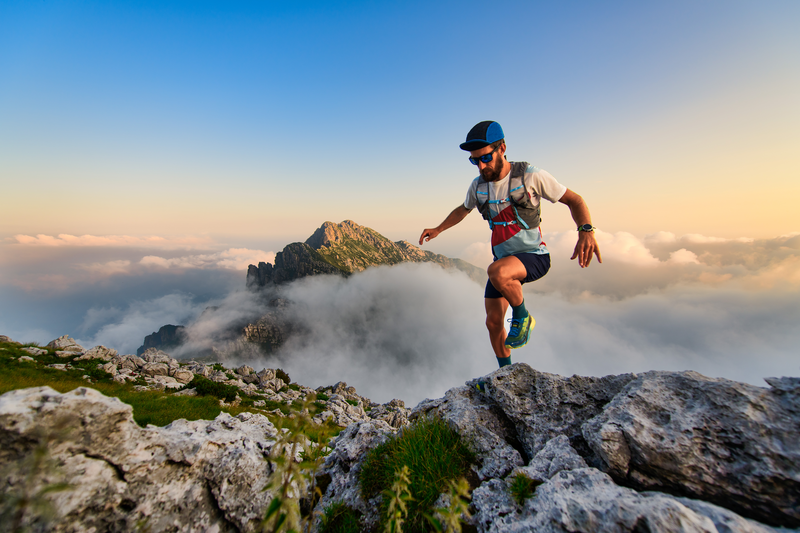
94% of researchers rate our articles as excellent or good
Learn more about the work of our research integrity team to safeguard the quality of each article we publish.
Find out more
REVIEW article
Front. Endocrinol. , 11 November 2021
Sec. Obesity
Volume 12 - 2021 | https://doi.org/10.3389/fendo.2021.778737
This article is part of the Research Topic Obesogens in the XXI century: emerging health challenges View all 8 articles
The exponential global increase in the incidence of obesity may be partly attributable to environmental chemical (EC) exposure. Humans are constantly exposed to ECs, primarily through environmental components. This review compiled human epidemiological study findings of associations between blood and/or urinary exposure levels of ECs and anthropometric overweight and obesity indices. The findings reveal research gaps that should be addressed. We searched MEDLINE (PubMed) for full text English articles published in 2006–2020 using the keywords “environmental exposure” and “obesity”. A total of 821 articles were retrieved; 102 reported relationships between environmental exposure and obesity indices. ECs were the predominantly studied environmental exposure compounds. The ECs were grouped into phenols, phthalates, and persistent organic pollutants (POPs) to evaluate obesogenic roles. In total, 106 articles meeting the inclusion criteria were summarized after an additional search by each group of EC combined with obesity in the PubMed and Scopus databases. Dose-dependent positive associations between bisphenol A (BPA) and various obesity indices were revealed. Both individual and summed di(2-ethylhexyl) phthalate (DEHP) and non-DEHP metabolites showed inconsistent associations with overweight and obesity indices, although mono-butyl phthalate (MBP), mono-ethyl phthalate (MEP), and mono-benzyl phthalate (MBzP) seem to have obesogenic roles in adolescents, adults, and the elderly. Maternal exposure levels of individual POP metabolites or congeners showed inconsistent associations, whereas dichlorodiphenyldichloroethylene (DDE) and perfluorooctanoic acid (PFOA) were positively associated with obesity indices. There was insufficient evidence of associations between early childhood EC exposure and the subsequent development of overweight and obesity in late childhood. Overall, human evidence explicitly reveals the consistent obesogenic roles of BPA, DDE, and PFOA, but inconsistent roles of phthalate metabolites and other POPs. Further prospective studies may yield deeper insights into the overall scenario.
Obesity is characterized by excess body fat, total body fat, or a particular depot of body fat (1). The most commonly evaluated anthropometric indices of obesity are body mass index (BMI), waist circumference (WC), hip circumference (HC), skinfold thickness (ST), percent body fat (%BF), fat mass (FM), and waist-to-height ratio (WHtR) (2–5). An adult individual is overweight if BMI ≥25 kg/m2 to <30 kg/m2, and obese if BMI ≥30 kg/m2 or WC ≥80 cm in women and WC ≥90 cm in men (6). Childhood overweight and obesity can be defined as BMI z-scores >1 and >2, respectively (3, 4, 6). Sex- and age-specific WC ≥90th percentile or WHtR ≥0.5 are also used to determine obesity in children (7, 8). Some alternative measurements are still available for both children and adults, and differ with age, gender, and country (9).
Whether obesity should be declared a disease is controversial (1). However, obesity leads to many aspects of ill health or functional impairment and several diseases (10–13), reduces health quality of life (14, 15), and increase mortality and morbidity (16–18). It is a complex condition with many causal contributors, including genetic factors and environmental factors (19–21). Recent epidemiological research has also reported the associations with overweight and obesity of environmental exposure sources that include environmental chemicals (ECs), air pollution, particulate matter, heavy metals, noise, green space, and others (22–31). According to the “obesogen hypothesis,” ECs, which are termed environmental obesogens (EOs), regulate lipid metabolism and adipogenesis, leading to obesity (32).
Over time, the use of synthetic chemicals has grown exponentially with the development of commerce and industry (33). Excessive usage results in environmental contamination. Humans are exposed to these ECs through environmental media by ingestion, inhalation, absorption, and even through transplacental transfer and breast milk (34–42). The human exposure levels of these ECs are generally estimated by biomonitoring of their metabolites or parent compounds in human urine or blood (cord blood or peripheral blood) as exposure biomarkers worldwide (43–47).
Recently, there has been increased interest in epidemiological studies of EC biomonitoring and subsequent evaluation of their obesogenic effects (4, 8, 34, 48–51). A concise view of the overall epidemiological findings is required to clarify whether obesogenic evidence of ECs is sufficient or consistent for the advancement of future research. Some previous reviews have explored the obesogenic role of ECs. However, most of these considered only a single group of ECs, and/or selected ECs based on their endocrine-disrupting properties, and/or considered limited exposure and outcome assessment period or age, and even not focused on epidemiological studies, and/or focused on a mechanism (52–59).
A further review addressing the current epidemiological evidence of the obesogenic effects of ECs at all stages of life from a public health perspective is needed. Accordingly, the objectives of the present review are to illuminate epidemiological study findings of the associations between EC exposure and anthropometric overweight and obesity indices, uncover the current research gap, and contemplate future research.
Research articles that demonstrated the associations between environmental exposure and obesity in MEDLINE of PubMed were searched for using “environmental exposure” AND “obesity” as keywords to select EOs (Figure 1). After additional filtering for full text, journal articles, inclusion of humans, English, and publication year (2006–2020), a total of 821 articles were retrieved. Of these, 719 articles were excluded owing to the following reasons: abstract not available (n=10); involved clinical trials (n=7), review/systematic review/meta-analysis (n=299); cell line studies (n=12); animal studies and statistical/computational models (n=21); editorial/commentary/protocol and approach (n=19); investigated associations of EC exposure with other adverse outcomes, including hypertension, puberty, diabetes, polycystic ovary syndrome, cardiovascular diseases, cancer risk, and others, and simple biomonitoring and ecological studies (n=351). In the remaining 102 articles, the ECs were predominantly studied environmental exposure (ECs = 62 and others = 40). Also, the production and uses of agricultural, industrials, and other synthetic chemicals are increasing, and recognized as major environmental pollutants over other environmental exposures namely heavy metals, noise or sound, green space and particulate matters. Therefore, we selected ECs as the major EOs apart from other environmental exposure and grouped them as follows: (i) phenols [bisphenol A (BPA), bisphenol S (BPS), bisphenol F (BPF), and others], (ii) phthalates (all phthalates and their metabolites), and (iii) persistent organic pollutants (POPs) [organochlorine compounds (OCs), polybrominated diphenyl ethers (PBDEs) and per- and polyfluoroalkyl substances (PFASs), and their metabolites or congeners] (Figure 1).
A primary search in PubMed and Scopus databases for each group of EO used the keywords “bisphenols” AND “obesity,” “phthalate” AND “obesity”, and “persistent organic pollutants” AND “obesity” to identify original research articles of human epidemiological studies. Additional PubMed filtering and Scopus refining were performed to select relevant articles (Figure 2). Articles were considered relevant when they investigated the associations of selected EOs with anthropometric overweight and obesity indices. The references of the selected primary research articles were also searched for relevant publications. A secondary search was also performed for each group of POPs combined with obesity (Figure 2).
Figure 2 Schematic diagram of study selection. *Cell line studies, animal/rodent/drosophila studies, investigation of other associations (e.g., growth, metabolic syndrome, fatty liver disease, diabetes, cardiometabolic risk, inflammation, polycystic ovary syndrome, prostate cancer, food intake, semen quality, puberty), ecological studies, and or simple biomonitoring studies.
All full-length articles, short communications, and brief reports of original research work from all over the world, irrespective of sex, religion, and race/ethnicity, were included in this review (Figure 1). Inclusion criteria included (i) epidemiological study (cohort, cross-sectional, and case-control); (ii) all ages and/or life-stage at exposure or outcome assessment; (iii) primary outcomes of overweight and/or obesity, or at least one anthropometric index of overweight or obesity; (iv) EO concentrations measured in urine or blood as human biomonitoring; (v) assessment of only non-occupational exposure levels of EOs; (vi) published after postulating “obesogen hypothesis”; and (vii) written in English. All other articles were excluded (Figure 2). Finally, 106 original research articles were included in this review.
Associations of EOs with overweight and/or obesity have been demonstrated in the aforementioned three groups. We grouped the early- and later-life exposure and outcome assessment age into seven categories (Matrix Tables 1–6): infants (up to 1 year), toddlers (>1– 2 years), preschoolers (>2– 5 years), school-aged (>5– 13 years), adolescents (>13– 19 years), adults (≥20– 60 years), and elderly (>60 years) as classified previously (60). Matrix tables were created according to categories.
We summarized a total of 33 human epidemiological studies, including 13 cohort studies and 20 cross-sectional studies that explored the association between prenatal and early- to later-life urinary phenols, especially bisphenol exposure levels with anthropometric overweight and obesity indices (Table 1 and Matrix Table 1). Most of the cohort studies were birth cohorts. The study subjects enrolled in the birth cohorts ranged from 173 to 1128 mother-child pairs. Among the 20 cross-sectional studies, 9 involved children and adolescents between the ages of 3 and 19 years, and 11 involved adults and elderly participants >18 years. Both the cohort and cross-sectional studies measured BPA, BPS, and BPF in spot urine other than the first morning void urine, or 24 h urine.
Maternal urinary BPA levels showed null or positive associations with one or more anthropometric obesity indices in infants and toddlers (61, 73, 80). Similar associations were also found between maternal BPA exposure levels and obesity measures in preschoolers and school-aged children. These associations were sex-specific (8, 50, 61, 70–73, 78, 80). Only one study reported negative associations between prenatal BPA exposure and BMI z-score and %BF (79). Toddler and preschooler exposure levels of BPA reported null or positive associations with overweight or obesity indices in toddlers, preschoolers, and school-aged children (8, 70, 72, 73, 78, 79). Associations were mostly null in children 5 to 9 years of age (72, 79). Urinary BPA concentrations among school-aged children showed inconsistent relationships with one or more obesity indices (8, 63, 68, 79, 81). However, several studies recruited children with ages ranging from 6 to 19 years and investigated the associations of urinary BPA, BPS, and BPF exposure levels with overweight and obesity indices. All these studies found positive associations with one or more anthropometric parameters of obesity (7, 34, 62, 66, 82, 83, 85). Adult exposure levels of BPA, BPS, and BPF were also positively associated with at least one anthropometric index of obesity in adults and elderly individuals (2, 41, 48, 64, 65, 67, 69, 75–77, 84, 86) with the exception of inconsistent associations in one study (63). One panel study (cohort) investigated the association between urinary BPA concentrations and overweight. The authors reported a positive association in the case of overall and female study participants, but not in male participants (74). Some other studies also observed a sex-stratified relationship between prenatal bisphenol exposure and overweight and obesity indices (8, 61, 72, 79). A few studies reported sex-dependent associations between childhood bisphenol exposure levels and obesity or adiposity measures (7, 66, 81). Race- or ethnicity-specific associations of urinary BPA concentrations with obesity indices were also reported, with a significant association of BPA levels only in non-Hispanic white subjects (83). Pubertal status was reported as a confounder of the associations between BPA concentrations and BMI, WC, and ST, especially in girls (70, 79). Maternal exposure levels of 2, 5-dichlorophenol, benzophenone-3, and triclosan showed null associations with %FM in children aged 4–9 years (71). In contrast, one study reported positive associations between urinary 2, 5-dichlorophenol levels in children aged 6–8 years and BMI, WC, and %BF in later childhood, which consistently increased up to 13 years of age (68).
BPA levels in urine varied among the studies and ranged from non-detectable to >2594 ng/ml (Table 1). Children and adolescents (6–19 years) with urinary BPA, BPS, and BPF concentrations of ≥2, ≥1.30, and ≥0.2 ng/ml, are susceptible to developing overweight or obesity (7, 34, 62, 81). In adults, BPA, BPS, and BPF showed obesogenic effects at concentrations ≥0.71, ≥1, and 1 ng/ml, respectively (41, 64, 67, 69, 77, 86). In addition, BPA concentrations ≥0.39 ng/ml may be responsible for subsequent development of overweight or obesity in elderly people (74).
A total of 32 studies (11 birth cohort, 19 cross-sectional, and 2 case-control studies) explored the association of both prenatal and postnatal urinary exposure levels of phthalate metabolites with overweight and obesity measures in human populations of different ages (Table 2, and Matrix Tables 2, 3). In the birth cohort studies, urine samples were collected from both the pregnant mother and their children aged 1–14 years. The study subjects ranged from 128 to 1128 mother-child pairs in the birth cohorts. Among the 19 cross-sectional studies, 11 involved children and adolescents, 8 involved only adults and elderly people (male and/or female) of different ages. Almost all the studies determined phthalate metabolites in the spot urine of the study participants.
Table 2 Associations of environmental phthalates with anthropometric overweight and obesity indices.
Associations between maternal 1st trimester DEHP exposure levels and obesity measures in preschoolers, school-aged children, and adolescents were inconsistent (50, 70, 87, 93). Similarly, the individual or sum of maternal 2nd trimester urinary DEHP metabolites showed both positive and null associations with different obesity indices in preschoolers, school-aged children, and adolescents (50, 87, 95, 96). However, negative or null associations were found between the 2nd and 3rd trimester DEHP exposure levels and anthropometric obesity indices in infants, preschoolers, school-aged children, and adolescents (50, 87, 98–101). Infant (1 year) exposure to DEHP was negatively associated with obesity indices at 8 years of age. In contrast, preschoolers exposed to DEHP (4 and 5 years) were negatively or positively associated (93, 96). Associations of DEHP exposure levels at 6–19 years of age (individual metabolite levels or sum of levels) with overweight or obesity indices in school-aged children and adolescents were very inconsistent (89–92, 94, 96, 102, 105–111). Most of the studies that recruited adults and elderly people reported positive or null associations between one or more DEHP metabolites or the sum of DEHP and different overweight and obesity indices in overall adult and elderly populations or after sex stratification (2, 48, 49, 65, 88, 97, 104, 106, 111, 112).
Similar inconsistent associations were also found among non-DEHP metabolites [mono-butyl phthalate (MBP), mono-ethyl phthalate (MEP), mono-methyl phthalate (MMP), mono-benzyl phthalate (MBzP), mono-isobutyl phthalate (MiBP), mono-(carboxylnonyl) phthalate (MCNP), mono-isononyl phthalate (MINP), and others], and obesity indices at different stages of life. First to 3rd-trimester maternal urinary concentrations of non-DEHP metabolites (except MCPP) displayed null or negative associations with anthropometric parameters of obesity in preschoolers, school-aged children, and adolescents (50, 70, 93, 98, 99, 101, 103). In contrast, one study found positive associations between maternal urinary concentrations of MEP, MBP, MBzP, and MiBP and obesity indices among all study participants (95). Another study also found positive associations after sex-stratified analysis in both males (MBzP) and females (MiBP and MBP) (87). Exposure levels of non-DEHP metabolites in toddlers and preschoolers showed null associations with their obesity measures (96, 101). However, one study described positive associations between MEP, MBP, and MBP, and obesity indices in girls, with negative associations in boys (93). Exposure levels of school-aged to adolescents to non-DEHP metabolites (MMP, MEP, MBP, MiBP, and MBzP) were mostly positively associated with one or more anthropometric indices in school-aged children or adolescents (89, 91, 92, 94, 96, 102, 105–110). In contrast, after sex stratification, inconsistent associations were evident (94, 102, 107, 110). One study recruited subjects 6–80 years old and found inconsistent associations among non-DEHP metabolite concentrations at different exposures (6-11, 12-19, 20-59 and 60-80 y) and corresponding outcome assessment ages (111). Exposure levels of non-DEHP metabolites in adults and the elderly also showed null or positive associations with their overweight and obesity indices (2, 48, 49, 65, 88, 97, 104, 106, 112). One study evaluated ethnicity-dependent association and found that higher maternal urinary concentrations of MCPP heightened the odds of being overweight or obese in Hispanics than in non-Hispanic blacks, although null associations were found with BMI (99). Prepubertal girls showed positive associations between %MEHHP and BMI, WC, and %BF, and showed significant odds increase in the 3rd and 4th quartiles compared to the 1st quartile. The relationship was null in pubertal girls (90).
Data regarding the lowest threshold levels of phthalate metabolites for overweight or obesity outcomes in humans are limited. Low molecular weight phthalate (LMWP) metabolite concentrations ≥0.27 µmol/ml were associated with significantly increased overweight or obesity indices in male children and adolescents (106). Another study reported increased BMI and WC for median urinary MEP concentrations ≥131 and ≥948 µg/g creatinine, respectively (110).
A total of 41 human epidemiological studies (33 cohort and 8 cross-sectional studies) explored the relationships between in utero and early life exposure to POPs and anthropometric indices of overweight and obesity among infants, children, adults, and elderly populations (Table 3). The studies assessed POP levels in blood (serum/plasma) or umbilical cord blood (whole blood, serum/plasma).
Table 3 Associations of environmental persistent organic pollutants with anthropometric overweight and obesity indices.
A total of 8 epidemiological studies (7 cohort and one cross-sectional study) investigated the associations of several PBDEs with anthropometric measures of obesity along with other POPs in children, adults, and elderly individuals. Inconsistent associations were documented (Table 3 and Matrix Table 4). PBDE congeners, including BDE28, BDE47, BDE99, BDE100, BDE153, and BDE154, were mainly associated with obesity indices. In most of the included studies, the BDE153 congener was negatively associated with one or more overweight or obesity indices in children and adults (4, 114, 117, 123, 127). All other PBDE congeners (BDE28, BDE47, BDE99, BDE100, BDE154, BDE209, and sum of PBDE), except BDE154, showed null associations with obesity indices (4, 103, 114, 117, 127, 141, 145). One study instead showed a positive association between BDE47 and BMI in adults ≥18 years of age (117). Associations of PBDE congeners with obesity in elderly people aged ≥70 years were null in two separate studies (141, 145). Early childhood exposure to PBDEs was negatively associated (BDE153) or inconsistent (others) with obesity indices, especially BMI and WC, at 7 years of age (114, 127).
Eleven birth cohort studies investigated the associations between in utero or maternal and childhood exposure to PFAS and obesity indices. Associations between maternal exposure levels of PFAS metabolites and obesity indices were inconsistent (Table 3 and Matrix Table 5). First- to 2nd-trimester exposure levels of PFOS and PFOA showed inconsistent associations with the obesity indices of infants and toddlers (51, 146). In contrast, almost all studies found positive associations between maternal PFOA concentrations and different obesity indices in overall and/or sex-stratified populations of preschoolers and school-aged children (3, 115, 121, 122, 124), with two exceptions: in utero PFOA exposure showed a null association with BMI, WC, and overweight in school-aged children (120, 137). In contrast, PFOS and other PFAS levels were inconsistently associated with anthropometric measures of overweight and obesity in preschoolers and school-aged children (113). Prenatal exposure levels of PFOA were positively associated only in adult females, but the associations were null for all PFASs when considering the overall population (138). However, exposure levels of PFAS in preschoolers and school-aged children mostly showed null or negative associations with overweight or obesity indices (3, 120, 121). Gestational exposure levels of PFOA ≥4.3–6.4 ng/ml were associated with increased WC in the children 2–8 years of age (122). In contrast, 1st and 2nd trimester exposure levels of PFOA (0.5– ≤7.10 ng/ml in boys and 1.10 to ≤6.70 ng/ml in girls) showed null associations with BMI or overweight at 7 years of age (137).
Positive, negative, or null associations have also been reported between in utero or prenatal and postnatal, and between early childhood to the elderly concerning exposure to OCs and overweight and obesity indices (Table 3 and Matrix Table 6) (103, 115–119, 121, 125, 126, 128–136, 139–145, 147–149). Maternal 1st to 3rd trimester blood and/or umbilical cord blood levels of OC metabolites, especially DDE and HCB levels, were positively associated with different anthropometric indices of obesity, whereas associations of PCBs, DDT metabolites, and β-HCH concentrations were null or positive in toddlers and preschoolers (115, 116, 126, 132, 142, 148). Inconsistent associations (positive and null) were also found between PCBs, DDT metabolites, DDE, HCB, and β-HCH levels in the 1st to 3rd trimester maternal blood or umbilical cord blood and obesity indices in school-aged children (103, 118, 130, 133–136, 139, 149). One study found positive associations of 2-week postpartum HCB levels, but not other OCs, with anthropometric indices in 18-month-old and 5-year-old children (121). Among the OCs, DDT and its metabolite DDE showed potent positive associations with obesity indices in the overall population or in school-aged boys and girls (103, 118, 130, 134, 135). Only one study investigated the relationship between prenatal exposure levels of DDE and adult obesity measures, and subsequently addressed the positive associations of adults aged 20–50 years. PCBs showed null associations in the same study participants (147). Again, associations sometimes varied among the countries within the study. A prospective cohort study of 412 Norwegian and Swedish mother-child pairs observed a non-monotonic dose-response relationship between PCB-153 concentrations and child overweight/obesity among Swedish children at 5 years of age, but not in Norwegian children (115). Exposure levels of PCB153 and DDE metabolites in infants were not associated with obesity measures in preschool and school-aged children (133). Early childhood or preschooler exposure levels of HCB, DDE, and PCBs were negatively associated with anthropometric parameters in preschoolers (121). School-aged exposure levels of PCBs, DDE, and HCB showed inconsistent associations with obesity indices in school-aged children, adolescents, and adults (125, 129). Exposure levels of DDE and β-HCH in adults (≥18 years) showed positive associations, PCBs showed inconsistent associations (positive and negative), and other OCs also showed null associations with different anthropometric indices of overweight and obesity (117, 119, 128, 131, 143, 144). OC exposure in elderly people also showed contradictory findings. DDE exposure levels showed positive or null associations, whereas PCBs showed very inconsistent associations (positive, negative, and null) with anthropometric indices in elderly people aged 50 to 75 years (140, 141, 145). Furthermore, cord blood HCB levels >1.03 ng/ml were associated with increased BMI in children at 6.5 years of age (149).
We present evidence of the relationship between urinary/blood levels of selected EOs and their metabolites or congeners, and anthropometric overweight and obesity indices. These relationships are contentious. Prenatal or in utero, newborn, and early childhood to elder life exposure to selected EOs might contribute to the development of adiposity at different stages of life, although the findings were inconsistent depending on exposure and outcome assessment periods. Some studies have clarified positive associations, whereas other studies described negative or null associations for the same EO exposure levels and the subsequent anthropometric indices of obesity (Tables 1–3).
A representative example is two separate birth cohort studies from China and the United States (8, 72) with almost the same number of children (430 and 408). The studies indicated contradictory associations of BPA concentrations at age 3 years with anthropometric obesity indices at age 7 years. The study from China found positive associations, whereas the US study found null associations, despite the same exposures and outcome ages (8, 72). Many other studies have also reported contradictory findings among the same exposure and outcome age groups (Tables 1–3). In contrast, some studies conducted in different countries recruiting different populations reported similar associations between the same or different EO and obesity outcomes (7, 34, 62, 82, 83, 89, 92). These conflicting findings across studies might be explained by methodological variations, particularly the characteristics of the study populations. Other potential reasons are exposure levels (low, medium, or high) and the timing and duration of EO exposure. Associations seem to differ between boys and girls, adult males, and females (Tables 1–3). Some studies reported ethnicity-specific associations between EO exposure and obesity indices (83, 99, 112). The reasons for racial and ethnic differences in overweight and obesity are largely unknown. Possible reasons might be the different patterns of calorie intake or energy consumption, physical activity, metabolic activity, endocrine, and genetic susceptibility among racial and ethnic groups (150, 151).
Among the environmental phenols, BPA has been widely investigated and has been positively associated with anthropometric overweight and obesity indices, mainly in school-aged children, adolescents, and adults. The use of BPA has been decreasing to reduce its negative health impact. This has led to increased use of BPS and BPF. Several studies investigated the association of BPS and BPF with obesity measures and described inconsistent relationships (7, 34, 48, 50, 67). These few studies might be insufficient to conclusively determine the reason for the contradictory associations. Both DEHP and non-DEHP metabolites showed inconsistent associations with overweight and obesity indices at different stages of life. Among the non-DEHP metabolites, MEP, MMP, and MBzP seem to have obesogenic roles in adult and elderly humans. Among the POPs, DDE and PFOA showed almost consistent positive associations with obesity. PFOS also seems to be positively associated with obesity measures, but the associations were sometimes inconsistent. Compared with DDE, DDT showed a weaker association with obesity indices. Although DDT and DDE have already been banned in many countries, the long half-lives of these EOs (7 and 10 years for DDT and DDE, respectively) in both the environment and humans might be responsible for the adverse effects (152–157). Similarly, PFAS metabolites are also very persistent in the environment (half-lives of 3–10 years) and humans are exposed through ingestion of contaminated food, drinking water, and ingestion or inhalation of PFAS from contaminated dust and soil, and even via transplacental and breast milk passage from mother to child (158–163).
Usually, a single EO or a group of similar EOs was included in previous studies, making the results straightforward and easily interpretable. The rising concern is that generalized linear regression can provide a simple relationship between a single chemical or a group of similar chemicals and outcomes, but cannot explore the joint effect of mixed exposure (48). In addition, to study causality, researchers need to consider mixed environmental exposures and their complex nonlinear interactions. Eventually, ignoring the joint effects of other chemicals could contribute to false-positive or false-negative results (164). We found only a limited number of studies that investigated the associations between cumulative exposure to EOs and overweight and obesity indices using a multipollutant approach. Findings were inconsistent (48, 103, 116, 117). In one study, the associations of phthalate metabolites and bisphenols with obesity indices varied when considering single and cumulative exposure levels using three different statistical models (48). Thus, the application of a multipollutant statistical model to clarify the joint effects of mixed EOs should be accepted and utilized to explore the effect of a cumulative exposure burden on the outcomes in one direction per occasion, and the exposure-response function of each chemical, while controlling other chemicals at certain levels.
Some EOs (e.g., bisphenols and phthalates) are lipophilic. They can accumulate in adipose tissue of obese women and can influence the development of obesity in their offspring. A recent population-based prospective cohort of 1396 mothers showed that women in highest group of pre-pregnancy BMI (>30kg/m2) had significant higher concentrations of BPS [OR=0.15 (0.01, 0.27)] total bisphenols (sum of BPA, BPS, and BPF) [OR= 1.88 (0.13, 4.78)], phthalic acid [OR=13.16 (2.51, 29.86)], high molecular weight phthalate (HMWP) [OR=46.73 (14.56, 93.72)] and DEHP [OR=32.34 (6.90, 70.75)] concentrations in comparison to women in normal pre-pregnancy BMI (20–24.9kg/m2) group (165). Another study found that prenatal exposure to PCBs (>1.95 µg/g lipid) was associated with increased BMI in girls from overweight mothers, but not in normal-weight mothers (130). Thus, pre-pregnancy BMI is an important confounder that must be considered when investigating obesity outcomes in a birth cohort. Three studies considered pre-pregnancy BMI as a confounder in birth cohorts (103, 132, 142). Adjustment of pre-pregnancy BMI might shed light on the relationship between EO exposure and obesity.
Daily consumable items (diet or foods and personal care products) are an important route of exposure to several EOs and are intrinsically related to energy balance. BPA and phthalate exposures occur primarily through ingestion and dermal absorption, as these compounds are found in common consumer goods, such as food containers, children’s toys, and personal care products (166–169). Thus, it can be predicted that those who consume or use more of these products are more likely to have higher exposure levels and, perhaps, are more likely to be obese. Several studies reported a direct link between dietary exposure to EOs and obesity (41, 83, 85). Most of the studies in the current review considered diet, calorie intake, energy consumption, and physical activity as potential confounders to address the relationships that strengthen the findings (Tables 1–3).
Puberty features hormonal transition. Both girls and boys undergo physical changes. Puberty has been associated with the development of obesity (170). Several studies evaluated the relationship between EOs and anthropometric measures of obesity in an age- and sex-specific manner before and after puberty (7, 61, 66, 72, 73, 78, 79, 81, 96). A sex-stratified analyses found that increased exposure to urinary concentrations of BPA was positively associated with the sum of skinfold thickness (ST) in girls, while exposure to MEHP, MEHHP, MECPP, and MEOHP were inversely related to BMI z-score, WC, and the sum of ST in boys (70). However, when the analyze was restricted to children who had not yet begun the pubertal transition, the results shifted and showed positive relationships between BPA in girls and MEHP in boys with the sum of ST. In the prenatal exposure period, the authors observed an inverse relationship between MBzP and a child’s BMI z-score, but this finding did not persist when the analyses were restricted to children prior to puberty. In a case-control study, prepubertal girls showed positive associations between %MEHHP and BMI, WC, and %BF, and showed significantly increased odds in the 3rd and 4th quartiles compare to the 1st quartile, whereas the relationship was null in pubertal girls (90). How the associations differ before and after puberty is not yet clearly understood. Knowledge of hormone levels related to pubertal growth, including thyroid hormones, leptin, adiponectin, and others, might provide more insight into the potential mechanisms of EO-mediated s adiposity (171).
One of the limitations of the birth cohort studies outlined here is the use of single spot urine during the 1st, 2nd, or 3rd trimester to estimate EO exposure. The biological half-lives of some of these chemicals are short and they are quickly excreted in urine (e.g., phthalates and bisphenols). Epidemiologists ideally prefer to use 24 h urine and repeated urine sampling when assessing these chemicals in relation to obesity, which occurs incrementally over time and has a multifactorial etiology (172, 173). The time of the day or season could account for some intrapersonal or interpersonal variations in urinary concentrations of analytes in single spot urine samples (174–177). However, single spot is the conventional test, despite these methodological limitations. In most of the included studies, biomonitoring EOs were done using methods lacking validated external quality assurance. Maintaining internal and external quality control and quality assurance might make study findings comparable and could strengthen the findings. Some studies had very limited information on pre-pregnancy BMI due to the availability of self-reported weight and the timing of recruitment in their original birth cohorts. These studies relied on maternal BMI. However, most of the studies collected data using self-reported questionnaires or home visits. Therefore, under- or over-estimated data could not be avoided. There was little or no data of phthalate metabolite (both DEHP and non-DEHP) levels and subsequent obesity assessment in infants and toddlers (Matrix Tables 2, 3). In addition, the assessment of obesity in adolescents related to OC (DDT, DDE, β-HCH, HCB, and PCBs) exposure was insufficient (Matrix Table 6). Very few studies investigated the relationship between cumulative EO exposure levels and overweight or obesity indices. Therefore, the possibility that prenatal and/or postnatal exposure to other unmeasured chemicals correlated with measured chemicals may have confounded the associations under study cannot be excluded. Finally, there are scant data concerning the trajectories of exposure and outcome assessments.
Environmental epidemiologists should clearly infer whether exposure to ECs might influence weight gain or obesity, or whether obese study participants might have greater exposure to, or excretion of, ECs by conducting long-term follow-up studies in child and adult populations. Further prospective studies should aim to collect data with repeated measures over extended periods to improve exposure classification, increase general understanding of the timing of exposure, and address the temporal relationship between ECs and obesity. Given the gradual decrease of some ECs and increase exposure to some alternate ECs in human populations, continued biomonitoring of these alternate ECs and further investigations on their obesogenic effects in humans could be undertaken. Researchers should target study participants at all stages of life to assess exposure and obesity outcomes at each age. Many other chemicals, including pesticides, heavy metals, and particulate matter, have been reportedly associated with obesity outcomes in vitro and in vivo in animal studies. However, their obesogenic effects have not yet been completely evaluated in humans (178–186). Thus, an exposome-based approach needs to be developed to investigate the possible obesogenic effects of chemicals, xenobiotics, and pollutants in humans to explore the overall scenario of cumulative exposure. Studies of the obesogenic effects of ECs in the context of diet, stress, ethnicity, gender, and other factors, using sophisticated statistical models to assess complex exposures should be done.
The collective data indicate that BPA, DDE, and PFOA have consistent obesogenic effects in humans. Other bisphenols, phthalates, and POP metabolites or congeners have contradictory relationships with obesity at different outcome assessment times. Further prospective cohort studies with cumulative exposure assessments are required. The findings of this review will increase the awareness of the obesogenic effects of ECs among the general population.
NCM: investigation, data curation, analysis, and writing-original draft. SK: data curation and writing–review and editing. YI and MK: conceptualization, project administration, funding acquisition, supervision, and writing–review and editing. All authors contributed to the article and approved the submitted version.
This research was conducted with the support of the Grants-in-Aid for Scientific Research 19H01078 and 19H03888 provided by the Japan Society for the Promotion of Science (JSPS).
The authors declare that the research was conducted in the absence of any commercial or financial relationships that could be construed as a potential conflict of interest.
All claims expressed in this article are solely those of the authors and do not necessarily represent those of their affiliated organizations, or those of the publisher, the editors and the reviewers. Any product that may be evaluated in this article, or claim that may be made by its manufacturer, is not guaranteed or endorsed by the publisher.
The first author is grateful to the Department of Biochemistry and Molecular Biology, Shahjalal University of Science and Technology, Sylhet, Bangladesh, for approval of his study leave.
The Supplementary Material for this article can be found online at: https://www.frontiersin.org/articles/10.3389/fendo.2021.778737/full#supplementary-material
BF, body fat; BMI, body mass index; BPA, bisphenol A; BPF, bisphenol F; BPS, bisphenol S; β-HCH, beta-hexachlorocyclohexane; DBP, dibutyl phthalate; DDT, dichlorodiphenyltrichloroethane; DDE, dichlorodiphenyldichloroethylene; DEHP; di(2-ethylhexyl) phthalate; EC, environmental chemical; EO, environmental obesogen; FM, fat mass; HC, hip circumference; HCB, hexachlorobenzene; HMWP, high molecular weight phthalate; LMWP, low molecular weight phthalate; MBP, mono-butyl phthalate/mono-n-butyl phthalate; MBzP, mono-benzyl phthalate; MCMHP, mono-2-carboxymethyl-hexyl phthalate; MCPP, mono-(3-carboxypropyl) phthalate; MEP, mono-ethyl phthalate; MEHP, mono-2-ethylhexyl phthalate; MEOHP, mono (2-ethyl-5-exohexyl) phthalate; MEHHP, mono (2-ethyl-5hydroxyhexyl) phthalate; MEHP, mono-ethylhexyl-phthalate; MECPP, mono-(2-ethyl-5-carboxypentyl) phthalate; MiBP, mono-isobutyl phthalate; MMP, mono-methyl phthalate; OC, organochlorine compound; PBDEs, polybrominated diphenyl ethers; PCB, polychlorinated biphenyl; PFAS, per- and polyfluoroalkyl substances; POPs, persistent organic pollutants; ST, skinfold thickness; WC, waist circumference; WHtR, waist-to-height ratio; WtHR, weight-to-height ratio.
1. Allison DB, Downey M, Atkinson RL, Billington CJ, Bray GA, Eckel RH, et al. Obesity as a Disease: A White Paper on Evidence and Arguments Commissioned by the Council of the Obesity Society. Obesity (Silver Spring) (2008) 16(6):1161–77. doi: 10.1038/oby.2008.231
2. Lim JE, Choi B, Jee SH. Urinary Bisphenol A, Phthalate Metabolites, and Obesity: Do Gender and Menopausal Status Matter? Environ Sci Pollut Res Int (2020) 27(27):34300–10. doi: 10.1007/s11356-020-09570-x
3. Liu Y, Li N, Papandonatos GD, Calafat AM, Eaton CB, Kelsey KT, et al. Exposure to Per- and Polyfluoroalkyl Substances and Adiposity at Age 12 Years: Evaluating Periods of Susceptibility. Environ Sci Technol (2020) 54(24):16039–49. doi: 10.1021/acs.est.0c06088
4. Guo J, Miao W, Wu C, Zhang J, Qi X, Yu H, et al. Umbilical Cord Serum PBDE Concentrations and Child Adiposity Measures at 7 Years. Ecotoxicol Environ Saf (2020) 203:111009. doi: 10.1016/j.ecoenv.2020.111009
5. Sommer I, Teufer B, Szelag M, Nussbaumer-Streit B, Titscher V, Klerings I, et al. The Performance of Anthropometric Tools to Determine Obesity: A Systematic Review and Meta-Analysis. Sci Rep (2020) 10(1):12699. doi: 10.1038/s41598-020-69498-7
6. World Health Organization. Obesity and Overweight. Available at: https://www.who.int/news-room/fact-sheets/detail/obesity-and-overweight (Accessed Accesssed August 30, 2021).
7. Liu B, Lehmler HJ, Sun Y, Xu G, Sun Q, Snetselaar LG, et al. Association of Bisphenol A and Its Substitutes, Bisphenol F and Bisphenol S, With Obesity in United States Children and Adolescents. Diabetes Metab J (2019) 43(1):59–75. doi: 10.4093/dmj.2018.0045
8. Guo J, Zhang J, Wu C, Xiao H, Lv S, Lu D, et al. Urinary Bisphenol A Concentrations and Adiposity Measures at Age 7 Years in a Prospective Birth Cohort. Chemosphere (2020) 251:126340. doi: 10.1016/j.chemosphere.2020.126340
9. James PT, Leach R, Kalamara E, Shayeghi M. The Worldwide Obesity Epidemic. Obes Res (2001) 9 Suppl 4:228s–33s. doi: 10.1038/oby.2001.123
10. Poirier P, Giles TD, Bray GA, Hong Y, Stern JS, Pi-Sunyer FX, et al. Obesity and Cardiovascular Disease: Pathophysiology, Evaluation, and Effect of Weight Loss. Arterioscler Thromb Vasc Biol (2006) 26(5):968–76. doi: 10.1161/01.ATV.0000216787.85457.f3
11. Patterson RE, Frank LL, Kristal AR, White E. A Comprehensive Examination of Health Conditions Associated With Obesity in Older Adults. Am J Prev Med (2004) 27(5):385–90. doi: 10.1016/j.amepre.2004.08.001
12. Klein S, Burke LE, Bray GA, Blair S, Allison DB, Pi-Sunyer X, et al. Clinical Implications of Obesity With Specific Focus on Cardiovascular Disease: A Statement for Professionals From the American Heart Association Council on Nutrition, Physical Activity, and Metabolism: Endorsed by the American College of Cardiology Foundation. Circulation (2004) 110(18):2952–67. doi: 10.1161/01.Cir.0000145546.97738.1e
13. Vincent HK, Vincent KR, Seay AN, Hurley RW. Functional Impairment in Obesity: A Focus on Knee and Back Pain. Pain Manag (2011) 1(5):427–39. doi: 10.2217/pmt.11.39
14. Pimenta FB, Bertrand E, Mograbi DC, Shinohara H, Landeira-Fernandez J. The Relationship Between Obesity and Quality of Life in Brazilian Adults. Front Psychol (2015) 6:966. doi: 10.3389/fpsyg.2015.00966
15. Busutil R, Espallardo O, Torres A, Martínez-Galdeano L, Zozaya N, Hidalgo-Vega Á. The Impact of Obesity on Health-Related Quality of Life in Spain. Health Qual Life Outcomes (2017) 15(1):197. doi: 10.1186/s12955-017-0773-y
16. Fontaine KR, Redden DT, Wang C, Westfall AO, Allison DB. Years of Life Lost Due to Obesity. Jama (2003) 289(2):187–93. doi: 10.1001/jama.289.2.187
17. Conway B, Rene A. Obesity as a Disease: No Lightweight Matter. Obes Rev (2004) 5(3):145–51. doi: 10.1111/j.1467-789X.2004.00144.x
18. Bray GA. Medical Consequences of Obesity. J Clin Endocrinol Metab (2004) 89(6):2583–9. doi: 10.1210/jc.2004-0535
19. Keith SW, Redden DT, Katzmarzyk PT, Boggiano MM, Hanlon EC, Benca RM, et al. Putative Contributors to the Secular Increase in Obesity: Exploring the Roads Less Traveled. Int J Obes (Lond) (2006) 30(11):1585–94. doi: 10.1038/sj.ijo.0803326
20. Bray GA, Champagne CM. Beyond Energy Balance: There Is More to Obesity Than Kilocalories. J Am Diet Assoc (2005) 105(5 Suppl 1):S17–23. doi: 10.1016/j.jada.2005.02.018
21. Eisenmann JC. Insight Into the Causes of the Recent Secular Trend in Pediatric Obesity: Common Sense Does Not Always Prevail for Complex, Multi-Factorial Phenotypes. Prev Med (2006) 42(5):329–35. doi: 10.1016/j.ypmed.2006.02.002
22. Patterson WB, Glasson J, Naik N, Jones RB, Berger PK, Plows JF, et al. Prenatal Exposure to Ambient Air Pollutants and Early Infant Growth and Adiposity in the Southern California Mother's Milk Study. Environ Health (2021) 20(1):67. doi: 10.1186/s12940-021-00753-8
23. Liu M, Tang W, Zhang Y, Wang Y, Baima K, Li Y, et al. Urban-Rural Differences in the Association Between Long-Term Exposure to Ambient Air Pollution and Obesity in China. Environ Res (2021) 201:111597. doi: 10.1016/j.envres.2021.111597
24. Bowe B, Gibson AK, Xie Y, Yan Y, Donkelaar AV, Martin RV, et al. Ambient Fine Particulate Matter Air Pollution and Risk of Weight Gain and Obesity in United States Veterans: An Observational Cohort Study. Environ Health Perspect (2021) 129(4):47003. doi: 10.1289/ehp7944
25. Zhong Q, Qin QR, Yang WJ, He JL, Zhu JL, Zhu ZY, et al. Multiple Metal Exposure and Obesity: A Prospective Cohort Study of Adults Living Along the Yangtze River, China. Environ Pollut (2021) 285:117150. doi: 10.1016/j.envpol.2021.117150
26. Jia P, Cao X, Yang H, Dai S, He P, Huang G, et al. Green Space Access in the Neighbourhood and Childhood Obesity. Obes Rev (2021) 22 Suppl 1(Suppl 1):e13100. doi: 10.1111/obr.13100
27. Foraster M, Eze IC, Vienneau D, Schaffner E, Jeong A, Héritier H, et al. Long-Term Exposure to Transportation Noise and Its Association With Adiposity Markers and Development of Obesity. Environ Int (2018) 121(Pt 1):879–89. doi: 10.1016/j.envint.2018.09.057
28. Berger K, Hyland C, Ames JL, Mora AM, Huen K, Eskenazi B, et al. Prenatal Exposure to Mixtures of Phthalates, Parabens, and Other Phenols and Obesity in Five-Year-Olds in the CHAMACOS Cohort. Int J Environ Res Public Health (2021) 18(4):1796. doi: 10.3390/ijerph18041796
29. Wang X, Mukherjee B, Park SK. Associations of Cumulative Exposure to Heavy Metal Mixtures With Obesity and its Comorbidities Among US Adults in NHANES 2003-2014. Environ Int (2018) 121(Pt 1):683–94. doi: 10.1016/j.envint.2018.09.035
30. Pyko A, Eriksson C, Lind T, Mitkovskaya N, Wallas A, Ögren M, et al. Long-Term Exposure to Transportation Noise in Relation to Development of Obesity—a Cohort Study. Environ Health Perspect (2017) 125(11):117005. doi: 10.1289/ehp1910
31. Shao W, Xu J, Xu C, Weng Z, Liu Q, Zhang X, et al. Early-Life Perfluorooctanoic Acid Exposure Induces Obesity in Male Offspring and the Intervention Role of Chlorogenic Acid. Environ Pollut (2021) 272:115974. doi: 10.1016/j.envpol.2020.115974
32. Grün F, Blumberg B. Perturbed Nuclear Receptor Signaling by Environmental Obesogens as Emerging Factors in the Obesity Crisis. Rev Endocr Metab Disord (2007) 8(2):161–71. doi: 10.1007/s11154-007-9049-x
33. Meeker JD. Exposure to Environmental Endocrine Disruptors and Child Development. Arch Pediatr Adolesc Med (2012) 166(10):952–8. doi: 10.1001/archpediatrics.2012.241
34. Jacobson MH, Woodward M, Bao W, Liu B, Trasande L. Urinary Bisphenols and Obesity Prevalence Among US Children and Adolescents. J Endocr Soc (2019) 3(9):1715–26. doi: 10.1210/js.2019-00201
35. Arbuckle TE, Davis K, Marro L, Fisher M, Legrand M, LeBlanc A, et al. Phthalate and Bisphenol A Exposure Among Pregnant Women in Canada–results From the MIREC Study. Environ Int (2014) 68:55–65. doi: 10.1016/j.envint.2014.02.010
36. González-Mariño I, Ares L, Montes R, Rodil R, Cela R, López-García E, et al. Assessing Population Exposure to Phthalate Plasticizers in Thirteen Spanish Cities Through the Analysis of Wastewater. J Hazard Mater (2021) 401:123272. doi: 10.1016/j.jhazmat.2020.123272
37. Katsikantami I, Sifakis S, Tzatzarakis MN, Vakonaki E, Kalantzi OI, Tsatsakis AM, et al. A Global Assessment of Phthalates Burden and Related Links to Health Effects. Environ Int (2016) 97:212–36. doi: 10.1016/j.envint.2016.09.013
38. Vizcaino E, Grimalt JO, Fernández-Somoano A, Tardon A. Transport of Persistent Organic Pollutants Across the Human Placenta. Environ Int (2014) 65:107–15. doi: 10.1016/j.envint.2014.01.004
39. Ahrens L, Bundschuh M. Fate and Effects of Poly- and Perfluoroalkyl Substances in the Aquatic Environment: A Review. Environ Toxicol Chem (2014) 33(9):1921–9. doi: 10.1002/etc.2663
40. Kim D, Ryu HY, Lee JH, Lee JH, Lee YJ, Kim HK, et al. Organochlorine Pesticides and Polychlorinated Biphenyls in Korean Human Milk: Contamination Levels and Infant Risk Assessment. J Environ Sci Health B (2013) 48(4):243–50. doi: 10.1080/03601234.2013.742413
41. Carwile JL, Michels KB. Urinary Bisphenol A and Obesity: NHANES 2003-2006. Environ Res (2011) 111(6):825–30. doi: 10.1016/j.envres.2011.05.014
42. Krysiak-Baltyn K, Toppari J, Skakkebaek NE, Jensen TS, Virtanen HE, Schramm KW, et al. Country-Specific Chemical Signatures of Persistent Environmental Compounds in Breast Milk. Int J Androl (2010) 33(2):270–8. doi: 10.1111/j.1365-2605.2009.00996.x
43. Fäys F, Hardy EM, Palazzi P, Haan S, Beausoleil C, Appenzeller BMR. Biomonitoring of Fast-Elimination Endocrine Disruptors - Results From a 6-Month Follow Up on Human Volunteers With Repeated Urine and Hair Collection. Sci Total Environ (2021) 778:146330. doi: 10.1016/j.scitotenv.2021.146330
44. Cao Z, Lin S, Zhao F, Lv Y, Qu Y, Hu X, et al. Cohort Profile: China National Human Biomonitoring (CNHBM)-A Nationally Representative, Prospective Cohort in Chinese Population. Environ Int (2021) 146:106252. doi: 10.1016/j.envint.2020.106252
45. Pollock T, Karthikeyan S, Walker M, Werry K, St-Amand A. Trends in Environmental Chemical Concentrations in the Canadian Population: Biomonitoring Data From the Canadian Health Measures Survey 2007-2017. Environ Int (2021) 155:106678. doi: 10.1016/j.envint.2021.106678
46. Karrer C, Andreassen M, von Goetz N, Sonnet F, Sakhi AK, Hungerbühler K, et al. The EuroMix Human Biomonitoring Study: Source-To-Dose Modeling of Cumulative and Aggregate Exposure for the Bisphenols BPA, BPS, and BPF and Comparison With Measured Urinary Levels. Environ Int (2020) 136:105397. doi: 10.1016/j.envint.2019.105397
47. Jeon HL, Hong S, Choi K, Lee C, Yoo J. First Nationwide Exposure Profile of Major Persistent Organic Pollutants Among Korean Adults and Their Determinants: Korean National Environmental Health Survey Cycle 3 (2015-2017). Int J Hyg Environ Health (2021) 236:113779. doi: 10.1016/j.ijheh.2021.113779
48. Zhang Y, Dong T, Hu W, Wang X, Xu B, Lin Z, et al. Association Between Exposure to a Mixture of Phenols, Pesticides, and Phthalates and Obesity: Comparison of Three Statistical Models. Environ Int (2019) 123:325–36. doi: 10.1016/j.envint.2018.11.076
49. Li YL, Lv J, Du ZP, Feng S, Sheng J, Jin ZX, et al. The Levels of Phthalate Exposure and Associations With Obesity in an Elderly Population in China. Ecotoxicol Environ Saf (2020) 201:110749. doi: 10.1016/j.ecoenv.2020.110749
50. Sol CM, Santos S, Duijts L, Asimakopoulos AG, Martinez-Moral MP, Kannan K, et al. Fetal Exposure to Phthalates and Bisphenols and Childhood General and Organ Fat. A Population-Based Prospective Cohort Study. Int J Obes (Lond) (2020) 44(11):2225–35. doi: 10.1038/s41366-020-00672-7
51. Jensen RC, Andersen MS, Larsen PV, Glintborg D, Dalgård C, Timmermann CAG, et al. Prenatal Exposures to Perfluoroalkyl Acids and Associations With Markers of Adiposity and Plasma Lipids in Infancy: An Odense Child Cohort Study. Environ Health Perspect (2020) 128(7):77001. doi: 10.1289/ehp5184
52. Egusquiza RJ, Blumberg B. Environmental Obesogens and Their Impact on Susceptibility to Obesity: New Mechanisms and Chemicals. Endocrinology (2020) 161(3):1–14. doi: 10.1210/endocr/bqaa024
53. Wu W, Li M, Liu A, Wu C, Li D, Deng Q, et al. Bisphenol A and the Risk of Obesity a Systematic Review With Meta-Analysis of the Epidemiological Evidence. Dose Response (2020) 18(2):1559325820916949. doi: 10.1177/1559325820916949
54. Cano-Sancho G, Salmon AG, La Merrill MA. Association Between Exposure to P,P'-DDT and Its Metabolite P,P'-DDE With Obesity: Integrated Systematic Review and Meta-Analysis. Environ Health Perspect (2017) 125(9):096002. doi: 10.1289/ehp527
55. Wassenaar PNH, Trasande L, Legler J. Systematic Review and Meta-Analysis of Early-Life Exposure to Bisphenol A and Obesity-Related Outcomes in Rodents. Environ Health Perspect (2017) 125(10):106001. doi: 10.1289/ehp1233
56. de Cock M, van de Bor M. Obesogenic Effects of Endocrine Disruptors, What do We Know From Animal and Human Studies? Environ Int (2014) 70:15–24. doi: 10.1016/j.envint.2014.04.022
57. Goodman M, Lakind JS, Mattison DR. Do Phthalates Act as Obesogens in Humans? A Systematic Review of the Epidemiological Literature. Crit Rev Toxicol (2014) 44(2):151–75. doi: 10.3109/10408444.2013.860076
58. Kim SH, Park MJ. Phthalate Exposure and Childhood Obesity. Ann Pediatr Endocrinol Metab (2014) 19(2):69–75. doi: 10.6065/apem.2014.19.2.69
59. Karoutsou E, Polymeris A. Environmental Endocrine Disruptors and Obesity. Endocr Regul (2012) 46(1):37–46. doi: 10.4149/endo_2012_01_37
60. Kail R. Children and Their Development. 6th Edition. Englewood Cliffs, N.J: Prentice Hall (2011).
61. Braun JM, Li N, Arbuckle TE, Dodds L, Massarelli I, Fraser WD, et al. Association Between Gestational Urinary Bisphenol a Concentrations and Adiposity in Young Children: The MIREC Study. Environ Res (2019) 172:454–61. doi: 10.1016/j.envres.2019.02.038
62. Amin MM, Ebrahim K, Hashemi M, Shoshtari-Yeganeh B, Rafiei N, Mansourian M, et al. Association of Exposure to Bisphenol A With Obesity and Cardiometabolic Risk Factors in Children and Adolescents. Int J Environ Health Res (2019) 29(1):94–106. doi: 10.1080/09603123.2018.1515896
63. Okubo Y, Handa A, Belin T. Serial Cross-Sectional Study for the Association Between Urinary Bisphenol A and Paediatric Obesity: Recent Updates Using NHANES 2003-2014. Pediatr Obes (2019) 14(12):e12566. doi: 10.1111/ijpo.12566
64. Do MT, Chang VC, Mendez MA, de Groh M. Urinary Bisphenol A and Obesity in Adults: Results From the Canadian Health Measures Survey. Health Promot Chronic Dis Prev Can (2017) 37(12):403–12. doi: 10.24095/hpcdp.37.12.02
65. Hong SH, Sung YA, Hong YS, Ha E, Jeong K, Chung H, et al. Urinary Bisphenol A is Associated With Insulin Resistance and Obesity in Reproductive-Aged Women. Clin Endocrinol (Oxf) (2017) 86(4):506–12. doi: 10.1111/cen.13270
66. Li J, Lai H, Chen S, Zhu H, Lai S. Gender Differences in the Associations Between Urinary Bisphenol A and Body Composition Among American Children: The National Health and Nutrition Examination Survey, 2003-2006. J Epidemiol (2017) 27(5):228–34. doi: 10.1016/j.je.2016.12.001
67. Liu B, Lehmler HJ, Sun Y, Xu G, Liu Y, Zong G, et al. Bisphenol A Substitutes and Obesity in US Adults: Analysis of a Population-Based, Cross-Sectional Study. Lancet Planet Health (2017) 1(3):e114–22. doi: 10.1016/s2542-5196(17)30049-9
68. Deierlein AL, Wolff MS, Pajak A, Pinney SM, Windham GC, Galvez MP, et al. Phenol Concentrations During Childhood and Subsequent Measures of Adiposity Among Young Girls. Am J Epidemiol (2017) 186(5):581–92. doi: 10.1093/aje/kwx136
69. Hao M, Ding L, Xuan L, Wang T, Li M, Zhao Z, et al. Urinary Bisphenol A Concentration and the Risk of Central Obesity in Chinese Adults: A Prospective Study. J Diabetes (2018) 10(6):442–8. doi: 10.1111/1753-0407.12531
70. Yang TC, Peterson KE, Meeker JD, Sánchez BN, Zhang Z, Cantoral A, et al. Bisphenol A and Phthalates In Utero and in Childhood: Association With Child BMI Z-Score and Adiposity. Environ Res (2017) 156:326–33. doi: 10.1016/j.envres.2017.03.038
71. Buckley JP, Herring AH, Wolff MS, Calafat AM, Engel SM. Prenatal Exposure to Environmental Phenols and Childhood Fat Mass in the Mount Sinai Children's Environmental Health Study. Environ Int (2016) 91:350–6. doi: 10.1016/j.envint.2016.03.019
72. Hoepner LA, Whyatt RM, Widen EM, Hassoun A, Oberfield SE, Mueller NT, et al. Bisphenol A and Adiposity in an Inner-City Birth Cohort. Environ Health Perspect (2016) 124(10):1644–50. doi: 10.1289/ehp205
73. Vafeiadi M, Roumeliotaki T, Myridakis A, Chalkiadaki G, Fthenou E, Dermitzaki E, et al. Association of Early Life Exposure to Bisphenol A With Obesity and Cardiometabolic Traits in Childhood. Environ Res (2016) 146:379–87. doi: 10.1016/j.envres.2016.01.017
74. Lee MR, Kim JH, Choi YH, Bae S, Park C, Hong YC. Association of Bisphenol A Exposure With Overweight in the Elderly: A Panel Study. Environ Sci Pollut Res Int (2015) 22(12):9370–7. doi: 10.1007/s11356-015-4087-5
75. Andra SS, Makris KC. Association Between Urinary Levels of Bisphenol A and its Monochlorinated Derivative and Obesity. J Environ Sci Health A Tox Hazard Subst Environ Eng (2015) 50(11):1169–79. doi: 10.1080/10934529.2015.1047674
76. Savastano S, Tarantino G, D'Esposito V, Passaretti F, Cabaro S, Liotti A, et al. Bisphenol-A Plasma Levels are Related to Inflammatory Markers, Visceral Obesity and Insulin-Resistance: A Cross-Sectional Study on Adult Male Population. J Transl Med (2015) 13:169. doi: 10.1186/s12967-015-0532-y
77. Ko A, Hwang MS, Park JH, Kang HS, Lee HS, Hong JH. Association Between Urinary Bisphenol A and Waist Circumference in Korean Adults. Toxicol Res (2014) 30(1):39–44. doi: 10.5487/tr.2014.30.1.039
78. Braun JM, Lanphear BP, Calafat AM, Deria S, Khoury J, Howe CJ, et al. Early-Life Bisphenol a Exposure and Child Body Mass Index: A Prospective Cohort Study. Environ Health Perspect (2014) 122(11):1239–45. doi: 10.1289/ehp.1408258
79. Harley KG, Aguilar Schall R, Chevrier J, Tyler K, Aguirre H, Bradman A, et al. Prenatal and Postnatal Bisphenol A Exposure and Body Mass Index in Childhood in the CHAMACOS Cohort. Environ Health Perspect (2013) 121(4):514–20. doi: 10.1289/ehp.1205548
80. Valvi D, Casas M, Mendez MA, Ballesteros-Gómez A, Luque N, Rubio S, et al. Prenatal Bisphenol a Urine Concentrations and Early Rapid Growth and Overweight Risk in the Offspring. Epidemiology (2013) 24(6):791–9. doi: 10.1097/EDE.0b013e3182a67822
81. Li DK, Miao M, Zhou Z, Wu C, Shi H, Liu X, et al. Urine Bisphenol-A Level in Relation to Obesity and Overweight in School-Age Children. PloS One (2013) 8(6):e65399. doi: 10.1371/journal.pone.0065399
82. Bhandari R, Xiao J, Shankar A. Urinary Bisphenol A and Obesity in US Children. Am J Epidemiol (2013) 177(11):1263–70. doi: 10.1093/aje/kws391
83. Trasande L, Attina TM, Blustein J. Association Between Urinary Bisphenol A Concentration and Obesity Prevalence in Children and Adolescents. Jama (2012) 308(11):1113–21. doi: 10.1001/2012.jama.11461
84. Wang T, Li M, Chen B, Xu M, Xu Y, Huang Y, et al. Urinary Bisphenol A (BPA) Concentration Associates With Obesity and Insulin Resistance. J Clin Endocrinol Metab (2012) 97(2):E223–7. doi: 10.1210/jc.2011-1989
85. Wang HX, Zhou Y, Tang CX, Wu JG, Chen Y, Jiang QW. Association Between Bisphenol A Exposure and Body Mass Index in Chinese School Children: A Cross-Sectional Study. Environ Health (2012) 11:79. doi: 10.1186/1476-069x-11-79
86. Shankar A, Teppala S, Sabanayagam C. Urinary Bisphenol a Levels and Measures of Obesity: Results From the National Health and Nutrition Examination Survey 2003-2008. ISRN Endocrinol (2012) 2012:965243. doi: 10.5402/2012/965243
87. Bowman A, Peterson KE, Dolinoy DC, Meeker JD, Sánchez BN, Mercado-Garcia A, et al. Phthalate Exposures, DNA Methylation and Adiposity in Mexican Children Through Adolescence. Front Public Health (2019) 7:162. doi: 10.3389/fpubh.2019.00162
88. Kang Y, Park J, Youn K. Association Between Urinary Phthalate Metabolites and Obesity in Adult Korean Population: Korean National Environmental Health Survey (KoNEHS), 2012-2014. Ann Occup Environ Med (2019) 31:e23. doi: 10.35371/aoem.2019.31.e23
89. Amin MM, Ebrahimpour K, Parastar S, Shoshtari-Yeganeh B, Hashemi M, Mansourian M, et al. Association of Urinary Concentrations of Phthalate Metabolites With Cardiometabolic Risk Factors and Obesity in Children and Adolescents. Chemosphere (2018) 211:547–56. doi: 10.1016/j.chemosphere.2018.07.172
90. Kim SH, On JW, Pyo H, Ko KS, Won JC, Yang J, et al. Percentage Fractions of Urinary Di(2-Ethylhexyl) Phthalate Metabolites: Association With Obesity and Insulin Resistance in Korean Girls. PloS One (2018) 13(11):e0208081. doi: 10.1371/journal.pone.0208081
91. Xia B, Zhu Q, Zhao Y, Ge W, Zhao Y, Song Q, et al. Phthalate Exposure and Childhood Overweight and Obesity: Urinary Metabolomic Evidence. Environ Int (2018) 121(Pt 1):159–68. doi: 10.1016/j.envint.2018.09.001
92. Amin MM, Parastar S, Ebrahimpour K, Shoshtari-Yeganeh B, Hashemi M, Mansourian M, et al. Association of Urinary Phthalate Metabolites Concentrations With Body Mass Index and Waist Circumference. Environ Sci Pollut Res Int (2018) 25(11):11143–51. doi: 10.1007/s11356-018-1413-8
93. Vafeiadi M, Myridakis A, Roumeliotaki T, Margetaki K, Chalkiadaki G, Dermitzaki E, et al. Association of Early Life Exposure to Phthalates With Obesity and Cardiometabolic Traits in Childhood: Sex Specific Associations. Front Public Health (2018) 6:327. doi: 10.3389/fpubh.2018.00327
94. Wu W, Wu P, Yang F, Sun DL, Zhang DX, Zhou YK. Association of Phthalate Exposure With Anthropometric Indices and Blood Pressure in First-Grade Children. Environ Sci Pollut Res Int (2018) 25(23):23125–34. doi: 10.1007/s11356-018-2447-7
95. Harley KG, Berger K, Rauch S, Kogut K, Claus Henn B, Calafat AM, et al. Association of Prenatal Urinary Phthalate Metabolite Concentrations and Childhood BMI and Obesity. Pediatr Res (2017) 82(3):405–15. doi: 10.1038/pr.2017.112
96. Shoaff J, Papandonatos GD, Calafat AM, Ye X, Chen A, Lanphear BP, et al. Early-Life Phthalate Exposure and Adiposity at 8 Years of Age. Environ Health Perspect (2017) 125(9):097008. doi: 10.1289/ehp1022
97. Dong R, Zhou T, Chen J, Zhang M, Zhang H, Wu M, et al. Gender- and Age-Specific Relationships Between Phthalate Exposures and Obesity in Shanghai Adults. Arch Environ Contam Toxicol (2017) 73(3):431–41. doi: 10.1007/s00244-017-0441-6
98. Buckley JP, Engel SM, Mendez MA, Richardson DB, Daniels JL, Calafat AM, et al. Prenatal Phthalate Exposures and Childhood Fat Mass in a New York City Cohort. Environ Health Perspect (2016) 124(4):507–13. doi: 10.1289/ehp.1509788
99. Buckley JP, Engel SM, Braun JM, Whyatt RM, Daniels JL, Mendez MA, et al. Prenatal Phthalate Exposures and Body Mass Index Among 4- to 7-Year-Old Children: A Pooled Analysis. Epidemiology (2016) 27(3):449–58. doi: 10.1097/ede.0000000000000436
100. Kim JH, Park H, Lee J, Cho G, Choi S, Choi G, et al. Association of Diethylhexyl Phthalate With Obesity-Related Markers and Body Mass Change From Birth to 3 Months of Age. J Epidemiol Community Health (2016) 70(5):466–72. doi: 10.1136/jech-2015-206315
101. Maresca MM, Hoepner LA, Hassoun A, Oberfield SE, Mooney SJ, Calafat AM, et al. Prenatal Exposure to Phthalates and Childhood Body Size in an Urban Cohort. Environ Health Perspect (2016) 124(4):514–20. doi: 10.1289/ehp.1408750
102. Deierlein AL, Wolff MS, Pajak A, Pinney SM, Windham GC, Galvez MP, et al. Longitudinal Associations of Phthalate Exposures During Childhood and Body Size Measurements in Young Girls. Epidemiology (2016) 27(4):492–9. doi: 10.1097/ede.0000000000000489
103. Agay-Shay K, Martinez D, Valvi D, Garcia-Esteban R, Basagaña X, Robinson O, et al. Exposure to Endocrine-Disrupting Chemicals During Pregnancy and Weight at 7 Years of Age: A Multi-Pollutant Approach. Environ Health Perspect (2015) 123(10):1030–7. doi: 10.1289/ehp.1409049
104. Yaghjyan L, Sites S, Ruan Y, Chang SH. Associations of Urinary Phthalates With Body Mass Index, Waist Circumference and Serum Lipids Among Females: National Health and Nutrition Examination Survey 1999-2004. Int J Obes (Lond) (2015) 39(6):994–1000. doi: 10.1038/ijo.2015.8
105. Hou JW, Lin CL, Tsai YA, Chang CH, Liao KW, Yu CJ, et al. The Effects of Phthalate and Nonylphenol Exposure on Body Size and Secondary Sexual Characteristics During Puberty. Int J Hyg Environ Health (2015) 218(7):603–15. doi: 10.1016/j.ijheh.2015.06.004
106. Buser MC, Murray HE, Scinicariello F. Age and Sex Differences in Childhood and Adulthood Obesity Association With Phthalates: Analyses of NHANES 2007-2010. Int J Hyg Environ Health (2014) 217(6):687–94. doi: 10.1016/j.ijheh.2014.02.005
107. Zhang Y, Meng X, Chen L, Li D, Zhao L, Zhao Y, et al. Age and Sex-Specific Relationships Between Phthalate Exposures and Obesity in Chinese Children at Puberty. PloS One (2014) 9(8):e104852. doi: 10.1371/journal.pone.0104852
108. Trasande L, Attina TM, Sathyanarayana S, Spanier AJ, Blustein J. Race/ethnicity-Specific Associations of Urinary Phthalates With Childhood Body Mass in a Nationally Representative Sample. Environ Health Perspect (2013) 121(4):501–6. doi: 10.1289/ehp.1205526
109. Wang H, Zhou Y, Tang C, He Y, Wu J, Chen Y, et al. Urinary Phthalate Metabolites are Associated With Body Mass Index and Waist Circumference in Chinese School Children. PloS One (2013) 8(2):e56800. doi: 10.1371/journal.pone.0056800
110. Teitelbaum SL, Mervish N, Moshier EL, Vangeepuram N, Galvez MP, Calafat AM, et al. Associations Between Phthalate Metabolite Urinary Concentrations and Body Size Measures in New York City Children. Environ Res (2012) 112:186–93. doi: 10.1016/j.envres.2011.12.006
111. Hatch EE, Nelson JW, Qureshi MM, Weinberg J, Moore LL, Singer M, et al. Association of Urinary Phthalate Metabolite Concentrations With Body Mass Index and Waist Circumference: A Cross-Sectional Study of NHANES Data, 1999-2002. Environ Health (2008) 7:27. doi: 10.1186/1476-069x-7-27
112. Stahlhut RW, van Wijngaarden E, Dye TD, Cook S, Swan SH. Concentrations of Urinary Phthalate Metabolites are Associated With Increased Waist Circumference and Insulin Resistance in Adult US Males. Environ Health Perspect (2007) 115(6):876–82. doi: 10.1289/ehp.9882
113. Chen Q, Zhang X, Zhao Y, Lu W, Wu J, Zhao S, et al. Prenatal Exposure to Perfluorobutanesulfonic Acid and Childhood Adiposity: A Prospective Birth Cohort Study in Shanghai, China. Chemosphere (2019) 226:17–23. doi: 10.1016/j.chemosphere.2019.03.095
114. Vuong AM, Braun JM, Wang Z, Yolton K, Xie C, Sjodin A, et al. Exposure to Polybrominated Diphenyl Ethers (PBDEs) During Childhood and Adiposity Measures at Age 8 Years. Environ Int (2019) 123:148–55. doi: 10.1016/j.envint.2018.11.050
115. Lauritzen HB, Larose TL, Øien T, Sandanger TM, Odland J, van de Bor M, et al. Prenatal Exposure to Persistent Organic Pollutants and Child Overweight/Obesity at 5-Year Follow-Up: A Prospective Cohort Study. Environ Health (2018) 17(1):9. doi: 10.1186/s12940-017-0338-x
116. Coker E, Chevrier J, Rauch S, Bradman A, Obida M, Crause M, et al. Association Between Prenatal Exposure to Multiple Insecticides and Child Body Weight and Body Composition in the VHEMBE South African Birth Cohort. Environ Int (2018) 113:122–32. doi: 10.1016/j.envint.2018.01.016
117. Warner M, Rauch S, Coker ES, Harley K, Kogut K, Sjödin A, et al. Obesity in Relation to Serum Persistent Organic Pollutant Concentrations in CHAMACOS Women. Environ Epidemiol (2018) 2(4):e032. doi: 10.1097/ee9.0000000000000032
118. Warner M, Ye M, Harley K, Kogut K, Bradman A, Eskenazi B. Prenatal DDT Exposure and Child Adiposity at Age 12: The CHAMACOS Study. Environ Res (2017) 159:606–12. doi: 10.1016/j.envres.2017.08.050
119. Henríquez-Hernández LA, Luzardo OP, Valerón PF, Zumbado M, Serra-Majem L, Camacho M, et al. Persistent Organic Pollutants and Risk of Diabetes and Obesity on Healthy Adults: Results From a Cross-Sectional Study in Spain. Sci Total Environ (2017) 607-608:1096–102. doi: 10.1016/j.scitotenv.2017.07.075
120. Mora AM, Oken E, Rifas-Shiman SL, Webster TF, Gillman MW, Calafat AM, et al. Prenatal Exposure to Perfluoroalkyl Substances and Adiposity in Early and Mid-Childhood. Environ Health Perspect (2017) 125(3):467–73. doi: 10.1289/ehp246
121. Karlsen M, Grandjean P, Weihe P, Steuerwald U, Oulhote Y, Valvi D. Early-Life Exposures to Persistent Organic Pollutants in Relation to Overweight in Preschool Children. Reprod Toxicol (2017) 68:145–53. doi: 10.1016/j.reprotox.2016.08.002
122. Braun JM, Chen A, Romano ME, Calafat AM, Webster GM, Yolton K, et al. Prenatal Perfluoroalkyl Substance Exposure and Child Adiposity at 8 Years of Age: The HOME Study. Obesity (Silver Spring) (2016) 24(1):231–7. doi: 10.1002/oby.21258
123. Vuong AM, Braun JM, Sjödin A, Webster GM, Yolton K, Lanphear BP, et al. Prenatal Polybrominated Diphenyl Ether Exposure and Body Mass Index in Children Up To 8 Years of Age. Environ Health Perspect (2016) 124(12):1891–7. doi: 10.1289/ehp139
124. Høyer BB, Ramlau-Hansen CH, Vrijheid M, Valvi D, Pedersen HS, Zviezdai V, et al. Anthropometry in 5- to 9-Year-Old Greenlandic and Ukrainian Children in Relation to Prenatal Exposure to Perfluorinated Alkyl Substances. Environ Health Perspect (2015) 123(8):841–6. doi: 10.1289/ehp.1408881
125. Tang-Péronard JL, Jensen TK, Andersen HR, Ried-Larsen M, Grøntved A, Andersen LB, et al. Associations Between Exposure to Persistent Organic Pollutants in Childhood and Overweight Up to 12 Years Later in a Low Exposed Danish Population. Obes Facts (2015) 8(4):282–92. doi: 10.1159/000438834
126. Vafeiadi M, Georgiou V, Chalkiadaki G, Rantakokko P, Kiviranta H, Karachaliou M, et al. Association of Prenatal Exposure to Persistent Organic Pollutants With Obesity and Cardiometabolic Traits in Early Childhood: The Rhea Mother-Child Cohort (Crete, Greece). Environ Health Perspect (2015) 123(10):1015–21. doi: 10.1289/ehp.1409062
127. Erkin-Cakmak A, Harley KG, Chevrier J, Bradman A, Kogut K, Huen K, et al. In Utero and Childhood Polybrominated Diphenyl Ether Exposures and Body Mass at Age 7 Years: The CHAMACOS Study. Environ Health Perspect (2015) 123(6):636–42. doi: 10.1289/ehp.1408417
128. Zong G, Grandjean P, Wu H, Sun Q. Circulating Persistent Organic Pollutants and Body Fat Distribution: Evidence From NHANES 1999-2004. Obesity (Silver Spring) (2015) 23(9):1903–10. doi: 10.1002/oby.21161
129. Delvaux I, Van Cauwenberghe J, Den Hond E, Schoeters G, Govarts E, Nelen V, et al. Prenatal Exposure to Environmental Contaminants and Body Composition at Age 7-9 Years. Environ Res (2014) 132:24–32. doi: 10.1016/j.envres.2014.03.019
130. Tang-Péronard JL, Heitmann BL, Andersen HR, Steuerwald U, Grandjean P, Weihe P, et al. Association Between Prenatal Polychlorinated Biphenyl Exposure and Obesity Development at Ages 5 and 7 Y: A Prospective Cohort Study of 656 Children From the Faroe Islands. Am J Clin Nutr (2014) 99(1):5–13. doi: 10.3945/ajcn.113.066720
131. Dirinck EL, Dirtu AC, Govindan M, Covaci A, Van Gaal LF, Jorens PG. Exposure to Persistent Organic Pollutants: Relationship With Abnormal Glucose Metabolism and Visceral Adiposity. Diabetes Care (2014) 37(7):1951–8. doi: 10.2337/dc13-2329
132. Valvi D, Mendez MA, Garcia-Esteban R, Ballester F, Ibarluzea J, Goñi F, et al. Prenatal Exposure to Persistent Organic Pollutants and Rapid Weight Gain and Overweight in Infancy. Obesity (Silver Spring) (2014) 22(2):488–96. doi: 10.1002/oby.20603
133. Høyer BB, Ramlau-Hansen CH, Henriksen TB, Pedersen HS, Góralczyk K, Zviezdai V, et al. Body Mass Index in Young School-Age Children in Relation to Organochlorine Compounds in Early Life: A Prospective Study. Int J Obes (Lond) (2014) 38(7):919–25. doi: 10.1038/ijo.2014.58
134. Warner M, Wesselink A, Harley KG, Bradman A, Kogut K, Eskenazi B. Prenatal Exposure to Dichlorodiphenyltrichloroethane and Obesity at 9 Years of Age in the CHAMACOS Study Cohort. Am J Epidemiol (2014) 179(11):1312–22. doi: 10.1093/aje/kwu046
135. Warner M, Aguilar Schall R, Harley KG, Bradman A, Barr D, Eskenazi B. In Utero DDT and DDE Exposure and Obesity Status of 7-Year-Old Mexican-American Children in the CHAMACOS Cohort. Environ Health Perspect (2013) 121(5):631–6. doi: 10.1289/ehp.1205656
136. Cupul-Uicab LA, Klebanoff MA, Brock JW, Longnecker MP. Prenatal Exposure to Persistent Organochlorines and Childhood Obesity in the US Collaborative Perinatal Project. Environ Health Perspect (2013) 121(9):1103–9. doi: 10.1289/ehp.1205901
137. Andersen CS, Fei C, Gamborg M, Nohr EA, Sørensen TI, Olsen J. Prenatal Exposures to Perfluorinated Chemicals and Anthropometry at 7 Years of Age. Am J Epidemiol (2013) 178(6):921–7. doi: 10.1093/aje/kwt057
138. Halldorsson TI, Rytter D, Haug LS, Bech BH, Danielsen I, Becher G, et al. Prenatal Exposure to Perfluorooctanoate and Risk of Overweight at 20 Years of Age: A Prospective Cohort Study. Environ Health Perspect (2012) 120(5):668–73. doi: 10.1289/ehp.1104034
139. Valvi D, Mendez MA, Martinez D, Grimalt JO, Torrent M, Sunyer J, et al. Prenatal Concentrations of Polychlorinated Biphenyls, DDE, and DDT and Overweight in Children: A Prospective Birth Cohort Study. Environ Health Perspect (2012) 120(3):451–7. doi: 10.1289/ehp.1103862
140. De Roos AJ, Ulrich CM, Sjodin A, McTiernan A. Adiposity, Body Composition, and Weight Change in Relation to Organochlorine Pollutant Plasma Concentrations. J Expo Sci Environ Epidemiol (2012) 22(6):617–24. doi: 10.1038/jes.2012.43
141. Lee DH, Lind L, Jacobs DR Jr., Salihovic S, van Bavel B, Lind PM. Associations of Persistent Organic Pollutants With Abdominal Obesity in the Elderly: The Prospective Investigation of the Vasculature in Uppsala Seniors (PIVUS) Study. Environ Int (2012) 40:170–8. doi: 10.1016/j.envint.2011.07.010
142. Mendez MA, Garcia-Esteban R, Guxens M, Vrijheid M, Kogevinas M, Goñi F, et al. Prenatal Organochlorine Compound Exposure, Rapid Weight Gain, and Overweight in Infancy. Environ Health Perspect (2011) 119(2):272–8. doi: 10.1289/ehp.1002169
143. Lee DH, Steffes MW, Sjödin A, Jones RS, Needham LL, Jacobs DR Jr. Low Dose Organochlorine Pesticides and Polychlorinated Biphenyls Predict Obesity, Dyslipidemia, and Insulin Resistance Among People Free of Diabetes. PloS One (2011) 6(1):e15977. doi: 10.1371/journal.pone.0015977
144. Dirinck E, Jorens PG, Covaci A, Geens T, Roosens L, Neels H, et al. Obesity and Persistent Organic Pollutants: Possible Obesogenic Effect of Organochlorine Pesticides and Polychlorinated Biphenyls. Obesity (Silver Spring) (2011) 19(4):709–14. doi: 10.1038/oby.2010.133
145. Rönn M, Lind L, van Bavel B, Salihovic S, Michaëlsson K, Lind PM. Circulating Levels of Persistent Organic Pollutants Associate in Divergent Ways to Fat Mass Measured by DXA in Humans. Chemosphere (2011) 85(3):335–43. doi: 10.1016/j.chemosphere.2011.06.095
146. Andersen CS, Fei C, Gamborg M, Nohr EA, Sørensen TI, Olsen J. Prenatal Exposures to Perfluorinated Chemicals and Anthropometric Measures in Infancy. Am J Epidemiol (2010) 172(11):1230–7. doi: 10.1093/aje/kwq289
147. Karmaus W, Osuch JR, Eneli I, Mudd LM, Zhang J, Mikucki D, et al. Maternal Levels of Dichlorodiphenyl-Dichloroethylene (DDE) may Increase Weight and Body Mass Index in Adult Female Offspring. Occup Environ Med (2009) 66(3):143–9. doi: 10.1136/oem.2008.041921
148. Verhulst SL, Nelen V, Hond ED, Koppen G, Beunckens C, Vael C, et al. Intrauterine Exposure to Environmental Pollutants and Body Mass Index During the First 3 Years of Life. Environ Health Perspect (2009) 117(1):122–6. doi: 10.1289/ehp.0800003
149. Smink A, Ribas-Fito N, Garcia R, Torrent M, Mendez MA, Grimalt JO, et al. Exposure to Hexachlorobenzene During Pregnancy Increases the Risk of Overweight in Children Aged 6 Years. Acta Paediatr (2008) 97(10):1465–9. doi: 10.1111/j.1651-2227.2008.00937.x
150. Kimbro RT, Brooks-Gunn J, McLanahan S. Racial and Ethnic Differentials in Overweight and Obesity Among 3-Year-Old Children. Am J Public Health (2007) 97(2):298–305. doi: 10.2105/ajph.2005.080812
151. Stryjecki C, Alyass A, Meyre D. Ethnic and Population Differences in the Genetic Predisposition to Human Obesity. Obes Rev (2018) 19(1):62–80. doi: 10.1111/obr.12604
152. Czaja K, Ludwicki JK, Góralczyk K, Struciński P. Relationship Between Two Consecutive Lactations and Fat Level in Persistent Organochlorine Compound Concentrations in Human Breast Milk. Chemosphere (2001) 43(4-7):889–93. doi: 10.1016/s0045-6535(00)00449-5
153. Jacobson JL, Fein GG, Jacobson SW, Schwartz PM, Dowler JK. The Transfer of Polychlorinated Biphenyls (PCBs) and Polybrominated Biphenyls (PBBs) Across the Human Placenta and Into Maternal Milk. Am J Public Health (1984) 74(4):378–9. doi: 10.2105/ajph.74.4.378
154. Longnecker MP. Invited Commentary: Why DDT Matters Now. Am J Epidemiol (2005) 162(8):726–8. doi: 10.1093/aje/kwi277
155. Porta M, Puigdomènech E, Ballester F, Selva J, Ribas-Fitó N, Llop S, et al. Monitoring Concentrations of Persistent Organic Pollutants in the General Population: The International Experience. Environ Int (2008) 34(4):546–61. doi: 10.1016/j.envint.2007.10.004
156. Shen H, Main KM, Virtanen HE, Damggard IN, Haavisto AM, Kaleva M, et al. From Mother to Child: Investigation of Prenatal and Postnatal Exposure to Persistent Bioaccumulating Toxicants Using Breast Milk and Placenta Biomonitoring. Chemosphere (2007) 67(9):S256–62. doi: 10.1016/j.chemosphere.2006.05.106
157. Woodruff T, Wolff MS, Davis DL, Hayward D. Organochlorine Exposure Estimation in the Study of Cancer Etiology. Environ Res (1994) 65(1):132–44. doi: 10.1006/enrs.1994.1026
158. Awad R, Zhou Y, Nyberg E, Namazkar S, Yongning W, Xiao Q, et al. Emerging Per- and Polyfluoroalkyl Substances (PFAS) in Human Milk From Sweden and China. Environ Sci Process Impacts (2020) 22(10):2023–30. doi: 10.1039/d0em00077a
159. Domingo JL, Nadal M. Human Exposure to Per- and Polyfluoroalkyl Substances (PFAS) Through Drinking Water: A Review of the Recent Scientific Literature. Environ Res (2019) 177:108648. doi: 10.1016/j.envres.2019.108648
160. Fromme H, Dreyer A, Dietrich S, Fembacher L, Lahrz T, Völkel W. Neutral Polyfluorinated Compounds in Indoor Air in Germany–the LUPE 4 Study. Chemosphere (2015) 139:572–8. doi: 10.1016/j.chemosphere.2015.07.024
161. Fromme H, Tittlemier SA, Völkel W, Wilhelm M, Twardella D. Perfluorinated Compounds–Exposure Assessment for the General Population in Western Countries. Int J Hyg Environ Health (2009) 212(3):239–70. doi: 10.1016/j.ijheh.2008.04.007
162. Monroy R, Morrison K, Teo K, Atkinson S, Kubwabo C, Stewart B, et al. Serum Levels of Perfluoroalkyl Compounds in Human Maternal and Umbilical Cord Blood Samples. Environ Res (2008) 108(1):56–62. doi: 10.1016/j.envres.2008.06.001
163. Schecter A, Colacino J, Haffner D, Patel K, Opel M, Päpke O, et al. Perfluorinated Compounds, Polychlorinated Biphenyls, and Organochlorine Pesticide Contamination in Composite Food Samples From Dallas, Texas, USA. Environ Health Perspect (2010) 118(6):796–802. doi: 10.1289/ehp.0901347
164. Czarnota J, Gennings C, Colt JS, De Roos AJ, Cerhan JR, Severson RK, et al. Analysis of Environmental Chemical Mixtures and Non-Hodgkin Lymphoma Risk in the NCI-SEER NHL Study. Environ Health Perspect (2015) 123(10):965–70. doi: 10.1289/ehp.1408630
165. Philips EM, Jaddoe VWV, Asimakopoulos AG, Kannan K, Steegers EAP, Santos S, et al. Bisphenol and Phthalate Concentrations and its Determinants Among Pregnant Women in a Population-Based Cohort in the Netherlands, 2004-5. Environ Res (2018) 161:562–72. doi: 10.1016/j.envres.2017.11.051
166. Lewis RC, Meeker JD, Peterson KE, Lee JM, Pace GG, Cantoral A, et al. Predictors of Urinary Bisphenol A and Phthalate Metabolite Concentrations in Mexican Children. Chemosphere (2013) 93(10):2390–8. doi: 10.1016/j.chemosphere.2013.08.038
167. Meeker JD, Sathyanarayana S, Swan SH. Phthalates and Other Additives in Plastics: Human Exposure and Associated Health Outcomes. Philos Trans R Soc Lond B Biol Sci (2009) 364(1526):2097–113. doi: 10.1098/rstb.2008.0268
168. Serrano SE, Braun J, Trasande L, Dills R, Sathyanarayana S. Phthalates and Diet: A Review of the Food Monitoring and Epidemiology Data. Environ Health (2014) 13(1):43. doi: 10.1186/1476-069x-13-43
169. Vandenberg LN, Hauser R, Marcus M, Olea N, Welshons WV. Human Exposure to Bisphenol A (BPA). Reprod Toxicol (2007) 24(2):139–77. doi: 10.1016/j.reprotox.2007.07.010
170. Li W, Liu Q, Deng X, Chen Y, Liu S, Story M. Association Between Obesity and Puberty Timing: A Systematic Review and Meta-Analysis. Int J Environ Res Public Health (2017) 14(10):1266. doi: 10.3390/ijerph14101266
171. Mantzoros CS, Rifas-Shiman SL, Williams CJ, Fargnoli JL, Kelesidis T, Gillman MW. Cord Blood Leptin and Adiponectin as Predictors of Adiposity in Children at 3 Years of Age: A Prospective Cohort Study. Pediatrics (2009) 123(2):682–9. doi: 10.1542/peds.2008-0343
172. Aylward LL, Hays SM, Zidek A. Variation in Urinary Spot Sample, 24 H Samples, and Longer-Term Average Urinary Concentrations of Short-Lived Environmental Chemicals: Implications for Exposure Assessment and Reverse Dosimetry. J Expo Sci Environ Epidemiol (2017) 27(6):582–90. doi: 10.1038/jes.2016.54
173. Grundy SM. Multifactorial Causation of Obesity: Implications for Prevention. Am J Clin Nutr (1998) 67(3 Suppl):563s–72s. doi: 10.1093/ajcn/67.3.563S
174. Frederiksen H, Kranich SK, Jørgensen N, Taboureau O, Petersen JH, Andersson AM. Temporal Variability in Urinary Phthalate Metabolite Excretion Based on Spot, Morning, and 24-H Urine Samples: Considerations for Epidemiological Studies. Environ Sci Technol (2013) 47(2):958–67. doi: 10.1021/es303640b
175. Fromme H, Bolte G, Koch HM, Angerer J, Boehmer S, Drexler H, et al. Occurrence and Daily Variation of Phthalate Metabolites in the Urine of an Adult Population. Int J Hyg Environ Health (2007) 210(1):21–33. doi: 10.1016/j.ijheh.2006.09.005
176. Preau JL Jr., Wong LY, Silva MJ, Needham LL, Calafat AM. Variability Over 1 Week in the Urinary Concentrations of Metabolites of Diethyl Phthalate and Di(2-Ethylhexyl) Phthalate Among Eight Adults: An Observational Study. Environ Health Perspect (2010) 118(12):1748–54. doi: 10.1289/ehp.1002231
177. Ye X, Wong LY, Bishop AM, Calafat AM. Variability of Urinary Concentrations of Bisphenol A in Spot Samples, First Morning Voids, and 24-Hour Collections. Environ Health Perspect (2011) 119(7):983–8. doi: 10.1289/ehp.1002701
178. Biserni M, Mesnage R, Ferro R, Wozniak E, Xenakis T, Mein CA, et al. Quizalofop-P-Ethyl Induces Adipogenesis in 3T3-L1 Adipocytes. Toxicol Sci (2019) 170(2):452–61. doi: 10.1093/toxsci/kfz097
179. Blanco J, Guardia-Escote L, Mulero M, Basaure P, Biosca-Brull J, Cabré M, et al. Obesogenic Effects of Chlorpyrifos and its Metabolites During the Differentiation of 3T3-L1 Preadipocytes. Food Chem Toxicol (2020) 137:111171. doi: 10.1016/j.fct.2020.111171
180. Brulport A, Le Corre L, Chagnon MC. Chronic Exposure of 2,3,7,8-Tetrachlorodibenzo-P-Dioxin (TCDD) Induces an Obesogenic Effect in C57BL/6J Mice Fed a High Fat Diet. Toxicology (2017) 390:43–52. doi: 10.1016/j.tox.2017.07.017
181. Chappell VA, Janesick A, Blumberg B, Fenton SE. Tetrabromobisphenol-A Promotes Early Adipogenesis and Lipogenesis in 3T3-L1 Cells. Toxicol Sci (2018) 166(2):332–44. doi: 10.1093/toxsci/kfy209
182. Lee HW, Pyo S. Acrylamide Induces Adipocyte Differentiation and Obesity in Mice. Chem Biol Interact (2019) 298:24–34. doi: 10.1016/j.cbi.2018.10.021
183. Li X, Pham HT, Janesick AS, Blumberg B. Triflumizole Is an Obesogen in Mice That Acts Through Peroxisome Proliferator Activated Receptor Gamma (PPARγ). Environ Health Perspect (2012) 120(12):1720–6. doi: 10.1289/ehp.1205383
184. Mesnage R, Biserni M, Genkova D, Wesolowski L, Antoniou MN. Evaluation of Neonicotinoid Insecticides for Oestrogenic, Thyroidogenic and Adipogenic Activity Reveals Imidacloprid Causes Lipid Accumulation. J Appl Toxicol (2018) 38(12):1483–91. doi: 10.1002/jat.3651
185. Smith A, Yu X, Yin L. Diazinon Exposure Activated Transcriptional Factors CCAAT-Enhancer-Binding Proteins α (C/Ebpα) and Peroxisome Proliferator-Activated Receptor γ (PPARγ) and Induced Adipogenesis in 3T3-L1 Preadipocytes. Pestic Biochem Physiol (2018) 150:48–58. doi: 10.1016/j.pestbp.2018.07.003
Keywords: environmental chemicals, phthalates, persistent organic pollutants, overweight, obesity, bisphenols, environmental obesogens, human biomonitoring
Citation: Mohanto NC, Ito Y, Kato S and Kamijima M (2021) Life-Time Environmental Chemical Exposure and Obesity: Review of Epidemiological Studies Using Human Biomonitoring Methods. Front. Endocrinol. 12:778737. doi: 10.3389/fendo.2021.778737
Received: 17 September 2021; Accepted: 23 October 2021;
Published: 11 November 2021.
Edited by:
Ana Catarina Sousa, University of Evora, PortugalReviewed by:
Yu Ait Bamai, Hokkaido University, JapanCopyright © 2021 Mohanto, Ito, Kato and Kamijima. This is an open-access article distributed under the terms of the Creative Commons Attribution License (CC BY). The use, distribution or reproduction in other forums is permitted, provided the original author(s) and the copyright owner(s) are credited and that the original publication in this journal is cited, in accordance with accepted academic practice. No use, distribution or reproduction is permitted which does not comply with these terms.
*Correspondence: Yuki Ito, eXVrZXlAbWVkLm5hZ295YS1jdS5hYy5qcA==
Disclaimer: All claims expressed in this article are solely those of the authors and do not necessarily represent those of their affiliated organizations, or those of the publisher, the editors and the reviewers. Any product that may be evaluated in this article or claim that may be made by its manufacturer is not guaranteed or endorsed by the publisher.
Research integrity at Frontiers
Learn more about the work of our research integrity team to safeguard the quality of each article we publish.