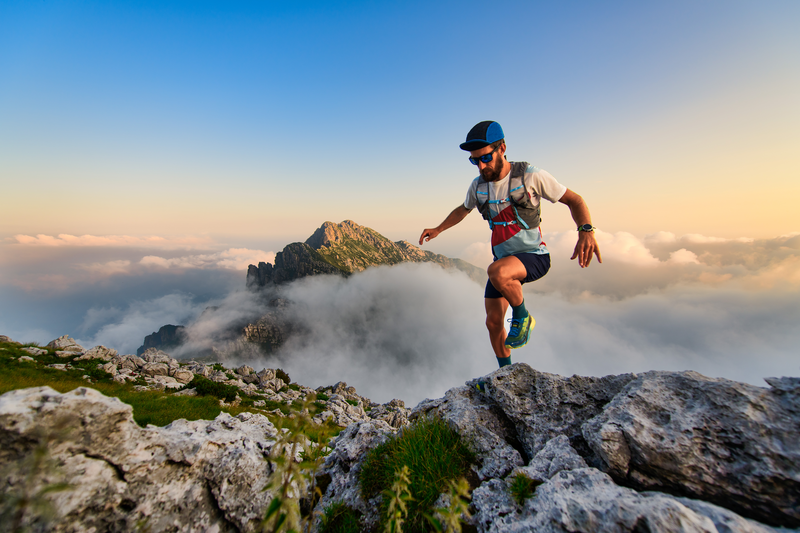
94% of researchers rate our articles as excellent or good
Learn more about the work of our research integrity team to safeguard the quality of each article we publish.
Find out more
ORIGINAL RESEARCH article
Front. Endocrinol. , 09 December 2021
Sec. Pediatric Endocrinology
Volume 12 - 2021 | https://doi.org/10.3389/fendo.2021.771193
This article is part of the Research Topic The Fetal Origins of Metabolic Disorders View all 15 articles
Background: Birth weight is associated with cardiometabolic factors at birth. However, it is unclear when these associations occur in fetal life. We aimed to investigate the associations between fetal growth in different gestational periods and cord blood cardiometabolic factors.
Methods: We included 1,458 newborns from the Born in Guangzhou Cohort Study, China. Z-scores of fetal size parameters [weight, abdominal circumference (AC), and femur length (FL)] at 22 weeks and growth at 22–27, 28–36, and ≥37 weeks were calculated from multilevel linear spline models. Multiple linear regression was used to examine the associations between fetal growth variables and z-scores of cord blood cardiometabolic factors.
Results: Fetal weight at each period was positively associated with insulin levels, with stronger association at 28–36 weeks (β, 0.31; 95% CI, 0.23 to 0.39) and ≥37 weeks (β, 0.15; 95% CI, 0.10 to 0.20) compared with earlier gestational periods. Fetal weight at 28–36 (β, −0.32; 95% CI, −0.39 to −0.24) and ≥37 weeks (β, −0.26; 95% CI, −0.31 to −0.21) was negatively associated with triglyceride levels, whereas weight at 28–36 weeks was positively associated with HDL levels (β, 0.12; 95% CI, 0.04 to 0.20). Similar results were observed for AC. Fetal FL at 22 and 22–27 weeks was associated with increased levels of insulin, glucose, and HDL.
Conclusions: Fetal growth at different gestational periods was associated with cardiometabolic factors at birth, suggesting that an interplay between fetal growth and cardiometabolic factors might exist early in pregnancy.
Fetal life is the period that an individual is most susceptible to environmental influences due to high plasticity of cells and organs (1, 2). Disturbed intrauterine development can have long-term health implications. According to the developmental origins of health and disease hypothesis, both fetal undernutrition and overnutrition may cause permanent changes to glucose–insulin and lipid metabolism and lead to cardiometabolic diseases in childhood and adulthood such as obesity, cardiovascular diseases, hyperlipidemia, and type 2 diabetes mellitus (1, 2).
Cardiometabolic markers in cord blood reflect the intrauterine metabolic environment and conditions of the fetus and may be an indicator of long-term changes in metabolic functions after birth. Previous studies (3–5), including ours (6), have shown that birth weight is associated with cardiometabolic markers in cord blood, such as insulin, glucose, and lipids. For example, birth weight z-score was positively related to cord blood insulin levels (6). In addition, compared with appropriate-for-gestational-age (AGA) infants, small-for-gestational-age (SGA) infants tend to have higher cord blood triglycerides (TG) and lower insulin, high-density lipoprotein cholesterol (HDL), total cholesterol, and low-density lipoprotein cholesterol (LDL) levels (7, 8). In contrast, large-for-gestational-age (LGA) infants have higher cord blood insulin level (9). All these studies have used birth weight as a proxy of fetal growth. However, birth weight is a summary indicator and does not reflect the longitudinal pattern of fetal growth. It is unclear when the association between fetal growth and cardiometabolic markers appears during prenatal period. Also, different fetal biometric parameters represent different dimensions of fetal growth. For instance, abdominal circumference (AC) is considered an indicator of body fat storage and is most predictive of birth weight, whereas femur length (FL) reflects fetal skeletal growth (10). These measures may have different implications for metabolic changes in the fetus. However, no study has examined the associations of different fetal biometric parameters and cardiometabolic markers in cord blood.
In the present study, we investigated the relationship between fetal growth measures (fetal weight, AC, and FL) at different gestational periods and cord blood cardiometabolic factors. The findings of our study may provide insights into the critical period for fetal programming of cardiometabolic health in childhood and adulthood.
This study was part of the Born in Guangzhou Cohort Study—BIGCS, a prospective study led by Guangzhou Women and Children’s Medical Center (GWCMC) that aimed to investigate the influence of environmental, genetic, and social factors on the health of pregnant women and the next generation. The protocol and cohort profile of BIGCS have been previously published (11). Briefly, BIGCS pregnant women are invited to participate when they undergo the first routine prenatal check-ups at GWCMC if they are less than 20 weeks of gestation, planned to give birth at GWCMC, and intended to stay in Guangzhou for ≥3 years after delivery. These women and their children are followed up at multiple time points to collect epidemiological and clinical information and biospecimens. BIGCS has been approved by the Ethics Committee of GWCMC. Written consent has been obtained from all participants.
The eligibility criteria for the current analysis include 1) availability of cord blood samples, 2) availability of maternal fasting blood samples at 14–27 weeks of gestation and data on 2-h 75-g oral glucose tolerance test (OGTT) of the mother, 3) no severe disease before pregnancy (e.g., type 1 or type 2 diabetes, hypertension, kidney diseases), and (4) availability of data on maternal demographic characteristics. This analysis included 1,744 mother–child pairs who were randomly selected from those eligible. Mother–child pairs with no fetal ultrasound growth data (n = 212) or covariates (n = 74) were excluded. This study finally included 1,458 pairs of mothers and children.
Data on fetal biometric measures, including biparietal diameter, head circumference, AC, and FL, were obtained through ultrasound scanning and extracted from the hospital electronic system. Fetal weight was estimated (EFW) using the INTERGROWTH-21st formula based on AC and FL (12). On average, each pregnant woman had three (range, 1–7) ultrasound examinations for fetal growth. An ultrasound examination is routinely performed in the first trimester to confirm gestational age based on crown–rump length. For pregnant women who do not attend antenatal care in the first trimester, gestational age is assessed in early second trimester based on fetal biometrics, including bilateral parietal diameter, head circumference, AC, FL, or cerebellar diameter (13, 14). Well-trained and licensed ultrasonographers in GWCMC conducted these ultrasound measurements. As our previous study shows (15), the mean error of EFW estimation ([EFW − birth weight]/birth weight × 100%) is low (about 4%), and the correlation between two consecutive measurements of fetal biometric parameters performed within a week was high (r > 0.95). These results indicate good quality of the ultrasound data.
Based on our previous study (15) and other studies (16–18), a multilevel linear spline model was used to evaluate the patterns of fetal growth in different gestational periods. Briefly, a fractional polynomial model was first used to determine the best-fitting curve for fetal size (including EFW and birth weight) by gestational age for the whole sample. Two knots (i.e., 28 and 37 weeks) were then selected to divide the entire gestation period into three intervals: “mid-pregnancy” (22–27 weeks), “early-third trimester” (28–36 weeks), and “late-third trimester” (≥37 weeks). We then fitted multilevel linear spline models to estimate fetal growth during each period. The resulting random effects from the models denote individual deviations from average size in early pregnancy (i.e., 22 weeks) and the average growth velocities in the three gestational intervals, which were converted into sex-specific z-scores before further analyses. More information on the modeling process can be found in our previous report (15). The same modeling approach was applied to generate AC and FL z-scores based on ultrasound measurements. Fetuses were also classified into “slow,” “normal,” and “fast” growth if they had a z-score ≤–1.28 (i.e., 10th percentile), –1.28 to 1.28, and >1.28 (i.e., 90th percentile), respectively. These cutoff values are equivalent to those for SGA, AGA, and LGA at birth which are commonly used in clinical practice.
Trained midwives collected venous umbilical cord blood using tubes with EDTA anticoagulants. The blood was centrifuged within 24 h. Plasma samples were obtained and stored at −80°C until analysis. A third-party medical laboratory assessed the levels of cardiometabolic factors in cord blood, including insulin, glucose, total cholesterol, HDL, LDL, and TG. Specifically, insulin levels (μIU/ml) were measured using the Roche Immunology Analyzer (cobas 8000 e602, Roche, Basel, Switzerland), and glucose (mmol/L), total cholesterol (mmol/L), HDL (mmol/L), LDL (mmol/L), and TG (mmol/L) were measured using the Roche Chemistry Analyzer (cobas 8000 c702, Roche, Basel, Switzerland). All tests had low (<2%) intraday and interday coefficients of variation. Glucose levels from 77 samples were below the lower limit (i.e., 0.11 mmol/L) of the linear detection range and were therefore excluded from further analysis.
We collected information on maternal age, height, pre-pregnancy weight, parity, education level, monthly income, and active/passive smoking during pregnancy using a self-reported questionnaire at recruitment (around 16 weeks of gestation). Height and pre-pregnancy weight were used to calculate pre-pregnancy body mass index (BMI = weight [kg]/height [m]2), which was then categorized into underweight (BMI < 18.5 kg/m2), normal weight (BMI 18.5–23.9), and overweight/obesity (BMI ≥ 24) based on the Chinese standard (19). In addition, we obtained information on pregnancy complications [hypertensive disorder in pregnancy and gestational diabetes mellitus (GDM)] from the medical records after delivery. Hypertensive disorder in pregnancy was identified using medical record data based on the International Classification of Diseases, Tenth Revision (ICD-10) (O13–O16). The diagnosis of GDM was made using the International Association of Diabetes and Pregnancy Study Group criteria (20).
The levels of cord blood cardiometabolic factors (insulin, glucose, total cholesterol, triglyceride, HDL, and LDL) were log-transformed to address non-normality of their distributions. Since different units and scales were used for different cardiometabolic factors, we then converted the log-transformed variables to z-scores (using internal means and standard deviations) for ease of comparison of results.
We used multiple linear regression model to examine the association between fetal growth variables (z-scores and growth categories for weight, AC, and FL) at each period and the levels of each cord blood cardiometabolic factor, and the regression coefficient (β) and 95% CI were calculated. Covariates included in these models were maternal age, education level, monthly income, parity, pre-pregnancy BMI, active/passive smoking during pregnancy, hypertensive disorder in pregnancy, and GDM. In the models for fetal growth velocity in the early-third and late-third trimesters, we further adjusted for growth variables in previous periods. However, fetal size in early pregnancy was not included for adjustment because it was highly correlated to growth velocity in mid-pregnancy. In the models of fetal growth categories, the group of “normal” growth was used as a referent and compared with the groups of “slow” and “fast” growth. We first included fetal weight, AC, and FL separately in the models.
We conducted exploratory stratified analyses to investigate whether the associations between fetal growth z-scores and cord blood cardiometabolic factor levels differed by maternal characteristics such as pre-pregnancy BMI (underweight vs. normal weight and overweight/obesity), parity (nulliparous vs. multiparous), and GDM (yes vs. no), which have been shown to affect fetal growth or fetal metabolic status. Multiplicative interaction term was included in the multiple linear regression model to assess whether the interaction between fetal growth z-scores and these maternal and neonatal characteristics exists. In addition, we conducted sensitivity analysis for the associations between continuous fetal growth variables and cardiometabolic factors, by excluding preterm infants and women with hypertensive disorder in pregnancy.
All data were analyzed by SPSS 21.0, and the statistical significance was set as a two-tailed P <0.05.
Participant characteristics are presented in Table 1. The mean age of the participants was 29.5 years. Most of them (80%) were nulliparous. About one in four were underweight before pregnancy, whereas around one in 10 were overweight or obese. About 12% of these women had GDM, 3% had hypertensive disorders in pregnancy, and 29.3% were exposed to active or passive smoking during pregnancy. The mean birth weight of their infants was 3,203 g.
Fetal weight z-scores at each gestational period were positively associated with cord blood insulin levels, with stronger associations observed in early- (β, 0.31; 95% CI, 0.23–0.39) and late-third trimesters (β, 0.15; 95% CI, 0.10 to 0.20) (Figure 1, upper section). Besides this, fetal weight z-scores in early- (β, −0.32; 95% CI, −0.39 to −0.24) and late-third (β, −0.26; 95% CI, −0.31 to −0.21) trimesters, but not early and mid-pregnancy, were negatively associated with cord blood TG levels. Fetal weight z-score in early-third trimester was also positively associated with HDL levels (β, 0.12; 95% CI, 0.04 to 0.20). No evident association was observed between fetal weight z-scores and glucose, total cholesterol, and LDL (Figure 1, upper section).
Figure 1 Associations between fetal weight growth in different periods and cord blood metabolic factors. *, >0 and <0.001.
Results for fetal weight categories (slow, normal, or fast) were consistent with overall findings for fetal weight z-scores. Compared with normal-growth fetuses, slow-growth fetuses had lower levels of cord blood insulin in early- and late-third trimester, whereas fast-growth fetuses had higher insulin levels in early and late trimesters (Figure 1, middle and lower sections). In addition, slow-growth fetuses had higher levels of TG in early- and late-third trimester and lower levels of HDL at birth, whereas fast-growth fetuses had lower levels of TG in each gestational period.
The patterns of associations between fetal AC z-scores and cardiometabolic factors were similar to those for fetal weight growth, although the magnitude of the associations appeared to be smaller (Figure 2). The associations for fetal AC categories (slow, normal, or fast) were also similar to the overall results for fetal weight growth. However, the associations between AC categories in late-third trimester and insulin and TG were close to null, whereas the associations for fetal weight categories remained statistically significant.
Figure 2 Association between fetal abdominal circumference growth in different periods and cord blood metabolic factors. *, >0 and <0.001.
Fetal FL z-scores in early and mid-pregnancy were associated with increased levels of cord blood insulin, glucose, and HDL (Figure 3, upper section). In line with these results, fetuses with slow FL growth in early and mid-pregnancy had lower levels of glucose and HDL at birth, while fetuses with fast FL growth had higher cord blood insulin and HDL levels (Figure 3, middle and lower sections). There was insufficient evidence for an association between FL continuous z-scores and cord blood TG levels. However, we found that babies with slow FL growth in early- and late-third trimesters had higher TG compared with those with normal FL growth.
Figure 3 Association between fetal femur length growth in different periods and cord blood metabolic factors. *, >0 and <0.001.
Stratified analysis by GDM revealed negative associations of fetal weight and AC z-scores before 37 weeks of gestation with cord blood TG being stronger among women without diabetes than those with diabetes (Pinteraction, <0.05) (Supplementary Tables 1, 2). No significant interaction was observed between FL and maternal diabetes status on cardiometabolic factors levels (Supplementary Table 3).
There was no evident interaction between fetal growth variables and maternal pre-pregnancy BMI (Supplementary Tables 4–6) and parity (Supplementary Tables 7–9) for any of the cardiometabolic factors.
In sensitivity analyses, the results were similar to the overall analysis when we excluded women with hypertensive disorder in pregnancy (n = 44) (Supplementary Table 10) or when we excluded preterm infants (n = 61) (Supplementary Table 11).
We found that fetal weight at each period was positively associated with cord blood insulin, with stronger associations observed in early- and late-third trimesters than early and mid-pregnancy. Fetal weight in early- and late-third trimesters was also negatively associated with TG and positively associated with HDL. Associations for fetal AC were similar to those for fetal weight. These results suggest that the third trimester could be the important period for fetal insulin and lipid metabolism. On the other hand, fetal FL in the early and mid-pregnancy was positively associated with cord blood insulin, glucose, and HDL, indicating that the first half of pregnancy might be a crucial stage for the interplay between fetal skeletal growth and these metabolic factors. To our knowledge, this is the first study to investigate the associations between fetal growth in different gestational periods and cord blood cardiometabolic factors.
Our observation that fetal weight and AC were positively associated with cord blood insulin is consistent with previous studies. Ong et al. found that cord blood insulin was positively associated with neonatal birth weight (21). Similarly, SGA neonates had hypoinsulinemia in cord blood at birth compared with AGA neonates (22), whereas insulin levels in LGA infants were significantly higher (21, 23). In addition, a previous study found gestational weight gain rates during the first and second trimesters, but not the third trimester, to be positively associated with cord blood insulin levels (24). In line with this study, we observed that fetal weight at early and mid-pregnancy was associated with insulin levels at birth; however, in contrast to this previous study (24), we found that fetal weight in the third trimester was also associated with cord blood insulin. Discrepancies in the results between the two studies might be related to the difference in exposure definition (e.g., self-reported gestational weight gain vs. ultrasound-based fetal growth), and thus, the results might be not directly comparable.
Our study revealed the association of fetal weight and AC with cord blood insulin to be stronger in late pregnancy than in early pregnancy. Insulin is an important hormone to promote fetal growth. It can not only stimulate fetal insulin sensitive cells to promote tissue utilization of glucose but also promote the storage of glycogen and fat, thereby promoting the growth of the fetus (25). Since fetal growth is fastest in late pregnancy (26), we speculate that fetuses in late pregnancy might be more sensitive to the growth-promoting effect of insulin than fetuses in early pregnancy. On the other hand, fetal growth may affect the levels of circulating insulin. It has been shown that fetal growth restriction can affect the maturation of fetal islet β cells, lead to the inhibition of fetal islet β-cell proliferation, reduce insulin secretion, hinder the activation of amino acid transport system, inhibit protein and fat synthesis, promote lipolysis, and inhibit fetal growth and development (27). These responses enable the fetus to adapt to the adverse intrauterine environment and redistribute nutrients, which ensures the growth and development of the main organs (e.g., brain) of the fetus but results in decreased fetal growth rate (28).
In addition, we found that in the second and third trimesters of pregnancy, fetal weight and AC were negatively associated with cord blood TG. This finding is consistent with previous studies which found that fetuses with intrauterine growth restriction had higher cord blood TG compared with normal-growth fetus (29, 30). Another study showed that AC was negatively associated with cord blood TG levels among growth-restricted fetuses (31). We also found that fetal weight and AC in late pregnancy were positively correlated with cord blood HDL. Interestingly, it has been shown that AC at birth was negatively associated with blood lipid levels in adulthood (32). Taken together, these findings suggest that abnormal fetal growth in late pregnancy might be critical for long-term lipid profile. A possible explanation for these findings is that decelerated fetal growth (especially for AC) may represent insufficient growth of the liver, which could result in dysregulation of lipid metabolism (32). However, the mechanisms for this association need to be studied in future studies.
The relationships between fetal FL or birth length and cord blood cardiometabolic factors have been rarely investigated previously. In the present study, we observed that femur growth in early and mid-pregnancy was positively associated with cord blood insulin and glucose. Fetal insulin, in addition to being a growth hormone, is also an osteogenic hormone, which can stimulate fetal bone mineralization and cartilage synthesis (33) and regulate fetal bone growth (34). Similarly, glucose is essential for fetal osteogenesis and cartilage formation. In the skeletal system, glucose is an important energy source for the development, growth, and maintenance of bone and articular cartilage (35). Therefore, insulin and glucose are two important nutrients for fetal FL growth in the early stage of pregnancy. We also found fetal FL in early and mid-pregnancy to be positively associated with cord blood HDL. The biological explanation for this association warrants further investigation.
In stratified analysis, we found that GDM modified the associations between fetal growth (weight and AC) and cord blood TG. For example, for pregnant women with diabetes, fetal weight growth before 28 weeks of gestation was positively associated with cord blood TG. By contrast, among women without diabetes, fetal weight growth in the same period was negatively associated with TG. This divergence of association was less pronounced after 28 weeks of gestation. We speculate that it could be related to the metabolic dysfunction induced by GDM. Fetal hyperinsulinemia, resulting from maternal GDM, can promote the synthesis of fat and leads to fetal overgrowth. This effect might be attenuated after 28 weeks when the dietary interventions are usually given to GDM patients for optimal glycemic control to improve pregnancy outcomes (36).
In this study, we found a positive association between fetal weight and the levels of insulin and high-density lipoprotein in cord blood metabolic factors in all gestational periods, especially during the third trimester. Specifically, slow-growth fetuses in the third trimester had an adverse lipid profile (i.e., high TG and low HDL) at birth, whereas fast-growth fetuses in the third trimester had higher insulin insensitivity (reflected by higher cord blood insulin level). Since adverse cardiometabolic profile at birth may be related to long-term cardiometabolic diseases, efforts should be made to ensure an optimal fetal growth during late pregnancy to improve the short- and long-term cardiometabolic health. However, future studies are still needed to validate our findings.
Based on a series of ultrasound measurements, we were able to assess longitudinal fetal growth patterns and their associations with cord blood cardiometabolic factors, which have not been reported previously. The prospective cohort design of the current study could reduce the recall bias for data collection. Compared with previous studies on birth weight and cord blood cardiometabolic factors (4, 9, 37), our study had relatively larger sample size and thus increased statistical power. A limitation of our study was that we could only measure metabolic factors in umbilical cord blood at birth. Information about the fetal metabolic status before birth would be useful, which allows the assessment for the dynamic changes of fetal metabolic status. However, it is impractical to collect cord blood samples before birth for normal pregnancies. Another limitation is that only a limited number of cardiometabolic factors were measured and data on adipokines (e.g., leptin and adiponectin), which may also play a role in fetal growth, were unavailable.
This study shows that fetal growth in different gestational periods was associated with cord blood cardiometabolic factors. These associations were more pronounced for fetal weight and AC growth after 28 weeks than those before 28 weeks, whereas associations for FL growth appeared to stronger before 28 weeks. Our findings suggest that fetal growth and cardiometabolic factors might interplay since early pregnancy. Further studies are needed to elucidate the mechanisms underlying these associations.
The original contributions presented in the study are included in the article/Supplementary Material. Further inquiries can be directed to the corresponding authors.
The studies involving human participants were reviewed and approved by the Ethics Committee of Guangzhou Women and Children’s Medical Center. The patients/participants provided their written informed consent to participate in this study.
J-RH and XQ conceptualized and designed the study, contributed to the data analysis, interpreted the data, and reviewed and revised the manuscript. J-SH, Q-ZC, S-YZ, J-YZ, and C-PZ analyzed and interpreted the data and draft and revised the manuscript. RR contributed to the data analysis and reviewed and revised the manuscript. Y-ML, Y-SK, and J-HL contributed to the data collection and reviewed and revised the manuscript. All authors approved the final manuscript as submitted and agree to be accountable for all aspects of the work.
The present study was supported by the grants from Ministry of Science and Technology of People's Republic of China (2019YFC0121905) and National Natural Science Foundation of China (grant number, 81703244 and 81673181).
The authors declare that the research was conducted in the absence of any commercial or financial relationships that could be construed as a potential conflict of interest.
All claims expressed in this article are solely those of the authors and do not necessarily represent those of their affiliated organizations, or those of the publisher, the editors and the reviewers. Any product that may be evaluated in this article, or claim that may be made by its manufacturer, is not guaranteed or endorsed by the publisher.
The authors are grateful to the pregnant women who participated in the BIGCS, to the management team, all obstetric care providers who assisted in the implementation of the study, and to all members of the BIGCS Study Group. We thank Ms. Yuting Yang for helping us with the English language editing on the first draft of the manuscript.
The Supplementary Material for this article can be found online at: https://www.frontiersin.org/articles/10.3389/fendo.2021.771193/full#supplementary-material
1. Perng W, Oken E, Dabelea D. Developmental Overnutrition and Obesity and Type 2 Diabetes in Offspring. Diabetologia (2019) 62:1779–88. doi: 10.1007/s00125-019-4914-1
2. Hoffman DJ, Reynolds RM, Hardy DB. Developmental Origins of Health and Disease: Current Knowledge and Potential Mechanisms. Nutr Rev (2017) 75:951–70. doi: 10.1093/nutrit/nux053
3. Xu M, Liu B, Wu MF, Chen HT, Cianflone K, Wang ZL. Relationships Among Acylation-Stimulating Protein, Insulin Resistance, Lipometabolism, and Fetal Growth in Gestational Diabetes Mellitus Women. J Obstet Gynaecol (2015) 35:341–5. doi: 10.3109/01443615.2014.960376
4. Terrazzan AC, Procianoy RS, Silveira RC. Neonatal Cord Blood Adiponectin and Insulin Levels in Very Low Birth Weight Preterm and Healthy Full-Term Infants. J Maternal-Fetal Neonatal Med (2014) 27:616–20. doi: 10.3109/14767058.2013.823939
5. Simón-Muela I, Näf S, Ballesteros M, Vendrell J, Ceperuelo-Mallafre V, de la Flor M, et al. Gender Determines the Actions of Adiponectin Multimers on Fetal Growth and Adiposity. Am J Obstet Gynecol (2013) 208:481.e1–7. doi: 10.1016/j.ajog.2013.02.045
6. Wang J, Shen S, Price MJ, Lu J, Sumilo D, Kuang Y, et al. Glucose, Insulin, and Lipids in Cord Blood of Neonates and Their Association With Birthweight: Differential Metabolic Risk of Large for Gestational Age and Small for Gestational Age Babies. J Pediatr (2020) 220:64–+. doi: 10.1016/j.jpeds.2020.01.013
7. Katragadda T, Mahabala RS, Shetty S, Baliga S. Comparison of Cord Blood Lipid Profile in Preterm Small for Gestational Age and Appropriate for Gestational Age Newborns. J Clin Diagn Res: JCDR (2017) 11:SC05–7. doi: 10.7860/jcdr/2017/24247.9197
8. Bansal N, Cruickshank JK, McElduff P, Durrington PN. Cord Blood Lipoproteins and Prenatal Influences. Curr Opin Lipidol (2005) 16:400–8. doi: 10.1097/01.mol.0000174154.61307.16
9. Hou RL, Jin WY, Chen XY, Jin Y, Wang XM, Shao J, et al. Cord Blood C-Peptide, Insulin, HbA1c, and Lipids Levels in Small- and Large-for-Gestational-Age Newborns. Med Sci Monit (2014) 20:2097–105. doi: 10.12659/msm.890929
10. Degani S. Fetal Biometry: Clinical, Pathological, and Technical Considerations. Obstet Gynecol Surv (2001) 56:159–67. doi: 10.1097/00006254-200103000-00023
11. Qiu X, Lu J-H, He J-R, Lam K-b, Shen S-Y, Guo Y, et al. The Born in Guangzhou Cohort Study (BIGCS). Eur J Epidemiol (2017) 32:337–46. doi: 10.1007/s10654-017-0239-x
12. Stirnemann J, Villar J, Salomon LJ, Ohuma E, Ruyan P, Altman DG, et al. International Estimated Fetal Weight Standards of the INTERGROWTH-21st Project. Ultrasound Obstet Gynecol (2017) 49:478–86. doi: 10.1002/uog.17347
13. C.U.S.D. ASSOCIATION. Prenatal Ultrasound Guidelines (2012). Chin J OF Med Ultrasound(Electronic Version) (2012) 9:574–80. doi: 10.3877/cma.j.issn.1672-6448.2012.07.002
14. American College of Obstetricians and Gynecologists’ Committee on Obstetric Practice. Committee Opinion No 700: Methods for Estimating the Due Date. Obstet Gynecol (2017) 129:e150–4. doi: 10.1097/aog.0000000000002046
15. He JR, Ramakrishnan R, Wei XL, Lu JH, Lu MS, Xiao WQ, et al. Fetal Growth at Different Gestational Periods and Risk of Impaired Childhood Growth, Low Childhood Weight and Obesity: A Prospective Birth Cohort Study. Bjog (2021) 128:1615–24. doi: 10.1111/1471-0528.16698
16. Norris T, Johnson W, Petherick E, Cameron N, Oddie S, Johnson S, et al. Investigating the Relationship Between Fetal Growth and Academic Attainment: Secondary Analysis of the Born in Bradford (BiB) Cohort. Int J Epidemiol (2018) 47:1475–84. doi: 10.1093/ije/dyy157
17. Howe LD, Tilling K, Matijasevich A, Petherick ES, Santos AC, Fairley L, et al. Linear Spline Multilevel Models for Summarising Childhood Growth Trajectories: A Guide to Their Application Using Examples From Five Birth Cohorts. Stat Methods Med Res (2016) 25:1854–74. doi: 10.1177/0962280213503925
18. Lampl M, Kusanovic JP, Erez O, Espinoza J, Gotsch F, Goncalves L, et al. Early Rapid Growth, Early Birth: Accelerated Fetal Growth and Spontaneous Late Preterm Birth. Am J Hum Biol (2009) 21:141–50. doi: 10.1002/ajhb.20840
19. Chen C, Lu FC. The Guidelines for Prevention and Control of Overweight and Obesity in Chinese Adults. BioMed Environ Sci (2004) 17 (Suppl):1–36.
20. Zhu W-w, Yang H-x. Diagnosis Gestational Diabetes Mellitus in China. Diabetes Care (2013) 36:E76–6. doi: 10.2337/dc12-2624
21. Ong K, Kratzsch J, Kiess W, Costello M, Scott C, Dunger D. Size at Birth and Cord Blood Levels of Insulin, Insulin-Like Growth Factor I (IGF-I), IGF-II, IGF-Binding Protein-1 (IGFBP-1), IGFBP-3, and the Soluble IGF-II/mannose-6-Phosphate Receptor in Term Human Infants. The ALSPAC Study Team. Avon Longitudinal Study of Pregnancy and Childhood. J Clin Endocrinol Metab (2000) 85:4266–9. doi: 10.1210/jc.85.11.4266
22. Okdemir D, Hatipoglu N, Kurtoglu S, Siraz UG, Akar HH, Muhtaroglu S, et al. The Role of Irisin, Insulin and Leptin in Maternal and Fetal Interaction. J Clin Res Pediatr Endocrinol (2018) 10:307–15. doi: 10.4274/jcrpe.0096
23. Dornhorst A, Nicholls JS, Ali K, Andres C, Adamson DL, Kelly LF, et al. Fetal Proinsulin and Birth Weight. Diabetic Med (1994) 11:177–81. doi: 10.1111/j.1464-5491.1994.tb02016.x
24. Rifas-Shiman SL, Fleisch A, Hivert MF, Mantzoros C, Gillman MW, Oken E. First and Second Trimester Gestational Weight Gains are Most Strongly Associated With Cord Blood Levels of Hormones at Delivery Important for Glycemic Control and Somatic Growth. Metabolism (2017) 69:112–9. doi: 10.1016/j.metabol.2017.01.019
25. Fowden AL, Hill DJ. Intra-Uterine Programming of the Endocrine Pancreas. Br Med Bull (2001) 60:123–42. doi: 10.1093/bmb/60.1.123
26. Grantz KL, Kim S, Grobman WA, Newman R, Owen J, Skupski D, et al. Fetal Growth Velocity: The NICHD Fetal Growth Studies. Am J Obstet Gynecol (2018) 219:285.e1–285.e36. doi: 10.1016/j.ajog.2018.05.016
27. Gatford KL, Simmons RA. Prenatal Programming of Insulin Secretion in Intrauterine Growth Restriction. Clin Obstet Gynecol (2013) 56:520–8. doi: 10.1097/GRF.0b013e31829e5b29
28. Saltiel AR, Kahn CR. Insulin Signalling and the Regulation of Glucose and Lipid Metabolism. Nature (2001) 414:799–806. doi: 10.1038/414799a
29. Milenkovic S, Jankovic B, Mirkovic L, Jovandaric MZ, Milenkovic D, Otasevic B. Lipids and Adipokines in Cord Blood and at 72h in Discordant Dichorionic Twins. Fetal Pediatr Pathol (2017) 36:106–22. doi: 10.1080/15513815.2016.1242675
30. Kelishadi R, Badiee Z, Adeli K. Cord Blood Lipid Profile and Associated Factors: Baseline Data of a Birth Cohort Study. Paediatric Perinatal Epidemiol (2007) 21:518–24. doi: 10.1111/j.1365-3016.2007.00870.x
31. Roberts A, Nava S, Bocconi L, Salmona S, Nicolini U. Liver Function Tests and Glucose and Lipid Metabolism in Growth-Restricted Fetuses. Obstet Gynecol (1999) 94:290–4. doi: 10.1016/s0029-7844(99)00235-5
32. Barker DJ, Martyn CN, Osmond C, Hales CN, Fall CH. Growth In Utero and Serum Cholesterol Concentrations in Adult Life. BMJ (Clin Res ed.) (1993) 307:1524–7. doi: 10.1136/bmj.307.6918.1524
33. Canalis EM, Dietrich JW, Maina DM, Raisz LG. Hormonal Control of Bone Collagen Synthesis In Vitro. Effects Insulin Glucagon Endocrinol (1977) 100:668–74. doi: 10.1210/endo-100-3-668
35. Mobasheri A. Glucose: An Energy Currency and Structural Precursor in Articular Cartilage and Bone With Emerging Roles as an Extracellular Signaling Molecule and Metabolic Regulator. Front Endocrinol (2012) 3:153. doi: 10.3389/fendo.2012.00153
36. Landon MB, Spong CY, Thom E, Carpenter MW, Ramin SM, Casey B, et al. Randomized Trial of Treatment for Mild Gestational Diabetes. N Engl J Med (2009) 361:1339–48. doi: 10.1056/NEJMoa0902430
37. Cianfarani S, Maiorana A, Geremia C, Scire G, Spadoni GL, Germani D. Blood Glucose Concentrations are Reduced in Children Born Small for Gestational Age (SGA), and Thyroid-Stimulating Hormone Levels are Increased in SGA With Blunted Postnatal Catch-Up Growth. J Clin Endocrinol Metab (2003) 88:2699–705. doi: 10.1210/jc.2002-021882
Keywords: fetal growth, cord blood, birth cohort, insulin, glucose, lipid
Citation: Huang J-S, Chen Q-Z, Zheng S-Y, Ramakrishnan R, Zeng J-Y, Zhuo C-P, Lai Y-M, Kuang Y-S, Lu J-H, He J-R and Qiu X (2021) Associations of Longitudinal Fetal Growth Patterns With Cardiometabolic Factors at Birth. Front. Endocrinol. 12:771193. doi: 10.3389/fendo.2021.771193
Received: 06 September 2021; Accepted: 16 November 2021;
Published: 09 December 2021.
Edited by:
Tomoko Aoyama, University of Auckland, New ZealandReviewed by:
Satoru Ikenoue, Keio University, JapanCopyright © 2021 Huang, Chen, Zheng, Ramakrishnan, Zeng, Zhuo, Lai, Kuang, Lu, He and Qiu. This is an open-access article distributed under the terms of the Creative Commons Attribution License (CC BY). The use, distribution or reproduction in other forums is permitted, provided the original author(s) and the copyright owner(s) are credited and that the original publication in this journal is cited, in accordance with accepted academic practice. No use, distribution or reproduction is permitted which does not comply with these terms.
*Correspondence: Xiu Qiu, eGl1LnFpdUBiaWdjcy5vcmc=; Jian-Rong He, amlhbnJvbmcuaGVAYmlnY3Mub3Jn
†These authors share first authorship
Disclaimer: All claims expressed in this article are solely those of the authors and do not necessarily represent those of their affiliated organizations, or those of the publisher, the editors and the reviewers. Any product that may be evaluated in this article or claim that may be made by its manufacturer is not guaranteed or endorsed by the publisher.
Research integrity at Frontiers
Learn more about the work of our research integrity team to safeguard the quality of each article we publish.