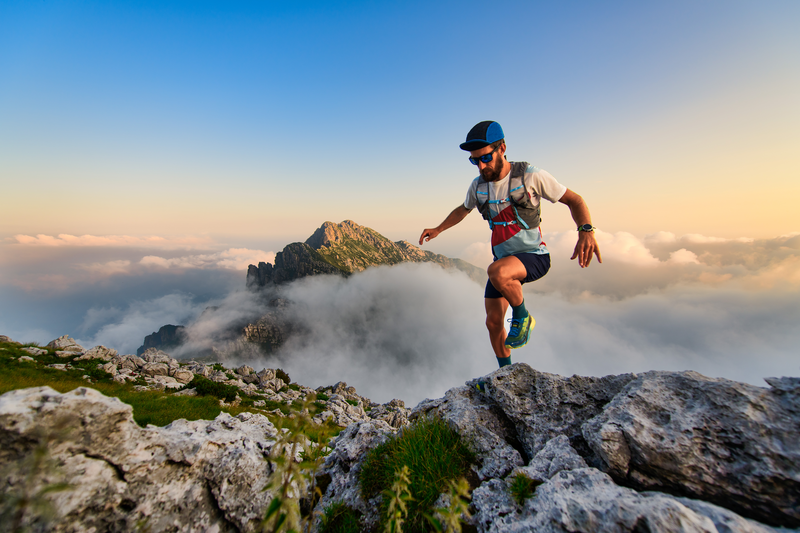
94% of researchers rate our articles as excellent or good
Learn more about the work of our research integrity team to safeguard the quality of each article we publish.
Find out more
REVIEW article
Front. Endocrinol. , 13 October 2022
Sec. Adrenal Endocrinology
Volume 13 - 2022 | https://doi.org/10.3389/fendo.2022.1001065
In recent years, vitamin D has become the protagonist in many studies. From cardiology to oncology the spotlight was on this vitamin. While in the past it was considered for its important role in phospho-calcium metabolism and skeletal disorders; today by studying it better, thousands of scenarios and facets have opened up on this vitamin which is actually a hormone in all respects. There are authoritative studies that demonstrate its activity in vitro and in vivo on: carcinogenesis, inflammation, autoimmunity and endocrinopathies. Its role has been studied in type 1 and type 2 diabetes mellitus, in Hashimoto or Graves’ thyroiditis and even in adrenal gland diseases. In fact, there are several studies that demonstrate the possible correlations between vitamin D and: Addison’s disease, Cushing disease, hyperaldosteronism or adrenocortical tumors. Moreover, this fascinating hormone and adrenal gland even seem to be deeply connected by common genetic pathways. This review aimed to analyze the works that have tried to study the possible influence of vitamin D on adrenal diseases. In this review we analyze the works that have tried to study the possible influence of vita-min D on adrenal disease.
Vitamin D has a fundamental role in mineral and bone metabolism: it promotes calcium absorption in the gut and maintains adequate serum calcium and phosphate concentrations to enable normal bone mineralization. It is also needed for bone growth and bone remodeling.
The production of vitamin D3 (cholecalciferol) in the skin is not an enzymatic process: cholecalciferol is a steroid hormone precursor synthesized in skin thanks to a photochemical reaction by ultraviolet B radiation of sunlight. Cholecalciferol is biologically inactive and needs two successive hydroxylation reactions for activation. The first hydroxylation occurs in the liver to form the 25-hydroxyvitamin D [25(OH)D or calcidiol]; the second occurs in the kidney to form 1,25-dihydroxyvitamin D, the biologically active vitamin, [1,25(OH)2D or calcitriol] this hydroxylation is promoted by the 1α-hydroxylase (CYP27B1), an enzyme which is dependent on parathyroid hormone (PTH). 25OHD and 1,25(OH)2D catabolism depends on CYP24A1 (1, 2) Figure 1.
Parathyroid hormone (PTH), calcium, phosphate and Fibroblast Growth Factor-23 (FGF-23) are the major regulators of the renal 1-hydroxylase (CYP27B1); regulation of the extra renal 1-hydroxylase is different from the kidney one and implies cytokines. Vitamin D and its metabolites are carried in the blood by albumin and vitamin D binding protein (DBP). Most, but not all actions of 1,25(OH)2D are mediated by the vitamin D receptor (VDR). VDR is a transcription factor that interacts with other transcription factors; calcitriol binds the nuclear VDR and regulates positively or negatively the transcription of several target genes depending on other cofactors to which it binds or relates. The VDR is found in most cells, not just those involved with bone and mineral homeostasis suggesting many actions of 1,25(OH)2D on different physiologic and pathologic processes (3, 4). The expression of 1α-hydroxylase and VDR in tissues involved in classical endocrine pathway, in breast, pancreas, colon, in malignant cells and immune cells, suggests that also other tissues, different from kidney, are able to synthesize the active form of vitamin D (5). So vitamin D has pleiotropic effects and may act in paracrine and autocrine manner in addition to its endocrine function. This suggests an important role of vitamin D in the pathogenesis and outcome of many common diseases, including endocrine diseases (6), chronic diseases (7) and cancer (8).
This review aimed to analyze the works that have tried to study the possible influence of vitamin D on adrenal diseases.
We searched the MEDLINE/PUBMED, Cochrane Library, ClinicalTrials.gov and SCOPUS databases up to 1975 to 2022 to identify all relevant English language medical literature for studies under the search text terms; Vitamin D AND (adrenal gland OR Cushing’s syndrome OR adrenal tumors OR primary aldosteronism OR primary adrenal insufficiency).
Across these databases, these search terms produced 942 results.
From these results, we excluded studies about adrenal medulla (74) and we excluded duplicates. We did a screen considering predominantly works that matched adrenal gland cortex and vitamin D (260). Among these works we select articles with greater rigor, we excluded case reports and articles not focus on the topic Figure 2.
Cushing’s syndrome (CS) is a rare endocrine disease characterized by high circulating levels of cortisol. There are two etiologies of CS: endogenous and exogenous. The most common cause of exogenous hypercortisolism is iatrogenic and depends on the prolonged use of glucocorticoids (GCs). Endogenous hypercortisolism depends on excessive production of cortisol by adrenal glands and it can be ACTH-dependent or ACTH-independent. ACTH-secreting pituitary adenomas (Cushing disease) and ectopic ACTH secretion by neoplasms are responsible for ACTH-dependent Cushing. Adrenal hyperplasia, adenoma and carcinoma are major causes of ACTH-independent Cushing syndrome. CS is causes severe morbidities and increases mortality, the most common complications are the cardiovascular ones. Signs and symptoms of CS are: severe fatigue, depression, anxiety, irritability, muscle weakness, cognitive difficulties, new or worsened high blood pressure, infections, skin darkening, bone loss, fractures, decreased sex drive, decreased fertility, erectile dysfunction, hirsutism, weight gain, “moon face”, “buffalo hump”, pink or purple stretch marks (striae) on the skin of the abdomen, thighs, breasts and arms, thinning, fragile skin that bruises easily, slow healing of cuts, infections, acne.
The relationship between CS and vitamin D is certainly the most complex and ambiguous one. The relationship between vitamin D and GCs has been extensively studied and the possibility that the use of GCs would alter the bioavailability of vitamin D has also been investigated (9, 10). The mechanism by which the GCs reduce the levels of 25 (OH) D seems indirect in fact GCs regulate the expression of the vitamin D receptor in many tissues and cells (11, 12). Studies in mice showed that use of dexamethasone was associated with a decrease in 1α-hydroxylase and an increase in 24-hydroxylase, which can lower the active form of calcitriol, thus decreasing the circulating levels of 25 (OH)D (13, 14).
The most important studies evaluating the Vitamin D and Cushing syndrome are summarized in Table 1.
Table 1 Main characteristics of the 4 studies included in the review on Cushing Syndrome and Vitamin D.
Between 70’s-80’s there was strong interest in glucocorticoids and vitamin D. Some works reported during GCs therapy, values of 1,25(OH)2D reduced, others increased, others unchanged (15, 18–20). The study by Seeman et al. in 1980 considered 14 patients with excess of endogenous or exogenous glucocorticoids. The dosage of vitamin D was performed both during euglucocorticoidism and during hyperglucocorticoidism; excess glucocorticoids was observed to cause small decreases in plasma concentrations of 25(OH)vitamin D (22 +/- 2-18 +/- 2 ng/ml; P <0.05) but no significant change in 1, 25-OHvitamin D (32 +/- 8- 23 +/- 6 pg/ml) (15). Another study by Kugai et al. (14) analyzed the impaired mineral metabolism and vitamin D metabolism in CS patients. In CS patients, plasma levels of 25OH vitamin D and 24,25(OH) vitamin D were lower than in normal subjects.
A recent study by Povalieva et al. evaluated vitamin D metabolism in patients with Cushing disease (CD) compared to healthy subjects receiving cholecalciferol bolus treatment. It studied: 30 adults with CD and 30 apparently healthy adults with similar age, gender and BMI. All participants received a single dose (150,000 IU) of oral cholecalciferol. The following data were determined: the serum metabolites of vitamin D, free 25 (OH) D, vitamin D binding protein (DBP) and PTH serum and urine biochemical parameters were performed prior to intake and on days 1, 3 and 7. The CD patients had lower baseline free 25 (OH) D levels (p <0.05) despite similar DBP levels (p>0.05) and lower albumin levels (p<0.05); 24-hour urinary free cortisol showed a significant correlation with the baseline 25 (OH) D/24.25 (OH) 2D ratio (r = 0.36, p <0.05). CD patients had a consistently higher 25(OH)D/24.25(OH) 2D ratio, which is indicative of a decrease in 24-hydroxylase activity. It was concluded that this altered activity of vitamin D catabolism could have influenced the effectiveness of treatment with cholecalciferol (16).
An even more recent study by Gurnotta et al. conducted on patients with CD is of considerable interest (17). The objectives of the study were to: 1. evaluate serum 25-OH- vitamin D in CD patients, compared to controls; 2. evaluate the response to a dose of 150,000 UI of cholecalciferol in patients with CD. We considered 50 patients with CD and 48 controls, in which the following data were evaluated: the anthropometric and biochemical parameters at baseline and after 6 weeks of therapy with cholecalciferol. At baseline, CD patients had a higher frequency of hypovitaminosis D deficiency (p=0.001) and lower serum 25(OH)D (p <0.001) than controls. Six weeks after cholecalciferol treatment, CD patients had increased serum calcium (p=0.017), 25 (OH) D (p<0.001), ISI-Matsuda (p=0.035), oral disposition index (p=0.045) and decrease in serum PTH (p = 0.004) and total cholesterol values (p=0.017) from baseline. Mean urinary free cortisol (mUFC) was negatively correlated independently with 25 (OH) D in CD. In conclusion, serum levels of 25 (OH) D were lower in patients with CD than in controls. Vitamin D deficiency is correlated with mUFC and mUFC values>240 nmol/24 h are associated with hypovitaminosis D. The supplementation of cholecalciferol had a positive impact on insulin sensitivity and lipids (17).
From this analysis, we can conclude that the data on CS and vitamin D are ambiguous and not unique. Some of these studies have been conducted on humans, others on animal models, but only a few studies have been conducted on subjects with endogenous hypercortisolism such as the study of Guarnotta et al. probably also this makes the reading of the data more ambiguous; the results obtained in Guarnotta’s study are very promising and open a window on future works and possible discoveries.
The term “adrenal tumor” describes benign and malignant mass lesions of the adrenal gland. The estimated incidence of adult adrenocortical carcinoma (ACC) is between 0.7 and 2.0 per million per year. ACC can occur at any age with a peak incidence between 40 and 60 years, women are more often affected (55-60%). ACC can be sporadic or part of familial cancer syndrome (Li-Fraumeni syndrome, Lynch syndrome, multiple endocrine neoplasia (MEN) 1 and familial adenomatous polyposis). Most of ACCs are sporadic. Although the molecular mechanisms of tumorigenesis in many of the hereditary syndromes are well known, the molecular pathogenesis of sporadic ACCs is not completely understood. ACC can present classically in three different ways: about one-third of patients present with symptoms of hormonal excess (hypercortisolism, hyperandrogenism or both), another third present with non-specific symptoms and the last third are diagnosed coincidentally when imaging studies are done for other medical conditions (21, 22).
In recent years there has been a lot of attention on the possible role of Vitamin D in oncology (23). Laboratory and animal studies have reported that vitamin D can inhibit carcinogenesis, slow tumor progression, including promotion of cell differentiation, inhibition of tumor cell proliferation; moreover, vitamin D would also seem to have anti-inflammatory, immunomodulatory, proapoptotic and antiangiogenic effects (24). The literature data are many and the results are really promising. Numerous studies have confirmed a significant benefit of vitamin D in reducing mortality (25, 26). In particular, several studies, reviews and meta-analyzes have shown a positive effect of vitamin D on breast (27), colon (28–30) and prostate cancer.
In the Table 2 we reported the most important studies about the possible effects of vitamin D on tumors of the adrenal gland.
Table 2 Main characteristics of the 4 studies included in the review on Adrenal Tumors and Vitamin D.
One of the fundamental studies on this topic was that by Pilon et al. (31). This study analyzed the role of the Vitamin D-VDR receptor axis in the growth of ACC, using the H295R human adrenocortical carcinoma cell line as a model. It was concluded that: slightly increased concentrations relative to physiological standards of 1,25(OH)2D resulted in a moderate anti-proliferative effect on H295R cells. The anti-proliferative effect was due to the arrest of the cell cycle in the G1 phase, without inducing apoptosis. VDR mRNA expression was lower in ACC than in benign adrenocortical lesions and VDR immunostaining was evident in benign lesions while it appeared weak in tumor tissues. These findings may suggest a protective role of VDR in the carcinogenesis of tumors of the adrenal cortex (31).
Another recent study by Rubin et al. aimed to evaluate the effects of mitotane (the only chemotherapeutic agent available for the treatment of ACC) and 1,25(OH)2D, singly or in combination, in an in vitro model with ACC H295R cells, and to elucidate the molecular events behind their effects involving the Wnt/beta-signaling of the chain. Multiple concentrations of mitotane and 1,25(OH)2D, were tested on H295R cells for 24–96 hours and the effects were analyzed by 3-(4,5-dimethylthiazol-2-yl)-2,5-diphenyltetrazolium bromide (MTT). A reduction in cell growth in a dose/time dependent manner was observed for both mitotane and 1,25(OH)2D. Furthermore, a combination of clinically subtherapeutic concentrations of mitotane with 1,25(OH)2D, had an additive anti-proliferative effect, thus showing an additive effect of mitotane and 1,25(OH)2D on inhibition growth of H295R ACC cells and suggested the presence of a functional link between the VDR and Wnt/beta-catenin pathways (33).
A paper by Pilon et al. aimed to analyze the methylation of GpG sites in the promoter of the VDR gene of a different and wider range of human adrenocortical tissues, comparing adrenocortical adenomas (ACA) with ACC tissues. The study considered: 3 normal adrenal and 23 adrenal cortical tumor samples (15 adenomas and 8 carcinomas). While recognizing the limitations of the study (low number of the sample analyzed) the results obtained are very interesting, in fact it is the first evidence of an association between the methylation of the promoter of the VDR gene and the reduced expression of VDR in the ACC. This suggests a potential role in the epigenetic inactivation of VDR in malignant adrenocortical tumorigenesis. The authors also set VDR promoter methylation as a possible target for pharmacological agents for the treatment of adrenal cancer in selected cases (32).
Starting from the conclusions of this paper, a very recent retrospective cross-sectional study by Bueno et al. enrolling pediatric patients with pediatric adrenocortical tumors (pACT) and studied VDR expression levels and methylation status in pACTs and their clinical and prognostic significance. It considered clinical and pathological features, mRNA (qPCR) and protein (immunohistochemistry) expression of VDR and methylation at the VDR level of adrenocortical tumor samples from 108 pediatric patients. Fourteen pediatric and 32 normal fetal and postnatal adrenal glands were used as controls. Tumors with high VDR methylation had lower mRNA levels and their patients had advanced disease and reduced disease-free survival. The study therefore concluded that VDR has a role in normal adrenocortical development and homeostasis, which is impaired during tumorigenesis. Hypermethylation and under-expression of VDR can be both predictive and prognostic biomarkers for pACT (34).
Studies in literature that investigate the correlation between vitamin D and tumors of the adrenal cortex, although few, seem to have a single direction, underlining the important role of VDR in carcinogenesis. The results are very promising; they should lay the basis for future clinical studies. It would be interesting to be able to investigate the effect of vitamin D reintegration in the prevention of these tumors or in the outcome in patients with ACC through longitudinal clinical studies.
The primary aldosteronism (PA) is the most common endocrine secondary form of hypertension, it represents the cause of the ≈5%-10% of hypertension in adults. Hyperaldosteronism can initially present as essential and refractory hypertension; it often goes undiagnosed and recent studies indicate that its prevalence may be at least 3-fold higher (35, 36). PA consists in a heterogeneous group of familial and sporadic disorders characterized by hypertension secondary to overproduction of aldosterone, low plasma renin activity, and low potassium levels. Symptoms can be: weakness, muscle spasms, temporary paralysis, tingling feelings and frequent urination. Sporadic PA is the most common form and it is caused by: Aldosterone Producing Adenoma (APA), unilateral adrenal hyperplasia (UAH) and bilateral adrenal hyperplasia (BAH) (37). PA is also linked to cardiovascular diseases and metabolic alterations (38). In literature, there are several in vitro-and-in vivo studies that suggest a connection between vitamin D and hyperaldosteronism.
The most important studies evaluating the Vitamin D and primary aldosteronism are summarized in Table 3.
The study by Li et al. shows that vitamin D works as a negative endocrine regulator of the renin-angiotensin system in animals. In the mice, in fact, with null vitamin D receptor (VDR-null) the expression of renin and angiotensin II has increased and it leads to hypertension, cardiac hypertrophy, and an increase in the intake of water. In wild-type mice, the inhibition of the synthesis of 1,25(OH)2D leads to an increase in the renin expression, while the injection of 1,25(OH)2D leads to renin suppression. Thus 1,25(OH)2D dramatically suppresses renin transcription thanks to a mechanism mediated by VDR in cellular cultivation (39). Other studies on animals show that VDR, linked to its ligand 1,25(OH)2D, negatively regulates the renin (39, 40).
Cross -sectional studies confirmed a connection between vitamin D metabolites and renin-angiotensin-aldosterone system (RAAS): 25(OH)D levels are inversely linked to plasma renin activity (PRA) as high circulating levels of angiotensin II (41). All these confirm that there’s more RAAS activation in case of vitamin D deficiency (52).
A study by Bi C et al. aimed at understanding endocrine mechanisms involved in APA formation, through the analysis of biological processes and signaling pathways related to APA. In this study 19 endocrine genes have been found that are involved in regulatory pathways but only VDR, POR, and RET were differentially expressed in APA specimens. Thus VDR would represent the most relevant transcription factor, and its target genes including CYP11B2 and KCNJ5 would play an important role in the endocrine mechanism of APA (46).
Moreover, a study by Lundqvist et al. showed as 1α,25-OHD2 plays a role in hormone production and in the expression of the steroidogenic enzymes that are crucial in the human adrenocortical cell line NCI-H295R; it has shown that cell treatment with 1α,25-OHD2 suppressed levels of corticosterone, aldosterone, DHEA, DHEA-sulphate and androstenedione in the culture medium (42).
Apart from molecular and in vitro studies, we analyzed clinical studies aimed at searching for a correlation between vitamin D and PA. The results of these studies are not unique. In fact, several studies reported that vitamin D levels were not different between patients with PA and essential hypertension (EH) (43, 44).
Moreover, Ceccoli et al. analyzed the picture of secondary hyperaldosteronism to the increase in the urinary excretion of calcium by comparing 116 patients with PA and 110 patients with EH. After medical or surgical treatment in 40 patients with PA, bone mineral density (BMD) significantly increased in the lumbar spine, femoral neck, and total hip; PTH levels were significantly reduced, and instead, 25OH vitamin D levels were unchanged before and after the treatment (25-OH vitamin D before treatment: 24 ± 16 ng/ml after treatment: 26 ± 12 ng/ml p value n.s.). In addition, there wasn’t a statistically significant difference between 25-OH vitamin D levels in both groups (PA ed EH) (47).
Another study by Salcuni et al. considered 188 people with adrenal incidentaloma, who were observed between November 2009 and October 2011. After confirmatory testing: 11 patients were diagnosed with PA and 15 patients were later taken as controls (nPA). Vitamin D levels were deficient in both groups, in PA group they were lower but not statistically significant. PA was associated with low bone mass, increased prevalence of osteoporosis, and vertebral fractures. So aldosterone excess lead not only to cardiovascular and renal injury but also to bone damage (45). Similarly, the study by Zhang et al. that recruited 142 patients with adrenal adenoma (84 with APA and 58 with non-functional adrenal adenoma (NFA) showed that vitamin D levels were low in both the two groups, and particularly in APA group the levels were slightly lower. Instead, the serum PTH levels were significantly higher in APA, thus PTH may be a promising auxiliary index for the clinical diagnosis of APA (49).
As we can see, the results are not clear and we should not forget the possibility, supported by many works, according to which vitamin D itself can play a role in EH pathogenesis: a great number of observational studies support a datum that vitamin D could have a protective effect against hypertension development (53).
A Mendelian randomization study of Vimaleswaran et al. has shown, in fact, a correlation between 25(OH)vitamin D concentration, blood pressure, and hypertension risk. The results of this study suggest that people who have genetic variation linked to low endogenous production of 25(OH)vitamin D have an increased risk of hypertension, it underlines the need for more randomized, controlled, and well-planned studies to assess the potential clinical benefits of vitamin D reintegration (54).
In literature, we have also found different authoritative studies that have found a link between vitamin D deficiency and hyperaldosteronism. For example, a randomized controlled study by Grübler MR performed on 188 patients with arterial hypertension and poor vitamin D levels, has shown that a supplement of this vitamin led to a significant reduction of plasmatic aldosterone compared to a control group with placebo (50). Other studies on patients with heart failure, to whom Vitamin D was administered, have shown a reduction in plasmatic aldosterone levels (55, 56). Moreover, the aim of the study by Petramala et al. was to evaluate the impact of an excess of aldosterone on mineral metabolism and BMD. Patients with PA had a higher plasmatic PTH, lower 25(OH)vitamin D serum levels, a higher prevalence of vitamin D deficiency, and a higher prevalence of osteopenia/osteoporosis compared to EH and controls (48).
A recent study by Ismail et al. (51) concluded that 70% of the patients with PA showed a significant deficiency in vitamin D. After a 3 months treatment with vitamin D determined: 1) a significant improvement of 25(OH) vitamin; 2) a significant decrease of aldosterone plasmatic concentration; 3) decrease of systolic blood pressure; 4) improvement of eGFR. Vitamin D treatment improved all these parameters, thus probably suggesting an interaction between vitamin D, aldosterone, and kidney function.
Ismail’s study certainly had some limits (for example the small sample size; observational, uncontrolled, nonblinded design; lack of a randomized design ensuring a balanced distribution of confounders between patients receiving vitamin D or a placebo), nevertheless, the obtained results are very interesting and promising, and they should be a starting point for new studies to investigate such a fascinating correlation between vitamin D, hyperaldosteronism and blood hypertension (57).
Primary Adrenal Insufficiency (PAI) is a rare but severe-threatening disease in children and adults; the diagnosis is frequently delayed. PAI is defined by the inability of the adrenal cortex to produce sufficient amounts of glucocorticoids, mineralocorticoids and adrenal androgen hormones. This condition was first described by Thomas Addison and for this reason it is also known as Addison’s disease (AD). It is a potentially critical condition due to the central role of these hormones in energy and fluid homeostasis. Cortisol deficiency results in a decrease in feedback to the hypothalamic-pituitary axis and subsequent enhanced stimulation of the adrenal cortex by elevated levels of plasma ACTH. Consequent to disruption of adrenal mineralocorticoid synthesis, renin release by the juxtaglomerular cells of the kidneys increases. The signs and symptoms of PAI are mainly based on the deficiency of gluco- and mineralocorticoids and they are: weakness, fatigue, hyponatremia, hyperkalemia, depression, anxiety, weight loss, abdominal pain, musculoskeletal pain, orthostatic hypotension, changes in blood count (anemia, eosinophilia, lymphocytosis), hypoglycemia, hyperpigmentation of the skin, loss of axillary and pubic hair (58). In adulthood, the most common etiologies include: autoimmune, hemorrhage, infiltrative disorders, metastases and infection, whereas congenital adrenal hyperplasia (usually due to 21‐hydroxylase deficiency) affects approximately 1:18000 infants and children (59). Although the pathogenesis of AD has not been made completely clear, a role of interaction between HLA aplotypes and environmental factors has been postulated: environmental factors seem to act as triggers in a context of genetic susceptibility (HLA), all of this leads to the destructive infiltration of the adrenal cortex by CD8-T lymphocytes and to the typical production of 21-OHase antibodies (60). The correlation between AD and vitamin D is really fascinating and can even be found at a genetic level: various genes responsible for metabolizing vitamin D and polymorphisms of the VDR gene are involved in the onset of AD (61, 62).
The most important studies evaluating the Vitamin D and Primary Adrenal Insufficiency are reported in Table 4.
Table 4 Main characteristics of the 10 studies included in the review on Primary Adrenal Insufficiency and Vitamin D.
The study of Pani et al. starts from the assumption that variants of the VDR were associated with type 1 diabetes and thyroidal autoimmunity and goes on to analyze the VDR polymorphisms in Addison’s disease. 95 patients and 220 controls were genotyped for VDR polymorphisms (Fok I, BsmI, Apa I e Taq I). The results demonstrated that the genotypes ‘ff’ e ‘tt’ were significantly more frequent in patients with PAI compared to the controls. The distribution of the genotype BsmI also differed significantly between the patients and the controls. It was therefore concluded that the VDR genotype was linked to Addison’s disease (61).
The study by Lopez et al. analyzed two polymorphisms with a single nucleotide in the hydroxylasis gene CYP27B1 for a link with AD, Hashimoto’s thyroiditis, Graves’ disease and type 1 mellitus diabetes. CYP27B1 hydroxylasis catalyzes the conversion of the 25OHD3 in 1,25(OH)2D3, that plays a role in immunity regulation and cell proliferation. One hundred twenty-four patients with AD were taken into consideration. A significant link has been found between allelic variation of the promotor (21260) C/apolimorphism and AD, concluding therefore that the polymorphism C/A of the promotor CYP27B1 (21260) seems to be linked to endocrinal autoimmune diseases. These results suggest a regulatory difference of the CYP27B1 hydroxylasis to predispose endocrinal autoimmunity (63). Moreover, this link was also later confirmed by the study by Ficna M et al. in 2010 (62)
Successively, Ramagopalan et al. studied the potential role of hypovitaminosis D in influencing the pathogenesis of immuno-mediated diseases (65). The authors observed that in the patients with hypovitaminosis D there were significantly higher levels of AD and other autoimmune diseases.
Korwutthikulrangsri et al. (66), studying the interrelationship between vitamin D deficiency and PAI in children in critical conditions, observed that about 50% of the children on entering intensive care showed a total concentration of 25(OH)D in the blood inferior to 50 nmol/L. Furthermore this condition of deficiency was correlated to the gravity of the illness, multiorgan dysfunction and increased mortality. Instead, no link was found between the 25(OH)D serum and adrenal function. Moreover, a study by McNally et al (68), conducted on 319 children in six Canadian pediatric intensive care units evaluated the potential relationship between vitamin D levels, the adrenal state and cardiovascular dysfunction linked to PAI. No link whatsoever was found between the state of the vitamin D and PAI. However, the cardiovascular dysfunction linked to PAI seemed to be influenced by the state of the vitamin D; in fact, the PAI in patients with vitamin D deficiency was linked to significantly higher risk of cardio vascular dysfunction. Another study by Pazderska et al. later demonstrated that people born in winter ran a greater risk of suffering from AD. These results suggested therefore that hypovitaminosis D, together with the exposition to seasonal viral infections, could have deregulated their innate immunity, increasing the risk of the insurgence of AD (67).
The study by Martinez M et al. represents the first randomized crossover study which investigates the integration of cholecalciferol in patients with AD. The work included 13 patients with AD who received reintegration with cholecalciferol (4000 UI/day) for 3 months. The following were monitored: the plasmatic levels of 25-OHD3 and the immunitary cells, and monocytes. The exploratory analysis included the correlation of the changes with the genic polymorphs correlated to the vitamin D and the antibody titles of the 21- hydroxylasis (69). The median concentrations of 25(OH)D3 were significantly increased after 3 months of treatment with cholecalciferol. Within the T cells, only the tardily activated T cells and the Tc cells activated tardily had diminished, while the monocytes had increased after the therapy with vitamin D. The changes in the T lymphocytes were linked to two polymorphisms (CYP27B1-rs108770012 and VDR-rs10735810); no changes were observed in the antibody titles of the 21-hydroxylasis. It was concluded that in 3 months of treatment with cholecalciferol the patients: 1. reached sufficient levels of 25(OH)D3; 2. this reintegration led to the regulation of the tardy activation of the T lymphocytes and of the monocytes in patients with AD. The esplorative analysis revealed potential genetic contributions. This pilot study provides new information about the immunomodulation of vitamin D in AD.
Another recent study by Zawadzka et al. (70) retrospectively analyzed medical records of 31 adult patients with the diagnosis of autoimmune AD and demonstrate a high incidence of vitamin D deficiency and a significant correlation between low levels of vitamin D and severe fatigue as well as limited exercise capacity in AD patients.
It can therefore be concluded that the data from the studies carried out up to now would suggest that hypovitaminosis D and AD could be correlated; this correlation would also be endorsed by the potential role of vitamin D in modulating the immune response and by the autoimmune nature of AD. New longitudinal clinical studies could further confirm this data and maybe give us further news on the question of a possible use of vitamin D in the prevention or as an adjuvant therapy in this illness as in other immune-based pathologies.
To sum up the studies carried out so far on the link between vitamin D status and adrenal gland diseases, although relatively scarce and heterogeneous, allow us to formulate the following considerations.
a. In patients with Cushing’s disease, levels of 25OHD are lower than in control subjects and appear to be negatively correlated with urinary cortisol levels.
b. Reduced levels of vitamin D and under-expression of VDR seem to have a role in tumors of the adrenal cortex.
c. Several studies have confirmed that a vitamin D deficiency is associated with an activation of the RAAS system; furthermore, some data from the literature have suggested that the administration of vitamin D may modulate hyperaldosteronism and the consequent hypertension.
d. Vitamin D due to its ability to modulate the immune response could play a protective role in the pathogenesis of an autoimmune disease such as Addison’s disease
Today the view that hormones have only one function is outdated. Thanks to thousands of molecular, biochemical and clinical studies, it has been observed that there is a correlation between hormones and cardio-vascular risk, autoimmunity, inflammation.
Vitamin D has been a surprising discovery in recent years and its many functions are even more surprising. The studies analyzed in this work are promising: they support a complex network of interactions between adrenal hormones and vitamin D, with implications in prevention and health. So after this long journey through clinical studies, hormones and biochemistry, let’s sum it up and go back to our original question: adrenal gland and vitamin D, myth or reality?
We have evidence that this link is more than just a myth. The topic is vast and fascinating, the potential is great: there would be the possibility of using this vitamin for therapeutic or even preventive purposes in adrenal diseases. Evidences seem to support a role of vitamin D deficiency in the adrenal diseases onset but nowadays they are limited and not conclusive, thus not allowing to formulate diagnostic and therapeutic conclusions for each of these pathologies.
Evidence on potential efficacy of vitamin D administration in clinical practice for patients with adrenal diseases is currently lacking, we have not confirmed results about quantity and duration of vitamin D supplementation or how vitamin D could change the development, the course and the prognosis of the adrenal pathologies examined.
It would be very interesting to deepen these clinical implications with new future studies and works.
AA and CC conceived the idea, AA, LB and MD drafted the manuscript, SG and CC supervised the process and contributed to editing. All authors contributed to the article and approved the submitted version.
The authors declare that the research was conducted in the absence of any commercial or financial relationships that could be construed as a potential conflict of interest.
All claims expressed in this article are solely those of the authors and do not necessarily represent those of their affiliated organizations, or those of the publisher, the editors and the reviewers. Any product that may be evaluated in this article, or claim that may be made by its manufacturer, is not guaranteed or endorsed by the publisher.
1. Morris HA, Anderson PH. Autocrine and paracrine actions of vitamin d. Clin Biochem Rev (2010) 31(4):129–38.
2. Chauss D, Freiwald T, McGregor R, Yan B, Wang L, Nova-Lamperti E, et al. Autocrine vitamin d signaling switches off pro-inflammatory programs of TH1 cells. Nat Immunol (2022) 23:62–74. doi: 10.1038/s41590-021-01080-3
3. Aranow C. Vitamin d, and the immune system. J Investig Med (2011) 59(6):881–6. doi: 10.2310/JIM.0b013e31821b8755
4. Bikle DD. Vitamin d, metabolism, mechanism of action, and clinical applications. Chem Biol (2014) 21(3):319–29. doi: 10.1016/j.chembiol.2013.12.016
5. Battault S, Whiting SJ, Peltier SL, Sadrin S, Gerber G, Maixent JM. Vitamin d metabolism, functions, and needs: from science to health claims. Eur J Nutr (2013) 52(2):429–41. doi: 10.1007/s00394-012-0430-5
6. Altieri B, Muscogiuri G, Barrea L, Mathieu C, Vallone CV, Mascitelli L, et al. Does vitamin d play a role in autoimmune endocrine disorders? a proof of concept. Rev Endocr Metab Disord (2017) 18(3):335–46. doi: 10.1007/s11154-016-9405-9
7. Muscogiuri G, Altieri B, Annweiler C, Balercia G, Pal HB, Boucher BJ, et al. Vitamin d and chronic diseases: the current state of the art. Arch Toxicol (2017) 91(1):97–107. doi: 10.1007/s00204-016-1804-x
8. Moukayed M, Grant WB. Molecular link between vitamin d and cancer prevention. Nutrients (2013) 5(10):3993–4021. doi: 10.3390/nu5103993
9. Davidson ZE, Walker KZ, Truby H. Clinical review: Do glucocorticosteroids alter vitamin d status? a systematic review with meta-analyses of observational studies. J Clin Endocrinol Metab (2012) 97:738–44. doi: 10.1210/jc.2011-2757
10. Skversky A, Kumar J, Abramowitz M, Kaskel F, Melamed M. Association of glucocor-ticoid use and low 25- hydroxyvitamin d levels: Results from the national health and nutrition examination survey (NHANES): 2001–2006. J Clin Endocrinol Metab (2011) 96:3838–45. doi: 10.1210/jc.2011-1600
11. Hidalgo A, Trump D, Johnson C. Glucocorticoid regulation of the vitamin d receptor. J Steroid Biochem Mol Biol (2010) 121:372–5. doi: 10.1016/j.jsbmb.2010.03.081
12. Hidalgo A, Deeb K, Pike J, Johnson C, Trump D. Dexamethasone enhances 1α,25 dihydroxyvitamin D3 effects by increasing vitamin d receptor transcription. J Biol Chem (2011) 286:36228–37. doi: 10.1074/jbc.M111.244061
13. Favus MJ, Kimberg DV, Millar GN, Gershon E. Effects of cortisone administration on the metabolism and localization of 25-hydroxycholecalciferol in the rat. J Clin Investig (1973) 52:1328–35. doi: 10.1172/JCI107304
14. Kugai N, Koide Y, Yamashita K, Shimauchi T, Nagata N, Takatani O. Impaired mineral metabolism in cushing’s syndrome:Parathyroid function, vitamin d metabolites and osteopenia. Endocrinol Jpn (1986) 33:345–52. doi: 10.1507/endocrj1954.33.345
15. Seeman E, Kumar R, Hunder GG, Scott M, Heath H 3rd, Riggs BL. Production, degradation, and circulating levels of 1,25- dihydroxyvitamin d in health and in chronic glucocorticoid excess. J Clin Invest (1980) 66:664–9. doi: 10.1172/JCI109902
16. Povaliaeva A, Bogdanov V, Pigarova E, Zhukov A, Dzeranova L, Belaya Z, et al. Assessment of vitamin d metabolism in patients with cushing's disease in response to 150,000 IU cholecalciferol treatment. Nutrients (2021) 13(12):4329. doi: 10.3390/nu1312432913
17. Guarnotta V, Di Gaudio F, Giordano C. Vitamin d deficiency in cushing’s disease: Before and after its supplementation. Nutrients (2022) 14(5):973. doi: 10.3390/nu1405097314
18. Hahn TJ, Halstead LR and Haddad JG. Serum 25-hydroxyvitamin d concentration in patients receiving chronic corticosteroid therapy. J Lab Clin Med (1977) 90:399–404.
19. Slovik DM, Ohman RM, Lowell FC, Clark MB, Segre GM and Potts JT. Parathyroid hormone and 25-hydroxy vitamin d levels in glucocorticoid treated patients. Clin Endocrinol (1980) 12:243–8. doi: 10.1111/j.1365-2265.1980.tb02706.x
20. Aloia JF, Roginsky M, Ellis K, Shukla K, Cohn S. Skeletal metabolism and body composition in cushing's syndrome. J Clin Endocrinol Metab (1974) 39:981–5. doi: 10.1210/jcem-39-6-981
21. Torti JF, Correa R. Adrenal cancer. 2021. In: StatPearls. Treasure Island (FL: StatPearls Publishing (2022).
22. Fassnacht M, Dekkers OM, Else T, Baudin E, Berruti A, de Krijger R, et al. European Society of endocrinology clinical practice guide-lines on the management of adrenocortical carcinoma in adults, in collaboration with the European network for the study of adrenal tumors. Eur J Endocrinol (2018) 179(4):G1–G46. doi: 10.1530/EJE-18-0608
23. Vuolo L, Di Somma C, Faggiano A, Colao A. Vitamin d and cancer. Front Endocrinol (Lausanne) (2012) 3:58. doi: 10.3389/fendo.2012.00058
24. Manson JE, Cook NR, Lee IM, Christen W, Bassuk SS, Mora S, et al. Vitamin d supplements and prevention of cancer and cardiovascular disease. N Engl J Med (2019) 380(1):33–44. doi: 10.1056/NEJMoa1809944
25. Keum N, Lee DH, Greenwood DC, Manson JE, Giovannucci E. Vitamin d supplementation and total cancer incidence and mortality: a meta-analysis of randomized controlled trials. Ann Oncol (2019) 30(5):733–43. doi: 10.1093/annonc/mdz059
26. Zhang Y, Fang F, Tang J, Jia L, Feng Y, Xu P, et al. Association between vitamin d supplementation and mortality: systematic review and meta-analysis. BMJ (2019) 366:l4673. doi: 10.1136/bmj.l4673
27. Hossain S, Beydoun MA, Beydoun HA, Chen X, Zonderman AB, Wood RJ. Vitamin d and breast cancer: A systematic review and meta-analysis of observational studies. Clin Nutr ESPEN (2019) 30:170–84. doi: 10.1016/j.clnesp.2018.12.085
28. Klampfer L. Vitamin d and colon cancer. World J Gastroint Oncol (2014) 6(11):430–7. doi: 10.4251/wjgo.v6.i11.430
29. Bao Y, Li Y, Gong Y, Huang Q, Cai S, Peng J, et al. Vitamin d status and survival in stage II-III colorectal cancer. Front Oncol (2020) 17. doi: 10.3389/fonc.2020.581597
30. Ferrer-Mayorga G, Larriba MJ, Crespo P, Muñoz A. Mechanisms of action of vitamin d in colon cancer. J Steroid Biochem Mol Biol (2019) 185:1–6. doi: 10.1016/j.jsbmb.2018.07.002
31. Pilon C, Urbanet R, Williams TA, Maekawa T, Vettore S, Sirianni R, et al. 1α,25-dihydroxyvitamin D3 inhibits the human H295R cell proliferation by cell cycle arrest: a model for a protective role of vitamin d receptor against adrenocortical cancer. J Steroid Biochem Mol Biol (2014) 140:26–33. doi: 10.1016/j.jsbmb.2013.11.008
32. Pilon C, Rebellato A, Urbanet R, Guzzardo V, Cappellesso R, Sasano H, et al. Methylation status of vitamin d receptor gene promoter in benign and malignant adrenal tumors. Int J Endocrinol (2015) 2015:375349. doi: 10.1155/2015/375349
33. Rubin B, Pilon C, Pezzani R, Rebellato A, Fallo F. The effects of mitotane and 1α,25-dihydroxyvitamin D3 on wnt/beta-catenin signaling in human adrenocortical carcinoma cells. J Endocrinol Invest (2020) 43(3):357–67. doi: 10.1007/s40618-019-01127-1
34. Bueno AC, Stecchini MF, Marrero-Gutiérrez J, More CB, Leal LF, Gomes DC, et al. Vitamin d receptor hypermethylation as a biomarker for pediatric adrenocortical tumors. Eur J Endocrinol (2022) 186(5):573–85. doi: 10.1530/EJE-21-0879
35. Funder JW, Carey RM. Primary aldosteronism: Where are we now? where to from here? Hypertension (2022) 79(4):726–35. doi: 10.1161/HYPERTENSIONAHA.121.18761
36. Stowasser M. Update in primary aldosteronism. J Clin Endocrinol Meta (2009) 94(10):3623–30. doi: 10.1210/jc.2009-1399
37. Rossi GP, Bisogni V, Bacca AV, Belfiore A, Cesari M, Concistrè A, et al. The 2020 Italian society of arterial hypertension (SIIA) practical guidelines for the management of primary aldosteronism. Int J Cardiol Hypertens (2020) 5:100029. doi: 10.1016/j.ijchy.2020.100029
38. Wu X, Yu J, Tian H. Cardiovascular risk in primary aldosteronism: A systematic review and meta-analysis. Med (Baltimore) (2019) 98(26):e15985. doi: 10.1097/MD.0000000000015985
39. Li YC, Kong J, Wei M, Chen ZF, Liu SQ, Cao LP. 1,25-dihydroxyvitamin D(3) is a negative endocrine regulator of the renin-angiotensin system. J Clin Invest (2002) 110(2):229–38. doi: 10.1172/JCI15219
40. Yuan W, Pan W, Kong J, Zheng W, Szeto FL, Wong KE, et al. 1,25-dihydroxyvitamin D3 suppresses renin gene transcription by blocking the activity of the cyclic AMP response element in the renin gene promoter. J Biol Chem (2007) 282(41):29821–30. doi: 10.1074/jbc.M705495200
41. Forman JP, Williams JS, Fisher ND. Plasma 25-hydroxyvitamin d and regulation of the renin-angiotensin system in humans. Hypertension (2010) 55:1283–8. doi: 10.1161/HYPERTENSIONAHA.109.148619
42. Lundqvist J, Norlin M, Wikvall K. 1alpha,25-dihydroxyvitamin D3 affects hormone production and expression of steroidogenic enzymes in human adrenocortical NCI-H295R cells. Biochim Biophys Acta (2010) 1801(9):1056–62. doi: 10.1016/j.bbalip.2010.04.009
43. Pilz S, Kienreich K, Drechsler C, Ritz E, Fahrleitner-Pammer A, Gaksch M, et al. Hyperparathyroidism in patients with primary aldosteronism: cross-sectional and interventional data from the GECOH study. J Clin Endocrinol Metab (2012) 97:E75–9. doi: 10.1210/jc.2011-2183
44. Maniero C, Fassina A, Seccia TM, Toniato A, Iacobone M, Plebani M, et al. Mild hyperparathyroidism: a novel surgically correctable feature of primary aldosteronism. J Hypertens (2012) 30:390–5. doi: 10.1097/hjh.0b013e32834f0451
45. Salcuni AS, Palmieri S, Carnevale V, Morelli V, Battista C, Guarnieri V, et al. Bone involvement in aldosteronism. J Bone Miner Res (2012) 27(10):2217–22. doi: 10.1002/jbmr.1660
46. Bi C, Li B, Du L, Wang L, Zhang Y, Cheng Z, et al. Vitamin d receptor, an important transcription factor associated with aldosterone-producing adenoma. PloS One (2013) 8(12):e82309. doi: 10.1371/journal.pone.0082309
47. Ceccoli L, Ronconi V, Giovannini L, Marcheggiani M, Turchi F, Boscaro M, et al. Bone health and aldosterone excess. Osteop Int (2013) 24:2801–7. doi: 10.1007/s00198-013-2399-1
48. Petramala L, Zinnamosca L, Settevendemmie A, Marinelli C, Nardi M, Concistrè A, et al. Bone and mineral metabolism in patients with primary aldosteronism. Int J Endocrinol (2014) 2014:836529. doi: 10.1155/2014/836529
49. Zhang LX, Gu WJ, Li YJ, Wang Y, Wang WB, Wang AP, et al. PTH is a promising auxiliary index for the clinical diagnosis of aldosterone-producing adenoma. Am J Hypertens (2016) 29(5):575–81. doi: 10.1093/ajh/hpv146
50. Grübler MR, Gaksch M, Kienreich K, Verheyen N, Schmid JÓ Hartaigh BW, Richtig G, et al. Effects of vitamin d supplementation on plasma aldosterone and renin-a randomized placebo- controlled trial. J Clin Hypertens (2016) 18(7):608–13. doi: 10.1111/jch.12825
51. Ismail NA, Kamaruddin NA, Azhar Shah S, Sukor N. The effect of vitamin d treatment on clinical and biochemical outcomes of primary aldosteronism. Clin Endocrinol (Oxf) (2020) 92(6):509–17. doi: 10.1111/cen.14177
52. Brown JM, Vaidya A. Interactions between adrenal-regulatory and calcium-regulatory hormones in human health. Curr Opin Endocrinol Diabetes Obes (2014) 21:193–201. doi: 10.1097/MED.0000000000000062
53. Latic N, Erben RG. Vitamin d and cardiovascular disease, with emphasis on hypertension, atherosclerosis, and heart failure. Int J Mol Sci (2020) 21(18):6483. doi: 10.3390/ijms21186483
54. Vimaleswaran KS, Cavadino A, Berry DJ, LifeLines Cohort Study investigators, Jorde R, Dieffenbach AK, et al. Association of vitamin d status with arterial blood pressure and hypertension risk: a mendelian randomisation study. Lancet Diabe Endocrinol (2014) 2(9):719–29. doi: 10.1016/S2213-8587(14)70113-5
55. Boxer RS, Hoit BD, Schmotzer BJ, Stefano GT, Gomes A, Negrea L. The effect of vitamin d on aldosterone and health status in patients with heart failure. J Card Fail (2014) 20(5):334–42. doi: 10.1016/j.cardfail.2014.01.019
56. Zittermann A, Ernst JB, Prokop S, Fuchs U, Dreier J, Kuhn J, et al. Effects of vitamin d supplementation on renin and aldosterone concentrations in patients with advanced heart failure: The EVITA trial. Int J Endocrinol (2018) 2018:5015417. doi: 10.1155/2018/5015417
57. Rossi GP, Lenzini L. Vitamin d supplementation: a novel therapy for aldosteronism? Nat Rev Endocrinol (2020) 16(6):303–4. doi: 10.1038/s41574-020-0359-3
58. Bornstein SR, Allolio B, Arlt W, Barthel A, Don-Wauchope A, Hammer GD, et al. Diagnosis and treatment of primary adrenal insufficiency: An endocrine society clinical practice guideline. J Clin Endocrinol Metab (2016) 101(2):364–89. doi: 10.1210/jc.2015-1710
59. Flück CE. Mechanisms in endocrinology: Update on pathogenesis of primary adrenal insufficiency: beyond steroid enzyme deficiency and autoimmune adrenal destruction. Eur J Endocrinol (2017) 177(3):R99–R111. doi: 10.1530/EJE-17-0128
60. Kraus AU, Penna-Martinez M, Meyer G, Badenhoop K. Vitamin d effects on monocytes' CCL-2, IL6 and CD14 transcription in addison's disease and HLA susceptibility. J Steroid Biochem Mol Biol (2018) 177:53–8. doi: 10.1016/j.jsbmb.2017.07.026
61. Pani MA, Seissler J, Usadel KH, Badenhoop K. Vitamin d receptor genotype is associated with addison's disease. Eur J Endocrinol (2002) 147(5):635–40. doi: 10.1530/eje.0.1470635
62. Fichna M, Zurawek M, Januszkiewicz-Lewandowska D, Gryczyñska M, Fichna P, Sowiñski J, et al. Association of the CYP27B1 c(-1260)A polymorphism with autoimmune addison's disease. Exp Clin Endocrinol Diabetes (2010) 118(8):544–9. doi: 10.1055/s-0029-1241206
63. Lopez ER, Zwermann O, Segni M, Meyer G, Reincke M, Seissler J, et al. A promoter polymorphism of the CYP27B1 gene is associated with addison's disease, hashimoto's thyroiditis, graves' disease and type 1 diabetes mellitus in germans. Eur J Endocrinol (2004) 151(2):193–7. doi: 10.1530/eje.0.1510193
64. Jennings CE, Owen CJ, Wilson V, Pearce SH. A haplotype of the CYP27B1 promoter is associated with autoimmune addison's disease but not with graves' disease in a UK population. J Mol Endocrinol (2005) 34:859–63. doi: 10.1677/jme.1.01760
65. Ramagopalan SV, Goldacre R, Disanto G, Giovannoni G, Goldacre MJ. Hospital admissions for vitamin d related conditions and subsequent immune-mediated disease: record-linkage studies. BMC Med (2013) 11:171. doi: 10.1186/1741-7015-11-171
66. Korwutthikulrangsri M, Mahachoklertwattana P, Lertbunrian R, Chailurkit LO, Poomthavorn P. Vitamin d deficiency and adrenal function in critically ill children. J Med Assoc Thai (2015) 98(4):365–72.
67. Pazderska A, Fichna M, Mitchell AL, Napier CM, Gan E, Ruchała M, et al. Impact of month of birth on the risk of development of autoimmune addison's disease. J Clin Endocrinol Metab (2016) 101(11):4214–8. doi: 10.1210/jc.2016-2392
68. McNally JD, Doherty DR, Lawson ML, Al-Dirbashi OY, Chakraborty P, Ramsay T, et al. The relationship between vitamin d status and adrenal insufficiency in critically ill children. J Clin Endocrinol Metab (2013) 98(5):E877–81. doi: 10.1210/jc.2013-1126
69. Penna-Martinez M, Filmann N, Bogdanou D, Shoghi F, Huenecke S, Schubert R, et al. High-dose vitamin d in addison's disease regulates T-cells and monocytes: A pilot trial. Nutrition (2018) 49:66–73. doi: 10.1016/j.nut.2017.10.021
Keywords: vitamin D, VDR polymorphisms, adrenal gland, Cushing’s syndrome, adrenal tumor, hyperaldosteronism, primary adrenal insufficiency
Citation: Al Refaie A, Baldassini L, De Vita M, Gonnelli S and Caffarelli C (2022) Vitamin D and adrenal gland: Myth or reality? A systematic review. Front. Endocrinol. 13:1001065. doi: 10.3389/fendo.2022.1001065
Received: 22 July 2022; Accepted: 28 September 2022;
Published: 13 October 2022.
Edited by:
Federica Saponaro, University of Pisa, ItalyReviewed by:
Elena Castellano, AO Santa Croce e Carle, ItalyCopyright © 2022 Al Refaie, Baldassini, De Vita, Gonnelli and Caffarelli. This is an open-access article distributed under the terms of the Creative Commons Attribution License (CC BY). The use, distribution or reproduction in other forums is permitted, provided the original author(s) and the copyright owner(s) are credited and that the original publication in this journal is cited, in accordance with accepted academic practice. No use, distribution or reproduction is permitted which does not comply with these terms.
*Correspondence: Carla Caffarelli, Y2FybGFjYWZmYXJlbGxpQHlhaG9vLml0
Disclaimer: All claims expressed in this article are solely those of the authors and do not necessarily represent those of their affiliated organizations, or those of the publisher, the editors and the reviewers. Any product that may be evaluated in this article or claim that may be made by its manufacturer is not guaranteed or endorsed by the publisher.
Research integrity at Frontiers
Learn more about the work of our research integrity team to safeguard the quality of each article we publish.