- 1School of Pharmacy, Macau University of Science and Technology, Macau, Macau SAR, China
- 2State Key Laboratory of Quality Research in Chinese Medicine, Macau, Macau SAR, China
- 3Department of Epidemiology and Biostatistics, School of Public Health, Jilin University, Changchun, China
- 4College of Pharmacy, Dalian Medical University, Dalian, China
Thyroid carcinoma is the most common endocrine cancer in the world, and its incidence has been steadily increasing in recent years. Despite its relatively good prognosis, therapies have not improved greatly in recent years. Therefore, exploring new therapies for thyroid carcinoma represents an unmet need. Nitric oxide (NO) is a short-term endogenous signaling molecule that plays a vital role in various physiological and pathological processes and is synthesized by nitric oxide synthase (NOS). Many studies have been conducted over the past decades to explain its correlation to cancer. NO exerts a wide range of effects on cancer, involving angiogenesis, apoptosis, cell cycle, invasion, and metastasis. It also serves a dual function by promoting and halting tumor development simultaneously. The relationship between NO and thyroid carcinoma has been intensively studied and discussed. This paper reviews the role and molecular mechanism of NO in thyroid carcinoma and discusses potentials of prevention and treatment of thyroid carcinoma.
1 Introduction
The thyroid tumor is the most common endocrine tumor. According to a population-wide epidemiological study of thyroid carcinoma, there are about 449,000 new cases of thyroid carcinoma in women and 137,000 in men in 2020. The corresponding age-standardized incidence is about 10.1 cases per 100,000 women and 3.1 cases per 100,000 men (1).There are many types of thyroid tumors. Classified by the cellular origin, they can be divided into two major categories. One of them is medullary thyroid carcinoma (MTC) caused by C cells (approximately 3-5%), another type of thyroid carcinoma is derived from follicular cells (about 95%) such as papillary thyroid cancer (PTC), follicular thyroid cancer (FTC), poorly differentiated thyroid cancer (PDTC) and aplastic thyroid cancer (ATC). PTC and FTC are considered to be well-differentiated thyroid carcinomas (2, 3). The prognosis of most thyroid carcinomas is reasonably good, and the 10-year survival rate among patients after surgery and other treatments is above 90% (4). Despite this, some other types of thyroid carcinomas are with a high degree of malignancy, and the survival rate is therefore reduced. For instance, the five-year survival rate of PDTC is 66% (5) and the ten-year survival rate of MTC is 62% (6).
The conventional treatment of thyroid carcinoma is usually surgery, which is divided into total thyroidectomy and unilateral thyroidectomy. This is the preferred treatment for most differentiated thyroid carcinomas (7). The choice of surgery, however, remains controversial. People who support total thyroidectomy believe that most patients with thyroid carcinoma have tumor tissue in both lobes of the thyroid gland (8), and thyroid recurrence occurs in the contralateral side in 5%-10% of patients (9). In contrast, proponents of unilateral thyroidectomy argue that more extensive surgery does not improve survival and unilateral surgery is less halmful to the patient than bilateral surgery.
Due to the great differences in the treatment and prognosis of different types of thyroid cancer, it has become an important topic to find a convenient and effective new treatment method.
In the last century, NO was known to be a toxic molecule. However, since the beginning of this century, NO has been increasingly recognized as a signaling molecule involved in a wide range of physiological activities in the human body, such as inflammation, immunity, hormone secretion, platelet aggregation, bronchiectasis, and so on (10, 11). Endogenous NO generation is regulated by nitric oxide synthases (NOS) (12). NO is closely associated with tumors and is often considered to have dual effects on cancer cells, that is, both promoting and inhibiting the growth of cancer cells (13). This review goes over the literature on thyroid carcinoma and NO to find a potential link between the two and to propose the basis for possible new treatment of thyroid carcinoma.
2 Physiological function of nitric oxide
Nitric oxide (NO) is an extremely short-lived diatomic free radical molecule with a half-life of less than 5 seconds in vivo (14). Nevertheless, NO has a wide range of effects on the human body. Ferid Murad was awarded the Nobel Prize in Physiology or Medicine in 1998 for his finding on cyclic guanosine monophosphate (GMP) dependence.In his early findings, NO was shown to activate the cytoplasmic isoform of guanylyl cyclase, which catalyzes the conversion of guanosine triphosphate (GTP) to cyclic guanosine monophosphate (GMP). In these circumstances, the increase of GMP level will lead to a series of phosphorylation processes, thereby causing the dilation of blood vessels and the change of blood flow velocity (15). This pathway is known as the GMP-dependent pathway. In addition to this, another pathway for NO is the GMP-independent pathway. It produces effects through S-nitrosylation of proteins, the processing which is mainly related to DNA damage and p53 mutation (16).
The effect of NO itself can be divided into direct and indirect effects in most cases. Direct effect means that NO binds to metal complexes of different proteins and interacts with each other to regulate physiological activities (17). The indirect effect refers to the reaction of NO with O2 and other endogenous free radicals and the production of reactive nitrogen. They are responsible for participating in other signaling and mediating toxic reactions (18).
Apart from that, NO also has a close relationship with immunity. It is widely considered to be participating in immune activities, regulating the functional activities, growth and death of macrophages, T lymphocytes, antigen-presenting cells, mast cells, neutrophils, NK cells, and other cells (19). NO plays a crucial role in allergy and autoimmune diseases by influencing immunity, especially T cell-mediated immune activity (20). Therefore, for diseases related to the immune system, NO may become a new therapeutic target.
3 Nitric oxide and nitric oxide synthase
Nitric oxide synthase (NOS) is a key enzyme in the production of NO in the human body. There are three main subtypes of NOS in the human body, namely nNOS, eNOS, and iNOS. Among them, nNOS is mainly found in the nervous system and is an essential enzyme for neuronal conduction, and eNOS was first discovered in endothelial cells and plays a crucial role in vasodilatation and blood pressure control. Both isomers depend on Ca2+ for concentration regulation and produce small amounts of NO for a short period (usually within seconds to minutes). The other type of iNOS is an isomer which is inducible and calcium-independent. It does not persist in cells and is only expressed when cells are stimulated. Unlike the previous two isomers, iNOS can be induced to produce NO continuously. Once induced, it produces large amounts of NO for hours to days (21, 22).
There are many controversies about the physiological role of NOS. Some believe it is a harmful enzyme and is involved in the development of inflammation. Atochina-Vasserman et al. (23), for instance, found that pneumonia in mice was reduced when iNOS was inhibited. Besides this, the findings of Miyoshi et al. (24) have shown that iNOS promotes low-density lipoprotein oxidation through the activation of smooth muscle cells, which is a key step in the formation of atherosclerosis. In the nervous system, NOS activity is high. When the expression of NOS itself is overactivated, large amounts of NO are produced, mediating cytotoxic effects and leading to nerve damage or even death (25).
With the in-depth study of NOS, more new findings have shown that NOS also brings many beneficial effects. nNOS is widely found in neurons, astrocytes, and cerebrovascular, and is believed to be involved in the regulation of learning, memory, neural development, and other physiological activities (26), though many previous studies have shown that NO produced by nNOS in the brain harms neural development, especially on neurons (27). Study by Lou et al. (28) have shown that when nNOS inhibitors are administered to neural stem cells (NSC), their ability to differentiate into neurons and astrocytes is inhibited. Weitzdoerfer et al. (29) found cognitive performance impairment in nNOS knockout mice, which suggests that nNOS may affect NSC differentiation. These findings indicate that both NOS and NO play a wide range of important roles in human physiological activities.
4 Nitric oxide and cancer
For a long time, a large number of researchers have been exploring the relationship between NO and cancer. Numerous studies have shown that the effects of NO on cancer cells are bidirectional. At low concentrations, it can protect cancer cells and accelerate tumor development. When the concentration of NO exceeds the ideal concentration for tumor growth, it results in growth arrest or apoptosis (30). In some in vitro experiments, it was found that the cell differentiation rate increased and the cell differentiation decreased at low doses of NO. However, at higher concentrations of NO, cell growth was shown to decrease, while cell differentiation was shown to increase (31). These findings also demonstrate that the effect of NO on tumors is bidirectional. NO can regulate all aspects of tumor physiological activities, including tumor growth, migration, invasion, survival, angiogenesis, and metastasis (32). These processes are usually determined by many factors, such as the concentration of NO, the time when tissue cells are exposed to NO, and the environment of redox reactions (30, 33). At low concentrations, it can stimulate the growth and proliferation of tumor cells (34) to achieve the purpose of promoting tumor growth. Apart from that, NO can also promote angiogenesis. It is well known that angiogenesis is the generation of new blood vessels from original blood vessels, which is an important step in tumor progression and metastasis (35). NO promotes angiogenesis, thereby helping tumor growth and metastasis (36). However, this mechanism is quite complicated and consists of the following processes. eNOS stimulates endothelial expression to produce and release NO. With the help of vascular endothelial growth factor (VEGF), eNOS expression is up-regulated, thereby releasing more NO and promoting the differentiation of quiescent endothelial cells into vessels (Figure 1) (37), to promote angiogenesis. At high concentrations, NO can mediate apoptosis. As a transcription factor, P53 is an important signaling molecule in cells, which regulates cell growth, apoptosis, DNA repair, and other processes by changing gene expression, and it is regulated by NO as well (38, 39). NO can lead to the accumulation of wild-type P53, thus achieving the purpose of inhibiting the growth of tumor cells (40). Interestingly, there is a negative feedback regulation between P53 and iNOS, in which P53 controls NOS2 activities. This relationship exists in many cancers (41, 42). These studies have proved that NO is a key factor in the physiological activities of tumor cells. It can be adjusted to control its flux in tumor tissues to achieve the purpose of tumor treatment, and it can achieve the purpose of tumor treatment by adjusting and controlling its flux in tumor tissues. In recent studies, NO has been used in cancer therapy alone or in combination, and has been shown to have immune-boosting, photodynamic therapeutic effects (43–45). Jiang et al. (46) found that NO is an immunogenic cell death (ICD) inducer, which can trigger a strong specific antitumor response, thereby enhancing the effect of tumor immunotherapy. Photodynamic therapy (PDT) is based on photosensitizers (PS) that transfer energy to tissue oxygen under specific laser irradiation to generate cytotoxic reactive oxygen species (ROS) that selectively kill tumor cells. The antioxidant glutathione (GSH) in tumor cells can effectively remove ROS and thus affect the effect of PDT. In addition, the study of Yao et al. (47) showed that NO could consume GSH, alleviate cellular hypoxia and improve the efficacy of PDT. The matter of the delivery mode of NO was examined in detail in the paper written by Alimoradi et al. (48).
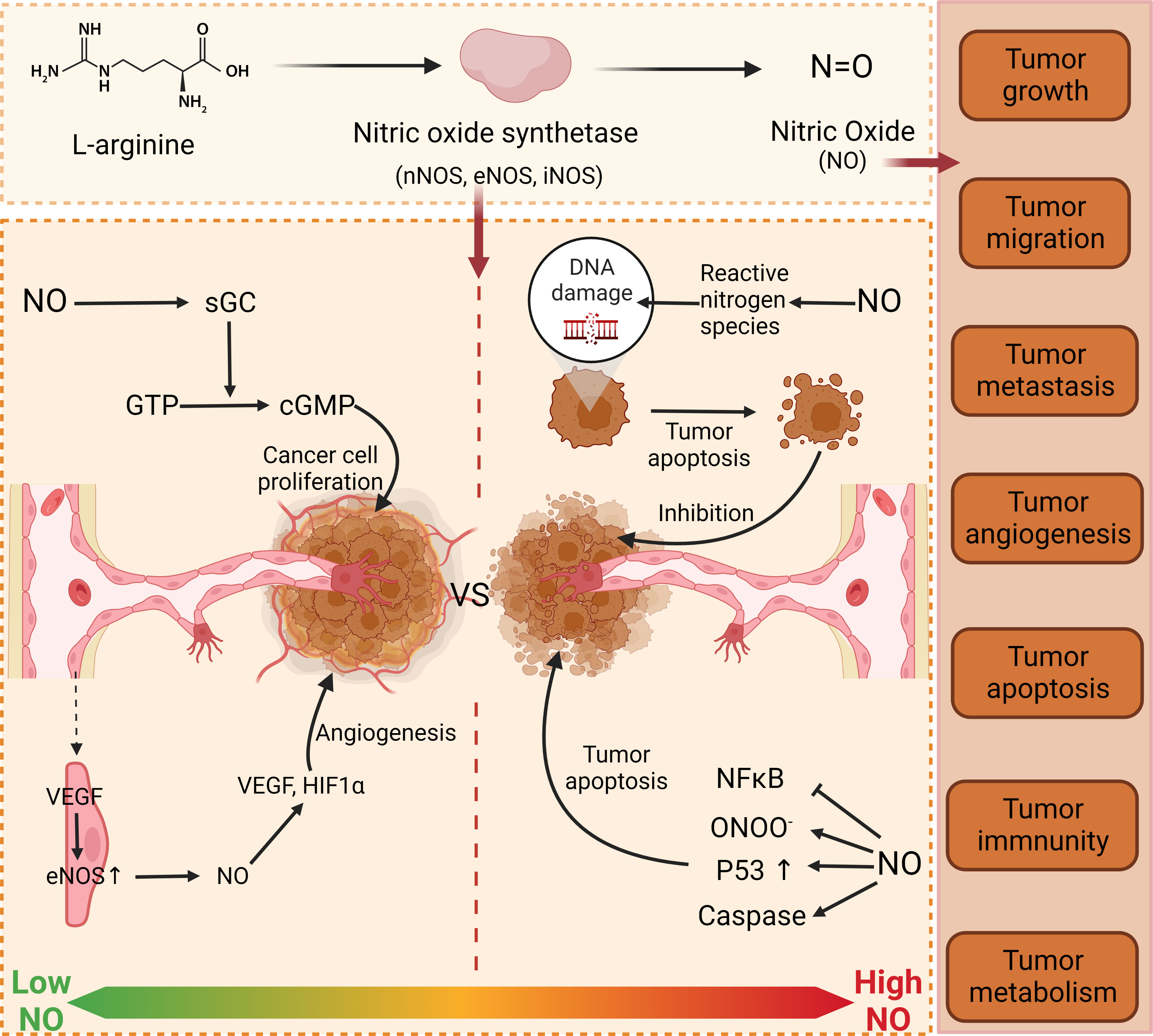
Figure 1 Function of NO in cancer. NO has dual properties on tumor cells. At low concentration, NO promotes tumor growth and proliferation through GMP-dependent pathway and angiogenesis. At high concentration, NO destroys the DNA of tumor cells by releasing active nitrogen, thus achieving the purpose of inhibiting tumor cells. Furthermore, NO can also accumulate P53 to affect the physiological processes of tumor cells. nNOS, neuronal nitric oxide synthase; eNOS, Endothelial Nitric Oxide Synthase; iNOS, Inducible Nitric Oxide Synthase; NO, nitrous oxide; sGC, Soluble guanylate cyclase; GTP, Guanosine Triphosphate; cGMP, cyclic guanosine monophosphate; VS, versus; VEGF, Vascular Endothelial Growth Factor; HIF1A, Hypoxia inducible factor-1α; DNA, deoxyribonucleic acid; NFkB, nuclear factor κB; ONOO, peroxynitrite anion.
5 Nitric oxide and thyroid carcinoma
The relationship between NO and thyroid is very intimate. NO is thought to be involved in many thyroid diseases, such as hypothyroidism and hyperthyroidism. In hypothyroidism, researchers have found that NO plays a quite important role in the damage of different tissues (49, 50). During the early stage of fetal development, maternal thyroid hormone (TH) is the crucial element to maintain normal brain and thyroid development (51, 52). In addition, nNOS is a key target gene of maternal TH in fetal cerebral cortex development. Maternal hypothyroidism may lead to fetal neural hypoplasia (53), while hyperthyroidism may affect cardiac function (54). Rodriguez et al. found that NO may modulate the response to hyperthyroidism, leading to cardiovascular dysfunction, even though this may be related to gender (55). The above studies proved that NO could regulate a large number of physiological processes of the thyroid and had a significant influence on it.
Thyroid carcinoma, as an endocrine tumor, is a malignant thyroid lesion. Kayser et al. detected the expression of NOS, iNOS and eNOS in different tissues, and found that the expression of NOS was much higher in thyroid tumor tissues (56). Choe et al. (57) found that iNOS expression was low in normal follicular epithelial cells, but was significantly enhanced in all follicular epithelial derived thyroid tumors. Maria et al. found that NOS enzyme can be expressed in WRO cells, which may be involved in the growth and progression of thyroid tumors (58). These studies indicate that there may be a clinical correlation between NO and thyroid tumors, but the specific molecular mechanism has not been elucidated. However, a study performed by Patel et al. (59) about the relationship between thyroid tumors and NOS in adolescents and children has shown that the contents of iNOS and eNOS in diseased thyroid tissues in PTC and FTC were higher than those in surrounding normal thyroid tissues, and this result was statistically significant. Additionally, they also found that VEGF expression was upregulated in tumor tissue. The study of Nakamura et al. (60) also confirmed that VEGF-D expression may be regulated by NO. This is consistent with the previously described evidence that NOS promotes angiogenesis to help tumor growth. As a consequence, it is demonstrated that NOS/NO plays a crucial part in thyroid tumors.
Since NO has a wide range of effects in thyroid tumors, regulation of NOS/NO may become a new convenient and economic treatment for thyroid tumors. Studies have shown that NO produced by iNOS has a great lethal effect on MTC cells and the volume of tumor tissues is significantly reduced (35%, P <0.05) by direct plasmid insertion of DNA-NOS II gene into MTC mice (61). The above experiments indicate that iNOS gene seems to be a promising gene therapy for human cancer. In another study, two nonsteroidal anti-inflammatory drugs (celecoxib and indomethacin) were added to the supply of NO to inhibit the growth of MTC cells. The results showed that compared with using a single drug, the therapeutic effect of any combination drug therapy was better than that of a single NO donor (62). The above studies indicated that the regulation of NOS/NO could play a role in the treatment of thyroid carcinoma. Beyond that, a new study in 2022 conducted by Sun et al. (63) explored the antitumor mechanism of nano-pulse stimulation (NPS) on human ATC cells in vitro. in vitro In this study, it was found that NPS could participate in the killing of ATC cells by iNOS. NPS can increase the activity of iNOS through the structure of enzyme, which greatly increases the concentration of NO. High levels of NO block the electron transport chain in mitochondria, leading to mitochondrial apoptosis and the release of cytochrome C, which activates the caspase process and guides cancer cell apoptosis. These findings also have a great reference value for researchers to develop new, convenient, effective, and cost-effective treatment regimens and therapeutic drugs (Figure 2).
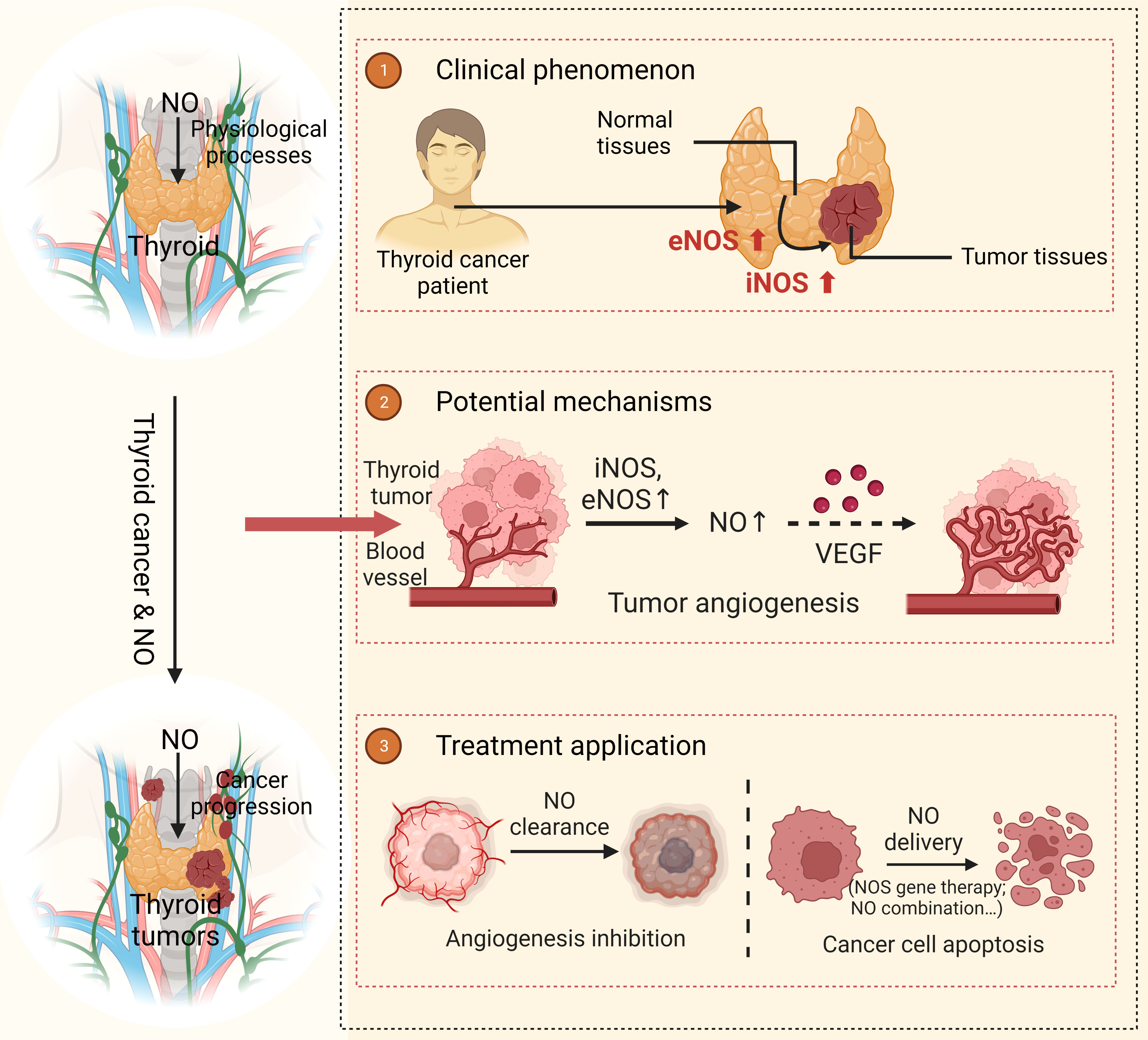
Figure 2 Function of NO in thyroid carcinoma The expressions of eNOS and iNOS in thyroid carcinoma tissues are higher than those in normal thyroid tissues. As a result, this increases the content of NO, which promotes tumor growth by regulating angiogenesis and VEGE. When NO is targeted to the thyroid tissue and maintained in high concentration, it can inhibit the growth and proliferation of thyroid tumors and therefore achieve therapeutic goals. NO, nitrous oxide; NOS, Nitric Oxide Synthase; eNOS, Endothelial Nitric Oxide Synthase; iNOS, Inducible Nitric Oxide Synthase; VEGF, Vascular Endothelial Growth Factor.
6 Conclusions
This paper reviews the literature on NO and thyroid carcinoma and explains the main association between the two. NO is a signaling molecule widely involved in human physiological activities. It is produced and released by the NOS enzyme family, and the effect is significantly bidirectional (64). It participates in every physiological process of various tumors, especially in the angiogenesis of tumor tissues, which has also been proved in thyroid tumors. At present, the mainstream treatments for thyroid tumors are surgery and the standard method of postoperative radioiodine (65). However, surgery often brings physical pain and psychological fear to patients in clinical practices. Beyond that, the need of receiving radioiodine therapy after surgery may lead to even more psychological stress on patients. Therefore, it is an unmet need to find a convenient, effective, and therapeutic potential strategy. on this ground t he therapeutic potential of NOS/NO in tumors is obvious. NO has been used in the treatment of various cancers, such as lung cancer, breast cancer, melanoma, and so on (66). If NO is to be considered as a therapeutic agent for thyroid tumors, two aspects should be considered due to its inherent biphasic nature. One aspect is to keep thyroid tumor tissue below the optimal NO concentration for growth. High expression of NOS is detected in diseased thyroid tissues, which also produce higher concentrations of NO than normal thyroid tissues. It is possible that NO at this concentration may stimulate cell proliferation and angiogenesis to promote the growth and development of tumor cells. Another aspect is to keep a high concentration of NO in thyroid tumor tissue to achieve the concentration of promoting cell apoptosis, to achieve the inhibitory effect on tumor cells. The animal experiments mentioned above also prove that this method is feasible. With the in-depth research on NO, researchers have realized its importance for tumor treatment, and more and more NO delivery systems have been developed (48). This greatly improves the target organ delivery in the treatment of different tissues and different types of tumors, making it more accurate. Meanwhile, it also reduces the effect of NO on other tissues and organs, improving the therapeutic effect. However, there have only been a few experiments on NO, and NO’s exact effects are still unknown. More experiments, especially in vivo, are needed to verify the real effects of the treatment in the future. On all accounts, the approach of NO pathway regulation should be recognized. Compared with conventional surgical radiotherapy and chemotherapy, NO pathway regulation has fewer side effects, and patients will suffer less mental and physical stress from it. As a consequence, the treatment of thyroid carcinoma with NO alone or in combination may be a potential better treatment method to improve the therapeutic effect of patients and improve their quality of life.
Author contributions
YL, XZ, NZ designed the structure of the article and collected the main reference; YH, MG and RS analyzed the data. JZ, YH, and XZ wrote the manuscript, WH substantially revised the manuscript. All authors contributed to the article and approved the submitted version.
Funding
This work was supported by the Macau Science and Technology Development fund (FDCT 0088/2021/A、0089/2021/A). The Faculty Research Grants of Macau University of Science and Technology (FRG-21-039、FRG-22-078).
Conflict of interest
The authors declare that the research was conducted in the absence of any commercial or financial relationships that could be construed as a potential conflict of interest.
Publisher’s note
All claims expressed in this article are solely those of the authors and do not necessarily represent those of their affiliated organizations, or those of the publisher, the editors and the reviewers. Any product that may be evaluated in this article, or claim that may be made by its manufacturer, is not guaranteed or endorsed by the publisher.
References
1. Pizzato M, Li M, Vignat J, Laversanne M, Singh D, La Vecchia C, et al. The epidemiological landscape of thyroid cancer worldwide: Globocan estimates for incidence and mortality rates in 2020. Lancet Diabetes Endocrinol (2022) 10(4):264–72. doi: 10.1016/s2213-8587(22)00035-3
2. Baloch ZW, Asa SL, Barletta JA, Ghossein RA, Juhlin CC, Jung CK, et al. Overview of the 2022 who classification of thyroid neoplasms. Endocr Pathol (2022) 33(1):27–63. doi: 10.1007/s12022-022-09707-3
3. Nagtegaal ID, Odze RD, Klimstra D, Paradis V, Rugge M, Schirmacher P, et al. The 2019 who classification of tumours of the digestive system. Histopathology (2020) 76(2):182–8. doi: 10.1111/his.13975
4. Doescher J, Veit JA, Hoffmann TK. [the 8th edition of the ajcc cancer staging manual : Updates in otorhinolaryngology, head and neck surgery]. Hno (2017) 65(12):956–61. doi: 10.1007/s00106-017-0391-3
5. Ibrahimpasic T, Ghossein R, Carlson DL, Nixon I, Palmer FL, Shaha AR, et al. Outcomes in patients with poorly differentiated thyroid carcinoma. J Clin Endocrinol Metab (2014) 99(4):1245–52. doi: 10.1210/jc.2013-3842
6. Kuo EJ, Sho S, Li N, Zanocco KA, Yeh MW, Livhits MJ. Risk factors associated with reoperation and disease-specific mortality in patients with medullary thyroid carcinoma. JAMA Surg (2018) 153(1):52–9. doi: 10.1001/jamasurg.2017.3555
7. Sherman SI. Thyroid carcinoma. Lancet (2003) 361(9356):501–11. doi: 10.1016/s0140-6736(03)12488-9
8. Katoh R, Sasaki J, Kurihara H, Suzuki K, Iida Y, Kawaoi A. Multiple thyroid involvement (Intraglandular metastasis) in papillary thyroid carcinoma. A Clinicopathologic Study 105 Consecutive Patients. Cancer (1992) 70(6):1585–90. doi: 10.1002/1097-0142(19920915)70:6<1585
9. Silverberg SG, Hutter RV, Foote FW Jr. Fatal carcinoma of the thyroid: Histology, metastases, and causes of death. Cancer (1970) 25(4):792–802. doi: 10.1002/1097-0142(197004)25:4<792
10. Choudhari SK, Chaudhary M, Bagde S, Gadbail AR, Joshi V. Nitric oxide and cancer: A review. World J Surg Oncol (2013) 11:118. doi: 10.1186/1477-7819-11-118
11. Lincoln J, Hoyle CH, Burnstock G. Nitric oxide in health and disease Cambridge university press. (1997) 3–11p.
12. Lirk P, Hoffmann G, Rieder J. Inducible nitric oxide synthase–time for reappraisal. Curr Drug Targets Inflammation Allergy (2002) 1(1):89–108. doi: 10.2174/1568010023344913
13. Wink DA, Mitchell JB. Chemical biology of nitric oxide: Insights into regulatory, cytotoxic, and cytoprotective mechanisms of nitric oxide. Free Radic Biol Med (1998) 25(4-5):434–56. doi: 10.1016/s0891-5849(98)00092-6
14. Hasan N, Cao J, Lee J, Naeem M, Hlaing SP, Kim J, et al. Pei/Nonoates-doped plga nanoparticles for eradicating methicillin-resistant staphylococcus aureus biofilm in diabetic wounds Via binding to the biofilm matrix. Mater Sci Eng C Mater Biol Appl (2019) 103:109741. doi: 10.1016/j.msec.2019.109741
15. Palmer RM, Ferrige AG, Moncada S. Nitric oxide release accounts for the biological activity of endothelium-derived relaxing factor. Nature (1987) 327(6122):524–6. doi: 10.1038/327524a0
16. Xu W, Liu LZ, Loizidou M, Ahmed M, Charles IG. The role of nitric oxide in cancer. Cell Res (2002) 12(5-6):311–20. doi: 10.1038/sj.cr.7290133
17. Cooper CE. Nitric oxide and iron proteins. Biochim Biophys Acta (1999) 1411(2-3):290–309. doi: 10.1016/s0005-2728(99)00021-3
18. Blough NV, Zafiriou OC. Reaction of superoxide with nitric oxide to form peroxonitrite in alkaline aqueous solution. Inorganic Chem (1985) 24(22):3502–4. doi: 10.1021/ic00216a003
19. Coleman JW. Nitric oxide in immunity and inflammation. Int Immunopharmacol (2001) 1(8):1397–406. doi: 10.1016/s1567-5769(01)00086-8
20. García-Ortiz A, Serrador JM. Nitric oxide signaling in T cell-mediated immunity. Trends Mol Med (2018) 24(4):412–27. doi: 10.1016/j.molmed.2018.02.002
21. Alderton WK, Cooper CE, Knowles RG. Nitric oxide synthases: Structure, function and inhibition. Biochem J (2001) 357(Pt 3):593–615. doi: 10.1042/0264-6021:3570593
22. Cinelli MA, Do HT, Miley GP, Silverman RB. Inducible nitric oxide synthase: Regulation, structure, and inhibition. Med Res Rev (2020) 40(1):158–89. doi: 10.1002/med.21599
23. Atochina-Vasserman EN, Beers MF, Kadire H, Tomer Y, Inch A, Scott P, et al. Selective inhibition of inducible no synthase activity in vivo reverses inflammatory abnormalities in surfactant protein d-deficient mice. J Immunol (2007) 179(12):8090–7. doi: 10.4049/jimmunol.179.12.8090
24. Miyoshi T, Li Y, Shih DM, Wang X, Laubach VE, Matsumoto AH, et al. Deficiency of inducible no synthase reduces advanced but not early atherosclerosis in apolipoprotein e-deficient mice. Life Sci (2006) 79(6):525–31. doi: 10.1016/j.lfs.2006.01.043
25. Dawson TM, Dawson VL. Nitric oxide signaling in neurodegeneration and cell death. Adv Pharmacol (2018) 82:57–83. doi: 10.1016/bs.apha.2017.09.003
26. Zhou L, Zhu DY. Neuronal nitric oxide synthase: Structure, subcellular localization, regulation, and clinical implications. Nitric Oxide (2009) 20(4):223–30. doi: 10.1016/j.niox.2009.03.001
27. Lu Q, Harris VA, Rafikov R, Sun X, Kumar S, Black SM. Nitric oxide induces hypoxia ischemic injury in the neonatal brain Via the disruption of neuronal iron metabolism. Redox Biol (2015) 6:112–21. doi: 10.1016/j.redox.2015.06.007
28. Luo CX, Jin X, Cao CC, Zhu MM, Wang B, Chang L, et al. Bidirectional regulation of neurogenesis by neuronal nitric oxide synthase derived from neurons and neural stem cells. Stem Cells (2010) 28(11):2041–52. doi: 10.1002/stem.522
29. Weitzdoerfer R, Hoeger H, Engidawork E, Engelmann M, Singewald N, Lubec G, et al. Neuronal nitric oxide synthase knock-out mice show impaired cognitive performance. Nitric Oxide (2004) 10(3):130–40. doi: 10.1016/j.niox.2004.03.007
30. Ridnour LA, Thomas DD, Donzelli S, Espey MG, Roberts DD, Wink DA, et al. The biphasic nature of nitric oxide responses in tumor biology. Antioxid Redox Signal (2006) 8(7-8):1329–37. doi: 10.1089/ars.2006.8.1329
31. Krischel V, Bruch-Gerharz D, Suschek C, Kröncke KD, Ruzicka T, Kolb-Bachofen V. Biphasic effect of exogenous nitric oxide on proliferation and differentiation in skin derived keratinocytes but not fibroblasts. J Invest Dermatol (1998) 111(2):286–91. doi: 10.1046/j.1523-1747.1998.00268.x
32. Ying L, Hofseth LJ. An emerging role for endothelial nitric oxide synthase in chronic inflammation and cancer. Cancer Res (2007) 67(4):1407–10. doi: 10.1158/0008-5472.Can-06-2149
34. Fukumura D, Kashiwagi S, Jain RK. The role of nitric oxide in tumour progression. Nat Rev Cancer (2006) 6(7):521–34. doi: 10.1038/nrc1910
35. Folkman J. Role of angiogenesis in tumor growth and metastasis. Semin Oncol (2002) 29(6 Suppl 16):15–8. doi: 10.1053/sonc.2002.37263
36. Cooke JP. No and angiogenesis. Atheroscler Suppl (2003) 4(4):53–60. doi: 10.1016/s1567-5688(03)00034-5
37. Babaei S, Teichert-Kuliszewska K, Monge JC, Mohamed F, Bendeck MP, Stewart DJ. Role of nitric oxide in the angiogenic response in vitro to basic fibroblast growth factor. Circ Res (1998) 82(9):1007–15. doi: 10.1161/01.res.82.9.1007
38. Zhou J, Brüne B. No and transcriptional regulation: From signaling to death. Toxicology (2005) 208(2):223–33. doi: 10.1016/j.tox.2004.11.021
39. Schmid T, Zhou J, Brüne B. Hif-1 and P53: Communication of transcription factors under hypoxia. J Cell Mol Med (2004) 8(4):423–31. doi: 10.1111/j.1582-4934.2004.tb00467.x
40. Forrester K, Ambs S, Lupold SE, Kapust RB, Spillare EA, Weinberg WC, et al. Nitric oxide-induced P53 accumulation and regulation of inducible nitric oxide synthase expression by wild-type P53. Proc Natl Acad Sci U.S.A. (1996) 93(6):2442–7. doi: 10.1073/pnas.93.6.2442
41. Ambs S, Merriam WG, Bennett WP, Felley-Bosco E, Ogunfusika MO, Oser SM, et al. Frequent nitric oxide synthase-2 expression in human colon adenomas: Implication for tumor angiogenesis and colon cancer progression. Cancer Res (1998) 58(2):334–41.
42. Ambs S, Ogunfusika MO, Merriam WG, Bennett WP, Billiar TR, Harris CC. Up-regulation of inducible nitric oxide synthase expression in cancer-prone P53 knockout mice. Proc Natl Acad Sci U.S.A. (1998) 95(15):8823–8. doi: 10.1073/pnas.95.15.8823
43. Fang X, Cai S, Wang M, Chen Z, Lu C, Yang H. Photogenerated holes mediated nitric oxide production for hypoxic tumor treatment. Angew Chem Int Ed Engl (2021) 60(13):7046–50. doi: 10.1002/anie.202015082
44. Fan W, Yung BC, Chen X. Stimuli-responsive no release for on-demand gas-sensitized synergistic cancer therapy. Angew Chem Int Ed Engl (2018) 57(28):8383–94. doi: 10.1002/anie.201800594
45. Sun T, Ding Y, Wang X, Zhang K, Zhang GP, Liang D, et al. Carry-on nitric-oxide luggage for enhanced chemotherapeutic efficacy. Nano Lett (2020) 20(7):5275–83. doi: 10.1021/acs.nanolett.0c01532
46. Jiang W, Dong W, Li M, Guo Z, Wang Q, Liu Y, et al. Nitric oxide induces immunogenic cell death and potentiates cancer immunotherapy. ACS Nano (2022) 16(3):3881–94. doi: 10.1021/acsnano.1c09048
47. Yao W, Wang K, Guo Y, Wei R, Luo S, Tang W, et al. Nitric oxide nano-prodrug platform with synchronous glutathione depletion and hypoxia relief for enhanced photodynamic cancer therapy. Biomater Adv (2022) 133:112616. doi: 10.1016/j.msec.2021.112616
48. Alimoradi H, Greish K, Gamble AB, Giles GI. Controlled delivery of nitric oxide for cancer therapy. Pharm Nanotechnol (2019) 7(4):279–303. doi: 10.2174/2211738507666190429111306
49. Sarati LI, Martinez CR, Artés N, Arreche N, López-Costa JJ, Balaszczuk AM, et al. Hypothyroidism: Age-related influence on cardiovascular nitric oxide system in rats. Metabolism (2012) 61(9):1301–11. doi: 10.1016/j.metabol.2012.01.022
50. Baghcheghi Y, Beheshti F, Salmani H, Soukhtanloo M, Hosseini M. Protective effect of pparγ agonists on cerebellar tissues oxidative damage in hypothyroid rats. Neurol Res Int (2016) 2016:1952561. doi: 10.1155/2016/1952561
51. Haddow JE, Palomaki GE, Allan WC, Williams JR, Knight GJ, Gagnon J, et al. Maternal thyroid deficiency during pregnancy and subsequent neuropsychological development of the child. N Engl J Med (1999) 341(8):549–55. doi: 10.1056/nejm199908193410801
52. Morreale de Escobar G, Obregon MJ, Escobar del Rey F. Role of thyroid hormone during early brain development. Eur J Endocrinol (2004) 151 Suppl 3:U25–37. doi: 10.1530/eje.0.151u025
53. Sinha RA, Pathak A, Mohan V, Bandyopadhyay S, Rastogi L, Godbole MM. Maternal thyroid hormone: A strong repressor of neuronal nitric oxide synthase in rat embryonic neocortex. Endocrinology (2008) 149(9):4396–401. doi: 10.1210/en.2007-1617
54. Purtell K, Roepke TK, Abbott GW. Cardiac arrhythmia and thyroid dysfunction: A novel genetic link. Int J Biochem Cell Biol (2010) 42(11):1767–70. doi: 10.1016/j.biocel.2010.07.013
55. Rodríguez L, Detomaso F, Braga P, Prendes M, Perosi F, Cernadas G, et al. Neonatal hyperthyroidism on rat heart: Interrelation with nitric oxide and sex. J Endocrinol Invest (2015) 38(6):685–94. doi: 10.1007/s40618-015-0244-4
56. Kayser L, Francis D, Broholm H. Immunohistochemical localization of inducible and endothelial constitutive nitric oxide synthase in neoplastic and autoimmune thyroid disorders. Apmis (2000) 108(11):785–91. doi: 10.1034/j.1600-0463.2000.d01-30.x
57. Choe W, Kim S, Hwang TS, Lee SS. Expression of inducible nitric oxide synthase in thyroid neoplasms: Immunohistochemical and molecular analysis. Pathol Int (2003) 53(7):434–9. doi: 10.1046/j.1440-1827.2003.01501.x
58. Sousa MS, Latini FR, Monteiro HP, Cerutti JM. Arginase 2 and nitric oxide synthase: Pathways associated with the pathogenesis of thyroid tumors. Free Radic Biol Med (2010) 49(6):997–1007. doi: 10.1016/j.freeradbiomed.2010.06.006
59. Patel A, Fenton C, Terrell R, Powers PA, Dinauer C, Tuttle RM, et al. Nitrotyrosine, inducible nitric oxide synthase (Inos), and endothelial nitric oxide synthase (Enos) are increased in thyroid tumors from children and adolescents. J Endocrinol Invest (2002) 25(8):675–83. doi: 10.1007/bf03345100
60. Nakamura Y, Yasuoka H, Zuo H, Takamura Y, Miyauchi A, Nakamura M, et al. Nitric oxide in papillary thyroid carcinoma: Induction of vascular endothelial growth factor d and correlation with lymph node metastasis. J Clin Endocrinol Metab (2006) 91(4):1582–5. doi: 10.1210/jc.2005-1790
61. Soler MN, Bobé P, Benihoud K, Lemaire G, Roos BA, Lausson S. Gene therapy of rat medullary thyroid cancer by naked nitric oxide synthase ii DNA injection. J Gene Med (2000) 2(5):344–52. doi: 10.1002/1521-2254(200009/10)2:5<344
62. Ragot T, Provost C, Prignon A, Cohen R, Lepoivre M, Lausson S. Apoptosis induction by combination of drugs or a conjugated molecule associating non-steroidal anti-inflammatory and nitric oxide donor effects in medullary thyroid cancer models: Implication of the tumor suppressor P73. Thyroid Res (2015) 8:13. doi: 10.1186/s13044-015-0025-3
63. Sun K, Lu X, Yin C, Guo J. In vitro antitumor activity of nano-pulse stimulation on human anaplastic thyroid cancer cells through nitric oxide-dependent mechanisms. Bioelectrochemistry (2022) 145:108093. doi: 10.1016/j.bioelechem.2022.108093
64. Robbins RA, Grisham MB. Nitric oxide. Int J Biochem Cell Biol (1997) 29(6):857–60. doi: 10.1016/s1357-2725(96)00167-7
65. Cabanillas ME, McFadden DG, Durante C. Thyroid cancer. Lancet (2016) 388(10061):2783–95. doi: 10.1016/s0140-6736(16)30172-6
Keywords: nitric oxide, thyroid carcinoma, endocrine system, eitric oxide synthase, treatment
Citation: Huang Y, Suguro R, Hu W, Zheng J, Liu Y, Guan M, Zhou N and Zhang X (2023) Nitric oxide and thyroid carcinoma: A review. Front. Endocrinol. 13:1050656. doi: 10.3389/fendo.2022.1050656
Received: 22 September 2022; Accepted: 14 December 2022;
Published: 09 January 2023.
Edited by:
Yicheng Mao, Fudan University, ChinaReviewed by:
Xiaocong Pang, First Hospital, Peking University, ChinaMinhua Zang, Shanghai Jiao Tong University, China
Copyright © 2023 Huang, Suguro, Hu, Zheng, Liu, Guan, Zhou and Zhang. This is an open-access article distributed under the terms of the Creative Commons Attribution License (CC BY). The use, distribution or reproduction in other forums is permitted, provided the original author(s) and the copyright owner(s) are credited and that the original publication in this journal is cited, in accordance with accepted academic practice. No use, distribution or reproduction is permitted which does not comply with these terms.
*Correspondence: Na Zhou, bnpob3VAbXVzdC5lZHUubW8=; Xin Zhang, eGluemhhbmdAbXVzdC5lZHUubW8=