- 1The Bartholin Institute, Department of Pathology, Rigshospitalet, Copenhagen Biocenter, Denmark
- 2Division of Pediatric and Adolescent Medicine, Oslo University Hospital, Oslo, Norway
- 3Faculty of Medicine, University of Oslo, Oslo, Norway
- 4Department of Medicine, University of Tennessee, Memphis, TN, United States
- 5Faculty of Health and Medical Sciences, University of Copenhagen, Copenhagen, Denmark
Background: At diagnosis of Type 1 Diabetes (T1D), 30% of the beta cells are dormant, i.e. alive, but inactive. This could reduce beta cell destruction, as cellular stress contributes to beta cell damage. However, the beta cells, that are still active, must produce more insulin and are therefore more vulnerable. The inactive beta cells represent a potential for restoring the insulin secretion.
Methods: We analyzed the expression of selected genes in islets from live, newly diagnosed T1D patients from the DiViD study and organ doners with longer duration of T1D, type 2 diabetes (T2D), or no diabetes from the nPOD study. Additionally, analysis of polymorphisms was performed on all the investigated genes.
Findings: Various possibilities were considered for the inactivity of the beta cells: secretion defect, fetal state, hibernation, and insulin resistance. We analyzed genes related to the ceramide and sphingomyelin synthesis and degradation, secretion, circadian rhythm and insulin action, and found changes in T1D islets that resemble fetal dedifferentiation and asynchrony. Furthermore, we found low levels of insulin receptor mRNA in the islets. No polymorphisms were found.
Interpretation: Our findings suggest a secretion defect, but also fetal dedifferentiation and desynchronization in the inactive beta cells. Together with previous evidence, that predisposing factors for T2D are also present for T1D development, we raise the idea to treat individuals with ongoing T1D development prophylactically with T2D medicine like GLP-1 receptor agonists, metformin, or others, combined with anti-inflammatory compounds, in order to reactivate the dormant beta cells, and to prevent autoimmune destruction. T2D mechanisms during T1D development should be investigated further.
Introduction
In the DiViD program (1) with biopsies from patients with newly diagnosed T1D, it has been found that 60% of the beta cells have been destroyed in the ongoing insulitis process, approximately 30% of the beta cells are preserved, but inactive, and approximately 10% actively secrete insulin for maintaining glucose homeostasis (2). However, these few remaining, actively secreting beta cells, must be considered at high risk of immune destruction for several reasons: the cells express high amounts of antigens (3), show ER-stress (4), produce high levels of immunogenic insulin molecules (5), express TTG (tissue trans-glutaminase), which deamidates glutamine to the more risky glutamate (6), and are more sensitive to cytokines (7). Overall, T1D development is seen more frequently in individuals with stressed beta cells, as is seen during the third trimester of pregnancy (8), during virus infections (9), and in association with psychological stress. It might be avoided, at least in animal models, by prophylactic insulin treatment (10), and in individuals with T1D, C-peptide values often increase substantially the first month after a T1D diagnose, when insulin injections are instituted. In humans, prophylactic insulin treatment has not been demonstrated to be efficient (11), possibly due to suboptimal insulin dosage.
A large fraction of the beta cells in the pancreas is inactive at the time of diagnosis. From an individual cell’s perspective, this is appropriate since the cell might be shielded from the destructive forces that attack active cells. The disadvantage is that higher secretory activity, and thus a higher risk of immune attack, is placed on the remaining, active beta cells.
The questions we address in this study are how the beta cells become dormant, and what can be done to prevent the T1D process in reaching clinical disease. We have analyzed islet mRNA expression from the DiViD and nPOD studies to suggest answers. Two of the possible reasons for the inactivity could be that the beta cells enter a fetal mode or a state of hibernation.
Methods
RNA analysis
Human pancreatic tissue was from the DiViD study (12) from newly onset (disease duration 35 days) T1D patients aged 24–35 years (n=5), or from the nPOD study (13): autoantibody-positive (n=12), T1D patients of 5 [0-21] years diabetes duration (n=20), T2D patients with 2 [0-15] years duration (n=8), pancreas-transplanted T1D patients with failure of the transplant (n=4), and healthy controls (n=18). The DiViD study was approved by The Norwegian government’s Regional Ethics Committee (reference 2009/1907) and the nPOD study by the University of Tennessee Health Science Center’s Local Institutional Review board (reference 10-00848-XM). Direct to lars.krogvold@gmail.com for requests to the datasets. In contrast to the living patients in the DiViD study, the nPOD study consists of pancreases from organ donors following accidental death. Frozen sections were used for laser capture dissection (14) and islets from two to five sections were pooled, and RNA was extracted, using the Arcturus PicoPure RNA Isolation Kit (Applied Biosystems, Grand Island, NY, USA), and quantified on a Bioanalyzer 2100 instrument (Agilent Technologies, Santa Clara, CA, USA). Gene expression analysis was carried out using Affymetrix expression arrays (GeneChip Human Gene 2.0 ST, Thermo Fisher) and normalized using global scaling (15). To maximize comparison, all tissue handling was performed in the same laboratory, by the same technicians, analyzed on the same equipment, and the RNA quality was certified by RIN value measurements (> 3.5).
GWAS analysis
GWAS analysis included the genes shown in Table 2. SNPs were obtained from Onengut-Gumuscu et al (16), using a cut-off p value <0.01. SNPs within ±250 kb flanking regions of the transcription start site of the examined genes were identified, and these SNPs, that were likely to regulate the expression of the associated genes, were identified using Encyclopedia of DNA Elements (ENCODE) from the University of California 186 Santa Cruz genome browser (17) (http://genome.ucsc.edu/), RegulomeDB (18), and data from multiple expression quantitative trait locus (eQTL) studies (19). The cis-eQTL effects were calculated using linear regression models in the selected tissues. Validated eQTLs from Westra et al. (20), and GTEx2015_v6. GTEx2015_v6 eQTLs were computed using a ±1 Mb cis window around the transcription start site. Significance was determined using a Q value threshold, using ≥ 70 samples per tissue to achieve the statistical power needed for this type of analysis. Predicted eQTL was calculated for pancreas and whole blood. Genotype–tissue expression predictions calculation was performed in tissues with at least ten samples. No Q value filtering was performed.
Statistical analysis
Statistical analysis for RNA expression was performed using GraphPad Prism 8.0.2 (GraphPad, La Jolla, Ca, USA) and data are shown as mean ± SEM. Outliers were detected in each group using the ROUT method and a total of 21 data points across all genes and groups were identified and removed. All groups were tested for normal distribution by the D’Agostino–Pearson and Anderson Darling test. For comparison between groups, one-way ANOVA was used with Dunnett’s multiple comparison test and a 95% CI. P <0.05 was considered significant (shown as *p < 0.05, **p < 0.01 and ***p < 0.001 in the figures).
Results
In the DiViD and nPOD material (see patient characteristics in Table 1) we initially looked at expression of genes (Figure 1) coding for enzymes that participate in the maintenance and degradation of ceramide and sphingomyelin and that were not previously examined (21). Interestingly, SMPD1 (also called ASM, acid sphingomyelinase) which degrades sphingomyelin, is reduced in all patient groups related to T1D. This is also the case for SPTSSA, although the reduction in antibody-positive individuals does not reach statistical significance. SGPP1 is also reduced at the diagnosis of T1D, whereas SGPP2 is only reduced in long term T1D individuals. SGMS1, SGPL1, and SPTSSB showed increased expression only in transplanted islets with recurrent diabetes (Tx). Tissue from T2D donors does not show changes in gene expression, except for SGPP1, where the gene is oppositely regulated than in tissue from T1D patients. In addition to the genes in Figure 1, ACER1, ACER3, CEPT1, CHPT1, and PCYT1A (see Table 2 for gene names), were also examined, but were not differentially regulated.
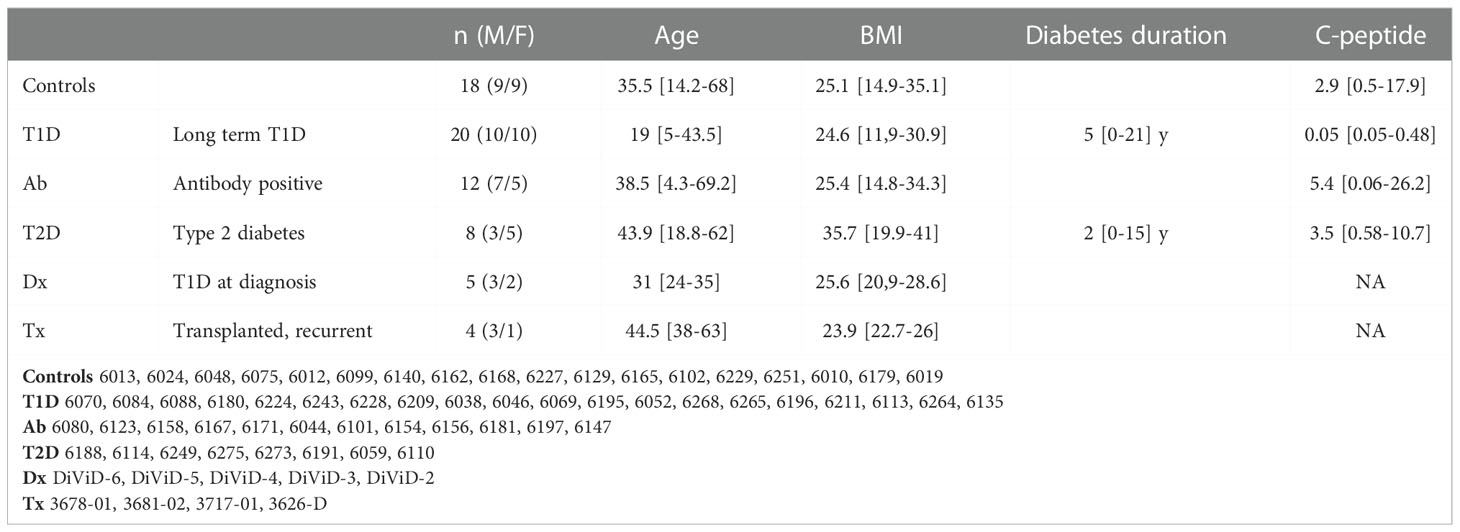
Table 1 Characteristics for the investigated patient groups. Below is shown identification number related to DiViD and nPOD repositories of analyzed samples.
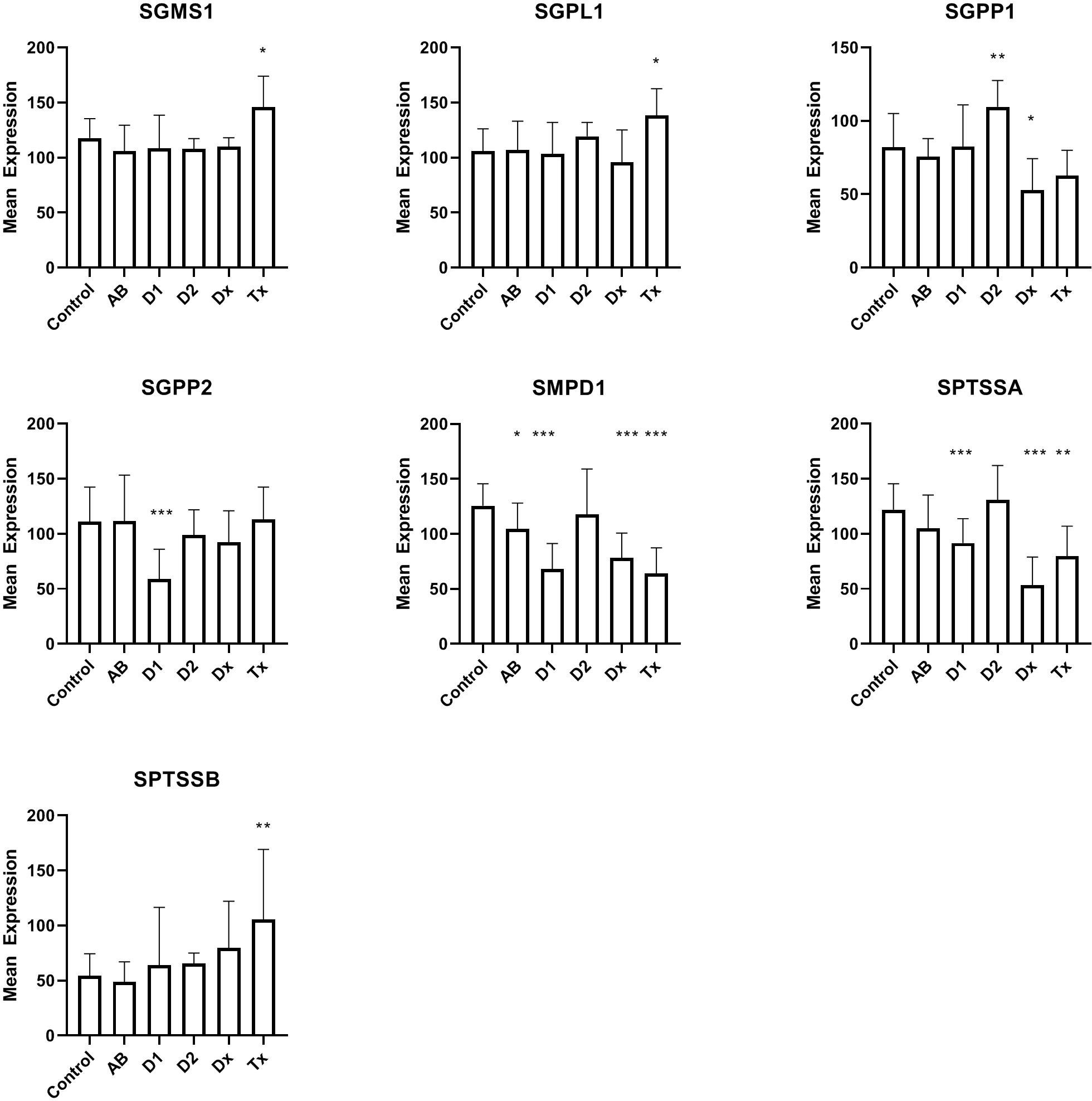
Figure 1 Expression levels of islet of Langerhans mRNA from genes related to synthesis or degradation of ceramide or sphingomyelin. For comparison between groups, one-way ANOVA was used with Dunnett’s multiple comparison test and a 95% CI. C: non-diabetic control patients, AB: non-diabetic subjects with auto-antibodies, D1, Type 1 diabetes of longer duration; D2, Type 2 diabetes; Dx, Newly diagnosed Type 1 DiViD samples; Tx, Biopsies from pancreatic transplants with recurrent diabetes. *p<0.05, **p<0.01, ***p<0.001.
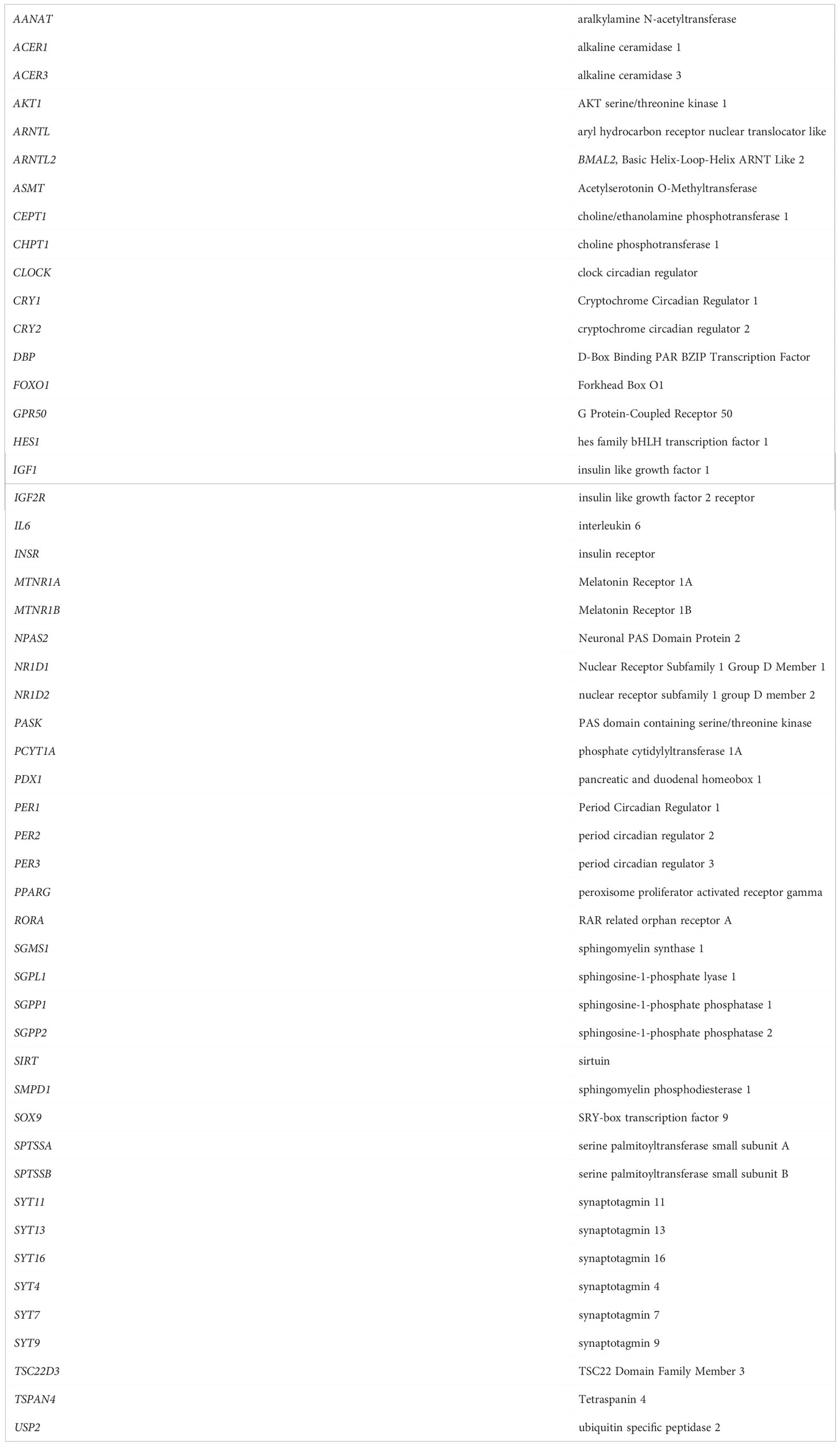
Table 2 List of abbreviations of examined genes and sample numbers from the nPOD and DiViD repository.
In Figure 2 we have analyzed the expression of selected synaptotagmins to investigate the hypothesis that the inactive state of many beta cells could simply be a result of a malfunctioning exocytotic mechanism. Thus, synaptotagmins, some of which binds Ca++, are assumed to be regulators of secretion, primarily in neuronal cells, but they have also been found in pancreatic beta cells. We found that synaptotagmin 4,7,11,13,16 are downregulated in long-standing T1D patients, whereas in newly diagnosed (DIVID) T1D patients only synaptotagmin 16 is downregulated.
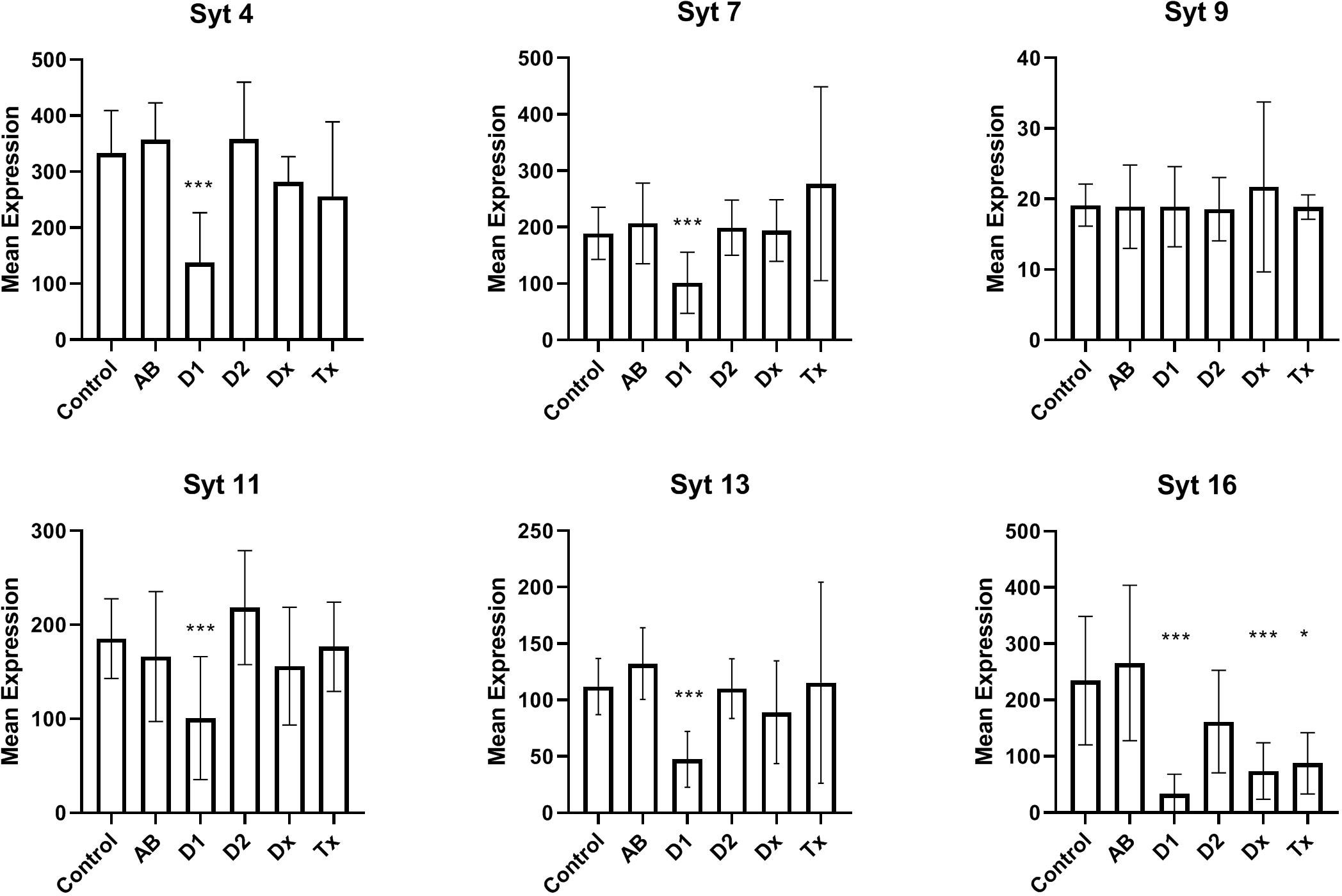
Figure 2 Expression levels of selected synaptotagmins in human islet of Langerhans. See Figure 1 for patient groups and additional information. *p<0.05, **p<0.01, ***p<0.001.
Dormant cells are well known in the animal kingdom during hibernation. This state, that enables species to overcome environmental changes, that is otherwise not compatible with their survival, is only partially understood, but changes in clock genes, otherwise known to control the circadian rhythm, can be detected in many organs during this state. This led us to examine if the inactive beta cells, at the time of T1D diagnosis, could bear resemblance to hibernation by investigating the expression of clock genes (Figure 3). Of 31 investigated genes, that were selected, either because they have been demonstrated as temporally regulated in beta cells or are genes that are well known to regulate the circadian rhythm in cells, 16 genes were differentially regulated, 10 in long lasting T1D, and 7 in tissue from T1D patients at the time of diagnosis (Figure 3). Only 2 genes were differentially regulated in islet tissue from T2D patients compared with controls. We did not find changes in NPAS2, PER1, CRY1, FOXO1, NR1D1, ARNTL2, DBP, TSC22D3, TSPAN4, ASMT, MTNR1A, MTNR1B, or GPR50.
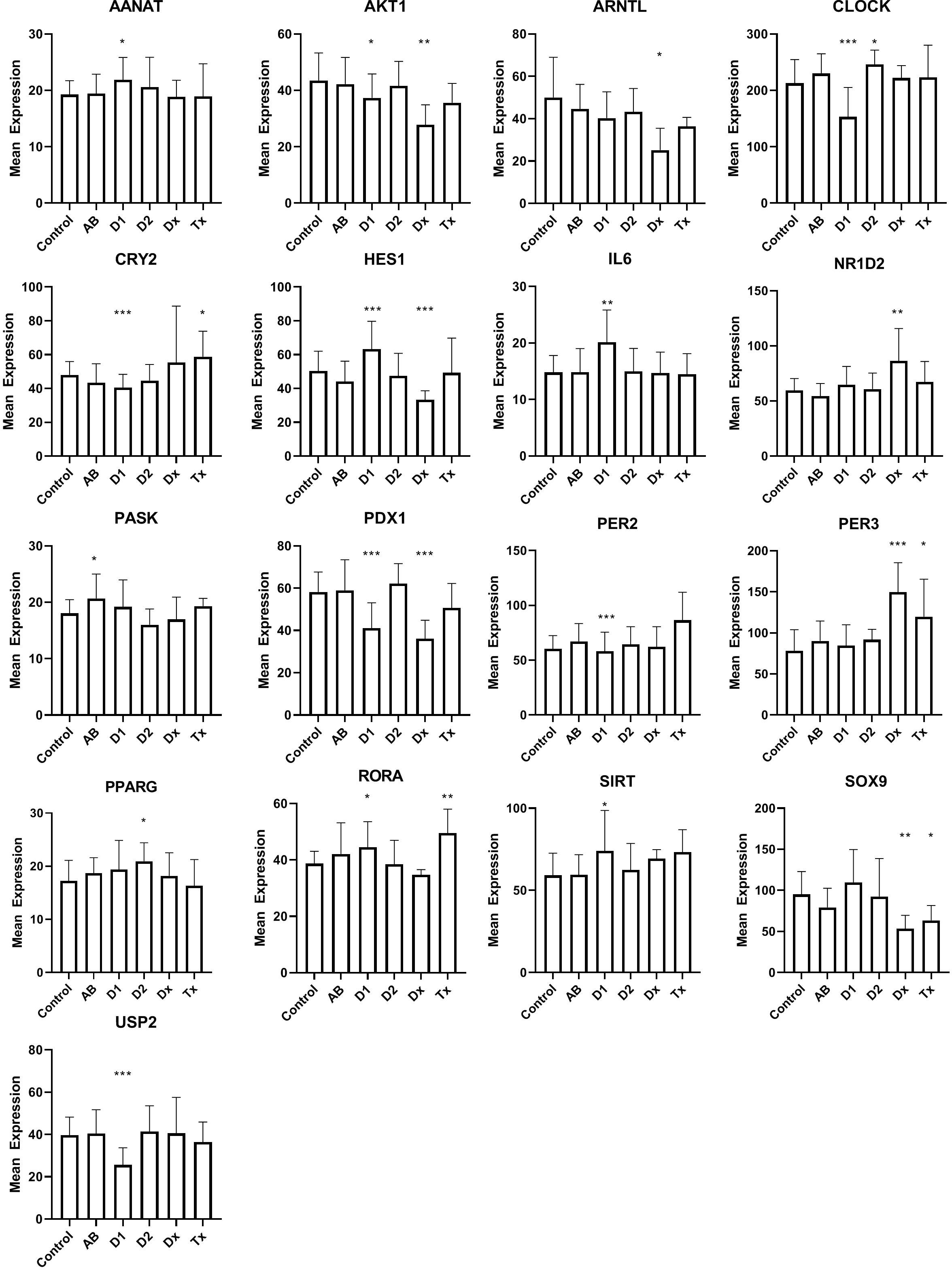
Figure 3 Expression levels of selected genes related to circadian rhythm in islet of Langerhans. See Figure 1 for patient groups and statistics. *p<0.05, **p<0.01, ***p<0.001.
We finally examined the insulin receptors in the beta cells to exclude that changes in their expression could be a reason for dormant state. We found that mRNA for the insulin receptor gene was down-regulated by a factor of two in newly diagnosed T1D patients, but increased in tissue from long lasting T1D patients (Figure 4). Tissue from T2D patients did not show any change. Likewise, the IGF2 receptor gene expression is down-regulated to 66% of the normal value, but no changes are seen for IGF1 receptor.
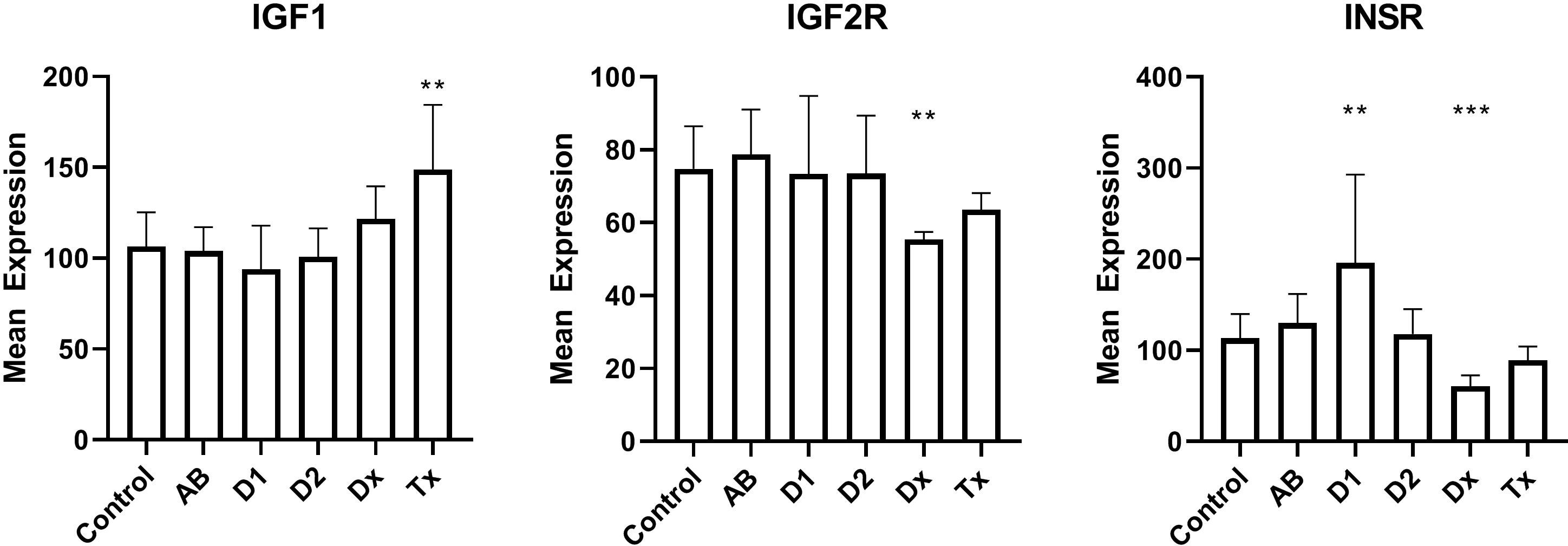
Figure 4 Islet mRNA expression of genes related to insulin action. Patient groups are identified in Figure 1, which also describes the statistical testing. *p<0.05, **p<0.01, ***p<0.001.
We also looked for polymorphisms among all the investigated genes mentioned in Table 2, but found no significant polymorphisms.
Discussion
In the present study we examined islets of Langerhans from live, newly diagnosed T1D humans and the results were compared to T1D patients with longer disease duration and other relevant controls. In the DiViD study enteroviruses were detected in all cases (22–24), and the inactive state of many beta cells could be a rational self-defense mechanism when infected with virus, as resting cells are less vulnerable than actively secreting cells; thus, enterovirus-infected mice with relaxed beta cells, induced by prophylactic insulin treatment, are less sensitive to diabetes development (25). The disadvantage of this inactivation state, however, is that the few remaining beta cells must be highly active and therefore are more vulnerable to immune attack. We therefore found it interesting to investigate the background for the dormant state.
We previously found that a number of genes related to the sphingomyelin synthesis were altered in T1D (21) and identified several additional genes in the present study. Based on expression levels of mRNA, it is not possible to predict changes in sphingomyelin levels, but a decrease in acid sphingomyelinase mRNA expression in tissue from all patient groups related to T1D (newly diagnosed, long standing, antibody-positive, transplantation tissue) compared to controls and T2D patients, is surprising, since decreased levels of acid sphingomyelinase is associated with higher levels of regulatory T cells (26), which is not expected in T1D-related conditions. For reference, increased sphingomyelinase has been found in serum from patients with rheumatoid arthritis (27).
We found decreased expression levels in five of six synaptotagmin mRNAs examined from T1D tissue and also in SYT16 expression from recently diagnosed T1D patients, still without detecting changes in tissue from T2D patients. While data are not available for all synaptotagmins in beta cells, SYT7 has previously been described as reduced in a beta cell model during cytokine stimulation (28) and further attributed to replenishing of insulin granules (29). Various data are available for SYT9, which we did not find altered, as it has both been described as a major Ca++ sensor (30), a regulator of early phase insulin secretion (31), and not involved in insulin secretion (32). SYT4 is decreased in tissue from T1D patients, which could be important, as an increase in SYT4 occurs during postnatal maturation of the beta cells, leading to binding of cellular Ca++ and resulting in a more specific glucose-stimulated insulin secretion. SYT11 and SYT13 were previously found reduced in human T2D islets (33), but not in the present study. In summary, the observed reductions in synaptotagmins could contribute to the diabetic phenotype. Although the SYT4 mRNA reduction is characteristic of undifferentiated, fetal beta cells, a fully, dedifferentiated state is unlikely, as it is not seen in adults (except in insulinomas), and since it is well known that fetal beta cells, exposed to glucose, as it takes place in pregnant T1D patients, develops into the adult phenotype.
As genes regulating the circadian rhythm are changed during hibernation (34), we used their expression to probe the hypothesis that the dormant state of the beta cells, found in human pancreatic T1D tissue, could be related to this condition. Beta cells display a distinct time-of-the-day circadian rhythm (35), so in principle the analysis is problematic, since the tissue is obtained from donors that deceased at different times of the day. If this was detrimental for the analysis, however, we would expect to see random expression levels of the circadian genes across the patient groups, and thus no significant differences. In contrast, we noticed a compelling number of circadian genes differentially regulated for T1D, either at diagnosis and/or in tissue from long-standing T1D patients, and significantly, only 2 genes that were differentially regulated in tissue from T2D patients. Regarding IL-6 we can not, of course, determine whether the signal arose from the beta cells or from infiltrating lymphocytes, but in any case, IL-6 was primarily included as a circadian gene, not as a marker of immunological activity. Together, we find that this suggests that the diabetic condition influences the expression of the circadian genes in the tissue collection. Their importance for insulin secretion is well established. Disruption of CLOCK and BMAL1 was previously shown cause to hypoinsulinaemia and diabetes in mice (36), and changes in the synchronization of the beta cells, resulting in loss of pulsatile or first phase insulin secretion, are predictive for both T1D and T2D (37, 38). Our data therefore support the hypothesis that desynchronized beta cells, also found during hibernation, are associated with the diabetes development.
We finally investigated the possibility that changes in beta cell insulin sensitivity could reduce the insulin secretion during diabetes development. We did see changes in insulin- and insulin-like growth factor 2-receptor expression in tissue from T1D patients (and not in tissue from T2D patients, as was also found by western blotting (39)), but it is not currently clear what the implication of the finding is, since it has been found that a lack of insulin stimulation on beta cells can both decrease (40) and increase (41) insulin stimulation. Interestingly, we and others have found that overweight adolescents, predisposed to T2D, also have a higher risk of developing T1D (42, 43). Furthermore, presence of insulin resistance can actually predict progression to T1D (44).
In summary, the human pancreatic beta cells that we investigated, show traits of embryonic dedifferentiation and changes in synchronization, similar to characteristics of T2D beta cells, and we previously found a relation between T1D development and predisposing factors to T2D disease. In this report, we primarily focused on cellular disease mechanisms, that could explain the inactivity of beta cells in T1D patients. If these mechanisms can be further established, it opens for prophylactic treatment of pre-T1D with GLP-1 analogues, metformin or potassium channel blockers to possibly activate the dormant beta cells. It could be done prophylactically in an early stage, following detection of T1D autoantibodies, and in combination with immunesuppressive medicine. Of note, GLP-1 improves glucose-stimulated insulin secretion and restores glucose competence in glucose-resistant beta-cells (45), and GLP-1 stimulation of SYT7 phosphorylation in pancreatic beta cells (46) might potentially overcome a decreased expression of the protein.
Data availability statement
The raw data supporting the conclusions of this article will be made available by the authors, without undue reservation.
Ethics statement
The studies involving human participants were reviewed and approved by The Norwegian government’s Regional Ethics Committee (reference 2009/1907). The patients/participants provided their written informed consent to participate in this study.
Author contributions
KB conceived the idea behind the study of T2D mechanism and together with KJ arranged the various examinations and wrote the original manuscript draft. KD-J and LK performed the DiViD study. IG performed the RNA expression analysis. FP did the GWAS studies. All authors contributed to the article and approved the submitted version.
Funding
The study was funded by the Axius and Bagger Sørensen foundations.
Conflict of interest
The authors declare that the research was conducted in the absence of any commercial or financial relationships that could be construed as a potential conflict of interest.
Publisher’s note
All claims expressed in this article are solely those of the authors and do not necessarily represent those of their affiliated organizations, or those of the publisher, the editors and the reviewers. Any product that may be evaluated in this article, or claim that may be made by its manufacturer, is not guaranteed or endorsed by the publisher.
References
1. Krogvold L, Wiberg A, Edwin B, Buanes T, Jahnsen FL, Hanssen KF, et al. Insulitis and characterisation of infiltrating T cells in surgical pancreatic tail resections from patients at onset of type 1 diabetes. Diabetologia (2016) 59(3):492–501. doi: 10.1007/s00125-015-3820-4
2. Seiron P, Wiberg A, Kuric E, Krogvold L, Jahnsen FL, Dahl-Jørgensen K, et al. Characterisation of the endocrine pancreas in type 1 diabetes: Islet size is maintained but islet number is markedly reduced. J Pathol Clin Res (2019) 5(4):248–55. doi: 10.1002/cjp2.140
3. Buschard K. The functional state of the beta cells in the pathogenesis of insulin-dependent diabetes mellitus. Autoimmunity (1991) 10(1):65–9. doi: 10.3109/08916939108997149
4. Sahin GS, Lee H, Engin F. An accomplice more than a mere victim: The impact of β-cell er stress on type 1 diabetes pathogenesis. Mol Metab (2021) 54:101365. doi: 10.1016/j.molmet.2021.101365
5. Kracht MJ, van Lummel M, Nikolic T, Joosten AM, Laban S, van der Slik AR, et al. Autoimmunity against a defective ribosomal insulin gene product in type 1 diabetes. Nat Med (2017) 23(4):501–7. doi: 10.1038/nm.4289
6. Marre ML, McGinty JW, Chow IT, DeNicola ME, Beck NW, Kent SC, et al. Modifying enzymes are elicited by er stress, generating epitopes that are selectively recognized by Cd4(+) T cells in patients with type 1 diabetes. Diabetes (2018) 67(7):1356–68. doi: 10.2337/db17-1166
7. Palmer JP, Helqvist S, Spinas GA, Mølvig J, Mandrup-Poulsen T, Andersen HU, et al. Interaction of beta-cell activity and il-1 concentration and exposure time in isolated rat islets of langerhans. Diabetes (1989) 38(10):1211–6. doi: 10.2337/diab.38.10.1211
8. Buschard K, Buch I, Mølsted-Pedersen L, Hougaard P, Kühl C. Increased incidence of true type I diabetes acquired during pregnancy. Br Med J (Clin Res ed) (1987) 294(6567):275–9. doi: 10.1136/bmj.294.6567.275
9. Unsworth R, Wallace S, Oliver NS, Yeung S, Kshirsagar A, Naidu H, et al. New-onset type 1 diabetes in children during covid-19: Multicenter regional findings in the U.K. Diabetes Care (2020) 43(11):e170–e1. doi: 10.2337/dc20-1551
10. Gotfredsen CF, Buschard K, Frandsen EK. Reduction of diabetes incidence of BB wistar rats by early prophylactic insulin treatment of diabetes-prone animals. Diabetologia (1985) 28(12):933–5. doi: 10.1007/bf00703140
11. Greenbaum CJ, Schatz DA, Haller MJ, Sanda S. Through the fog: Recent clinical trials to preserve β-cell function in type 1 diabetes. Diabetes (2012) 61(6):1323–30. doi: 10.2337/db11-1452
12. Krogvold L, Edwin B, Buanes T, Ludvigsson J, Korsgren O, Hyöty H, et al. Pancreatic biopsy by minimal tail resection in live adult patients at the onset of type 1 diabetes: Experiences from the divid study. Diabetologia (2014) 57(4):841–3. doi: 10.1007/s00125-013-3155-y
13. Campbell-Thompson M, Wasserfall C, Kaddis J, Albanese-O’Neill A, Staeva T, Nierras C, et al. Network for pancreatic organ donors with diabetes (Npod): Developing a tissue biobank for type 1 diabetes. Diabetes/Metabol Res Rev (2012) 28(7):608–17. doi: 10.1002/dmrr.2316
14. Richardson SJ, Rodriguez-Calvo T, Gerling IC, Mathews CE, Kaddis JS, Russell MA, et al. Islet cell hyperexpression of hla class I antigens: A defining feature in type 1 diabetes. Diabetologia (2016) 59(11):2448–58. doi: 10.1007/s00125-016-4067-4
15. Wu J, Kakoola DN, Lenchik NI, Desiderio DM, Marshall DR, Gerling IC. Molecular phenotyping of immune cells from young nod mice reveals abnormal metabolic pathways in the early induction phase of autoimmune diabetes. PloS One (2012) 7(10):e46941. doi: 10.1371/journal.pone.0046941
16. Onengut-Gumuscu S, Chen WM, Burren O, Cooper NJ, Quinlan AR, Mychaleckyj JC, et al. Fine mapping of type 1 diabetes susceptibility loci and evidence for colocalization of causal variants with lymphoid gene enhancers. Nat Genet (2015) 47(4):381–6. doi: 10.1038/ng.3245
17. Rosenbloom KR, Sloan CA, Malladi VS, Dreszer TR, Learned K, Kirkup VM, et al. Encode data in the ucsc genome browser: Year 5 update. Nucleic Acids Res (2013) 41(Database issue):D56–63. doi: 10.1093/nar/gks1172
18. Boyle AP, Hong EL, Hariharan M, Cheng Y, Schaub MA, Kasowski M, et al. Annotation of functional variation in personal genomes using regulomedb. Genome Res (2012) 22(9):1790–7. doi: 10.1101/gr.137323.112
19. Consortium G. Human genomics. the genotype-tissue expression (Gtex) pilot analysis: Multitissue gene regulation in humans. Science (2015) 348(6235):648–60. doi: 10.1126/science.1262110
20. Westra HJ, Peters MJ, Esko T, Yaghootkar H, Schurmann C, Kettunen J, et al. Systematic identification of trans eqtls as putative drivers of known disease associations. Nat Genet (2013) 45(10):1238–43. doi: 10.1038/ng.2756
21. Holm LJ, Krogvold L, Hasselby JP, Kaur S, Claessens LA, Russell MA, et al. Abnormal islet sphingolipid metabolism in type 1 diabetes. Diabetologia (2018) 61(7):1650–61. doi: 10.1007/s00125-018-4614-2
22. Krogvold L, Genoni A, Puggioni A, Campani D, Richardson SJ, Flaxman CS, et al. Live enteroviruses, not other viruses, detected in human pancreas at the onset of type 1 diabetes in the divid-study. Diabetologia (2022). doi: 10.1007/s00125-022-05779-2
23. Krogvold L, Edwin B, Buanes T, Frisk G, Skog O, Anagandula M, et al. Detection of a low-grade enteroviral infection in the islets of langerhans of living patients newly diagnosed with type 1 diabetes. Diabetes (2015) 64(5):1682–7. doi: 10.2337/db14-1370
24. Krogvold L, Leete P, Mynarek I, Russell MA, Gerling IC, Lenchik NI, et al. Detection of antiviral tissue responses and increased cell stress in the pancreatic islets of newly diagnosed T1d patients: Results from the divid study. Front Endocrinol (2022) 3:881997. doi: 10.3389/fendo.2022.881997
25. Bock T, Hartoft-Nielsen M-L, Kaas A, Buschard K. Prophylactic insulin treatment reduces diabetes incidence in EMC-M virus-induced diabetes mellitus. Diabetologia (1995) 38:A97.
26. Wiese T, Dennstädt F, Hollmann C, Stonawski S, Wurst C, Fink J, et al. Inhibition of acid sphingomyelinase increases regulatory T cells in humans. Brain Commun (2021) 3(2):fcab020. doi: 10.1093/braincomms/fcab020
27. Chung HY, Wickel J, Stuhlmüller B, Kinne RW, Claus RA. Acid sphingomyelinase activity is elevated in the serum of rheumatoid arthritis patients, suppressed by anti-Tnf-α treatment. Clin Exp Rheumatol (2019) 37 Suppl 122(6):18–9.
28. Kreutzberger AJB, Kiessling V, Doyle CA, Schenk N, Upchurch CM, Elmer-Dixon M, et al. Distinct insulin granule subpopulations implicated in the secretory pathology of diabetes types 1 and 2. eLife (2020) 9:e62506. doi: 10.7554/eLife.62506
29. Dolai S, Xie L, Zhu D, Liang T, Qin T, Xie H, et al. Synaptotagmin-7 functions to replenish insulin granules for exocytosis in human islet β-cells. Diabetes (2016) 65(7):1962–76. doi: 10.2337/db15-1436
30. Gauthier BR, Wollheim CB. Synaptotagmins bind calcium to release insulin. Am J Physiol Endocrinol Metab (2008) 295(6):E1279–86. doi: 10.1152/ajpendo.90568.2008
31. Patak A, Alsharif H, Trombley JE, Rahman M, Bhatnagar S. Synaptotagmin 9 regulates tomosyn-2 protein abundance to affect early phase of insulin secretion. Diabetes (2021) 70(Supp. 1):125–OR.
32. Gustavsson N, Wang X, Wang Y, Seah T, Xu J, Radda GK, et al. Neuronal calcium sensor synaptotagmin-9 is not involved in the regulation of glucose homeostasis or insulin secretion. PloS One (2010) 5(11):e15414. doi: 10.1371/journal.pone.0015414
33. Ofori JK, Karagiannopoulos A, Barghouth M, Nagao M, Andersson ME, Salunkhe VA, et al. The highly expressed calcium-insensitive synaptotagmin-11 and synaptotagmin-13 modulate insulin secretion. Acta Physiol (Oxf) (2022) 236(1):e13857. doi: 10.1111/apha.13857
34. Revel FG, Herwig A, Garidou ML, Dardente H, Menet JS, Masson-Pévet M, et al. The circadian clock stops ticking during deep hibernation in the European hamster. Proc Natl Acad Sci United States America (2007) 104(34):13816–20. doi: 10.1073/pnas.0704699104
35. Pozzilli P. The dpt-1 trial: A negative result with lessons for future type 1 diabetes prevention. Diabetes/Metabol Res Rev (2002) 18(4):257–9. doi: 10.1002/dmrr.316
36. Marcheva B, Ramsey KM, Buhr ED, Kobayashi Y, Su H, Ko CH, et al. Disruption of the clock components clock and Bmal1 leads to hypoinsulinaemia and diabetes. Nature (2010) 466(7306):627–31. doi: 10.1038/nature09253
37. Chase HP, Cuthbertson DD, Dolan LM, Kaufman F, Krischer JP, Schatz DA, et al. First-phase insulin release during the intravenous glucose tolerance test as a risk factor for type 1 diabetes. J Pediatr (2001) 138(2):244–9. doi: 10.1067/mpd.2001.111274
38. Pørksen N. Early changes in beta-cell function and insulin pulsatility as predictors for type 2 diabetes. Diabetes Nutr Metab (2002) 15(6 Suppl):9–14.
39. Folli F, Okada T, Perego C, Gunton J, Liew CW, Akiyama M, et al. Altered insulin receptor signalling and β-cell cycle dynamics in type 2 diabetes mellitus. PloS One (2011) 6(11):e28050. doi: 10.1371/journal.pone.0028050
40. Kulkarni RN, Brüning JC, Winnay JN, Postic C, Magnuson MA, Kahn CR. Tissue-specific knockout of the insulin receptor in pancreatic beta cells creates an insulin secretory defect similar to that in type 2 diabetes. Cell (1999) 96(3):329–39. doi: 10.1016/s0092-8674(00)80546-2
41. Skovsø S, Panzhinskiy E, Kolic J, Cen HH, Dionne DA, Dai XQ, et al. Beta-cell specific insr deletion promotes insulin hypersecretion and improves glucose tolerance prior to global insulin resistance. Nat Commun (2022) 13(1):735. doi: 10.1038/s41467-022-28039-8
42. Antvorskov JC, Aunsholt L, Buschard K, Gamborg M, Kristensen K, Johannesen J, et al. Childhood body mass index in relation to subsequent risk of type 1 diabetes-a Danish cohort study. Pediatr Diabetes (2018) 19(2):265–70. doi: 10.1111/pedi.12568
43. Zucker I, Zloof Y, Bardugo A, Tsur AM, Lutski M, Cohen Y, et al. Obesity in late adolescence and incident type 1 diabetes in young adulthood. Diabetologia (2022). doi: 10.1007/s00125-022-05722-5
44. Xu P, Cuthbertson D, Greenbaum C, Palmer JP, Krischer JP. Role of insulin resistance in predicting progression to type 1 diabetes. Diabetes Care (2007) 30(9):2314–20. doi: 10.2337/dc06-2389
45. Buteau J. Glp-1 receptor signaling: Effects on pancreatic beta-cell proliferation and survival. Diabetes Metab (2008) 34 Suppl 2:S73–7. doi: 10.1016/s1262-3636(08)73398-6
Keywords: type 1 diabetes, type 2 diabetes, pathogenesis, beta cells, GLP-1, metformin
Citation: Josefsen K, Krogvold L, Gerling IC, Pociot F, Dahl-Jørgensen K and Buschard K (2022) Development of Type 1 Diabetes may occur through a Type 2 Diabetes mechanism. Front. Endocrinol. 13:1032822. doi: 10.3389/fendo.2022.1032822
Received: 31 August 2022; Accepted: 01 December 2022;
Published: 14 December 2022.
Edited by:
David Funda, Institute of Microbiology, Academy of Sciences of the Czech Republic (ASCR), CzechiaReviewed by:
Volker Burkart, German Diabetes Center (DDZ), GermanyJacob Petersen, Novo Nordisk (Denmark), Denmark
Marie-louise Hartoft-Nielsen, Novo Nordisk (Denmark), Denmark
Copyright © 2022 Josefsen, Krogvold, Gerling, Pociot, Dahl-Jørgensen and Buschard. This is an open-access article distributed under the terms of the Creative Commons Attribution License (CC BY). The use, distribution or reproduction in other forums is permitted, provided the original author(s) and the copyright owner(s) are credited and that the original publication in this journal is cited, in accordance with accepted academic practice. No use, distribution or reproduction is permitted which does not comply with these terms.
*Correspondence: Karsten Buschard, buschard@dadlnet.dk