- Department of Urology, Ruijin Hospital, Shanghai Jiao Tong University School of Medicine, Shanghai, China
Adrenocortical carcinoma (ACC) is a rare endocrine malignancy with frequent metastatic spread and poor prognosis. The disease can occur at any age with unexpected biological behavior. Recent genome-wide studies of ACC have contributed to our understanding of the disease, but diagnosis of ACC remains a challenge, even for multidisciplinary expert teams. Patients with ACC are frequently diagnosed in advanced stages and have limited therapeutic options. Therefore, for earlier diagnosis and better clinical management of adrenocortical carcinoma, specific, sensitive, and minimal invasive markers are urgently needed. Over several decades, great efforts have been made in discovering novel and reliable diagnostic and prognostic biomarkers including microRNAs, steroid profilings, circulating tumor cells, circulating tumor DNAs and radiomics. In this review, we will summarize these novel noninvasive biomarkers and analyze their values for diagnosis, predicting prognosis, and disease monitoring. Current problems and possible future application of these non-invasive biomarkers will also be discussed.
Introduction
Adrenal tumors are common diseases, affecting 3~10% human population (1–3). The majority of adrenal tumors are small benign nonfunctional adrenocortical adenomas, and a small fraction of adrenal tumors are adrenocortical carcinomas (1, 2). Adrenocortical carcinoma is a rare malignant tumor with an annual incidence of 0.7-2 cases per million individuals (4–6). It occurs at any age, with two peak incidences: the first one in childhood, mainly derived from hereditary syndromes such as the Li-Fraumeni and Beckwith-Wiedemann syndrome, and the second one between 40 and 60 years of age (3, 4, 7). Women are more frequently affected (55%-60%) than men (8, 9).
ACCs often show aggressive biological behavior, and 50%-70% of patients with ACC have symptoms and signs of hormone excess. Two thirds of them have glucocorticoids and or androgens overproductions (3, 4, 8). A small fraction of these patients have pure androgen excess while estrogen or mineralocorticoid excess are very rare (3). Symptoms of ACC patients who have hormone overproduction usually present with an abdominal mass, weight loss, and other constitutional symptoms (1, 10). One thirds of patients presents with nonspecific symptoms that are related to local tumor growth or spread of tumor to surrounding or distant tissues (2). The German ACC Registry has demonstrated that the median age of primary diagnosis of adrenocortical carcinoma is 46.7 years (10). The conventional diagnosis of adrenocortical carcinoma often relies on imaging and hormonal features and has significant limitations. Owing to the development of imaging techniques, more and more adrenal incidentalomas have been discovered. After exclusion of nonfunctioning benign adrenocortical adenoma, a multidisciplinary discussion is warranted. A multidisciplinary team is able to provide all physicians required for diagnosis and treatment of ACC, which could provide a close and personalized management for patients with ACC (11). Early diagnosis associated with surgical tumor removal has been proven as the best option for ACC treatment (12–14).
Genetic analysis and studies have made great advances in the understanding of the disease. However, the patients with ACC are still diagnosed in advanced stage and have poor prognosis with a 5-year mortality rate of approximately 75% to 90% (15–17). Therefore, for better management of ACC, it is mandatory to discover sensitive, specific, and noninvasive biomarkers that can contribute to early diagnosis of ACC. The best biomarkers should not only be able to distinguish malignancy from benign lesions, but also to provide prognostic information and monitor the disease. The aim of this review was to summarize non-invasive biomarkers of adrenocortical cancer, including microRNAs, steroid metabolomics, circulating tumor cells, circulating tumor DNAs, and radiomics. Meanwhile, current problems and possible future applications will also be discussed.
MicroRNAs
Biogenesis and Function of miRNAs
MicroRNAs (miRNA) are small non-coding(~19-24 nucleotides) RNAs (18), most of which undergo a sophisticated maturation process and interact with the 3’untranslated region(3’-UTR) of target messenger RNAs to regulate gene expression at posttranscriptional levels (19, 20). MiRNAs regulate gene expression by inhibiting translation of mRNAs or inducing the degradation of mRNAs (19) (Figure 1). Interaction of miRNAs with other regions of mRNA (the 5’ UTR, coding sequence, gene promoters) has also been discovered (19). The same miRNAs can bind with different mRNAs, and miRNAs often act in a synergistic manner to regulate genes expression (21). Expressions of miRNA are tissue specific and the same miRNA can function as oncogenic or tumor suppressor in different tissues (22). One third coding genes are regulated by miRNAs, which means miRNAs take part in a majority of biological processes including proliferation, differentiation and apoptosis (20, 23). Changes in miRNA expression may be associated with tumor development and progression in adrenocortical carcinoma (16, 24).
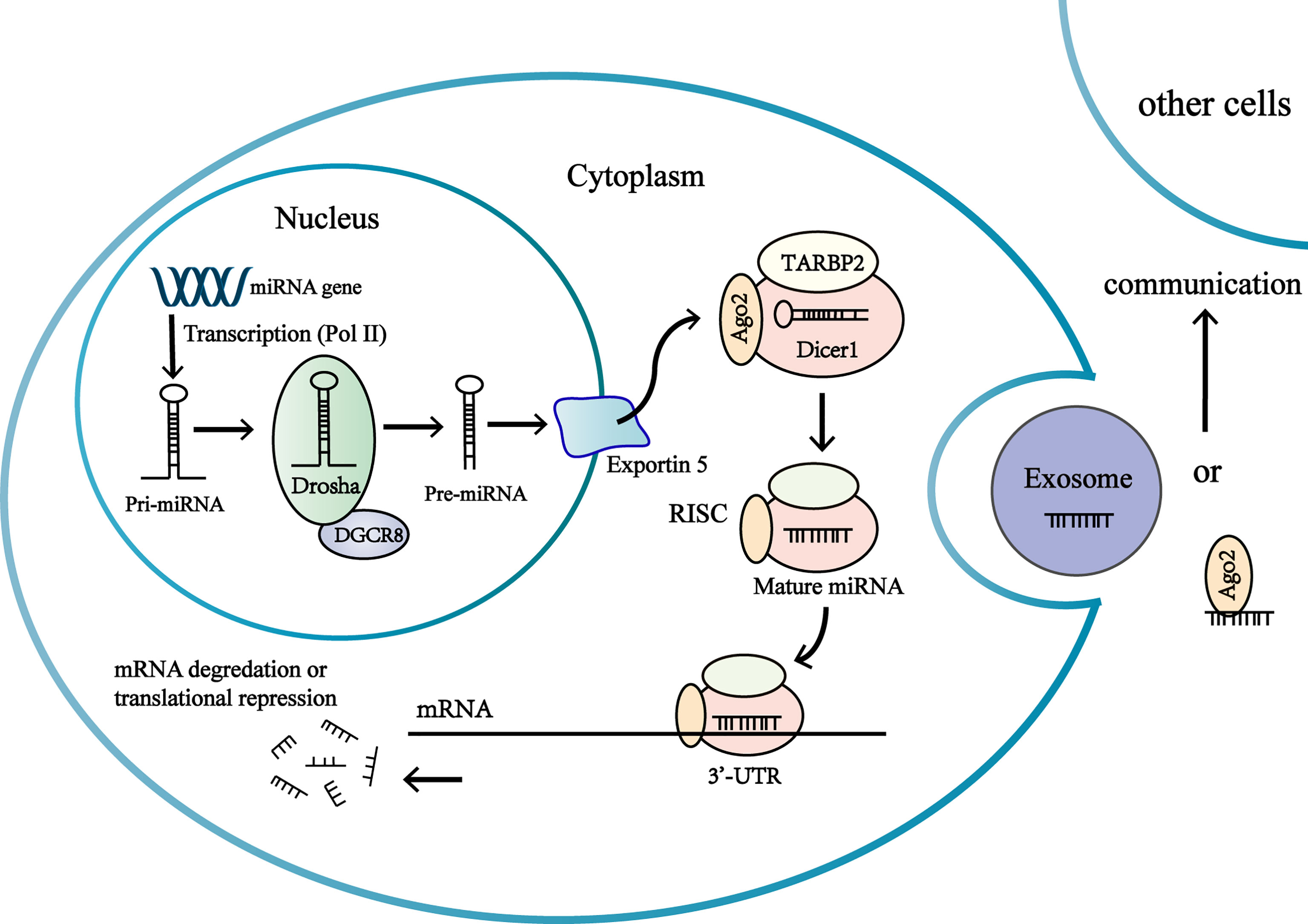
Figure 1 Biogenesis and function of miRNAs in the adrenocortical tumor cells. MiRNA genes are transcribed as primary miRNA (pri-miRNA) in nucleus. Pri-miRNA is processed by multiprotein complex to produce a precursor miRNA (pre-miRNA). The multiprotein complex consists of a double-stranded RNA (dsRNA)-binding protein DGCR8, a nuclear RNase III enzyme Drosha. The pre-miRNAs are transported from nucleus to the cytoplasm by exportin-5 (Exp-5). In the cytoplasm, the pre-miRNA are processed by the RNase III endonuclease Dicer protein and the double-stranded transactivation-responsive RNA-binding protein (TRBP), producing a short double-stranded (ds) miRNA duplex. One strand of miRNA duplex is next loaded into an Ago protein to form RISC, while the other one is degraded by cellular nuclease. RISC complex consists of Dicer, Argonaute 2 (Ago2), and the dsRNA-binding protein TRBP. The association of the miRNA-RISC complex binding to 3’-untranslated region (3’-UTR) of target mRNA leads to inhibition of protein translation or degradation of the mRNA. Extracellular miRNAs in extracellular vesicles or associated with RNA-binding proteins are involved in intercellular communications.
Over the past decade, novel studies have found that miRNAs can be extracted from various body fluids (blood, urine, feces, breast milk, saliva, tears, etc.) and can be detected with minimal invasion (23, 25–27). Observations have revealed that microRNAs enter body fluids via passive release (inflammation or necrosis) or via active secretion. Actively secreted miRNAs are either packed in extracellular membrane vesicles (EV) (microvesicles, exosomes, apoptotic bodies) or attached into macromolecular complexes like Argonaute 2 (AGO2) protein and high density lipoprotein (HDL) (27–29) (Figure 1). Extracellular miRNAs in extracellular vesicles or associated with high density lipoprotein have been discovered to be transferred to another cell and regulate gene expressions, thus circulating miRNAs might act as epigenetic ‘hormones’ by influencing gene expressions, even in distant cell or tissues (21, 29, 30) (Figure 1). However, the mechanisms of regulating miRNA to be secreted into body fluids are poorly understood (21).
Circulating MiRNAs
To find miRNAs biomarkers for diagnosis of ACC, several studies have investigated the different expressions of tissue miRNAs in benign and malignant adrenocortical tumors. Among these studies, miR-483-5p, miR-483-3p, miR-210, and miR-503 are most consistently overexpressed in ACC, while miR-195 are found to be underexpressed in ACC (24, 31–38). The results have suggested that miRNAs may be a promising biomarkers for diagnosis of adrenocortical carcinoma.
Circulating counterparts of these miRNAs in ACC have been detected in several studies, as well (Table 1). By analyzing the results of these studies, a great number of deregulated miRNAs were identified and validated (Figure 2). Deregulated plasma or serum miRNAs have been found by comparing miRNA absolute levels or using dCT method (△CT value equals target miRNA’s CT minus internal control miRNA’s CT) between ACC and ACA (24, 36, 39–44).
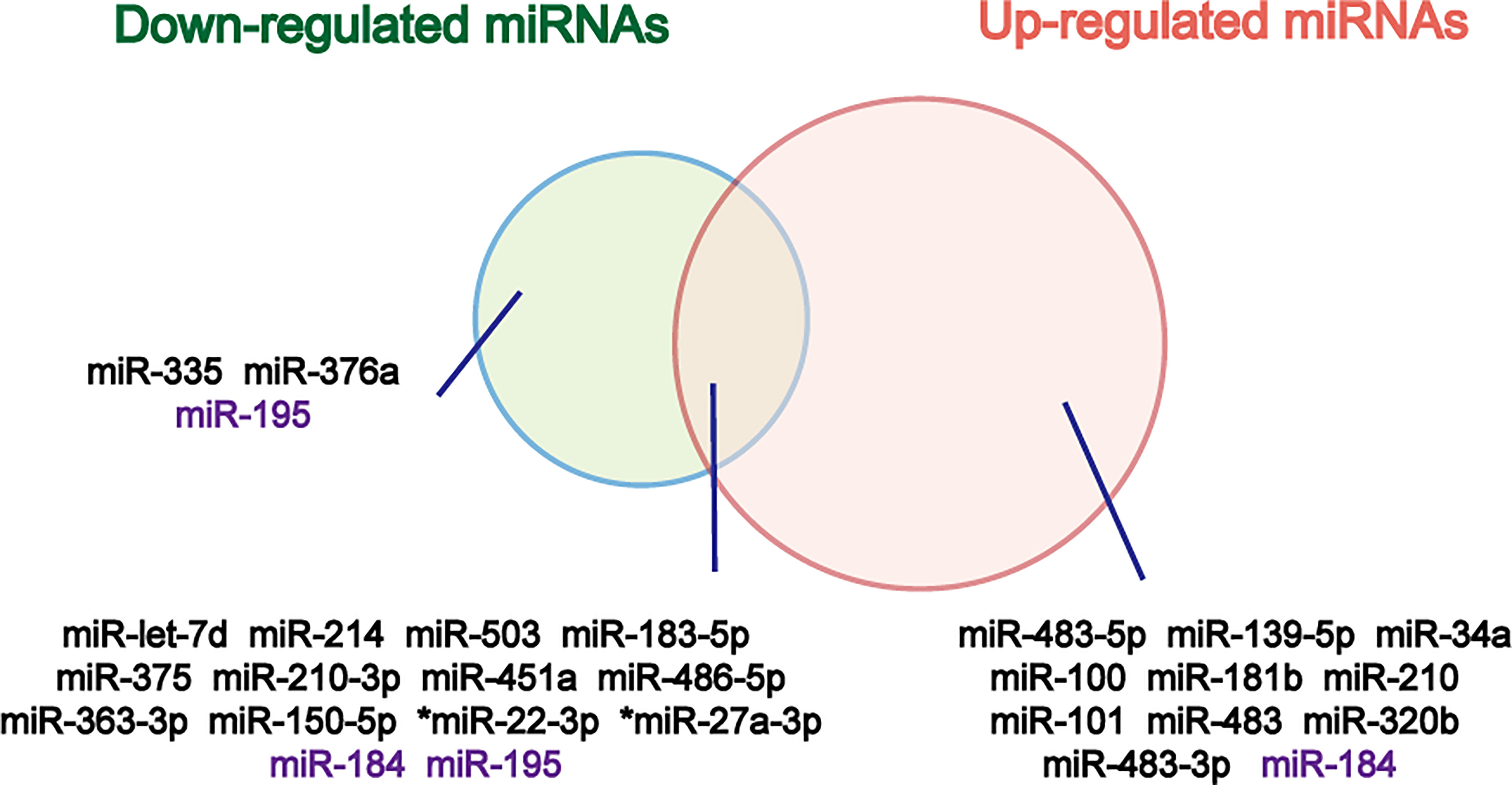
Figure 2 Venn diagram of down- and up-regulated miRNAs in ACC relative to ACA. The miRNAs that don’t show same results in different studies are marked purple. *Overexpressed in both cortisol-producing ACC and cortisol-producing ACA.
Overexpressed plasma or serum miR-483-5p has been found in nearly all studies, and the expression of circulating miR-483-5p was not influenced by hormonal tests used in routine diagnosis of cortisol overproduction (22). Based on the findings above, circulating miR-483-5p can be served as the best biomarker for diagnosis of ACC. However, in Chabre et al. study, ACCs were subdivided into patients with aggressive ACC (aACC) and patients with no-aggressive ACC (naACC). Serum miR-483-5p was detected neither in healthy people nor in ACA or naACA patients. On the contrary, serum from patients with aACC displayed a high level of miR-483-5p (median value: 472 000 copies/ml) (24). In another study, circulating miRNAs were evaluated in patients with ACC, ACA and adrenal myelolipoma (AML), and no significant difference of miR-483-5p expression was found in ACC and AML samples (36). Results of these two studies might limit the clinical utility of circulating miR-483-5p for diagnosis of malignancy.
Salvianti et al. have measured miR483 and miR-483-5p absolute levels in plasm samples, and significant overexpressions of miR-483 and miR-483-5p were detected in advanced stages of ACC (stage III-IV) relative to early stages (stage I-II), ACA and normal adrenal cortices (42). A correlation between miR-483-5p levels and the number of circulating tumor cells has also been discovered (42). In another study, a correlation between urinary and plasma expression of miR-483-5p has been found (44). However, no significant difference of expression of miR-483-5p has been identified in urine samples between ACC and ACA (44).
There is no recommendation whether plasma or serum is more suitable for evaluation of extracellular miRNAs. A study observed RNAs in serum samples and in the corresponding plasma samples, the higher RNA concentration in serum samples have been found. The reason for this phenomenon was that addition RNA was released from cell during the coagulation process, which demonstrated the miRNAs in serum could be affected by this process (45). Using plasma to extract miRNAs was not affected by above process, but cellular contamination such as platelets can occur easily (46). Several studies have investigated the expression of EV-associated miRNAs, which are secreted actively and could be more sensitive and specific (41). Perge et al. have investigated the expression of EV-associated miRNAs and their applicability for diagnosis of ACC. Overexpressed miR-483-5p and miR-101 have been found in ACC compared to ACA in this study (41).
As for reference genes, synthetic spike-in-RNAs (e.g. cel-miR-39) has been used as reference in several studies (24, 40, 41), and Circulating miRNAs with relatively stable expression like miR-16 was useful in some studies, as well (20, 39, 40). In previous studies, researchers have found that miR-16 and cel-miR-39 were applicable for normalization. However, it is still a debate which is the most optimum method for miRNA normalization in qRT-PCR analysis.
The diagnostic accuracy of these miRNAs was different (Table 2). Extracellular vesicle (EV) associated miR-483-5p appeared to be the most promising minimally invasive biomarker of ACC with the highest accuracy (41). Meanwhile, miR-195 in serum had a high diagnostic accuracy as well (24). However, the sensitivity and specificity reported for these circulating miRNAs are not high enough for clinical diagnosis of malignancy at present.
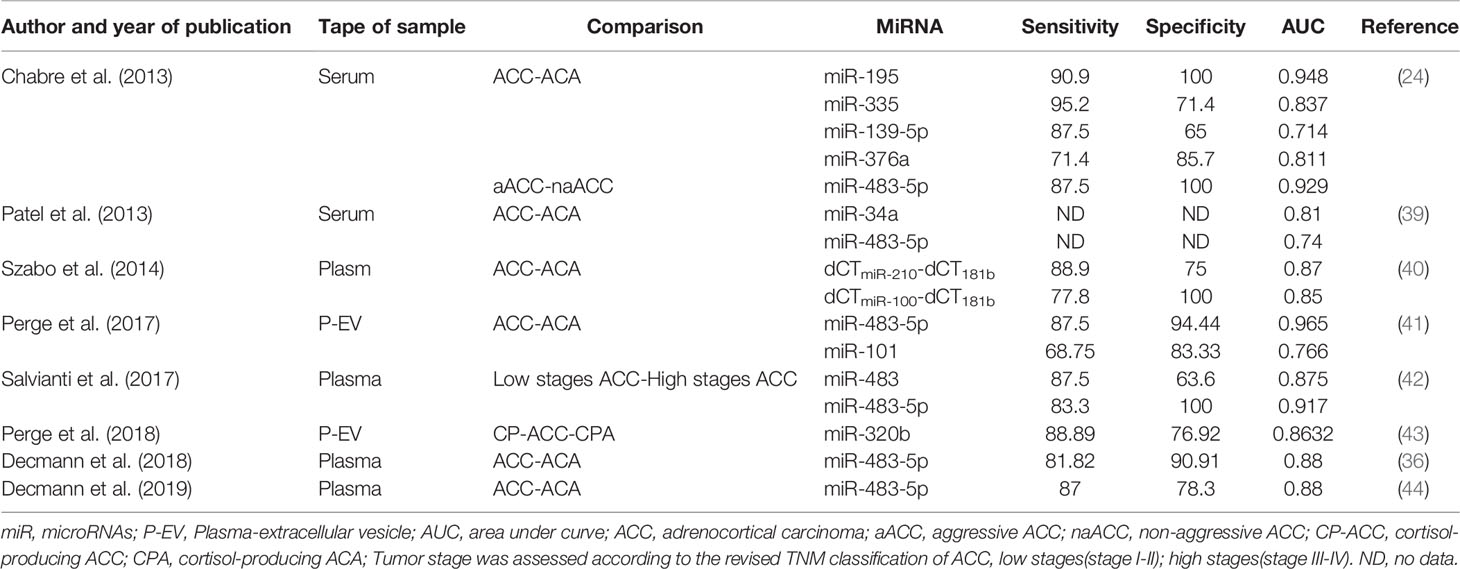
Table 2 Diagnostic accuracy of circulating miRNA for differentiating adrenocortical carcinoma from adrenocortical adenoma.
The expression of some circulating miRNA might be associated with cortisol secretion. Perge et al. evaluated the expression of plasma extracellular vesicle (EV)-associated miRNAs in patients with non-functioning adrenocortical carcinoma (NFA), cortisol-producing adrenocortical adenoma (CPA) and cortisol-producing adrenocortical carcinoma(CP-ACC). The expressions of miR-22-3p, miR-27a-3p, and miR-320b were significantly increased in both CP-ACC and CPA compared to NFA. The expression of miR-320b was detected to be overexpressed in CP-ACC relative to CPA (43). Igaz et al. have investigated the effect of adrenocorticotropin and dexamethasone on the expression of miRNAs. MiR-27a expression can be regulated by hormone both in vitro and in vivo (47). Based on these findings, it is supposed that cortisol overproduction might play a role in expressions of some miRNAs.
The role of miRNAs as prognostic markers in patients of ACC have been investigated in several studies. Chabre et al. has reported for the first time that shorter overall survival was associated with increased miR-483-5p and decreased miR-195 expression in circulation (24). A recent study, measuring miR-483-5p levels three months after surgery, has found that serum miR-483-5p levels were higher in patients with poor prognosis (recurrence or death within 3 years after surgery) than patients with good prognosis (no recurrence for at least 3 years) (48).
Moreover, circulating miRNAs could be applied as non-invasive biomarkers for evaluating treatment efficacy. This application of miRNAs has been investigated in two xenograft studies. The expression of circulating miR-483-5p was significantly reduced by effective mitotane and 9-cis retinoic acid treatment in a mouse NCI-H295R xenograft model (49). MiR-210 ratio in an SW-13 xenograft model was altered after liposomal etoposide-doxorubicin-platina-mitotane (LEDP-M) chemotherapy treatment, which indicated that plasm miR-210 might be a promising biomarker for monitoring treatment efficiency (50).
Challenges and Perspectives
It must be noted that despite these promising results, applying circulating miRNAs for diagnosis, prognosis, and follow-up is still difficult. It is related to different platforms, different analytical methodologies, lack of standard genes, and the low concentration of miRNAs. Meanwhile, miRNAs are not specific for ACC, which means the same variation could be found in another disease. Furthermore, the number of the patients involved in previous studies was small. In the future, further studies with large cohorts, standard methodologies, and recommended reference genes are warranted.
Steroid Metabolite Profiling
Steroidogenesis in Adrenocortical Carcinoma
50-70% of ACC are found to produce adrenal hormones in excess, though this is not clinically apparent in many cases (4, 7). Serum and urinary steroid analysis generally play an important part in diagnosis of adrenal hormone excess and disorders of steroidogenesis. Based on traditional serum steroid analysis, which mostly assesses end products of steroidogenesis, a large number of patients with ACCs are defined as nonfunctioning. However, the steroidogenic pattern produced by dedifferentiated and immature malignant ACC cells is characterized by many steroid precursors and metabolites rather than end products of complete steroidogenesis (51), and precursors and metabolites are often accumulated in patients with ACC (52) (Figure 3). Researchers have investigated steroid profiling and have found that steroid profiling has great potential in diagnosis, prognosis and monitoring recurrence in ACC.
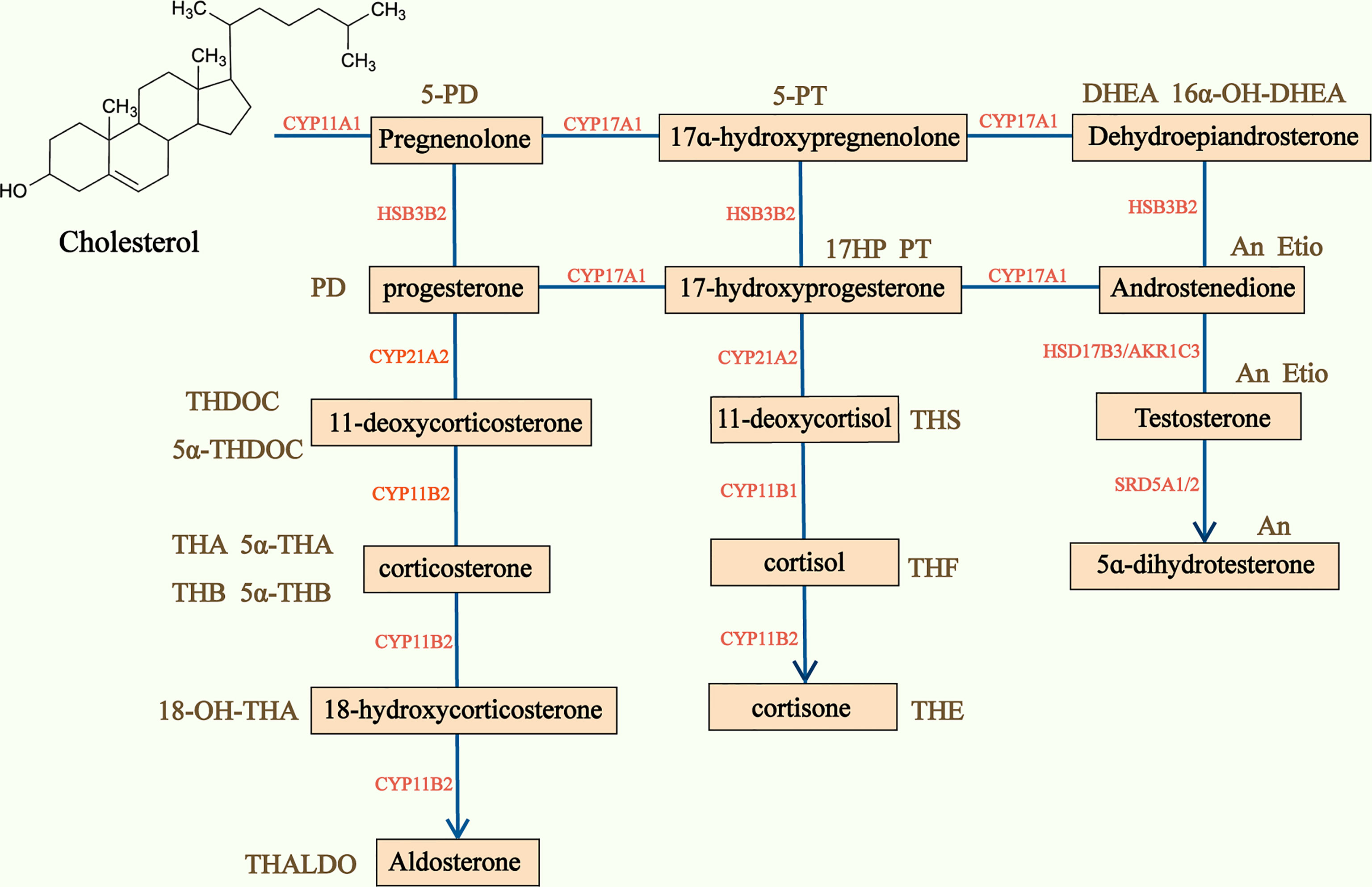
Figure 3 Adrenal steroidogenesis and main corresponding urine steroid metabolites. Steroidogenesis pathway is represented in the center of the figure and the main urine steroid metabolites on the sides of the picture. CYP, Cytochrome P450; HSD, hydroxysteroid dehydrogenase; 5-PD, pregnenediol; PD, pregnandiol; THDOC, tetrahydrodeoxycorticosterone; THA, tetrahydro-11-dehydrocorticosterone; THB, tetrahydrocorticosterone; THALDO, tetrahydroaldosterone; 5-PT, pregenetriol; PT, pregnanetriol; THS, tetrahydro-11-deoxycortisol; THF, tetrahydrocortisol; Et, etiocholanolone; THE, tetrahydrocortisone; DHEA, dehydroepiandrosterone; An, androsterone; Etio, Etiocholanolone.
Urine Steroid Profiling
Several decades ago, Grondal et al. have shown that the urine metabolomics might be reliable to identify ACC with high sensitivity and specificity and to predict their recurrence during follow-up (53). More recently, several retrospective studies have described patients with adrenal tumors showing significant differences of steroid excretions between ACCs and ACAs (Table 3), which suggests significant value of urine steroid metabolite profiling for a highly accurate diagnostic test of adrenocortical tumors.
The first large research of steroid profiling was conducted by Arlt et al. with 45 ACC patients and 102 ACA patients (54). Quantifications of 32 distinct adrenal steroids were carried out by gas chromatography-mass spectrometry(GC-MS) in urine samples. The study indicated that the glucocorticoid precursor metabolite tetrahydro-11-deoxycortisol(THS) and androgen precursor metabolites pregnanediol and pregnanetriol were the most informative steroids in discriminating ACC from ACA. Nine most discriminative steroids for differentiating ACC from ACA have been identified by using the machine learning-based algorithm called generalized matrix learning vector quantization (GMLVQ). Receiver-operating characteristics (ROC) analysis demonstrated that the diagnostic information of 32 steroids resulted in 90% sensitivity and specificity, while the nine most discriminative and the top three steroids provided only slightly lower diagnostic accuracies (87.7% and 87.2%, respectively) (54). Receiver-operating characteristics revealed great performance of GMLVQ.
In Kerkhofs et al. study, they performed GC-MS analysis but did not apply computational analysis of 22 steroid metabolites in 27 ACCs, 107 ACAs, and 8 other adrenal conditions. They found 15 steroid markers with a sensitivity of at least 90% in diagnosing ACCs, but specificities varied from 2% to 83% (55). They showed that THS was the most informative markers again and its excretion was significantly associated with ACC tumor size and stage. In addition, THS at a cut-off value of 2.35umol/24h can detect ACC with a 100% sensitivity and 99% specificity (55).
Velikanova et al. conducted a research to analyze urine steroid profiling by using high-performance liquid chromatography(HPLC) and gas chromatography-mass spectrometry(GC-MS) methods in 31 ACCs and 108 ACAs. By combining several parameters of urine steroids, including THS, pregnanediol, 5-pregnanetriol, and 5-pregnanediol, 100% sensitivity and specificity of ACC, and ACA differential diagnosis can be achieved (56). THS was also confirmed to be the most informative markers. However, increased urinary excretion of THS was only in 74% of ACC patients, and DHEA and its metabolites in 67.7% patients with ACC (56). Hines et al. have developed a novel multiple-steroid assay to quantify 26 urine steroid metabolites by using HRAM LC-MS, and have shown that patients with ACC had 11 steroid metabolites with increased Z scores, especially tetrahydro-11-deoxycortisol (14 vs 0.5, P<0.001), pregnanetriol (7.5 vs -0.4, P=0.001), and 5-pregnenetriol (5.4 vs -0.4, P=0.01), which might improve diagnosis for patients with ACC (57).
These studies found that tetrahydro-11-deoxycortisol (THS) was the most informative steroid marker to discriminate ACC from ACA (54–58). THS is the metabolite of 11-deoxycortisol, which is converted to cortisol by 11β-hydroxylase. The mechanism for THS overproduction in ACC remains unclear, but it seems to be related with deficiency of 11β-hydroxylase and or dysfunction of the enzyme itself. The mechanism of accumulation of other precursors may be similar with THS (59).
We can find that in all the studies above, the excretion of steroid precursor metabolites in ACC is higher than metabolites in ACA. Urine steroid profiling is a promising prognostic method to distinguish ACCs from ACAs with high sensitivity and specificity. However, above studies were based on retrospective studies with small numbers of patients. In order to use urine steroid profiling for distinguishing malignancy from benign, a large prospective study is needed.
Recently, to validate the use of urine steroid metabolomic testing, a multicenter Evaluation of Urine Steroid metabolomics in the Differential Diagnosis of Adrenocortical Tumor(EURINE-ACT) study was conducted. Bancos et al. have validated the diagnostic utility of urine steroid metabolomics in detecting ACCs (59). Previous studies of urine steroid metabolomics for ACC detection analyzed urinary steroid profiling by gas chromatography-mass spectrometry, which is a low-throughput method requiring highly specialized expertise. In this study, Bancos et al. used the high-throughput LC-MS/MS approach, which is more widely available in clinical routine. The accuracy of diagnosis of urine metabolomics was higher than maximum diameter and imaging characteristics in this study. When combining these three parameters, the positive predictive value was 76.4% (59).
Urine steroid metabolites can not only hold promise for early diagnosis but also provide a non-invasive biomarker for the follow-up of patients with ACC. Several studies have shown that the detection of steroid metabolites in urine can predict recurrence before imaging evidence is found; and can be used to assess completeness of resection after surgery (60, 61). Early detection of recurrence may allow timely cytotoxic chemotherapy and radical surgery, which can improve the overall survival. It is noted that the levels of urine steroid are associated with the bulk of malignant lesions. Mitotane is the most widely used chemotherapy medications in adrenocortical carcinoma, which interferes with steroidogenesis in various ways. Nevertheless, Chortis et al. have found that the diagnostic performance of the steroid profile was not influenced by adjuvant mitotane treatment (60).
Plasma Steroid Profiling
Steroid profile in urine samples allows a non-invasive integrated assessment of daily steroid production, which provides a high sensitivity and specificity in differentiating ACC from non-ACC lesions (62). However, the analysis of steroid profile in urine still has many limitation. GC-MS poor practicability and the inaccuracy of 24-h urine collection restrict to transfer this technique into clinical practice (63).
Plasma samples are easier to collect than 24h urine samples. Two studies have examined steroid profiling by using LC-MS/MS in serum to investigate their value in diagnosis and monitoring recurrence of ACC. Liquid-chromatography mass spectrum were used in both studies, which is readily accessible in clinical routine (64). In a very small study with only ten ACC patients, Taylor et al. have developed an LC-MS/MS method for measurement of a 13-steroid panel. 4-7 steroids (median=6) were increased in ACC cases, while 11-Deoxycortisol was markedly increased in all cases of ACC. A complete separation between ACC and non-ACC group could be achieved by using the whole 13-steroid panel. 11-deoxycorticol and 17-hydroxypregnenolone were the best markers to discriminate ACC from non-ACC (65).
More recently, Schweizer et al. have quantified 15 steroid hormones in 66 ACA and 42 ACC plasma samples by combining multiple statistics into a machine-learning model. Higher abundances of 11-deoxycorticosterone, progesterone, 17-hydroxyprogesterone, 11-deoxycortisol, DHEA, DHEAS and estradiol have been observed in ACC (66). In comparison with the study of Arlt’s group, the AUC values of their model (six steroid hormones) are significantly smaller than those obtained with nine steroid metabolites in 24h urine. The model could not be improved by inclusion of all measured plasma steroids (66). It may be that the large proportion of tumors in Arlt et al. study were in advanced stages, which may be associated with more prominent alterations of steroid hormones and precursors. Meanwhile, in Alrt et al. study, a larger number of ACAs were included (54). A conclusion regarding the optimal matrix and method of analysis cannot be drawn yet.
Challenges and Perspectives
Based on what’s been discussed above, the diagnostic and prognostic value of steroid profiling in ACC has been confirmed. However, there are still many challenges. The majority of measurements of steroid profiling are rely on GC-MS. The GC-MS can only be manufactured by a small number of laboratories and institutions. Meanwhile, a lack of harmonization between laboratories has limited its further investigations and clinical applications of it. Detection of a steroid profiling is a time-consuming examination, so it cannot be used for screening of all patients with ACC at present. Therefore, developing simple methods for analysis of specific steroid profiling is crucially important. Owing to the low incidence of adrenocortical carcinoma, studies often conclude a few cases. Prior to implementation in routine clinical practice, steroid profiling as a diagnostic tool need to be validated in a larger cohort.
Circulating Tumor Cells
Another prospective area of ACC is the study of circulating tumor cells(CTCs). Circulating tumor cells (CTCs) are neoplastic cells shed into the blood stream from either primary tumor or metastases (67–70). They can be differentiated from patients’ surrounding normal hematopoietic cells. Approaches to CTCs isolation include a large panel of technologies, which were based on different properties (physical and biological properties) of CTCs. Physical properties of CTCs include density, size, electric charges, deformability and so on, while biological properties include cell surface protein expression and viability (71). The advantage of using physical properties is that they allow CTC separation without labeling (71). Detection of circulating CTCs in peripheral blood is a useful tool for diagnosis, prognosis, and follow-up in several solid cancers, especially breast cancer (67, 68, 72–74).
A preliminary study has revealed that CTCs are present in circulation of patients with ACC but not in patients with ACA, providing the first evidence that CTCs might be a valid and useful presurgical marker to differentiate between malignant and benign adrenocortical tumors (67). Meanwhile, a significant decrease in the number of CTCs has been found after surgery compared to presurgical samples, which illustrates that CTCs can be a promising biomarker for follow-up (67).
The results of the recently published research have shown that CTCs (obtained from liquid blood biopsy) were presents in 68% of pre-surgery and in 38% of post-surgery blood samples, which illustrates that CTCs can be a promising marker for monitoring disease in ACC (75). Patients were stratified in high and low pre-surgery CTC number groups (75th percentile CTC value as cut-off), and CTCs significantly predicted the patients’ overall survival (75). Meanwhile, CTCs provide a minimal invasive method that can be used to study genetic information, which will contribute to our understanding of adrenocortical tumors (76). Both studies above isolated CTCs from blood by ScreenCell devices system (ScreenCell), which were based on cell size and morphological criteria. Immunocytochemistry was performed on enriched CTCs and their origins of ACC were confirmed. Although with the steady development of techniques, CTC isolation has become more feasible over the past years, CTC identification and characterization remain challenging.
These studies provide a promising utility of CTCs for diagnosis and follow-up of ACC. However, they were both based on small cohorts of patients. Further studies with larger cohorts of patients are needed to confirm the diagnostic and prognostic value of CTCs in adrenocortical carcinoma before we can establish its clinical utility.
Circulating Cell-Free Tumor DNA
Circulating cell-free DNAs (cfDNAs) are short extracellular DNA fragments (approximately 160-180bp) in body fluids that are derived from the remnants of “healthy cells”, malignant cells, and tumor microenvironmental cells (77, 78). Circulating cell-free DNA is predominantly resulted from enzymatic degradation during or after cell death (79). In patients with ACC, a variable fraction of cfDNA in body fluids is contributed by cancer cells. These cfDNAs, known as circulating tumor DNA(ctDNA), carrying tumor-specific somatic genetic alterations, can be released by the primary tumor, metastasis, and circulating tumor cells. The amount of ctDNA is largely depends on the tumor type, diameter and disease stage (80). The mechanism by which the tumor sheds DNA into the blood is still obscure (81). Detection of somatic mutations that are specific to cancer cells helps discriminate circulating cell-free tumor DNA(ctDNA) from ccfDNA of non-tumoral origin (82).
The poor quality of ctDNA and its extremely low levels makes qualification and detection quite difficult (79). The development of highly sensitive technologies, such as digital PCR and Next Generation Sequencing enables researchers to obtain ctDNA profiling and compare it with the profiling of the primary lesions to gain deep insight into the heterogeneity and clonal evolution of the tumor (77).
The role of ctDNA as a biomarker for diagnostic, prognostic, and therapeutic monitoring, has already been investigated in many types of cancers (82, 83). Recently, ctDNA has been investigated in ACC as well. There are two studies that have detected the mutation in cfDNA within serum samples of patients with ACC.
Creemers et al. performed a pilot study including six patients with ACC. Mutations have been found in primary tumors in three of them by Next-generation sequence (NGS). Cell-free circulating DNA (cfDNA) was isolated from blood samples of these patients, which were collected before (1 to 2 weeks) and after surgery. Tumor-specific mutations were only found in one of these three patients who had metastatic ACC at diagnosis (84). Preoperative cfDNA showed the same mutations as primary tumor by NGS, while postoperative cfDNA showed the same mutations but at lower frequencies. In the cfDNA from two patients with known mutations in primary tumor, tumor-specific mutations can’t be detected, which suggests that this minimally invasive approach will only be suitable for detecting disease progression in a subgroup of patients with ACC (84). They detect mutations in primary tumor by NGS to identify these tumor-specific mutations in cfDNA. The limitation of this method is that the tumor-specific mutations, which were detected in cfDNA, must be present in primary tumor and can be detected by NGS (84).
In another study, ctDNA was detected by highly sensitive technologies (deep NGS and droplet digital RCR). Garinet et al. also found that ctDNA only presented in a subset of ACC patients. In this study, eight patients were detected of at least one mutation in the tumor, while mutations in the cfDNA were only found in two out of these eight patients. Though two of the currently most sensitive molecular biology technologies were used, it remains challenging to conclude whether ctDNA detection was negative because of a limited sensitivity (85).
These two results indicate that different ACC patients were associated with different levels of ctDNA. Compared with CTCs, ctDNA is much easier to isolate and more sensitive to detect. However, application of ctDNA as a potential biomarker for ACC remains a problem. Further studies with larger cohorts, longitudinal monitoring, and standardized methodologies are needed in the future.
Radiomics
The main challenge in the management of adrenal incidentalomas is to differentiate malignant from benign lesions. CT is the dominant imaging tool for evaluating the adrenocortical tumors. Size is thought to be the most important predictor for malignancy, and a size of > 4cm is a crucial feature (86). A large retrospective single-center study has found that 70% larger adrenal tumors (> 4cm) turn out to be non-malignant lesions (87). Therefore, this risk factor (diameter) should not be taken in isolation for diagnosis of malignancy. Machine learning has been used to differentiate large (> 4cm) adrenocortical carcinoma from other large adrenocortical lesions on contrasted-enhanced CT. The radiomics, obtained by machine learning had a diagnostic accuracy (82%) for malignant tumor exceeding that of radiologists (68.5%) (88). Meanwhile, Torresan et al. have also found that CT texture analysis by an unsupervised machine learning approach could predict malignancy in nearly all patients (89). These two studies have illustrated that radiomics could be served as a novel non-invasive tool to differentiate benign from malignant adrenocortical tumors. For clinical perspective, other non-invasive biomarkers (miRNA, steroid profiling and so on) requires a long time to collect and analyze data. Meanwhile these biomarkers are not available in all countries. On the contrary, computed tomography is used in all clinics and only a dedicated software is required. However, these two studies have limitations related to their design and relatively small sample size. Further studies on larger patient populations are warranted.
Conclusion
In spite of all the progress that has been made, it seems that we are far from utilizing non-invasive biomarkers to diagnose a malignant adrenocortical tumor and predict prognosis. Although many problems remain unsolved, future research directions of early diagnosis and detection of recurrence of ACC may still revolve around these non-invasive biomarkers. We expect that non-invasive biomarkers will be used in clinical practice in the future and will improve the management of adrenocortical carcinoma.
Author Contributions
YZ designed and revised the manuscript. YC, WK, DZ, and XY wrote the manuscript and made the figures. All authors contributed to the article and approved the submitted version.
Funding
This work was supported by the grants from the National Science Foundation of Shanghai (NO.21ZR1440700).
Conflict of Interest
The authors declare that the research was conducted in the absence of any commercial or financial relationships that could be construed as a potential conflict of interest.
Publisher’s Note
All claims expressed in this article are solely those of the authors and do not necessarily represent those of their affiliated organizations, or those of the publisher, the editors and the reviewers. Any product that may be evaluated in this article, or claim that may be made by its manufacturer, is not guaranteed or endorsed by the publisher.
Acknowledgments
We would like to thank all members in our group and apologize to researchers whose work could not be cited or discussed owing to space limitations.
References
1. Else T, Kim AC, Sabolch A, Raymond VM, Kandathil A, Caoili EM, et al. Adrenocortical Carcinoma. Endocr Rev (2014) 35(2):282–326. doi: 10.1210/er.2013-1029
2. Mansmann G, Lau J, Balk E, Rothberg M, Miyachi Y, Bornstein SR. The Clinically Inapparent Adrenal Mass: Update in Diagnosis and Management. Endocr Rev (2004) 25(2):309–40. doi: 10.1210/er.2002-0031
3. Fassnacht M, Dekkers OM, Else T, Baudin E, Berruti A, de Krijger R, et al. European Society of Endocrinology Clinical Practice Guidelines on the Management of Adrenocortical Carcinoma in Adults, in Collaboration With the European Network for the Study of Adrenal Tumors. Eur J Endocrinol (2018) 179(4):G1–G46. doi: 10.1530/EJE-18-0608
4. Libe R. Adrenocortical Carcinoma (ACC): Diagnosis, Prognosis, and Treatment. Front Cell Dev Biol (2015) 3:45. doi: 10.3389/fcell.2015.00045
5. Xing Z, Luo Z, Yang H, Huang Z, Liang X. Screening and Identification of Key Biomarkers in Adrenocortical Carcinoma Based on Bioinformatics Analysis. Oncol Lett (2019) 18(5):4667–76. doi: 10.3892/ol.2019.10817
6. Fassnacht M, Kroiss M, Allolio B. Update in Adrenocortical Carcinoma. J Clin Endocrinol Metab (2013) 98(12):4551–64. doi: 10.1210/jc.2013-3020
7. Vaidya A, Nehs M, Kilbridge K. Treatment of Adrenocortical Carcinoma. Surg Pathol Clin (2019) 12(4):997–1006. doi: 10.1016/j.path.2019.08.010
8. Allolio B, Fassnacht M. Clinical Review: Adrenocortical Carcinoma: Clinical Update. J Clin Endocrinol Metab (2006) 91(6):2027–37. doi: 10.1210/jc.2005-2639
9. Kebebew E, Reiff E, Duh QY, Clark OH, McMillan A. Extent of Disease at Presentation and Outcome for Adrenocortical Carcinoma: Have We Made Progress? World J Surg (2006) 30(5):872–8. doi: 10.1007/s00268-005-0329-x
10. Fassnacht M, Johanssen S, Quinkler M, Bucsky P, Willenberg HS, Beuschlein F, et al. Limited Prognostic Value of the 2004 International Union Against Cancer Staging Classification for Adrenocortical Carcinoma: Proposal for a Revised TNM Classification. Cancer (2009) 115(2):243–50. doi: 10.1002/cncr.24030
11. Voltan G, Boscaro M, Armanini D, Scaroni C, Ceccato F. A Multidisciplinary Approach to the Management of Adrenal Incidentaloma. Expert Rev Endocrinol Metab (2021) 16(4):201–12. doi: 10.1080/17446651.2021.1948327
12. Luton JP, Cerdas S, Billaud L, Thomas G, Guilhaume B, Bertagna X, et al. Clinical Features of Adrenocortical Carcinoma, Prognostic Factors, and the Effect of Mitotane Therapy. N Engl J Med (1990) 322(17):1195–201. doi: 10.1056/nejm199004263221705
13. Redlich A, Boxberger N, Strugala D, Fruhwald MC, Leuschner I, Kropf S, et al. Systemic Treatment of Adrenocortical Carcinoma in Children: Data From the German GPOH-MET 97 Trial. Klin Padiatr (2012) 224(6):366–71. doi: 10.1055/s-0032-1327579
14. Terzolo M, Angeli A, Fassnacht M, Daffara F, Tauchmanova L, Conton PA, et al. Adjuvant Mitotane Treatment for Adrenocortical Carcinoma. N Engl J Med (2007) 356(23):2372–80. doi: 10.1056/NEJMoa063360
15. Ayala-Ramirez M, Jasim S, Feng L, Ejaz S, Deniz F, Busaidy N, et al. Adrenocortical Carcinoma: Clinical Outcomes and Prognosis of 330 Patients at a Tertiary Care Center. Eur J Endocrinol (2013) 169(6):891–9. doi: 10.1530/EJE-13-0519
16. Cherradi N. microRNAs as Potential Biomarkers in Adrenocortical Cancer: Progress and Challenges. Front Endocrinol (Lausanne) (2015) 6:195. doi: 10.3389/fendo.2015.00195
17. Nakamura Y, Yamazaki Y, Felizola SJ, Ise K, Morimoto R, Satoh F, et al. Adrenocortical Carcinoma: Review of the Pathologic Features, Production of Adrenal Steroids, and Molecular Pathogenesis. Endocrinol Metab Clin North Am (2015) 44(2):399–410. doi: 10.1016/j.ecl.2015.02.007
18. Vannini I, Fanini F, Fabbri M. Emerging Roles of microRNAs in Cancer. Curr Opin Genet Dev (2018) 48:128–33. doi: 10.1016/j.gde.2018.01.001
19. O’Brien J, Hayder H, Zayed Y, Peng C. Overview of MicroRNA Biogenesis, Mechanisms of Actions, and Circulation. Front Endocrinol (Lausanne) (2018) 9:402. doi: 10.3389/fendo.2018.00402
20. Brase JC, Wuttig D, Kuner R, Sültmann H. Serum microRNAs as Non-Invasive Biomarkers for Cancer. Mol Cancer (2010) 9:306. doi: 10.1186/1476-4598-9-306
21. Igaz P. Circulating microRNAs in Adrenal Tumors. Curr Opin Endocrinol Diabetes Obes (2019) 26(3):155–9. doi: 10.1097/MED.0000000000000472
22. Igaz P, Igaz I, Nagy Z, Nyiro G, Szabo PM, Falus A, et al. MicroRNAs in Adrenal Tumors: Relevance for Pathogenesis, Diagnosis, and Therapy. Cell Mol Life Sci (2015) 72(3):417–28. doi: 10.1007/s00018-014-1752-7
23. Decmann A, Perge P, Nagy Z, Butz H, Patocs A, Igaz P. Circulating microRNAs in the Diagnostics of Endocrine Neoplasms. Orv Hetil (2017) 158(13):483–90. doi: 10.1556/650.2017.30708
24. Chabre O, Libe R, Assie G, Barreau O, Bertherat J, Bertagna X, et al. Serum miR-483-5p and miR-195 Are Predictive of Recurrence Risk in Adrenocortical Cancer Patients. Endocr Relat Cancer (2013) 20(4):579–94. doi: 10.1530/ERC-13-0051
25. Igaz I, Igaz P. Diagnostic Relevance of microRNAs in Other Body Fluids Including Urine, Feces, and Saliva. Exp Suppl (2015) 106:245–52. doi: 10.1007/978-3-0348-0955-9_11
26. Chen X, Ba Y, Ma L, Cai X, Yin Y, Wang K, et al. Characterization of microRNAs in Serum: A Novel Class of Biomarkers for Diagnosis of Cancer and Other Diseases. Cell Res (2008) 18(10):997–1006. doi: 10.1038/cr.2008.282
27. Redis RS, Calin S, Yang Y, You MJ, Calin GA. Cell-To-Cell miRNA Transfer: From Body Homeostasis to Therapy. Pharmacol Ther (2012) 136(2):169–74. doi: 10.1016/j.pharmthera.2012.08.003
28. Zen K, Zhang CY. Circulating microRNAs: A Novel Class of Biomarkers to Diagnose and Monitor Human Cancers. Med Res Rev (2012) 32(2):326–48. doi: 10.1002/med.20215
29. Perge P, Nagy Z, Igaz I, Igaz P. Suggested Roles for microRNA in Tumors. Biomol Concepts (2015) 6(2):149–55. doi: 10.1515/bmc-2015-0002
30. Decmann A, Perge P, Turai PI, Patocs A, Igaz P. Non-Coding RNAs in Adrenocortical Cancer: From Pathogenesis to Diagnosis. Cancers (Basel) (2020) 12(2):461. doi: 10.3390/cancers12020461
31. Ozata DM, Caramuta S, Velazquez-Fernandez D, Akcakaya P, Xie H, Hoog A, et al. The Role of microRNA Deregulation in the Pathogenesis of Adrenocortical Carcinoma. Endocr Relat Cancer (2011) 18(6):643–55. doi: 10.1530/ERC-11-0082
32. Soon PS, Tacon LJ, Gill AJ, Bambach CP, Sywak MS, Campbell PR, et al. miR-195 and miR-483-5p Identified as Predictors of Poor Prognosis in Adrenocortical Cancer. Clin Cancer Res (2009) 15(24):7684–92. doi: 10.1158/1078-0432.CCR-09-1587
33. Patterson EE, Holloway AK, Weng J, Fojo T, Kebebew E. MicroRNA Profiling of Adrenocortical Tumors Reveals miR-483 as a Marker of Malignancy. Cancer (2011) 117(8):1630–9. doi: 10.1002/cncr.25724
34. Tombol Z, Szabo PM, Molnar V, Wiener Z, Tolgyesi G, Horanyi J, et al. Integrative Molecular Bioinformatics Study of Human Adrenocortical Tumors: microRNA, Tissue-Specific Target Prediction, and Pathway Analysis. Endocr Relat Cancer (2009) 16(3):895–906. doi: 10.1677/ERC-09-0096
35. Schmitz KJ, Helwig J, Bertram S, Sheu SY, Suttorp AC, Seggewiss J, et al. Differential Expression of microRNA-675, microRNA-139-3p and microRNA-335 in Benign and Malignant Adrenocortical Tumours. J Clin Pathol (2011) 64(6):529–35. doi: 10.1136/jcp.2010.085621
36. Decmann A, Perge P, Nyiro G, Darvasi O, Liko I, Borka K, et al. MicroRNA Expression Profiling in Adrenal Myelolipoma. J Clin Endocrinol Metab (2018) 103(9):3522–30. doi: 10.1210/jc.2018-00817
37. Feinmesser M, Benbassat C, Meiri E, Benjamin H, Lebanony D, Lebenthal Y, et al. Specific MicroRNAs Differentiate Adrenocortical Adenomas From Carcinomas and Correlate With Weiss Histopathologic System. Appl Immunohistochem Mol Morphol (2015) 23(7):522–31. doi: 10.1097/pai.0000000000000117
38. Koperski Ł, Kotlarek M, Świerniak M, Kolanowska M, Kubiak A, Górnicka B, et al. Next-Generation Sequencing Reveals microRNA Markers of Adrenocortical Tumors Malignancy. Oncotarget (2017) 8(30):49191–200. doi: 10.18632/oncotarget.16788
39. Patel D, Boufraqech M, Jain M, Zhang L, He M, Gesuwan K, et al. MiR-34a and miR-483-5p Are Candidate Serum Biomarkers for Adrenocortical Tumors. Surgery (2013) 154(6):1224–8; discussion 9. doi: 10.1016/j.surg.2013.06.022
40. Szabo DR, Luconi M, Szabo PM, Toth M, Szucs N, Horanyi J, et al. Analysis of Circulating microRNAs in Adrenocortical Tumors. Lab Invest (2014) 94(3):331–9. doi: 10.1038/labinvest.2013.148
41. Perge P, Butz H, Pezzani R, Bancos I, Nagy Z, Paloczi K, et al. Evaluation and Diagnostic Potential of Circulating Extracellular Vesicle-Associated microRNAs in Adrenocortical Tumors. Sci Rep (2017) 7(1):5474. doi: 10.1038/s41598-017-05777-0
42. Salvianti F, Canu L, Poli G, Armignacco R, Scatena C, Cantini G, et al. New Insights in the Clinical and Translational Relevance of Mir483-5p in Adrenocortical Cancer. Oncotarget (2017) 8(39):65525–33. doi: 10.18632/oncotarget.19118
43. Perge P, Decmann Á, Pezzani R, Bancos I, Fassina A, Luconi M, et al. Analysis of Circulating Extracellular Vesicle-Associated microRNAs in Cortisol-Producing Adrenocortical Tumors. Endocrine (2018) 59(2):280–7. doi: 10.1007/s12020-017-1506-z
44. Decmann A, Bancos I, Khanna A, Thomas MA, Turai P, Perge P, et al. Comparison of Plasma and Urinary microRNA-483-5p for the Diagnosis of Adrenocortical Malignancy. J Biotechnol (2019) 297:49–53. doi: 10.1016/j.jbiotec.2019.03.017
45. Wang K, Yuan Y, Cho JH, McClarty S, Baxter D, Galas DJ. Comparing the MicroRNA Spectrum Between Serum and Plasma. PloS One (2012) 7(7):e41561. doi: 10.1371/journal.pone.0041561
46. Yamada A, Cox MA, Gaffney KA, Moreland A, Boland CR, Goel A. Technical Factors Involved in the Measurement of Circulating microRNA Biomarkers for the Detection of Colorectal Neoplasia. PloS One (2014) 9(11):e112481. doi: 10.1371/journal.pone.0112481
47. Igaz I, Nyiro G, Nagy Z, Butz H, Nagy Z, Perge P, et al. Analysis of Circulating MicroRNAs In Vivo Following Administration of Dexamethasone and Adrenocorticotropin. Int J Endocrinol (2015) 2015:589230. doi: 10.1155/2015/589230
48. Oreglia M, Sbiera S, Fassnacht M, Guyon L, Denis J, Cristante J, et al. Early Postoperative Circulating miR-483-5p Is a Prognosis Marker for Adrenocortical Cancer. Cancers (Basel) (2020) 12(3):724. doi: 10.3390/cancers12030724
49. Nagy Z, Baghy K, Hunyadi-Gulyás É, Micsik T, Nyírő G, Rácz G, et al. Evaluation of 9-Cis Retinoic Acid and Mitotane as Antitumoral Agents in an Adrenocortical Xenograft Model. Am J Cancer Res (2015) 5(12):3645–58. doi: 10.1530/endoabs.37.GP.29.04
50. Jung S, Nagy Z, Fassnacht M, Zambetti G, Weiss M, Reincke M, et al. Preclinical Progress and First Translational Steps for a Liposomal Chemotherapy Protocol Against Adrenocortical Carcinoma. Endocr Relat Cancer (2016) 23(10):825–37. doi: 10.1530/ERC-16-0249
51. Bancos I, Arlt W. Diagnosis of a Malignant Adrenal Mass: The Role of Urinary Steroid Metabolite Profiling. Curr Opin Endocrinol Diabetes Obes (2017) 24(3):200–7. doi: 10.1097/MED.0000000000000333
52. Pereira SS, Costa MM, Gomez-Sanchez CE, Monteiro MP, Pignatelli D. Incomplete Pattern of Steroidogenic Protein Expression in Functioning Adrenocortical Carcinomas. Biomedicines (2020) 8(8):256. doi: 10.3390/biomedicines8080256
53. Gröndal S, Eriksson B, Hagenäs L, Werner S, Curstedt T. Steroid Profile in Urine: A Useful Tool in the Diagnosis and Follow Up of Adrenocortical Carcinoma. Acta Endocrinol (Copenh) (1990) 122(5):656–63. doi: 10.1530/acta.0.1220656
54. Arlt W, Biehl M, Taylor AE, Hahner S, Libe R, Hughes BA, et al. Urine Steroid Metabolomics as a Biomarker Tool for Detecting Malignancy in Adrenal Tumors. J Clin Endocrinol Metab (2011) 96(12):3775–84. doi: 10.1210/jc.2011-1565
55. Kerkhofs TM, Kerstens MN, Kema IP, Willems TP, Haak HR. Diagnostic Value of Urinary Steroid Profiling in the Evaluation of Adrenal Tumors. Horm Cancer (2015) 6(4):168–75. doi: 10.1007/s12672-015-0224-3
56. Velikanova LI, Shafigullina ZR, Lisitsin AA, Vorokhobina NV, Grigoryan K, Kukhianidze EA, et al. Different Types of Urinary Steroid Profiling Obtained by High-Performance Liquid Chromatography and Gas Chromatography-Mass Spectrometry in Patients With Adrenocortical Carcinoma. Horm Cancer (2016) 7(5-6):327–35. doi: 10.1007/s12672-016-0267-0
57. Hines JM, Bancos I, Bancos C, Singh RD, Avula AV, Young WF, et al. High-Resolution, Accurate-Mass (HRAM) Mass Spectrometry Urine Steroid Profiling in the Diagnosis of Adrenal Disorders. Clin Chem (2017) 63(12):1824–35. doi: 10.1373/clinchem.2017.271106
58. Shafigullina ZR, Velikanova LI, Vorokhobina NV, Shustov SB, Lisitsin AA, Malevanaia EV, et al. Urinary Steroid Profiling by Gas Chromatography Mass Spectrometry: Early Features of Malignancy in Patients With Adrenal Incidentalomas. Steroids (2018) 135:31–5. doi: 10.1016/j.steroids.2018.04.006
59. Bancos I, Taylor AE, Chortis V, Sitch AJ, Jenkinson C, Davidge-Pitts CJ, et al. Urine Steroid Metabolomics for the Differential Diagnosis of Adrenal Incidentalomas in the EURINE-ACT Study: A Prospective Test Validation Study. Lancet Diabetes Endocrinol (2020) 8(9):773–81. doi: 10.1016/s2213-8587(20)30218-7
60. Chortis V, Bancos I, Nijman T, Gilligan LC, Taylor AE, Ronchi CL, et al. Urine Steroid Metabolomics as a Novel Tool for Detection of Recurrent Adrenocortical Carcinoma. J Clin Endocrinol Metab (2020) 105(3):e307–18. doi: 10.1210/clinem/dgz141
61. Khorram-Manesh A, Ahlman H, Jansson S, Wängberg B, Nilsson O, Jakobsson CE, et al. Adrenocortical Carcinoma: Surgery and Mitotane for Treatment and Steroid Profiles for Follow-Up. World J Surg (1998) 22(6):605–11; discussion 11-2. doi: 10.1007/s002689900442
62. Araujo-Castro M, Valderrabano P, Escobar-Morreale HF, Hanzu FA, Casals G. Urine Steroid Profile as a New Promising Tool for the Evaluation of Adrenal Tumors. Literature Review. Endocrine (2021) 72(1):40–8. doi: 10.1007/s12020-020-02544-6
63. Fanelli F, Di Dalmazi G. Serum Steroid Profiling by Mass Spectrometry in Adrenocortical Tumors: Diagnostic Implications. Curr Opin Endocrinol Diabetes Obes (2019) 26(3):160–5. doi: 10.1097/MED.0000000000000475
64. Crona J, Beuschlein F. Adrenocortical Carcinoma - Towards Genomics Guided Clinical Care. Nat Rev Endocrinol (2019) 15(9):548–60. doi: 10.1038/s41574-019-0221-7
65. Taylor DR, Ghataore L, Couchman L, Vincent RP, Whitelaw B, Lewis D, et al. A 13-Steroid Serum Panel Based on LC-MS/MS: Use in Detection of Adrenocortical Carcinoma. Clin Chem (2017) 63(12):1836–46. doi: 10.1373/clinchem.2017.277624
66. Schweitzer S, Kunz M, Kurlbaum M, Vey J, Kendl S, Deutschbein T, et al. Plasma Steroid Metabolome Profiling for the Diagnosis of Adrenocortical Carcinoma. Eur J Endocrinol (2019) 180(2):117–25. doi: 10.1530/eje-18-0782
67. Pinzani P, Scatena C, Salvianti F, Corsini E, Canu L, Poli G, et al. Detection of Circulating Tumor Cells in Patients With Adrenocortical Carcinoma: A Monocentric Preliminary Study. J Clin Endocrinol Metab (2013) 98(9):3731–8. doi: 10.1210/jc.2013-1396
68. Lalli E, Sasano H. 5th International ACC Symposium: An Outlook to Current and Future Research on the Biology of Adrenocortical Carcinoma: Diagnostic and Therapeutic Applications. Horm Cancer (2016) 7(1):44–8. doi: 10.1007/s12672-015-0240-3
69. Ignatiadis M, Lee M, Jeffrey SS. Circulating Tumor Cells and Circulating Tumor DNA: Challenges and Opportunities on the Path to Clinical Utility. Clin Cancer Res (2015) 21(21):4786–800. doi: 10.1158/1078-0432.CCR-14-1190
70. Qin Z, Ljubimov VA, Zhou C, Tong Y, Liang J. Cell-Free Circulating Tumor DNA in Cancer. Chin J Cancer (2016) 35:36. doi: 10.1186/s40880-016-0092-4
71. Alix-Panabieres C, Pantel K. Circulating Tumor Cells: Liquid Biopsy of Cancer. Clin Chem (2013) 59(1):110–8. doi: 10.1373/clinchem.2012.194258
72. Tsoli M, Daskalakis K, Kassi E, Kaltsas G, Tsolakis AV. A Critical Appraisal of Contemporary and Novel Biomarkers in Pheochromocytomas and Adrenocortical Tumors. Biol (Basel) (2021) 10(7):580. doi: 10.3390/biology10070580
73. Sun YF, Yang XR, Zhou J, Qiu SJ, Fan J, Xu Y. Circulating Tumor Cells: Advances in Detection Methods, Biological Issues, and Clinical Relevance. J Cancer Res Clin Oncol (2011) 137(8):1151–73. doi: 10.1007/s00432-011-0988-y
74. Cristofanilli M, Budd GT, Ellis MJ, Stopeck A, Matera J, Miller MC, et al. Circulating Tumor Cells, Disease Progression, and Survival in Metastatic Breast Cancer. N Engl J Med (2004) 351(8):781–91. doi: 10.1056/NEJMoa040766
75. Cantini G, Canu L, Armignacco R, Salvianti F, De Filpo G, Ercolino T, et al. Prognostic and Monitoring Value of Circulating Tumor Cells in Adrenocortical Carcinoma: A Preliminary Monocentric Study. Cancers (Basel) (2020) 12(11):3176. doi: 10.3390/cancers12113176
76. Cai X, Janku F, Zhan Q, Fan JB. Accessing Genetic Information With Liquid Biopsies. Trends Genet (2015) 31(10):564–75. doi: 10.1016/j.tig.2015.06.001
77. Perdigones N, Murtaza M. Capturing Tumor Heterogeneity and Clonal Evolution in Solid Cancers Using Circulating Tumor DNA Analysis. Pharmacol Ther (2017) 174:22–6. doi: 10.1016/j.pharmthera.2017.02.003
78. Thierry AR, El Messaoudi S, Gahan PB, Anker P, Stroun M. Origins, Structures, and Functions of Circulating DNA in Oncology. Cancer Metastasis Rev (2016) 35(3):347–76. doi: 10.1007/s10555-016-9629-x
79. Jiang P, Chan CW, Chan KC, Cheng SH, Wong J, Wong VW, et al. Lengthening and Shortening of Plasma DNA in Hepatocellular Carcinoma Patients. Proc Natl Acad Sci USA (2015) 112(11):E1317–25. doi: 10.1073/pnas.1500076112
80. Bettegowda C, Sausen M, Leary RJ, Kinde I, Wang Y, Agrawal N, et al. Detection of Circulating Tumor DNA in Early- and Late-Stage Human Malignancies. Sci Transl Med (2014) 6(224):224ra24. doi: 10.1126/scitranslmed.3007094
81. Lalli E, Luconi M. The Next Step: Mechanisms Driving Adrenocortical Carcinoma Metastasis. Endocr Relat Cancer (2018) 25(2):R31–48. doi: 10.1530/ERC-17-0440
82. Alix-Panabieres C, Schwarzenbach H, Pantel K. Circulating Tumor Cells and Circulating Tumor DNA. Annu Rev Med (2012) 63:199–215. doi: 10.1146/annurev-med-062310-094219
83. Mizdrak M, Ticinovic Kurir T, Bozic J. The Role of Biomarkers in Adrenocortical Carcinoma: A Review of Current Evidence and Future Perspectives. Biomedicines (2021) 9(2):174. doi: 10.3390/biomedicines9020174
84. Creemers SG, Korpershoek E, Atmodimedjo PN, Dinjens WNM, van Koetsveld PM, Feelders RA, et al. Identification of Mutations in Cell-Free Circulating Tumor DNA in Adrenocortical Carcinoma: A Case Series. J Clin Endocrinol Metab (2017) 102(10):3611–5. doi: 10.1210/jc.2017-00174
85. Garinet S, Nectoux J, Neou M, Pasmant E, Jouinot A, Sibony M, et al. Detection and Monitoring of Circulating Tumor DNA in Adrenocortical Carcinoma. Endocr Relat Cancer (2018) 25(3):L13–L7. doi: 10.1530/ERC-17-0467
86. Angeli A, Osella G, Alì A, Terzolo M. Adrenal Incidentaloma: An Overview of Clinical and Epidemiological Data From the National Italian Study Group. Horm Res (1997) 47(4-6):279–83. doi: 10.1159/000185477
87. Iniguez-Ariza NM, Kohlenberg JD, Delivanis DA, Hartman RP, Dean DS, Thomas MA, et al. Clinical, Biochemical, and Radiological Characteristics of a Single-Center Retrospective Cohort of 705 Large Adrenal Tumors. Mayo Clin Proc Innov Qual Outcomes (2018) 2(1):30–9. doi: 10.1016/j.mayocpiqo.2017.11.002
88. Elmohr MM, Fuentes D, Habra MA, Bhosale PR, Qayyum AA, Gates E, et al. Machine Learning-Based Texture Analysis for Differentiation of Large Adrenal Cortical Tumours on CT. Clin Radiol (2019) 74(10):818.e1–e7. doi: 10.1016/j.crad.2019.06.021
Keywords: adrenocortical carcinoma, non-invasive biomarker, microRNA, steroid profiling, diagnosis, prognosis, disease monitoring
Citation: Cheng Y, Kou W, Zhu D, Yu X and Zhu Y (2022) Future Directions in Diagnosis, Prognosis and Disease Monitoring of Adrenocortical Carcinoma: Novel Non-Invasive Biomarkers. Front. Endocrinol. 12:811293. doi: 10.3389/fendo.2021.811293
Received: 08 November 2021; Accepted: 23 December 2021;
Published: 01 February 2022.
Edited by:
Toru Hosoi, Sanyo-Onoda City University, JapanReviewed by:
Matthias Kroiß, Ludwig Maximilian University of Munich, GermanyFilippo Ceccato, University of Padua, Italy
Copyright © 2022 Cheng, Kou, Zhu, Yu and Zhu. This is an open-access article distributed under the terms of the Creative Commons Attribution License (CC BY). The use, distribution or reproduction in other forums is permitted, provided the original author(s) and the copyright owner(s) are credited and that the original publication in this journal is cited, in accordance with accepted academic practice. No use, distribution or reproduction is permitted which does not comply with these terms.
*Correspondence: Yu Zhu, enkxMDQ3OEByamguY29tLmNu