- 1Division of Endocrinology, Department of Internal Medicine, Faculty of Medicine, Chiang Mai University, Chiang Mai, Thailand
- 2Thailand Excellence Center for Tissue Engineering and Stem Cells, Department of Biochemistry, Faculty of Medicine, Chiang Mai University, Chiang Mai, Thailand
Preclinical studies have found impaired osteogenic differentiation to be associated with type 2 diabetes (T2DM), which is related to skeletal accumulation of advanced glycation end products (AGEs). Our previous study also showed impaired osteogenic differentiation in peripheral blood-derived mononuclear cells (PBMC) isolated from patients with long-standing T2DM, which is conceivably due to the overexpression of receptor of advance glycation end products (RAGE) and the enhancement of cellular apoptosis. However, the existence of RAGE overexpression in earlier stages of diabetes remains unclear, as do the factors influencing that RAGE overexpression. This cross-sectional study enrolled 40 patients with T2DM treated with metformin monotherapy and 30 age-matched non-diabetic controls (NDM) to investigate the overexpression of RAGE in PBMC derived from patients with earlier stage diabetes, as well as to explore its determining factors. Almost all (90%) PBMC-isolated from NDM (NDM-pD) expressed osteoblast-specific genes including ALPL, BGLAP, COL1A1, and RUNX2/PPAR while only 40% of PBMC-derived from diabetic patients (DM-pD) expressed those genes. By using age- and pentosidine-matched NDM-pD as a reference, AGER and BAX/BCL2 expression in PBMC isolated from diabetic patients showing impaired osteoblast-specific gene expression (DM-iD) were 6.6 and 5 folds higher than the reference while AGER and BAX/BCL2 expression in DM-pD were comparable to the reference. AGER expression showed a significant positive correlation with age (r=0.470, p=0.003). The multivariate analysis demonstrated that both age and AGER expression correlated with the potential for osteogenic differentiation in the PBMC isolated from patients with diabetes. In conclusion, this study showed osteogenic differentiation impairment in approximately half of PBMC derived from type 2 diabetic patients receiving metformin monotherapy. Both AGER and BAX/BCL2 overexpression were demonstrated only in PBMC-isolated from diabetic patients with poor osteogenic differentiation. Therefore, this study not only illustrated the existence of RAGE overexpression in PBMC derived from patients with early stages of T2DM but also strengthened the linkage between that RAGE overexpression and the retardation of osteogenic differentiation. Age was also shown to be a positive influencing factor for RAGE overexpression. Furthermore, both age and RAGE overexpression were demonstrated as independent risk factors for determining osteogenic differentiation potential of the PBMC-isolated from T2DM.
Introduction
Type 2 diabetes (T2DM) is a major health issue worldwide. It is a metabolic disorder characterized by insulin resistance and chronic hyperglycemia, contributing to multiple devastating microvascular and macrovascular complications. It is well documented that chronic hyperglycemia accelerates the accumulation of advanced glycation end products (AGEs) (1–3). These are non-enzymatic modifications of proteins which usually slowly accumulate in long-lived substrates in aging animals (4) and humans (5, 6). The accumulation of AGEs is one of the main mechanisms linking hyperglycemia and the chronic microvascular and macrovascular complications which develop in cases of diabetes (7–9).
T2DM is associated with a decrease in bone turnover (10, 11), changes in bone microarchitecture (12) and increases in the risk of fragility fractures (13–18) with a preserved bone mineral density (15, 16, 18). These indicate an adverse effect of diabetes on bone quality. Furthermore, the risk of fragility fractures increases with poorer glycemic control (19–21), suggesting an impact of hyperglycemia on fragility fractures, the most serious complication of osteoporosis. The skeletal accumulation of AGEs, which is accelerated in the presence of hyperglycemia, may contribute to those phenomena in diabetic patients. The non-enzymatic glycation of type 1 collagen of the bone competes with the enzymatic collagen crosslinking, yielding a skeletal accumulation of AGEs such as pentosidine. Multiple preclinical studies have demonstrated that the accumulation of AGEs altered the mechanical properties of bone (22–24) and interferes with the functions of bone cells including osteoblasts (25–30). In clinical studies Furst and colleagues (31) demonstrated an inverse correlation between skin AGE accumulation and bone material strength in patients with T2DM. Furthermore, a positive correlation has been shown between serum pentosidine and vertebral fractures (32–34). In contrast, an endogenous secretory receptor of AGE (esRAGE), a neutralizing molecule of AGE, showed a negative correlation with vertebral fractures in T2DM (34, 35).
AGEs act via binding to their specific receptor, the receptor of AGE (RAGE). Following binding with AGEs, RAGEs initiate multiple signal cascades including inflammatory and apoptotic pathways. Several in vitro studies in osteoblast lineage cells have demonstrated that AGEs-dependent RAGE activation inhibited osteoblast differentiation (36–39) and enhanced osteoblast apoptosis (25, 28, 39, 40). In our previous study in human subjects with T2DM, impaired osteogenic differentiation and enhanced cellular apoptotic signals were demonstrated, both of which are possibly linked to cellular RAGE overexpression in individuals with T2DM (41).
Our previous study illustrated higher AGER expression in peripheral-blood derived mononuclear cells (PBMC)-isolated from patients with long-standing T2DM compared to those in a matched non-diabetic control group, suggesting higher cellular RAGE activation in individuals with long-standing T2DM (41). Moreover, the cellular RAGE overexpression was positively associated with enhanced cellular apoptotic signals and then was potentially involved in the impairment of osteogenic differentiation potential of the cells (41). However, the existence of cellular RAGE overexpression in earlier stages of diabetes remains to be elucidated, as do the factors influencing that RAGE overexpression. It is known that the peripheral blood-derived mesenchymal stem cells can differentiate into multiple cell types, including adipocytes, chondrocytes and osteoblasts (42–44). Our previous study also showed the osteogenic differentiation potential of the PBMC-isolated from both healthy volunteers and patients with T2DM (41). To determine osteogenic differentiation of stem cells using the least invasive measures, this study was conducted using PBMC-derived from participants to investigate: 1) the osteogenic differentiation potential of PBMC-isolated from T2DM patients receiving metformin monotherapy, 2) the existence of cellular RAGE overexpression and the effects of that RAGE overexpression on osteogenic differentiation and cellular apoptotic signals, and 3) factors influencing that cellular RAGE overexpression.
Materials and Methods
Ethical Statements
This study was a cross-sectional study, performed at Maharaj Nakorn Chiang Mai Hospital, Chiang Mai University, Chiang Mai, Thailand. The study was approved by the Research Ethics Committee of the Faculty of Medicine, Chiang Mai University (MED-2557-02609). All participants signed an informed consent agreement before they were enrolled on the study.
Study Population and Sample Collection
Metformin is the first-line medication recommended for treatment of T2DM by the majority of international guidelines. In Thailand, metformin monotherapy is mostly used as initial therapy for patients with T2DM. Therefore, in this study, T2DM patients taking metformin monotherapy were enrolled to be representative of early-stage diabetes. Age-matched non-diabetic individuals were enrolled as a control group. The exclusion criteria were as follows: females with serum creatinine higher than 1.4 mg/dL or males with serum creatinine above 1.5 mg/dL; individuals who use thiazolidinedione, steroids, immunosuppressive agents, anti-resorptive agents or anabolic agents for osteoporosis, and individuals with metastatic or hematologic malignancy. Venous blood (35-40 mL) was collected from all enrolled participants to isolate the PBMC, and to determine serum levels of pentosidine (Elabscience Biotechnology, WuHan, Hubei, China) and sRAGE (R&D, Minneapolis, MN, USA). Fasting plasma glucose (FPG), glycated hemoglobin (HbA1c), low-density lipoprotein cholesterol (LDL-C) and serum creatinine were assessed using standardized procedures at a central laboratory of the Faculty of Medicine, Chiang Mai University. Glomerular filtration rate (eGFR) was calculated using the Chronic Kidney Disease Epidemiology Collaboration (CKD-EPI) method. Fracture risk estimation was estimated from The Fracture Risk Assessment Tool (FRAX®) using the Thailand database (45).
Isolation and Culture of Human Peripheral Blood-Derived Mononuclear Cells (PBMC)
PBMC were isolated from the 35-40 mL of peripheral venous blood using density gradient centrifugation as described in our previous study (41). In brief, the venous blood was centrifuged at 1500 rpm for 5 minutes. After plasma was removed, the remaining fraction was first diluted with an equal volume of DMEM (Gibco, Grand Islands, NY, USA) and then overlaid on Histopaque (specific gravity 1.077 g/mL; Sigma-Aldrich, St Louis, MO, USA) and finally centrifuged at 4000 rpm for 30 minutes. The PBMC were isolated from the mononuclear cell layer and plated in 24-well culture plates. These were then cultured in RPMI supplemented with 10% (v/v) fetal bovine serum (Gibco, Grand Islands, NY, USA). After removing the floating cells, the plastic-adhered cells were cultured in DMEM supplemented with 10% (v/v) fetal bovine serum (Gibco, Grand Islands, NY, USA), called non-osteogenic-inducing medium in the present study, for 7-10 days until confluence. To induce osteogenic differentiation, the plastic-adhered cells were cultured in non-osteogenic-inducing medium until reaching 50% confluence. They were then changed to an osteogenic-inducing medium (DMEM supplemented with 10-7 M dexamethasone, 60 μM ascorbic acid and 10 mM β-glycerophosphate) and cultured for a further 21 days.
Analysis for the Expression of Osteoblast-Specific Genes, AGER, and Cellular Apoptotic-Associated Genes
To examine gene expression total RNA was extracted using the illutraRNA spin Mini Kit (GE Healthcare Life Science, Buckinghamshire, UK) in accordance with the manufacturer’s instructions. Total RNA (500 ng) of each sample was used for reverse transcription into cDNA using an iScript™cDNA Synthesis Kit (Bio-Rad, Hercules, CA, USA) in accordance with the manufacturer’s protocol. Afterwards, the cDNA was analyzed by real-time quantitative polymerase chain reaction (real-time qPCR)(SsoFast EvaGreen Supremixes; Bio-Rad, Hercules, CA, USA). The reaction took place at 45 cycles of 5 seconds at 95°C, 10 seconds at 60°C and 30 seconds at 72°C using the Applied Biosystems 7500/7500 Fast Real-Time PCR system. The total RNA extracted from the PBMC, cultured both in non-osteogenic and osteogenic-inducing media, was used to determine: 1) osteoblast-specific genes including ALPL, BGLAP, COL1A1 and RUNX2 for representing osteoblast differentiation and 2) PPAR-γ which is a transcription factor driving towards adipocytes for evaluating signals against osteoblast differentiation. Differentiation towards osteoblasts was defined by the increment of expression of all osteoblast-specific marker genes including ALPL, BGLAP and COL1A1, as well as the increment of the RUNX2/PPARγ ratio. In contrast, the total RNA extracted only from the PBMC cultured in the non-osteogenic-inducing medium was used to determine: 1) AGER expression to elucidate cellular RAGE overexpression, and 2) BAX and BCL2 expression for the evaluation of cellular apoptotic signals. The primers were purchased from Invitrogen (Table 1). In the real-time qPCR, the GAPDH gene was used for normalization of the relative expression levels for each primer set by the 2(-ΔΔCT) method.
Statistical Analysis
All descriptive data are reported as mean ± standard deviation. An independent t-test was used to compare all continuous parameters while a Chi square test was used to compare binary parameters. Linear regression analysis was used to demonstrate factors correlating with osteoblast differentiation. Pearson’s correlation was used to identify the correlation between parameters, the exception being the correlation between AGER expression and other parameters which was done using Spearman’s correlation. A p-value of less than 0.05 was used as a measure of statistical significance. All statistical analyses were done with SPSS version 23.0.
Results
Demographic Data and Clinical Characters of Study Participants
The study included 40 patients with T2DM treated with metformin monotherapy (DM) and 30 age-matched participants without diabetes (NDM). All patients with T2DM were diagnosed as DM using the FPG criteria with a cut-off value of 126 mg/dL as recommended by the American Diabetes Association. Age, gender, body mass index (BMI), systolic blood pressure (SBP), diastolic blood pressure (DBP), LDL-C, eGFR, and 10-year fracture risk as determined by FRAX® were comparable in both NDM and DM groups (Table 2). With comparable levels of blood pressure in both DM and NDM groups, the usage rates of anti-hypertensive agents, including angiotensin-converting enzyme inhibitors (ACEI), angiotensin II receptor blockers (ARB), dihydropyridine calcium channel blockers (DHP-CCB) and thiazide-like diuretics, were higher in DM group than in NDM group. With comparable levels of LDL-C in both groups, the rate of statin use was higher in DM group than in NDM group. However, the difference in anti-hypertensive drugs and statins usage rates did not reach statistical significance (Table 2). Metformin was not applicable to NDM group. In the group with diabetes, the duration of diabetes was 5.5 ± 4.1 years with a 47.5% prevalence of microvascular complications and 10% prevalence of macrovascular complications. Metformin was the only anti-hyperglycemic agent used in all diabetic participants with a dosage of 1652.5 ± 627.4 mg/day. Both FPG and HbA1c levels were significantly higher in the DM group than those in NDM (Table 2). Serum pentosidine was significantly elevated in DM compared to that in NDM (6.1 ± 3.6 ng/mL vs 4.0 ± 2.1 ng/mL, p=0.03), suggesting accelerated AGEs accumulation in T2DM (Table 2). In contrast to pentosidine, serum sRAGE, a decoy receptor of AGEs, was comparable in both DM and NDM groups (527.1 ± 249.7 pg/mL vs 599.4 ± 422.1 pg/mL, p=0.374). Even though FPG, HbA1c and serum pentosidine levels were significantly higher in DM than in NDM group, serum pentosidine level showed no correlation with either FPG (r=0.065, p =0.598) or HbA1c (r=0.062, p=0.668). In addition, serum pentosidine level showed no correlation with other parameters, including age, BMI, eGFR and sRAGE level. Fracture risk as estimated by FRAX using the Thailand database was comparable between DM and NDM groups (Table 2).
Age Was an Independent Risk Factor for Preserving Osteogenic Differentiation Potential in Type 2 Diabetes
The PBMC-derived from diabetic patients showed a reduced potential to differentiate towards osteoblasts compared with those from non-diabetic controls. By using osteoblast-specific gene expression as a marker for osteogenic differentiation potential, the isolated PBMC from DM were divided into 2 groups including DM with preserved osteogenic differentiation potential (DM-pD) and DM with impaired osteogenic differentiation potential (DM-iD). Forty percent (16/40) of the PBMC-isolated from diabetic patients showed expression of osteoblast-specific genes including ALPL, BGLAP, COL1A1 and RUNX2/PPAR; therefore, the isolated-PBMC in this group were classified as DM-pD. The other 60% (24/40) of PBMC-isolated from DM did not express those osteoblast-specific genes, so the isolated-PBMC in this group were classified as DM-iD. While almost all the isolated PBMC from NDM expressed osteoblast-specific genes (NDM-pD), only 40% of the isolated PBMC from DM expressed osteoblast-specific genes (DM-pD) (90% vs 40%; p<0.0001). As shown by the RUNX2/PPAR ratio, the PBMC-derived from DM-pD expressed RUNX2, a master transcription factor for osteogenic differentiation, and PPARγ, a transcription factor driving differentiation against osteoblasts, at a similar extent to that of NDM-pD but at 3.8 times higher than that of DM-iD (Figure 1). Moreover, the PBMC-isolated from DM-pD showed the expression of ALPL, COL1A1 and BGLAP to be comparable to those of NDM-pD but at 7.3, 5.9, and 4.3 times higher than those of DM-iD (Figure 1). Factors determining osteoblast differentiation were analyzed in both NDM and DM groups. The multivariate analysis demonstrated that being diabetic is the only factor determining differentiation towards osteoblasts. Being diabetic increased the risk of osteogenic differentiation impairment 13.5 times (OR 13.5; 95% CI 3.21-77.91; p<0.001).
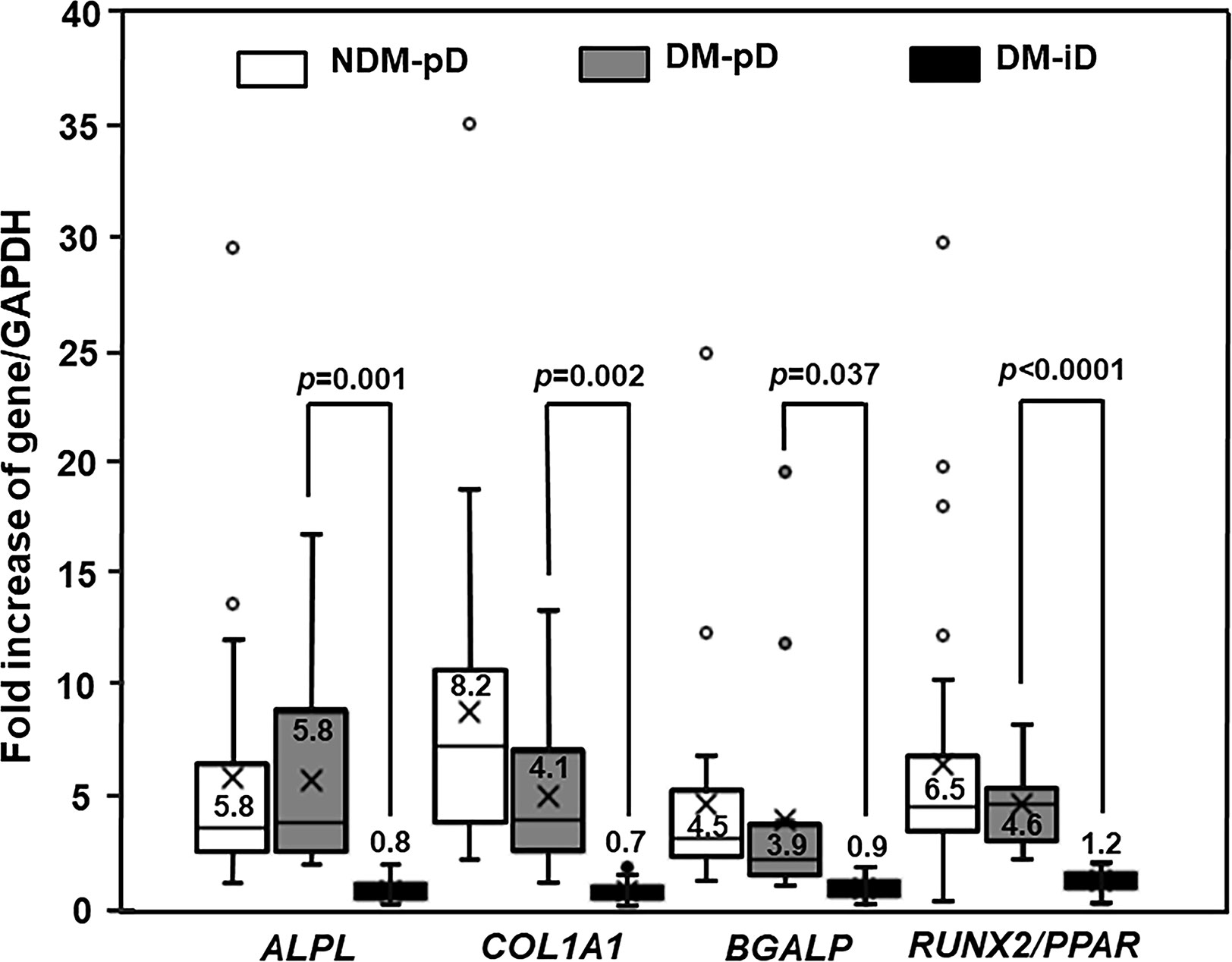
Figure 1 Osteogenic differentiation marker expression. Box and whisker plots to show comparison of osteoblast-specific gene expression in participants without diabetes showing preserved osteogenic differentiation potential (NDM-pD), patients with diabetes showing preserved osteogenic differentiation potential (DM-pD) and patients with diabetes showing impaired osteogenic differentiation potential (DM-iD)(mean ± SD). DM-pD had higher levels of expression of ALPL, COL1A1, BGLAP and RUNX2/PPARγ ratio (RUNX2/PPAR) than those in DM-iD by 7.3, 5.9, 4.3 and 3.8 times, respectively. DM-pD had significantly higher levels of expression of all osteoblast-specific genes than those in DM-iD but had comparable levels of expression of all osteoblast-specific genes to those in NMD-pD.
Forty percent of the PBMC-isolated from diabetic patients demonstrated a similar level of osteoblast-specific gene expression compared to those from non-diabetic individuals, indicating the preservation of osteogenic differentiation potential only in some individuals with type 2 diabetes. We next determined the factors influencing the preservation of osteogenic differentiation potential in the PBMC-derived from diabetic patients. Ten percent of PBMC-isolated from non-diabetic controls (3/30) which did not express osteoblast-specific genes, was excluded from the following analysis. When comparing DM-pD and NDM-pD, patients in the DM-pD group had significantly higher FPG (137.7 ± 30.2 mg/dL vs 96.1 ± 9.4 mg/dL, p<0.0001), HbA1c (7.5 ± 1.1% vs 6.0 ± 0.4%, p<0.0001) and serum pentosidine (7.3 ± 4.7 ng/mL vs 3.7 ± 1.8 ng/mL, p=0.008) than those in the NDM-pD group (Table 3). In addition, individuals with DM-pD were younger (54.4 ± 3.2 years vs 59.7 ± 7.9 years, p=0.004) than those in NDM-pD group (Table 3). When comparing within diabetic groups, FPG (137.7 ± 30.2 mg/dL vs 139.2 ± 23.49, p=0.859) and HbA1c (7.5 ± 1.1% vs 7.5 ± 0.86%, p=0.963) were comparable in both DM-pD and DM-iD groups. Serum pentosidine level was slightly higher in DM-pD in comparison to those in DM-iD group (7.3 ± 4.7 ng/mL vs 5.2 ± 2.53 ng/mL, p=0.120); however, the difference did not reach statistical significance (Table 3). Because FPG, HbA1c and serum pentosidine level were comparable between DM-pD and DM-iD, those factors should not influence the maintenance of osteogenic differentiation ability in cases of diabetes. There were also no differences in other parameters between DM-pD and DM-iD, including BMI, SBP, DBP, duration of being diabetic, the dosage of metformin, the usage rate of anti-hypertensive agents and statins, LDL-C and eGFR (Table 3). In contrast, age showed significant differences between DM-pD and DM-iD. Individuals in DM-iD were older than those in DM-pD group (60.5 ± 7.4 years vs 54.4 ± 3.2 years, p=0.001) but were at the same age as those in NDM-pD (60.5 ± 7.4 years vs 59.7 ± 7.9 years, p=0.701) (Table 3). The multivariate analysis demonstrated that age correlated with the potential for osteogenic differentiation in the PBMC-isolated from patients with diabetes, indicating that age is an independent risk factor for determining the differentiation potential toward osteoblasts of the PBMC-isolated from individuals with T2DM. Therefore, younger age was a protective factor for the preservation of osteoblast differentiation potential in T2DM.
AGER Overexpression Was Associated With Enhanced Cellular Apoptotic Signals and Impaired Osteogenic Differentiation, and Was Influenced by Age
Our previous study demonstrated higher AGER expression in patients with long-standing type 2 diabetes compared to that in non-diabetic controls, as well as the association of that RAGE overexpression with cellular apoptotic signal enhancement and osteogenic differentiation impairment. In this study, we analyzed PBMC isolated from 40 diabetic participants with metformin-monotherapy and 27 non-diabetic participants showing osteogenic differentiation (NDM-pD) to explore whether there was: 1) cellular RAGE overexpression in early-stage diabetes, 2) an association between RAGE overexpression with cellular apoptotic signal enhancement and osteogenic differentiation impairment, and 3) factors influencing cellular RAGE overexpression.
Since pentosidine has been documented in other studies as being an AGER enhancer, and age was confirmed earlier as an independent risk factor for the determination of osteogenic differentiation potential, the expression of AGER in both DM-iD and DM-pD was compared with the level of AGER expression in age- and pentosidine-matched NDM-pD individuals. This comparison aimed to explore if there was only a higher level of AGER expression in DM-iD, which would in turn suggest a link between cellular RAGE overexpression and defects in osteogenic differentiation. Using NDM-pD as a reference group, AGER expression in DM-iD was 6.6 times higher than that in the reference while the AGER expression in DM-pD was comparable to that in the reference (Figure 2). The AGER expression in DM-iD was significantly higher than that in DM-pD (6.6-fold vs 0.7-fold, p<0.0001), suggesting cellular RAGE overexpression in DM-iD as well as a connection between that RAGE overexpression and osteogenic differentiation defects in the PBMC. Consistent with higher AGER expression, the BAX/BCL2 expression ratio in DM-iD was 5.0 times higher than that in the reference while the BAX/BCL2 expression ratio in DM-pD was comparable to that in the reference (Figure 2). The BAX/BCL2 expression ratio in DM-iD was also significantly higher than in DM-pD (5.0-fold vs 0.6-fold, p=0.003), suggesting higher cellular apoptotic rate only in cases of diabetes with impaired osteogenic differentiation ability. Furthermore, AGER expression showed a strongly positive correlation with the BAX/BCL2 expression ratio (r=0.735, p<0.001), but showed a negative correlation with multiple osteoblast-specific gene expression including ALPL (r= -0.757, p<0.001), BGLAP (r= -0.670, p<0.001), COL1A1 (r= -0.478, p=0.003) and RUNX2/PPARγ ratio (r= -0.770, p<0.001) (Table 4). These association analyses suggest a direct relationship between cellular RAGE overexpression and cellular apoptotic signals, as well as an inverse relationship between cellular RAGE overexpression and osteogenic differentiation ability in the PBMC derived from diabetic patients. Interestingly, AGER expression showed a positive correlation with age (r=0.470, p=0.003) (Table 4), suggesting that age influences cellular RAGE overexpression.
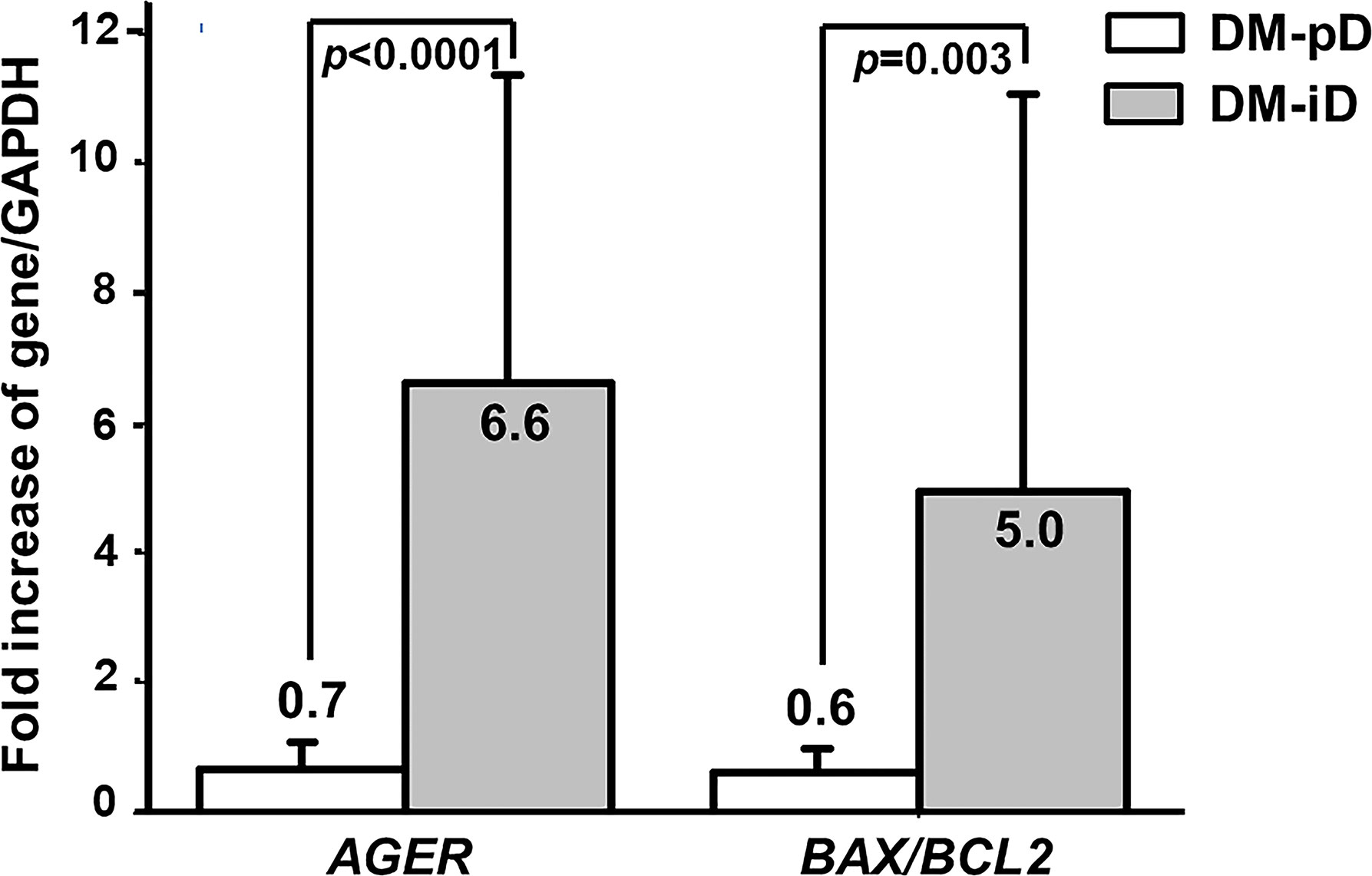
Figure 2 The expression of AGER, BAX and BCL2 genes. Comparison of the expression of the AGER, and BAX/BCL2 ratio between PBMC-isolated from subjects with diabetes with preserved osteogenic differentiation potential (DM-pD) and PBMC-isolated from diabetics with impaired osteogenic differentiation potential (DM-iD) by using age- and pentosidine-matched PBMC-isolated from non-diabetics with preserved osteogenic differentiation (NDM-pD) as a reference group. DM-iD showed higher expression of AGER and BAX/BCL2 ratio than the reference by 6.6 and 5.0 times, respectively, while DM-pD showed similar expression of AGER and BAX/BCL2 ratio to the reference. The expression of AGER and BAX/BCL2 ratio in DM-iD was significantly higher than that in DM-pD.
Discussion
This study demonstrated the preservation of osteogenic differentiation in 40% of PBMC-derived from type 2 diabetic patients who have had diabetes for an average of 5 years and are being treated with metformin monotherapy. Higher AGER expression was demonstrated only in PBMC-isolated from diabetics with poor osteogenic differentiation. This study not only demonstrated the existence of cellular RAGE overexpression in early stages of type 2 diabetes but also strengthened the link between that cellular RAGE overexpression and osteogenic differentiation retardation. This study also provided evidence to suggest that cellular RAGE overexpression increased with age. In addition, age and AGER expression were shown to be independent risk factors determining osteogenic differentiation potential of the PBMC-derived from T2DM.
In animal models with T2DM, a dramatic decrease in osteoblast/osteoid surface and bone mineral apposition rate has been demonstrated which indicates osteoblast differentiation and function impairment (46). Consistent with findings from animal studies, a reduction in osteoblast/osteoid surface, osteoid volume and thickness, which indicates a low bone formation state, has also been shown in humans with T2DM (10, 11, 47). Our previously study also demonstrated an impairment in osteogenic differentiation of the PBMC-isolated from long-standing type 2 diabetic patients (41). In agreement with previous studies, the present study illustrated impaired osteogenic differentiation in cases of type 2 diabetes. In the multivariate analysis, being diabetic significantly increased the risk of osteogenic differentiation impairment by 13.5 times (OR 13.5; 95% CI 3.21-77.91; p<0.001). In this study, 40% of cells from diabetic patients expressed osteoblast differentiation markers to a similar extent of those in non-diabetic individuals, suggesting a preserved potential of osteogenic differentiation only in some of the population among the whole diabetic group. Even though being diabetic increases the risk of osteogenic differentiation defects, diabetes at the different stages may have different degrees of that defect. Our previous study, which included long-standing type 2 diabetic subjects with an average of 10.7 ± 7.7 years of diagnosis, demonstrated that only 7.3% of PBMC-isolated from these diabetic patients expressed osteoblast-specific genes while 86.7% in non-diabetic controls expressed those genes (7.3% vs 86.7%, p<0.0001) (41). However, the present study was carried out with cells from patients at an earlier stage of diabetes with an average of 5.5 ± 4.1 years of diagnosis and showed that 40% of PBMC-isolated from diabetic subjects expressed osteoblast-specific genes while 90% in non-diabetic controls expressed those genes (40% vs 90%, p<0.0001). The present study showed a much higher number of PBMC with preserved osteogenic differentiation potential than those in the previous study which was done at a later stage of diabetes. However, it remains to be elucidated whether newly diagnosed type 2 diabetes still results in an impaired osteogenic differentiation.
Our previous study demonstrated cellular RAGE overexpression in type 2 diabetes which may lead to higher cellular apoptosis and poorer differentiation toward osteoblasts in T2DM. Because most PBMC-isolated from diabetic patients lose their ability to differentiate into osteoblasts, no firm conclusion can be made regarding the influence of cellular RAGE overexpression shown in type 2 diabetes on cellular apoptosis and the poorer differentiation found in type 2 diabetes. In this study, we focused on the earlier stage of diabetes and we demonstrated that only 60% (24/40) of the PBMC-isolated from diabetic patients lose their osteogenic differentiation potential (DM-iD). Therefore, we had the opportunity to explore whether there was only cellular RAGE overexpression in PBMC-isolated from diabetic patients showing impaired osteogenic differentiation. As pentosidine is an AGER enhancer and age is an independent risk factor for determining osteogenic differentiation as described above, AGER expression in an age- and pentosidine-matched NDM-pD group was used as a reference to compare the level of AGER expression between DM-iD and DM-pD for determining whether higher cellular AGER expression occurred only in the DM-iD group. Interestingly, higher AGER expression was demonstrated in DM-iD group but not in DM-pD, suggesting the existence of RAGE overexpression only in PBMC with poor osteogenic differentiation ability that isolated from diabetic patients. To further explore the association between cellular apoptosis and osteogenic differentiation, BAX and BCL2 expression in DM-iD and DM-pD were compared by using BAX/BCL2 expression in an age- and pentosidine-matched NDM-pD group as a reference. As seen with AGER expression, the ratio of BAX/BCL2 expression was higher only in the DM-iD group, suggesting a link between cellular apoptotic signal enhancement and osteogenic differentiation impairment. Consistent with our previous study (41), the expression of AGER and BAX/BCL2 ratio showed a strong correlation with each other (r=0.735, p<0.001). Therefore, the present study not only confirmed the existence of RAGE overexpression in PBMC with poor osteogenic differentiation potential but also strengthened the link between the cellular RAGE overexpression with osteogenic differentiation impairment and cellular apoptotic signal enhancement in the PBMC-isolated from T2DM patients.
Since the hyperglycemic state in diabetes accelerates the accumulation of AGEs in various tissues including those in the skeleton, accumulation of AGEs is one of the factors proposed as being responsible for the impairment in bone quality associated with diabetes and may be a useful predictor of fracture in diabetic individuals. Several studies have shown that the skeletal accumulation of AGEs alters bone mechanical properties, leading to fragility fractures (22–24). In addition, studies in primary culture osteoblasts and mesenchymal stem cells demonstrated that AGEs attenuated osteoblast differentiation and enhanced osteoblast apoptosis (25–29). In humans, serum pentosidine showed a positive correlation with fracture risk in T2DM (32–34). In contrast, esRAGE, an AGE neutralizer, showed a negative correlation with fracture risk in T2DM (34, 35). In this study, serum pentosidine was significantly higher in the group with diabetes than that in the age-matched non-diabetic group, implying accelerated accumulation of AGEs in diabetes mellitus. However, the levels of serum pentosidine in the group of diabetics with preserved osteogenic differentiation was comparable to the group of diabetics with impaired osteogenic differentiation. Moreover, a higher cellular AGER expression was illustrated only in the cells from the group of diabetics with impaired osteogenic differentiation, and that higher AGER expression was shown to be associated with the differentiation potential toward osteoblasts. Therefore, it is conceivable that serum pentosidine level has poor potential for the prediction of osteogenic differentiation in diabetes. Nevertheless, due to a small sample size and the wide standard deviation of serum pentosidine concentration found, the results of our study cannot exclude its potential for predicting osteoblast differentiation ability.
As 40% of PBMC-isolated from the diabetic group were shown to maintain their osteogenic differentiation potential, there was a potential for the exploration of the factors determining the preservation of osteogenic differentiation ability in our present study. These factors will be valuable in future exploration for guidance of fracture prevention in type 2 diabetes. Using the results of the multivariate analysis, this study demonstrated that age was a factor showing a correlation with the osteogenic differentiation potential of the PBMC-isolated from individuals with diabetes, indicating that age is an independent risk factor for determining the differentiation potential towards osteoblasts of the PBMC in diabetics. There is a higher probability for the preservation of the osteogenic differentiation potential in the younger diabetic individuals. In addition to age, AGER expression was also a risk factor for determining osteogenic differentiation, suggesting the contribution of higher cellular RAGE overexpression to the potential of differentiation of the PBMC towards osteoblasts. Interestingly, this study also demonstrated a positive correlation between age and AGER expression. Since age was an independent risk factor for osteogenic differentiation, as well as age showing a positive correlation with AGER expression, age itself is conceivably a contributor to cellular RAGE overexpression which in turn negatively affects osteogenic differentiation. Son and colleagues (48) demonstrated that accumulation of tissue AGEs and AGE-RAGE binding intensity increased with age and were different in organs in a non-diabetic mice model. In the liver and kidney, AGEs progressively accumulated with age, and AGE-RAGE binding intensity also increased with age, leading to an increase in its downstream signaling cascade. To the contrary, the accumulation of AGEs and AGE-RAGE binding intensity did not increase with age in skeletal muscles. Therefore, it remains a need for further elucidation as to whether RAGE activation in the skeleton conforms to an age-dependent pattern, leading to the probability of higher RAGE activation with increasing age. If AGEs-RAGE activation in the skeleton is an age-dependent pattern, being diabetic would drive accelerated accumulation of AGEs and even perpetuate RAGE activation as individuals inevitable grow older, resulting in the accelerated impairment of bone quality and increased fragility fracture in type 2 diabetes. From the evidence shown in this study, being diabetic increased the risk of osteogenic differentiation impairment and younger age is the single protective factor identified for preservation of osteogenic differentiation in T2DM, it is pertinent to state that prevention of becoming diabetic may be the most effective way to preserve the potential for osteogenic differentiation of the PBMC.
Several different types of medication prescribed for participants in this study were shown to influence bone metabolism. Metformin was the only anti-hyperglycemia agent given to all diabetic individuals in this study and it was not taken by non-diabetic controls. Metformin has been shown to promote the differentiation of osteoblasts from mesenchymal stem cells through the activation of the AMPK pathway (49, 50). Zhou Z and colleague (51) also demonstrated that metformin suppresses AGE-dependent RAGE activation in bone marrow derived macrophages. Even though all diabetic participants in this study may get benefit from metformin therapy, this medication did not overcome the detrimental effects of diabetes on osteogenic differentiation in 60% of cases. Metformin was used at the same dosage in both DM-pD and DM-iD, suggesting that the quantity of metformin did not influence the preservation of osteogenic differentiation ability found in this study. ACEI and ARB have been previously shown to have beneficial effects on bone metabolism and fractures (52–54). Liu YY and colleagues (52) showed that captopril, a type of ACEI, promoted osteoblast differentiation of primary cultured osteoblast cells as well as enhanced bone formation and bone strength in ovariectomized rats. In human, Kao YT and colleagues (53) showed that hypertensive individuals treated with ACEI or ARB had a decreased risk of osteoporotic fracture compared to those treated with other medications. Even though the difference did not reach statistical significance, participants in diabetic group used ACEI or ARB at a higher rate than those in non-diabetic group in this study (67.5% vs 44.8%, p=0.084). Therefore, it is reasonable to state that ACEI and ARB do not contribute to a lower rate of osteogenic differentiation in the diabetic group. In patients with diabetes, ACEI and ARB were used in the DM-pD group at a higher rate than those in DM-iD (75% vs 62.5%, p=0.503); however, the difference was nowhere near statistical significance. Therefore, it is reasonable to state that ACEI or ARB usage is not a contributory factor in the maintenance of osteogenic differentiation shown in DM-pD. Statins have been previously shown to have beneficial effects on bone metabolism and osteoporosis (55–58). Statins have been demonstrated to promote osteoblast differentiation and bone formation by stimulating the Akt/PI3 kinase and the β-catenin/Wnt signaling pathway, as well as to inhibit osteoblast apoptosis via activation of the TGFβ/Smad3 pathway (55). Zhang M and colleagues (56) demonstrated that simvastatin promoted differentiation of rat mesenchymal stem cells toward osteoblasts through the up-regulation of β-catenin (56). In humans, Lin TK and colleagues (57) carried out a nation-wide population cohort study to illustrate that statin use was associated with the decreased risk of osteoporosis in both females and males. In this study, participants in the diabetic group used statins at a higher rate than those in non-diabetic controls (79.5% vs 66.7%, p=0.275); however, the difference did not reach statistical significance. Therefore, from these findings the higher usage rate of statins in the diabetic group should not contribute to a lower rate of osteogenic differentiation in diabetic group.
This study provides evidence to support that cellular RAGE overexpression leads to osteogenic differentiation impairment in early stages type 2 diabetes, and that cellular RAGE overexpression is influenced by age. However, this evidence should be carefully interpreted due to several limitations. First, this study only demonstrated signal activation by mRNA level not protein expression due to the limited number of isolated cells from the relatively small 35-40 mL sample of peripheral blood collected from recruited patients. This raises the possibility the chance that transcription is activated but not translated into proteins. Therefore, it is probable that AGER overexpression will not lead to higher RAGE activation. The further studies involving RAGE knock-down would clarify whether cellular RAGE overexpression directly entail an impaired osteoblast differentiation. Second, this study presented data to show the pattern of association between parameters, so the causes and effects of those parameters cannot be definitely concluded. Finally, this study was a cross-sectional study which had several unexpected confounding factors by the nature of this type of study, for examples, AGER polymorphisms. Even though all baseline characters of the enrolled participants were generally comparable, those unexpected confounding factors might influence the results of the study. Multiple single nucleotide polymorphisms (SNPs) of AGER gene have been reported for association with diabetes and chronic diabetic complications (58–60). Cheng H and colleagues (58) showed that rs1800624 and rs2070600 SNPs associated with an increased risk of type 2 diabetes in South Asians and Caucasian, respectively. The rs1800624 SNP was documented for increasing AGER expression in vitro by enhancing the binding affinity of the transcription factor site while the rs207600 SNP was documented for enhancing the affinity of RAGE for its ligands (59). Since AGER polymorphism was not determined in this study, the contribution of AGER polymorphism to RAGE overexpression in PBMC was still possible. However, the evidence showing detrimental effects of AGER polymorphism on bone metabolism remains to be elucidated in cases of diabetes. To date, Raska Jr I and colleagues (60) showed that both rs1800624 and rs2070600 did not associate with sRAGE level, bone mineral density and fractures in postmenopausal women with T2DM.
Data Availability Statement
The original contributions presented in the study are included in the article/supplementary material. Further inquiries can be directed to the corresponding author.
Ethics Statement
The studies involving human participants were reviewed and approved by the Research Ethics Committee of the Faculty of Medicine, Chiang Mai University. The patients/participants provided their written informed consent to participate in this study.
Author Contributions
MP was involved in conceptualization, funding acquisition, methodology, formal analysis, original draft writing, review and editing of the manuscript. PP was involved in the methodology, original draft writing, review and editing of the manuscript. PK was involved in original draft writing, as well as review and editing of the manuscript. All authors contributed to the article and approved the submitted version.
Funding
This work is supported by Merck. The funder had no roles in the study design, data collection and analysis, preparation of the manuscript or decision to publish.
Conflict of Interest
The authors declare that the research was conducted in the absence of any commercial or financial relationships that could be construed as a potential conflict of interest.
Publisher’s Note
All claims expressed in this article are solely those of the authors and do not necessarily represent those of their affiliated organizations, or those of the publisher, the editors and the reviewers. Any product that may be evaluated in this article, or claim that may be made by its manufacturer, is not guaranteed or endorsed by the publisher.
References
2. Singh R, Barden A, Mori T, Beilin L. Advanced Glycation End-Products: A Review. Diabetologia (2001) 44(2):129–46. doi: 10.1007/s001250051591
3. Saito M, Fujii K, Mori Y, Marumo K. Role of Collagen Enzymatic and Glycation Induced Cross-Links as a Determinant of Bone Quality in Spontaneously Diabetic WBN/Kob Rats. Osteoporos Int (2006) 17(10):1514–23. doi: 10.1007/s00198-006-0155-5
4. Tomasek JJ, Meyers SW, Basinger JB, Green DT, Shew RL. Diabetic and Age-Related Enhancement of Collagen-Linked Fluorescence in Cortical Bones of Rats. Life Sci (1994) 55(11):855–61. doi: 10.1016/0024-3205(94)90041-8
5. Odetti P, Rossi S, Monacelli F, Poggi A, Cirnigliaro M, Federici M, et al. Advanced Glycation End Products and Bone Loss During Aging. Ann NY Acad Sci (2005) 1043:710–17. doi: 10.1196/annals.1333.082
6. Hein G, Weiss C, Lehmann G, Niwa T, Stein G, Franke S. Advanced Glycation End Product Modification of Bone Proteins and Bone Remodelling: Hypothesis and Preliminary Immunohistochemical Findings. Ann Rhem Dis (2006) 65(1):101–4. doi: 10.1136/ard.2004.034348
7. Garay-Sevilla ME, Regalado JC, Malacara JM, Nava LE, Wróbel-Zasada K, Castro-Rivas A, et al. Advanced Glycosylation End Products in Skin, Serum, Saliva and Urine and Its Association With Complications of Patients With Type 2 Diabetes Mellitus. J Endocrinol Invest (2005) 28(3):223–30. doi: 10.1007/BF03345377
8. Kilhovd BK, Juutilainen A, Lehto S, Rönnemaa T, Torjesen PA, Hassen KF, et al. Increased Serum Levels of Advanced Glycation End Products Predict Total, Cardiovascular and Coronary Mortality in Women With Type 2 Diabetes: A Population-Based 18 Year Follow-Up Study. Diabetogia (2007) 50(7):1409–17. doi: 10.1007/s00125-007-0687-z
9. Yamanaka M, Matsumura T, Ohno R, Fujiwara Y, Shinagawa M, Sugawa H, et al. Non-Invasive Measurement of Skin Fluorescence to Evaluate Diabetic Complications. J Clin Biochem Nutr (2016) 58(2):135–40. doi: 10.3164/jcbn.15-132
10. Shu A, Yin MT, Stein E, Cremers S, Dworakowski E, Ives R, et al. Bone Structure and Turnover in Type 2 Diabetes Mellitus. Osteoporos Int (2012) 23(2):635–41. doi: 10.1007/s00198-011-1595-0
11. Reyes-García R, Rozas-Moreno P, López-Gallardo G, García-Martín A, Varsavsky M, Avilés-Perez MD, et al. Serum Levels of Bone Resorption Markers Are Decreased in Patients With Type 2 Diabetes. Acta Diabetol (2013) 50(1):47–52. doi: 10.1007/s00592-011-0347-0
12. Paccou J, Ward KA, Jameson KA, Dennison EM, Cooper C, Edwards MH. Bone Microarchitecture in Men and Women With Diabetes: The Importance of Cortical Porosity. Calcif Tissue Int (2016) 98(5):465–73. doi: 10.1007/s00223-015-0100-8
13. Bonds DE, Larson JC, Schwartz AV, Strotmeyer ES, Robbins J, Rodriquez BL, et al. Risk of Fracture in Women With Type 2 Diabetes: The Women’s Health Initiative Observational Study. J Clin Endocrinol Metab (2006) 91(9):3404–10. doi: 10.1210/jc.2006-0614
14. Janghorbani M, Van Dam RM, Willett WC, Hu FB. Systematic Review of Type 1 and Type 2 Diabetes Mellitus and Risk of Fracture. Am J Epidemiol (2007) 166(5):495–505. doi: 10.1093/aje/kwm106
15. Vestergaard P. Discrepancies in Bone Mineral Density and Fracture Risk in Patients With Type 1 and Type 2 Diabetes–A Meta-Analysis. Osteoporos Int (2007) 18(4):427–44. doi: 10.1007/s00198-006-0253-4
16. Yamamoto M, Yamaguchi T, Yamauchi M, Kaji H, Sugimoto T. Bone Mineral Density Is Not Sensitive Enough to Assess the Risk of Vertebral Fractures in Type 2 Diabetic Women. Calcif Tissue Int (2007) 80(6):353–58. doi: 10.1007/s00223-007-9003-7
17. Melton LJ 3rd, Leibson CL, Achenbach SJ, Therneau TM, Khosla S. Fracture Risk in Type 2 Diabetes: Update of a Population-Based Study. J Bone Miner Res (2008) 23(8):1334–42. doi: 10.1359/jbmr.080323
18. Ma L, Oei L, Jiang L, Estrada K, Chen H, Wang Z, et al. Association Between Bone Mineral Density and Type 2 Diabetes Mellitus: A Meta-Analysis of Observational Studies. Eur J Epidemiol (2012) 27(5):319–32. doi: 10.1007/s10654-012-9674-x
19. Oei L, Zillikens MC, Dehghan A, Buitendijk GH, Castano-Betancourt MC, Estrada K, et al. High Bone Mineral Density and Fracture Risk in Type 2 Diabetes as Skeletal Complications of Inadequate Glucose Control: The Rotterdam Study. Diabetes Care (2013) 36(6):1619–28. doi: 10.2337/dc12-1188
20. Schneider AL, Williams EK, Brancati FL, Blecker S, Coresh J, Selvin E. Diabetes and Risk of Fracture-Related Hospitalization: The Atherosclerosis Risk in Communities Study. Diabetes Care (2013) 36(5):1153–8. doi: 10.2337/dc12-1168
21. Li CI, Liu CS, Lin WY, Meng NH, Chen CC, Yang SY, et al. Glycated Hemoglobin Level and Risk of Hip Fracture in Older People With Type 2 Diabetes: A Competing Risk Analysis of Taiwan Diabetes Cohort Study. J Bone Mineral Res (2015) 30(7):1338–46. doi: 10.1002/jbmr.2462
22. Tang SY, Zeenath U, Vashishth D. Effects of Non-Enzymatic Glycation on Cancellous Bone Fragility. Bone (2007) 40(4):1144–51. doi: 10.1016/j.bone.2006.12.056
23. Poundarik AA, Wu PC, Evis Z, Sroga GE, Ural A, Rubin M, et al. A Direct Role of Collagen Glycation in Bone Fracture. J Mech Behav BioMed Mater (2015) 52:120–30. doi: 10.1016/j.jmbbm.2015.08.012
24. Epstein S, Defeudis G, Manfrini S, Napoli N, Pozzilli P. Scientific Committee of the First International Symposium on Diabetes and Bone. Diabetes and Disordered Bone Metabolism (Diabetic Osteodystrophy): Time for Recognition. Osteoporos Int (2016) 27(6):1931–51. doi: 10.1007/s00198-015-3454-x
25. Alikhani M, Alikhani Z, Boyd C, MacLellan CM, Raptis M, Liu R, et al. Advanced Glycation End Products Stimulate Osteoblast Apoptosis via the MAP Kinase and Cytosolic Apoptotic Pathways. Bone (2007) 40(2):345–53. doi: 10.1016/j.bone.2006.09.011
26. Franke S, Siggelkow H, Wolf G, Hein G. Advanced Glycation Endproducts Influence the mRNA Expression of RAGE, RANKL and Various Osteoblastic Genes in Human Osteoblasts. Arch Physiol Biochem (2007) 113(3):154–61. doi: 10.1080/13813450701602523
27. Sanguineti R, Storace D, Monacelli F, Federici A, Odetti P. Pentosidine Effects on Human Osteoblasts In Vitro. Ann NY Acad Sci (2008) 1126:166–72. doi: 10.1196/annals.1433.044
28. Okazaki K, Yamaguchi T, Tanaka K, Notsu M, Ogawa N, Yano S, et al. Advanced Glycation End Products (AGEs), But Not High Glucose, Inhibit the Osteoblastic Differentiation of Mouse Stromal ST2 Cells Through the Suppression of Osterix Expression, and Inhibit Cell Growth and Increasing Cell Apoptosis. Calcif Tissue Int (2012) 91(4):286–96. doi: 10.1007/s00223-012-9641-2
29. Notsu M, Yamaguchi T, Okazaki K, Tanaka K, Ogawa N, Kanazawa I, et al. Glycation End Product 3 (AGE3) Suppresses the Mineralization of Mouse Stromal ST2 Cells and Human Mesenchymal Stem Cells by Increasing TGF-β Expression and Secretion. Endocrinology (2014) 155(7):2402–10. doi: 10.1210/en.2013-1818
30. Miranda C, Giner M, Montoya MJ, Vázquez MA, Miranda MJ, Pérez-Cano R. Influence of High Glucose and Advanced Glycation End-Products (Ages) Levels in Human Osteoblast-Like Cells Gene Expression. BMC Musculoskelet Disord (2016) 17:377. doi: 10.1186/s12891-016-1228-z
31. Furst JR, Bandeira LC, Fan WW, Agarwal S, Nishiyama KK, McMahon DJ, et al. Advanced Glycation End Products and Bone Material Strength in Type 2 Diabetes. J Clin Endocrinol Metab (2016) 101(6):2502–10. doi: 10.1210/jc.2016-1437
32. Yamamoto M, Yamaguchi T, Yamauchi M, Yano S, Sugimoto T. Serum Pentosidine Levels Are Positively Associated With the Presence of Vertebral Fractures in Postmenopausal Women With Type 2 Diabetes. J Clin Endocrinol Metab (2008) 93(3):1013–19. doi: 10.1210/jc.2007-1270
33. Schwartz AV, Garnero P, Hillier TA, Sellmeyer DE, Strotmeyer ES, Feingold KR, et al. Health, Aging, and Body Composition Study. Pentosidine and Increased Fracture Risk in Older Adults With Type 2 Diabetes. J Clin Endocrinol Metab (2009) 94(7):2380–6. doi: 10.1210/jc.2008-2498
34. Tamaki J, Kouda K, Fujita Y, Iki M, Yura A, Miura M, et al. Ratio of Endogenous Secretory Receptor for Advanced Glycation End Products to Pentosidine Predicts Fractures in Men. J Clin Endocrinol Metab (2018) 103(1):85–94. doi: 10.1210/jc.2017-00929
35. Yamamoto M, Yamaguchi T, Yamauchi M, Sugimoto T. Low Serum Level of the Endogenous Secretory Receptor for Advanced Glycation End Products (esRAGE) Is a Risk Factor for Prevalent Vertebral Fractures Independent of Bone Mineral Density in Patients With Type 2 Diabetes. Diabetes Care (2009) 32(12):2263–8. doi: 10.2337/dc09-0901
36. Xie J, Méndez JD, Méndez-Valenzuela V, Aguilar-Hernández MM. Cellular Signaling of the Receptor for Advanced Glycation End Products (RAGE). Cell Signal (2013) 25(11):2185–97. doi: 10.1016/j.cellsig.2013.06.013
37. Suryavanshi SV, Kulkarni YA. NF-Кβ: A Potential Target in the Management of Vascular Complications of Diabetes. Front Pharmacol (2017) 8:798. doi: 10.3389/fphar.2017.00798
38. Yao D, Brownlee M. Hyperglycemia-Induced Reactive Oxygen Species Increase Expression of the Receptor for Advanced Glycation End Products (RAGE) and RAGE Ligands. Diabetes (2010) 59(1):249–55. doi: 10.2337/db09-0801
39. Mercer N, Ahmed H, Etcheverry SB, Vasta GR, Cortizo AM. Regulation of Advanced Glycation End Product (AGE) Receptors and Apoptosis by AGEs in Osteoblast-Like Cells. Mol Cell Biochem (2007) 306(1-2):87–94. doi: 10.1007/s11010-007-9557-8
40. Liu J, Mao J, Jiang Y, Xia L, Mao L, Wu Y, et al. AGEs Induced Apoptosis in Rat Osteoblast Cells by Activating the Caspase-3 Signaling Pathway Under a High-Glucose Environment In Vitro. Appl Biochem Biotechnol (2016) 178(5):1015–27. doi: 10.1007/s12010-015-1925-3
41. Phimphilai M, Pothachareon P, Kongtawelert P, Chattipakorn N. Impaired Osteogenic Differentiation and Enhanced Cellular Receptor of Advanced Glycation End Products Sensitivity in Patients With Type 2 Diabetes. J Bone Miner Metab (2017) 35(6):631–41. doi: 10.1007/s00774-016-0800-9
42. Cesselli D, Beltrami AP, Rigo S, Bergamin N, D'Aurizio F, Verardo R, et al. Multipotent Progenitor Cells Are Present in Human Peripheral Blood. Circ Res (2009) 104(10):1225–34. doi: 10.1161/CIRCRESAHA.109.195859
43. Chong PP, Selvaratnam L, Abbas AA, Kamarul T. Human Peripheral Blood Derived Mesenchymal Stem Cells Demonstrate Similar Characteristics and Chondrogenic Differentiation Potential to Bone Marrow Derived Mesenchymal Stem Cells. J Orthop Res (2012) 30(4):634–42. doi: 10.1002/jor.21556
44. Yang HS, Kim GH, La WG, Bhang SH, Lee TJ, Lee JH, et al. Enhancement of Human Peripheral Blood Mononuclear Cell Transplantation-Mediated Bone Formation. Cell Transplant (2011) 20(9):1445–52. doi: 10.3727/096368910X557272
45. Centre for Metabolic Bone Diseases, University of Sheffield, UK[Internet]: The Fracture Risk Assessment Tool (FRAX®). Available at: https://www.sheffield.ac.uk/FRAX/tool.aspx?country=57.
46. Verhaeghe J, van Herck E, Visser WJ, Suiker AM, Thomasset M, Einhorn TA, et al. Bone and Mineral Metabolism in BB Rats With Long-Term Diabetes. Decreased Bone turnover osteoporosis Diabetes (1990) 39(4):477–82. doi: 10.2337/diab.39.4.477
47. Leite Duarte ME, da Silva RD. Histomorphometric Analysis of the Bone Tissue in Patients With Non-Insulin-Dependent Diabetes (DMNID). Rev Hosp Clin Fac Med Sao Paulo (1996) 51(1):7–11.
48. Son M, Chung WJ, Oh S, Ahn H, Choi CH, Hong S, et al. Age Dependent Accumulation Patterns of Advanced Glycation End Product Receptor (RAGE) and Binding Intensities Between RAGE and Its Ligands Differ in the Liver, Kidney, and Skeletal Muscle. Immun Ageing (2017) 14:12. doi: 10.1186/s12979-017-0095-2
49. Molinuevo MS, Schurman L, McCarthy AD, Cortizo AM, Tolosa MJ, Gangoiti MV, et al. Effect of Metformin on Bone Marrow Progenitor Cell Differentiation: In Vivo and In Vitro Studies. J Bone Miner Res (2010) 25(2):211–21. doi: 10.1359/jbmr.090732
50. Shaik AR, Singh P, Shaik C, Kohli S, Vohora D, Ferrari SL. Metformin: Is It the Well Wisher of Bone Beyond Glycemic Control in Diabetes Mellitus? Calcified Tissue Int (2021) 108:693–707. doi: 10.1007/s00223-021-00805-8
51. Zhou Z, Tang Y, Jin X, Chen C, Lu Y, Liu L, et al. Metformin Inhibits Advanced Glycation End Products-Induced Inflammatory Response in Murine Macrophages Partly Through AMPK Activation and RAGE/NF κ B Pathway Suppression. J Diabetes Res (2016) 2016:4847812. doi: 10.1155/2016/4847812
52. Liu YY, Yao WM, Wu T, Xu BL, Chen F, Cui L. Captopril Improves Osteopenia in Ovariectomized Rats and Promotes Bone Formation in Osteoblasts. J Bone Miner Metab (2011) 29(2):149–58. doi: 10.1007/s00774-010-0209-9
53. Kao YT, Huang CY, Fang YA, Liu JC. The Association Between Renin Angiotensin Aldosterone System Blockers and Future Osteoporotic Fractures in a Hypertensive Population – A Population-Based Cohort Study in Taiwan. Int J Cardiol (2020) 305:147–53. doi: 10.1016/j.ijcard.2019.12.069
54. Mo C, Ke J, Zhao D, Zhang B. Role of the Renin-Angiotensin-Aldosterone System in Bone Metabolism. J Bone Miner Metab (2020) 38(6):772–9. doi: 10.1007/s00774-020-01132-y
55. Chamani S, Liberale L, Mobasheri L, Montecucco F, Al-Rasadi K, Jamialahmadi T, et al. The Role of Statins in the Differentiation and Function of Bone Cells. Eur J Clin Invest (2021) 51:e13534. doi: 10.1111/eci.13534
56. Zhang M, Bian YQ, Tao HM, Yang XF, Mu WD. Simvastatin Induces Osteogenic Differentiation of MSCs via Wnt/β-Catenin Pathway to Promote Fracture Healing. Eur Rev Med Pharmacol Sci (2018) 22(9):2896–905. doi: 10.26355/eurrev_201805_14992
57. Lin TK, Chou P, Lin CH, Hung YJ, Jong GP. Long-Term Effect of Statins on the Risk of New-Onset Osteoporosis: A Nationwide Population-Based Cohort Study. PloS One (2018) 13(5):e0196713. doi: 10.1371/journal.pone.0196713
58. Cheng H, Zhu W, Zhu M, Sun Y, Sun X, Jia D, et al. Susceptibility of Six Polymorphisms in the Receptor for Advanced Glycation End Products to Type 2 Diabetes: A Systematic Review and Meta-Analysis. Endocr J (2021). doi: 10.1507/endocrj.EJ21-0130
59. Serveaux-Dancer M, Jabaudon M, Creveaux I, Belville C, Blondonnet R, Gross C, et al. Pathological Implications of Receptor for Advanced Glycation End-Product (AGER) Gene Polymorphism. Dis Markers (2019). doi: 10.1155/2019/2067353
Keywords: advanced glycation end products, receptor of advanced glycation end products, osteogenic differentiation, peripheral blood derived mononuclear cells, type 2 diabetes
Citation: Phimphilai M, Pothacharoen P and Kongtawelert P (2021) Age-Influenced Receptors of Advanced Glycation End Product Overexpression Associated With Osteogenic Differentiation Impairment in Patients With Type 2 Diabetes. Front. Endocrinol. 12:726182. doi: 10.3389/fendo.2021.726182
Received: 16 June 2021; Accepted: 10 August 2021;
Published: 26 August 2021.
Edited by:
Guillaume Mabilleau, Université d’Angers, FranceReviewed by:
Philip C. Trackman, The Forsyth Institute, United StatesArmando Rojas, Catholic University of the Maule, Chile
Copyright © 2021 Phimphilai, Pothacharoen and Kongtawelert. This is an open-access article distributed under the terms of the Creative Commons Attribution License (CC BY). The use, distribution or reproduction in other forums is permitted, provided the original author(s) and the copyright owner(s) are credited and that the original publication in this journal is cited, in accordance with accepted academic practice. No use, distribution or reproduction is permitted which does not comply with these terms.
*Correspondence: Mattabhorn Phimphilai, mphimphi@hotmail.com