepatocytes. Gastroenterology (2002) 122(4):1088–100. doi: 10.1053/gast.2002.32363
39. Subramanian M, Metya SK, Sadaf S, Kumar S, Schwudke D, Hasan G. Altered lipid homeostasis in drosophila InsP3 receptor mutants leads to obesity and hyperphagia. Dis Models Mechanisms (2013) 6(3):734–44. doi: 10.1242/dmm.010017
40. Khamphaya T, Chukijrungroat N, Saengsirisuwan V, Mitchell-Richards KA, Robert ME, Mennone A, et al. Nonalcoholic fatty liver disease impairs expression of the type II inositol 1,4,5-trisphosphate receptor. Hepatol (Baltimore Md) (2018) 67(2):560–74. doi: 10.1002/hep.29588
41. Martin N, Bernard D. Calcium signaling and cellular senescence. Cell Calcium (2018) 70:16–23. doi: 10.1016/j.ceca.2017.04.001
42. Wiel C, Lallet-Daher H, Gitenay D, Gras B, Le Calvé B, Augert A, et al. Endoplasmic reticulum calcium release through ITPR2 channels leads to mitochondrial calcium accumulation and senescence. Nat Commun (2014) 5:3792. doi: 10.1038/ncomms4792
43. Ma X, Warnier M, Raynard C, Ferrand M, Kirsh O, Defossez PA, et al. The nuclear receptor RXRA controls cellular senescence by regulating calcium signaling. Aging Cell (2018) 17(6):e12831. doi: 10.1111/acel.12831
44. Borodkina AV, Shatrova AN, Deryabin PI, Griukova AA, Abushik PA, Antonov SM, et al. Calcium alterations signal either to senescence or to autophagy induction in stem cells upon oxidative stress. Aging (2016) 8(12):3400–18. doi: 10.18632/aging.101130
45. Murakami Y, Saigo K, Takashima H, Minami M, Okanoue T, Bréchot C, et al. Large Scaled analysis of hepatitis b virus (HBV) DNA integration in HBV related hepatocellular carcinomas. Gut (2005) 54(8):1162–8. doi: 10.1136/gut.2004.054452
46. Paterlini-Bréchot P, Saigo K, Murakami Y, Chami M, Gozuacik D, Mugnier C, et al. Hepatitis b virus-related insertional mutagenesis occurs frequently in human liver cancers and recurrently targets human telomerase gene. Oncogene (2003) 22(25):3911–6. doi: 10.1038/sj.onc.1206492
47. Thivolet C, Vial G, Cassel R, Rieusset J, Madec AM. Reduction of endoplasmic reticulum- mitochondria interactions in beta cells from patients with type 2 diabetes. PloS One (2017) 12(7):e0182027. doi: 10.1371/journal.pone.0182027
48. Prell T, Lautenschläger J, Grosskreutz J. Calcium-dependent protein folding in amyotrophic lateral sclerosis. Cell Calcium (2013) 54(2):132–43. doi: 10.1016/j.ceca.2013.05.007
49. Pfaffenseller B, Wollenhaupt-Aguiar B, Fries GR, Colpo GD, Burque RK, Bristot G, et al. Impaired endoplasmic reticulum stress response in bipolar disorder: cellular evidence of illness progression. Int J Neuropsychopharmacol (2014) 17(9):1453–63. doi: 10.1017/S1461145714000443
50. Blanco FJ, Möller I, Romera M, Rozadilla A, Sánchez-Lázaro JA, Rodríguez A, et al. Improved prediction of knee osteoarthritis progression by genetic polymorphisms: the arthrotest study. Rheumatol (Oxford England) (2015) 54(7):1236–43. doi: 10.1093/rheumatology/keu478
51. Guo XB, Ma WL, Liu LJ, Huang YL, Wang J, Huang LH, et al. Effects of gene polymorphisms in the endoplasmic reticulum stress pathway on clinical outcomes of chemoradiotherapy in Chinese patients with nasopharyngeal carcinoma. Acta Pharmacologica Sinica (2017) 38(4):571–80. doi: 10.1038/aps.2016.148
52. Balasus D, Way M, Fusilli C, Mazza T, Morgan MY, Cervello M, et al. The association of variants in PNPLA3 and GRP78 and the risk of developing hepatocellular carcinoma in an Italian population. Oncotarget (2016) 7(52):86791–802. doi: 10.18632/oncotarget.13558
53. Wang C, Zhang X, Ling Q, Zheng S, Xu X. A model integrating donor gene polymorphisms predicts fibrosis after liver transplantation. Aging (2020) 13(1):1264–75. doi: 10.18632/aging.202302
54. Szalai G, Krishnamurthy R, Hajnóczky G. Apoptosis driven by IP(3)-linked mitochondrial calcium signals. EMBO J (1999) 18(22):6349–61. doi: 10.1093/emboj/18.22.6349
55. Szado T, Vanderheyden V, Parys JB, De Smedt H, Rietdorf K, Kotelevets L, et al. Phosphorylation of inositol 1,4,5-trisphosphate receptors by protein kinase B/Akt inhibits Ca2+ release and apoptosis. Proc Natl Acad Sci USA (2008) 105(7):2427–32. doi: 10.1073/pnas.0711324105
56. Hu XY, Li Y, Li LQ, Zheng Y, Lv JH, Huang SC, et al. Risk factors and biomarkers of non-alcoholic fatty liver disease: an observational cross-sectional population survey. BMJ Open (2018) 8(4):e019974. doi: 10.1136/bmjopen-2017-019974
57. Bruno Ade S, Rodrigues MH, Alvares MC, Nahas-Neto J, Nahas EA. Non-alcoholic fatty liver disease and its associated risk factors in Brazilian postmenopausal women. Climacteric J Int Menopause Society (2014) 17(4):465–71. doi: 10.3109/13697137.2014.881353
58. Lonardo A, Nascimbeni F, Ballestri S, Fairweather D, Win S, Than TA, et al. Sex differences in nonalcoholic fatty liver disease: State of the art and identification of research gaps. Hepatol (Baltimore Md) (2019) 70(4):1457–69. doi: 10.1002/hep.30626
59. Ullah R, Rauf N, Nabi G, Ullah H, Shen Y, Zhou YD, et al. Role of nutrition in the pathogenesis and prevention of non-alcoholic fatty liver disease: Recent updates. Int J Biol Sci (2019) 15(2):265–76. doi: 10.7150/ijbs.30121
Keywords: non-alcoholic fatty liver disease (NAFLD), heat shock 70-kDa protein 5 (HSPA5), inositol 1,4,5-trisphosphate receptor type 2 (ITPR2), genetic polymorphism, calcium homeostasis
Citation: Tang Z, Ding Y, Zhang R, Zhang M, Guan Q, Zhang L, Wang H, Chen Y, Jiang R, Zhang W and Wang J (2023) Genetic polymorphisms of Ca2+ transport proteins and molecular chaperones in mitochondria-associated endoplasmic reticulum membrane and non-alcoholic fatty liver disease. Front. Endocrinol. 13:1056283. doi: 10.3389/fendo.2022.1056283
Received: 28 September 2022; Accepted: 13 December 2022;
Published: 04 January 2023.
Edited by:
Kerry Loomes, The University of Auckland, New ZealandReviewed by:
Valentina María Parra, University of Chile, ChileChristopher Stuart Walker, The University of Auckland, New Zealand
Copyright © 2023 Tang, Ding, Zhang, Zhang, Guan, Zhang, Wang, Chen, Jiang, Zhang and Wang. This is an open-access article distributed under the terms of the Creative Commons Attribution License (CC BY). The use, distribution or reproduction in other forums is permitted, provided the original author(s) and the copyright owner(s) are credited and that the original publication in this journal is cited, in accordance with accepted academic practice. No use, distribution or reproduction is permitted which does not comply with these terms.
*Correspondence: Jie Wang, d2FuZ2ppZW5qbXVAMTI2LmNvbQ==
†These authors have contributed equally to this work