- 1Center of Geriatrics, Hainan General Hospital (Hainan Affiliated Hospital of Hainan Medical University), Haikou, China
- 2Clinical College, Hainan Medical University, Haikou, China
Ischemia with non-obstructive coronary artery (INOCA) is a blind spot of coronary artery disease (CAD). Such patients are often reassured but offered no specific care, that lead to a heightened risk of adverse cerebrovascular disease (CVD) outcomes. Epicardial adipose tissue (EAT) is proven to correlate independently with CAD and its severity, but it is unknown whether EAT is a specific and sensitive indicator of INOCA. This review focuses on the INOCA epidemiology and related factors, as well as the association between EAT.
1 Background
Ischemia with non-obstructive coronary artery (INOCA) has become the focus of global attention. In tradition myocardial ischemia is caused by coronary atherosclerosis-induced obstructive lesions. However, many patients exhibit chest pain and ischemia without significant vascular disease (≥50%) (1). Such patients are often neglected, resulting in frequent hospitalization and adverse clinical outcome (2–6). The mechanisms of INOCA are multifactorial, including coronary microvascular dysfunction (CMD), coronary vascular spasm, endothelial dysfunction, myocardial bridge, both alone or co-exist (7). CMD may be the main reason, but it is difficult to detect sensitivity (3–6). Epicardial adipose tissue (EAT) occupies a special place within the heart. During the progression of atherosclerosis (AS), the paracrine function of EAT changes from a nutritional source to lesions with dysfunction, inflammation, and fibrosis (8). We suspect that the thickness or density of EAT could become a new diagnostic criterion for INOCA. Extensive studies have confirmed that EAT is not only independently associated with the severity of CAD but also with the plaque calcification (9). However, others have some inconsistent and women with CAD are susceptible (5, 10, 11). This illustrates whether these differences are early features of CAD, while EAT is a sensitive predictor for INOCA. This review focuses on INOCA epidemiology and related factors, as well as its association between EAT.
2 Ischemia with Non-obstructive Coronary Artery (INOCA)
INOCA is characterized by clinical evidence of myocardial ischemia with either normal or less than 50% stenotic coronary arteries on angiography. Causes of INOCA include CMD, coronary vascular spasm, endothelial dysfunction, where CMD is the main (50%). Traditionally invasive and noninvasive CMD detection have corresponding blind spots: blockage under invasive coronary angiography may have no dysfunction, and FFR <0.8 may be overrated.
2.1 INOCA and CMD
Balaji T. found that approximately half of the women with INOCA had CMD (age 57 ± 11; MPRI <1.84) (2). CMD is a disease with injured epicardium endothelium, limiting perfusion of the myocardium. It is characterized by impaired coronary flow reserve (CFR), which may be due to obstructive or nonobstructive CAD and myocardial infarction. Usually, CMD has a relatively long asymptomatic period, but 30-60% can both incite angina (6). As reflected in CFR, there is little correlation between anatomical severity and functional impairment. Thus, CMD should be recognized as the main cause of INOCA.
Among the sites controlling blood flow resistance and perfusion is the coronary artery microcirculation (diameter<300 mm), including arterioles and small veins. A major part of circulation is the delivery of oxygen and nutrients to tissues, the removal of waste and the regulation of inflammation and repair. Because of the adaptation of their morphology and function, R Pries proposed the model of “vascular adaptation” to describe microvascular network adaptation in meeting tissue requirements in 1998. The adaptation of the vascular cavity to the circulatory system ensures that the vascular cavity and all tissues are equally perfused and thus ensures adequate perfusion reserves and good perfusion at rest. Nevertheless, microcirculation is poorly adapted, resulting in insufficient blood flow distribution and degradation of microcirculation.
In clinical, myocardial ischemia mainly caused by damage of the epicardial coronary artery and downstream microvascular network (4). Total perfusion in the larger myocardial area is controlled by the epicardial artery. Due to the flow limitation, there will be a mismatch between myocardial supply and demand, thereby increasing the arteriovenous oxygen difference (AVD-O2). Within the terminal vascular network, microcirculation controls blood flow distribution. Microvascular dysfunction is usually associated with a functional shunt, hypoxia, and an average AVD-O2 reduction (12). Additionally, diffuse nonobstructive coronary AS is often underestimated since uneven distribution of microvessel results in less sensitivity of clinical imaging methods to detect heterogeneity (4). According to studies, patients with limited nonobstructive diseases have a worse prognosis than those with normal coronary arteries but better prognosis than those with extensive nonobstructive diseases. In patients with extensive nonobstructive disease, risk equals those with single-vessel obstructive CAD (13). The breakthrough for detecting INOCA early may be the sensitive detection of diffuse nonobstructive coronary AS. The clinical diagnostic assessment of INOCA is as followed (Figure1).
2.2 Diagnostic assessment of INOCA
Electrocardiograms can be used to detect myocardial ischemia in the diagnosis of INOCA. Cardiac magnetic resonance (CMR) and pressure echocardiography (left anterior descending arterial flow imaging) detected abnormal wall movement. Additionally, myocardial contrast ultrasound, single-photon emission CT (SPECT), and positron emission tomography (PET) can detect changes in perfusion (Table 1). Ischemia is usually caused by exercise or drugs that increase myocardial oxygen demand or by imbalanced perfusion of the heart due to vasodilation. In clinical, adenosine injections were used to measure the ratio between distal coronary artery stenosis and aortic root pressure. A flow reserve fraction (FFR) less than 0.8 indicates myocardial ischemia. In INOCA patients, customized treatment guided by the results of intracoronary trials [CFR, index of microcirculatory resistance (IMR), and acetylcholine trials] (IMR ≥ 25 units or CFR<2.32 suggests microcirculation abnormalities, is the best predictor) can significantly reduce angina symptoms (5). With the use of vasodilators such as adenosine, CFR and IMR are commonly measured. For testing the arterioles, however, acetylcholine must be injected into the coronary arteries to ascertain endothelial function. During reactive hyperemia, systemic endothelial dysfunction can be observed in INOCA patients (1).
An intravenous injection of contrast agent was used to evaluate the coronary artery lumen wall by coronary artery CTA. Since both tests are anatomically based, it provides a high degree of accuracy for detecting coronary artery stenosis. A stenosis of 50-90% is not necessarily diagnostic, as it does not always cause myocardial ischemia (14, 21). Coronary computed tomography angiography (CCTA) is recommended as a primary or secondary diagnosis for patients at moderate risk of CAD, which due to its sensitivity and specificity (15). CCTA can be used as a pre-diagnostic tool to detect early stages of AS in healthy or mildly symptomatic patients. The 2021 ESC Guide noted that CCTA is used to exclude CAD. For clinically ST-segment elevation angina, invasive coronary angiography (ICA) will continue to be the best option. In contrast, in patients with clinically highly susceptible unstable angina, the use of imaging or coronary CCTA would be the best option (22). The 2019 ESC Guide noted that in patients with extensive CAD, CT-based FFR is not inferior to ICA and FFR in deciding and identifying vascular reconstruction targets (1). A 26-monthes follow-up study showed that CCTA is more discriminating than functional tests in predicting major adverse cardiovascular events (MACE) (23). For CCTA or invasive angiography stenosis, further evaluation using noninvasive or invasive functional tests may be necessary before extremely severe stenosis (diameter stenosis >90%) detected (15).
3 EAT and INOCA
3.1 EAT and CMD
EAT has a microcirculation that is share with the heart. Its unique properties include increased fatty acid metabolism and transcripts, as well as genes associated with inflammation and endothelial function. In physiological conditions, EAT have metabolic, thermal production (similar to brown fat), and mechanical (heart protection) properties, where mitochondrial brown fat uncoupling protein-1 (UCP-1)-positive small single atrial fat cells have been described in epicardial fat (24). The metabolic of EAT has been confirmed, while brown adipose tissue (BAT)-specific genes, such as UCP-1 and PRD1-BF1-RIZ1 homologous domain containing 16 (PRDM-16), were also found at high levels of expression in human EAT (8). The histological characteristics of EAT also resemble those of brown and beige adipocytes. Studies have shown that EAT is rich in saturated fatty acids with high protein content and has the strongest release and absorption capacity of free fatty acids (FFAs) compared to other visceral fat libraries (24, 25); this may be due to EAT containing mesenchymal cell-derived myocardial stem cells (9, 26). Physiological conditions enable FFAs to spread bidirectionally from epicardial fat to the myocardium along the concentration gradient, and the vascular active cytokines secreted by EAT allow FFAs to enter the coronary artery and influence coronary tension. Epicardial fat acts as a buffer to prevent excessive exposure to FFAs in the heart muscle. The oxidation of FFAs in the heart provides most of their energy. Therefore, metabolic disorders and FFA enrichment interact with the heart muscle.
CAD patients tend to have more brown fat than white fat. This is demonstrated by a significant decrease in the expression of brown fat-like genes (peroxidase proliferator activated receptor γ coactivator Factor 1α (PGC-1α), UCP-1 and PRDM-16 and by increased expression of white fat-like genes (insulin-like growth factor binding protein (IGFBP) 3 and homologous frame (HOX) C8 and 9) (8). In addition to increased reactive oxygen content and proinflammatory gene expression in EAT, this process also reduced plasma fatty acid clearance. Thus, EAT whitening and subsequent localized dysfunction may trigger vascular inflammation and coronary AS. But the mechanism of EAT white differentiation and produces inflammation remains unclear. Additionally, hypoxia and abnormal changes interact with EAT cytokines (such as TNF, IL-6 and FFAs) to alter atrial tissue conductance, accelerate fat decomposition, and play a role in AS at different stages. Vascular tension and remodeling, smooth muscle cell proliferation and migration, oxidative stress, congenital inflammatory response, and plaque instability are among them (8). In the early stages of these diseases, EAT acts as promoters of AS. This suggests that EAT might have a greater effect earlier than in the later stages of the disease. Many experts believe that thickened epicardial fat will cause physical obstruction of diastolic filling, which will lead to atrial enlargement, impaired ventricular diastolic filling, and ischemia (24). This may be a link between structure and dysfunction in the later stages of the disease.
EAT contributes to AS, and focal obstructive lesions occur in the coronary segments where there are the most dysfunctional electrophysiological properties adjacent to areas of thick epicardial fat. This inflammation can affect the structure and function of adjacent tissues through paracrine (27). In the Tomasz Mazurek et al. study, increased local inflammation in patients with severe CAD did not correlate with plasma circulating cytokine concentrations. It confirmed that bioactive molecules in peri coronary tissue may alter arterial homeostasis and the inflammatory cells in adipose tissue is only indicative of an approaching plaque rupture (28). This can only account for the interaction of CAD with inflammatory changes in EAT, but not for the sequence between them.
3.2 Mechanism of EAT causes INOCA
At present, the mechanisms of INOCA are mostly believed to be endothelial injury and oxidative stress (Figures 2, 3). Several studies have confirmed that INOCA occurs due to decreased microvascular density, mesenchymal fibrosis, contraction of smooth muscle cells, and deposition of subcutaneous plaque lipids. The local vascular environment is changed by EAT during inflammation, as it activates adipokines and proinflammatory factors. The relationship between EAT and CAD has been well established, but no studies have expressed the relationship between EAT and INOCA. Several mechanisms by which the inflammatory state of EAT may lead to INOCA are discussed below.
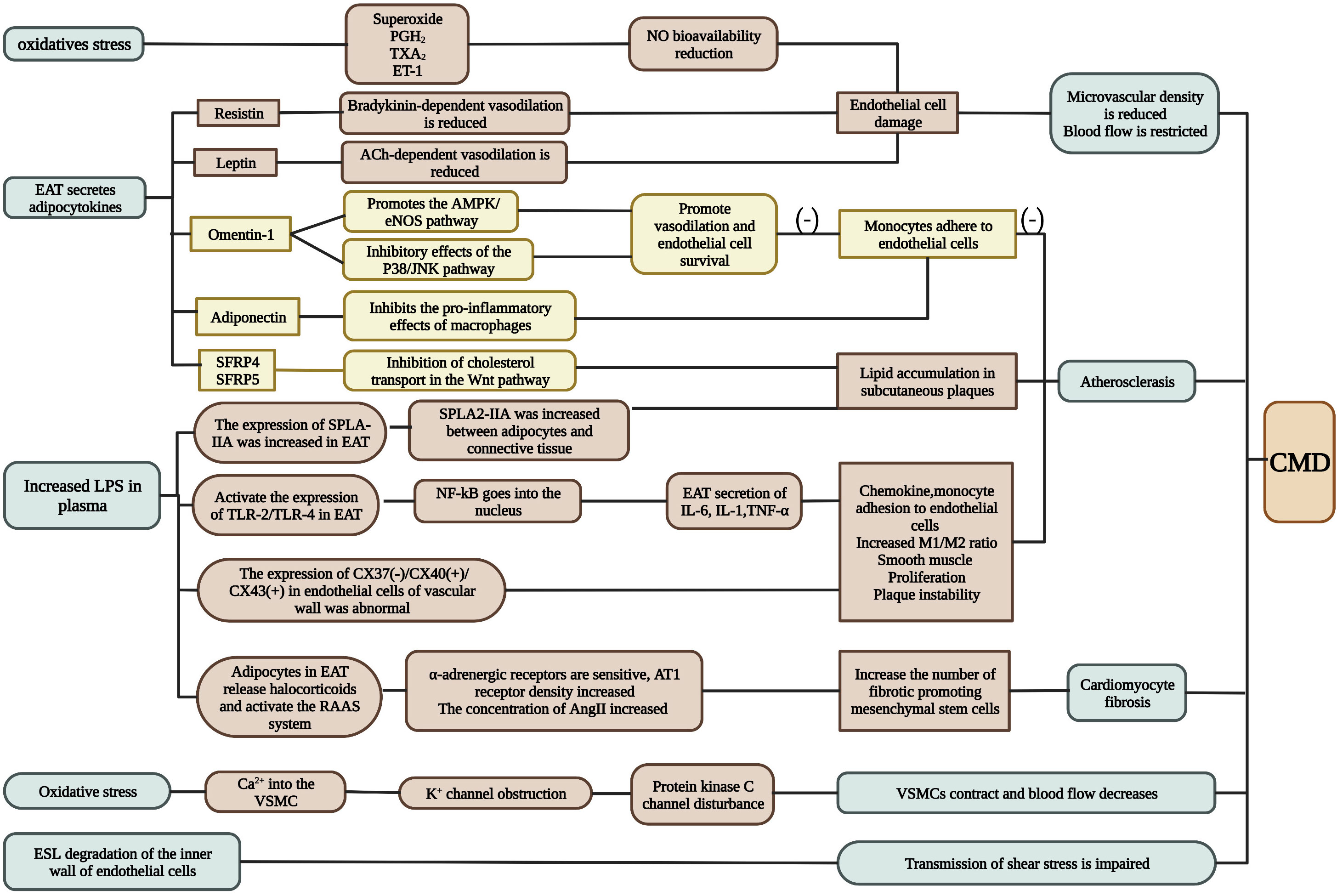
Figure 2 The mechanism of INOCA. INOCA is represented by CMD. It can be caused by oxidative stress, adipokines produced by EAT, changes in circulating factor concentrations in plasma, and changes in ESL. CMD is determined by a combination of factors including endothelial dysfunction, vascular smooth muscle cell hyperreactivity, vascular remodeling, vascular fibrosis, and changes in macrophage function (see text for details). A possible adjustment procedure is proposed for this. CMD: coronary microvascular dysfunction.*Green represents several conditions that produce CMD under abnormal EAT; brown represents pathways that promote inflammation; yellow represents pathways that inhibit inflammation. (Created with BioRender.com).
3.2.1 Inflammation
CAD is a disease characterized by low-grade, chronic inflammation of the arterial wall. The early characteristics of the coronary microvascular response to inflammation are oxidative stress, reduced nitric oxide bioavailability, and endothelial activation. C-reactive protein is increased among subjects with microvascular angina compared with control subjects, further supporting a possible role of inflammation and endothelial dysfunction in causing CMD. CFR is reduced among patients with normal or minimally diseased coronary arteries and either systemic lupus erythematosus or rheumatoid arthritis, and prolonged systemic inflammation may also contribute to premature CAD in these patients (29).
3.2.2 Endothelial function damage
When the endothelium is under stress, it may produce substances known as endothelin, thromboxane A2, prostaglandin H2 (30–33), and superoxide that restrict arterial blood flow. Net expansionary responses to various stimuli shift into contractile responses. Endothelin 1 (ET-1) is a potent vasoconstrictor of human coronary arteries. In microvascular angina (MVA)/vascular angina (VSA), Thomas J. et al. (31) used the ACh/adenosine thermodilution technique to confirm widespread endothelial damage. MVA and VSA were generally susceptible to ET-1. So, they (30) conducted another experiment in which the correlation of ET-1-related genes was investigated for 391 individuals with stable angina. A rs9349379-G allele of the ET-1 gene was independently associated with cardiomyopathy. In the population with G alleles, the likelihood of having high levels of ET-1 and myocardial dysfunction was more than twice (impaired myocardial perfusion, and impaired exercise endurance). As a result, inflammation may cause EAT to release ET-1 in CMD, thereby reducing NO bioavailability. Endothelial cell migrated, angiogenesis impaired, and angiogenesis and remodeling are unbalance, meaning that microvessel density decreases. It is unclear whether these changes are the results or the cause of CMD, so we assume they are reciprocal.
Indirectly or directly, EAT can also affect endothelial cells. Low-density lipoproteins (LDLs) are retained in the subcutaneous space by the enzyme secretory type II phospholipase A2 (SPLA2-IIA). In human atherosclerotic lesions, SPLA2-IIA expression was higher in epicardial fat (34). Expression of the human SPLA2-IIA gene in mouse models increased sensitivity to AS (35). EAT may cause lipogenesis by secreting SPLA2-IIA by its lipids into the stroma, resulting in conjugated fatty acids.
A transition from a resting to an active state is also involved in endothelial dysfunction. Several studies have shown that phenotypic transformation of macrophages can also cause endothelial dysfunction (25). Yoichiro Hirata’s team (36) confirmed that the level of proinflammatory cytokines in EAT of CAD patients negatively correlated with the level of anti-inflammatory cytokines. As the M1/M2 macrophage count increased with the Gensini score, it was evident that the M1/M2 macrophage count increased with CAD severity. A. R. Baker et al. (37) found that whole body lipopolysaccharide levels increased among patients with CAD. Additionally, TLR-2 and TLR-4 expression levels (markers of macrophage activation) were higher in EATs of CAD patients. Lipopolysaccharides trigger inflammation by activating TLRs (24) (Figure 3).
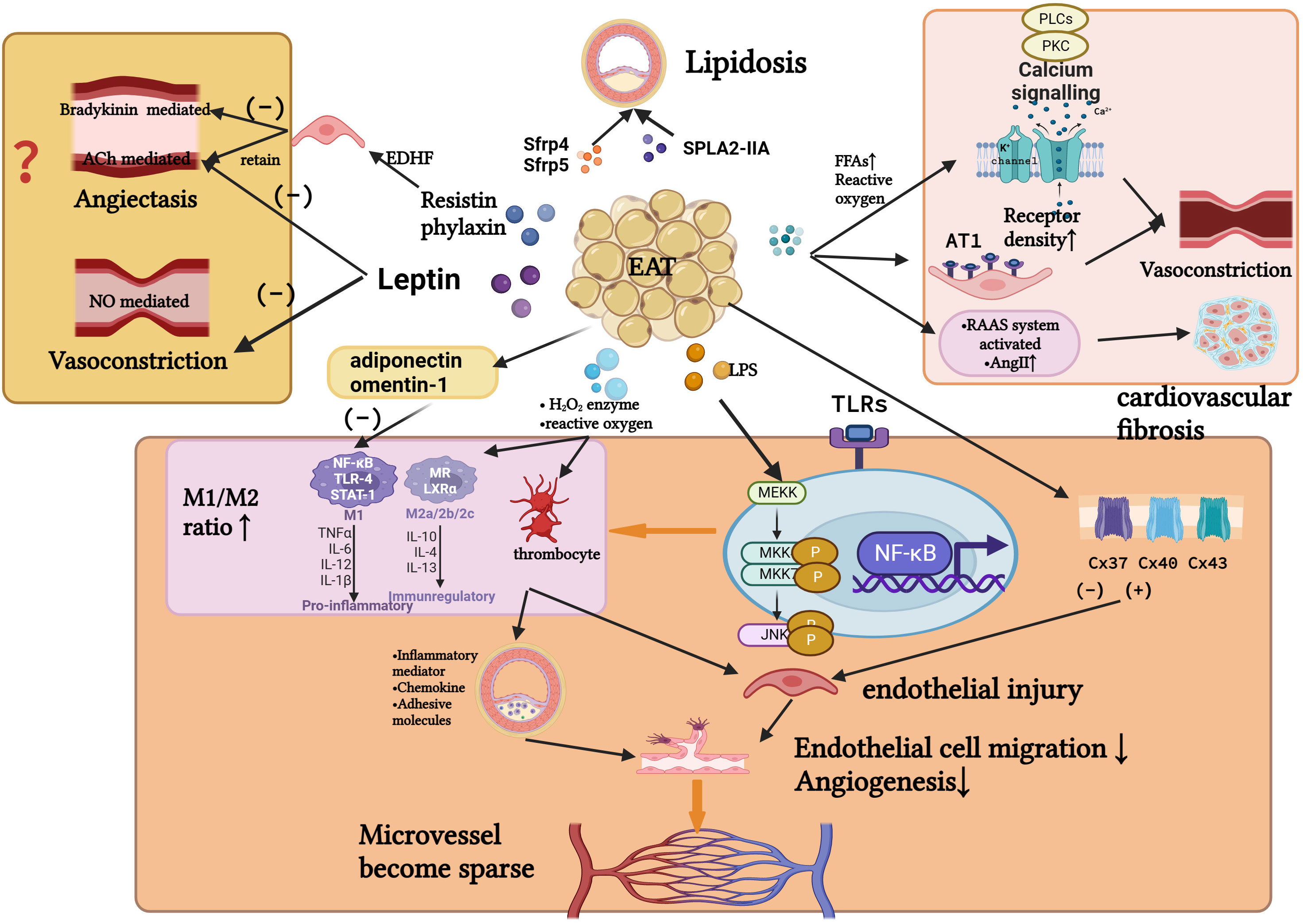
Figure 3 Several possible relationships between inflammatory EAT and the pathogenesis of INOCA. Inflammation causes EAT to secrete cytokines and adipokines, altering the function of the vascular endothelium and the phenotype of macrophages. In turn, it affects the function of vascular smooth muscle, the remodeling of microvascular density, deposition of subcutaneous plaque lipids, and the mesenchymal fibrosis. These may affect perfusion of myocardial blood flow, leading to the development of INOCA. (Created with BioRender.com).
Additionally, connexins (a family of proteins), semi-channels and gap junction channels, have been shown to play a role in atherosclerosis (38). Nonetheless, in Cx40del mice, endothelial cells on the surface of advanced atherosclerotic lesions did not express CX40. Near lesions, endothelial cells not affected by lesions exhibited large amounts of Cx40 protein. It is suggested that Cx40 may be a marker of early endothelial injury.
3.2.3 Adipokines regulation
EAT can release adipokines to regulate the progression of AS. Various studies have found (33) that resistin does not affect coronary blood flow, although it damages endothelial function. It is believed that resistin delays bradykinin-mediated vasodilation but permits ACh-mediated vasodilation. ACh-mediated vasodilation is decreased by leptin. Additionally, leptin inhibits contractile responses induced by AngII on aortic vascular smooth muscle cells (VSMCs) (9, 24). Intriguingly, EAT also expresses AngII and leptin. It is unclear whether EAT can affect the coronary endothelium via these factors, but it deserves further investigation.
The M1/M2 ratio of CAD patients was negatively correlated with the expression of adiponectin in EAT, but this correlation was not statistically significant (36). G. Iacobellis et al. (25) found that adiponectin levels near left coronary artery (LCA) were associated with the expression in EAT of CAD patients, whereas have no relationship with serum adiponectin levels. Yu Du et al. showed that adiponectin in the CAD group was lower than in the non-CAD group (39). Both stenosis and non-stenosis the difference was statistically significant in the CAD group. They also detected another adipokine of omentin-1. EAT omentin-1 level was markedly decreased in CAD patients compared with NCAD patients. In CAD patients with and without stenosis, omentin-1 ratios were 0.01:0.14 (P=0.0127) (39).
Wnt pathway-related proteins are highly expressed in AS lesions. It plays a major role in AS by transporting cholesterol and regulating inflammation. In the single-factor analysis of Q. Ji, Secreted frizzled-related protein 4 (Sfrp4) in EAT of CAD and non-CAD cardiac surgery subjects was a significant difference. Sfrp4 mRNA levels in EAT correlated with CAD, a multivariable linear regression model found that the association was independent of fasting glucose, age, and BMI (40). Sfrp5, which competitively binds with wingless-type family member 5a (Wnt5a), controls chronic inflammation, inhibits atherosclerosis, and represses metabolic conditions (41, 42). In our previous study, Wnt5a mRNA expression in EAT was found to correlate with the presence of CAD. Also, Sfrp5 mRNA and protein levels in EAT of CAD patients were shown to be reduced for the first time. And these inverse correlations were further confirmed after adjustment for other conventional risk factors (41). It means low Sfrp5 and high Wnt5a levels were associated with Chinese CAD patients.
3.2.4 Neurohumoral regulation
Endothelial cells and the autonomic nervous system interact closely. Activation of β-adrenergic receptors in vascular smooth muscle cells causes vasodilation, activation of α-adrenergic receptors causes vasoconstriction, and activation of toxicological receptors causes vasoconstriction. Studies have confirmed that chronic metabolic disorders activate the RAAS system (33). The flow of AngII, combined with the AT1 receptors, significantly increase vasoconstriction. The sensitivity of alpha-adrenergic receptors also affects the coronary blood flow.
Ana M et al. found a correlation between EAT inflammatory, increased aldosterone and impaired vasodilation in obese people (43). An adrenal gland and adipose tissues over-synthesis corticosteroid signals, revealing a proinflammatory state in adipose tissue.
A study has shown that hypoxia causes microcirculation vessels to weaken to vasodilators, and this is due to cyclooxygenase (44). It was also observed that in the high-fat model, the impairment of upstream KATP channels was associated with impaired vasodilator response to acidosis (33). Inhibition of coronary arteriolar vasoconstriction by protein kinase C (via the KATP channel). Oxidative stress decreased the coronary response to ischemia-induced vasodilators. Therefore, changes in cardiac perfusion will likely occur after the development of oxidative stress and endothelial dysfunction.
3.2.5 Emerging theory
Among endothelial cells, there is a membrane-bound layer of macromolecules (polysaccharide calyx) and another relatively thick layer of plasma components at the lumen surface, called the endothelial surface layer (ESL) (45). Accordingly, it hypothesized that CMD might be associated with lower arterial-mediated vasodilation of NO (4).The ESL regulates blood flow by transmitting shear stress (the transmission of shear force). ESL is very unstable and easily degraded by oxygen free radicals, ischemia, inflammation, or altered plasma composition.
4 EAT and the traditional risk factors affecting INOCA
4.1 EAT and metabolic disorders
EAT thickness is associated with metabolic syndrome in a significant and independent way, according to several clinical studies, it is independently on other cardiometabolic risk factors, such as insulin resistance, fasting glucose, CRP, liver enzymes, and carotid intima-media thickness (3, 24). On the other hand, some studies showing EAT were not correlated with traditional factors or AS (Table 2). Patients with CAD (48) had low mRNA levels of glucose transporter-4 (GLUT4) and abnormal levels of retinol binding protein 4 (RBP4). Cells in EAT release higher levels of RBP4 than subcutaneous fat cells. Thus, it is speculated that EAT may lead to locally adverse lipid and glucose spectra and insulin resistance. Studies have shown that (25) EAT is associated with an increase in serum transaminase levels and the incidence of hepatic steatosis in obese individuals. However, these changes are not related to obesity but to excessive visceral fat levels in these individuals. Due to increased FFA production and intrinsic insulin resistance, we conclude that epicardial and intrahepatic fat are directly linked. Nevertheless, Antonopoulos AS demonstrated that perivascular adipocyte lipid accumulation was positively correlated with atherosclerotic plaque load but not with systemic insulin resistance (49). It appears that perivascular adipocyte lipid accumulation is caused by local inflammation rather than systemic metabolic disorders (such as insulin resistance). A study by M. Shimabukuro et al. discovered that EAT volume is associated with coronary artery calcification independent of CAD (50). Interestingly, inflammatory cells in adipose tissue reflect only plaque rupture, in a similar manner to inflammatory infiltration of the outer membrane and surrounding blood vessels in advanced AS. Additionally, dipeptidyl peptidase-4 inhibitors have been shown to decrease EAT accumulation (27). However, it did not affect the anti-inflammatory properties or secretion of proinflammatory factors in EAT. In this case, insulin resistance is not the initiator, but the promoter of inflammation caused by EAT. Furthermore, adipose changes occurred before insulin resistance and CAD.
In patients with advanced CAD, there was no strong correlation between inflammatory signals from EAT and plasma inflammatory biomarkers (28). Similarly, circulating inflammatory biomarkers were not significantly associated with EAT concentrations. There was no significant relationship between inflammation caused by EAT in CAD patients and systemic inflammation (obesity, diabetes). It may therefore appropriate to speculate that the local inflammation caused by EAT is not associated with traditional risk factors.
4.2 Gender differences
Even though the prevalence of heart disease is higher in men than in women, the overall in-hospital mortality rate is higher than in men. This may be due to the atypical symptoms of heart attack in women (chest discomfort, weakness, dizziness) (51). Women in INOCA are approximately four times more likely than men to be readmitted to the hospital with ACS/chest pain within 180 days (3). It shows that gender difference is a unique characteristic of INOCA, women for its susceptible population. Several studies have found that women with CMD have higher myocardial triglyceride content, which may translate into ectopic fatty acid deposition in cardiomyocytes, resulting in myocardial mechanical damage in the diastolic period (3). Estrogen might control in vivo vasodilation by inducing cardiomyocyte hyperpolarization, altering ATP-sensitive potassium channel dynamics in myocytes, and inhibiting Ca2+ and ET-1 controlled contractions of cardiomyocytes. At the same time, estrogen can induce cardiomyocyte-mediated endothelin-independent vasodilation by stimulating prostacyclin production (52). Since adipocytes, immune cells, and mitochondria express estrogen receptors specifically, and free fatty acid metabolism in women is more complex than in men. Estrogen receptors are expressed on the surface of immune cells and modulate immune activity. β-Estradiol inhibits immune activity by inhibiting the differentiation of proinflammatory helper T cells and M1 macrophages. Estrogen is also a determinant of adipose tissue metabolism and function. In a mouse model (53), estrogen has been shown to prevent impaired glucose tolerance and obesity by regulating adipogenesis and decomposition. Estrogen can also increase the expression of this gene through estrogen receptor-α in the mitochondria of brown adipose tissue and affect mitochondrial production (3, 54). Studies have shown (8) that visceral adipose tissue (VAT) includes less insulin-sensitive tissue, and inflammatory cytokines and immune cells in the infiltrating blood vessels express more glucocorticoids and androgen receptors. VAT has been proven to be more sensitive than subcutaneous adipose tissue (32), and male fat is easily deposited in VAT due to its unique composition of androgens. FFAs is first deposited in female cardiomyocytes by the expression of estrogen receptors. Studies have shown that myocardial dysfunction is independently associated with metabolic syndrome, and men are at greater risk than women (54). In INOCA, however, females are more prevalent than males and EAT thickness in women was associated with more risk factors (32, 55, 56). Therefore, is it possible to speculate that obstructive CAD occurs after INOCA? Are women more inclined to have INOCA due to diffuse lipid deposition compared to men who are prone to focal obstruction? Further researches are needed.
5 EAT detection
Researchers have confirmed that the quantity and quality of EAT are both associated with AS in CAD patients by different ways (Figure 4; Table 3). Its thickness, a noninvasive echocardiographic assessment, proved to be the best independent predictor of carotid IMT (8). 5.0 mm thick EAT in diastolic identified a higher likelihood of vascular disease in the presence of carotid plaque (57). EAT was positively associated with coronary artery calcium (CAC) in the study, even after adjusting for other CAD risk factors and BMI (58). EAT is also closely associated with the presence of “soft” or “mixed” vulnerable plaques and is associated with negative outcomes (59). Moreover, there was a weak correlation between EAT and the Framingham risk score (57) and EAT thickness (>3.0 mm) was an independent predictor of CAD. In the same cohort, a 4.5 mm definition of EAT thickness identified five elements of metabolic syndrome, with 9.5 mm and 7.5 mm predicting metabolic syndrome in men and women, respectively. EAT thickness of 8.0 mm and 7.1 mm (men and women) indicate abnormal fasting glucose (60). A higher value is more predictive of heart disease after systemic metabolic disorders. However, in a study of middle-aged subjects with suspected metabolic syndrome (54), the thickness of EAT was associated with hs-CRP level, left ventricular mass, and subclinical myocardial dysfunction only in men. This suggests that the inflammatory activity of EAT induces myocardial remodeling and dysfunction in middle-aged subjects but is attenuated in female subjects. Therefore, it is speculated that the thickness of EAT may be a marker of the early process of cardiac remodeling and dysfunction in these patients.
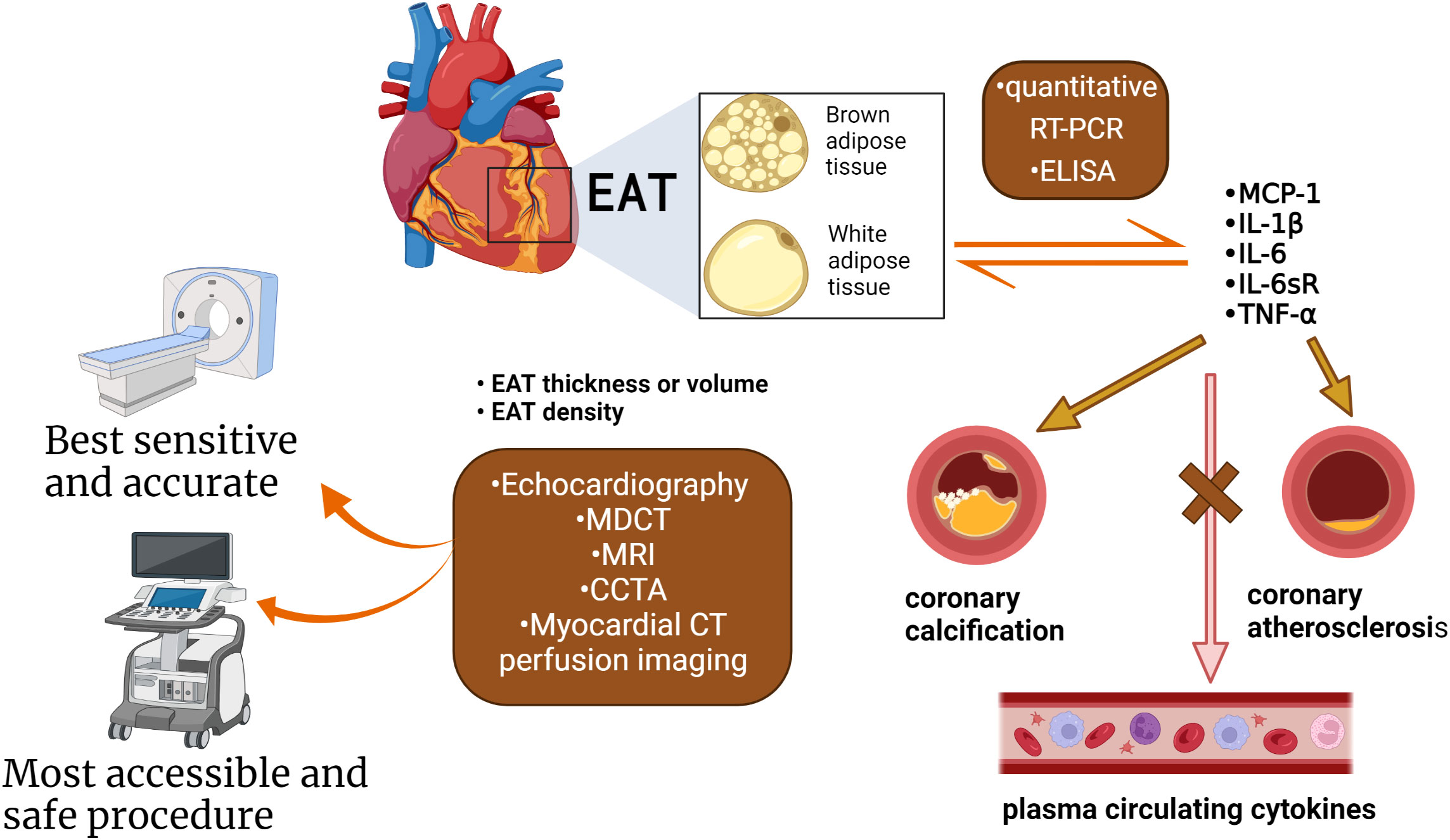
Figure 4 The methods used to measure the quantity and quality of EAT. Quantification of EAT has seen improvements over the years attributed to the advancements in non-invasive imaging techniques: CT, as well as CMR, PET. Evaluation indicators as thickness, volume, and density. EAT under chronic inflammation shifts from brown fat to white fat. The quality of the EAT was changed, such as inflammatory status, the type of adipokines, and microRNA. The cytokines and adipokines within the EAT can be measured by quantitative RT-PCR and ELISA. Cytokines correlate with the presence of CAD, severity, and coronary artery calcification, but not with circulating cytokines in plasma. EAT inflammation was independent of several clinical variables (obesity, diabetes, or chronic therapy with statins or ACE inhibitors). (Created with BioRender.com).
EAT density detected by echocardiography, magnetic resonance imaging (MRI) and PET/CT may be used as a biomarker for early coronary atherosclerosis, as it is an explanation for EAT composition and its effect on CAD (8, 61). The radioactive density of adipose tissue detected by CT is associated with differences in adipose tissue composition, which may promote the development of coronary atherosclerosis independent of the volume of EAT. The relationship between EAT attenuation and CAC distribution and density was not affected by gender (61). In men with severe CAD, lower CT attenuation at EAT was associated with higher CAC (14), independent of EAT volume and BMI. Interestingly, the attenuation of EAT was independent of the distribution range of calcium in the four major coronary arteries but related to the calcium density of each plaque (61). This may indicate that EAT may not be an instigator of CAC development but merely a catalyst for the rupture of stable atherosclerotic plaques.
6 Conclusions and outlook
INOCA has now entered the public eye for its undervalued cardiogenic deaths. Its microcirculation system is like the coronary artery, which is also a research hotspot. In recent studies, it has been found that the EAT calcification fraction is independently correlated with EAT thickness. However, some studies have confirmed that EAT is not correlated with traditional risk factors. As CAD deterioration, inflammation of the coronary system and microcirculation are isolated from systemic inflammatory changes. The high sensitivity of EAT relative to subcutaneous fat makes it possible to detect early dysfunction of the coronary artery and microcirculation system. In conclusion, maybe EAT could be a goalkeeper for INOCA, and more reliable clinical research and basic experiment are needed.
Author contributions
TS contributed to the conception of the review. ZS wrote the first draft of the manuscript. WH wrote some sections of the manuscript. ZM and TS wrote and modified the review.Both authors have reviewed the final version of this manuscript before submitting it to topic editors of the journal.
Acknowledgments
This work was Supported by National Natural Science Foundation of China (Regional Program) (82060087), Natural Science Foundation of Hainan Province of China (Young Scholars program) (820QN384), Hainan Clinical Medical Research Center Project (LCYX202207), Hainan Provincial Health Industry Research Project (20A200396), and Innovation and Entrepreneurship Project for College Students (Correlation between Elderly Non-Obstructive Coronary Artery and Epicardial adipose tissue Thickness).
Conflict of interest
The authors declare that the research was conducted in the absence of any commercial or financial relationships that could be construed as a potential conflict of interest.
Publisher’s note
All claims expressed in this article are solely those of the authors and do not necessarily represent those of their affiliated organizations, or those of the publisher, the editors and the reviewers. Any product that may be evaluated in this article, or claim that may be made by its manufacturer, is not guaranteed or endorsed by the publisher.
Abbreviations
AS, Atherosclerosis; Ang II, Angiotensin II; BAT, Brown adipose tissue; CCTA, Coronary computed tomography angiography; CT, Computed tomography; CAD, Coronary artery disease; CVD, Cerebrovascular disease; CMD, Coronary microvascular dysfunction; CFR, Coronary flow reserve; ESL, Endothelial surface layer; EAT, Epicardial adipose tissue; ET-1, Endothelin 1; FFR, Flow reserve fraction; FFAs, Free fatty acids; GLUT4, Glucose transporter – 4; INOCA, Ischemia with non-obstructive coronary artery; IMR, Index of microcirculatory resistance; ICA, Invasive coronary angiography; LDLs, Low-density lipoproteins; LCA, Left coronary artery; MACE, Major adverse cardiovascular events; MVA, Microvascular angina; PET, Positron emission tomography; SPECT, Single-photon emission CT; Sfrp4, Secreted frizzled-related protein 4; Uncoupling protein - 1; UCP-1, VSA, Vascular angina; VSMCs, Vascular smooth muscle cells; Wnt5a, Wingless-type family member 5a.
References
1. Knuuti J, Wijns W, Saraste A, Capodanno D, Barbato E, Funck-Brentano C, et al. ESC Guidelines for the diagnosis and management of chronic coronary syndromes. Eur Heart J (2019 2020) 41(3):407–77. doi: 10.1093/eurheartj/ehz425
2. Tamarappoo B, Samuel TJ, Elboudwarej O, Thomson LEJ, Aldiwani H, Wei J, et al. Left ventricular circumferential strain and coronary microvascular dysfunction: A report from the women's ischemia syndrome evaluation coronary vascular dysfunction (WISE-CVD) project. Int J Cardiol (2021) 327:25–30. doi: 10.1016/j.ijcard.2020.11.006
3. Bairey Merz CN, Pepine CJ, Walsh MN, Fleg JL. Ischemia and no obstructive coronary artery disease (INOCA): Developing evidence-based therapies and research agenda for the next decade. Circulation (2017) 135(11):1075–92. doi: 10.1161/CIRCULATIONAHA.116.024534
4. Pries AR, Reglin B. Coronary microcirculatory pathophysiology: can we afford it to remain a black box? Eur Heart J (2017) 38(7):478–88. doi: 10.1093/eurheartj/ehv760
5. Sara JD, Widmer RJ, Matsuzawa Y, Lennon RJ, Lerman LO, Lerman A. Prevalence of coronary microvascular dysfunction among patients with chest pain and nonobstructive coronary artery disease. JACC Cardiovasc Interv. (2015) 8(11):1445–53. doi: 10.1016/j.jcin.2015.06.017
6. Taqueti VR, Di Carli MF. Coronary microvascular disease pathogenic mechanisms and therapeutic options: JACC state-of-the-Art review. J Am Coll Cardiol (2018) 72(21):2625–41. doi: 10.1016/j.jacc.2018.09.042
7. Bonanni A, d'Aiello A, Pedicino D, Di Sario M, Vinci R, Ponzo M, et al. Mol hallmarks ischemia non-obstructive coronary arteries: "INOCA versus Obstructive CCS" challenge. J Clin Med (2022) 11(6). doi: 10.3390/jcm11061711
8. Madonna R, Massaro M, Scoditti E, Pescetelli I, De Caterina R. The epicardial adipose tissue and the coronary arteries: dangerous liaisons. Cardiovasc Res (2019) 115(6):1013–25. doi: 10.1093/cvr/cvz062
9. Hassan M, Latif N, Yacoub M. Adipose tissue: friend or foe? Nat Rev Cardiol (2012) 9(12):689–702. doi: 10.1038/nrcardio.2012.148
10. Lavi S, Prasad A, Yang EH, Mathew V, Simari RD, Rihal CS, et al. Smoking is associated with epicardial coronary endothelial dysfunction and elevated white blood cell count in patients with chest pain and early coronary artery disease. Circulation (2007) 115(20):2621–7. doi: 10.1161/CIRCULATIONAHA.106.641654
11. Ong P, Athanasiadis A, Borgulya G, Vokshi I, Bastiaenen R, Kubik S, et al. Clinical usefulness, angiographic characteristics, and safety evaluation of intracoronary acetylcholine provocation testing among 921 consecutive white patients with unobstructed coronary arteries. Circulation (2014) 129(17):1723–30. doi: 10.1161/CIRCULATIONAHA.113.004096
12. Ostergaard L, Kristiansen SB, Angleys H, Frøkiær J, Michael Hasenkam J, Jespersen SN, et al. The role of capillary transit time heterogeneity in myocardial oxygenation and ischemic heart disease. Basic Res Cardiol (2014) 109(3):409. doi: 10.1007/s00395-014-0409-x
13. Van Mieghem CAG. CT as gatekeeper of invasive coronary angiography in patients with suspected CAD. Cardiovasc Diagn Ther (2017) 7(2):189–95. doi: 10.21037/cdt.2017.04.03
14. Schuijf JD, Matheson MB, Ostovaneh MR, Arbab-Zadeh A, Kofoed KF, Scholte A, et al. Ischemia and no obstructive stenosis (inoca) at ct angiography, ct myocardial perfusion, invasive coronary angiography, and spect: the core320 study. Radiology (2020) 294(1):61–73. doi: 10.1148/radiol.2019190978
15. Knuuti J, Ballo H, Juarez-Orozco LE, Saraste A, Kolh P, Rutjes AWS, et al. The performance of non-invasive tests to rule-in and rule-out significant coronary artery stenosis in patients with stable angina: a meta-analysis focused on post-test disease probability. Eur Heart J (2018) 39(35):3322–30. doi: 10.1093/eurheartj/ehy267
16. Pepine CJ, Anderson RD, Sharaf BL, Reis SE, Smith KM, Handberg EM, et al. Coronary microvascular reactivity to adenosine predicts adverse outcome in women evaluated for suspected ischemia results from the national heart, lung and blood institute WISE (Women's ischemia syndrome evaluation) study. J Am Coll Cardiol (2010) 55(25):2825–32. doi: 10.1016/j.jacc.2010.01.054
17. Ahmadi A, Leipsic J, Øvrehus KA, Gaur S, Bagiella E, Ko B, et al. Lesion-specific and vessel-related determinants of fractional flow reserve beyond coronary artery stenosis. JACC Cardiovasc Imaging. (2018) 11(4):521–30. doi: 10.1016/j.jcmg.2017.11.020
18. Rahman H, Scannell CM, Demir OM, Ryan M, McConkey H, Ellis H, et al. High-resolution cardiac magnetic resonance imaging techniques for the identification of coronary microvascular dysfunction. JACC Cardiovasc Imaging. (2021) 14(5):978–86. doi: 10.1016/j.jcmg.2020.10.015
19. Thomson LE, Wei J, Agarwal M, Haft-Baradaran A, Shufelt C, Mehta PK, et al. Cardiac magnetic resonance myocardial perfusion reserve index is reduced in women with coronary microvascular dysfunction. a national heart, lung, and blood institute-sponsored study from the women's ischemia syndrome evaluation. Circ Cardiovasc Imaging (2015) 8(4). doi: 10.1161/CIRCIMAGING.114.002481
20. Ford TJ, Stanley B, Good R, Rocchiccioli P, McEntegart M, Watkins S, et al. Stratified medical therapy using invasive coronary function testing in angina: The CorMicA trial. J Am Coll Cardiol (2018) 72. doi: 10.1016/j.jacc.2018.09.006
21. Tonino PA, Fearon WF, De Bruyne B, Oldroyd KG, Leesar MA, Ver Lee PN, et al. Angiographic versus functional severity of coronary artery stenoses in the FAME study fractional flow reserve versus angiography in multivessel evaluation. J Am Coll Cardiol (2010) 55(25):2816–21. doi: 10.1016/j.jacc.2009.11.096
22. Collet JP, Thiele H, Barbato E, Barthélémy O, Bauersachs J, Bhatt DL, et al. ESC Guidelines for the management of acute coronary syndromes in patients presenting without persistent ST-segment elevation. Eur Heart J (2020 2021) 42(14):1289–367. doi: 10.1093/eurheartj/ehaa575
23. Hoffmann U, Ferencik M, Udelson JE, Picard MH, Truong QA, Patel MR, et al. Prognostic value of noninvasive cardiovascular testing in patients with stable chest pain: insights from the promise trial (prospective multicenter imaging study for evaluation of chest pain). Circulation (2017) 135(24):2320–32. doi: 10.1161/CIRCULATIONAHA.116.024360
24. Iacobellis G, Bianco AC. Epicardial adipose tissue: emerging physiological, pathophysiological and clinical features. Trends Endocrinol Metab (2011) 22(11):450–7. doi: 10.1016/j.tem.2011.07.003
25. Iacobellis G. Local and systemic effects of the multifaceted epicardial adipose tissue depot. Nat Rev Endocrinol (2015) 11(6):363–71. doi: 10.1038/nrendo.2015.58
26. Dueñas A, Aranega AE, Franco D. More than just a simple cardiac envelope; cellular contributions of the epicardium. Front Cell Dev Biol (2017) 5:44. doi: 10.3389/fcell.2017.00044
27. Packer M. Epicardial adipose tissue may mediate deleterious effects of obesity and inflammation on the myocardium. J Am Coll Cardiol (2018) 71(20):2360–72. doi: 10.1016/j.jacc.2018.03.509
28. Mazurek T, Zhang L, Zalewski A, Mannion JD, Diehl JT, Arafat H, et al. Human epicardial adipose tissue is a source of inflammatory mediators. Circulation (2003) 108(20):2460–6. doi: 10.1161/01.CIR.0000099542.57313.C5
29. Murthy VL, Naya M, Taqueti VR, Foster CR, Gaber M, Hainer J, et al. Effects of sex on coronary microvascular dysfunction and cardiac outcomes. Circulation (2014) 129(24):2518–27. doi: 10.1161/CIRCULATIONAHA.113.008507
30. Ford TJ, Corcoran D, Padmanabhan S, Aman A, Rocchiccioli P, Good R, et al. Genetic dysregulation of endothelin-1 is implicated in coronary microvascular dysfunction. Eur Heart J (2020) 41(34):3239–52. doi: 10.1093/eurheartj/ehz915
31. Ford TJ, Rocchiccioli P, Good R, McEntegart M, Eteiba H, Watkins S, et al. Systemic microvascular dysfunction in microvascular and vasospastic angina. Eur Heart J (2018) 39(46):4086–97. doi: 10.1093/eurheartj/ehy529
32. Gaborit B, Venteclef N, Ancel P, Pelloux V, Gariboldi V, Leprince P, et al. Human epicardial adipose tissue has a specific transcriptomic signature depending on its anatomical peri-atrial, peri-ventricular, or peri-coronary location. Cardiovasc Res (2015) 108(1):62–73. doi: 10.1093/cvr/cvv208
33. Sorop O, van de Wouw J, Chandler S, Ohanyan V, Tune JD, Chilian WM, et al. Experimental animal models of coronary microvascular dysfunction. Cardiovasc Res (2020) 116(4):756–70. doi: 10.1093/cvr/cvaa002
34. Dutour A, Achard V, Sell H, Naour N, Collart F, Gaborit B, et al. Secretory type II phospholipase A2 is produced and secreted by epicardial adipose tissue and overexpressed in patients with coronary artery disease. J Clin Endocrinol Metab (2010) 95(2):963–7. doi: 10.1210/jc.2009-1222
35. Ivandic B, Castellani LW, Wang XP, Qiao JH, Mehrabian M, Navab M, et al. Role of group II secretory phospholipase A2 in atherosclerosis: 1. increased atherogenesis and altered lipoproteins in transgenic mice expressing group IIa phospholipase A2. Arterioscler Thromb Vasc Biol (1999) 19(5):1284–90. doi: 10.1161/01.atv.19.5.1284
36. Hirata Y, Tabata M, Kurobe H, Motoki T, Akaike M, Nishio C, et al. Coronary atherosclerosis is associated with macrophage polarization in epicardial adipose tissue. J Am Coll Cardiol (2011) 58(3):248–55. doi: 10.1016/j.jacc.2011.01.048
37. Baker AR, Harte AL, Howell N, Pritlove DC, Ranasinghe AM, da Silva NF, et al. Epicardial adipose tissue as a source of nuclear factor-kappaB and c-jun n-terminal kinase mediated inflammation in patients with coronary artery disease. J Clin Endocrinol Metab (2009) 94(1):261–7. doi: 10.1210/jc.2007-2579
38. Chadjichristos CE, Scheckenbach KE, van Veen TA, Richani Sarieddine MZ, de Wit C, Yang Z, et al. Endothelial-specific deletion of connexin40 promotes atherosclerosis by increasing CD73-dependent leukocyte adhesion. Circulation (2010) 121(1):123–31. doi: 10.1161/CIRCULATIONAHA.109.867176
39. Du Y, Ji Q, Cai L, Huang F, Lai Y, Liu Y, et al. Association between omentin-1 expression in human epicardial adipose tissue and coronary atherosclerosis. Cardiovasc Diabetol (2016) 15:90. doi: 10.1186/s12933-016-0406-5
40. Ji Q, Zhang J, Du Y, Zhu E, Wang Z, Que B, et al. Human epicardial adipose tissue-derived and circulating secreted frizzled-related protein 4 (SFRP4) levels are increased in patients with coronary artery disease. Cardiovasc Diabetol (2017) 16(1):133. doi: 10.1186/s12933-017-0612-9
41. Tong S, Du Y, Ji Q, Dong R, Cao J, Wang Z, et al. Expression of Sfrp5/Wnt5a in human epicardial adipose tissue and their relationship with coronary artery disease. Life Sci (2020) 245:117338. doi: 10.1016/j.lfs.2020.117338
42. Tong S, Ji Q, Du Y, Zhu X, Zhu C, Zhou Y. Sfrp5/Wnt pathway: A protective regulatory system in atherosclerotic cardiovascular disease. J Interferon Cytokine Res (2019) 39(8):472–82. doi: 10.1089/jir.2018.0154
43. Briones AM, Nguyen Dinh Cat A, Callera GE, Yogi A, Burger D, He Y, et al. Adipocytes produce aldosterone through calcineurin-dependent signaling pathways: implications in diabetes mellitus-associated obesity and vascular dysfunction. Hypertension (2012) 59(5):1069–78. doi: 10.1161/HYPERTENSIONAHA.111.190223
44. Nakhostine N, Nadeau R, Lamontagne D. Altered hypoxia-induced coronary vasodilatation in diabetic rabbit heart. Can J Physiol Pharmacol (1997) 75(12):1267–72. doi: 10.1139/y97-167
45. Pries AR, Secomb TW, Gaehtgens P. The endothelial surface layer. Pflugers Arch (2000) 440(5):653–66. doi: 10.1007/s004240000307
46. Stenström I, Maaniitty T, Uusitalo V, Pietilä M, Ukkonen H, Kajander S, et al. Frequency and angiographic characteristics of coronary microvascular dysfunction in stable angina: a hybrid imaging study. Eur Heart J Cardiovasc Imaging. (2017) 18(11):1206–13. doi: 10.1093/ehjci/jex193
47. Shaw JL, Nelson MD, Wei J, Motwani M, Landes S, Mehta PK, et al. Inverse association of MRI-derived native myocardial T1 and perfusion reserve index in women with evidence of ischemia and no obstructive CAD: A pilot study. Int J Cardiol (2018) 270:48–53. doi: 10.1016/j.ijcard.2018.06.086
48. Salgado-Somoza A, Teijeira-Fernández E, Rubio J, Couso E, González-Juanatey JR, Eiras S. Coronary artery disease is associated with higher epicardial retinol-binding protein 4 (RBP4) and lower glucose transporter (GLUT) 4 levels in epicardial and subcutaneous adipose tissue. Clin Endocrinol (Oxf). (2012) 76(1):51–8. doi: 10.1111/j.1365-2265.2011.04140.x
49. Antonopoulos AS, Sanna F, Sabharwal N, Thomas S, Oikonomou EK, Herdman L, et al. Detecting human coronary inflammation by imaging perivascular fat. Sci Transl Med (2017) 9(398). doi: 10.1126/scitranslmed.aal2658
50. Shimabukuro M, Hirata Y, Tabata M, Dagvasumberel M, Sato H, Kurobe H, et al. Epicardial adipose tissue volume and adipocytokine imbalance are strongly linked to human coronary atherosclerosis. Arterioscler Thromb Vasc Biol (2013) 33(5):1077–84. doi: 10.1161/ATVBAHA.112.300829
51. Virani SS, Alonso A, Benjamin EJ, Bittencourt MS, Callaway CW, Carson AP, et al. Heart disease and stroke statistics-2020 update: a report from the american heart association. Circulation (2020) 141(9):e139–596. doi: 10.1161/CIR.0000000000000757
52. Reis SE, Holubkov R, Conrad Smith AJ, Kelsey SF, Sharaf BL, Reichek N, et al. Coronary microvascular dysfunction is highly prevalent in women with chest pain in the absence of coronary artery disease: results from the NHLBI WISE study. Am Heart J (2001) 141(5):735–41. doi: 10.1067/mhj.2001.114198
53. Stubbins RE, Holcomb VB, Hong J, Núñez NP. Estrogen modulates abdominal adiposity and protects female mice from obesity and impaired glucose tolerance. Eur J Nutr (2012) 51(7):861–70. doi: 10.1007/s00394-011-0266-4
54. Cho DH, Joo HJ, Kim MN, Lim DS, Shim WJ, Park SM. Association between epicardial adipose tissue, high-sensitivity c-reactive protein and myocardial dysfunction in middle-aged men with suspected metabolic syndrome. Cardiovasc Diabetol (2018) 17(1):95. doi: 10.1186/s12933-018-0735-7
55. Rosito GA, Massaro JM, Hoffmann U, Ruberg FL, Mahabadi AA, Vasan RS, et al. Pericardial fat, visceral abdominal fat, cardiovascular disease risk factors, and vascular calcification in a community-based sample: the framingham heart study. Circulation (2008) 117(5):605–13. doi: 10.1161/CIRCULATIONAHA.107.743062
56. Koilpillai P, Aggarwal NR, Mulvagh SL. State of the art in noninvasive imaging of ischemic heart disease and coronary microvascular dysfunction in women: indications, performance, and limitations. Curr Atheroscler Rep (2020) 22(12):73. doi: 10.1007/s11883-020-00894-0
57. Nelson MR, Mookadam F, Thota V, Emani U, Al Harthi M, Lester SJ, et al. Epicardial fat: an additional measurement for subclinical atherosclerosis and cardiovascular risk stratification? J Am Soc Echocardiogr (2011) 24(3):339–45. doi: 10.1016/j.echo.2010.11.008
58. Kim BJ, Kang JG, Lee SH, Lee JY, Sung KC, Kim BS, et al. Relationship of echocardiographic epicardial fat thickness and epicardial fat volume by computed tomography with coronary artery calcification: Data from the CAESAR study. Arch Med Res (2017) 48(4):352–9. doi: 10.1016/j.arcmed.2017.06.010
59. Alexopoulos N, McLean DS, Janik M, Arepalli CD, Stillman AE, Raggi P. Epicardial adipose tissue and coronary artery plaque characteristics. Atherosclerosis (2010) 210(1):150–4. doi: 10.1016/j.atherosclerosis.2009.11.020
60. Iacobellis G, Barbaro G, Gerstein HC. Relationship of epicardial fat thickness and fasting glucose. Int J Cardiol (2008) 128(3):424–6. doi: 10.1016/j.ijcard.2007.12.072
61. Franssens BT, Nathoe HM, Visseren FL, van der Graaf Y, Leiner T. Relation of epicardial adipose tissue radiodensity to coronary artery calcium on cardiac computed tomography in patients at high risk for cardiovascular disease. Am J Cardiol (2017) 119(9):1359–65. doi: 10.1016/j.amjcard.2017.01.031
Keywords: epicardial adipose tissue, coronary microvascular dysfunction, ischemic non-obstructive coronary artery disease, adipocytokine, inflammatory mediators
Citation: Shan T, Shuwen Z, Hengbin W and Min Z (2023) Can EAT be an INOCA goalkeeper. Front. Endocrinol. 13:1028429. doi: 10.3389/fendo.2022.1028429
Received: 26 August 2022; Accepted: 16 December 2022;
Published: 20 January 2023.
Edited by:
Frederic Castinetti, Aix-Marseille Université, FranceReviewed by:
ELisabetta Ricottini, Campus Bio-Medico University, ItalyGeorge Nikov Chaldakov, Medical University of Varna, Bulgaria
Copyright © 2023 Shan, Shuwen, Hengbin and Min. This is an open-access article distributed under the terms of the Creative Commons Attribution License (CC BY). The use, distribution or reproduction in other forums is permitted, provided the original author(s) and the copyright owner(s) are credited and that the original publication in this journal is cited, in accordance with accepted academic practice. No use, distribution or reproduction is permitted which does not comply with these terms.
*Correspondence: Zeng Min, hndzm6@126.com
†These authors have contributed equally to this work