- 1Radboud Institute for Molecular Life Sciences, Department of Physiology, Radboudumc, Nijmegen, Netherlands
- 2Karolinska Institutet, Division of Renal Medicine, Department of Clinical Science, Intervention and Technology, Stockholm, Sweden
- 3Linköpings universitet Hälsouniversitetet, Department of Health, Medicine and Caring Sciences, Linköping, Sweden
- 4Karolinska Institutet, Division of Clinical Geriatrics, Department of Neurobiology, Department of Care Sciences and Society, Stockholm, Sweden
Background: Disturbances in magnesium homeostasis are common in patients with chronic kidney disease (CKD) and are associated with increased mortality. The kidney is a key organ in maintaining normal serum magnesium concentrations. To this end, fractional excretion of magnesium (FEMg) increases as renal function declines. Despite recent progress, the hormonal regulation of renal magnesium handling is incompletely understood. Fibroblast Growth Factor 23 (FGF23) is a phosphaturic hormone that has been linked to renal magnesium handling. However, it has not yet been reported whether FGF23 is associated with renal magnesium handling in CKD patients.
Methods: The associations between plasma FGF23 levels, plasma and urine magnesium concentrations and FEMg was investigated in a cross-sectional cohort of 198 non-dialysis CKD patients undergoing renal biopsy.
Results: FGF23 was significantly correlated with FEMg (Pearson’s correlation coefficient = 0.37, p<0.001) and urinary magnesium (-0.14, p=0.04), but not with plasma magnesium. The association between FGF23 and FEMg remained significant after adjusting for potential confounders, including estimated glomerular filtration rate (eGFR), parathyroid hormone and 25-hydroxyvitamin D.
Conclusions: We report that plasma FGF23 is independently associated with measures of renal magnesium handling in a cohort of non-dialysis CKD patients. A potential causal relationship should be investigated in future studies.
1 Introduction
Magnesium ions (Mg2+) are essential in several biological processes such as cell signaling, energy metabolism, growth and proliferation. Normomagnesemia is maintained between 0.7 and 1.1 mmol/L through an elaborate coordination between the kidney, bone and intestine. Renal excretion of Mg2+ is a controlled process (1) fine-tuned in the distal convoluted tubule (DCT). In the DCT, regulated Mg2+ reabsorption occurs through the transient receptor potential melastatin (TRPM) 6 (2). 10-25% filtered Mg2+ is reabsorbed in the proximal tubule and 50-70% is reabsorbed in the thick ascending limb of Henle’s loop. Fine-tuning of Mg2+ reabsorption occurs in the DCT (10%) via an active transcellular process (1). The Mg2+ handling in the kidney is influenced by several factors. Generally, peptide hormones, such as parathyroid hormone (PTH) or vasopressin, increase the Mg2+ transport (3). Interestingly, vitamin D does not influence, in physiological conditions, the Mg2+ homeostasis, yet rodents treated with vitamin D showed an increase in serum levels of Mg2+ and a decrease in renal Mg2+ excretion (4). Electrolytes play an important role in the transport of Mg2+. Calcium (Ca2+) and Mg2+ ions have been shown to activate Calcium Sensing Receptor (CaSR) and regulate paracellular Mg2+ transport (5), while potassium (K+) indirectly influences the Mg2+ transport in the DCT through the voltage-gated channel Kv1.1, which provides an electrical driving force for transcellular Mg2+ transport (6, 7). Nevertheless, the exact regulation of renal Mg2+ handling remains largely unkown. Mutations in the TRPM6 gene results in primary hypomagnesemia with secondary hypocalcemia (PHSH, OMIM# 602014), an autosomal recessive condition characterized by abnormally low serum Mg2+. However, the precise hormonal regulation of TRPM6 in health and in disease has not yet been characterized (8).
Chronic kidney disease (CKD) affects approximately 10% of the population globally and is associated with increased cardiovascular morbidity and mortality (9). Vascular calcification is a key mechanism behind the increased cardiovascular risk associated with CKD (10, 11). In CKD patients the fractional excretion of Mg2+ (FEMg) gradually increases to compensate for the reduced number of functioning nephrons (12). In patients with end stage renal disease (ESRD) the adaptive mechanisms are no longer sufficient to fully compensate for the loss of glomerular filtration capacity, which can lead to hypermagnesemia (13). Also, hypomagnesemia is commonly observed in CKD patients due to reduced intestinal uptake or increased renal losses caused by use of medication such as diuretics and proton-pump inhibitors (14, 15), comorbidities including diabetes and hypertension, or low dietary Mg2+ intake (1).
Both hyper- and hypomagnesemia have been linked to cardiovascular disease and higher overall mortality in patients with CKD (16, 17). Mechanistically, Mg2+ has been demonstrated to inhibit phosphate-induced vascular calcification, and Mg2+ supplementation or Mg2+-based therapies have shown promise in reducing cardiovascular disease in CKD patients (18–22). A better understanding of the hormonal regulation of renal Mg2+ handling, especially in the setting of reduced renal function, is therefore of outmost clinical importance.
Fibroblast Growth Factor 23 (FGF23) is a bone-derived hormone that reduces the apical abundance of the sodium-phosphate co-transporters NPT2A and NPT2C in the proximal tubule, thereby lowering phosphate (Pi) reabsorption and increasing urinary Pi excretion. FGF23 concentrations increase dramatically in CKD patients, likely as an adaptive mechanism to counteract Pi retention (23–25). FGF23 plays an active role in the inhibition of renal 1,25-dihydroxyvitamin D (1,25(OH)2D) synthesis by increasing the expression of the calcitriol-inactivating enzyme 24-hydroxylase, whereas PTH stimulates the 25-hydroxyvitamin D-1α-hydroxylase, which generates 1,25(OH)2D (26). FGF23 and 1,25(OH)2D regulate each other through a feedback loop, as 1,25(OH)2D stimulates FGF23 synthesis in osteoblasts through the presence of a vitamin D response element in the FGF23 promoter (27). Animal studies show that FGF23 inhibits PTH secretion by activating the MAPK pathway, which decreases PTH expression and secretion (28, 29). This mechanism may not be important in humans, as CKD patients display a simultaneous increase in PTH and FGF23 levels (30–32).
Many epidemiological studies have linked increased FGF23 concentrations to worse clinical outcomes, including cardiovascular morbidity and mortality (33, 34), although the evidence of a causal relationship from prospective trials is still lacking.
In addition to regulating Pi balance, FGF23 and its co-receptor Klotho have been linked to renal handling of other electrolytes, including Ca2+, sodium (Na+) and K+ (35–37). There are also some indications that FGF23 may be involved in controlling renal Mg2+ reabsorption. First, mice with diet-induced hypomagnesemia have increased serum FGF23 levels and reduced renal Klotho expression (38, 39), suggesting that dietary Mg2+ can influence the regulation of the FGF23-Klotho axis. Similarly, low plasma Mg2+ concentrations are associated with high plasma FGF23 concentrations and high mortality rates in azotemic cats with CKD (40). Second, a negative association between serum FGF23 and Mg2+ was observed in patients undergoing hemodialysis, supporting a possible interrelationship (41). Third, Mg2+ supplementation was recently reported to reduce vascular calcification in hyperphosphatemic Klotho-deficient mice with high FGF23 concentrations (8).
Herein we set out to test whether FGF23 could be involved in renal Mg2+ handling in patients with non-dialysis CKD, and particularly we hypothesize that FGF23 and FEMg are positively associated.
2 Materials and methods
2.1 Study design and patient inclusion
For the present study we used a cohort of non-dialysis patients with CKD stage 1-5 undergoing kidney biopsy at the Karolinska University Hospital between 2011 and 2017 (KaroKidney – karokidney.org) (23). All patients in which plasma and spot urine Mg2+ were available were included in the analysis. The study received ethical approval (Ethical Review Board, Stockholm, Sweden, DNR 2010/579-31), and informed consent was obtained from all participants.
2.2 Exposure, outcome and covariates
Prior to the kidney biopsy, blood and urine samples were collected and directly analyzed or frozen and stored at -80°C for later biochemical measurements. Intact FGF23 was measured in plasma using a second-generation enzyme-linked immunosorbent assay (ELISA) (Immutopics, San Clemente, CA, USA). Plasma (normal values equal to 0.7-1.1 mmol/L) and urine Mg2+ concentrations were measured using a colorimetric assay (Roche/Hitachi, Tokyo, Japan) according to the manufacturer’s protocol. Absorbance was measured at a 600 nm wavelength on a microplate spectrophotometer (Bio-Rad Laboratories, CA, USA). All Mg2+ measurements were carried out in triplicate. The FEMg (normal range defined as FEMg under 4%) was calculated using the formula [(UMg2+ x PCrea)/(UCrea x PMg2+ x 0.7)] x 100 (where U = urinary, P = plasma, Mg2+ = ionized magnesium, Crea = creatinine) (42). Soluble Klotho and aldosterone were analyzed in serum using ELISA methods (IBL International, Germany and DRG Diagnostics, Germany, respectively). Estimated eGFR was calculated using plasma Cystatin C values.
2.3 Statistical analysis
Data were expressed as mean ± standard deviation (SD) or median with interquartile range (IQR) for continuous variables and percentage of total for categorical variables. Plasma FGF23, Klotho, 25-hydroxyvitamin D, PTH, aldosterone, fractional excretion of Ca2+ (FECa), FEMg, fraction excretion of K+ (FEK), plasma Pi, Ca2+, creatinine, and urinary Ca2+, Mg2+, Pi and creatinine were log10 transformed when used in models, to improve their distribution towards normal. Missing data were imputed by using Predictive Mean Matching with 15 iterations and can be found in Supplementary Table 1.
The cohort was stratified into quartiles, based on the plasma levels of FGF23, and the quartiles were tested for group comparisons using one-way ANOVA and Tukey’s post-hoc test to correct for multiple comparisons.
Pearson’s correlation was used to explore the relationships between FGF23, FEMg, eGFR, age, sex, aldosterone, PTH, Klotho, 25-hydroxyvitamin D, FEK, fractional excretion of Pi (FEPi), FECa, plasma Na+, K+, Ca2+, Pi, Mg2+, urinary Ca2+, and Pi concentrations. All data were compiled in a correlation matrix with all covariates included.
Univariate linear regression models were performed to investigate the effect of FGF23 on FEMg values. Data were expressed as regression coefficient (β), 95% confidence intervals (CI), adjusted R2 and p-value. Multivariate general linear regression models were run to investigate the effect of FGF23 on FEMg. The first model (model 1) consisted of age (years), sex and eGFR (mL/min/1.73 m2). The second model (model 2) included the same parameters as model 1 plus Klotho (pg/mL), 25-hydroxyvitamin D (nmol/L), and PTH (ng/L). The third model (model 3) included model 1 and medication (yes/no): loop diuretics, thiazide, PPI, and beta blockers. The fourth model (model 4) included model 2 and model 3, and the fifth model (model 5) included model 4 and comorbidities (yes/no): hypertension and diabetes. The sixth model (model 6) included model 2 and FECa, while the seventh model (model 7) included model 1 and FECa, FEK and FEPi.
The cohort was divided into quartiles of FGF23 concentrations and quartiles of eGFR, to investigate if the log10FEMg associations are consistent even if the cohort is divided and we see the same trend. Linear regression analysis was used to show the associations.
Differences with a p-value of <0.05 were considered statistically significant. Statistical analyses were performed using statistical software SPSS for Windows, version 25, release 25.0.0.1, 64-bit edition and GraphPad Prism 8 for macOS, version 8.4.3 (471).
3 Results
3.1 Baseline characteristics of study population
The study cohort included a total of 198 CKD patients. Clinical characteristics and relevant laboratory results for the full cohort and FGF23 strata are reported in Table 1. Of the 198 patients, 62.7% were male, the mean age was 50.5 ( ± 17.0) years, the median for FGF23 was 81 (58 – 154) pg/mL and the mean eGFR was 48.7 ( ± 22.3) mL/min/1.73m2. Of the patients, 13.1% were hypomagnesemic and 57.1% presented FEMg values higher than the normal range. Of note, FEMg, FEPi and FEK increased over quartiles of FGF23. All plasma and urine electrolyte values are shown in Supplementary Figure 1.
3.2 Associations between FGF23, covariates and renal magnesium handling
Initially, the relationships between FGF23 and plasma Mg2+, urinary Mg2+ concentrations, and FEMg were explored using Pearson’s correlation (as shown in Figure 1 and Supplementary Table 2). This analysis showed a significant correlation between FGF23, FEMg and urinary Mg2+, but no significant correlation with plasma Mg2+ concentration. Furthermore, plasma FGF23 was negatively correlated with plasma Klotho (r=-0.281, p<0.001), and positively correlated with FEPi (r=0.527, p<0.001) (shown in Figure 1 and Supplementary Table 2).
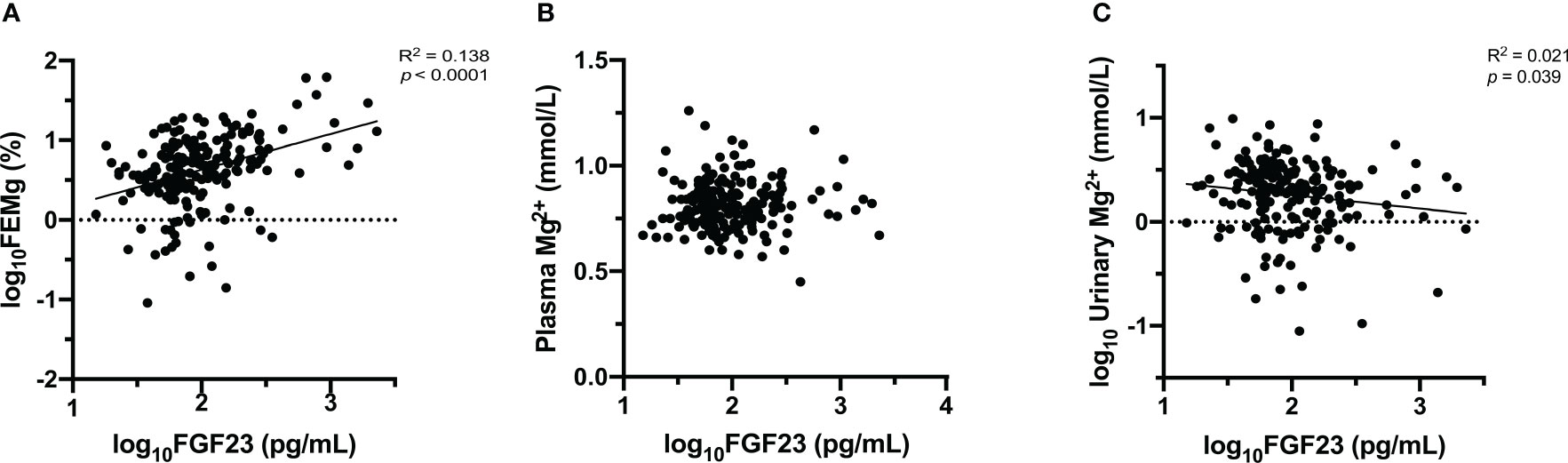
Figure 1 Association between FGF23 and Mg2+ [FEMg (A), plasma Mg2+ (B), urinary Mg2+ (C)]. Data are the outcomes of simple linear regression analysis.
Next, Pearson’s correlation was used to assess the relationship between FEMg and several clinical characteristics. The bivariate analysis demonstrated a negative correlation between FEMg and eGFR (r=-0.397, p<0.001) and an inversed correlation with plasma Klotho (r=-0.261, p<0.001), as seen in Figure 1 and Supplementary Table 2. Further, FEMg was positively and moderately correlated with FEPi (r=0.394, p<0.001) and plasma Pi (r=0.229, p=0.001) (shown in Figure 1 and Supplementary Table 2).
3.3 Univariate and multivariate analyses between FGF23 and renal magnesium handling
Univariate linear regression analysis revealed a significant correlation between plasma FGF23 and FEMg (in Table 2). Conversely, there was no significant correlation between FGF23 and plasma Mg2+ concentration and only a very weak correlation between FGF23 and urinary Mg2+ concentration (as seen in Figure 2).
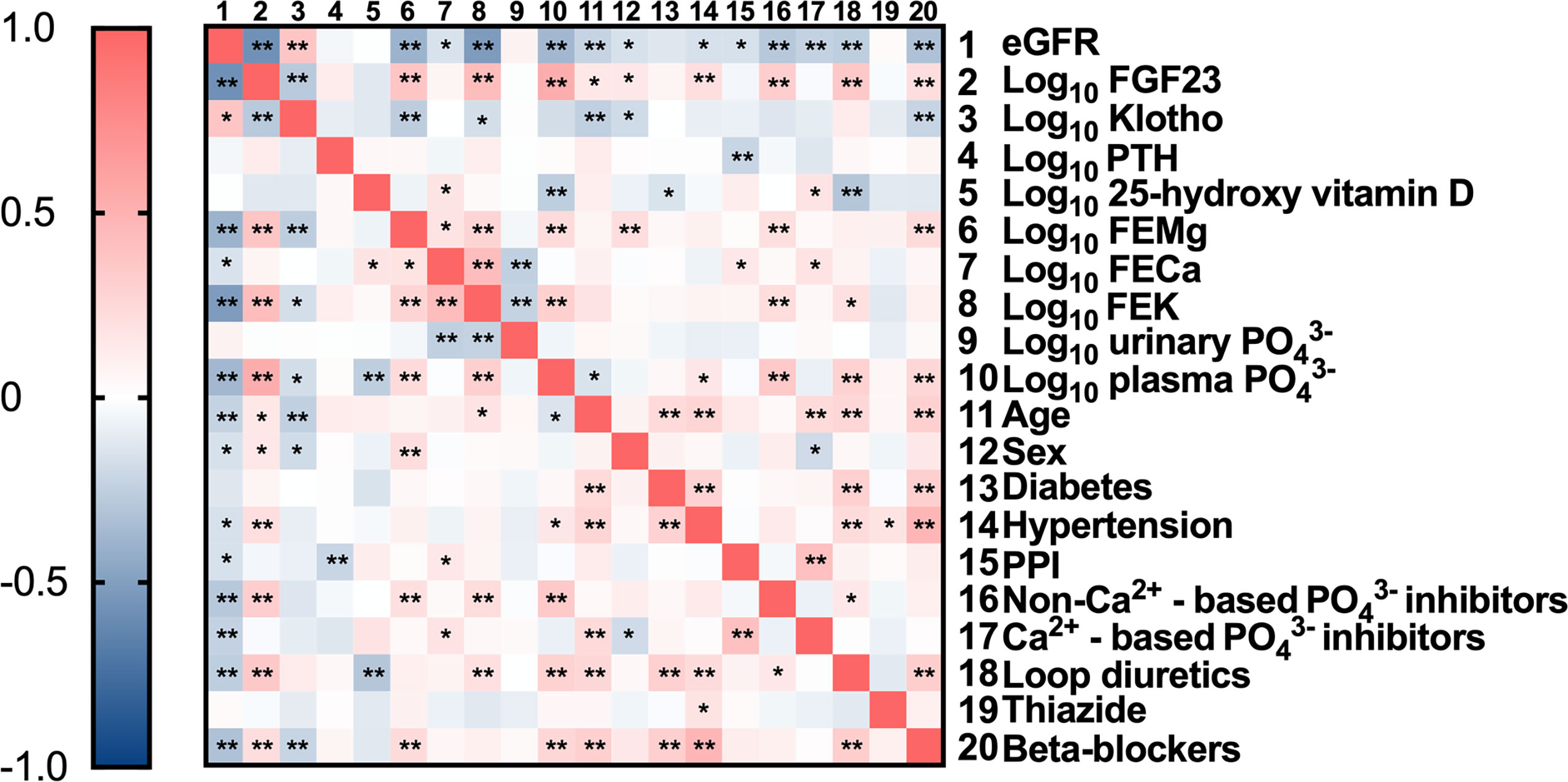
Figure 2 Correlation matrix with relevant parameters included. Data are the outcomes of bivariate analysis; *Correlation is significant at the 0.05 level; **Correlation is significant at the 0.01 level.
Next, we explored the relationship between FEMg and FGF23 after adjustment for potential confounders. In crude linear regression, FGF23 accounts for 37.4% of the variation in FEMg, with an adjusted R2 of 0.135 (p<0.001). After adjustment for age, sex and eGFR (model 1) there was an overall adjusted R2 of 0.184 (p=0.022). In a multiple adjusted linear regression model including age, sex, eGFR, Klotho, 25-hydroxyvitamin D, PTH, there was an overall adjusted R2 of 0.189, (p=0.059). In model 3 (adjusted for use of medication) there was an adjusted R2 of 0.179 (p=0.016), while model 4 showed an overall adjusted R2 of 0.174 (p=0.032). In the adjustment for medication and comorbidities (model 5) there was an adjusted R2 of 0.164 (p=0.029), while in the adjustment for fractional excretions (model 7) there was an adjusted R2 of 0.203 (p=0.080). The adjustment for the Klotho-FGF23 axis (model 6) showed an overall adjusted R2 of 0.197 (p=0.046). Supplementary Tables 3, 4 show that the models in Table 2 were not over-adjusted, using the variance inflation factor and two linear regression models.
3.4 Subgroup analysis by different eGFR and FGF23 stages
To investigate if the associations to FEMg are consistent over the full spectra of FGF23 concentrations and eGFR observed in our cohort we performed sub-group analysis in the different strata of FGF23 and eGFR (shown in Figure 3). The results show that, in the FGF23 strata, the association between FEMg and FGF23 is significant only in the fourth quartile, while the association between FEMg and eGFR is significant in the first, third and fourth quartile. The division in eGFR quartiles demonstrated that FEMg correlates only in the first quartile with FGF23 (in Table 3). The pattern of FEMg is similar throughout FGF23 and eGFR strata – it decreases with the increase of eGFR and it simultaneously increases with FGF23 (Figure 3). Supplementary Figures 2, 3 explore Klotho in CKD using similar analysis.
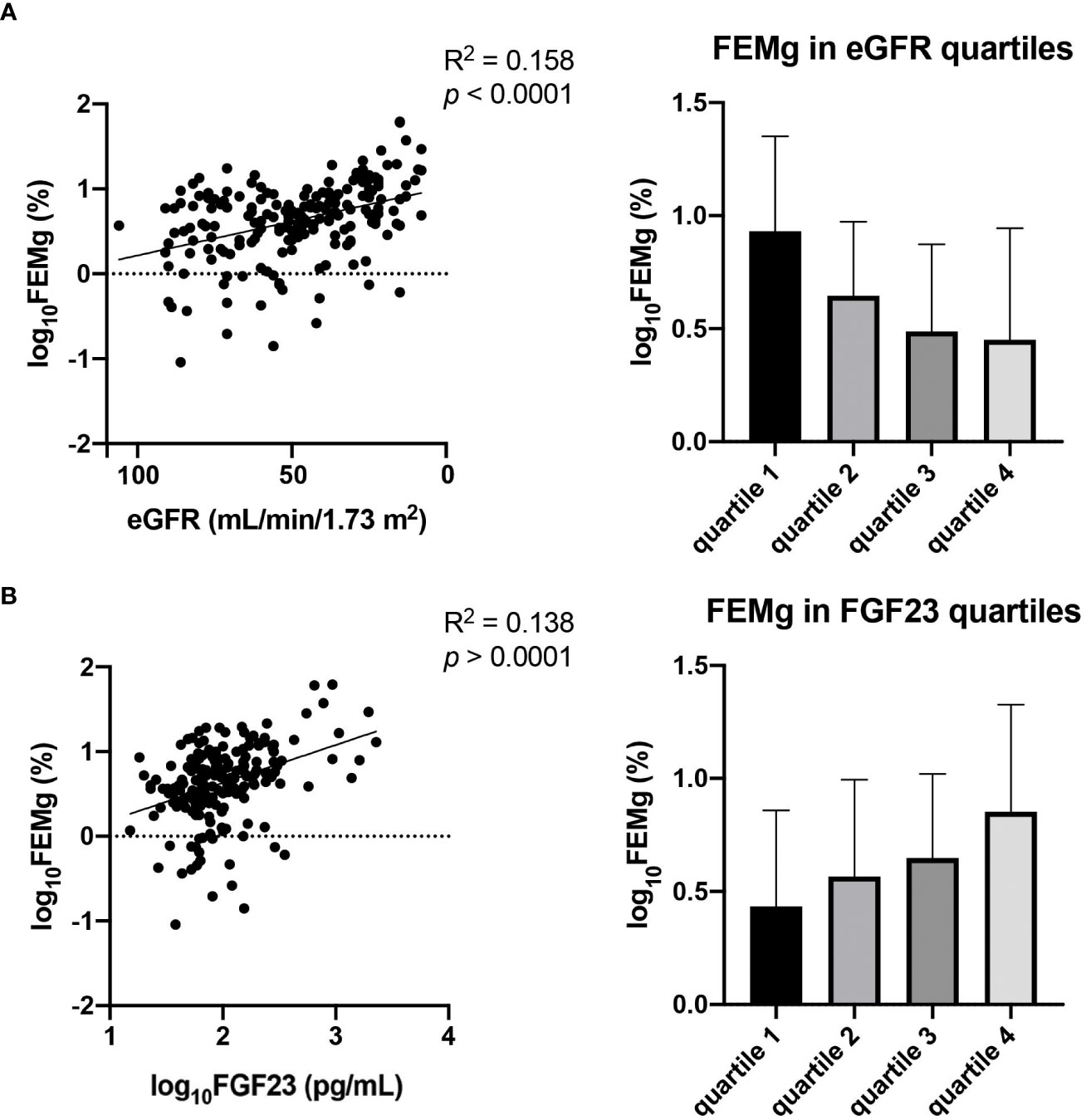
Figure 3 Association between eGFR and FEMg (A) in comparison with the association of FGF23 and FEMg (B). Data are the outcomes of simple linear regression analysis.
4 Discussion
In this study of 198 non-dialysis CKD patients, we demonstrate that FGF23 is positively associated with FEMg independently of renal function and other potential confounders. This finding is consistent with the hypothesis that FGF23 may influence renal magnesium handling in patients with decreased renal function, and/or vice versa (23, 33, 34, 43).
The role of FGF23 in CKD and the associated cardiovascular disease has been the subject of many studies over the last decade (18, 39, 44, 45). As a master regulator of phosphate (46), it is well established that the increase in FGF23 contributes to maintaining phosphate homeostasis in early stages of CKD, and as such protects against vascular calcification. This is evidenced by studies (47) in which FGF23 neutralizing antibodies were given to rats with CKD, where the reduction of FGF23 resulted in hyperphosphatemia and significantly worsen vascular calcification. However, it is still debated whether the dramatically increased FGF23 concentrations commonly observed in late-stage CKD patients have direct and detrimental effects on the cardiovascular system.
Similarly, the role of Mg2+ homeostasis in CKD patients has received increasing attention over the last few years, especially in the context of vascular calcification and other cardiovascular morbidities (17, 20, 48). There have been some indications that Mg2+ is involved in controlling FGF23 levels (38, 39), and that FGF23-Klotho signaling might influence renal magnesium handling. A potential mechanism for such relationship might be that FGF23-Klotho signaling in the DCT controls TRPM6 expression and/or activity. This is supported by the co-expression of Klotho and TRPM6 in distal tubular cells (49, 50), and by the fact that the renal expression of Klotho and TRPM6 are strongly correlated in healthy individuals as well as in patients with diabetic nephropathy (51).
Although a few studies have reported on associations between FGF23 and Mg2+ in animals or in patients with ESRD, no studies have explored their potential relationship in human non-dialysis CKD (40, 41). In the present study we report a direct and independent association between FGF23 and FEMg, whereas there are no or only weak associations to plasma or urinary Mg2+. This is supportive of the hypothesis that FGF23 may participate in controlling renal Mg2+ reabsorption, to maintain Mg2+ homeostasis as renal function declines (23, 33, 34, 43). The plasma Mg2+ levels were stable across all quartiles, which indicates a sustained intestinal absorption of Mg2+ due to dietary Mg2+ intake (4, 52). However, the cross-sectional nature of this study restricts us from drawing conclusions about any possible causality behind the observed relationship between FEMg and FGF23.
Importantly, as the hormonal regulation of Mg2+ handling is incompletely understood, there could be confounders that we did not consider in our models and that might explain the observed relationship, which could provide a potential mechanism and explanation to the observed associations.
In conclusion, our study demonstrates that FGF23 is positively associated with FEMg in patients with CKD, independently of renal function and other potential confounders. These finding warrants further investigations of the FGF23-Klotho-Pi-Mg2+ axis in patients with CKD and vascular calcification.
Data availability statement
The data that support the findings of this study are not publicly available due to their containing information that could compromise the privacy of research participants, but are available from KaroKidney upon reasonable request.
Ethics statement
The study received ethical approval from the Ethical Review Board, Stockholm, Sweden (DNR 2010/579-31), and informed consent was obtained from all participants.
Author contributions
TG, HO, and JH were involved in the conception and design of the study. TG performed experiments and statistical analysis. AWi, PB, AWe, AB and HO created the biobank. TG, MZ, HO and JH drafted the manuscript. TG, MZ, HX, AWi, PB, AWe, AB, HO and JH critically revised the manuscript. TG, MZ, AWi, PB, AWe, AB, HX, HO and JH approved the final version of the manuscript. All authors contributed to the article and approved the submitted version.
Funding
This work was supported by grants from Region Stockholm (ALF), CIMED, the Swedish Kidney Foundation and Radboud Institute for Molecular Life Sciences.
Acknowledgments
The authors would like to thank Wouter van Megen for his valuable advice when processing the samples.
Conflict of interest
The authors declare that the research was conducted in the absence of any commercial or financial relationships that could be construed as a potential conflict of interest.
Publisher’s note
All claims expressed in this article are solely those of the authors and do not necessarily represent those of their affiliated organizations, or those of the publisher, the editors and the reviewers. Any product that may be evaluated in this article, or claim that may be made by its manufacturer, is not guaranteed or endorsed by the publisher.
Supplementary material
The Supplementary Material for this article can be found online at: https://www.frontiersin.org/articles/10.3389/fendo.2022.1046392/full#supplementary-material
References
1. de Baaij JH, Hoenderop JG, Bindels RJ. Magnesium in man: Implications for health and disease. Physiol Rev (2015) 95(1):1–46. doi: 10.1152/physrev.00012.2014
2. Cao G, Hoenderop JG, Bindels RJ. Insight into the molecular regulation of the epithelial magnesium channel Trpm6. Curr Opin Nephrol Hypertens (2008) 17(4):373–8. doi: 10.1097/MNH.0b013e328303e184
3. Dai LJ, Ritchie G, Kerstan D, Kang HS, Cole DE, Quamme GA. Magnesium transport in the renal distal convoluted tubule. Physiol Rev (2001) 81(1):51–84. doi: 10.1152/physrev.2001.81.1.51
4. Lameris AL, Nevalainen PI, Reijnen D, Simons E, Eygensteyn J, Monnens L, et al. Segmental transport of Ca(2)(+) and Mg(2)(+) along the gastrointestinal tract. Am J Physiol Gastrointest Liver Physiol (2015) 308(3):G206–16. doi: 10.1152/ajpgi.00093.2014
5. Gong Y, Renigunta V, Himmerkus N, Zhang J, Renigunta A, Bleich M, et al. Claudin-14 regulates renal ca(+)(+) transport in response to casr signalling Via a novel microrna pathway. EMBO J (2012) 31(8):1999–2012. doi: 10.1038/emboj.2012.49
6. Curry JN, Yu ASL. Magnesium handling in the kidney. Adv Chronic Kidney Dis (2018) 25(3):236–43. doi: 10.1053/j.ackd.2018.01.003
7. Glaudemans B, van der Wijst J, Scola RH, Lorenzoni PJ, Heister A, van der Kemp AW, et al. A missense mutation in the Kv1.1 voltage-gated potassium channel-encoding gene Kcna1 is linked to human autosomal dominant hypomagnesemia. J Clin Invest (2009) 119(4):936–42. doi: 10.1172/JCI36948
8. Ter Braake AD, Smit AE, Bos C, van Herwaarden AE, Alkema W, van Essen HW, et al. Magnesium prevents vascular calcification in klotho deficiency. Kidney Int (2020) 97(3):487–501. doi: 10.1016/j.kint.2019.09.034
9. Jha V, Garcia-Garcia G, Iseki K, Li Z, Naicker S, Plattner B, et al. Chronic kidney disease: Global dimension and perspectives. Lancet (2013) 382(9888):260–72. doi: 10.1016/S0140-6736(13)60687-X
10. Guerin AP, London GM, Marchais SJ, Metivier F. Arterial stiffening and vascular calcifications in end-stage renal disease. Nephrol Dial Transplant (2000) 15(7):1014–21. doi: 10.1093/ndt/15.7.1014
11. Floege J, Ketteler M. Vascular calcification in patients with end-stage renal disease. Nephrol Dial Transplant (2004) 19(Suppl 5):V59–66. doi: 10.1093/ndt/gfh1058
12. Rodelo-Haad C, Pendon-Ruiz de Mier MV, Diaz-Tocados JM, Martin-Malo A, Santamaria R, Munoz-Castaneda JR, et al. The role of disturbed mg homeostasis in chronic kidney disease comorbidities. Front Cell Dev Biol (2020) 8:543099. doi: 10.3389/fcell.2020.543099
13. Coburn JW, Popovtzer MM, Massry SG, Kleeman CR. The physicochemical state and renal handling of divalent ions in chronic renal failure. Arch Intern Med (1969) 124(3):302–11. doi: 10.1001/archinte.1969.00300190042007
14. Mikolasevic I, Milic S, Stimac D, Zaputovic L, Lukenda Zanko V, Gulin T, et al. Is there a relationship between hypomagnesemia and proton-pump inhibitors in patients on chronic hemodialysis? Eur J Intern Med (2016) 30:99–103. doi: 10.1016/j.ejim.2016.01.026
15. Nakashima A, Ohkido I, Yokoyama K, Mafune A, Urashima M, Yokoo T. Proton pump inhibitor use and magnesium concentrations in hemodialysis patients: A cross-sectional study. PloS One (2015) 10(11):e0143656. doi: 10.1371/journal.pone.0143656
16. Celi LA, Scott DJ, Lee J, Nelson R, Alper SL, Mukamal KJ, et al. Association of hypermagnesemia and blood pressure in the critically ill. J Hypertens (2013) 31(11):2136–41. doi: 10.1097/HJH.0b013e3283642f18
17. Azem R, Daou R, Bassil E, Anvari EM, Taliercio JJ, Arrigain S, et al. Serum magnesium, mortality and disease progression in chronic kidney disease. BMC Nephrol (2020) 21(1):49. doi: 10.1186/s12882-020-1713-3
18. Ter Braake AD, Shanahan CM, de Baaij JHF. Magnesium counteracts vascular calcification: Passive interference or active modulation? Arterioscler Thromb Vasc Biol (2017) 37(8):1431–45. doi: 10.1161/ATVBAHA.117.309182
19. Turgut F, Kanbay M, Metin MR, Uz E, Akcay A, Covic A. Magnesium supplementation helps to improve carotid intima media thickness in patients on hemodialysis. Int Urol Nephrol (2008) 40(4):1075–82. doi: 10.1007/s11255-008-9410-3
20. Massy ZA, Drueke TB. Magnesium and outcomes in patients with chronic kidney disease: Focus on vascular calcification, atherosclerosis and survival. Clin Kidney J (2012) 5(Suppl 1):i52–61. doi: 10.1093/ndtplus/sfr167
21. Leenders NHJ, Bos C, Hoekstra T, Schurgers LJ, Vervloet MG, Hoenderop JGJ. Dietary magnesium supplementation inhibits abdominal vascular calcification in an experimental animal model of chronic kidney disease. Nephrol Dial Transplant (2022) 37(6):1049–58. doi: 10.1093/ndt/gfac026
22. Xu C, Smith ER, Tiong MK, Ruderman I, Toussaint ND. Interventions to attenuate vascular calcification progression in chronic kidney disease: A systematic review of clinical trials. J Am Soc Nephrol (2022) 33(5):1011–32. doi: 10.1681/ASN.2021101327
23. Xu H, Hashem A, Witasp A, Mencke R, Goldsmith D, Barany P, et al. Fibroblast growth factor 23 is associated with fractional excretion of sodium in patients with chronic kidney disease. Nephrol Dial Transplant (2019) 34(12):2051–7. doi: 10.1093/ndt/gfy315
24. Vervloet MG. Fgf23 measurement in chronic kidney disease: What is it really reflecting? Clin Chim Acta (2020) 505:160–6. doi: 10.1016/j.cca.2020.03.013
25. Larsson T, Nisbeth U, Ljunggren O, Juppner H, Jonsson KB. Circulating concentration of fgf-23 increases as renal function declines in patients with chronic kidney disease, but does not change in response to variation in phosphate intake in healthy volunteers. Kidney Int (2003) 64(6):2272–9. doi: 10.1046/j.1523-1755.2003.00328.x
26. Prie D, Friedlander G. Reciprocal control of 1,25-dihydroxyvitamin d and Fgf23 formation involving the Fgf23/Klotho system. Clin J Am Soc Nephrol (2010) 5(9):1717–22. doi: 10.2215/CJN.02680310
27. Liu S, Tang W, Zhou J, Stubbs JR, Luo Q, Pi M, et al. Fibroblast growth factor 23 is a counter-regulatory phosphaturic hormone for vitamin d. J Am Soc Nephrol (2006) 17(5):1305–15. doi: 10.1681/ASN.2005111185
28. Ben-Dov IZ, Galitzer H, Lavi-Moshayoff V, Goetz R, Kuro-o M, Mohammadi M, et al. The parathyroid is a target organ for Fgf23 in rats. J Clin Invest (2007) 117(12):4003–8. doi: 10.1172/JCI32409
29. Olauson H, Lindberg K, Amin R, Sato T, Jia T, Goetz R, et al. Parathyroid-specific deletion of klotho unravels a novel calcineurin-dependent Fgf23 signaling pathway that regulates pth secretion. PloS Genet (2013) 9(12):e1003975. doi: 10.1371/journal.pgen.1003975
30. Isakova T, Wahl P, Vargas GS, Gutierrez OM, Scialla J, Xie H, et al. Fibroblast growth factor 23 is elevated before parathyroid hormone and phosphate in chronic kidney disease. Kidney Int (2011) 79(12):1370–8. doi: 10.1038/ki.2011.47
31. Galitzer H, Ben-Dov IZ, Silver J, Naveh-Many T. Parathyroid cell resistance to fibroblast growth factor 23 in secondary hyperparathyroidism of chronic kidney disease. Kidney Int (2010) 77(3):211–8. doi: 10.1038/ki.2009.464
32. Komaba H, Fukagawa M. Fgf23-parathyroid interaction: Implications in chronic kidney disease. Kidney Int (2010) 77(4):292–8. doi: 10.1038/ki.2009.466
33. Fragoso A, Silva AP, Gundlach K, Buchel J, Neves PL. Magnesium and fgf-23 are independent predictors of pulse pressure in pre-dialysis diabetic chronic kidney disease patients. Clin Kidney J (2014) 7(2):161–6. doi: 10.1093/ckj/sfu003
34. Musgrove J, Wolf M. Regulation and effects of Fgf23 in chronic kidney disease. Annu Rev Physiol (2020) 82:365–90. doi: 10.1146/annurev-physiol-021119-034650
35. Cha SK, Hu MC, Kurosu H, Kuro-o M, Moe O, Huang CL. Regulation of renal outer medullary potassium channel and renal k(+) excretion by klotho. Mol Pharmacol (2009) 76(1):38–46. doi: 10.1124/mol.109.055780
36. Imura A, Tsuji Y, Murata M, Maeda R, Kubota K, Iwano A, et al. Alpha-klotho as a regulator of calcium homeostasis. Science (2007) 316(5831):1615–8. doi: 10.1126/science.1135901
37. Hu MC, Kuro-o M, Moe OW. Renal and extrarenal actions of klotho. Semin Nephrol (2013) 33(2):118–29. doi: 10.1016/j.semnephrol.2012.12.013
38. Matsuzaki H, Katsumata S, Maeda Y, Kajita Y. Changes in circulating levels of fibroblast growth factor 23 induced by short-term dietary magnesium deficiency in rats. Magnes Res (2016) 29(2):48–54. doi: 10.1684/mrh.2016.0401
39. Matsuzaki H, Kajita Y, Miwa M. Magnesium deficiency increases serum fibroblast growth factor-23 levels in rats. Magnes Res (2013) 26(1):18–23. doi: 10.1684/mrh.2013.0331
40. van den Broek DHN, Chang YM, Elliott J, Jepson RE. Prognostic importance of plasma total magnesium in a cohort of cats with azotemic chronic kidney disease. J Vet Intern Med (2018) 32(4):1359–71. doi: 10.1111/jvim.15141
41. Iguchi A, Watanabe Y, Iino N, Kazama JJ, Iesato H, Narita I. Serum magnesium concentration is inversely associated with fibroblast growth factor 23 in haemodialysis patients. Nephrol (Carlton) (2014) 19(11):667–71. doi: 10.1111/nep.12287
42. Ayuk J, Gittoes NJ. Contemporary view of the clinical relevance of magnesium homeostasis. Ann Clin Biochem (2014) 51(Pt 2):179–88. doi: 10.1177/0004563213517628
43. Felsenfeld AJ, Levine BS, Rodriguez M. Pathophysiology of calcium, phosphorus, and magnesium dysregulation in chronic kidney disease. Semin Dial (2015) 28(6):564–77. doi: 10.1111/sdi.12411
44. Olauson H, Qureshi AR, Miyamoto T, Barany P, Heimburger O, Lindholm B, et al. Relation between serum fibroblast growth factor-23 level and mortality in incident dialysis patients: Are gender and cardiovascular disease confounding the relationship? Nephrol Dial Transplant (2010) 25(9):3033–8. doi: 10.1093/ndt/gfq191
45. Sakaguchi Y, Fujii N, Shoji T, Hayashi T, Rakugi H, Isaka Y. Hypomagnesemia is a significant predictor of cardiovascular and non-cardiovascular mortality in patients undergoing hemodialysis. Kidney Int (2014) 85(1):174–81. doi: 10.1038/ki.2013.327
46. Bacchetta J, Bardet C, Prie D. Physiology of Fgf23 and overview of genetic diseases associated with renal phosphate wasting. Metabolism (2020) 103S:153865. doi: 10.1016/j.metabol.2019.01.006
47. Shalhoub V, Shatzen EM, Ward SC, Davis J, Stevens J, Bi V, et al. Fgf23 neutralization improves chronic kidney disease-associated hyperparathyroidism yet increases mortality. J Clin Invest (2012) 122(7):2543–53. doi: 10.1172/JCI61405
48. Massy ZA, Drueke TB. Magnesium and cardiovascular complications of chronic kidney disease. Nat Rev Nephrol (2015) 11(7):432–42. doi: 10.1038/nrneph.2015.74
49. Chen L, Chou CL, Knepper MA. A comprehensive map of mrnas and their isoforms across all 14 renal tubule segments of mouse. J Am Soc Nephrol (2021) 32(4):897–912. doi: 10.1681/ASN.2020101406
50. He B, Chen P, Zambrano S, Dabaghie D, Hu Y, Moller-Hackbarth K, et al. Single-cell rna sequencing reveals the mesangial identity and species diversity of glomerular cell transcriptomes. Nat Commun (2021) 12(1):2141. doi: 10.1038/s41467-021-22331-9
51. Levin A, Reznichenko A, Witasp A, Liu P, Greasley PJ, Sorrentino A, et al. Novel insights into the disease transcriptome of human diabetic glomeruli and tubulointerstitium. Nephrol Dial Transplant (2020) 35(12):2059–72. doi: 10.1093/ndt/gfaa121
Keywords: FGF23, FEMg, CKD, Klotho, phosphate
Citation: Grigore TV, Zuidscherwoude M, Witasp A, Barany P, Wernerson A, Bruchfeld A, Xu H, Olauson H and Hoenderop J (2023) Fibroblast growth factor 23 is independently associated with renal magnesium handling in patients with chronic kidney disease. Front. Endocrinol. 13:1046392. doi: 10.3389/fendo.2022.1046392
Received: 16 September 2022; Accepted: 28 November 2022;
Published: 09 January 2023.
Edited by:
Seerapani Gopaluni, University of Cambridge, United KingdomReviewed by:
Lorenza Magagnoli, University of Milan, ItalyErica Clinkenbeard, Purdue University Indianapolis, United States
Copyright © 2023 Grigore, Zuidscherwoude, Witasp, Barany, Wernerson, Bruchfeld, Xu, Olauson and Hoenderop. This is an open-access article distributed under the terms of the Creative Commons Attribution License (CC BY). The use, distribution or reproduction in other forums is permitted, provided the original author(s) and the copyright owner(s) are credited and that the original publication in this journal is cited, in accordance with accepted academic practice. No use, distribution or reproduction is permitted which does not comply with these terms.
*Correspondence: Joost Hoenderop, am9vc3QuaG9lbmRlcm9wQHJhZGJvdWR1bWMubmw=
†These authors have contributed equally to this work and share last authorship