- 1Laboratorio de Fisiopatología Renal, Instituto de Ciencias Biomédicas, Universidad Autónoma de Chile, Santiago, Chile
- 2Facultad de Medicina y Ciencia, Universidad San Sebastián, Santiago, Chile
In recent studies, primary aldosteronism (PA) has been reported as the most common etiology for secondary hypertension of endocrine origin, accounting for approximately 10% of cases. In PA, excess aldosterone production can lead to deleterious effects at the cardiovascular (CV) and renal levels by activating mineralocorticoid receptors, which involves an increase in pro-inflammatory and pro-fibrotic mediators. Among these mediators, neutrophil gelatinase–associated lipocalin (NGAL), a secretion glycoprotein belonging to the lipocalin superfamily, has been closely linked to CV and renal damage in several pathological conditions. Because NGAL can be detected in biofluids such as plasma and urine, it has been proposed as a damage biomarker for target tissues and has also been studied for its role in hypertension and associated with PA. NGAL is produced by many different cell types, can be carried on extracellular vesicles, and is modulated by microRNAs, which would support its use as a biomarker for endocrine hypertension due to PA. Over the last decade, studies have shown that NGAL is necessary for the development of aldosterone-induced hypertension and that is associated with end-organ damage. In addition, it has been proposed that some mechanisms are dependent on the activation of immune cells, such as dendritic cells and macrophages, where the release of specific cytokines (i.e., interleukin [IL]-23) or chemokines (i.e., CCL-5) induced by aldosterone would depend on NGAL. Subsequently, this activates the T helper (Th) lymphocytes, such as Th17 and Th2, resulting in CV and renal fibrosis due to the high aldosterone levels. Although the immune system has been closely associated with essential hypertension, its participation in endocrine hypertension has not been fully elucidated. This review discusses the link between NGAL and endocrine hypertension, particularly in the context of PA, and their possible regulators and mechanisms, with a focus on its role as an immunomodulator.
Introduction
Arterial hypertension (AH) affects 31.1% of the global population (1). Its etiology is multifactorial, and many cases are classified as essential AH because their precise origin is unknown. Approximately 10%–15% of hypertensive patients have secondary AH, with renal arterial disease and endocrine AH as the most common etiologies. Primary aldosteronism (PA) is the most common etiology for endocrine AH (2).
Neutrophil gelatinase–associated lipocalin (NGAL), also known as lipocalin-2 (Lcn2) or 24p3, is a 25-kDa secretion protein belonging to the lipocalin superfamily, which can bind siderophores for iron import to different cell types (3). Although its expression may vary in some physiological conditions (4, 5), a significant increase in NGAL levels has been closely related to several renal and cardiovascular (CV) disorders, involving the activation of the mineralocorticoid receptors (MR). Thus, NGAL has emerged as a possible biomarker for acute and chronic sub-inflammatory conditions, including PA.
Here, we present several conditions that can modify NGAL abundance depending on aldosterone (Aldo) levels. Our discussion will mainly focus on the mechanisms where NGAL may function as a modulator of the immune response driving tissue fibrosis, in hopes of providing new insight into its role as a biomarker for PA.
Endocrine arterial hypertension and primary aldosteronism
PA is clinically defined as inappropriate synthesis and secretion of Aldo, independent of the renin–angiotensin system, that cannot be suppressed by sodium loading (6). The prevalence of PA varies depending on the diagnostic criteria, but approximately 10% of patients with AH present PA (7), and its prevalence may be increased in patients with resistant AH (8). Patients with PA have a higher risk of CV and renal complications, as well as a higher risk mortality as compared to those with essential AH (9–11). Excess Aldo production favors MR activation in different cell types, which can increase the risk of morbidity and mortality (9, 12) even in normotensive patients (13). Thus, increased MR activity due to PA is seen in AH and in target organ damage.
Current guidelines recommend using the Aldo to renin ratio for PA analysis (14). However, sensitive technology (e.g., liquid chromatography–mass spectrometry) is required for quantification because of the low plasma concentrations of these components. This relationship may also be modified by different factors (15). Therefore, early identification of PA, with emphasis on Aldo target tissues and cells, both represents a challenge related to its diagnosis (16) and is also relevant for the stratification of risk related to the pro-inflammatory and pro-fibrotic that can be caused by Aldo–MR interaction in different cell types (17).
NGAL is a sensitive target gene to the complex Aldo–MR, which has allowed to consider it as a biomarker for CV and renal disease associated with PA (18, 19). Moreover, recent findings suggest that extracellular vesicles (EVs) isolated from liquid biopsies may carry NGAL, representing a new diagnostic tool for PA (20).
NGAL as a biomarker of renal damage and arterial hypertension
Kjeldsen et al. initially described NGAL in human neutrophils (21, 22), where it is expressed in forms of mRNA and protein change based on the granulopoiesis stage (23). However, NGAL can originate from many sites: the hematopoietic organs (24); adipocytes; hepatocytes (25); neoplastic cells; lungs (26); mammary glands and uterus (4); neurons; and endothelial, cardiac, and smooth muscle cells (27).
Recently, we characterized the relative abundance of NGAL in different mouse immune cells. We observed that its expression in CD11chigh antigen-presenting cells (APCs) is higher than that in B and T lymphocytes (28). This observation is relevant considering that these immune cells can potentially promote AH (18) and end-organ damage associated with AH (29).
In the kidney, NGAL expression has been described in specific nephron regions augmented in different experimental and clinical settings of renal dysfunction, suggesting that NGAL is a specific biomarker for kidney injury (30–33). In particular, NGAL increases considerably in response to ischemic or obstructive renal disease in mice (31, 34, 35), suggesting a direct correlation between inflammatory processes and oxidative stress in the development of kidney disease. Furthermore, Viau et al. showed the prevention of tubular dilation and glomerular and tubular fibrosis in an experimental model of chronic kidney disease (CKD) in NGAL knock-out (KO) mice (36), indicating its critical role.
In terms of NGAL’s potential as a biomarker, studies have shown that high urinary NGAL urinary levels of NGAL (uNGAL) levels are directly associated with tubulointerstitial fibrosis and tubular atrophy in patients and experimental animals. In contrast, it is inversely correlated with the glomerular filtration rate in kidney disease (36, 37). Over the last few years, studies have proposed that early identification of uNGAL or serum NGAL (sNGAL) would be helpful not only in the early diagnosis of kidney disease (37–39) but also in tracking the progression of acute kidney injury (31) and its transition to CKD in real time (40). Thus, using NGAL as a biomarker in the early stages of renal damage could be crucial for the prognosis of patients progressing to CKD (41).
Because CKD and AH are closely linked, several studies published during the last decade have considered using sNGAL and/or uNGAL as biomarkers to determine the CV and renal risk in patients with AH. For instance, uNGAL levels (normalized to urine creatinine) were found to correlate with systolic blood pressure (SBP) and diastolic blood pressure (DBP) in a study of 100 healthy volunteers from Hong Kong (42). Similarly, Wu et al. demonstrated that sNGAL was significantly associated with SBP and DBP in a cohort of 707 patients, comprising those with CV disease and those who were CV disease–free (43). Malyszko et al. showed that uNGAL and sNGAL levels in hypertensive patients were higher than in normotensive and healthy volunteers (44). Because the NGAL levels in serum and urine may be modified by a patient's renal function, the increase in sNGAL and uNGAL levels may also be affected, considering the differences in renal function among the study participants. Finally, Lindberg et al. revealed that plasma NGAL levels were positively correlated with SBP and neutrophil count and inversely correlated with renal function (45). All of these studies defined AH as SBP > 140 mmHg without specifying its etiology. The origin of the increase in uNGAL and sNGAL levels observed in endocrine AH therefore remains to be clarified. However, the origin of these increased levels in the context of PA is well understood, and the mechanisms underlying this phenomenon will be discussed in the following sections.
Recently, the use of NGAL carried on EVs as a biomarker in hypertensive patients has also been considered. Several studies in renal transplantation have demonstrated that exosome cargo obtained from urine involves NGAL in the forms of mRNA (46) and protein (47). Other studies in central and peripheral inflammatory conditions have shown NGAL expression in EVs isolated from biofluids such as plasma (48) and saliva (49). In the case of AH, Barros et al. showed that alpha-1-acid-glycoprotein (AGP1, also known as orosomucoid-1) is upregulated in urinary EVs from hypertensive patients compared with those of normotensive patients (50). AGP1 is an inflammation-sensitive plasma protein that is increased in patients with higher CV risk (51) and is related to lipocalins, including NGAL. However, NGAL upregulation in the EVs of hypertensive patients remains to be explored.
NGAL and endocrine arterial hypertension
To date, the hormonal regulation of NGAL expression associated with AH has been poorly investigated. In this context, excess glucocorticoid production due to an endogenous or exogenous origin induces AH (52) by activating the glucocorticoid receptors (GR) in peripheral tissues (53). In particular, the GR in vascular smooth muscle cells has been considered crucial for increasing blood pressure during glucocorticoid excess (54). This evidence is relevant, considering that the GR at the CV level modulate NGAL abundance during dexamethasone treatment (55). In addition, an experimental study in rats showed that 2-week oral administration of corticosterone increased NGAL protein expression in the glomeruli and renal tubule by 75% and 30%, respectively (56).
In terms of other “non-classic” tissues (or cells) in AH, Vizzardelli et al. reported that glucocorticoids have a synergic effect on lipopolysaccharides involved in NGAL production in dendritic cells (DCs) (57), which are the principal APCs involved in AH. Because high glucocorticoid levels can also activate the MR, it is worth mentioning that some of the effects described could occur through this receptor (58). This hypothesis is supported by a recent study that reported that hypertensive patients diagnosed with non-classic apparent mineralocorticoid excess plus PA presented higher NGAL plasma levels than those with PA only (59). The regulation of NGAL by the glucocorticoid–MR complex in other immune cell types in endocrine AH remains to be explored further.
NGAL modulation due to MR activation by Aldo during AH has been better described, particularly at the experimental level. In clinical settings, patients with PA presented high sNGAL levels associated with metalloproteinase (MMP)-9 (60). However, potential modulation of sNGAL levels by MR antagonism or adrenalectomy has not been reported. Kozlowki et al. reported that uNGAL levels are increased in patients undergoing elective posterior retroperitoneal adrenalectomy; however, uNGAL was used in this case as a biomarker for kidney injury associated with the procedure and not for PA (61). This study did not report levels of sNGAL or adrenal hormones before the intervention. Therefore, new studies are needed to clarify this relationship and to support the role of sNGAL as a biomarker of PA.
At the experimental level, Latouche et al. initially demonstrated that mRNA NGAL is strongly induced in cardiac and vascular smooth muscle cells after mineralocorticoid infusion in rodents (27). However, spironolactone, an MR antagonist, prevented these effects, suggesting that the MR directly controls the NGAL expression in CV cells. Subsequently, Tarjus et al. demonstrated that high blood pressure and CV fibrosis, also characterized by galectin-3 (Gal3) and collagen (Col)-1 upregulation, induced by the “nephrectomy Aldo–salt (NAS) model,” are prevented in NGAL–KO mice, suggesting a direct link between NGAL and endocrine AH due to excess Aldo production (60). Another study showed that AH, CV fibrosis, and the pro-inflammatory phenotype in NAS mice are attenuated not only by the complete genetic deficiency of NGAL but also by depletion confined to the myeloid compartment (62). This study attained new insights regarding the effect of NGAL on APCs, considering their myeloid origin.
Recently, a study showed that Aldo induces mRNA NGAL expression in DCs by MR activation (18). In addition, CD11chigh APCs are necessary for developing cardiac hypertrophy and CV fibrosis in NAS mice. Buonafine et al. showed that NGAL levels were increased in macrophages (Mϕ), DCs, and peripheral blood mononuclear cells in NAS mice compared with control mice. By contrast, NGAL levels in B lymphocytes and CD4+ and CD8+ T lymphocytes of NAS-treated mice did not differ from those of control mice, even when NAS increased the recruitment of these cells in the lymph nodes (62). These findings suggest that NGAL from Mϕ and DCs is secreted during mineralocorticoid excess, which may contribute to the CV remodeling that has previously been reported. Additionally, a positive correlation was found between NGAL mRNA levels and MR in all the immune cells studied at the basal state (62), indicating a strong association between MR and NGAL in the immune cells. Notably, NGAL modulation seems specific to MR activation. Preliminary studies from our group have revealed that NGAL is not significantly modified in target CV tissues and other immune cells after stimulation of angiotensin II (AngII), another critical hormone in AH (data not published).
The role of NGAL in the immune system during aldosterone-dependent hypertension
The link between the immune system and AH was first suggested in the 1960s, when autoantibodies were identified in the arteries of hypertensive cadavers (63). Since then, this finding has been supported by additional experimental studies (64, 65). The causal role of the immune system in secondary AH was supported by a study by Grollman and White that demonstrated that immunosuppressants could control blood pressure in rats with partial renal infarction (66). Later, additional evidence implicated the immune system in the development of AH and its association with end-organ damage (29).
Many different immune cells have been directly associated with blood pressure control. The most notable among them are monocytes/Mϕ, DCs, and T lymphocytes, which participate in AngII- or Aldo-dependent AH (18, 67–74) and NaCl-sensitive AH (75–79). Part of the mechanism proposed for T-cell activation is the formation of neoantigens from APCs (80–82) through increased reactive oxygen species formation and subsequent protein modifications (83, 84). By contrast, polymorphonuclear leukocytes, such as neutrophils, have also been considered to be among the immune cells contributing to AH (85). An increased blood neutrophil-to-lymphocyte ratio (NLR) has been observed in hypertensive patients. Moreover, one multicentric study found that NLR was positively correlated with plasma Aldo concentrations and identified it as a significant predictor of CKD among patients with PA (86). Although Aldo may directly activate the p38, ERK1/2, and PI3K pathways in human neutrophils (87, 88), which favors MMP-9/NGAL upregulation, its role in Aldo-dependent AH is still controversial, considering that Aldo inhibits nuclear factor κB (NF-κB) through MR activation in neutrophils (89). Therefore, further studies are needed to explore the Aldo–MR–NGAL relationship in neutrophil-related inflammation.
Aldo can bind to the MR in DCs and Mϕ because it is mainly present in APCs. Ko et al. demonstrated that blood pressure elevation was prevented during treatment with mineralocorticoids and salt (the DOCA–salt model) in mice deficient in Mϕ colony-stimulating factor (M-CSF), a relevant protein for Mϕ differentiation (90). Later, Rickard et al. demonstrated that myeloid MR deletion prevents AH induced by DOCA–salt. They used the Cre/LoxP recombination system, along with a lysozyme M promoter for selective MR depletion on monocytes/Mϕ (My–MR–KO), during 8 weeks of DOCA–salt treatment in mice. Cardiac remodeling associated with endocrine AH was reduced in the My–MR–KO mice (91). Subsequently, the same group demonstrated that cardiac Mϕ isolated from DOCA–salt mice showed an increment of tumor necrosis factor (TNF)-α and the chemokine C-X-C motif ligand (CXCL)-9, which promotes T lymphocyte infiltration and cardiac fibrosis, while these increases were prevented in My–MR–KO animals (92).
Based on previous evidence, the effects of the MR on APCs associated with endocrine AH can be explained by NGAL induction and its secondary impact as an immunomodulator. Recently, our group demonstrated that NGAL mediates the upregulation of IL-23p19 and IL-23p40 subunits in CD11chigh DCs treated with Aldo in an MR-dependent way (18). Notably, we observed that NGAL does not affect the in vitro differentiation of CD11chigh DCs from mouse bone marrow (18), which was supported by other studies in terms of maturation (93). Interestingly, IL-23p19 and IL-23p40 subunits have been reported as crucial for Th17 polarization from naïve T-CD4+ lymphocytes (94), suggesting that IL-17A secretion from Th17 lymphocytes may be affected by NGAL levels during hyperaldosteronism (Figure 1). Leopold proposed a similar mechanism in CV remodeling that considered post-translational NGAL modifications that may affect the CV abundance of Gal3 and Col-1 (95).
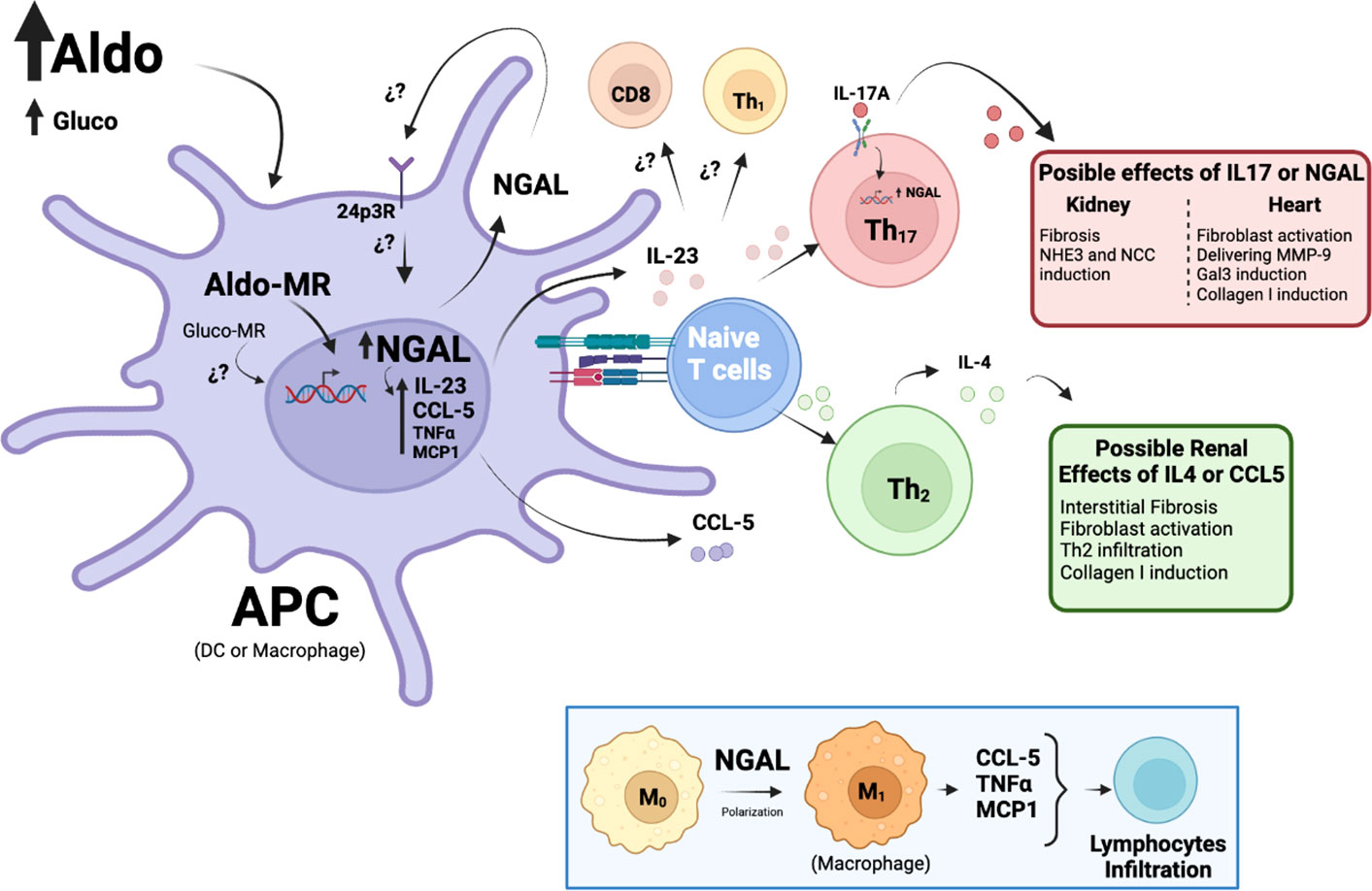
Figure 1 Proposed mechanisms of NGAL as an immunomodulator on APCs during Aldo-dependent endocrine hypertension. Elevated Aldo (or Gluco) levels can activate the MR in APCs (DCs or Mϕ) and promote the transcription of several genes, such as NGAL. NGAL may promote the release of pro-inflammatory factors from APCs, such as MCP-1, TNFα, and CCL-5, triggering lymphocyte infiltration in target tissues, particularly Th2 lymphocytes. Th2 lymphocytes release IL-4, favoring interstitial fibrosis and augmenting renal damage. By contrast, NGAL modulates the production of IL-23, a cytokine essential for Th17 lymphocyte polarization, which has been closely related to renal and CV fibrosis, as well as NHE3 and NCC induction and transcriptional increases of NGAL. Finally, NGAL may directly activate the Mϕ to M1 pro-inflammatory phenotype, which has also been associated with MR-dependent AH (see insert).
During kidney injury (96) and AH (97), NGAL is dependent on the IL-17A axis. Norlander et al. demonstrated that IL-17A upregulates the abundance of the sodium-hydrogen exchanger 3 (NHE3) protein and promotes the activity of sodium chloride cotransporter (NCC) in the human proximal tubule and mouse distal convoluted tubule cells, respectively (98). This suggests that NGAL may be involved in sodium reabsorption at the tubular level through IL-17A in PA. However, further studies will be needed to determine the IL-17 dependence on NGAL from APCs during MR activation.
In addition, Bonnard et al. demonstrated that NGAL from Mϕ is also necessary for the renal expression of extracellular matrix proteins, such as Col-1, αSMA, and fibronectin, which are associated with interstitial fibrosis (99). They demonstrated that NGAL is required for chemokine ligand (CCL)-5 induction in Mϕ stimulated with Aldo and salt in vitro, a relevant finding given that the pharmacological blockade of the CCL5 receptor reduced renal Th2–CD4+ lymphocyte infiltration induced by NAS. Finally, they observed that this blockade and the resulting neutralization of IL-4, a cytokine secreted by Th2-CD4+ lymphocytes, prevents interstitial fibrosis in the kidney, suggesting that these Th cells are also involved because of MR activation by Aldo (Figure 1). The previous findings indicate that NGAL may act as an immunomodulator in AH-associated PA, triggering a pro-inflammatory phenotype related to target organ damage, where the action of APCs (mainly DCs and Mϕ) is required.
Limited information is available regarding the cell types targeted by NGAL and the pathways involved. Recombinant NGAL has been shown to promote M1 polarization in microglia (100), which are the resident Mϕ in the central nervous system. This is relevant given that M1 Mϕ are responsible for producing a wide range of pro-inflammatory cytokines and chemokines, including TNF-α, CCL5, and monocyte chemokine protein (MCP)-1 (101–103). Similarly, Pawar et al. reported that 3 days of intraperitoneal injections of recombinant NGAL upregulated MCP-1 in the kidneys of wild-type mice (104). These mediators are critical for Th cell infiltration during Aldo-dependent AH, which would be modulated by NGAL (Figure 1, insert).
Finally, whether NGAL can activate a specific receptor on APCs and target cells at the CV and renal levels in the MR–Aldo complex remains unknown. In physiological conditions, NGAL acts mainly through its endocytic 24p3 receptor (24p3R), which is present in neurons (105), the intestinal epithelium (106), the distal and collecting tubules (107), and immune cells, such as Mϕ and neutrophils (108). However, additional studies will be needed to determine whether 24p3R is modulated by the MR–Aldo complex in the APCs involved in AH.
Concluding remarks
Over the last 20 years, several studies have shed light on the role of the immune system in the development of AH and target organ damage, emphasizing some emerging mechanisms by which APCs activate Th cells, which has also been extensible for endocrine hypertension. Among the various origins of endocrine AH, PA seems to be the principal etiology.
Experimental studies have demonstrated that in the presence of abnormally elevated levels of Aldo, the APCs, mainly CD11chigh DCs and Mϕ, may potentiate additional mechanisms for antigen presentation driving T lymphocyte polarization through molecules, such as NGAL by MR activation. However, the effects of these APCs in endocrine AH, and the mechanisms by which NGAL modulates T cells, have not been fully clarified. Additional pre-clinical and clinical studies are needed to answer this question.
NGAL after MR activation in APCs would favor the differentiation and recruitment of Th17 and Th2 lymphocytes through the release of IL-23 and CCL-5, respectively. However, whether NGAL modulates polarization toward other Th lymphocytes strongly associated with AH, such as Th1 lymphocytes and T CD8+-lymphocytes, in the context of endocrine hypertension and high Aldo levels is still unknown.
Author contributions
Conceptualization, PA and CAA; Investigation, PA and CAA; Writing—original draft preparation, PA and CAA; Writing—review and editing, CAA; Figures, PA and CAA; Supervision, CAA; Funding acquisition, PA and CAA Both authors have read and approved the published version of the manuscript.
Funding
This work was supported by the National Agency of Research and Development (ANID), Fondecyt Regular #1201251 (CAA) and Fondecyt de Postdoctorado #3201016 (PA).
Acknowledgments
We thank Pamela Torres for the administrative assistance at Universidad Autónoma de Chile.
Conflict of interest
The authors declare that the research was conducted without any commercial or financial relationships that can be construed as a potential conflict of interest.
Publisher’s note
All claims expressed in this article are solely those of the authors and do not necessarily represent those of their affiliated organizations, or those of the publisher, the editors and the reviewers. Any product that may be evaluated in this article, or claim that may be made by its manufacturer, is not guaranteed or endorsed by the publisher.
References
1. Mills KT, Bundy JD, Kelly TN, Reed JE, Kearney PM, Reynolds K, et al. Global disparities of hypertension prevalence and control: A systematic analysis of population-based studies from 90 countries. Circulation (2016) 134(6):441–50. doi: 10.1161/CIRCULATIONAHA.115.018912
2. Rimoldi SF, Scherrer U, Messerli FH. Secondary arterial hypertension: when, who, and how to screen? Eur Heart J (2014) 35(19):1245–54. doi: 10.1093/eurheartj/eht534
3. Flo TH, Smith KD, Sato S, Rodriguez DJ, Holmes MA, Strong RK, et al. Lipocalin 2 mediates an innate immune response to bacterial infection by sequestrating iron. Nature (2004) 432(7019):917–21. doi: 10.1038/nature03104
4. Nilsen-Hamilton M, Liu Q, Ryon J, Bendickson L, Lepont P, Chang Q. Tissue involution and the acute phase response. Ann N Y Acad Sci (2003) 995:94–108. doi: 10.1111/j.1749-6632.2003.tb03213.x
5. Borregaard N, Sehested M, Nielsen BS, Sengelov H, Kjeldsen L. Biosynthesis of granule proteins in normal human bone marrow cells. gelatinase is a marker of terminal neutrophil differentiation. Blood (1995) 85(3):812–7. doi: 10.1182/blood.V85.3.812.bloodjournal853812
6. Mulatero P, Monticone S, Deinum J, Amar L, Prejbisz A, Zennaro MC, et al. Genetics, prevalence, screening and confirmation of primary aldosteronism: a position statement and consensus of the working group on endocrine hypertension of the European society of hypertension. J Hypertens (2020) 38(10):1919–28. doi: 10.1097/HJH.0000000000002510
7. Funder JW, Carey RM. Primary aldosteronism: Where are we now? Where To From Here? Hypertension (2022) 79(4):726–35. doi: 10.1161/HYPERTENSIONAHA.121.18761
8. Brown JM, Siddiqui M, Calhoun DA, Carey RM, Hopkins PN, Williams GH, et al. The unrecognized prevalence of primary aldosteronism: A cross-sectional study. Ann Intern Med (2020) 173(1):10–20. doi: 10.7326/M20-0065
9. Hundemer GL, Curhan GC, Yozamp N, Wang M, Vaidya A. Cardiometabolic outcomes and mortality in medically treated primary aldosteronism: a retrospective cohort study. Lancet Diabetes Endocrinol (2018) 6(1):51–9. doi: 10.1016/S2213-8587(17)30367-4
10. Hundemer GL, Curhan GC, Yozamp N, Wang M, Vaidya A. Renal outcomes in medically and surgically treated primary aldosteronism. Hypertension (2018) 72(3):658–66. doi: 10.1161/HYPERTENSIONAHA.118.11568
11. Meng Z, Dai Z, Huang K, Xu C, Zhang YG, Zheng H, et al. Long-term mortality for patients of primary aldosteronism compared with essential hypertension: A systematic review and meta-analysis. Front Endocrinol (Lausanne) (2020) 11:121. doi: 10.3389/fendo.2020.00121
12. Monticone S, D'Ascenzo F, Moretti C, Williams TA, Veglio F, Gaita F, et al. Cardiovascular events and target organ damage in primary aldosteronism compared with essential hypertension: a systematic review and meta-analysis. Lancet Diabetes Endocrinol (2018) 6(1):41–50. doi: 10.1016/S2213-8587(17)30319-4
13. Wannachalee T, Turcu AF. Primary aldosteronism: a continuum from normotension to hypertension. Curr Cardiol Rep (2021) 23(8):105. doi: 10.1007/s11886-021-01538-8
14. Funder JW, Carey RM, Mantero F, Murad MH, Reincke M, Shibata H, et al. The management of primary aldosteronism: Case detection, diagnosis, and treatment: An endocrine society clinical practice guideline. J Clin Endocrinol Metab (2016) 101(5):1889–916. doi: 10.1210/jc.2015-4061
15. Hung A, Ahmed S, Gupta A, Davis A, Kline GA, Leung AA, et al. Performance of the aldosterone to renin ratio as a screening test for primary aldosteronism. J Clin Endocrinol Metab (2021) 106(8):2423–35. doi: 10.1210/clinem/dgab348
16. Ruhle BC, White MG, Alsafran S, Kaplan EL, Angelos P, Grogan RH. Keeping primary aldosteronism in mind: Deficiencies in screening at-risk hypertensives. Surgery (2019) 165(1):221–7. doi: 10.1016/j.surg.2018.05.085
17. Nguyen Dinh Cat A, Jaisser F. Extrarenal effects of aldosterone. Curr Opin Nephrol Hypertens (2012) 21(2):147–56. doi: 10.1097/MNH.0b013e32834fb25b
18. Araos P, Prado C, Lozano M, Figueroa S, Espinoza A, Berger T, et al. Dendritic cells are crucial for cardiovascular remodeling and modulate neutrophil gelatinase-associated lipocalin expression upon mineralocorticoid receptor activation. J Hypertens (2019) 37(7):1482–92. doi: 10.1097/HJH.0000000000002067
19. Barnett AM, Babcock MC, Watso JC, Migdal KU, Gutierrez OM, Farquhar WB, et al. High dietary salt intake increases urinary NGAL excretion and creatinine clearance in healthy young adults. Am J Physiol Renal Physiol (2022) 322(4):F392–402. doi: 10.1152/ajprenal.00240.2021
20. Carvajal CA, Tapia-Castillo A, Perez JA, Fardella CE. Primary aldosteronism, aldosterone, and extracellular vesicles. Endocrinology (2022) 163(1): 1–12. doi: 10.1210/endocr/bqab240
21. Kjeldsen L, Johnsen AH, Sengelov H, Borregaard N. Isolation and primary structure of NGAL, a novel protein associated with human neutrophil gelatinase. J Biol Chem (1993) 268(14):10425–32. doi: 10.1016/S0021-9258(18)82217-7
22. Kjeldsen L, Bainton DF, Sengelov H, Borregaard N. Identification of neutrophil gelatinase-associated lipocalin as a novel matrix protein of specific granules in human neutrophils. Blood (1994) 83(3):799–807. doi: 10.1182/blood.V83.3.799.799
23. Le Cabec V, Cowland JB, Calafat J, Borregaard N. Targeting of proteins to granule subsets is determined by timing and not by sorting: The specific granule protein NGAL is localized to azurophil granules when expressed in HL-60 cells. Proc Natl Acad Sci U S A (1996) 93(13):6454–7. doi: 10.1073/pnas.93.13.6454
24. Aigner F, Maier HT, Schwelberger HG, Wallnofer EA, Amberger A, Obrist P, et al. Lipocalin-2 regulates the inflammatory response during ischemia and reperfusion of the transplanted heart. Am J Transpl (2007) 7(4):779–88. doi: 10.1111/j.1600-6143.2006.01723.x
25. Ariza X, Graupera I, Coll M, Sola E, Barreto R, Garcia E, et al. Neutrophil gelatinase-associated lipocalin is a biomarker of acute-on-chronic liver failure and prognosis in cirrhosis. J Hepatol (2016) 65(1):57–65. doi: 10.1016/j.jhep.2016.03.002
26. Wang Y, Jia M, Yan X, Cao L, Barnes PJ, Adcock IM, et al. Increased neutrophil gelatinase-associated lipocalin (NGAL) promotes airway remodelling in chronic obstructive pulmonary disease. Clin Sci (Lond) (2017) 131(11):1147–59. doi: 10.1042/CS20170096
27. Latouche C, El Moghrabi S, Messaoudi S, Nguyen Dinh Cat A, Hernandez-Diaz I, Alvarez de la Rosa D, et al. Neutrophil gelatinase-associated lipocalin is a novel mineralocorticoid target in the cardiovascular system. Hypertension (2012) 59(5):966–72. doi: 10.1161/HYPERTENSIONAHA.111.187872
28. Buonafine M, Martinez-Martinez E, Jaisser F. More than a simple biomarker: the role of NGAL in cardiovascular and renal diseases. Clin Sci (Lond) (2018) 132(9):909–23. doi: 10.1042/CS20171592
29. Madhur MS, Elijovich F, Alexander MR, Pitzer A, Ishimwe J, Van Beusecum JP, et al. Hypertension: Do inflammation and immunity hold the key to solving this epidemic? Circ Res (2021) 128(7):908–33. doi: 10.1161/CIRCRESAHA.121.318052
30. Ix JH, Shlipak MG. The promise of tubule biomarkers in kidney disease: A review. Am J Kidney Dis (2021) 78(5):719–27. doi: 10.1053/j.ajkd.2021.03.026
31. Paragas N, Qiu A, Zhang Q, Samstein B, Deng SX, Schmidt-Ott KM, et al. The ngal reporter mouse detects the response of the kidney to injury in real time. Nat Med (2011) 17(2):216–22. doi: 10.1038/nm.2290
32. Mishra J, Ma Q, Prada A, Mitsnefes M, Zahedi K, Yang J, et al. Identification of neutrophil gelatinase-associated lipocalin as a novel early urinary biomarker for ischemic renal injury. J Am Soc Nephrol (2003) 14(10):2534–43. doi: 10.1097/01.ASN.0000088027.54400.C6
33. Supavekin S, Zhang W, Kucherlapati R, Kaskel FJ, Moore LC, Devarajan P. Differential gene expression following early renal ischemia/reperfusion. Kidney Int (2003) 63(5):1714–24. doi: 10.1046/j.1523-1755.2003.00928.x
34. Berger T, Togawa A, Duncan GS, Elia AJ, You-Ten A, Wakeham A, et al. Lipocalin 2-deficient mice exhibit increased sensitivity to escherichia coli infection but not to ischemia-reperfusion injury. Proc Natl Acad Sci U S A (2006) 103(6):1834–9. doi: 10.1073/pnas.0510847103
35. Amador CA, Bertocchio JP, Andre-Gregoire G, Placier S, Duong Van Huyen JP, El Moghrabi S, et al. Deletion of mineralocorticoid receptors in smooth muscle cells blunts renal vascular resistance following acute cyclosporine administration. Kidney Int (2016) 89(2):354–62. doi: 10.1038/ki.2015.312
36. Viau A, El Karoui K, Laouari D, Burtin M, Nguyen C, Mori K, et al. Lipocalin 2 is essential for chronic kidney disease progression in mice and humans. J Clin Invest (2010) 120(11):4065–76. doi: 10.1172/JCI42004
37. Kuwabara T, Mori K, Mukoyama M, Kasahara M, Yokoi H, Saito Y, et al. Urinary neutrophil gelatinase-associated lipocalin levels reflect damage to glomeruli, proximal tubules, and distal nephrons. Kidney Int (2009) 75(3):285–94. doi: 10.1038/ki.2008.499
38. Nickolas TL, Forster CS, Sise ME, Barasch N, Sola-Del Valle D, Viltard M, et al. NGAL (Lcn2) monomer is associated with tubulointerstitial damage in chronic kidney disease. Kidney Int (2012) 82(6):718–22. doi: 10.1038/ki.2012.195
39. Singer E, Marko L, Paragas N, Barasch J, Dragun D, Muller DN, et al. Neutrophil gelatinase-associated lipocalin: pathophysiology and clinical applications. Acta Physiol (Oxf) (2013) 207(4):663–72. doi: 10.1111/apha.12054
40. Ko GJ, Grigoryev DN, Linfert D, Jang HR, Watkins T, Cheadle C, et al. Transcriptional analysis of kidneys during repair from AKI reveals possible roles for NGAL and KIM-1 as biomarkers of AKI-to-CKD transition. Am J Physiol Renal Physiol (2010) 298(6):F1472–83. doi: 10.1152/ajprenal.00619.2009
41. Woodson BW, Wang L, Mandava S, Lee BR. Urinary cystatin c and NGAL as early biomarkers for assessment of renal ischemia-reperfusion injury: a serum marker to replace creatinine? J Endourol (2013) 27(12):1510–5. doi: 10.1089/end.2013.0198
42. Li D, Li H, Bauer C, Hu Y, Lewis JR, Xu A, et al. Lipocalin-2 variants and their relationship with cardio-renal risk factors. Front Endocrinol (Lausanne) (2021) 12:781763. doi: 10.3389/fendo.2021.781763
43. Wu G, Li H, Fang Q, Jiang S, Zhang L, Zhang J, et al. Elevated circulating lipocalin-2 levels independently predict incident cardiovascular events in men in a population-based cohort. Arterioscler Thromb Vasc Biol (2014) 34(11):2457–64. doi: 10.1161/ATVBAHA.114.303718
44. Malyszko J, Bachorzewska-Gajewska H, Malyszko JS, Pawlak K, Dobrzycki S. Serum neutrophil gelatinase-associated lipocalin as a marker of renal function in hypertensive and normotensive patients with coronary artery disease. Nephrol (Carlton) (2008) 13(2):153–6. doi: 10.1111/j.1440-1797.2007.00899.x
45. Lindberg S, Jensen JS, Mogelvang R, Pedersen SH, Galatius S, Flyvbjerg A, et al. Plasma neutrophil gelatinase-associated lipocalinin in the general population: association with inflammation and prognosis. Arterioscler Thromb Vasc Biol (2014) 34(9):2135–42. doi: 10.1161/ATVBAHA.114.303950
46. Peake PW, Pianta TJ, Succar L, Fernando M, Pugh DJ, McNamara K, et al. A comparison of the ability of levels of urinary biomarker proteins and exosomal mRNA to predict outcomes after renal transplantation. PloS One (2014) 9(2):e98644. doi: 10.1371/journal.pone.0098644
47. Alvarez S, Suazo C, Boltansky A, Ursu M, Carvajal D, Innocenti G, et al. Urinary exosomes as a source of kidney dysfunction biomarker in renal transplantation. Transplant Proc (2013) 45(10):3719–23. doi: 10.1016/j.transproceed.2013.08.079
48. Pineles B, Mani A, Sura L, Rossignol C, Albayram M, Weiss MD, et al. Neuronal exosome proteins: novel biomarkers for predicting neonatal response to therapeutic hypothermia. Arch Dis Child Fetal Neonatal Ed (2022) 107(1):60–4. doi: 10.1136/archdischild-2020-321096
49. Aqrawi LA, Galtung HK, Guerreiro EM, Ovstebo R, Thiede B, Utheim TP, et al. Proteomic and histopathological characterisation of sicca subjects and primary sjogren's syndrome patients reveals promising tear, saliva and extracellular vesicle disease biomarkers. Arthritis Res Ther (2019) 21(1):181. doi: 10.1186/s13075-019-1961-4
50. Barros ER, Rigalli JP, Tapia-Castillo A, Vecchiola A, Young MJ, Hoenderop JGJ, et al. Proteomic profile of urinary extracellular vesicles identifies AGP1 as a potential biomarker of primary aldosteronism. Endocrinology (2021) 162(4): 1–11. doi: 10.1210/endocr/bqab032
51. Engstrom G, Stavenow L, Hedblad B, Lind P, Eriksson KF, Janzon L, et al. Inflammation-sensitive plasma proteins, diabetes, and mortality and incidence of myocardial infarction and stroke: a population-based study. Diabetes (2003) 52(2):442–7. doi: 10.2337/diabetes.52.2.442
52. Goodwin JE, Geller DS. Glucocorticoid-induced hypertension. Pediatr Nephrol (2012) 27(7):1059–66. doi: 10.1007/s00467-011-1928-4
53. Liu B, Zhang TN, Knight JK, Goodwin JE. The glucocorticoid receptor in cardiovascular health and disease. Cells (2019) 8(10)1227: 1–21. doi: 10.3390/cells8101227
54. Goodwin JE, Zhang J, Geller DS. A critical role for vascular smooth muscle in acute glucocorticoid-induced hypertension. J Am Soc Nephrol (2008) 19(7):1291–9. doi: 10.1681/ASN.2007080911
55. Oakley RH, Ren R, Cruz-Topete D, Bird GS, Myers PH, Boyle MC, et al. Essential role of stress hormone signaling in cardiomyocytes for the prevention of heart disease. Proc Natl Acad Sci U S A (2013) 110(42):17035–40. doi: 10.1073/pnas.1302546110
56. Langreck C, Wauson E, Nerland D, Lamb B, Folkerts T, Winter L, et al. Hippocampal mitogen-activated protein kinase phosphatase-1 regulates behavioral and systemic effects of chronic corticosterone administration. Biochem Pharmacol (2021) 190:114617. doi: 10.1016/j.bcp.2021.114617
57. Vizzardelli C, Pavelka N, Luchini A, Zanoni I, Bendickson L, Pelizzola M, et al. Effects of dexamethazone on LPS-induced activationand migration of mouse dendritic cells revealed by a genome-wide transcriptional analysis. Eur J Immunol (2006) 36(6):1504–15. doi: 10.1002/eji.200535488
58. Carvajal CA, Tapia-Castillo A, Fardella CE. Extending the endocrine hypertension spectrum: novel non-classic apparent mineralocorticoid excess. Endocrine (2021) 74(2):437–9. doi: 10.1007/s12020-021-02783-1
59. Tapia-Castillo A, Carvajal CA, Perez JA, Fardella CE. Clinical, biochemical, and miRNA profile of subjects with positive screening of primary aldosteronism and non-classic apparent mineralocorticoid excess. Endocrine (2022) 77(2):380–91. doi: 10.1007/s12020-022-03103-x
60. Tarjus A, Martinez-Martinez E, Amador C, Latouche C, El Moghrabi S, Berger T, et al. Neutrophil gelatinase-associated lipocalin, a novel mineralocorticoid biotarget, mediates vascular profibrotic effects of mineralocorticoids. Hypertension (2015) 66(1):158–66. doi: 10.1161/HYPERTENSIONAHA.115.05431
61. Kozlowski T, Rydzewska-Rosolowska A, Mysliwiec J, Choromanska B, Wojskowicz P, Dadan J, et al. The impact of laparoscopic adrenalectomy on renal function. results of a prospective randomised clinical trial. Endokrynol Pol (2019) 70(5):409–16. doi: 10.5603/EP.a2019.0029
62. Buonafine M, Martinez-Martinez E, Amador C, Gravez B, Ibarrola J, Fernandez-Celis A, et al. Neutrophil gelatinase-associated lipocalin from immune cells is mandatory for aldosterone-induced cardiac remodeling and inflammation. J Mol Cell Cardiol (2018) 115:32–8. doi: 10.1016/j.yjmcc.2017.12.011
63. Koroskenyi K, Juba F, Vajda G. Human vascular antigen complement consumption test of hypertensive patients (preliminary report). Experientia (1961) 17:91–2. doi: 10.1007/BF02171445
64. Ebringer A, Doyle AE. Raised serum IgG levels in hypertension. Br Med J (1970) 2(5702):146–8. doi: 10.1136/bmj.2.5702.146
65. Montecucco F, Pende A, Quercioli A, Mach F. Inflammation in the pathophysiology of essential hypertension. J Nephrol (2011) 24(1):23–34. doi: 10.5301/JN.2010.4729
66. White FN, Grollman A. Autoimmune factors associated with infarction of the kidney. Nephron (1964) 1:93–102. doi: 10.1159/000179322
67. Guzik TJ, Hoch NE, Brown KA, McCann LA, Rahman A, Dikalov S, et al. Role of the T cell in the genesis of angiotensin II induced hypertension and vascular dysfunction. J Exp Med (2007) 204(10):2449–60. doi: 10.1084/jem.20070657
68. Crowley SD, Frey CW, Gould SK, Griffiths R, Ruiz P, Burchette JL, et al. Stimulation of lymphocyte responses by angiotensin II promotes kidney injury in hypertension. Am J Physiol Renal Physiol (2008) 295(2):F515–24. doi: 10.1152/ajprenal.00527.2007
69. Trott DW, Thabet SR, Kirabo A, Saleh MA, Itani H, Norlander AE, et al. Oligoclonal CD8+ T cells play a critical role in the development of hypertension. Hypertension (2014) 64(5):1108–15. doi: 10.1161/HYPERTENSIONAHA.114.04147
70. Itani HA, McMaster WG Jr., Saleh MA, Nazarewicz RR, Mikolajczyk TP, Kaszuba AM, et al. Activation of human T cells in hypertension: Studies of humanized mice and hypertensive humans. Hypertension (2016) 68(1):123–32. doi: 10.1161/HYPERTENSIONAHA.116.07237
71. Wenzel P, Knorr M, Kossmann S, Stratmann J, Hausding M, Schuhmacher S, et al. Lysozyme m-positive monocytes mediate angiotensin II-induced arterial hypertension and vascular dysfunction. Circulation (2011) 124(12):1370–81. doi: 10.1161/CIRCULATIONAHA.111.034470
72. Amador CA, Barrientos V, Peña J, Herrada AA, González M, Valdés S, et al. Spironolactone decreases DOCA-salt-induced organ damage by blocking the activation of T helper 17 and the downregulation of regulatory T lymphocytes. Hypertension (2014) 63(4):797–803. doi: 10.1161/HYPERTENSIONAHA.113.02883
73. Hevia D, Araos P, Prado C, Fuentes Luppichini E, Rojas M, Alzamora R, et al. Myeloid CD11c+ antigen-presenting cells ablation prevents hypertension in response to angiotensin II plus high-salt diet. Hypertension (2018) 71(4):709–18. doi: 10.1161/HYPERTENSIONAHA.117.10145
74. Lu X, Rudemiller NP, Privratsky JR, Ren J, Wen Y, Griffiths R, et al. Classical dendritic cells mediate hypertension by promoting renal oxidative stress and fluid retention. Hypertension (2020) 75(1):131–8. doi: 10.1161/HYPERTENSIONAHA.119.13667
75. Mattson DL, Lund H, Guo C, Rudemiller N, Geurts AM, Jacob H. Genetic mutation of recombination activating gene 1 in Dahl salt-sensitive rats attenuates hypertension and renal damage. Am J Physiol Regul Integr Comp Physiol (2013) 304(6):R407–14. doi: 10.1152/ajpregu.00304.2012
76. Rudemiller N, Lund H, Jacob HJ, Geurts AM, Mattson DL, Program PK. CD247 modulates blood pressure by altering T-lymphocyte infiltration in the kidney. Hypertension (2014) 63(3):559–64. doi: 10.1161/HYPERTENSIONAHA.113.02191
77. Van Beusecum JP, Barbaro NR, McDowell Z, Aden LA, Xiao L, Pandey AK, et al. High salt activates CD11c(+) antigen-presenting cells via SGK (Serum glucocorticoid kinase) 1 to promote renal inflammation and salt-sensitive hypertension. Hypertension (2019) 74(3):555–63. doi: 10.1161/HYPERTENSIONAHA.119.12761
78. Ferguson JF, Aden LA, Barbaro NR, Van Beusecum JP, Xiao L, Simmons AJ, et al. High dietary salt-induced dendritic cell activation underlies microbial dysbiosis-associated hypertension. JCI Insight (2019) 5:e126241. doi: 10.1172/jci.insight.126241
79. Pitzer A, Elijovich F, Laffer CL, Ertuglu LA, Sahinoz M, Saleem M, et al. DC ENaC-dependent inflammasome activation contributes to salt-sensitive hypertension. Circ Res (2022); 131:328–344. doi: 10.1161/CIRCRESAHA.122.320818
80. Kirabo A, Fontana V, de Faria AP, Loperena R, Galindo CL, Wu J, et al. DC Isoketal-modified proteins activate T cells and promote hypertension. J Clin Invest (2014) 124(10):4642–56. doi: 10.1172/JCI74084
81. Xiao L, Patrick DM, Aden LA, Kirabo A. Mechanisms of isolevuglandin-protein adduct formation in inflammation and hypertension. Prostaglandins Other Lipid Mediat (2018) 139:48–53. doi: 10.1016/j.prostaglandins.2018.09.008
82. Dixon KB, Davies SS, Kirabo A. Dendritic cells and isolevuglandins in immunity, inflammation, and hypertension. Am J Physiol Heart Circ Physiol (2017) 312(3):H368–H74. doi: 10.1152/ajpheart.00603.2016
83. Barbaro NR, Foss JD, Kryshtal DO, Tsyba N, Kumaresan S, Xiao L, et al. Dendritic cell amiloride-sensitive channels mediate sodium-induced inflammation and hypertension. Cell Rep (2017) 21(4):1009–20. doi: 10.1016/j.celrep.2017.10.002
84. Ruggeri Barbaro N, Van Beusecum J, Xiao L, do Carmo L, Pitzer A, Loperena R, et al. Sodium activates human monocytes via the NADPH oxidase and isolevuglandin formation. Cardiovasc Res (2021) 117(5):1358–71. doi: 10.1093/cvr/cvaa207
85. Araos P, Figueroa S, Amador CA. The role of neutrophils in hypertension. Int J Mol Sci (2020) 21(22):8536. doi: 10.3390/ijms21228536
86. Libianto R, Hu J, Chee MR, Hoo J, Lim YY, Shen J, et al. A multicenter study of neutrophil-to-Lymphocyte ratio in primary aldosteronism. J Endocr Soc (2020) 4(12):bvaa153. doi: 10.1210/jendso/bvaa153
87. Walczak C, Gaignier F, Gilet A, Zou F, Thornton SN, Ropars A. Aldosterone increases VEGF-a production in human neutrophils through PI3K, ERK1/2 and p38 pathways. Biochim Biophys Acta (2011) 1813(12):2125–32. doi: 10.1016/j.bbamcr.2011.07.010
88. Gilet A, Zou F, Boumenir M, Frippiat JP, Thornton SN, Lacolley P, et al. Aldosterone upregulates MMP-9 and MMP-9/NGAL expression in human neutrophils through p38, ERK1/2 and PI3K pathways. Exp Cell Res (2015) 331(1):152–63. doi: 10.1016/j.yexcr.2014.11.004
89. Bergmann A, Eulenberg C, Wellner M, Rolle S, Luft F, Kettritz R. Aldosterone abrogates nuclear factor kappaB-mediated tumor necrosis factor alpha production in human neutrophils via the mineralocorticoid receptor. Hypertension (2010) 55(2):370–9. doi: 10.1161/HYPERTENSIONAHA.109.141309
90. Ko EA, Amiri F, Pandey NR, Javeshghani D, Leibovitz E, Touyz RM, et al. Resistance artery remodeling in deoxycorticosterone acetate-salt hypertension is dependent on vascular inflammation: evidence from m-CSF-deficient mice. Am J Physiol Heart Circ Physiol (2007) 292(4):H1789–95. doi: 10.1152/ajpheart.01118.2006
91. Rickard AJ, Morgan J, Tesch G, Funder JW, Fuller PJ, Young MJ. Deletion of mineralocorticoid receptors from macrophages protects against deoxycorticosterone/salt-induced cardiac fibrosis and increased blood pressure. Hypertension (2009) 54(3):537–43. doi: 10.1161/HYPERTENSIONAHA.109.131110
92. Shen JZ, Morgan J, Tesch GH, Rickard AJ, Chrissobolis S, Drummond GR, et al. Cardiac tissue injury and remodeling is dependent upon MR regulation of activation pathways in cardiac tissue macrophages. Endocrinology (2016) 157(8):3213–23. doi: 10.1210/en.2016-1040
93. Floderer M, Prchal-Murphy M, Vizzardelli C. Dendritic cell-secreted lipocalin2 induces CD8+ T-cell apoptosis, contributes to T-cell priming and leads to a TH1 phenotype. PloS One (2014) 9(7):e101881. doi: 10.1371/journal.pone.0101881
94. Hirahara K, Ghoreschi K, Laurence A, Yang XP, Kanno Y, O'Shea JJ. Signal transduction pathways and transcriptional regulation in Th17 cell differentiation. Cytokine Growth Factor Rev (2010) 21(6):425–34. doi: 10.1016/j.cytogfr.2010.10.006
95. Leopold JA. The central role of neutrophil gelatinase-associated lipocalin in cardiovascular fibrosis. Hypertension (2015) 66(1):20–2. doi: 10.1161/HYPERTENSIONAHA.115.05479
96. Schmidt T, Luebbe J, Kilian C, Riedel JH, Hiekmann S, Asada N, et al. IL-17 receptor c signaling controls CD4(+) TH17 immune responses and tissue injury in immune-mediated kidney diseases. J Am Soc Nephrol (2021) 32(12):3081–98. doi: 10.1681/ASN.2021030426
97. Orejudo M, Rodrigues-Diez RR, Rodrigues-Diez R, Garcia-Redondo A, Santos-Sanchez L, Randez-Garbayo J, et al. Interleukin 17A participates in renal inflammation associated to experimental and human hypertension. Front Pharmacol (2019) 10:1015. doi: 10.3389/fphar.2019.01015
98. Norlander AE, Saleh MA, Kamat NV, Ko B, Gnecco J, Zhu L, et al. Interleukin-17A regulates renal sodium transporters and renal injury in angiotensin II-induced hypertension. Hypertension (2016) 68(1):167–74. doi: 10.1161/HYPERTENSIONAHA.116.07493
99. Bonnard B, Ibarrola J, Lima-Posada I, Fernandez-Celis A, Durand M, Genty M, et al. Neutrophil gelatinase-associated lipocalin from macrophages plays a critical role in renal fibrosis Via the CCL5 (Chemokine ligand 5)-Th2 cells-IL4 (Interleukin 4) pathway. Hypertension (2022) 79(2):352–64. doi: 10.1161/HYPERTENSIONAHA.121.17712
100. Jang E, Lee S, Kim JH, Kim JH, Seo JW, Lee WH, et al. Secreted protein lipocalin-2 promotes microglial M1 polarization. FASEB J (2013) 27(3):1176–90. doi: 10.1096/fj.12-222257
101. Gordon S. Alternative activation of macrophages. Nat Rev Immunol (2003) 3(1):23–35. doi: 10.1038/nri978
102. Barish GD, Downes M, Alaynick WA, Yu RT, Ocampo CB, Bookout AL, et al. A nuclear receptor atlas: macrophage activation. Mol Endocrinol (2005) 19(10):2466–77. doi: 10.1210/me.2004-0529
103. Zhuang Y, Zhao X, Yuan B, Zeng Z, Chen Y. Blocking the CCL5-CCR5 axis using maraviroc promotes M1 polarization of macrophages cocultured with irradiated hepatoma cells. J Hepatocell Carcinoma (2021) 8:599–611. doi: 10.2147/JHC.S300165
104. Pawar RD, Pitashny M, Gindea S, Tieng AT, Levine B, Goilav B, et al. Neutrophil gelatinase-associated lipocalin is instrumental in the pathogenesis of antibody-mediated nephritis in mice. Arthritis Rheumatol (2012) 64(5):1620–31. doi: 10.1002/art.33485
105. Dekens DW, Naude PJ, Engelborghs S, Vermeiren Y, Van Dam D, Oude Voshaar RC, et al. Neutrophil gelatinase-associated lipocalin and its receptors in alzheimer's disease (AD) brain regions: Differential findings in AD with and without depression. J Alzheimers Dis (2017) 55(2):763–76. doi: 10.3233/JAD-160330
106. Langelueddecke C, Roussa E, Fenton RA, Thevenod F. Expression and function of the lipocalin-2 (24p3/NGAL) receptor in rodent and human intestinal epithelia. PloS One (2013) 8(8):e71586. doi: 10.1371/journal.pone.0071586
107. Langelueddecke C, Roussa E, Fenton RA, Wolff NA, Lee WK, Thevenod F. Lipocalin-2 (24p3/neutrophil gelatinase-associated lipocalin (NGAL)) receptor is expressed in distal nephron and mediates protein endocytosis. J Biol Chem (2012) 287(1):159–69. doi: 10.1074/jbc.M111.308296
Keywords: NGAL, endocrine hypertension, aldosterone, immune system, mineralocorticoid receptors
Citation: Araos P and Amador CA (2022) Neutrophil gelatinase–associated lipocalin as an immunomodulator in endocrine hypertension. Front. Endocrinol. 13:1006790. doi: 10.3389/fendo.2022.1006790
Received: 29 July 2022; Accepted: 04 October 2022;
Published: 25 October 2022.
Edited by:
Cristian A. Carvajal, Pontificia Universidad Católica de Chile, ChileReviewed by:
Xiaohan Lu, Duke University, United StatesMorag J. Young, Baker Heart and Diabetes Institute, Australia
David Patrick, Vanderbilt University, United States
Copyright © 2022 Araos and Amador. This is an open-access article distributed under the terms of the Creative Commons Attribution License (CC BY). The use, distribution or reproduction in other forums is permitted, provided the original author(s) and the copyright owner(s) are credited and that the original publication in this journal is cited, in accordance with accepted academic practice. No use, distribution or reproduction is permitted which does not comply with these terms.
*Correspondence: Cristián A. Amador, cristian.amador@uss.cl