- Department of Plant Science, North Dakota State University, Fargo, ND, United States
Plant-based foods containing phenolic bioactives have human health protective functions relevant for combating diet and lifestyle-influenced chronic diseases, including type 2 diabetes (T2D). The molecular structural features of dietary phenolic bioactives allow antioxidant functions relevant for countering chronic oxidative stress-induced metabolic breakdown commonly associated with T2D. In addition to antioxidant properties, phenolic bioactives of diverse plant foods have therapeutic functional activities such as improving insulin sensitivity, reducing hepatic glucose output, inhibiting activity of key carbohydrate digestive enzymes, and modulating absorption of glucose in the bloodstream, thereby subsequently improving post-prandial glycemic control. These therapeutic functional properties have direct implications and benefits in the dietary management of T2D. Therefore, plant-based foods that are rich in phenolic bioactives are excellent dietary sources of therapeutic targets to improve overall glycemic control by managing chronic hyperglycemia and chronic oxidative stress, which are major contributing factors to T2D pathogenesis. However, in studies with diverse array of plant-based foods, concentration and composition of phenolic bioactives and their glycemic control relevant bioactivity can vary widely between different plant species, plant parts, and among different varieties/genotypes due to the different environmental and growing conditions, post-harvest storage, and food processing steps. This has allowed advances in innovative strategies to screen and optimize whole and processed plant derived foods and their ingredients based on their phenolic bioactive linked antioxidant and anti-hyperglycemic properties for their effective integration into T2D focused dietary solutions. In this review, different pre-harvest and post-harvest strategies and factors that influence phenolic bioactive-linked antioxidant and anti-hyperglycemic properties in diverse plant derived foods and derivation of extracts with therapeutic potential are highlighted and discussed. Additionally, novel bioprocessing strategies to enhance bioavailability and bioactivity of phenolics in plant-derived foods targeting optimum glycemic control and associated T2D therapeutic benefits are also advanced.
Type 2 Diabetes Etiology: Role of Chronic Oxidative Stress, Hyperglycemia and Gut Health
Growing prevalence of diet and lifestyle-influenced non-communicable chronic diseases (NCDs) is severely affecting communities leading to breakdown in public health solutions across the globe. Since the beginning of 21st century, NCD-associated morbidity and mortality has increased significantly contributing to emerging global public health crisis and health disparities (1). Due to its etiological link to sustained and accumulated unhealthy diets and lifestyle, poor urban communities living on subsidized and hyper-processed foods (calorie dense and nutritionally imbalanced) have become more vulnerable and susceptible to NCDs (2). This public health challenge has been exposed and has worsened with COVID-19 pandemic, as poor urban and suburban communities with underlying NCD related health conditions are being the most susceptible and has affected population globally (3, 4). Among common NCDs, type 2 diabetes (T2D) is one of the major contributors for poor public health outcomes and widening health disparities worldwide irrespective of current crisis with COVID-19 pandemic.
In 2019, there were 463 million people or 9.3% of the world’s population living with T2D and now estimated that 700 million or 10.9% will have the disease by 2045 (5). There are additional populations, who are undiagnosed or at pre-diabetic stage, making this chronic disease epidemic even more severe than what the current statistical estimation reflect. In addition to the higher prevalence and mortality rates associated with T2D, there is also the burden of economic stress due to health expenditure involved in the treatment of T2D and its associated complications. Between 2015 and 2019, the global expenditure on T2D was estimated to be around 673 - 760 billion USD (6, 7). Therefore, the complexity of T2D and its burden towards society across continents and countries is multifaceted, affecting all spheres of human life and wider community well-being. To find solutions towards this public health challenge and for designing well-rounded preventative strategies and interventions, it is important to understand the diet-linked metabolic breakdowns and common risk factors associated with pathogenesis and etiology of T2D.
Type 2 diabetes is largely a diet-linked metabolic disorder that is associated with insulin resistance, β cell dysfunction, abnormal glucose and lipid metabolism, and chronic oxidative stress-induced inflammation (8). Altered carbohydrate absorption, depletion of stored glycogen, increased gluconeogenesis, β cell dysfunction, insulin resistance in peripheral tissues, and altered insulin signaling pathways resulting from poor diet, associated lifestyle influenced metabolic breakdowns coupled with genetic factors, can contribute towards the risks of developing chronic hyperglycemia (8). These risk factors can potentially cause epigenetic changes via histone modification mechanisms such as DNA methylation, which in turn can impair the action and secretion of insulin thereby contributing towards chronic hyperglycemia and progression of T2D (9). A review of numerous studies has indicated that differential DNA methylation and gene expression can occur in muscle, adipose, liver, and pancreatic islets in individuals with T2D, when compared to non-diabetic individuals (9). Histone modification via DNA methylation of the PDX1 gene, which codes for a transcription factor involved in pancreatic development and β cell maturation, can lead to lower expression of this gene thereby contributing towards the pathogenesis of T2D, and gestational diabetes (9, 10).
Like chronic hyperglycemia, chronic oxidative stress resulting from cellular oxygen malfunction can also play a key role in the pathogenesis of T2D and its associated complications. Breakdown in cellular redox homeostasis under hyperglycemic conditions can lead to the generation of excessive reactive oxygen species (ROS) which impedes the normal functioning of cells (pancreatic β cells) and tissues (liver, adipose, and muscle) (11, 12). Therefore, cellular and tissue impairment due to oxidative stress represents an underlying mechanism of glucose toxicity in T2D and its related micro- and macro-vascular complications (13). Significantly higher levels of oxidative stress markers (malondialdehyde and catalase) and inflammatory markers (TNF-α and IL1-α) were observed in blood samples of patients affected with T2D, indicating that oxidative stress and inflammation can be triggered in the pathogenesis of diabetes (14). In recent times, the role of impaired mitochondrial function in the pathogenesis of T2D is gaining increasing relevance through various in vivo and ex vivo metabolic studies that have highlighted mitochondrial dysfunction as a predisposing condition for ectopic lipid deposition and insulin resistance (15). However, further clinical trials are needed to determine if the measurement of oxidative stress markers can be useful in the detection and can be integrated in therapeutic interventions against T2D (16).
Additionally, cellular and circulatory glucose and redox homeostasis critical for preventing and managing T2D are also associated with improved human gut health. Therefore, examining the role of gut microbiota in the pathogenesis T2D also has merit. The human gut microbiota consists of 6 major phyla which are Firmicutes, Bacteroidetes, Actinobacteria, Proteobacteria, Fusobacteria, and Verrucomicrobia, of which Firmicutes and Bacteroidetes are the dominant phyla that account for 90% of the gut microbiome (17). Studies have shown that loss of microbial diversity or dysbiosis can act as a factor in the rapid progression of insulin resistance in T2D (18). Dysbiosis can result in increased gut permeability, which allows for translocation of microbial pathogens and their products (e.g., lipopolysaccharides) into the blood stream circulation leading to metabolic endotoxemia, low grade inflammation, and insulin resistance, thereby contributing towards the pathogenesis of T2D (19, 20). Results from over 42 human studies including preclinical studies or clinical trials have shown that the genera of Bifidobacterium, Bacteroides, Faecalibacterium, Akkermansia and Roseburia were inversely associated with development of T2D through improved metabolism and gut health, while the genera of Ruminococcus, Fusobacterium, and Blautia contributed to T2D (20). However, more clinical, and epidemiological studies are needed to examine the specific role of gut microbiota in regulating carbohydrate metabolism and for improving overall glycemic control.
The current understanding on etiology and pathogenesis of T2D clearly indicates that maintaining cellular and circulatory glucose, redox homeostasis, and improving gut health are critical for countering chronic oxidative stress and hyperglycemia. Healthy diet coupled with regular exercise can help to maintain optimum metabolic regulation and functions, especially to support favorable cellular conditions for oxygen and nutrient coupling during energy (ATP) synthesis (oxidative phosphorylation) in the mitochondria (16). Therefore, the major aim of this review is to examine the role of plant food sources from different ecological origins with high health protective bioactive metabolites, specifically anti-diabetic functional role of phenolics, for countering chronic oxidative stress, chronic hyperglycemia, and for improving human digestive health, which are essential preventative measures to reduce the risks of developing T2D.
Phenolic Bioactives of Plant Foods: Role in Human Metabolism and Glycemic Control
Nutritionally balanced and healthy diet has a major role in the prevention and management of T2D, and several studies suggest that greater consumption of whole grains, legumes, vegetables, and fruits with balanced nutritional profile and health protective bioactive compounds can potentially decrease the risk of T2D (21, 22). The current evidence clearly indicates that type of diet, dietary composition, and diversity of plant foods that are rich in micro-nutrients, dietary fiber, vitamins, and health protective bioactive compounds like dietary antioxidants are potentially beneficial to improve glycemic control and help counter T2D associated complications (23). However, understanding and optimizing different food sources and processing variables is essential to effectively integrate them into dietary and therapeutic interventions targeting T2D prevention and its management. Such T2D benefits targeted optimization can be achieved through identification of key nutritional biomarkers and functional bioactive components of plant foods that have the potential to modulate carbohydrate metabolism which are beneficial in maintaining cellular glucose homeostasis, redox homeostasis, and for improving human gut health. Plant-based foods are good sources of many bioactive compounds including phenolics, carotenoids, vitamins, alkaloids, saponins, and polysaccharides with diverse human health protective bioactivities (24). However, stress inducible phenolic bioactives of plant food sources are gaining wider attention in dietary and therapeutic interventions due to their specific role in countering chronic oxidative stress, hyperglycemia, dyslipidemia and improving digestive health, which are relevant for preventing T2D progression and pathogenesis (25). Therefore, examining the specific glycemic control relevant and health protective functional role of phenolic bioactives of plant foods from different ecological origins and their subsequent optimization based on sound metabolic rationale for dietary and therapeutic applications has significant merit.
Phenolic metabolites are widely distributed across and within all plant families (23). Therefore, improving diversity of plant foods also improves the diversity of these protective phenolic bioactive compounds in our daily diet (26). Such improvements in intake of plant foods enriched in health protective phenolics are relevant in reducing risks of T2D. However, composition and content of these phenolic bioactives vary widely among different plant foods and their bioavailability and functionality changes with different pre and post-harvest conditions (23, 27, 28).
Overall, phenolic bioactives of plant foods can be broadly classified into two major groups, flavonoid, and non-flavonoid compounds (29). When consumed through plant-based diet, these phenolic bioactives are partially absorbed in the stomach and small intestine while rest of the phenolics are utilized by microflora in the large intestine for microbial growth (prebiotic effect) and/or are converted into other phenolic derivatives through microbial action, followed by their absorption in the large intestine (30). It is estimated that 90–95% of consumed phenolics are not absorbed in the small intestine, but accumulate in the large intestinal lumen, where the gut microbiota have the metabolic capacity to convert the phenolic compounds into active metabolites, which in turn are responsible for the human health protective functions (31).
Following absorption in the stomach and small intestine, phenolic compounds and their derivatives are transported to the liver via enterohepatic circulation, where they undergo biotransformation and the resulting metabolites are transported via the bloodstream to other tissues and organs including pancreas, muscle, liver, and adipose tissue and/or are excreted from the body via urine (30). The rate or extent of absorption of phenolics is influenced by several factors such as the chemical structure of the compound as well as its gastrointestinal digestion, absorption, metabolic processing, and degree of conjugation (8, 32). The bioavailability and absorption of phenolics dictate their human health protective functionalities including properties relevant for T2D benefits.
Phenolic bioactives of plant foods have potential to modulate specific anti-hyperglycemic properties in small intestine, liver, muscle, adipose and pancreatic tissue (29). The anti-diabetic relevant anti-hyperglycemic functionalities of phenolics is due to their ability to modulate the activity of enzymes involved in glucose metabolism, as well as their ability to improve β cell function (insulin synthesis and secretion), reduce hepatic glucose output, and alter efficiency of glucose transporters and insulin receptors (8, 29) (Figure 1). Plant foods such as cocoa (Theobroma cacao), coffee (Coffea spp.), fruits, berries, nuts, vegetables, spices, medicinal herbs, tea (Camellia sinensis) and wine, that are a rich source of phenolic bioactives, have shown anti-hyperglycemic activity through different mechanisms of action, especially through the inhibition of the key enzymes like α-amylase, α-glucosidase, and aldose reductase (23, 33). Modification of the chemical structure of phenolic compounds via hydroxylation, methylation, glycosylation, hydrogenation or galloylation, can in turn increase or decrease the associated inhibitory activity against α-amylase and α-glucosidase enzymes (33). Modulation of activity of these key enzymes can help to slow down the breakdowns of complex carbohydrates and absorption of glucose into the bloodstream, which concurrently helps to maintain post-prandial glucose homeostasis leading to T2D benefits.
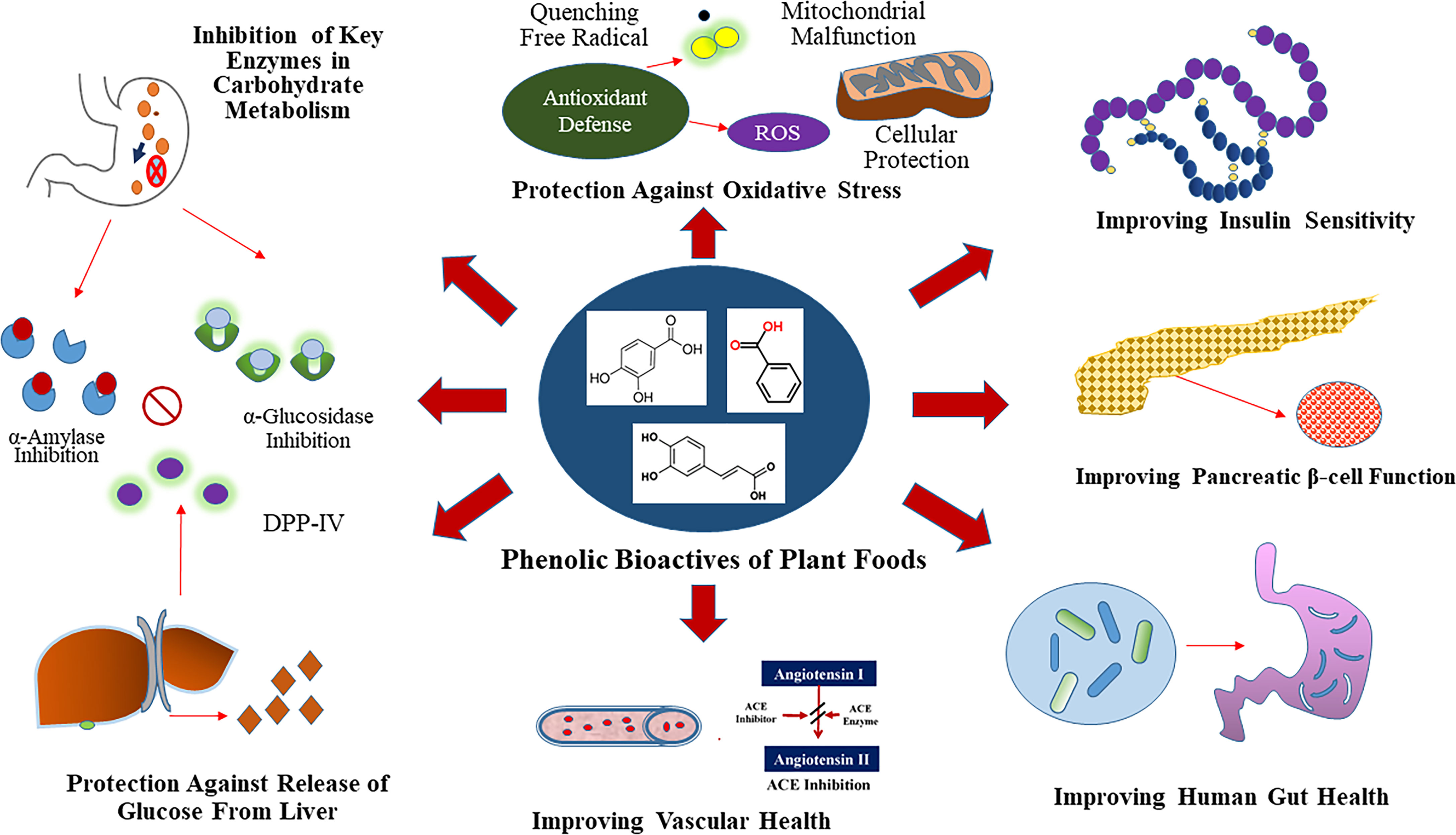
Figure 1 Glycemic Control Benefit Relevant Functional Properties of Plant Phenolics. Phenolics are dietary inhibitors of key enzymes involved in carbohydrate metabolism like α-amylase and α-glucosidase. Additionally, phenolics potentially inhibit dipeptidyl peptidase IV (DPP-IV), which is a dietary and therapeutic target to maintain glucose homeostasis. Select plant foods rich in phenolics also provide protection against chronic hypertension through inhibition of angiotensin-I-converting (ACE) enzyme.
Additionally, phenolic metabolites can improve pancreatic β cell function (insulin synthesis and secretion) via activation of Sirtuin 1(Sirt 1) and glucose transporter 2 (Glut 2) through modulation of insulin dependent pathways that involves modifying cellular redox status and cell signaling activity (29, 32). Phenolics can also improve peripheral insulin sensitivity via activation of phosphoinositide 3-kinases (PI3K)/protein kinase B (AKT), Glut 4, and peroxisome proliferator-activated receptor gamma (PPAR-γ) in muscle, adipose and other tissues (29, 32). Furthermore, through insulin independent pathways, phenolics exhibit anti-hyperglycemic activity via activation of AMP-activated protein kinase (AMPK) in liver, muscle, and adipose tissues (29). The effect of phenolic compounds on these pathways are often correlated with improved β cell function and insulin sensitivity, reduced inflammation and lipotoxicity, as well as reduced hepatic glucose output and glucose absorption, all of which help to maintain normal glucose homeostasis (32). The molecular mechanisms by which phenolic compounds modulate activity against T2D can be broadly classified into four main categories that include transcriptional modulation, translational modulation, modulation of enzyme activity, and epigenetic regulation (34).
Another promising strategy to help lower postprandial blood glucose is through the inhibition of the enzyme dipeptidyl peptidase IV (DPP-IV), which inactivates the incretin hormones glucagon-like peptide-1 (GLP-1) and glucose-dependent insulinotrophic polypeptide (GIP), that are responsible for the stimulation of insulin secretion from pancreatic β cells as well as modulation of glucagon release from pancreatic α cells (35). Anthocyanins isolated from blueberry (Vaccinium spp.) and blackberry (Rubus spp.) wine blends, as well as several pure phenolic compounds like resveratrol, luteolin, apigenin, and flavone showed potent inhibitory activity against DPP-IV (36).
Therefore, phenolic metabolites that are widely distributed in plant foods have diverse anti-diabetic properties, which can be harnessed in functional food design and dietary strategies targeting wider T2D benefits. However, the phenolic profile and their anti-diabetic relevant functional properties vary widely among different plant foods, and plant parts as well as among different pre-harvest growing, post-harvest storage, food processing, and cooking conditions.
Factors Influencing Phenolic Bioactive-Linked Antioxidant and Anti-Hyperglycemic Functionalities of Plant Foods
Consumers across the globe are increasingly making informed choices and integrating whole plant-based and minimally processed foods in their daily diet (37). However, the current food and nutrition model is mostly designed based on macro-nutrient content and whole calorie estimation and neglect the importance of other nutritional and bioactive components of plant foods like health protective phenolic bioactives (28). Therefore, to improve our understanding about nutritional and health protective functional benefits of foods, which will subsequently provide well-rounded nutritional information to consumers, it is necessary to know the phenolic bioactive composition and specific functional benefits of wide arrays of plant foods from different ecological origins. However, due to wide variability in the ecological diversity and distribution of phenolic bioactives in different plant food systems and even within different varieties/genotypes of same species, it can be challenging to design health-targeted foods with optimum phenolic level and composition (28). Additionally, as phenolics are stress inducible secondary metabolites of plants, pre and post-harvest factors like growing condition, cultivation practices, biotic and abiotic stress pressures, storage condition, different food processing and cooking methods significantly influence and alter the composition and overall content of phenolics in plant derived foods and beverages. Therefore, screening and optimization of different factors in the farm to fork food chain that have significant impact on phenolic bioactive linked functional quality of plant foods is essential to target them in health focused food solutions countering diet and lifestyle influenced T2D (Table 1).
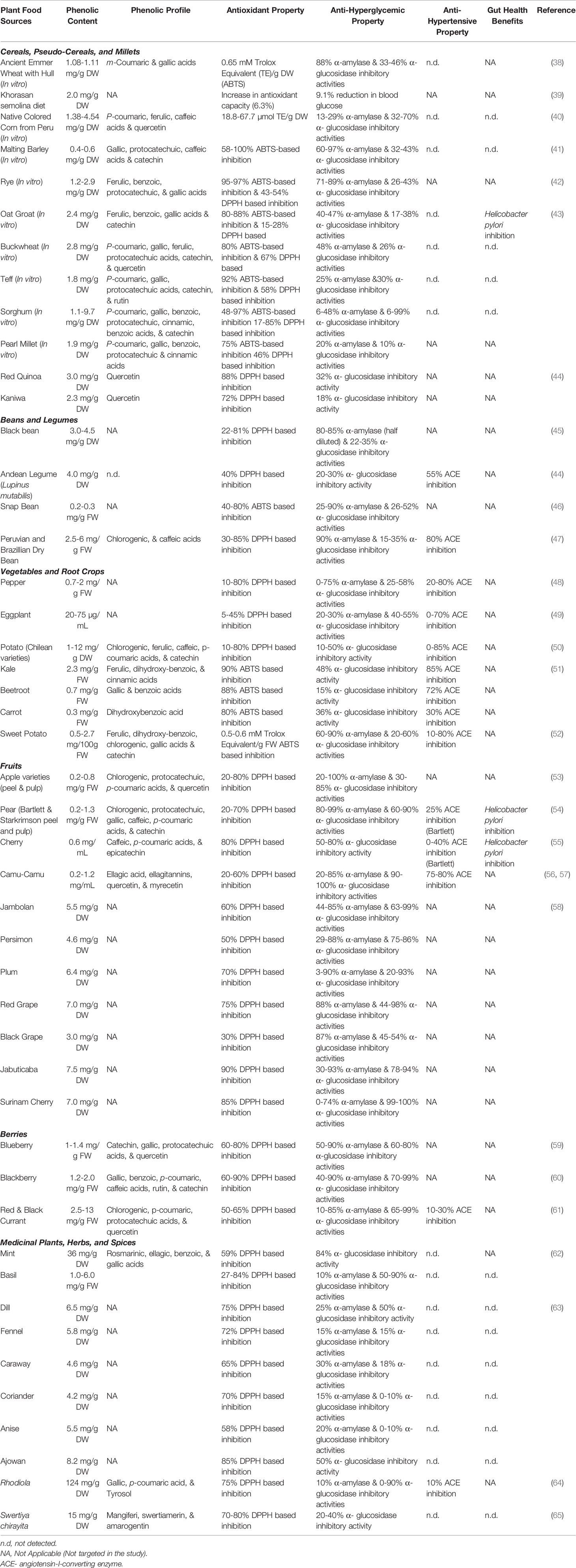
Table 1 Antioxidant, anti-hyperglycemic, anti-hypertensive, and human gut health benefits of select plant foods from different geographic and ecological origins that are rich dietary sources for phenolic bioactives.
Screening of Food Plant Species and Varieties/Genotypes
Cereals, Pseudo-Cereals, and Millets
Cereals, pseudo-cereals, and millets are major sources of carbohydrates in the human diet, and they support the essential calorie needs as well as play a critical role in addressing food and nutritional insecurity solutions, especially to counter chronic hunger and malnutrition globally. However, higher intake of hyper-processed and refined cereals as simple sources of dense soluble macronutrient carbohydrates also increases the risks of developing T2D (66). Therefore, there is a growing interest to integrate whole grain cereals with balanced nutritional profile and higher concentration of fiber and health protective bioactives for optimum dietary benefits and health protection. The current scientific evidence clearly indicates that there is a substantial inverse relationship between higher consumption of whole grains and risks of developing T2D (67). The anti-hyperglycemic benefits of whole grains are associated with low energy intake, prevention of weight gain, and has direct influence on insulin resistance (68). The higher dietary fiber content and phenolic bioactive content of whole grain is also relevant in supporting beneficial gut microbiome as well as providing protection against chronic oxidative stress, inflammation, and hyperglycemia (69).
In vitro studies have shown that ancient accessions of cereal grains, like ancient wheat (emmer- Triticum dicoccon, einkorn-Triticum monococcum and spelt-Triticum spelta) have wider diet-linked health benefits such as capacity to reduce ROS formation, lower blood glucose and cholesterol levels, and reduce circulating vascular endothelial growth factors and interleukins levels, which are relevant for countering T2D (39, 70). In a recent in vitro model based screening study, higher phenolic linked antioxidant and anti-hyperglycemic properties (high α-amylase and moderate α-glucosidase enzyme inhibitory activities) were observed in emmer wheat (Triticum dicoccon) with hull both before and after milling, when compared to modern wheat (Triticum aestivum) varieties (Barlow and Coteau) (38). The emmer wheat with hull had significantly high m-coumaric acid, which was not present in dehulled emmer and modern wheat varieties (38). Like the results of ancient wheat, higher phenolic content and associated antioxidant and anti-hyperglycemic functional qualities were observed in different colored corn (Zea mays) races and accessions, which are indigenous native corn of North and South America (40, 71). Colored corn accessions with high anthocyanin content and high antioxidant activity also exhibited high α-glucosidase and lipase enzyme inhibitory activities (40). In Peruvian purple corn accession (Zea mays subsp. indurata), higher concentration of anthocyanin, hydroxy-cinnamic acids like p-coumaric acid and caffeic acid derivatives were found as free phenolic fractions, while ferulic acid was the predominant bound phenolic fraction in these colored corn accessions (71).
High antioxidant and anti-hyperglycemic properties were also observed in other cereal grains with high phenolic bioactive content like barley (Hordeum vulgare), rye (Secale cereale), and oat (Avena sativa) (41–43, 72). Among different barley (Hordeum vulgare) varieties, high anti-hyperglycemic property relevant α-glucosidase and lipase enzyme inhibitory was found in black barley type, while high phenolic-linked antioxidant activity was observed in malting barley cultivar Pinnacle (41). Overall, barley is a good dietary source of phenolic compounds and other functional constituents like β-glucans (insoluble and soluble fiber) and can potentially play health protective role in mitigating T2D associated risk factors (73). Like barley, rye (Secale cereale) is also rich source of phenolic bioactives and dietary fiber and has exhibited anti-cholesterol and glycemic control relevant properties (42). In a previous in vitro screening study, high total soluble phenolic content, high antioxidant activity, very high α-amylase and moderate to high α-glucosidase enzyme inhibitory activities were observed in rye varieties (42). High ferulic acid, benzoic acid, protocatechuic acid, and gallic acid content were found in the aqueous food grade extracts of most rye varieties (42). In another study, high phenolic-linked antioxidant and α-amylase enzyme inhibitory activity was observed in organic whole grain oat (Avena sativa) (43). Additionally, same whole grain oat extracts also exhibited inhibitory activity against ulcer causing bacteria Helicobacter pylori (43). Therefore, barley, rye, and whole grain oat is addition to being good dietary sources of phenolic antioxidants are relevant for managing chronic oxidative stress and chronic hyperglycemia and also have potential to counter human gut infection, which is pertinent for addressing T2D associated gut infection.
In addition to whole grain oat, higher antioxidant and anti-hyperglycemic properties were observed in buckwheat (Fagopyrum esculentum), teff (Eragrostis tef), and pearl millet (Pennisetum glaucum) (43). The results of phenolic compound characterization revealed that ferulic acid and catechin were major phenolic compounds in whole grain oat and buckwheat, while teff and pearl millet had higher protocatechuic acid and gallic acid (43). Similarly, very high phenolic content and positive correlation with antioxidant and α-glucosidase enzyme inhibitory activity was observed in different sorghum (Sorghum bicolor) genotypes (43). Cinnamic acid was the predominant phenolic bioactive detected in all sorghum genotypes (43). Previously, higher content of quercetin and very high antioxidant activity were found in pseudo-cereals, Quinoa (Chenopodium quinoa) and Kaniwa (Chenopodium pallidicaule) of Peruvian Andean origin (44). Results of these previous screening studies indicated that whole grain cereals, especially ancient cereal grains with cultural and ecological relevance are rich sources of health protective phenolic bioactives and can be rationally integrated in dietary and therapeutic interventions to counter chronic inflammation, hyperglycemia, and gut infection associated with T2D.
Beans and Other Legumes
The current scientific evidence clearly suggests that legumes with balanced profiles of dietary fiber, micronutrients, and phenolic bioactives in a high protein matrix have human metabolism-linked protective function such as favorable glucose metabolism related benefits. Previously, high phenolic bioactive associated antioxidant and anti-hyperglycemic properties were found in black bean (Phaseolus vulgaris) varieties (45). Black beans are also a rich source of micronutrients including zinc, essential amino acids, and dietary fibers and has potential to support healthy immunity and wider functional benefits that are relevant to counter malnutrition, infection as well as different metabolic disorders (74).
In a recent screening study, high phenolic-linked antioxidant, α-amylase, and α-glucosidase enzyme inhibitory activities were observed in different snap bean (Phaseolus vulgaris) genotypes (46). Higher content of quercetin, catechin, and gallic acid were also found in all snap bean genotypes (46). Similarly, Randhir et al. (75) observed high antioxidant, anti-hyperglycemic, and anti-hypertensive property relevant functional qualities in thermally processed select legume sprouts and seedlings (fava bean-Vicia faba, mung bean-Vigna radiata, and soybean-Glycine max). In another study, high antioxidant, and moderate anti-diabetic (α-amylase, and α-glucosidase enzyme inhibition) and low anti-hypertensive (ACE inhibition) property were found in Peruvian and Brazilian bean (Phaseolus vulgaris) varieties (47). All these studies suggest that traditional as well as modern legume varieties with rich phenolic bioactive content in high protein matrix are excellent dietary targets to address chronic oxidative stress, hyperglycemia, and hypertension, which are major risk factors of T2D.
Vegetables and Root Crops
Like legumes, vegetables are also extremely relevant dietary targets to address current diet and lifestyle influenced T2D challenges. Higher intake of vegetables has been associated with a reduced incidence of T2D and can help to reduce other NCD related health risks (76, 77). In addition to phenolic bioactives, vegetables are also rich source of dietary fiber, folate, and several micronutrients, which are relevant for diverse human health protective functional qualities including oxidative stress protection, glycemic control, and supporting human digestive health. A dose response meta-analysis-based cohort study indicated that 2-3 servings/day of vegetables were associated with lower risk of T2D (78). In another study, reduction of T2D by 9% was observed with increase in intake of vegetables up to 300 g/day (79).
One of the major plant families with many popular vegetable crops is Solanaceae, which include pepper (Capsicum spp.), eggplant (Solanum melongena), tomato (Solanum lycopersicum), and potato (Solanum tuberosum). Previously, Kwon et al. (48) screened several types of pepper (Capsicum annuum) (green, red, orange, yellow, Cubanelle, red sweet, yellow sweet, long hot and Jalapeño) and found high α-glucosidase and low α-amylase enzyme inhibitory activities in aqueous extracts. In the same study, ACE inhibitory activity was also observed in select peppers. Potential anti-diabetic benefits of eggplant (Solanum melongena) were also examined using in vitro assay models (49). Eggplant varieties with high phenolic content exhibited very high α-glucosidase and moderate to high ACE enzyme inhibitory activities. Like eggplant, extracts of Chilean potato (Solanum crispum) of diverse colors also showed moderate α-glucosidase and moderate to high ACE inhibitory activity, relevant for managing chronic hyperglycemia and hypertension (50).
In a recent study, similar antioxidant, antidiabetic, and anti-hypertensive benefit relevant functional qualities were observed in vegetables, which were targeted as part of Native American “Three Sisters Crop” community garden concept (51). Very high phenolic bioactive-linked antioxidant, moderate α-glucosidase, and high ACE enzyme inhibitory activity was found in kale (Brassica oleracea var. sabellica) followed by purple potato (Solanum tuberosum), beetroot (Beta vulgaris), pepper (Capsicum annuum), and carrot (Daucus carota) (51). Higher concentration of dihydroxybenzoic acid, ferulic acid, and cinnamic acid were found in kale extracts, which are potentially relevant for its high antioxidant, anti-hyperglycemic and anti-hypertensive functional qualities (51). A recent screening study with different colored (white, orange, and purple) sweet potato (Ipomoea batatas) varieties found high phenolic bioactive linked antioxidant activity in purple fleshed variety, while high anti-hyperglycemic (α-amylase and α-glucosidase enzyme inhibition) and anti-hypertensive properties (ACE inhibition) were found in orange and white-fleshed sweet potato varieties (52). All these screening studies indicated that vegetables with rich source of phenolic bioactives are good dietary targets to counter chronic hyperglycemia and oxidative stress induced metabolic breakdowns as well as potentially help to manage chronic hypertension, which is a common health complication of T2D.
Fruits
Fruits are rich sources of stress protective bioactive compounds and other essential nutrients with diverse health benefits. Therefore, higher consumption of fruits with rich dietary antioxidant profiles is also relevant in countering oxidative stress-induced metabolic breakdowns and related chronic diseases like T2D. In a prospective longitudinal cohort study, Muraki et al. (80) found that higher intake of specific whole fruits, like blueberry (Vaccinium spp.), grapes (Vitis vinifera), and apple (Malus domestica) was positively correlated with reduced risk of T2D, and high dietary fiber and bioactive antioxidant content of these fruits might have contributed towards such anti-diabetic relevant benefits. In a clinical study, reduction of HbA1C, low blood pressure and reduced risk of coronary heart disease were observed with higher consumption of fruits with low glycemic index such as apple (Malus domestica), orange (Citrus × aurantium), pear (Pyrus communis), tangerine (Citrus reticulata), blueberry (Vaccinium spp.), strawberry (Fragaria × ananassa), nectarine (Prunus persica var. nucipersica), plum (Prunus domestica), peach (Prunus persica), grapefruit (Citrus paradisi), raspberry (Rubus idaeus), blackberry (Rubus spp.), and breadfruit (Artocarpus altilis) (81). In a recent meta-analysis of prospective cohort studies revealed that increase in consumption at a rate of one serving of apple and pear per week resulted into 3% reduction of T2D risk (82). Both apple and pear are good sources of dietary fiber and natural antioxidants like flavonoids and phenolic acids and have shown anti-hyperglycemic and anti-hypertensive properties relevant for dietary interventions to counter T2D associated complications (83).
Significant impact of varietal influence on phenolic-linked antioxidant and in vitro anti-hyperglycemic properties were observed in select apple varieties (53). Additionally, higher phenolic content, antioxidant activity, and α-glucosidase enzyme inhibitory activity were found in well preserved apple (Red Delicious, Honey Crisp, Cortland) peel extracts, while pulp extracts exhibited higher α-amylase enzyme inhibitory activity (53, 84). The major phenolic compounds found in apples are protocatechuic acid, chlorogenic acid, p-coumaric acid, and gallic acid. Similarly, carbohydrate enzyme modulating properties (α-glucosidase and α-amylase enzyme inhibitory activities) relevant to counter chronic hyperglycemia were observed in varieties (Mcintosh, Empire, Cortland, and Mutsu) of well-preserved stored apple (85).
High phenolic-linked antioxidant and anti-hyperglycemic functional properties were also observed in pear (54). Additionally, anti-hypertensive property relevant ACE inhibitory activity was observed in pulp extracts of Bartlett pear (54). Another previous in vitro study (86), also reported similar phenolic-linked antioxidant, anti-hyperglycemic (α-glucosidase and α-amylase enzyme inhibitory activities), and anti-hypertensive (ACE inhibition) properties in freshly harvested and long term stored pear varieties (Bartlett, Bosc, Comice, Seckel, Red D’ Anjou, and Green D’ Anjou). Major phenolic compounds found in pear peel were p-coumaric acid, protocatechuic acid, caffeic acid, and gallic acid, while pear pulp was a good source of chlorogenic acid, catechin and gallic acid (54). Another Rosaceae family fruit, cherry (Prunus cerasus) is also rich source of bioactive phenolics including anthocyanin. Inhibition of α-amylase, α-glucosidase, cyclooxygenase-1, lipoxygenase, cyclooxygenase-2, and xanthine oxidase were observed with tart cherry (Montmorency) extracts (87). Above studies indicated that whole fresh and well-preserved fruits like apple, pear, and cherry are a rich source of phenolic antioxidants that have diverse health protective functional qualities such as improving glucose metabolism and overall glycemic control.
In addition to these popular fruits, underutilized and more localized fruits from diverse ecological and geographical origin across the globe have also shown anti-diabetic and cardiovascular health protective properties. In a study with native Peruvian fruits, Pinto et al. (88) found high α-glucosidase enzyme inhibitory activity and a positive correlation with phenolic content and antioxidant activity in food grade aqueous extracts of Lucuma (Pouteria lucuma) and Algarrobo (Prosopis pallida). Additionally, high ACE inhibitory activity was observed in Papayita arequipeňa (Vasconcellea pubescens) and Algarrobo relevant for managing chronic hypertension associated with type 2 diabetes and cardiovascular diseases (88). Very high in vitro α-glucosidase enzyme inhibitory activity and high antioxidant potential were observed in another Amazonian fruit, camu-camu (Myrciaria dubia) (56). In an in vitro screening study, high anti-hyperglycemic (α-amylase and α-glucosidase enzyme inhibitory activities) and high antioxidant properties and their proportional positive correlation with soluble phenolic content were observed in peel, pulp, and seed extracts of select Brazilian fruits (Jabuticaba- Plinia peruviana, Surinam Cherry- Eugenia uniflora, Jambolan plum-Syzygium cumini, Persimmon-Diospyros kaki, plum- Prunus domestica, red grape- Vitis vinifera L., and black grape-Vitis labrusca) (58). All these screening studies indicate that different parts of fruits (peel, seed, and pulp) are rich sources of a wide array of phenolic bioactives with high antioxidant and glucose metabolism modulating potential and can be targeted in dietary and therapeutic support strategies to improve glycemic control, and subsequently reduce the risk of T2D.
Berries
The superior dietary and nutritional benefits of berries are mostly attributed to their unique health promoting phenolic bioactive profile and related high antioxidant activity. Berries are a good source of dietary antioxidants like anthocyanin, flavonoids, and ellagitannins and can be integrated into fresh, frozen, and processed foods in dietary and therapeutic support strategies to counter chronic oxidative stress associated diseases. Beyond their high antioxidant property, berries also have wider human health protective functional qualities including potential for improving glucose metabolism and glycemic control and for providing protection against lipid and protein oxidation (60, 89, 90).
Consistent body of previously published literature based on both in vitro and in vivo studies have revealed high phenolic bioactive-linked antioxidant and anti-hyperglycemic (α-amylase and α-glucosidase enzyme inhibitory activities) functional qualities in berries (59–61, 90–95). Among different berries, strawberry is one of the most popular choices among consumers and demand for this fruit as a fresh and processed food is increasing rapidly. High α-glucosidase, moderate α-amylase, and moderate ACE enzyme inhibitory activities were observed in aqueous and ethanolic extracts of select strawberry (Fragaria × ananassa) varieties (92). In another in vitro study, anti-proliferative, anti-hyperglycemic, and anti-hypertensive properties of purified ellagitannins from strawberries were investigated and compared with ellagic acid and strawberry extracts (94). High anti-proliferative activity was observed with ellagic acid, while purified ellagitannins from strawberry had high α-amylase and ACE inhibitory activities (94).
Like strawberry, glucose metabolism supporting functional benefits relevant for anti-diabetic application were also observed in blueberries (59, 93, 95, 96). In an in vitro model-based screening study to understand the effect of highbush blueberry fruit developmental stages on phenolic-linked anti-hyperglycemic functional qualities, higher soluble phenolic content and high chlorogenic acid content were found in green fruit (before completely ripe), while extracts of fully ripe berries had high antioxidant, high α-glucosidase and moderate α-amylase enzyme inhibitory activities (93). Similarly, significant impact of different varieties/genotypes on phenolic bioactive-linked (based on positive correlation between parameters) antioxidant and glucose metabolism relevant functional benefits (high in vitro α-amylase and α-glucosidase enzyme inhibitory activities) were also observed in blueberries (Vaccinium spp.) (59). The major phenolic compounds found in food grade aqueous extracts of these varieties were catechin, quercetin, protocatechuic acid, and gallic acid (59). High phenolic bioactive-linked antioxidant and anti-hyperglycemic functional properties as well as significant impacts of different varieties/genotypes on these health-targeted qualities were also found in blackberry (Rubus spp.) (60, 97).
Similarly, significant in vitro α-glucosidase and ACE enzyme inhibitory activities were observed in cranberry (Vaccinium microcarpum) powders having high quercetin and p-coumaric acid content (98). In another in vitro screening study, da Silva Pinto et al. (61) found moderate to high α-glucosidase, α-amylase, and ACE enzyme inhibitory activities in red (Ribes rubrum) and black currant (Ribes nigrum) varieties. Recently, Espe (97) observed very high phenolic content, antioxidant, anti-hyperglycemic, and anti-hypertensive properties in several genotypes of serviceberry/juneberry (Amelanchier spp.) grown under cold temperate climate in the Northern Great Plains of the United States. Phenolic enriched berries have also shown other health protective functional qualities such as protection against gut microbiome dysbiosis and dyslipidemia, the common risk factors associated with T2D (99, 100). Due to such high source of dietary antioxidants and associated glucose metabolism supporting functional properties, metabolically screened and optimized berries can be incorporated into food synergies and functional food design for anti-diabetic benefits.
Medicinal Herbs and Spices
Medicinal herbs and spices have long been used in traditional medicine for wider health benefits, including managing post-prandial blood glucose levels, which is essential to counter chronic hyperglycemia and associated metabolic breakdowns of T2D. Among popular and common medicinal herbs, plants from the family Lamiaceae have been found in every region of the world and are widely used in food and therapeutic applications for centuries (62). Medicinal herbs from this plant family are high in biphenolics, like rosmarinic acid and have a wide range of flavonoids and mono-phenolics (62). In a recent in vitro study, high phenolic bioactive linked antioxidant and α-glucosidase enzyme inhibitory activity were found in food grade extracts of select clonal lines of Asian basil (Ocimum spp.) (62).
Another important plant family with health relevant therapeutic properties is Apiaceae, which has many common spices and condiments like coriander (Coriandrum sativum), caraway (Carum carvi), anise (Pimpenella anisum), fennel (Foeniculum vulgare), dill (Anethum graveolens), and ajowan (Trachyspermum ammi). Moderate to high α-glucosidase, moderate α-amylase, and high antioxidant activity were observed in seed extracts of dill, fennel, caraway, coriander, and anise, while ajowan extracts showed high antioxidant and moderate α-glucosidase enzyme inhibitory activity (63). Similarly, crude ethanol extracts of cumin (Cuminum cyminum) seeds with high phenolic and flavonoid content exhibited reduction in glycemic level and inflammation, as well as improved plasma lipid profile in diabetes induced rats (101).
There are many medicinal plants, herbs, and spices from diverse geographical and ecological origins, especially from rich biodiversity regions (Himalayas, Andes, Amazon, Western Ghats of India and Borneo in South East Asia), that have shown wider anti-diabetic and human health protective functional properties. High in vitro α-glucosidase and moderate ACE enzyme inhibitory activities were observed in aqueous and ethanolic extracts of Rhodiola crenulata and Rhodiola rosea, a rich source of tyrosol and other phenolic bioactives (102). Similarly, moderate inhibition of α-glucosidase enzyme and its positive correlation with phenolic content was also observed in ethanolic extracts of Ginkgo biloba leaf (103). Medicinal herbs and spices from Andean region like Chancapiedra (Phyllanthus niruri L.), Zarazaparrilla (Smilax officinalis), Yerba Mate (Ilex paraguariensis St-Hill), and Huacatay (Tagetes minuta) have shown high antioxidant and high α-glucosidase enzyme inhibitory activity in an in vitro model based screening study (104). In another study, flower, and leaf extracts of Loquat (Eriobotrya japonica Lindl.) with high chlorogenic acid and quercetin content exhibited significantly high anti-hyperglycemic activity (α-amylase and α-glucosidase enzyme inhibitory activities) (105). Crude extracts of a medicinal plant Swertia chirayita from Himalayas (Nepal) showed high phenolic-linked antioxidant and moderate α-glucosidase enzyme inhibitory activities (65). Mangiferin, swertiamarin, and amarogentin were major phenolic compounds found in extracts of Swertia chirayita (65). All these screening studies indicate that many medicinal plants, herbs, and spices are rich sources of phenolic bioactives with wider glycemic control benefits (Table 1).
Impact of Cultivation Practices and Growing Environments
Phenolic metabolites of food and medicinal plants are stress-inducible, and their composition, content, and health protective functional qualities vary widely due to differences in growing environment and across different agricultural and horticultural production practices (Figure 2 and Table 2A). Variations of such stress inducible phenolic metabolites impose significant challenges to consistently find plant-based edible materials with uniform consistency and optimum bioactive profile and associated functional qualities relevant for health-focused dietary solutions (28). There is limited understanding and insufficient empirical evidence to a find clear relationship between agricultural production practices and specific health targeted phenolic bioactive-linked nutritional qualities of plant foods. One of the rapidly growing agriculture markets is organic food, which is mostly driven by a wider perception among consumers about their superior nutritional and sensory qualities. However, many previous studies were inconclusive and did not find significant differences in bioactive phenolic content and associated nutritional qualities of plant foods between organic and conventional production systems (143–145).
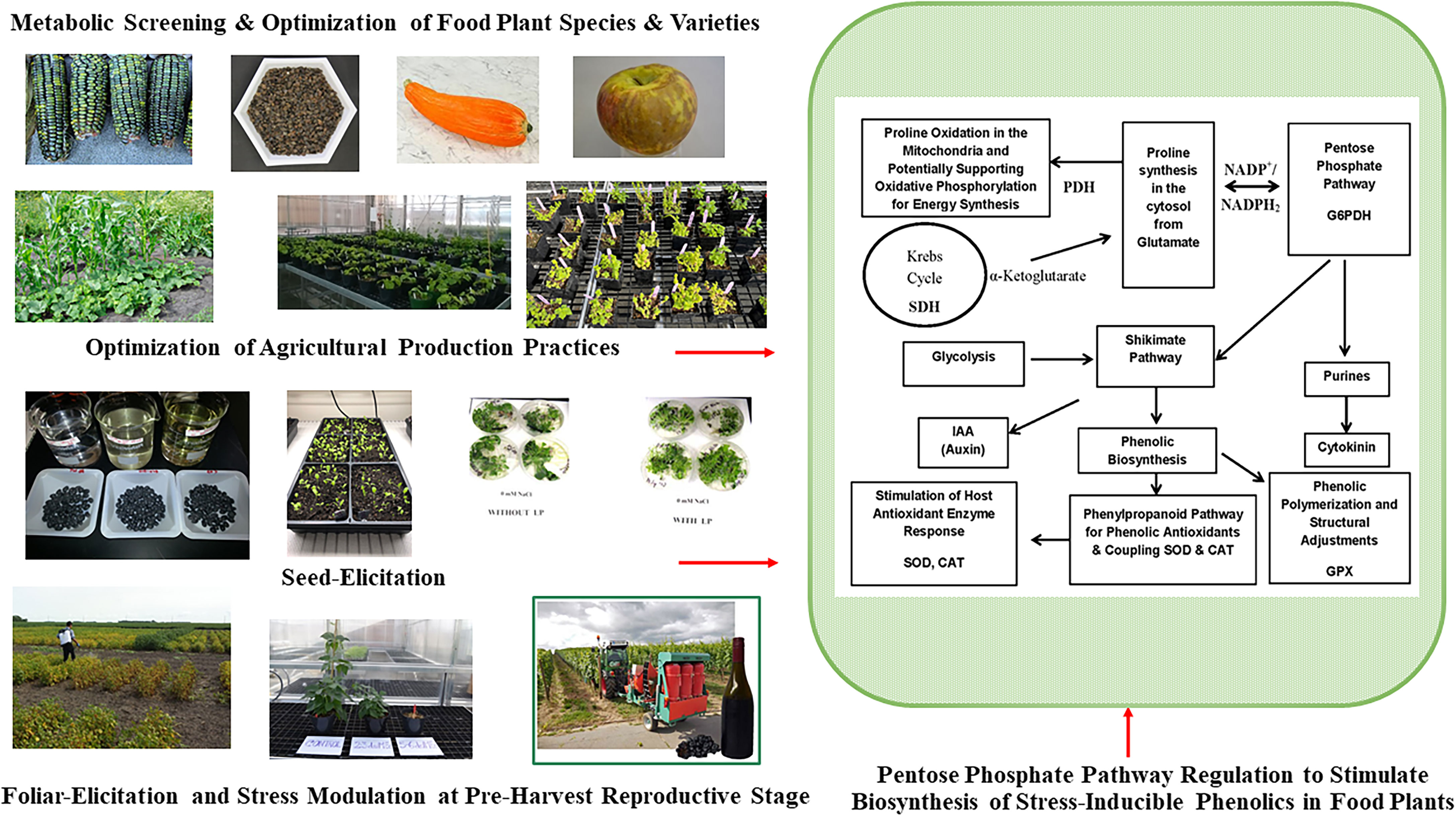
Figure 2 Pre-Harvest Stress Inducible Strategies to Stimulate Biosynthesis of Human Health Protective Phenolic Bioactives in Food Plants. Mild stress induction at critical reproductive stage drive carbon flux towards biosynthesis of stress protective metabolites like phenolics through upregulation of pentose phosphate pathway (PPP), shikimate pathway, and phenylpropanoid pathway. Metabolically driven screening also help to find plant foods with optimum phenolic bioactive profile and associated anti-diabetic functionalities. Glucose-6-phopshate dehydrogenase (G6PDH); proline dehydrogenase (PDH); succinate dehydrogenase (SDH); superoxide dismutase (SOD), catalase (CAT), guaiacol peroxidase (GPX).
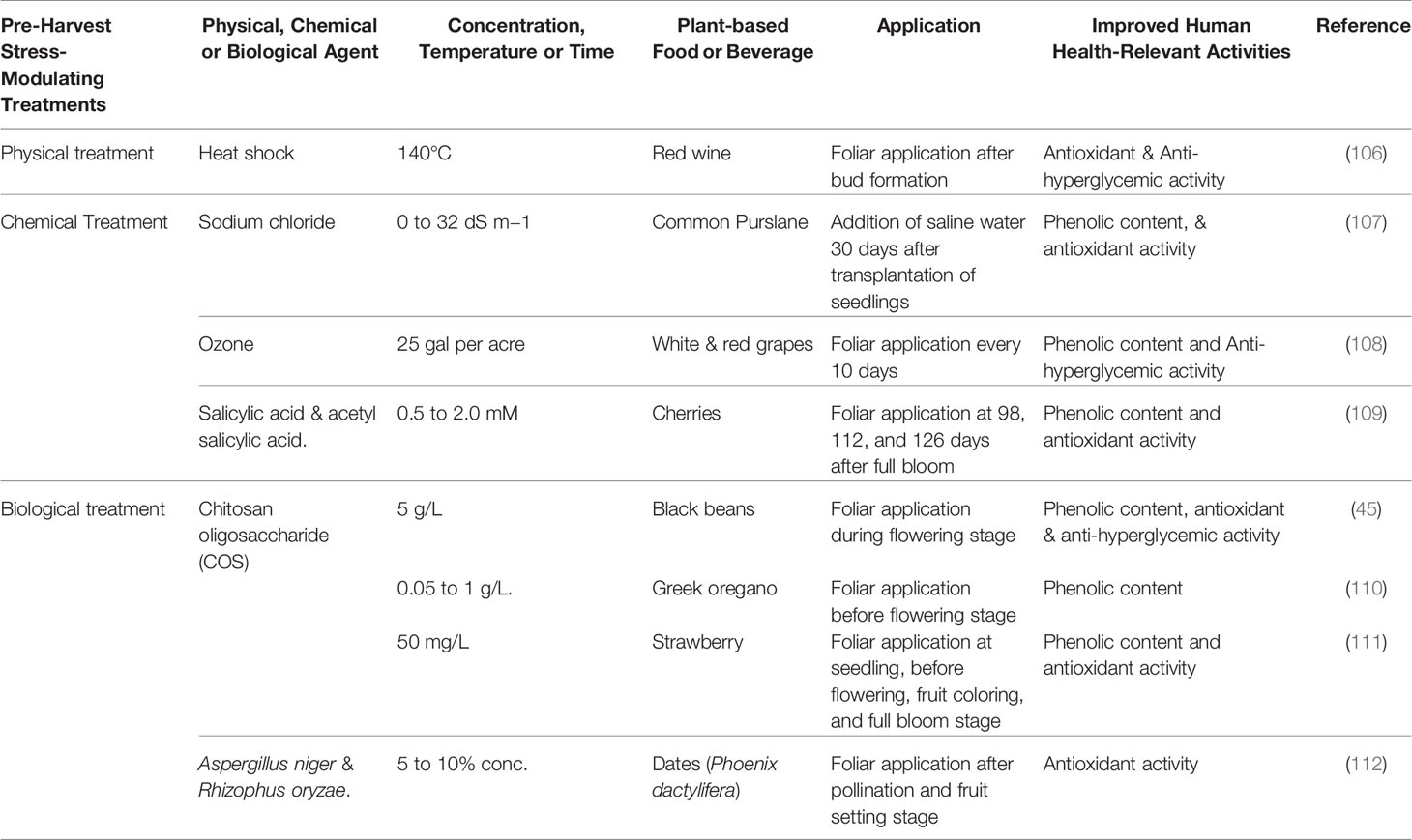
Table 2A Pre-harvest strategies to improve the glycemic control-relevant bioactivity of plant-based foods and beverages.
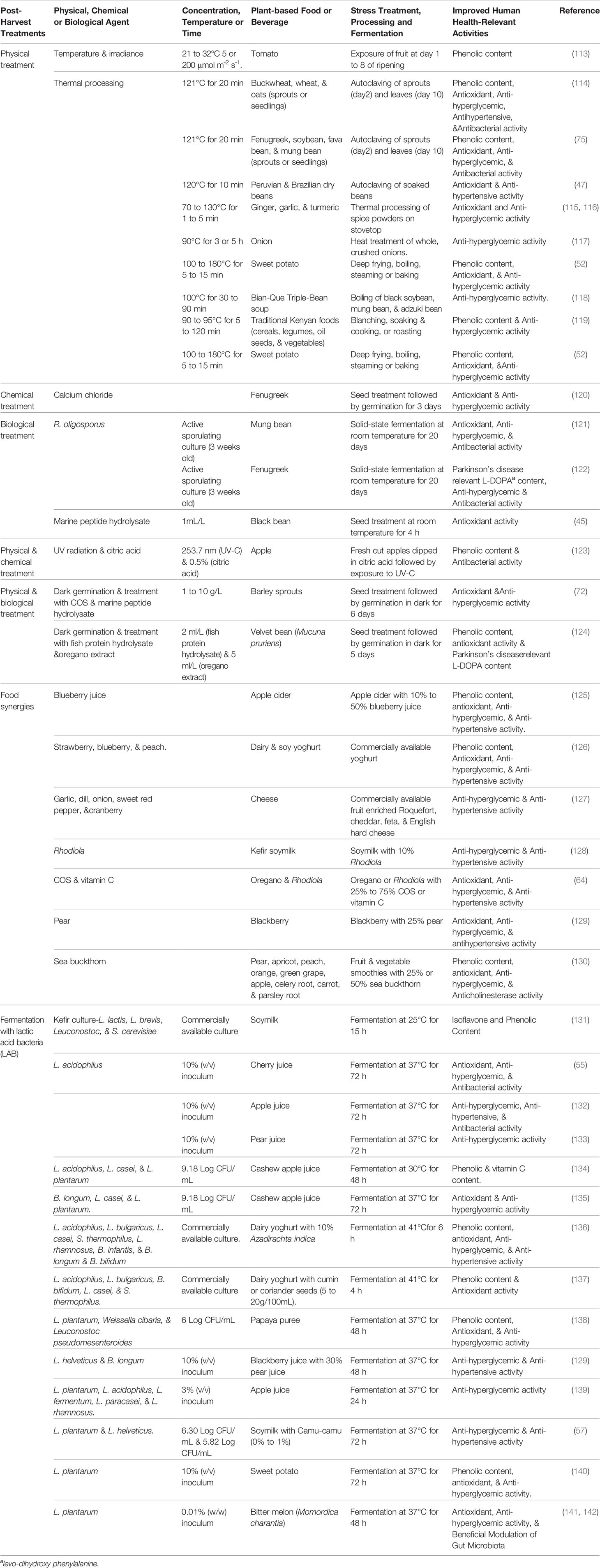
Table 2B Post-harvest strategies to improve the glycemic control-relevant bioactivity of plant-based foods and beverages.
In a study with different rye varieties, high ferulic and benzoic acid content, as well as improved α-amylase enzyme inhibitory activity were observed in organically grown rye, while conventional production practices resulted in higher phenolic-linked antioxidant activity (42). In another study, higher catechin content was found in organic oat groats and rolled oat, when compared to the sample from conventional production practices (43). In a recent single crop-year study, higher phenolic bioactive-linked antioxidant and anti-hyperglycemic (α-amylase and α-glucosidase enzyme inhibitory activities) functional properties were observed in blackberry varieties with organic weed management and fertilization practices (mulching with feather meal) (97).
Oliveira et al. (146) reported high phenolic content, vitamin C, and soluble sugar content in organic tomato and indicated that the improvement was due to abiotic stress during fruit development stage under organic production practices. Therefore, effect of micro and macro environments both in soil rhizosphere and plant phyllosphere, which significantly varies between different crop years and due to different geographic regions might have greater influence on stress inducible and bioactive phenolic content which in turn dictates the health relevant nutritional qualities of plant foods. Significant effect of different crop-year and growing locations on phenolic-linked antioxidant and anti-hyperglycemic properties of rabbit-eye blueberry varieties were previously observed (59). Similarly, different crop years (environmental variations) resulted in significant variations in phenolic antioxidant content and altered anti-hyperglycemic functional qualities in serviceberry genotypes and oats in two different studies (43, 97). Furthermore, significant differences in phenolic linked antioxidant and anti-hyperglycemic functional qualities were observed in Tendergreen snap bean genotype between field and greenhouse growing conditions (46).
Impact of seasonal variations on phenolic-linked antioxidant, anti-diabetic and anti-hypertensive properties were also observed in leaf extracts of different Ginkgo trees (103). In another study, higher antioxidant activity was observed in extracts of Swertia chirayita grown in the wild, while high α-glucosidase enzyme inhibitory activity was found in extracts of cultivated plants (147). Therefore, results of all these studies indicated that growing environment and cultivation practices significantly influence phenolic bioactive content and optimum health protective functional qualities of plant foods. However, such variations also provide a unique opportunity to modulate and stimulate stress-inducible phenolic bioactives and to improve their functional benefits. Novel pre-harvest strategies targeting critical metabolic pathway regulation involved in the biosynthesis of stress-inducible phenolic bioactives can be advanced to enhance health-targeted functional qualities including glycemic control benefits in plant foods.
Pre-Harvest Bio-Elicitation Strategy
An important metabolically modulating pre-harvest strategy is bio-elicitation with natural stress mimicking compounds to divert carbon flux towards the protective endogenous defense pathways in plants and concurrently stimulating stress inducible production of phenolic metabolites. Such stimulation of phenolic metabolite biosynthesis at critical reproductive and maturity stages can be harnessed to improve overall bioactive and nutritional qualities including chronic disease preventive functional properties in plant foods. In such stress response modulation harnessing strategies, non-toxic and water-soluble elicitors can be targeted as exogenous application or the plant can be exposed to controlled (in moderation) wounding, heat and cold shock, irradiation, UV or ozone treatments to improve stress inducible phenolic bioactive contents in plants (28).
In a field-based study, improvement of phenolic content, antioxidant activity, and anti-hyperglycemic property relevant in vitro α-amylase enzyme inhibitory activity were observed in select black bean genotypes with foliar application of water-soluble chitosan oligosaccharide during flowering stage (45). Foliar application of chitosan oligosaccharide 2 weeks before flowering also resulted into improvement in phenolic and flavonoid content in Greek oregano (Origanum vulgare) (110). Similarly, improvement in fruit quality and enhanced phenolic-linked antioxidant activity were observed in strawberry with pre-harvest application of water-soluble chitosan (111).
Application of short-term mild abiotic stress have also shown improvement of phenolic content and associated human health relevant functional qualities in food plants, especially in high-value specialty fruits. Manduri et al. (106) reported improvement in phenolic-linked antioxidant and anti-hyperglycemic properties in red wine derived from instantaneous heat-shock treated grapes (Pinot Noir). Similarly, enhanced phenolic content and higher anti-hyperglycemic properties (in vitro α-amylase and α-glucosidase enzyme inhibitory activities) were observed in white and red wine grape varieties following foliar application of ozone during fruit maturity stage (108). Though not many studies have investigated mild stress-induced improvement in human health relevant glycemic control protective functional properties of plant foods, stimulation of phenolic biosynthesis pathways and enhanced phenolic antioxidant content were observed with natural elicitor, ozone, heat and cold shock, salt stress, wounding, and UV-radiation treatments (107, 109, 112, 123) (Table 2A and Figure 2). Therefore, it is important to have field-based, multi-location, and multi-year studies in the future to better understand the efficacy of stress-modulating pre-harvest strategies for enhancing phenolic linked human health protective functional qualities especially targeting and harnessing wider T2D benefits.
Post-Harvest Strategies to Improve Phenolic Bioactive-Linked Antioxidant and Anti-Diabetic Functionalities of Plant-Derived Foods and Beverages
Globally, there is a growing interest in plant-based functional foods, food ingredients, and nutraceuticals targeting wider human health benefits and specifically to counter NCD-linked public health disparities. With modern scientific innovation and improved understanding on plant metabolites and their mobilization and bioavailability in plant food matrix, many strategies have evolved to enhance bioactive profiles and associated human health promoting functional qualities in foods and beverages derived from different plant sources (23, 148) (Figure 3 and Table 2B). With increasing consumer awareness about health benefits of plant bioactives and dietary antioxidants, food industries are also investing in novel technologies to improve antioxidant and other bioactive-linked functional properties in plant-based processed foods. In this review, novel post-harvest approaches, which are safe, inexpensive, simple, and potentially effective strategies to improve phenolic linked anti-diabetic, anti-hypertensive, and human gut health benefits in plant foods and beverages are highlighted (Table 2B).
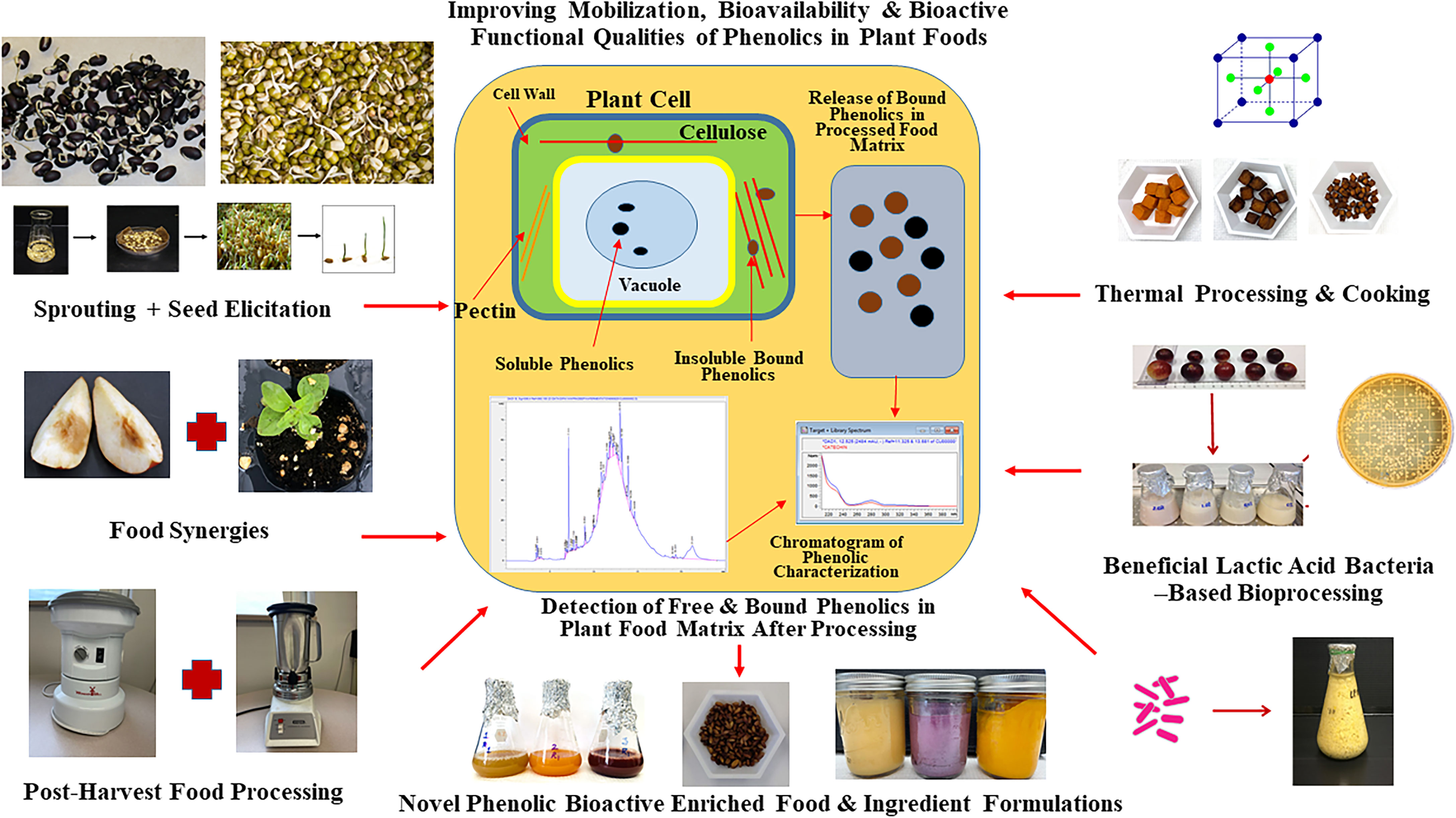
Figure 3 Post-harvest Bioprocessing Strategies to Enhance Mobilization, Bioavailability, and Bioactive Functionalities of Phenolics in Plant Foods. Sprouting, bio-elicitation, beneficial lactic acid bacteria-based fermentation, and different food processing and cooking strategies can be optimized and targeted to improve phenolic bioactive-linked antioxidant, anti-hyperglycemic and digestive health relevant benefits in plant foods from different geographical and ecological origins.
Sprouting, Bioconversion and Biological Elicitation of Seeds
Sprouting is a traditional and inexpensive processing method that helps to biologically activate seeds upon the imbibition of moisture and trigger subsequent physiological and biochemical modification of seed biochemical components. During sprouting, enzymatic hydrolysis converts complex nutrient reserve of seeds into simple sugars and amino acids and such mobilization and solubilization of macronutrients also influence synthesis, mobilization, and bioavailability of free and bound bioactive compounds like phenolics. When integrated with novel seed elicitation approach, the bioavailability, and functional qualities of bioactives in sprouted seeds can be further enhanced. Seed elicitation with natural elicitors in combination with biological activation during sprouting can upregulate plant defense response pathways like anabolic pentose phosphate, shikimic acid, and phenylpropanoid pathways, which in turn improves biosynthesis of stress inducible phenolic metabolites and their bioactivity (Figure 2) (23, 27, 28).
In a recent study, improvement of in vitro α-glucosidase enzyme inhibitory activity and enhanced gallic acid content were observed in dark germinated (6 day) malting barley sprouts (72). Furthermore, improvement in phenolic-linked antioxidant and type 2 diabetes relevant anti-hyperglycemic properties were also found in dark germinated barley sprouts following seed elicitation with natural bioprocessed elicitors (chitosan oligosaccharide and fish protein hydrolysate) (72). Such an improvement in phenolic-linked health protective functional qualities in bio-elicited and sprouted barley was through upregulation of defense response pathways such as anabolic pentose phosphate pathway (73). Similar trend in improvement of phenolic –linked antioxidant functionalities through upregulation of anabolic pentose phosphate pathway was observed in bio-elicited (chitosan oligosaccharide and fish protein hydrolysate) and dark germinated black bean sprouts (45).
Previously, bioconversion of mung bean with Rhizopus oligosporus exhibited higher phenolic –linked antioxidant, α-amylase enzyme inhibition and anti-bacterial activity against ulcer causing bacteria Helicobacter pylori (121). Similar improvement in α-amylase enzyme inhibitory activity and anti-bacterial activity against H. pylori was found in Rhizopus oligosporus bioprocessed fenugreek (Trigonella foenum-graecum) extracts (122). Enhanced antioxidant and anti-diabetic functional qualities (in vitro α-amylase and α-glucosidase enzyme inhibition) was observed in sprouted (3 day) and bio-elicited (seed treatment with calcium chloride) fenugreek (120). In another study, improvement of phenolic-linked antioxidant activity was observed in dark germinated and bio-elicited Mucuna pruriens sprouts, while optimum anti-hyperglycemic relevant α-amylase and α-glucosidase enzyme inhibitory activity was found in cotyledon prior to the sprouting and bio-elicitation (124).
In a recent rat model-based study, improvement of glycemic control through reduced blood glucose level and hepato-protective properties were observed with treatment of methanolic extracts of broccoli (Brassica cretica) sprouts (149). Improved anti-hyperglycemic and anti-hyperlipidemic properties relevant for anti-diabetic benefits were also observed in rat model with sprouted quinoa-based yogurt (150). All these studies indicated that sprouting alone or in combination with bio-elicitation and food grade bioconversion are novel and effective approaches to enhance phenolic bioactive-linked antioxidant, anti-diabetic, and lipid metabolism benefits of plant foods. Such strategies can be targeted towards design functional food ingredients and nutraceuticals for wider T2D benefits.
Thermal Processing and Cooking Optimization
Like sprouting, health-targeted optimization of cooking methods and food processing strategies can provide inexpensive and simple tools to the consumers for improving overall nutritional qualities of diverse foods. Such an optimization strategy can specifically target improved bioavailability and stability of bioactive compounds and their health protective functional qualities like glycemic control relevant benefits in cooked and processed foods. One of the most common and simple method is to use thermal treatments for mobilizing bioactive compounds and improving their functional properties in nutritionally relevant food matrix.
Previously, improvement of phenolic content, antioxidant activity, α-amylase, α-glucosidase, and ACE enzyme inhibitory activities were observed in thermal processed (autoclaved for 20 min) buckwheat and oat sprouts (2 day dark germinated) and seedlings (10 day leaves light germinated) (114). Additionally, anti-bacterial activity against H. pylori was also found in thermal processed sprouts and seedling extracts (114). Similar improvement in anti-diabetic functional qualities and anti-bacterial activity against H. pylori were observed in thermal processed fava bean, mung bean, soybean, and fenugreek sprouts (dark germinated) and micro seedlings (light germinated) (75). In another study, improved antioxidant and ACE inhibitory activity were found in thermal processed Peruvian and Brazilian bean varieties (47). The improvement in human health protective antioxidant, anti-hyperglycemic, anti-hypertensive, and anti-microbial functional qualities in sprouts and beans following thermal processing might be due to oxidation and polymerization of phenolic bioactives and subsequent modification in their structure-function relationship. Previously, thermally treated (toasted in conventional stove-top) spices (ginger-Zingiber officinale, garlic-Allium sativum, and turmeric-Curcuma longa) showed high phenolic linked antioxidant and enhanced α-glucosidase enzyme inhibitory activity (116). In another study, Hester et al. (115) found induced antioxidant activity in Wistar male rat following feeding with thermally treated garlic, ginger, and turmeric. Thermally treated onion (Allium cepa) extracts also showed improvement in anti-hyperglycemic functional qualities by suppressing carbohydrate absorption via inhibition of intestinal sucrose (117).
In addition to thermal processing, it is also important to optimize cooking conditions and related variables based on the bioactive stability and associated health targeted functional qualities of cooked foods. Common cooking methods like boiling, steaming, baking, frying, and microwave cooking can help to release embedded bioactive compounds in plant food matrix and potentially improve health-protective functional qualities such as antioxidant, anti-hyperglycemic, anti-hypertensive, and gut health benefits. However, without proper optimization cooking can also lead to loss of essential functional and nutritional qualities of food through significant physical and chemical changes. In a recent study with orange–fleshed sweet potato, Chintha (52) found higher retention of phenolic bioactives and improvement of associated antioxidant and anti-hyperglycemic functional qualities under optimized (cooking time, size of sample, and cooking temperature) cooking conditions. Higher antioxidant and anti-diabetic property relevant functional qualities were observed in oven baked and steamed sweet potato, when compared to boiled and deep-fried samples (52). Previously, Song et al. (118) optimized boiling time (30 min) of Bian-Que Triple Bean soup based on its α-glycosidase inhibitory activity and antioxidant property. Similarly, minimal processing (soaking + cooking and roasting) resulted into higher retention of phenolic compounds and associated antioxidant, and anti-hyperglycemic (in vitro α-amylase and α-glucosidase enzyme inhibitory activity) functional qualities in traditional Kenyan foods (finger millet-Eleusine coracana, amaranth-Amaranthus spp., pigeonpea- Cajanus cajan, field bean- Vicia faba, groundnut-Arachis hypogea, pumpkin-Cucurbita pepo, and sunflower seeds-Helianthus annuus) (119). Therefore, these studies indicated that optimizing cooking and processing conditions is essential to retain and improve bioactive-linked health functional qualities of diverse plant food matrix. Such health-targeted cooking and food processing optimization can provide critical insights into optimum bioactive and nutritional qualities supporting human health benefits.
Health-Targeted Food Synergies
Addition of food ingredients that are rich source of bioactives to improve overall food and nutritional qualities is part of traditional food practices across the globe. In recent times, advanced knowledge on bioactive and nutritional benefits of specific food ingredients and improvement in food processing technologies have further expanded the use of combining different food matrices and ingredients to design novel foods with improved food quality and keeping quality. Food industries are also advancing strategies to find compatible food synergies for wider nutritional and bioactive targeted health benefits. Previous studies have advanced novel food synergy strategy by incorporating bioactive enriched edible plants to improve phenolic bioactive-linked anti-diabetic functional qualities of different plant and dairy-based foods (125, 129).
In a study targeting cranberry-based herbal synergy, high in vitro α-glucosidase and α-amylase enzyme inhibitory activities were observed in cranberry: Rhodiola rosea (75:25) combination, while cranberry: rosemary (Salvia rosmarinus) (75:25) combination had high anti-hypertensive property relevant ACE inhibitory activity (151). In another study, improvement in phenolic bioactive mobilization and associated anti-hyperglycemic and anti-hypertensive functional qualities were observed when Kefir-culture mediated fermentation of soymilk was supplemented with Rhodiola (2%) extracts (128). Improved anti-hyperglycemic functional qualities of Kefir-culture mediated soymilk was positively correlated with tyrosol and salidroside content, two major health protective phenolic bioactive compounds of Rhodiola (128). Like fermented and Rhodiola supplemented soymilk, cranberry enrichment of cheese (Cheddar, feta, and Roquefort) also showed improvement in α-amylase and α-glucosidase enzyme inhibitory activity relevant for anti-hyperglycemic benefits (127). High anti-hyperglycemic and anti-hypertensive property were found in yogurt enriched with different plant extracts like neem (Azadirachta indica) (136), coriander, and cumin seeds (137). Integration of chitosan oligosaccharide resulted in enhanced antioxidant activity in medicinal herb extracts (oregano and Rhodiola), while vitamin C and herb combinations exhibited improved α-glucosidase and ACE inhibitory activity (64). Many recent reviews have highlighted the benefits of integrating bioactive enriched medicinal plants and herbs for improving anti-diabetic and anti-hypertensive relevant functional properties of foods and for therapeutic applications (152, 153).
Berries that are a rich source of dietary and water-soluble antioxidants are also excellent targets for novel food synergies, specifically to improve anti-inflammatory, anti-hyperglycemic, and anti-dyslipidemic properties of foods and beverages. Addition of blueberry juice (20%) in apple cider (80%) showed improvement in phenolic-linked antioxidant and in vitro α-glucosidase enzyme inhibitory activity (125). Similarly, higher antioxidant, anti-hyperglycemic, and anti-hypertensive properties were observed in 30:70 blackberry: pear combination (129, 154). In a recent study, enrichment with sea buckthorn berry (Elaeagnus rhamnoides L.) resulted in higher anti-cholinesterase, anti-hyperglycemic, and antioxidant properties in fruit (75%) and vegetable (25%) combined smoothies (130). Such integration of compatible fruit extracts with complementary bioactive profile is an effective approach to enhance human health protective functional qualities of minimally processed fruit-based foods and beverages. In addition to compatible fruits, phenolic enriched dried berry extracts can also be added to other plant-based food matrices to improve wider health benefits. Significant reduction of glucose (24-74%) production was observed in rat hepatocytes, when blueberry phenolic enriched defatted soybean flour was incorporated in very high fat diet formulations (155). Similarly, addition of Saskatoon (serviceberry-Amelanchier spp.) berry powder (5%) in regular mouse diet resulted into reduction of blood and urine glucose level in diabetic mouse model (156). A recent meta-analysis-based study indicated that consumption of phenolic enriched foods alone or in combination with anti-diabetic drugs has significant potential to lower blood glucose levels in individuals with T2D or at pre-diabetic state (157). Therefore, health targeted food synergy by combining phenolic enriched food matrices is an effective dietary and therapeutic approach to counter breakdown of glucose metabolism and associated health risks for prevention and management of type 2 diabetes.
Bioprocessing Using Lactic Acid Bacteria (LAB)-Based Fermentation
Fermentation of plant and dairy-based food substrates with beneficial and edible microorganisms is a widely popular traditional food practice, which can be found in all cultures and geographic regions across the globe. There is a renewed interest in fermented foods due to the growing perception about its wider health benefits, specifically with more informed understanding about the role of improved gut health in reducing risks of chronic diseases (NCDs) (158). In the context of improved food quality through benefits of fermentation helps to enhance mobilization and bioavailability of bioactive metabolites in fermented end-products and thus increase overall nutritional and health protective functional properties. Improved isoflavone and phenolic acid content was reported in Kefir-fermented soymilk (131). Advancement in food technologies has also supported optimization of more controlled fermentation both for small domestic and pilot scale (food industries) to improve specific health targeted benefits like redox and glycemic control protective properties in fermented foods and beverages. Among beneficial microorganisms, probiotic relevant lactic acid bacteria (LAB) based fermentation is most widely used bioprocessing tool to improve nutritional and keeping quality of dairy and plant-based foods (148).
Many previous studies have found improvement in glucose metabolism, anti-hypertensive, antioxidant, anti-dyslipidemia, and vascular health protective functional properties in dairy and plant-based food substrates following controlled fermentation with LAB (55, 132–134, 159, 160). Cohort study based meta-analysis also revealed reduced risk of T2D with higher intake of yogurt, the most popular and traditional LAB fermented food (161, 162). In addition to improvement in mobilization of phenolics that influence health relevant functional qualities of fermented dairy foods, release of other bioactive compounds like peptides in fermented end products also enhance glycemic control and anti-hypertensive property relevant functional benefits (163, 164).
Like the results of dairy-based food substrates, LAB-based fermentation is also an effective dietary strategy to improve health protective bioactive functional qualities such as anti-hyperglycemic and anti-hypertensive properties of diverse plant-based food substrates (148). However, the improvement of bioavailability and bio-accessibility of bioactive compounds of fermented plant food largely depends on type and source of plant substrate, type of LAB strains, and total fermentation time, and methods of bioprocessing. A study with in vitro digestion of papaya (Carica papaya) puree revealed higher recovery of individual phenolic compounds like chlorogenic, vanillic, syringic, ellagic, ferulic acids, catechin, epicatechin, and quercetin following fermentation with Lactoplantibacillus plantarum (L75*D7) and Weiissella cibaria64 (W64*D7) strains (138). Improvement in antioxidant and anti-hyperglycemic functional qualities were observed with LAB-based fermentation of aqueous extracts of cherry (Prunus avium), pear, and apple (55, 132, 133). Similarly, improved anti-hyperglycemic and anti-hypertensive property were observed in pear: blackberry (70:30) fruit synergy following fermentation with beneficial LAB (L. helveticus, and Bifidobacterium longum) (129). Significant improvement in α-glucosidase enzyme inhibitory activity was found with LAB fermentation of Aksu apple juice (139).
In addition to common fruits and berries, LAB fermentation is particularly relevant in improving bioactive functional qualities and to remove anti-nutrients of underutilized fruits and plant food byproducts. Enhanced in vitro α-glucosidase, α-amylase, and moderate ACE enzyme inhibitory activities were observed in camu-camu and soymilk combination after fermenting with L. plantarum and L. helveticus (57). Similarly, improvement in antioxidant and anti-hyperglycemic functional properties were also observed in LAB fermented cashew (Anacardium occidentale) apple juice (135). Improvement in phenolic bioactive-linked antioxidant, and anti-hyperglycemic functional qualities were also observed in different flesh-colored sweet potato following L. plantarum based fermentation (140). In recent rat model-based studies, Momordica charantia extracts fermented with L. plantarum showed improvement in lipid metabolism, glycemic control, and beneficial alteration in gut microbiota composition relevant for wider T2D benefits (141, 142). Therefore, LAB based fermentation can be rationally recruited as a novel bioprocessing tool to improve bioactive functional qualities like anti-diabetic, anti-dyslipidemia, and cardiovascular health protective properties in dairy and plant-based food substrates. Such fermentation based bioprocessing strategy are also relevant to develop functional food ingredients and nutraceuticals targeting T2D benefits. Additionally, due to wider use of fermentation in traditional food practices, this bioprocessing approach is also pertinent in developing culturally relevant foods with bioactive enriched health benefits.
Conclusion
Narrow dietary choices high in macronutrients with higher intake of refined and hyper-processed soluble carbohydrate rich foods, unhealthy lifestyles like physical inactivity, and genetic pre-disposition are major contributing factors in increasing prevalence of T2D and related mortality worldwide. To counter and reduce the risks of common T2D and its co-morbidity, it is important to improve our daily diet by widening plant food diversity with balanced and superior bioactive and wider nutritional quality rich profiles. Dietary preventive strategies to counter T2D at early stages of disease development is specifically important for addressing growing health care costs and economic burden. Among health protective and health promoting bioactives, stress-inducible phenolic bioactives of plant foods are drawing wider attention in advancing healthy dietary strategies based on their diverse functional properties like antioxidant, anti-hyperglycemic, anti-hypertensive, anti-dyslipidemic, and gut health benefits. However, optimization of wide variability and diversity in content and composition of phenolic bioactives among plant food sources is essential for delivery consistency and therefore is a major challenge for effective integration in dietary and therapeutic strategies to deliver optimum functional health benefits. Therefore, it is necessary to screen and optimize phenolic bioactive content, composition, and functional qualities of plant food sources across farm to fork food chain to incorporate them in health targeted food applications. Advancements in metabolically relevant screening strategies and growing empirical evidence on specific health protective role of phenolic bioactives of plant foods against chronic diseases like T2D have widened the scope of finding suitable plant food and food ingredients with optimum bioactive profile and health relevant functional qualities for delivery. Additionally, novel pre-harvest and post-harvest strategies based on improved understanding of biosynthesis, mobilization, bioavailability, and bioaccessibility of stress inducible phenolics have enabled to further enhance bioactive content and health promoting nutritional qualities in wide arrays of plant foods. Studies highlighted in this review clearly indicate that phenolic bioactive enriched plant foods are safe and inexpensive dietary targets to counter common health risks associated with T2D. However, extensive clinical and epidemiological studies are required to improve our understanding on glycemic control protective mechanisms of plant phenolics relevant for wider dietary and therapeutic solutions against T2D. Achieving health targeted dietary benefits with bioactive enriched plant food diversity in diverse global ecologies are extremely important to address wider T2D-linked public health disparities, and specifically to improve nutrition, health, and well-being of marginalized and less developed health care communities globally.
Author Contributions
DS and KS: Conceptualization. DS and AC: Review literature collection. DS, AC, and KS: writing—original draft. DS and AC: Creation of figures and tables. KS and DS: Review and Editing. All authors contributed to the article and approved the submitted version.
Conflict of Interest
The authors declare that the research was conducted in the absence of any commercial or financial relationships that could be construed as a potential conflict of interest.
Publisher’s Note
All claims expressed in this article are solely those of the authors and do not necessarily represent those of their affiliated organizations, or those of the publisher, the editors and the reviewers. Any product that may be evaluated in this article, or claim that may be made by its manufacturer, is not guaranteed or endorsed by the publisher.
References
1. Allen L. Are We Facing a Noncommunicable Disease Pandemic? J Epidemiol Global Health (2017) 7(1):5–9. doi: 10.1016/j.jegh.2016.11.001
2. Khandelwal S, Kurpad A, Narayan KM. Global non-Communicable Diseases—the Nutrition Conundrum. Front Public Health (2018) 6:9. doi: 10.3389/fpubh.2018.00009
3. Kluge HHP, Wickramasinghe K, Rippin HL, Mendes R, Peters DH, Kontsevaya A, et al. Prevention and Control of non-Communicable Diseases in the COVID-19 Response. Lancet (2020) 395(10238):1678–80. doi: 10.1016/S0140-6736(20)31067-9
4. Karmakar M, Lantz PM, Tipirneni R. Association of Social and Demographic Factors With COVID-19 Incidence and Death Rates in the US. JAMA Network Open (2021) 4(1):e2036462–e2036462. doi: 10.1001/jamanetworkopen.2020.36462
5. Pinchevsky Y, Butkow N, Raal FJ, Chirwa T, Rothberg A. Demographic and Clinical Factors Associated With Development of Type 2 Diabetes: A Review of the Literature. Int J Gen Med (2020) 13:121. doi: 10.2147/2FIJGM.S226010
6. Ogurtsova K, da Rocha Fernandes JD, Huang Y, Linnenkamp U, Guariguata L, Cho NH, et al. IDF Diabetes Atlas: Global Estimates for the Prevalence of Diabetes for 2015 and 2040. Diabetes Res Clin Pract (2017) 128:40–50. doi: 10.1016/j.diabres.2017.03.024
7. Williams R, Karuranga S, Malanda B, Saeedi P, Basit A, Besançon S, et al. Global and Regional Estimates and Projections of Diabetes-Related Health Expenditure: Results From the International Diabetes Federation Diabetes Atlas. Diabetes Res Clin Pract (2020) 162:108072. doi: 10.1016/j.diabres.2020.108072
8. Bahadoran Z, Mirmiran P, Azizi F. Dietary Polyphenols as Potential Nutraceuticals in Management of Diabetes: A Review. J Diabetes Metab Disord (2013) 12(1):1–9. doi: 10.1186/2251-6581-12-43
9. Ling C. Epigenetic Regulation of Insulin Action and Secretion–Role in the Pathogenesis of Type 2 Diabetes. J Internal Med (2020) 288(2):158–67. doi: 10.1111/joim.13049
10. Rosik J, Szostak B, Machaj F, Pawlik A. The Role of Genetics and Epigenetics in the Pathogenesis of Gestational Diabetes Mellitus. Ann Hum Genet (2020) 84(2):114–24. doi: 10.1111/ahg.12356
11. Benáková Š., Holendová B, Plecitá-Hlavatá L. Redox Homeostasis in Pancreatic β-Cells: From Development to Failure. Antioxidants (2021) 10(4):526. doi: 10.3390/antiox10040526
12. Parker L, Shaw CS, Stepto NK, Levinger I. Exercise and Glycemic Control: Focus on Redox Homeostasis and Redox-Sensitive Protein Signaling. Front Endocrinol (2017) 8:87. doi: 10.3389/fendo.2017.00087
13. Wu N, Shen H, Liu H, Wang Y, Bai Y, Han P. Acute Blood Glucose Fluctuation Enhances Rat Aorta Endothelial Cell Apoptosis, Oxidative Stress and Pro-Inflammatory Cytokine Expression In Vivo. Cardiovasc Diabetol (2016) 15(1):1–13. doi: 10.1186/s12933-016-0427-0
14. Mistry KN, Dabhi BK, Joshi BB. Evaluation of Oxidative Stress Biomarkers and Inflammation in Pathogenesis of Diabetes and Diabetic Nephropathy. Indian J Biochem Biophys (IJBB) (2020) 57(1):45–50.
15. Sangwung P, Petersen KF, Shulman GI, Knowles JW. Mitochondrial Dysfunction, Insulin Resistance, and Potential Genetic Implications: Potential Role of Alterations in Mitochondrial Function in the Pathogenesis of Insulin Resistance and Type 2 Diabetes. Endocrinology (2020) 161(4):bqaa017. doi: 10.1210/endocr/bqaa017
16. Bigagli E, Lodovici M. Circulating Oxidative Stress Biomarkers in Clinical Studies on Type 2 Diabetes and its Complications. Oxid Med Cell Longev (2019) 2019:1–17. doi: 10.1155/2019/5953685
17. Sikalidis AK, Maykish A. The Gut Microbiome and Type 2 Diabetes Mellitus: Discussing a Complex Relationship. Biomedicines (2020) 8(1):8. doi: 10.3390/biomedicines8010008
18. Sharma S, Tripathi P. Gut Microbiome and Type 2 Diabetes: Where We are and Where to Go? J Nutr Biochem (2019) 63:101–8. doi: 10.1016/j.jnutbio.2018.10.003
19. Adeshirlarijaney A, Gewirtz AT. Considering Gut Microbiota in Treatment of Type 2 Diabetes Mellitus. Gut Microbes (2020) 11(3):253–64. doi: 10.1080/19490976.2020.1717719
20. Gurung M, Li Z, You H, Rodrigues R, Jump DB, Morgun A, et al. Role of Gut Microbiota in Type 2 Diabetes Pathophysiology. EBioMedicine (2020) 51:102590. doi: 10.1016/j.ebiom.2019.11.051
21. Becerra-Tomás N, Blanco Mejía S, Viguiliouk E, Khan T, Kendall CW, Kahleova H, et al. Mediterranean Diet, Cardiovascular Disease and Mortality in Diabetes: A Systematic Review and Meta-Analysis of Prospective Cohort Studies and Randomized Clinical Trials. Crit Rev Food Sci Nutr (2020) 60(7):1207–27. doi: 10.1080/10408398.2019.1565281
22. O’Connor LE, Hu EA, Steffen LM, Selvin E, Rebholz CM. Adherence to a Mediterranean-Style Eating Pattern and Risk of Diabetes in a US Prospective Cohort Study. Nutr Diabetes (2020) 10(1):1–9. doi: 10.1038/s41387-020-0113-x
23. Sarkar D, Shetty K. Metabolic Stimulation of Plant Phenolics for Food Preservation and Health. Annu Rev Food Sci Technol (2014) 5:395–413. doi: 10.1146/annurev-food-030713-092418
24. Jiang LL, Gong X, Ji MY, Wang CC, Wang JH, Li MH. Bioactive Compounds From Plant-Based Functional Foods: A Promising Choice for the Prevention and Management of Hyperuricemia. Foods (2020) 9(8):973. doi: 10.3390/foods9080973
25. Salau VF, Erukainure OL, Islam MS. Phenolics: Therapeutic Applications Against Oxidative Injury in Obesity and Type 2 Diabetes Pathology. In: In Pathology. Cambridge, MA: Academic Press (2020). p. 297–307.
26. Shetty K, Sarkar D. Introduction: Metabolic-Driven Ecological Rationale to Advance Biotechnological Approaches for Functional Foods. In: In Functional Foods and Biotechnology. Boca Raton, FL, USA: CRC Press (2019). p. 1–4.
27. Shetty K, Wahlqvist M. A Model for the Role of the Proline-Linked Pentose-Phosphate Pathway in Phenolic Phytochemical Bio-Synthesis and Mechanism of Action for Human Health and Environmental Applications. Asia Pac J Clin Nutr (2004) 13(1):1–24.
28. Sarkar D, Shetty K. Metabolic Modulation of Abiotic Stress Response for Improvement of Functional Ingredients in Food Plants. In: Functional Foods and Biotechnology: Biotransformation and Analysis of Functional Foods and Ingredients. Boca Raton, FL, USA: CRC Press (2020). p. 3.
29. Burton-Freeman B, Brzeziński M, Park E, Sandhu A, Xiao D, Edirisinghe I. A Selective Role of Dietary Anthocyanins and Flavan-3-Ols in Reducing the Risk of Type 2 Diabetes Mellitus: A Review of Recent Evidence. Nutrients (2019) 11(4):841. doi: 10.3390/nu11040841
30. Anhê FF, Desjardins Y, Pilon G, Dudonné S, Genovese MI, Lajolo FM, et al. Polyphenols and Type 2 Diabetes: A Prospective Review. PharmaNutrition (2013) 1(4):105–14. doi: 10.1016/j.phanu.2013.07.004
31. Calderón-Pérez L, Llauradó E, Companys J, Pla-Pagà L, Pedret A, Rubió L, et al. Interplay Between Dietary Phenolic Compound Intake and the Human Gut Microbiome in Hypertension: A Cross-Sectional Study. Food Chem (2021) 344:128567. doi: 10.1016/j.foodchem.2020.128567
32. Kang GG, Francis N, Hill R, Waters D, Blanchard C, Santhakumar AB. Dietary Polyphenols and Gene Expression in Molecular Pathways Associated With Type 2 Diabetes Mellitus: A Review. Int J Mol Sci (2020) 21(1):140. doi: 10.3390/ijms21010140
33. Xiao JB, Hogger P. Dietary Polyphenols and Type 2 Diabetes: Current Insights and Future Perspectives. Curr Med Chem (2015) 22(1):23–38.
34. Hoda M, Hemaiswarya S, Doble M. Mechanisms of Action of Phenolic Phytochemicals in Diabetes Management. In: In Role of Phenolic Phytochemicals in Diabetes Management. Singapore: Springer (2019). p. 83–121. Available at: https://doi.org/10.1007/978-981-13-8997-9_4.
35. Nauck MA, Meier JJ. Incretin Hormones: Their Role in Health and Disease. Diabetes Obes Metab (2018) 20:5–21. doi: 10.1111/dom.13129
36. Fan J, Johnson MH, Lila MA, Yousef G, De Mejia EG. Berry and Citrus Phenolic Compounds Inhibit Dipeptidyl Peptidase IV: Implications in Diabetes Management. Evid Based Complement Alternat Med (2013) 2013:1–13. doi: 10.1155/2013/479505
37. Galbreth MR, Ghosh B. Competition and Sustainability: The Impact of Consumer Awareness. Decis Sci (2013) 44(1):127–59. doi: 10.1111/j.1540-5915.2012.00395.x
38. Christopher A, Sarkar D, Zwinger S, Shetty K. Ethnic Food Perspective of North Dakota Common Emmer Wheat and Relevance for Health Benefits Targeting Type 2 Diabetes. J Ethnic Foods (2018) 5(1):66–74. doi: 10.1016/j.jef.2018.01.002
39. Whittaker A, Dinu M, Cesari F, Gori AM, Fiorillo C, Becatti M, et al. A Khorasan Wheat-Based Replacement Diet Improves Risk Profile of Patients With Type 2 Diabetes Mellitus (T2DM): A Randomized Crossover Trial. Eur J Nutr (2017) 56(3):1191–200. doi: 10.1007/s00394-016-1168-2
40. Ranilla LG, Huamán-Alvino C, Flores-Báez O, Aquino-Méndez EM, Chirinos R, Campos D, et al. Evaluation of Phenolic Antioxidant-Linked In Vitro Bioactivity of Peruvian Corn (Zea Mays L.) Diversity Targeting for Potential Management of Hyperglycemia and Obesity. J Food Sci Technol (2019) 56(6):2909–24. doi: 10.1007/s13197-019-03748-z
41. Ramakrishna R, Sarkar D, Schwarz P, Shetty K. Phenolic Linked Anti-Hyperglycemic Bioactives of Barley (Hordeum Vulgare L.) Cultivars as Nutraceuticals Targeting Type 2 Diabetes. Ind Crops Prod (2017) 107:509–17. doi: 10.1016/j.indcrop.2017.03.03
42. Mishra LK, Sarkar D, Zwinger S, Shetty K. Phenolic Antioxidant-Linked Anti-Hyperglycemic Properties of Rye Cultivars Grown Under Conventional and Organic Production Systems. J Cereal Sci (2017) 76:108–15. doi: 10.1016/j.jcs.2017.06.002
43. Khalaf E. Phenolic-Linked Antioxidant and Anti-Hyperglycemic Properties of Selected Cereal, Pseudo-Cereal, and Millet Using In Vitro Screening Methods. MS Thesis. Fargo, ND, USA: Cereal Science, North Dakota State University (2018).
44. Ranilla LG, Apostolidis E, Genovese MI, Lajolo FM, Shetty K. Evaluation of Indigenous Grains From the Peruvian Andean Region for Antidiabetes and Antihypertension Potential Using In Vitro Methods. J Med Food (2009) 12(4):704–13. doi: 10.1089/jmf.2008.0122
45. Orwat J. Phenolic Antioxidant-Linked Bioactive Enrichment in Black Beans (Phaseolus Vulgaris L.) to Screen for Health Benefits and Enhancement of Salinity Resilience. MS Thesis. Fargo, ND, USA: Cereal Science, North Dakota State University (2016).
46. de Andrade FA, Sarkar D, Miglioranza É., Gonçalves LSA, Miglioranza LHDS, Shetty K. Screening of Snap Bean (Phaseolus Vulgaris L.) Genotypes for Phenolic Bioactive-Linked Anti-Hyperglycemic Properties Using In Vitro Models. J Med Act Plant (2019) 8(3):20–32.
47. Ranilla LG, Kwon YI, Genovese MI, Lajolo FM, Shetty K. Effect of Thermal Treatment on Phenolic Compounds and Functionality Linked to Type 2 Diabetes and Hypertension Management of Peruvian and Brazilian Bean Cultivars (Phaseolus Vulgaris L.) Using In Vitro Methods. J Food Biochem (2010) 34(2):329–55. doi: 10.1111/j.1745-4514.2009.00281.x
48. Kwon YI, Apostolidis E, Shetty K. Evaluation of Pepper (Capsicum Annuum) for Management of Diabetes and Hypertension. J Food Biochem (2007) 31(3):370–85. doi: 10.1111/j.1745-4514.2007.00120.x
49. Kwon YI, Apostolidis E, Shetty K. In Vitro Studies of Eggplant (Solanum Melongena) Phenolics as Inhibitors of Key Enzymes Relevant for Type 2 Diabetes and Hypertension. Bioresour Technol (2008) 99(8):2981–8. doi: 10.1016/j.biortech.2007.06.035
50. Saleem F, Eid AH, Shetty K. Potato–Herb Synergies as Food Designs for Hyperglycemia and Hypertension Management. Funct Foods Nutraceuticals Degenerative Dis Prev (2011) 12:325–40. doi: 10.1002/9780470960844.ch12
51. Mishra LK, Walker-Swaney J, Sarkar D, Shetty K. Bioactive Vegetables Integrated Into Ethnic “Three Sisters Crops” Garden Targeting Foods for Type 2 Diabetes-Associated Health Disparities of American Indian Communities. J Ethnic Foods (2017) 4(3):163–71. doi: 10.1016/j.jef.2017.08.007
52. Chintha P. Functional Bioactive Compounds From Sweet Potatoes for Human Health Benefits, Doctoral Dissertation, Department of Plant Sciences. Fargo, ND, USA: North Dakota State University (2020).
53. Barbosa ACL, Pinto MDS, Sarkar D, Ankolekar C, Greene D, Shetty K. Varietal Influences on Antihyperglycemia Properties of Freshly Harvested Apples Using In Vitro Assay Models. J Med Food (2010) 13(6):1313–23. doi: 10.1089/jmf.2009.0273
54. Sarkar D, Ankolekar C, Pinto M, Shetty K. Dietary Functional Benefits of Bartlett and Starkrimson Pears for Potential Management of Hyperglycemia, Hypertension and Ulcer Bacteria Helicobacter Pylori While Supporting Beneficial Probiotic Bacterial Response. Food Res Int (2015) 69:80–90. doi: 10.1016/j.foodres.2014.12.014
55. Ankolekar C, Pinto M, Greene D, Shetty K. Phenolic Bioactive Modulation by Lactobacillus Acidophilus Mediated Fermentation of Cherry Extracts for Anti-Diabetic Functionality, Helicobacter Pylori Inhibition and Probiotic Bifidobacterium Longum Stimulation. Food Biotechnol (2011) 25(4):305–35. doi: 10.1080/08905436.2011.617255
56. Fujita A, Sarkar D, Wu S, Kennelly E, Shetty K, Genovese MI. Evaluation of Phenolic-Linked Bioactives of Camu-Camu (Myrciaria Dubia Mc. Vaugh) for Antihyperglycemia, Antihypertension, Antimicrobial Properties and Cellular Rejuvenation. Food Res Int (2015) 77(part 2):194–203. doi: 10.1016/j.foodres.2015.07.009
57. Fujita A, Sarkar D, Genovese MI, Shetty K. Improving Anti-Hyperglycemic and Anti-Hypertensive Properties of Camu-Camu (Myriciaria Dubia Mc. Vaugh) Using Lactic Acid Bacterial Fermentation. Process Biochem (2017) 59:133–40. doi: 10.1016/j.procbio.2017.05.017
58. Ramalho SA, Narain N, Andrade JKS, de Oliveira CS, Sarkar D, Shetty K. Evaluation of Phenolic-Linked Anti-Hyperglycemic Properties of Tropical Brazilian Fruits for Potential Management of Early Stages Type 2 Diabetes. Fruits (2018) 73(5):273–82. doi: 10.17660/th2018/73.5.3
59. Sarkar D, Agustinah W, Woods F, Coneva E, Vinson E, Shetty K. In Vitro Screening and Evaluation of Phenolic Antioxidant-Linked Anti-Hyperglycemic Functions of Rabbit-Eye Blueberry (Vaccinium Ashei) Cultivars. J Berry Res (2017) 7(3):163–77. doi: 10.3233/JBR-170154
60. Sarkar D, Orwat J, Hurburt T, Woods F, Pitts JA, Shetty K. Evaluation of Phenolic Bioactive-Linked Functionality of Blackberry Cultivars Targeting Dietary Management of Early Stages Type-2 Diabetes Using. Vitro models Sci Hortic (2016) 212:193–202. doi: 10.1016/j.scienta.2016.10.003
61. da Silva Pinto M, Kwon YI, Apostolidis E, Lajolo FM, Genovese MI, Shetty K. Evaluation of Red Currants (Ribes Rubrum L.), Black Currants (Ribes Nigrum L.), Red and Green Gooseberries (Ribes Uva-Crispa) for Potential Management of Type 2 Diabetes and Hypertension Using In Vitro Models. J Food Biochem (2010) 34(3):639–60. doi: 10.1111/j.1745-4514.2009.00305.x
62. Mishra LK, Sarkar D, Mentreddy R, Shetty K. Evaluation of Phenolic Bioactive-Linked Anti-Hyperglycemic and Helicobacter Pylori Inhibitory Activities of Asian Basil (Ocimum Spp.) Varieties. J Herbal Med (2020) 20:100310. doi: 10.1016/j.hermed.2019.100310
63. Saleem F, Sarkar D, Ankolekar C, Shetty K. Phenolic Bioactives and Associated Antioxidant and Anti-Hyperglycemic Functions of Select Species of Apiaceae Family Targeting for Type 2 Diabetes Relevant Nutraceuticals. Ind Crops Prod (2017) 107:518–25. doi: 10.1016/j.indcrop.2017.06.023
64. Sarkar D, Pinto MDS, Shetty K. Targeted Screening and Improvement of the Medicinal Properties of Oregano and Rhodiola With Chitosan Oligosaccharide and Vitamin C Using in Vitro Assays for Hyperglycemia and Hypertension Linked to Type 2 Diabetes. J Herbs Spices Med Plants (2017) 23(4):347–62. doi: 10.1080/10496475.2017.1341859
65. Phoboo S, Pinto MDS, Barbosa ACL, Sarkar D, Bhowmik PC, Jha PK, et al. Phenolic-Linked Biochemical Rationale for the Anti-Diabetic Properties of Swertia Chirayita (Roxb. Ex Flem.) Karst. Phytother Res (2013) 27(2):227–35. doi: 10.1002/ptr.4714
66. McMacken M, Shah S. A Plant-Based Diet for the Prevention and Treatment of Type 2 Diabetes. Journal of Geriatric Cardiology. JGC (2017) 14(5):342–54. doi: 10.11909/2Fj.issn.1671-5411.2017.05.009
67. Aune D, Keum N, Giovannucci E, Fadnes LT, Boffetta P, Greenwood DC, et al. Whole Grain Consumption and Risk of Cardiovascular Disease, Cancer, and All Cause and Cause Specific Mortality: Systematic Review and Dose-Response Meta-Analysis of Prospective Studies. BMJ (2016) 353:342–54. doi: 10.1136/bmj.i2716
68. Parker ED, Liu S, Van Horn L, Tinker LF, Shikany JM, Eaton CB, et al. The Association of Whole Grain Consumption With Incident Type 2 Diabetes: The Women's Health Initiative Observational Study. Ann Epidemiol (2013) 23(6):321–7. doi: 10.1016/j.annepidem.2013.03.010
69. Belobrajdic DP, Bird AR. The Potential Role of Phytochemicals in Wholegrain Cereals for the Prevention of Type-2 Diabetes. Nutr J (2013) 12(1):1–12. doi: 10.1186/1475-2891-12-62
70. Christopher A, Sarkar D, Shetty K. “Human Health-Relevant Bioactive Functionalities of Ancient Emmer Wheat.” In Functional Foods and Biotechnology. CRC Press (2019). pp. 47–59.
71. Galvez Ranilla L, Christopher A, Sarkar D, Shetty K, Chirinos R, Campos D. Phenolic Composition and Evaluation of the Antimicrobial Activity of Free and Bound Phenolic Fractions From a Peruvian Purple Corn (Zea Mays L.) Accession. J Food Sci (2017) 82(12):2968–76. doi: 10.1111/1750-3841.13973
72. Ramakrishna R, Sarkar D, Manduri A, Iyer SG, Shetty K. Improving Phenolic Bioactive-Linked Anti-Hyperglycemic Functions of Dark Germinated Barley Sprouts (Hordeum Vulgare L.) Using Seed Elicitation Strategy. J Food Sci Technol (2017) 54(11):3666–78. doi: 10.1007/s13197-017-2828-
73. Ramakrishna R, Sarkar D, Shetty K. Metabolic Stimulation of Phenolic Biosynthesis and Antioxidant Enzyme Response in Dark Germinated Barley (Hordeum Vulgare L.) Sprouts Using Bioprocessed Elicitors. Food Sci Biotechnol (2019) 28(4):1093–106. doi: 10.1007/s10068-018-0535-6
74. Moloto RM, Moremi LH, Soundy P, Maseko ST. Biofortification of Common Bean as a Complementary Approach to Addressing Zinc Deficiency in South Africans. Acta Agric Scand Sect B—Soil Plant Sci (2018) 68(7):575–84. doi: 10.1080/09064710.2018.1454507
75. Randhir R, Kwon YI, Lin YT, Shetty K. Effect of Thermal Processing on the Phenolic Associated Health-Relevant Functionality of Selected Legume Sprouts and Seedlings. J Food Biochem (2009) 33(1):89–112. doi: 10.1111/j.1745-4514.2008.00210.x
76. Cooper AJ, Sharp SJ, Luben RN, Khaw KT, Wareham NJ, Forouhi NG. The Association Between a Biomarker Score for Fruit and Vegetable Intake and Incident Type 2 Diabetes: The EPIC-Norfolk Study. Eur J Clin Nutr (2015) 69(4):449–54. doi: 10.1038/ejcn.2014.246
77. Wang PY, Fang JC, Gao ZH, Zhang C, Xie SY. Higher Intake of Fruits, Vegetables or Their Fiber Reduces the Risk of Type 2 Diabetes: A Meta-Analysis. J Diabetes Invest (2016) 7(1):56–69. doi: 10.1111/jdi.12376
78. Wu Y, Zhang D, Jiang X, Jiang W. Fruit and Vegetable Consumption and Risk of Type 2 Diabetes Mellitus: A Dose-Response Meta-Analysis of Prospective Cohort Studies. Nutr Metab Cardiovasc Dis (2015) 25(2):140–7. doi: 10.1016/j.numecd.2014.10.004
79. Schwingshackl L, Hoffmann G, Lampousi AM, Knüppel S, Iqbal K, Schwedhelm C, et al. Food Groups and Risk of Type 2 Diabetes Mellitus: A Systematic Review and Meta-Analysis of Prospective Studies. Eur J Eepidemiol (2017) 32(5):363–75. doi: 10.1007/s10654-017-0246-y
80. Muraki I, Imamura F, Manson JE, Hu FB, Willett WC, van Dam RM, et al. Fruit Consumption and Risk of Type 2 Diabetes: Results From Three Prospective Longitudinal Cohort Studies. BMJ (2013) 347:1–15. doi: 10.1136/bmj.f5001
81. Jenkins DJA, Srichaikul K, Kendall CWC, Sievenpiper JL, Abdulnour S, Mirrahimi A, et al. The Relation of Low Glycaemic Index Fruit Consumption to Glycaemic Control and Risk Factors for Coronary Heart Disease in Type 2 Diabetes. Diabetologia (2011) 54(2):271–9. doi: 10.1007/s00125-010-1927-1
82. Guo H, Gao X, Ma R, Liu J, Ding Y, Zhang M, et al. Prevalence of Metabolic Syndrome and its Associated Factors Among Multi-Ethnic Adults in Rural Areas in Xinjiang, China. Sci Rep (2017) 7(1):1–9. doi: 10.1038/s41598-017-17870-5
83. Da Porto A, Cavarape A, Colussi G, Casarsa V, Catena C, Sechi LA. Polyphenols Rich Diets and Risk of Type 2 Diabetes. Nutrients (2021) 13(5):1445. doi: 10.3390/nu13051445
84. Ankolekar C, Sarkar D, Greene D, Shetty K. Using Biological Elicitation to Improve Type 2 Diabetes Targeted Food Quality of Stored Apple. Front Sustain Food Syst (2021) 5:709384. doi: 10.3389/fsufs.2021.709384
85. Adyanthaya I, Kwon YI, Apostolidis E, Shetty K. Health Benefits of Apple Phenolics From Postharvest Stages for Potential Type 2 Diabetes Management Using In Vitro Models. J Food Biochem (2010) 34(1):31–49. doi: 10.1111/j.1745-4514.2009.00257.x
86. Barbosa ACL, Sarkar D, Pinto MDS, Ankolekar C, Greene D, Shetty K. Type 2 Diabetes Relevant Bioactive Potential of Freshly Harvested and Long-Term Stored Pears Using In Vitro Assay Models. J Food Biochem (2013) 37(6):677–86. doi: 10.1111/j.1745-4514.2012.00665.x
87. Kirakosyan A, Gutierrez E, Solano BR, Seymour EM, Bolling SF. The Inhibitory Potential of Montmorency Tart Cherry on Key Enzymes Relevant to Type 2 Diabetes and Cardiovascular Disease. Food Chem (2018) 252:142–6. doi: 10.1016/j.foodchem.2018.01.084
88. Pinto MDS, Ranilla LG, Apostolidis E, Lajolo FM, Genovese MI, Shetty K. Evaluation of Antihyperglycemia and Antihypertension Potential of Native Peruvian Fruits Using In Vitro Models. J Med Food (2009) 12(2):278–91. doi: 10.1089/jmf.2008.0113
89. Kowalska K, Olejnik A. Current Evidence on the Health-Beneficial Effects of Berry Fruits in the Prevention and Treatment of Metabolic Syndrome. Curr Opin Clin Nutr Metab Care (2016) 19(6):446–52. doi: 10.1097/MCO.0000000000000322
90. Zhang AJ, Rimando AM, Fish W, Mentreddy SR, Mathews ST. Serviceberry [Amelanchier Alnifolia (Nutt.) Nutt. Ex. M. Roem (Rosaceae)] Leaf Extract Inhibits Mammalian α-Glucosidase Activity and Suppresses Postprandial Glycemic Response in a Mouse Model of Diet-Induced Obesity and Hyperglycemia. J Ethnopharmacol (2012) 143(2):481–7. doi: 10.1016/j.jep.2012.06.054
91. Bispo K, Amusquivar E, García-Seco D, Ramos-Solano B, Gutierrez-Mañero J. And Herrera, E). Supplementing Diet With Blackberry Extract Causes a Catabolic Response With Increments in Insulin Sensitivity in Rats. Plant Foods Hum Nutr (2015) 70(2):170–5. doi: 10.1007/s11130-015-0474-9
92. Cheplick S, Kwon YI, Bhowmik P, Shetty K. Phenolic-Linked Variation in Strawberry Cultivars for Potential Dietary Management of Hyperglycemia and Related Complications of Hypertension. Bioresour Technol (2010) 101(1):404–13. doi: 10.1016/j.biortech.2009.07.068
93. Cheplick S, Sarkar D, Bhowmik P, Shetty K. Phenolic Bioactives From Developmental Stages of Highbush Blueberry (Vaccinium Corymbosum) for Hyperglycemia Management Using In Vitro Models. Can J Plant Sci (2015) 95(4):653–62. doi: 10.4141/cjps-2014-352
94. da Silva Pinto M, de Carvalho JE, Lajolo FM, Genovese MI, Shetty K. Evaluation of Antiproliferative, Anti-Type 2 Diabetes, and Antihypertension Potentials of Ellagitannins From Strawberries (Fragaria× Ananassa Duch.) Using In Vitro Models. J Med Food (2010) 13(5):1027–35. doi: 10.1089/jmf.2009.0257
95. Wang SY, Camp MJ, Ehlenfeldt MK. Antioxidant Capacity and α-Glucosidase Inhibitory Activity in Peel and Flesh of Blueberry (Vaccinium Spp.) Cultivars. Food Chem (2012) 132(4):1759–68. doi: 10.1016/j.foodchem.2011.11.134
96. Pranprawit A, Heyes JA, Molan AL, Kruger MC. Antioxidant Activity and Inhibitory Potential of Blueberry Extracts Against Key Enzymes Relevant for Hyperglycemia. J Food Biochem (2015) 39(1):109–18. doi: 10.1111/jfbc.12094
97. Espe AA. Phenolic Bioactive-Linked Antioxidant, Anti-Hyperglycemic, and Anti-Hypertensive Properties of Serviceberry and Blackberry. Fargo, ND, USA: Master Thesis, Department of Plant Sciences, North Dakota State University (2019).
98. Pinto MDS, Ghaedian R, Shinde R, Shetty K. Potential of Cranberry Powder for Management of Hyperglycemia Using In Vitro Models. J Med Food (2010) 13(5):1036–44. doi: 10.1089/jmf.2009.0225
99. Hameed A, Galli M, Adamska-Patruno E, Krętowski A, Ciborowski M. Select Polyphenol-Rich Berry Consumption to Defer or Deter Diabetes and Diabetes-Related Complications. Nutrients (2020) 12(9):2538. doi: 10.3390/nu12092538
100. Nakano H, Wu S, Sakao K, Hara T, He J, Garcia S, et al. Bilberry Anthocyanins Ameliorate NAFLD by Improving Dyslipidemia and Gut Microbiome Dysbiosis. Nutrients (2020) 12(11):3252. doi: 10.3390/nu12113252
101. Mohamed DA, Hamed IM, Fouda KA. Research Article Antioxidant and Anti-Diabetic Effects of Cumin Seeds Crude Ethanol Extract. J Biol Sci (2018) 18(5):251–9. doi: 10.3923/jbs.2018.251.259
102. Kwon YI, Jang HD, Shetty K. Evaluation of Rhodiola Crenulata and Rhodiola Rosea for Management of Type II Diabetes and Hypertension. Asia Pac J Clin Nutr (2006) 15(3):425–32.
103. Pinto MDS, Kwon YI, Apostolidis E, Lajolo FM, Genovese MI, Shetty K. Potential of Ginkgo Biloba L. Leaves in the Management of Hyperglycemia and Hypertension Using In Vitro Models. Bioresour Technol (2009) 100(24):6599–609. doi: 10.1016/j.biortech.2009.07.021
104. Ranilla LG, Kwon YI, Apostolidis E, Shetty K. Phenolic Compounds, Antioxidant Activity and In Vitro Inhibitory Potential Against Key Enzymes Relevant for Hyperglycemia and Hypertension of Commonly Used Medicinal Plants, Herbs and Spices in Latin America. Bioresour Technol (2010) 101(12):4676–89. doi: 10.1016/j.biortech.2010.01.093
105. Ahumada J, Fuentealba C, Olaeta JA, Undurraga P, Pedreschi R, Shetty K, et al. Bioactive Compounds of Loquat (Eriobotrya Japonica Lindl.) Cv. Golden Nugget and Analysis of the In Vitro Functionality for Hyperglycemia Management. Int J Agric Natural Resour (2017) 44(3):272–84. doi: 10.7764/rcia.v44i3.1816
106. Manduri A, Sarkar D, Fischer M, Vargas C, Shetty K. Instantaneous Heat Shock Treatment in Grape During Pre-Harvest Stages Enhances Phenolic-Linked Medicinal Properties in Red Wine. J Med Act Plant (2017) 5(2):36–46.
107. Alam MA, Juraimi AS, Rafii MY, Hamid AA, Aslani F, Alam MZ. Effects of Salinity and Salinity-Induced Augmented Bioactive Compounds in Purslane (Portulaca Oleracea L.) for Possible Economical Use. Food Chem (2015) 169:439–47. doi: 10.1016/j.foodchem.2014.08.019
108. Christopher A, Orwat J, Sarkar D, Hatterman-Valenti H, Shetty K. Ozone Elicited Phenolic Bioactives in Grapes and Health Relevant Screening Targeted for Type 2 Diabetes Using In Vitro Assay Models. J Med Act Plant (2018) 7(1):1–11.
109. Giménez MJ, Valverde JM, Valero D, Guillén F, Martínez-Romero D, Serrano M, et al. Quality and Antioxidant Properties on Sweet Cherries as Affected by Preharvest Salicylic and Acetylsalicylic Acids Treatments. Food Chem (2014) 160:226–32. doi: 10.1016/j.foodchem.2014.03.107
110. Yin H, Fretté XC, Christensen LP, Grevsen K. Chitosan Oligosaccharides Promote the Content of Polyphenols in Greek Oregano (Origanum Vulgare Ssp. Hirtum). J Agric Food Chem (2012) 60(1):136–43. doi: 10.1021/jf204376j
111. He Y, Bose SK, Wang W, Jia X, Lu H, Yin H. Pre-Harvest Treatment of Chitosan Oligosaccharides Improved Strawberry Fruit Quality. Int J Mol Sci (2018) 19(8):2194. doi: 10.3390/ijms19082194
112. Bhatt K, Jampala SSM. Influence of Pre-Harvest Foliar Spray of Fungal Culture Filtrates on Post-Harvest Biology of Date Fruit Harvested at Khalal Stage. Postharvest Biol Technol (2020) 166:111220. doi: 10.1016/j.postharvbio.2020.111220
113. Gautier H, Diakou-Verdin V, Bénard C, Reich M, Buret M, Bourgaud F, et al. How Does Tomato Quality (Sugar, Acid, and Nutritional Quality) Vary With Ripening Stage, Temperature, and Irradiance? J Agric Food Chem (2008) 56(4):1241–50. doi: 10.1021/jf072196t
114. Randhir R, Kwon YI, Shetty K. Effect of Thermal Processing on Phenolics, Antioxidant Activity and Health-Relevant Functionality of Select Grain Sprouts and Seedlings. Innovative Food Sci Emerging Technol (2008) 9(3):355–64. doi: 10.1016/j.ifset.2007.10.004
115. Hester F, Verghese M, Willis S, Baldwin A, Reid M, Shackelford L, et al. The Anti-Diabetic Potential of Thermally Treated Garlic, Turmeric, and Ginger in Pre-Diabetic Male Wistar Rat Model. Food Nutr Sci (2018) 9(04):420. doi: 10.4236/fns.2018.94033
116. Hester F, Verghese M, Sunkara R, Willis S, Walker LT. A Comparison of the Antioxidative and Anti-Diabetic Potential of Thermally Treated Garlic, Turmeric, and Ginger. Food Nutr Sci (2019) 10(2):207–19. doi: 10.4236/fns.2019.102016
117. Kang YR, Choi HY, Lee JY, Jang SI, Kang H, Oh JB, et al. Calorie Restriction Effect of Heat-Processed Onion Extract (ONI) Using In Vitro and In Vivo Animal Models. Int J Mol Sci (2018) 19(3):874. doi: 10.3390/ijms19030874
118. Song Y, Xu B, Cai W. Active Substances and In Vitro Anti-Diabetic Effects of a Traditional Folk Remedy Bian-Que Triple-Bean Soup as Affected by the Boiling Time. Food Funct (2013) 4(4):635–43. doi: 10.1039/C3FO30303A
119. Kunyanga CN, Imungi JK, Okoth MW, Biesalski HK, Vadivel V. Total Phenolic Content, Antioxidant and Antidiabetic Properties of Methanolic Extract of Raw and Traditionally Processed Kenyan Indigenous Food Ingredients. LWT-Food Sci Technol (2012) 45(2):269–76. doi: 10.1016/j.lwt.2011.08.006
120. Gupta SK, Mandal P. Involvement of Calcium Ion in Enhancement of Antioxidant and Antidiabetic Potential of Fenugreek Sprouts. Free Radic Antioxid (2015) 5(2):74–82. doi: 10.5530/fra.2015.2.5
121. Randhir R, Shetty K. Mung Beans Processed by Solid-State Bioconversion Improves Phenolic Content and Functionality Relevant for Diabetes and Ulcer Management. Innovative Food Sci Emerging Technol (2007) 8(2):197–204. doi: 10.1016/j.ifset.2006.10.003
122. Randhir R, Shetty K. Improved α-Amylase and Helicobacter Pylori Inhibition by Fenugreek Extracts Derived via Solid-State Bioconversion Using Rhizopus Oligosporus. Asia Pac J Clin Nutr (2007) 16(3):382–92.
123. Chen C, Hu W, He Y, Jiang A, Zhang R. Effect of Citric Acid Combined With UV-C on the Quality of Fresh-Cut Apples. Postharvest Biol Technol (2016) 111:126–31. doi: 10.1016/j.postharvbio.2015.08.005
124. Randhir R, Kwon YI, Shetty K. Improved Health-Relevant Functionality in Dark Germinated Mucuna Pruriens Sprouts by Elicitation With Peptide and Phytochemical Elicitors. Bioresour Technol (2009) 100(19):4507–14. doi: 10.1016/j.biortech.2009.01.078
125. Agustinah W, Sarkar D, Woods F, Shetty K. Apple and Blueberry Synergies for Designing Bioactive Ingredients for the Management of Early Stages of Type 2 Diabetes. J Food Qual (2016) 39(4):370–82. doi: 10.1111/jfq.12206
126. Apostolidis E, Kwon YI, Shetty K. Potential of Select Yogurts for Diabetes and Hypertension Management. J Food Biochem (2006) 30(6):699–717. doi: 10.1111/j.1745-4514.2006.00091.x
127. Apostolidis E, Kwon YI, Shetty K. Inhibitory Potential of Herb, Fruit, and Fungal-Enriched Cheese Against Key Enzymes Linked to Type 2 Diabetes and Hypertension. Innovative Food Sci Emerging Technol (2007) 8(1):46–54. doi: 10.1016/j.ifset.2006.06.001
128. Kwon YI, Apostolidis E, Shetty K. Anti-Diabetes Functionality of Kefir Culture-Mediated Fermented Soymilk Supplemented With Rhodiola Extracts. Food Biotechnol (2006) 20(1):13–29. doi: 10.1080/08905430500522055
129. Pucel N, Sarkar D, Labbe RG, Khanongnuch C, Shetty K. Improving Health Targeted Food Quality of Blackberry: Pear Fruit Synergy Using Lactic Acid Bacterial Fermentation. Front Sustain Food Syst (2021) 5:703672. doi: 10.3389/fsufs.2021.703672
130. Tkacz K, Wojdyło A, Turkiewicz IP, Nowicka P. Anti-Diabetic, Anti-Cholinesterase, and Antioxidant Potential, Chemical Composition and Sensory Evaluation of Novel Sea Buckthorn-Based Smoothies. Food Chem (2021) 338:128105. doi: 10.1016/j.foodchem.2020.128105
131. da Silva Fernandes M, Lima FS, Rodrigues D, Handa C, Guelfi M, Garcia S, et al. Evaluation of the Isoflavone and Total Phenolic Contents of Kefir-Fermented Soymilk Storage and After the In Vitro Digestive System Simulation. Food Chem (2017) 229:373–80. doi: 10.1016/j.foodchem.2017.02.095
132. Ankolekar C, Johnson K, Pinto M, Johnson D, Labbe RG, Greene D, et al. Fermentation of Whole Apple Juice Using Lactobacillus Acidophilus for Potential Dietary Management of Hyperglycemia, Hypertension, and Modulation of Beneficial Bacterial Responses. J Food Biochem (2012) 36(6):718–38. doi: 10.1111/j.1745-4514.2011.00596.x
133. Ankolekar C, Pinto M, Greene D, Shetty K. In Vitro Bioassay Based Screening of Antihyperglycemia and Antihypertensive Activities of Lactobacillus Acidophilus Fermented Pear Juice. Innovative Food Sci Emerging Technol (2012) 13:221–30. doi: 10.1016/j.ifset.2011.10.008
134. Kaprasob R, Kerdchoechuen O, Laohakunjit N, Sarkar D, Shetty K. Fermentation-Based Biotransformation of Bioactive Phenolics and Volatile Compounds From Cashew Apple Juice by Select Lactic Acid Bacteria. Process Biochem (2017) 59:141–9. doi: 10.1016/j.procbio.2017.05.019
135. Kaprasob R, Sarkar D, Kerdchoechuen O, Laohakunjit N, Khanongnuch C, Shetty K. Beneficial Lactic Acid Bacteria Based Bioprocessing of Cashew Apple Juice for Targeting Antioxidant Nutraceutical Inhibitors as Relevant Antidotes to Type 2 Diabetes. Process Biochem (2019) 82:40–50. doi: 10.1016/j.procbio.2019.05.005
136. Shori AB, Baba AS. Antioxidant Activity and Inhibition of Key Enzymes Linked to Type-2 Diabetes and Hypertension by Azadirachta Indica-Yogurt. J Saudi Chem Soc (2013) 17(3):295–301. doi: 10.1016/j.jscs.2011.04.006
137. Shori AB. Proteolytic Activity, Antioxidant, and α-Amylase Inhibitory Activity of Yogurt Enriched With Coriander and Cumin Seeds. LWT (2020) 133:109912. doi: 10.1016/j.lwt.2020.109912
138. Mashitoa FM, Akinola SA, Manhevi VE, Garcia C, Remize F, Slabbert R, et al. Influence of Fermentation of Pasteurised Papaya Puree With Different Lactic Acid Bacterial Strains on Quality and Bioaccessibility of Phenolic Compounds During. In Vitro Digestion Foods (2021) 10(5):962. doi: 10.3390/foods10050962
139. Wang X, Han M, Zhang M, Wang Y, Ren Y, Yue T, et al. In Vitro Evaluation of the Hypoglycemic Properties of Lactic Acid Bacteria and its Fermentation Adaptability in Apple Juice. LWT (2021) 136:110363. doi: 10.1016/j.lwt.2020.110363
140. Chintha P, Sarkar D, Pecota K, Dogramaci M, Shetty K. Improving Phenolic Bioactive-Linked Functional Qualities of Sweet Potatoes Using Beneficial Lactic Acid Bacteria-Based Biotransformation Strategy. Horticulturae (2021) 7(10):367. doi: 10.3390/horticulturae7100367
141. Gao H, Wen JJ, Hu JL, Nie QX, Chen HH, Xiong T, et al. Fermented Momordica Charantia L. Juice Modulates Hyperglycemia, Lipid Profile, and Gut Microbiota in Type 2 Diabetic Rats. Food Res Int (2019) 121:367–78. doi: 10.1016/j.foodres.2019.03.055
142. Wen JJ, Li MZ, Gao H, Hu JL, Nie QX, Chen HH, et al. Polysaccharides From Fermented Momordica Charantia L. With Lactobacillus Plantarum NCU116 Ameliorate Metabolic Disorders and Gut Microbiota Change in Obese Rats. Food Funct (2021) 12(6):2617–30. doi: 10.1039/D0FO02600J
143. Herencia JF, García-Galavís PA, Dorado JAR, Maqueda C. Comparison of Nutritional Quality of the Crops Grown in an Organic and Conventional Fertilized Soil. Sci Hortic (2011) 129(4):882–8. doi: 10.1016/j.scienta.2011.04.008
144. Smith-Spangler C, Brandeau ML, Hunter GE, Bavinger JC, Pearson M, Eschbach PJ, et al. Are Organic Foods Safer or Healthier Than Conventional Alternatives? A Systematic Review. Ann Internal Med (2012) 157(5):348–66. doi: 10.7326/0003-4819-157-5-201209040-00007
145. You Q, Wang B, Chen F, Huang Z, Wang X, Luo PG. Comparison of Anthocyanins and Phenolics in Organically and Conventionally Grown Blueberries in Selected Cultivars. Food Chem (2011) 125(1):201–8. doi: 10.1016/j.foodchem.2010.08.063
146. Oliveira AB, Moura CF, Gomes-Filho E, Marco CA, Urban L, Miranda MRA. The Impact of Organic Farming on Quality of Tomatoes is Associated to Increased Oxidative Stress During Fruit Development. PloS One (2013) 8(2):e56354. doi: 10.1371/journal.pone.0056354
147. Phoboo S, Bhowmik PC, Jha PK, Shetty K. Phenolic-Linked Antioxidant, Anti-Diabetic, and Anti-Hypertensive Potential of Wild and Cultivated Swertia Chirayita (Roxb. Ex Flem.) Karst. Using In Vitro Assays. J Herbs Spices Med Plants (2014) 20(1):55–69. doi: 10.1080/10496475.2013.822448
148. Sarkar D, Ankolekar C, Shetty K. Benefcial Lactic Acid Bacteria (LAB)-Based Biotransformation of Plant and Dairy Substrates to Enhance Type 2 Diabetes-Relevant Health Benefts. In: Functional Foods and Biotechnology: Biotransformation and Analysis of Functional Foods and Ingredients. Boca Raton, FL, USA: CRC Press (2020). p. 345.
149. Sahai V, Kumar V. Anti-Diabetic, Hepatoprotective and Antioxidant Potential of Brassica Oleracea Sprouts. Biocatal Agric Biotechnol (2020) 25:101623. doi: 10.1016/j.bcab.2020.101623
150. Obaroakpo JU, Liu L, Zhang S, Lu J, Liu L, Pang X, et al. In Vitro Modulation of Glucagon-Like Peptide Release by DPP-IV Inhibitory Polyphenol-Polysaccharide Conjugates of Sprouted Quinoa Yoghurt. Food Chem (2020) 324:126857. doi: 10.1016/j.foodchem.2020.126857
151. Apostolidis E, Kwon YI, Shetty K, Apostolidis E, Kwon YI. Potential of Cranberry-Based Herbal Synergies for Diabetes and Hypertension Management. Asia Pac J Clin Nutr (2006) 15(3):433–41.
152. Chukwuma CI, Matsabisa MG, Ibrahim MA, Erukainure OL, Chabalala MH, Islam MS. Medicinal Plants With Concomitant Anti-Diabetic and Anti-Hypertensive Effects as Potential Sources of Dual Acting Therapies Against Diabetes and Hypertension: A Review. J Ethnopharmacol (2019) 235:329–60. doi: 10.1016/j.jep.2019.02.024
153. Gupta RC, Chang D, Nammi S, Bensoussan A, Bilinski K, Roufogalis BD. Interactions Between Antidiabetic Drugs and Herbs: An Overview of Mechanisms of Action and Clinical Implications. Diabetol Metab Syndrome (2017) 9(1):1–12. doi: 10.1186/s13098-017-0254-9
154. Warner SD. Evaluation of Blackberry Cultivars and Blackberry-Pear Synergies for Potential Management of Type Ii Diabetes and Hypertension. Master Thesis, Food Science. Amherst, MA, USA: University of Massachusetts (2012).
155. Roopchand DE, Kuhn P, Rojo LE, Lila MA, Raskin I. Blueberry Polyphenol-Enriched Soybean Flour Reduces Hyperglycemia, Body Weight Gain and Serum Cholesterol in Mice. Pharmacol Res (2013) 68(1):59–67. doi: 10.1016/j.phrs.2012.11.008
156. Moghadasian MH, Masisi K, Le K, Beta T, Shen GX, Fischer G. The Potential Anti-Diabetic Effects of Saskatoon Berry in Experimental Mouse Models. Austin J Nutr Food Sci (2019) 7(1). doi: 10.26420/austinjnutrifoodsci.2019.1111
157. Raimundo AF, Félix F, Andrade R, García-Conesa MT, González-Sarrías A, Gilsa-Lopes J, et al. Combined Effect of Interventions With Pure or Enriched Mixtures of (Poly) Phenols and Anti-Diabetic Medication in Type 2 Diabetes Management: A Meta-Analysis of Randomized Controlled Human Trials. Eur J Nutr (2020) 59(4):1329–43. doi: 10.1007/s00394-020-02189-1
158. Gille D, Schmid A, Walther B, Vergères G. Fermented Food and non-Communicable Chronic Diseases: A Review. Nutrients (2018) 10(4):448. doi: 10.3390/nu10040448
159. Chen Y, Liu W, Xue J, Yang J, Chen X, Shao Y, et al. Angiotensin-Converting Enzyme Inhibitory Activity of Lactobacillus Helveticus Strains From Traditional Fermented Dairy Foods and Antihypertensive Effect of Fermented Milk of Strain H9. J Dairy Sci (2014) 97(11):6680–92. doi: 10.3168/jds.2014-7962
160. Eussen SJ, van Dongen MC, Wijckmans N, den Biggelaar L, Elferink SJO, Singh-Povel CM, et al. Consumption of Dairy Foods in Relation to Impaired Glucose Metabolism and Type 2 Diabetes Mellitus: The Maastricht Study. Br J Nutr (2016) 115(8):1453–61. doi: 10.1017/S0007114516000313
161. Gijsbers L, Ding EL, Malik VS, De Goede J, Geleijnse JM, Soedamah-Muthu SS. Consumption of Dairy Foods and Diabetes Incidence: A Dose-Response Meta-Analysis of Observational Studies. Am J Clin Nutr (2016) 103(4):1111–24. doi: 10.3945/ajcn.115.123216
162. O’Connor LM, Lentjes MA, Luben RN, Khaw KT, Wareham NJ, Forouhi NG. Dietary Dairy Product Intake and Incident Type 2 Diabetes: A Prospective Study Using Dietary Data From a 7-Day Food Diary. Diabetologia (2014) 57(5):909–17. doi: 10.1007/s00125-014-3176-1
163. Patil P, Mandal S, Tomar SK, Anand S. Food Protein-Derived Bioactive Peptides in Management of Type 2 Diabetes. Eur J Nutr (2015) 54(6):863–80. doi: 10.1007/s00394-015-0974-2
Keywords: alpha-amylase, alpha-glucosidase, antioxidant, anti-hyperglycemia, bioprocessing strategies, carbohydrate metabolism, phenolics, plant foods
Citation: Sarkar D, Christopher A and Shetty K (2022) Phenolic Bioactives From Plant-Based Foods for Glycemic Control. Front. Endocrinol. 12:727503. doi: 10.3389/fendo.2021.727503
Received: 18 June 2021; Accepted: 21 December 2021;
Published: 18 January 2022.
Edited by:
Gelsomina Fico, University of Milan, ItalyReviewed by:
Zhifeng Xue, Tianjin University of Traditional Chinese Medicine, ChinaZihao Chen, National University of Singapore Suzhou Research Institute, China
Copyright © 2022 Sarkar, Christopher and Shetty. This is an open-access article distributed under the terms of the Creative Commons Attribution License (CC BY). The use, distribution or reproduction in other forums is permitted, provided the original author(s) and the copyright owner(s) are credited and that the original publication in this journal is cited, in accordance with accepted academic practice. No use, distribution or reproduction is permitted which does not comply with these terms.
*Correspondence: Kalidas Shetty, a2FsaWRhcy5zaGV0dHlAbmRzdS5lZHU=