- 1Department of Clinical Nutrition, Xinhua Hospital, School of Medicine, Shanghai Jiao Tong University, Shanghai, China
- 2Shanghai Key Laboratory of Pediatric Gastroenterology and Nutrition, Shanghai, China
- 3Shanghai Institute for Pediatric Research, Shanghai, China
Objective: This study aimed to evaluate whether serum uric acid (SUA) plays a mediating role in the development of insulin resistance (IR) in obese children and adolescents.
Methods: A total of 369 participants aged 4-17 years with obesity who attended the Nutrition Outpatient Clinic for Obesity at Xinhua Hospital from January 2012 to January 2019 were recruited for this retrospective study. We classified participants into two groups on the basis of HOMA-IR values: the low HOMA-IR group (< 3.16) (n = 222) and the high HOMA-IR group (≥ 3.16) (n = 147).
Results: The univariate analysis found that the high HOMA-IR group had higher BMI, SUA, and fasting insulin (FINS) (P < 0.05). Multiple linear regression analysis and mediating effect analysis indicated that body mass index (BMI) could directly regulate FINS and HOMA-IR (both P < 0.05). The results from the mediating effect analysis found that UA partially played an indirect role in the link between BMI, FINS and HOMA-IR (both P < 0.05) but had no effect on fasting blood glucose (P > 0.05).
Conclusions: SUA should be investigated in obesity and plays a partial mediating role in insulin resistance induced by obesity in obese children and adolescents.
Introduction
Obesity is an increasingly serious clinical and public health problem with more than 100 million obese people worldwide (1). The prevalence of obesity is as high as 20.3% (7-12 y) and 9.6% (13-17 y), respectively, in Chinese children and adolescents (2). Obesity is a driving factor of some endocrine diseases, such as insulin resistance (IR), type 2 diabetes, hypertension, hyperuricemia, and metabolic syndrome (3–6), which places a heavy burden on patients, families, and the public health system (7). Researchers have confirmed that IR is commonly present in obese children and adolescents and is associated with an increased risk of developing type 2 diabetes in adulthood (3, 8). Therefore, identifying and modifying risk factors for IR should be beneficial in preventing diabetes and other metabolic diseases in these high-risk youth.
Obesity diagnosed by body mass index (BMI) has been reported to result in hyperuricemia in more cross-sectional studies (9, 10). Recent studies have found that hyperuricemia not only leads to gout arthritis and nephropathy but may also be related to IR, type 2 diabetes and cardiovascular morbid events (9, 11–13). Thus, SUA interacts with other factors in the modulation of obesity and IR.
However, the mechanism of IR in obese children is still not clear. In addition, to our knowledge, there is no previous epidemiologic study that investigated whether hyperuricemia exerts a mediating effect on the relationship between obesity and IR in children. Therefore, we hypothesized that a BMI-SUA-IR pathway is present in obese children and adolescents. In the current study, we examined the associations among IR, SUA and BMI with multiple linear regression analysis and mediation analysis.
Methods
Subjects
A retrospective study focused on 369 outpatients with obesity, aged 4-17 years, who attended the Nutrition Outpatient Clinic for Obesity at Xinhua Hospital from January 2012 to January 2019 (Figure 1). According to the World Health Organization standards, obesity is defined as BMI ≥ the 95th percentile of children of the same sex and age (14). Participants with severe kidney and liver damage and obesity caused by endocrine diseases or genetic metabolic diseases were excluded from this study. This study was reviewed and approved by the Ethics Committee of Xinhua Hospital, School of Medicine, Shanghai Jiao Tong University (No. XHEC-D-2021-113).
Data Collection and Measurement
All data from the first clinical visit were collected at Xinhua Hospital, located in Shanghai, China. Basic characteristics, including sex, age, height, and weight, were recorded. Accordingly, BMI (kg/m2) was determined as weight in kg divided by height in meters squared.
Triglyceride (TG), total cholesterol (TC), high-density lipoprotein cholesterol (HDL-C), low-density lipoprotein cholesterol (LDL-C), fasting blood glucose (FBG), and fasting insulin (FINS) were tested at the first clinical visit. All of these parameters were measured in the hospital laboratory centre using a Hitachi 7180 automatic biochemical analyzer (Hitachi, Japan). Serum uric acid (SUA) was analyzed using the urase method.
Homeostasis model assessment-β (HOMA-β) and homeostasis model assessment-insulin resistance (HOMA-IR) were calculated to evaluate β-cell function and IR using the following equations: HOMA-β = 20 × FINS (mIU/L)/[FBG (mmol/L)‐3.5]%; HOMA-IR = FBG (mmol/L) × FINS (mIU/L)/22.5 (15). At present, there is no determination of the cut-off point of IR in children. Most studies have defined IR with a HOMA-IR value of 3.16 in children and adolescents (16, 17). Thus, we classified participants into two groups on the basis of HOMA-IR values: the low HOMA-IR group (< 3.16) (n = 222) and the high HOMA-IR group (≥ 3.16) (n = 147).
Statistical Analysis
All statistical analyses were carried out using SPSS V.25.0 statistical software. Descriptive participant characteristics are presented as the mean ± SD for continuous variables and median (p25, p75) for non-normally distributed data.
For the categorical variables, the chi-square test and percentage (%) were used. Univariate analysis was performed to analyze the correlation between IR and variables and the correlation between SUA and variables. Furthermore, multiple linear regression analysis was conducted to evaluate the direct impacts of BMI and SUA on IR using different models: Model 1 (crude model), Model 2 (adjusted for age and sex), and Model 3 (adjusted for age, sex, TG, TC, HDL-C, and LDL-C) and to evaluate the direct impacts of BMI on SUA using different models: Model 1 (crude model), Model 2 (adjusted for age and sex), and Model 3 (adjusted for age, sex, TG, TC, HDL-C, LDL-C, FBG and FINS).
Advanced analysis of the mediating effect was conducted by constructing two causal pathways, a BMI → SUA → IR pathway (Figure 2). For this pathway, BMI was fitted as an independent variable, SUA was the potential mediator, and IR was the outcome of interest. In the pathway, the “total effect” consisted of a “direct effect” (not mediated by SUA) and an “indirect effect” (completely or partly mediated by SUA). If the direct impact was significant [p(c’) < 0.05] and the indirect impact was not significant [both p(a) and p(b) > 0.05], then the link between BMI and IR was not mediated by SUA. The association between BMI and IR was fully mediated by SUA, with the indirect effects being significant [both p(a) and p(b) < 0.05] but the direct effects being not significant [p(c’) > 0.05]. When both the indirect effects and the direct effects were significant [p(a), p(b) and p(c’) < 0.05], SUA played a partial mediating effect on the relationship between BMI and IR. Path analyses were performed using Process v2.16.3 by Andrew F. Hayes (18, 19). All two-sided P values < 0.05 were considered statistically significant.
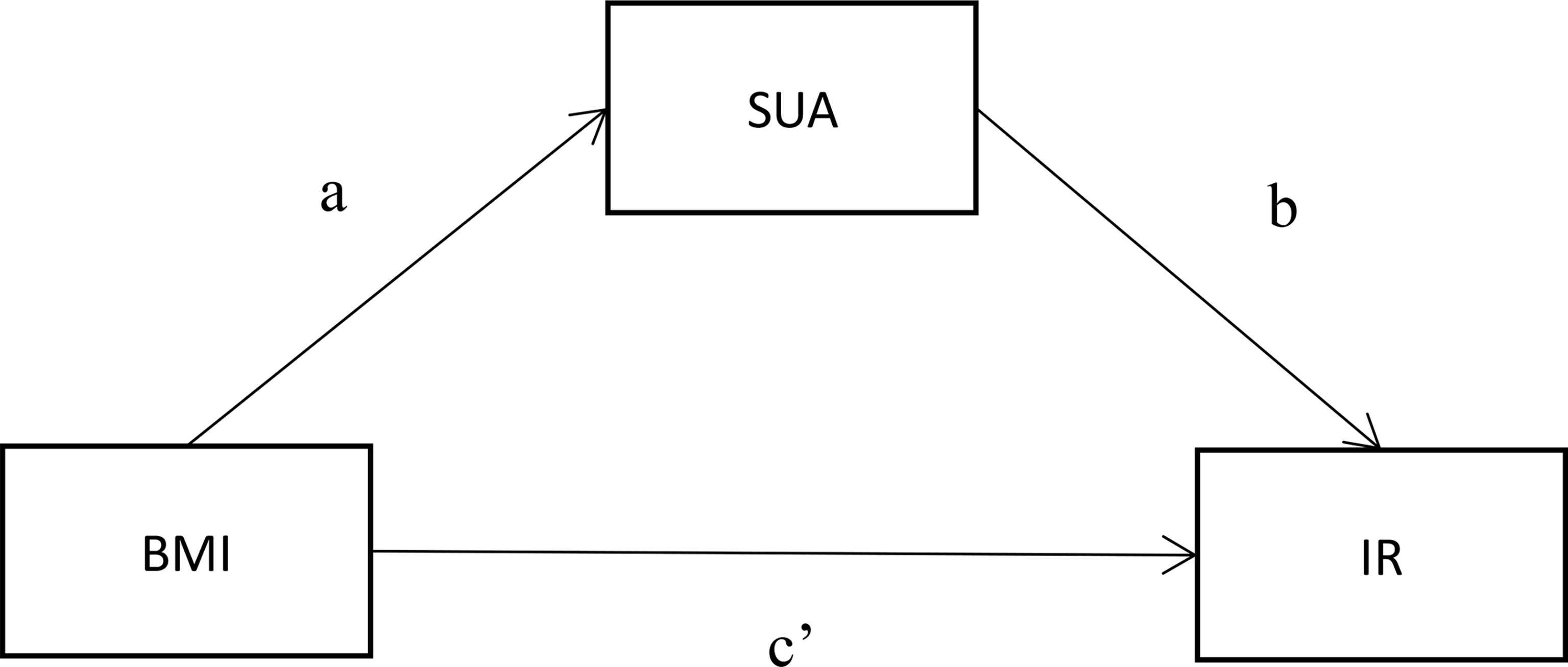
Figure 2 Mediation model for the association between BMI and IR with SUA as a mediator. (A) represents the regression coefficients for the association between BMI and SUA; (B) represents the regression coefficients for the association between SUA and glucose metabolism; (C’) represents the total effect between BMI and glucose metabolism.
Results
Participants Characteristics and Factors Associated With IR Among the Total Sample
A total of 369 participants, with a mean age of 10.53 ± 2.43 years, were recruited in this study, including 252 (68.29%) boys and 117 (31.71%) girls. Compared with the low HOMA-IR group (< 3.16), the high HOMA-IR group (≥3.16) was older and had higher BMI, SUA, TG, TC, LDL-C, FINS, and HOMA-IR but lower HDL-C (all p < 0.05, Table 1).
Correlation Analysis of BMI and SUA With HOMA-IR
The correlation analysis showed that HOMA-IR was closely associated with BMI, SUA, TG, TC, HDL-C, LDL-C and FINS (all p < 0.05, Table 2). To further explore the correlations between HOMA-IR and BMI, HOMA-IR and SUA, the results of the multiple linear regression analysis are presented in Table 3. The results indicated that both BMI and SUA were risk factors for HOMA-IR with the crude model and when adjusted for age and sex (all p < 0.05, Table 3). However, after adjusting for age, sex, TG, TC, HDL-C, LDL-C, and BMI (p < 0.05, Table 3), there was no correlation between SUA and HOMA-IR (p > 0.05, Table 3).
Correlation Analysis of BMI With SUA
The correlation analysis showed that BMI, TG, TC, HDL-C and FINS were closely associated with SUA (all p < 0.05, Table 2). Further multiple linear regression analysis found that BMI was a risk factor for SUA with the crude model adjusted for age and sex and for age, sex, TG, TC, HDL-C, LDL-C, FBG and FINS (all p < 0.05, Table 4).
Direct and Indirect Effects of BMI on Markers of Glucose Metabolism With SUA As a Mediator
As displayed in Table 5, the mediation analysis based on a causal pathway revealed the mediating role of SUA on the relationship between BMI and glucose metabolism. BMI was directly associated with FINS and HOMA-IR [both p (c’)< 0.05, Table 5]. In addition, the potential causal effect of BMI on FINS and HOMA-IR mediated by SUA was also presented [All p (a) and p (b)< 0.05, Table 5], which implied that SUA could have a mediating impact on the link between BMI and glucose metabolism. However, BMI was not directly associated with FBG and not indirectly associated with FBG with SUA as a mediator (all p > 0.05, Table 5).
Discussion
This study has demonstrated several unexpected findings with important clinical implications. The results showed that obesity had direct impacts on regulating FINS and HOMA-IR, and the results of mediating effect analysis also highlighted that the link between obesity and IR was partly mediated by SUA in obese children and adolescents. However, the present study did not find that obesity directly or indirectly affects FBG.
Obesity is an ongoing global epidemic that severely affects adults and children. Many risk factors for IR have been identified in obese children and adolescents, and one of them is serum uric acid. However, it remains controversial whether SUA plays a pathogenic role in IR. In our study, it was found that SUA was a risk factor for IR and exerted a mediating role in the process of obesity-induced IR, which implied that SUA may not only directly affect IR but also be the intermediate link leading to IR. That is, a BMI → SUA → IR pathway is present in obese individuals.
It is known that obesity is strongly associated with IR in obese children and adolescents (10, 20), which was in line with our study. However, the mechanisms of obesity leading to IR are not fully understood to date. There has been growing evidence suggesting that IR is induced through chronic low-grade inflammation, which is promoted by adipocytokines (21–23). A previous study suggested that leptin and adiponectin could modulate the relationship between obesity and IR (24). Other studies also showed a positive association between leptin and IR after controlling for potential confounding factors in children and adolescents (22, 25). Thus, these studies could indicate that adipocytokines are the direct pathway of obesity-insulin resistance.
Furthermore, cross-sectional studies showed that an excessive increase in BMI and waist circumference was associated with significant SUA elevation and hyperuricemia (26–28). It has been demonstrated that mature adipocytes and adipose tissue produce and secrete uric acid (29). Thus, obesity could increase the mRNA expression and activity of xanthine oxidoreductase from adipose tissue (29, 30).
Viewed separately, a close relationship between SUA and IR has long been appreciated, which may be another mechanism to explain IR in obese people. Emerging evidence has shown that hyperuricemia is linked with IR and the subsequent promotion and development of T2DM (9, 31, 32). Similar to these studies, we found that the SUA level was higher in the high HOMA-IR group, and SUA was also an independent risk factor for HOMA-IR. Furthermore, another major finding of the present study was that advanced causal mediating analysis supported that IR was partly mediated by SUA, which implies that an obesity → SUA → IR indirect pathway exists in obese children and adolescents.
Regarding the mechanism involved, the causal relationship between hyperuricemia and IR has not been completely illuminated and is still under investigation. In an animal study with eight-week-old male C57BL/6J mice, increased SUA levels might inhibit IRS1 and Akt insulin signaling and induce IR, which indicates a key role of the reactive oxygen species pathway in high SUA-induced IR (29). There are also reports that SUA-induced IR is caused by an increase in tissue NADPH oxidase or hs-CRP levels (33–35).
In the present study, there was no direct or indirect correlation between obesity and FBG. This result is not surprising, as IR precedes the development of diabetes. This hints that obese children oversecrete insulin in response to increasing insulin resistance to maintain the FBG in the normal range. However, this condition is strongly associated with alterations that represent an increased risk for the development of metabolic disorders and the occurrence of diabetes in adulthood (3, 9).
Nevertheless, there are several limitations in this study that deserve comment. First, because of the retrospective study design, other potential mediators could not be fully taken into account. Second, as obesity in our study was evaluated by BMI, which cannot differentiate excess body weight from increased fat mass or fat-free mass, further studies will be conducted to investigate the effects of different body compositions on hyperuricemia and IR.
In conclusion, SUA plays a partial mediating role in IR induced by obesity in obese children and adolescents. SUA should be investigated in obesity, and the results of the present study indicate this avenue is worth pursuing. In addition, the long-term direct and indirect effects of obesity and SUA on FBG need to be investigated in the future.
Data Availability Statement
The raw data supporting the conclusions of this article will be made available by the authors, without undue reservation.
Ethics Statement
This study was reviewed and approved by the Ethic Committee of Xinhua Hospital, School of Medicine, Shanghai Jiao Tong University (No. XHEC-D-2021-113). Written informed consent to participate in this study was provided by the participants’ legal guardian/next of kin.
Authors Contributions
YN, QT, XuaZ, WC, and YF conceptualized and designed the study, drafted the initial manuscript, and reviewed and revised the manuscript. XueZ, XM, and JS designed the data collection instruments, collected data, carried out the initial analyses, and reviewed and revised the manuscript. All authors contributed to the article and approved the submitted version.
Conflict of Interest
The authors declare that the research was conducted in the absence of any commercial or financial relationships that could be construed as a potential conflict of interest.
Publisher’s Note
All claims expressed in this article are solely those of the authors and do not necessarily represent those of their affiliated organizations, or those of the publisher, the editors and the reviewers. Any product that may be evaluated in this article, or claim that may be made by its manufacturer, is not guaranteed or endorsed by the publisher.
Acknowledgments
The authors thank the participants and the parents of participants.
References
1. NCD Risk Factor Collaboration (NCD-RisC) Worldwide Trends in Body-Mass Index, Underweight, Overweight, and Obesity From 1975 to 2016: A Pooled Analysis of 2416 Population-Based Measurement Studies in 128.9 Million Children, Adolescents, and Adults. Lancet (2017) 390(10113):2627–42. doi: 10.1016/S0140-6736(17)32129-3
2. Zhou Y, Zhang Q, Wang T, Zhang Y, Xu B. Prevalence of Overweight and Obesity in Chinese Children and Adolescents From 2015. Ann Hum Biol (2017) 44(7):642–3. doi: 10.1080/03014460.2017.1371224
3. Romualdo M, Nóbrega FJD, Escrivão MAMS. Insulin Resistance in Obese Children and Adolescents. J Pediatr (Rio J) (2014) 90(6):600–7. doi: 10.1016/j.jped.2014.03.005
4. Kuwahara E, Murakami Y, Okamura T, Komatsu H, Nakazawa A, Ushiku H, et al. Increased Childhood BMI is Associated With Young Adult Serum Uric Acid Levels: A Linkage Study From Japan. Pediatr Res (2017) 81(2):293–8. doi: 10.1038/pr.2016.213
5. Kubota M, Nagai A. Factors Associated With Childhood Obesity in Asian Countries: A Review of Recent Literature. In: . Recent Advances in Obesity in Children (Chapter 2). India: Avid Science, Hyderabad (2015).
6. Weihrauch-Blüher S, Schwarz P, Klusmann JH. Childhood Obesity: Increased Risk for Cardiometabolic Disease and Cancer in Adulthood. Metabolism (2019) 03(92):1–24. doi: 10.1016/j.metabol.2018.12.001
7. Klingelhöfer D, Braun M, Quarcoo D, Brüggmann D, Groneberg DA. Epidemiological Influences and Requirements of Global Childhood Obesity Research. Obes Facts (2021) 14:382–96. doi: 10.1159/000516777
8. Bjerregaard LF, Jensen BW, Ängquist L, Osler M, Sorensen T, Baker JL. Change in Overweight From Childhood to Early Adulthood and Risk for Type 2 Diabetes. N Engl J Med (2018) 378(14):1302–12. doi: 10.1056/NEJMoa1713231
9. Wu WC, Lai YW, Chou YC, Liao YC, You SL, Bai CH, et al. Serum Uric Acid Level as a Harbinger of Type 2 Diabetes: A Prospective Observation. Int J Environ Res Public Health (2020) 17(7):2277. doi: 10.3390/ijerph17072277
10. Thomazini F, de Carvalho BS, de Araujo PX, Franco MDC. High Uric Acid Levels in Overweight and Obese Children and Their Relationship With Cardiometabolic Risk Factors: What is Missing in This Puzzle? J Pediatr Endocrinol Metab (2021) 30:1–7. doi: 10.1515/jpem-2021-0211
11. Cui D, Liu S, Tang M, Lu Y, Zhao M, Mao R, et al. Phloretin Ameliorates Hyperuricemia-Induced Chronic Renal Dysfunction Through Inhibiting NLRP3 Inflammasome and Uric Acid Reabsorption. Phytomedicine (2020) 66:1–10. doi: 10.1016/j.phymed.2019.153111
12. Han T, Lan L, Qu R, Xu Q, Jiang R, Na L, et al. Temporal Relationship Between Hyperuricemia and Insulin Resistance and its Impact on Future Risk of Hypertension. Hypertension (2017) 70(4):703e11. doi: 10.1161/HYPERTENSIONAHA.117.09508
13. Bjelakovic B, Stefanutti C, Bonic D, Vukovic V, Kavaric N, Saranac L, et al. Serum Uric Acid and Left Ventricular Geometry Pattern in Obese Children. Atheroscl Suppl (2019) 40):88–93. doi: 10.1016/j.atherosclerosissup.2019.08.035
14. Cole TJ, Bellizzi MC, Flegal KM, Dietz WH. Establishing a Standard Definition for Child Overweight and Obesity Worldwide: International Survey. BMJ (2000) 320:1240–3. doi: 10.1136/bmj.320.7244.1240
15. Matthews DR, Hosker JP, Rudenski AS, Naylor BA, Treacher DF, Turner RC. Homeostasis Model Assessment: Insulin Resistance and Beta-Cell Function From Fasting Plasma Glucose and Insulin Concentrations in Man. Diabetologia (1985) 28:412–9. doi: 10.1007/BF00280883
16. Keskin M, Kurtoglu S, Kendirci M, Atabek ME, Yazici C. Homeostasis Model Assessment is More Reliable Than the Fasting Glucose/Insulin Ratio and Quantitative Insulin Sensitivity Check Index for Assessing Insulin Resistance Among Obese Children and Adolescents. Pediatrics (2005) 115(4):e500–3. doi: 10.1542/peds.2004-1921
17. Lim SM, Choi DP, Rhee Y, Kim HC. Association Between Obesity Indices and Insulin Resistance Among Healthy Korean Adolescents: The JS High School Study. PloS One (2015) 10(5):e0125238. doi: 10.1371/journal.pone.0125238
18. VanderWeele TJ. A Three-Way Decomposition of a Total Effect Into Direct, Indirect, and Interactive Effects. Epidemiology (2013) 24(2):224–32. doi: 10.1097/EDE.0b013e318281a64e
19. Preacher KJ, Hayes AF. Asymptotic and Resampling Strategies for Assessing and Comparing Indirect Effects in Multiple Mediator Models. Behav Res Methods (2008) 40(3):879–91. doi: 10.3758/brm.40.3.879
20. Sánchez-Escudero V, García Lacalle C, González Vergaz A, Mateo LR, Marqués Cabrero A. The Triglyceride/Glucose Index as an Insulin Resistance Marker in the Pediatric Population and its Relation to Eating Habits and Physical Activity. Endocrinol Diabetes Nutr (Engl Ed) (2021) 68(5):296–303. doi: 10.1016/j.endien.2020.08.015
21. Al-Daghri NM, Al-Attas OS, Alokail M, Alkharfy K, Wani K, Amer OE, et al. Does Visceral Adiposity Index Signify Early Metabolic Risk in Children and Adolescents?: Association With Insulin Resistance, Adipokines, and Subclinical Inflammation. Pediatr Res (2014) 75(3):459–63. doi: 10.1038/pr.2013.229
22. Brand C, Gaya ACA, Dias AF, Agostinis-Sobrinho C, Farinha JB, Boeno FP, et al. Relationship Between Insulin Resistance and Adipocytokines: The Mediator Role of Adiposity in Children. Ann Hum Biol (2020) 47(3):1–7. doi: 10.1080/03014460.2020.1740320
23. Klisic A, Kavaric N, Kotur J, Ninic A. Serum Soluble Transferrin Receptor Levels are Independently Associated With Homeostasis Model Assessment of Insulin Resistance in Adolescent Girls. Arch Med Sci (2021) 1–7. doi: 10.5114/aoms/132757
24. Lopez-Jaramillo P, Gomez-Arbelaez D, Lopez-Lopez J, Lopez-Lopez C, Martínez-Ortega J, Gómez-Rodríguez A, et al. The Role of Leptin/Adiponectin Ratio in Metabolic Syndrome and Diabetes. Horm Mol Biol Clin Invest (2014) 18(1):37–45. doi: 10.1515/hmbci-2013-0053
25. Agostinis-Sobrinho CA, Lacerda E, Moreira C, Abreu S, Lopes L, OliveiraSantos J, et al. Association Between Leptin, Adiponectin, and Leptin/Adiponectin Ratio With Clustered Metabolic Risk Factors in Portuguese Adolescents: The Labmed Physical Activity Study. Ann Nutr Metab (2017) 70(4):321–8. doi: 10.1159/000477328
26. Tamba S, Nishizawa H, Funahashi T, Okauchi Y, Ogawa T, Noguchi M, et al. Relationship Berween the Serum Uric Acid Level, Visceral Fat Accumulation and Serum Adiponectin Concentration in Japanese Men. Internal Med (2008) 47(13):1175–80. doi: 10.2169/internalmedicine.47.0603
27. Zhang ZQ, Deng J, He LP, Ling WH, Su YX, Chen YM. Comparison of Various Anthropometric and Body Fat Indices in Identifying Cardiometabolic Disturbances in Chinese Men and Women. PloS One (2013) 8:e70893. doi: 10.1371/journal.pone.0070893
28. Palmer TM, Nordestgaard BG, Benn M, Tybjaerg-Hansen A, Davey Smith G, Lawlor DA, et al. Association of Plasma Uric Acid With Ischaemic Heart Disease and Blood Pressure: Mendelian Randomisation Analysis of Two Large Cohorts. BMJ (2013) 347:f4262. doi: 10.1136/bmj.f4262
29. Tsushima Y, Nishizawa H, Tochino Y, Nakatsuji H, Sekimoto R, Nagao H, et al. Uric Acid Secretion From Adipose Tissue and its Increase in Obesity. J Biol Chem (2013) 288:27138–49. doi: 10.1074/jbc.M113.485094
30. Cheung KJ, Tzameli I, Pissios P, Rovira I, Gavrilova O, Ohtsubo T, et al. Xanthine Oxidoreductase is a Regulator of Adipogenesis and Ppargamma Activity. Cell Metab (2007) 5(2):115–28. doi: 10.1016/j.cmet.2007.01.005
31. Zhu Y, Hu Y, Huang T, Zhang Y, Li Z, Luo C, et al. High Uric Acid Directly Inhibits Insulin Signalling and Induces Insulin Resistance. Biochem Biophys Res Commun (2014) 447(4):707–14. doi: 10.1016/j.bbrc.2014.04.080
32. Anothaisintawee T, Lertrattananon D, Thamakaison S, Reutrakul S, Ongphiphadhanakul B, Thakkinstian A. Direct and Indirect Effects of Serum Uric Acid on Blood Sugar Levels in Patients With Prediabetes: A Mediation Analysis. J Diabetes Res (2017) 2017. doi: 10.1155/2017/6830671
33. Kelly CC, Lyall H, Petrie JR, Gould GW, Connell JM, Sattar N. Low Grade Chronic Inflflammation in Women With Polycystic Ovarian Syndrome. J Clin Endocrinol Metab (2001) 86(6):2453–5. doi: 10.1210/jcem.86.6.7580
34. Festa A, D'Agostino R, Howard G, Mykkänen L, Tracy RP, Haffner SM. Chronic Subclinical Inflflammation as Part of the Insulin Resistance Syndrome: The Insulin Resistance Atherosclerosis Study (IRAS). Circulation (2000) 102(1):42–7. doi: 10.1161/01.cir.102.1.42
Keywords: insulin resistance, uric acid, obesity, children, adolescents
Citation: Niu Y, Tang Q, Zhao X, Zhao X, Mao X, Sheng J, Cai W and Feng Y (2021) Obesity-Induced Insulin Resistance Is Mediated by High Uric Acid in Obese Children and Adolescents. Front. Endocrinol. 12:773820. doi: 10.3389/fendo.2021.773820
Received: 10 September 2021; Accepted: 15 November 2021;
Published: 03 December 2021.
Edited by:
Elpis Vlachopapadopoulou, Panagiotis & Aglaia Kyriakou Children’s Hospital, GreeceReviewed by:
Evelyn Frias-Toral, Catholic University of Santiago de Guayaquil, EcuadorAleksandra Klisic, Primary Health Care Center Podgorica, Montenegro
Piotr Fichna, Poznan University of Medical Sciences, Poland
Copyright © 2021 Niu, Tang, Zhao, Zhao, Mao, Sheng, Cai and Feng. This is an open-access article distributed under the terms of the Creative Commons Attribution License (CC BY). The use, distribution or reproduction in other forums is permitted, provided the original author(s) and the copyright owner(s) are credited and that the original publication in this journal is cited, in accordance with accepted academic practice. No use, distribution or reproduction is permitted which does not comply with these terms.
*Correspondence: Wei Cai, Y2FpdzE5NzhAMTYzLmNvbQ==; Yi Feng, ZmVuZ3lpQHhpbmh1YW1lZC5jb20uY24=
†These authors have contributed equally to this work